- 1Forest Pathology Laboratory, School of Forest, Fisheries and Geomatics Sciences, University of Florida, Gainesville, FL, United States
- 2Department of Plant Pathology, University of Minnesota, St. Paul, MN, United States
- 3Faculty of Education, Ibaraki University, Mito, Ibaraki, Japan
- 4Department of Botany and Plant Pathology, Purdue University, West Lafayette, IN, United States
In the late fall of 2018, foliar rust (referred to as ironwood/hophornbeam leaf rust [IHLR]) was discovered in several counties in Florida, United States, on ironwood (Carpinus caroliniana) and hophornbeam (Ostrya virginiana), both members of the Betulaceae. Uredinia were observed on leaves and, in some cases, samaras of both species at numerous locations on trees of all age classes. Similar reports across the southeastern United States (Georgia, the Carolinas, Tennessee, and Texas) were detected the following year, with European hornbeam (Carpinus betulus) being reported as an additional host, while Ostrya virginiana var. guatemalensis in El Salvador was also discovered showing signs of uredinial infection. Field observations and analyses of morphological data obtained with light and scanning electron microscopy on IHLR and related Melampsoridium herbarium samples and combined molecular data from the ITS and LSU loci indicate that (i) IHLR across samples from the southeastern United States and El Salvador belong to the same taxon; (ii) IHLR is closely affiliated to M. asiaticum; and (iii) some taxonomic modifications might be necessary at the genus level. No alternative (gametophyte) host has been identified, and the rust is likely overwintering in the uredinial stage. This disease represents a novel leaf pathogen on these hosts in North America, and efforts are needed to monitor future disease epidemiology and impacts on these native and cultivated tree species.
1. Introduction
Betulaceae species, American hornbeam or ironwood (Carpinus caroliniana Walt.) and Eastern hophornbeam (Ostrya virginiana Mill. K. Koch), are distributed in eastern North America, more precisely east of a Minnesota–Louisiana axis plus Texas and Oklahoma, as well as Ontario and Quebec in Canada (Nesom et al., 2003). Ostrya virginiana also has disjunct populations in Wyoming (USA), Manitoba (Canada), Mexico, Guatemala, Honduras, and El Salvador (https://www.fs.fed.us/database/feis/plants/tree/ostvir). However, populations south of the United States are considered either a different species, O. guatemalensis, or a variety, O. virginiana var. guatemalensis (https://powo.science.kew.org/taxon/urn:lsid:ipni.org:names:295249-1). Naturally occurring as forest understory components, these two species are also planted for ornamental landscaping purposes. Both are important to wildlife, notably O. virginiana, whose buds and catkins constitute winter food for a variety of bird species and other wildlife (Burns and Honkala, 1990).
Yellow pustules developing on the lower (abaxial) surface of the leaves of Carpinus caroliniana and Ostrya virginiana were first observed in the fall of 2018 near Gainesville in Alachua County, Florida (Figures 1A–E). There was no record of rust occurring on these two Betulaceae species in Florida prior to these observations. Similar observations across the southeastern United States were reported by collaborators in the following year (J.A. Smith, personal communication). Melampsoridium species are obligate biotrophic fungi and have their telial (sporophytic) stage on Betulaceae hosts across the globe: M. alni on Alnus spp., M. asiaticum on Carpinus spp. and Ostrya japonica, M. betulinum on Betula spp. and Alnus spp., M. carpini on Carpinus spp. and O. virginiana, and M. hiratsukanum on Alnus spp. (https://nt.ars-grin.gov/fungaldatabases/fungushost/fungushost.cfm).
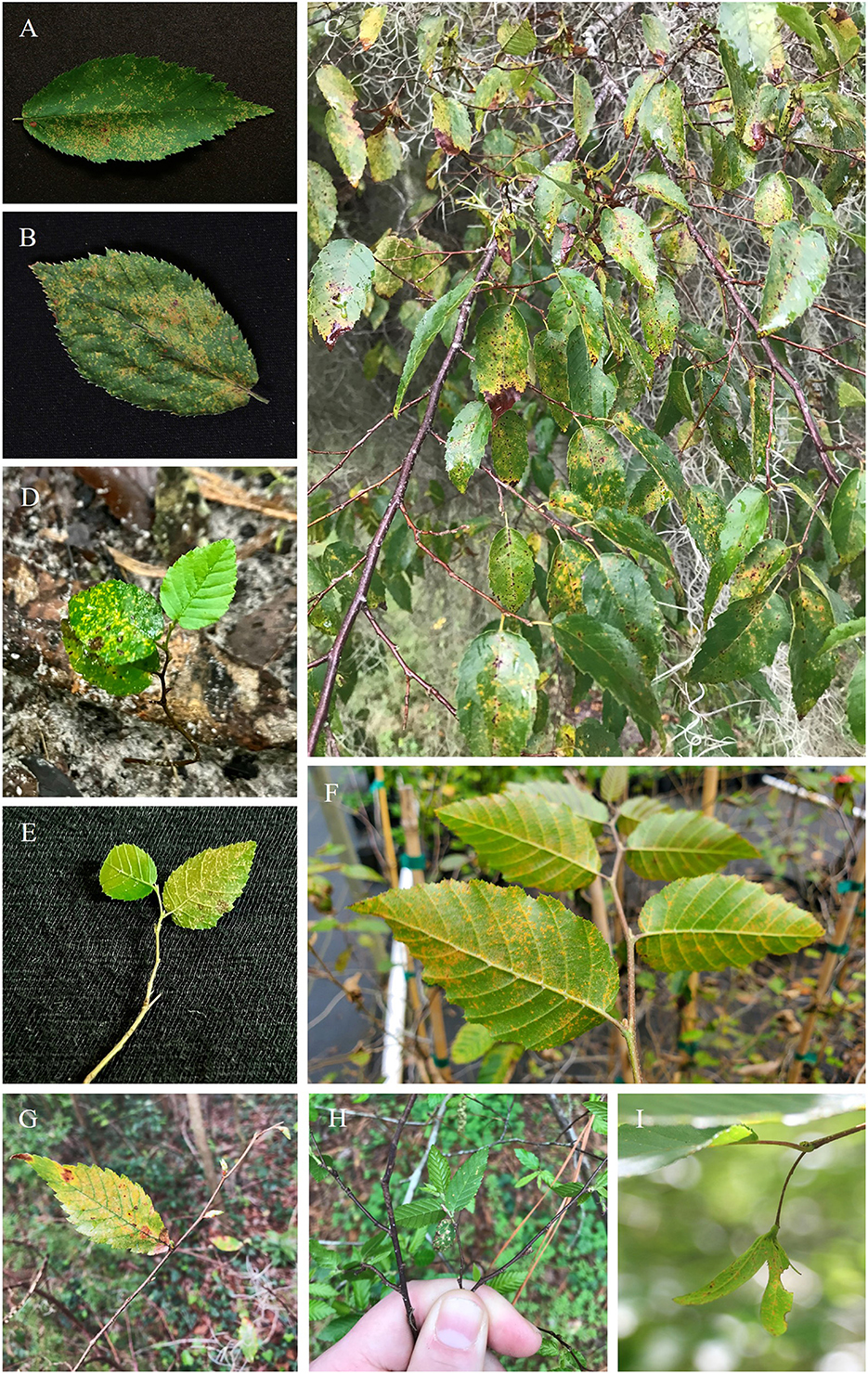
Figure 1. Uredinial infection of IHLR on Betulaceae hosts in the southeastern United States. (A) Carpinus caroliniana leaf upper surface, (B) Ostrya virginiana leaf upper surface, (C) severe sporulation on C. caroliniana leaves, (D, E) C. caroliniana seedlings, (F) abaxial surface of Carpinus betulus leaves grown in a nursery, (G) C. caroliniana samaras, (H, I) C. caroliniana new leaves coexisting with leaves from previous growth year.
The genus Melampsoridium was erected by Klebahn in 1899 and was segregated from Melampsora based on the presence of a peridium on both aecia and uredinia and the absence of paraphyses (Engler and Prantl, 1899). In addition, Klebahn mentioned that Melampsoridium species are heteroecious, although sporophytic hosts have only been reported for M. alni, M. hiratsukanum, and M. betulinum (Hiratsuka et al., 1992). No alternative host has been recorded for M. carpini and M. asiaticum (the only known taxa to occur on Carpinus and Ostrya), although the distribution of some Larix species overlaps the distribution of these two fungal taxa (Mamet et al., 2019). A key for the genus was created by Kaneko and Hiratsuka (1981), and species delimitation relied on urediniospore morphological comparisons: length, width, echinulation pattern (echinulated opposed to smooth apex), and number and position of germ pores. These features facilitated the description of M. asiaticum in Kaneko and Hiratsuka (1983), which is present in Japan and China. This also separated rusts from both Carpinus and Ostrya species into two morphological species: M. asiaticum and M. carpini. Some records for the genus worldwide have been made based on these morphological characteristics but require re-examination and, in some cases, have been re-annotated. A record of Melampsoridium carpini from New York State (Accession number PUR006358, collected by Mains and Kauffman in 1914), the only record of rust on Ostrya from North America, was originally annotated as having been collected from “Ostrya virginiana”. However, our examination determined it was M. betulinum based on the length of urediniospores and position of germ pores (Kaneko and Hiratsuka, 1983), and the host is more likely to be yellow birch (Betula alleghaniensis). Kurkela et al. (1999) used, for the first time in the study of this genus, genetic data (ITS rDNA sequence data) and morphology (urediniospore roundness) to determine that the rust species infecting alder in northeastern Europe (Estonia and Finland) was M. hiratsukanum and not M. betulinum. This study underlined the limitation in the use of this locus for broader phylogenetic studies among the Pucciniales order due to a lack of variation, notably within Cronartium, Pucciniastrum, and Chrysomyxa genera (Aime et al., 2017). However, it remains pertinent for species delimitation between species in the genus Melampsoridium in combination with morphological data. Hantula et al. (2009) also used a combination of ITS data and morphological observations to better distinguish the two rust species co-occurring on Alnus leaves in Europe: the native species M. betulinum and the introduced species M. hiratsukanum.
Therefore, using morphological and genetic data, we propose in this study to test the hypotheses (i) that the observed epidemic in the southeastern United States referred to as IHLR is caused by a Melampsoridium rust species, and (ii) that this taxon represents a novel taxon previously undescribed.
2. Methods
2.1. Fungal material studied
2.1.1. Emergent IHLR taxon
Uredinia from the lower leaf surface of Carpinus caroliniana (Cc—American hornbeam), Ostrya virginiana (Ov—Eastern hophornbeam), and Carpinus betulus (Cb—European hornbeam) (Figure 1F) were collected on 11 infected samples from across the southeastern United States: three from Texas, three from Tennessee, two from Florida, one from Georgia, one from South Carolina, and one from North Carolina. Cc and Ov samples are from natural areas and botanical gardens, while Cb samples are from nursery and landscape settings. The locations of IHLR samples are mapped in Figure 2 and listed in Table 1. Ostrya virginiana var. guatemalensis-infected leaves from El Salvador were requested as well from a collaborator on site and shipped to colleagues at the University of Minnesota (USDA-APHIS/PPQ Permit P526P-18-04404). All isolates were given a code that included the state where they were collected and letters referring to their host (e.g., FLCc1). Details about samples and their name codes are listed in Table 1.
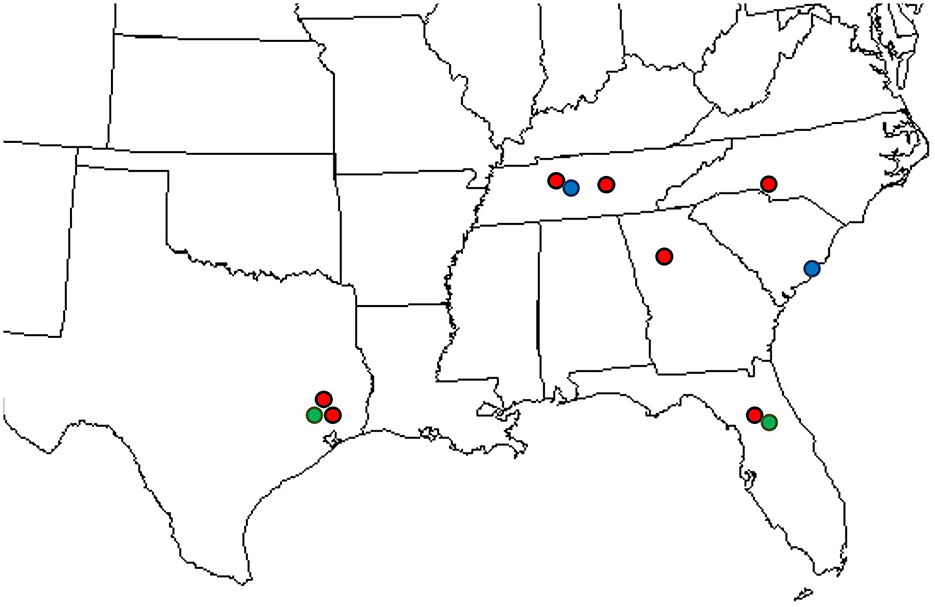
Figure 2. Locations where IHLR was sampled across the southeastern United States: red dots on Carpinus caroliniana, blue dots on Carpinus betulus, and green dots on Ostrya virginiana hosts.
One of the diagnostic tools in the genus Melampsoridium consists of plotting roundness against the length of the urediniospores (Kurkela et al., 1999; Hantula et al., 2009, 2012). We therefore used it to make the comparison between the sample from El Salvador and a selection of the ones obtained across the southeastern United States in 2019 from both C. caroliniana and O. virginiana hosts in Gainesville, FL (FLCc1 and FlOv1, respectively), C. caroliniana in Harris County, TX (TXCc2), and C. betulus in a nursery setting in 2019 in Tennessee (TNCb1). Two more samples from 2020 were added from another C. betulus host in Charleston, SC, and another C. caroliniana host in Atlanta, GA (SCCb1 and GACc1, respectively).
Given that these hosts are closely related within the Betulaceae, the synchronicity of the emergence of these samples, the nature of their host (Ostrya virginiana var. guatemalensis), and the conspecificity of the sample obtained from El Salvador with the taxon sampled across the Southeastern United States are discussed below.
2.1.2. Herbarium specimens
We requested 27 herbarium specimens belonging to all accepted taxa in the genus Melampsoridium for morphological and molecular investigations. Three specimens were obtained from the United States Department of Agriculture National Fungus Collections (BPI), 14 from the Arthur Fungarium at Purdue University (PUR), six from the University of Michigan Herbarium (MICH), one from the Tottori Mycological Institute (TMI), and three from the Ibaraki University Herbarium (Table 1). Among them, several were likely incorrectly identified. As mentioned above, M. asiaticum was described in Kaneko and Hiratsuka (1983), and some specimens were erroneously treated as M. carpini. This is the case for specimens PUR 006369 from 1931, PUR 006371 from 1929, and PUR 006375 from 1939, which were labeled as M. carpini but likely represent M. asiaticum. These specimens were not included in the study.
2.2. Incidence and severity
Incidence and severity data were determined by processing digital pictures of the host tissue with Leaf Doctor software (Pethybridge and Nelson, 2015), optimized for Apple's iOS operating system. Twenty leaves from each of 10 Carpinus caroliniana and 10 Ostrya virginiana individuals were sampled in July 2020 at two sites: Loblolly Woods and John Mahon Nature Park in Gainesville, Florida. As uredinial infection was observed on the samaras of two additional Carpinus caroliniana individuals at Loblolly Woods later in the 2020 summer season, severity measurements were also recorded on 20 leaves from these two individuals, as well as on five samaras on both trees. Average severity values were determined for each host at each location. The severity value for each leaf corresponds to a disease percentage, i.e., the proportion of symptomatic tissue area infected by the rust pathogen divided by the total leaf tissue area. The incidence was recorded as positive when at least one yellow pustule was observed on the sampled leaves/samaras.
2.3. Morphological observations
2.3.1. Light microscopy
All IHLR isolates from the southeastern United States, one from El Salvador, and all the requested herbarium specimens were systematically used for urediniospore measurements, including length, width, perimeter, and area. These measurements were also used to investigate the conspecificity of the sample from El Salvador with the emergent taxon across the southeastern United States. Urediniospore morphological characters of each collected sample or herbarium specimen were visualized on slides in 5% KOH under 400 × , 600 × , or 1,000 × magnification using a Nikon Eclipse 55i light microscope (Melville, NY). Cell measurements were performed using ImageJ2 software (http://imagej.net). Up to 20 urediniospores were measured per specimen. Urediniospore roundness was determined as described in Kurkela et al. (1999) using the following formula:
where 1 is the minimum value that indicates a perfect circle.
2.3.2. Scanning electron microscopy
In order to generate higher-resolution images of the urediniospores of the different taxa used in this study, specimens were examined using scanning electron microscopy (SEM) at the University of Minnesota Imaging Center. Leaf tissue infected with Melampsoridium was mounted on 1.5-mm aluminum stubs with carbon tape and sputter-coated with gold/palladium using a Cressington 108 Auto Sputter Coater (Cressington Scientific Instruments, Watford, United Kingdom). Samples were then examined using a Hitachi S3500N scanning electron microscope (Hitachi, Tokyo, Japan). This allowed for a consistent observation of the urediniospore echinulation pattern (homogeneous echinulation vs. smooth apex) and the measurement of interspine distance. We measured 10 interspine distances on 10 spores per sample/herbarium specimen. The echinulation pattern was observed and noted on these same 10 spores per sample/herbarium specimen.
2.4. Statistical analysis
Morphological characteristics average values were calculated for each specimen (IHLR and herbarium) to establish statistical comparisons. The principal component analysis (PCA), based on urediniospore morphological measurements (length, width, perimeter, area, and roundness), pairwise tests (Welsh two-sample t-test for normally distributed data), and Kruskal–Wallis tests (non-parametric test) were carried out using R software version 4.1.1 (R Core Team, 2023). When the original data were not normally distributed, logarithmic base 10 and square root transformations were attempted for pairwise comparisons. The PCA analysis is used to test affinities between the different taxa in the genus based on morphological characteristics.
2.5. DNA extraction, amplification, and sanger sequencing
DNA was extracted by crushing uredinia between a clean microscope slide and a clean cover slip in 50 μL of Extract-N-Amp DNA extraction buffer (Millipore Sigma, St. Louis, MO), placing the solution for 1 h at 37°C, 10 min at 90°C, and at 4°C. This protocol is based on the work of Liang et al. (2006). When recalcitrant herbarium samples were processed, the QIAGEN DNeasy Plant Mini Kit (Qiagen, Hilden, Germany) was used instead, and the extraction process was optimized by soaking samples in liquid nitrogen and disrupting them with glass beads in a shaker. The incubation time in the extraction buffer was also increased from 10 min to up to 4 h at 90°C. All amplification reactions were run in a MJ Mini thermocycler (Bio-Rad Inc., Hercules, CA) in 25 μL reaction volumes with 12 μL Immomix Red Master Mix (Bioline, London, UK), 9 μL of PCR-grade H2O, 1 μL Bovine Serum Albumine (BSA) (3% w/v), 1 μL of each primer, and 1 ng/μL of DNA template. For the nuclear large subunit (28S) locus of the ribosomal DNA, amplification was achieved using the primers Rust2Inv (forward) (Aime, 2006)/LR6 (reverse) (Vilgalys and Hester, 1990) with the following conditions: initial denaturation step of 2 min at 94°C, 40 cycles of 30 s at 94°C, 1 min at 57°C, and 1.5 min at 72°C, for a final extension of 7 min at 72°C. The internal transcribed spacer (ITS) region was amplified using ITS1F (White et al., 1990) and ITS4b (Gardes and Bruns, 1993) primer pairs. Amplicons were sequenced with the same primers. A nested PCR was performed on weaker LSU PCR amplicons with Rust28SF (Aime et al., 2018)/LR5 (Vilgalys and Hester, 1990). Primers were designed for specimens recalcitrant to the ITS locus amplification, notably M. asiaticum specimens from Japan and M. carpini specimens from Austria. These primers, based on the universal ITS2 and ITS3 primers (White et al., 1990), are named ITS2_Mel and ITS3_Mel (see Table 2 for primer sequences). These same primers were used for Sanger sequencing on amplicons obtained from nested PCRs. PCR amplicons were purified with the ExoSAP-IT purification kit (ThermoFisher, Waltham, MA) and sequenced by Genewiz (Genewiz LLC, NJ). Forward and reverse sequences were analyzed in GENEIOUS software v. 10.2.6 (Kearse et al., 2012) for manual editing and alignment.
2.6. Amplicon-based identification
First investigations on the identity of the rust sampled across the southeastern United States were undertaken by using manually curated ITS and LSU sequences for BLASTn searches against the GenBank database (https://blast.ncbi.nlm.nih.gov/Blast.cgi) and aligned for comparison to validate affiliation to the genus Melampsoridium.
2.7. Phylogenetic analysis
LSU and ITS sequences were used to reconstruct the phylogenetic position of the IHLR taxon observed across the southeastern United States, notably resolving its position within the genus Melampsoridium. Sequences obtained in this study and voucher sequences from previously published work (Liang et al., 2006; McKenzie et al., 2013; Bubner et al., 2019) were aligned using the MUSCLE (Edgar, 2004) plugin for GENEIOUS 10. Sequences were visually edited to treat ambiguities and sequencing errors. Independent phylogenetic analyses based on single locus data were undertaken using the RAxML plugin (Stamatakis, 2014) and the MrBayes plugin (Huelsenbeck and Ronquist, 2001) within GENEIOUS 10. Sixteen sequences were aligned for an ITS-based analysis (505 nucleotides) and 15 sequences for an LSU-based analysis (455 nucleotides). The general time reversal (GTR) evolutionary model was used with rapid bootstrapping and 1,000 bootstrapping replications. Pucciniastrum coryli isolate TSH-R4237 (IBA8641) was used as an outgroup to root the trees, given that it is a closely related taxon in the Pucciniastraceae (Aime et al., 2018). All the taxa used in this phylogenetic study and their GenBank accession numbers are reported in Table 1. A multilocus approach was also undertaken by using a concatenated dataset of 960 nucleotides to run Bayesian and maximum likelihood phylogenetic analysis.
3. Results
3.1. Incidence and severity
The disease survey and sample collection in this study include locations in the states of Florida, Georgia, the Carolinas, Tennessee, and Texas (Figure 2, Table 1). In this survey, the rust disease incidence and severity on Carpinus caroliniana and Ostrya virginiana were recorded at two locations in Gainesville, Alachua County, Florida, in July 2020. The sample tree selection was arbitrary. At the time of observation, all sampled trees from the two locations (n = 42; 100% incidence) showed uredinial sporulation on the leaves. The average severity recorded on Carpinus caroliniana leaves was 13.17% at John Mahon Nature Park and 7.25% at Loblolly Woods, while on Ostrya virginiana, it was 14.64% at the former and 11.34% at the latter. In addition to the foliar infection, uredinia were produced on samara wings on Carpinus caroliniana in late summer (Figure 1G). Rust severity data were recorded for the samara infection on two Carpinus caroliniana individuals at the Loblolly Woods location (n = 5 per tree), where the average value was 33.3%. No comprehensive survey for the incidence and severity of rust on the two tree species was undertaken at the locations in the other five states. However, the level of rust sporulation across the samples collected at all sites appears consistent.
3.2. Morphological observations
3.2.1. Light microscopy
3.2.1.1. Conspecificity of El Salvador sample with IHLR from the southeastern United States
While testing the conspecificity of the El Salvador sample with IHLR, only the width of urediniospores was statistically different (Welch t-test p-value = 0.0003) between the sample from El Salvador (average of 10.88 μm ± 1.12) and specimens collected in the southeastern US (12.03 μm ± 1.44). A principal component analysis including IHLR and M. asiaticum specimens on the same morphological traits allows further comparisons to investigate the affinities between the two taxa. The first two components represent more than 87% of the variance in the original five-dimensional dataset and allow a similarly reliable assessment using this technique. The ellipse centroids are close to each other, while the point projections appear to be slightly spread for M. asiaticum (Supplementary Figure 1). This reflects the pairwise statistical analysis where width, perimeter, and area traits are comparable at a 5% confidence level and roundness at a 1% confidence level. However, the length trait is significantly different between the two groups.
A similar pattern of variability is observed when plotting roundness against the length of the urediniospores from the El Salvador sample (n = 20) and a selection of samples from across the southeastern US (n = 120) (Supplementary Figure 2). This is confirmed by a comparison of roundness:length ratios that are comparable for all IHLR samples (n = 215 urediniospores) and the El Salvador sample (n = 20 urediniospores), with an average value of 0.056 for both.
3.2.1.2. Pairwise comparison of Melampsoridium
Pairwise comparisons of morphological traits between every recognized species in the genus Melampsoridium revealed diagnostic features and allowed for the observation of morphological affinities. Urediniospore length was statistically different for all taxa except between IHLR and M. carpini specimens (Welch t-test p-value on log10 transformed data = 0.6724) and between M. alni and M. hiratsukanum specimens (Welch t-test p-value on log10 transformed data = 0.3875). Urediniospore width is diagnostic for most taxa but could not discriminate between M. alni/M. hiratsukanum (Welch t-test p-value = 0.2966), M. asiaticum/M. carpini (Kruskal–Wallis p-value = 0.3875), and IHLR/M. asiaticum (Welch t-test p-value = 0.7023). Urediniospore perimeter and area in a similar way segregate all taxa combinations except M. betulinum/M. hiratsukanum (Kruskal–Wallis p-value = 0.1169 and Welch t-test p-value = 0.8450, respectively) and IHLR/M. asiaticum (Welch t-test p-values = 0.2517 and 0.9047, respectively). The urediniospore roundness trait significantly discriminates species in only 7 out of the 15 possible combinations in the genus and does not appear to be a reliable diagnostic tool by itself. Statistical differences and similarities among taxa in pairwise comparisons are presented in a heat map (Figure 3). A more detailed heat map including p-values is presented in Supplementary Figure 3. Its visual observation allows a preliminary interpretation of the morphological affinities of the different taxa within the genus, i.e., taxa with comparable traits. The overall size of the spores (area) is comparable for M. hiratsukanum (286.67 μm2) and M. betulinum (288.19 μm2) (Welch t-test p-value = 0.8450). However, the specimens from these two taxa differ in their length (Welch t-test p-value on square root transformed data = 1.35 E-05, 5.163664 and 5.436891 values, respectively) and width (Welch t-test p-value = 0.0002, 13.82 μm and 12.76 μm values, respectively). Specimens of M. alni (13.98 μm) and M. hiratsukanum (13.81 μm) are similar in terms of width (Welch t-test p-value = 0.6823). Their overall size (area) is also not statistically different at a 1% significance level (Welch t-test p-value = 0.0218, 319.71 μm2 and 288.19 μm2 values, respectively). Finally, M. alni and M. betulinum specimens have comparable lengths as explained above with log10 transformed average measurements of 1.46 and 1.47, but also area values that are not statistically different at a 1% significance level (Welch t-test p-value = 0.0156, 319.71 μm2 and 286.67 μm2 values, respectively).
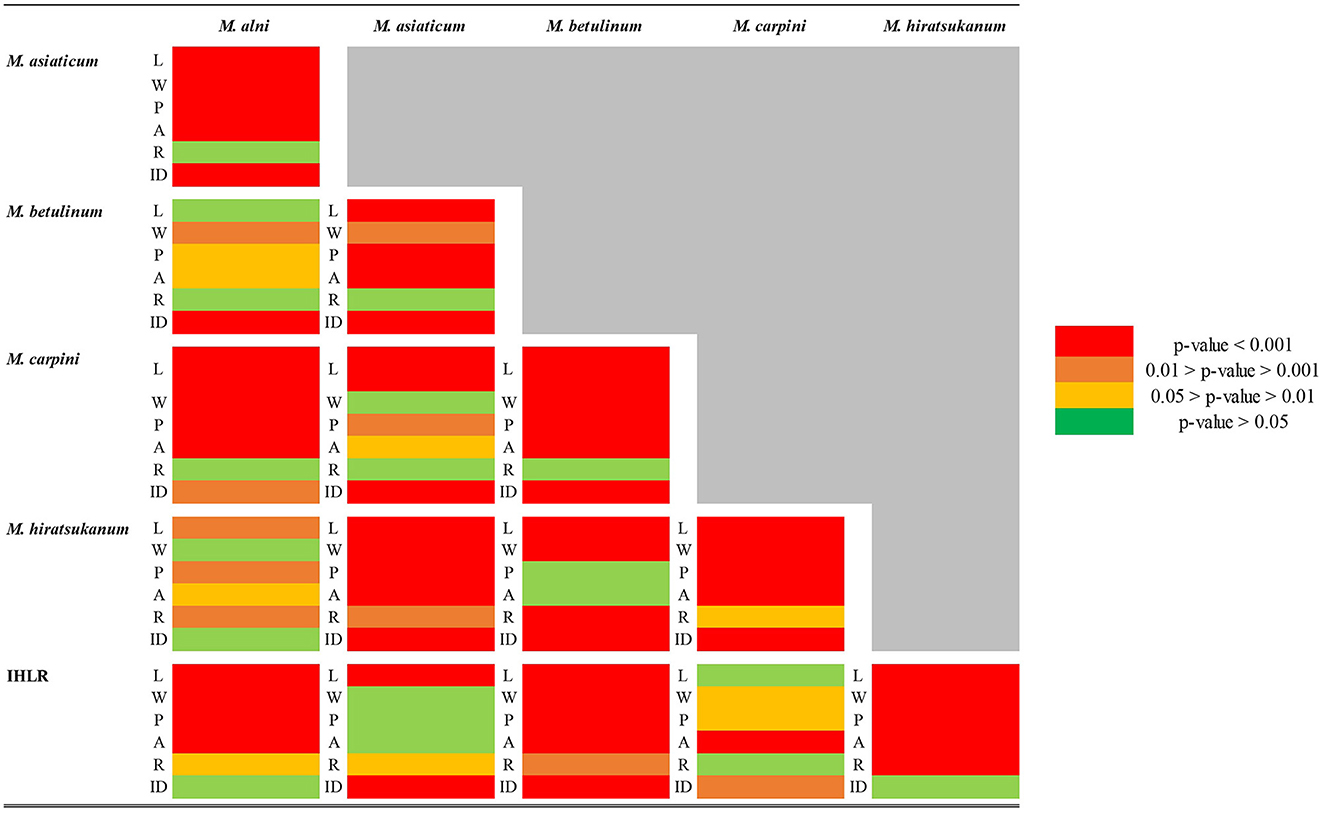
Figure 3. Heat map table of the p-values obtained from pairwise comparison of different urediniospore morphological traits (L = length; W = width; P = perimeter; A = area; and R = roundness) among the Melampsoridium genus. Welch t-test was used when data normality and variance homogeneity assumptions were satisfied. Kruskal–Wallis test was used when one or both of the data normality and/or variance homogeneity assumptions were not satisfied. When not satisfied with the original measurements, the normality of the distribution was tested on logarithm base 10 (log10) and square root (SQRT) transformed data.
The two taxa with the most comparable traits appear to be IHLR and M. asiaticum, with statistically comparable width (11.93 μm and 12.03 μm values, respectively, Welch t-test p-value = 0.7023), perimeter (56.91 μm and 57.91 μm values, respectively, Welch t-test p-value = 0.2517), and area values (203.42 and 204.16 values, respectively, Welch t-test p-value = 0.9047). Moreover, there is no statistical difference at a significance level of 1% regarding the roundness trait (1.20 and 1.26 values, respectively, Kruskal–Wallis p-value = 0.0174). IHLR specimen measurements are similar to those of M. carpini on urediniospore length log10 transformed values (Welch t-test p-value on log10 transformed data = 0.6724), where both values = 1.34. Finally, morphological affinities are also observed between M. carpini and M. asiaticum, whose average width is comparable (Kruskal–Wallis p-value = 0.2966, values of 11.39 μm and 12.03 μm, respectively). The average area is also comparable between the two taxa at a significance level of 1% (Kruskal–Wallis p-value = 0.0238, values of 188.30 μm and 204.16 μm, respectively). The pooled average values for each trait and per taxon are presented in Table 3.
The principal component analysis (PCA) based on five morphological traits (length, width, perimeter, area, and roundness) allows for a visual assessment of the relationships among the genus (Figure 4). The two first components represent more than 90% of the variance in the original five-dimensional dataset and provide a reliable assessment using this technique. The ellipse centroids appear to group in two clusters. The first includes M. alni, M. betulinum, and M. hiratsukanum species, while the second includes IHLR specimens of M. asiaticum and M. carpini. This is congruent with the results obtained from the pairwise comparisons above. This trend is also observable from the boxplot graph of the urediniospore area in the Supplementary Figure 4.
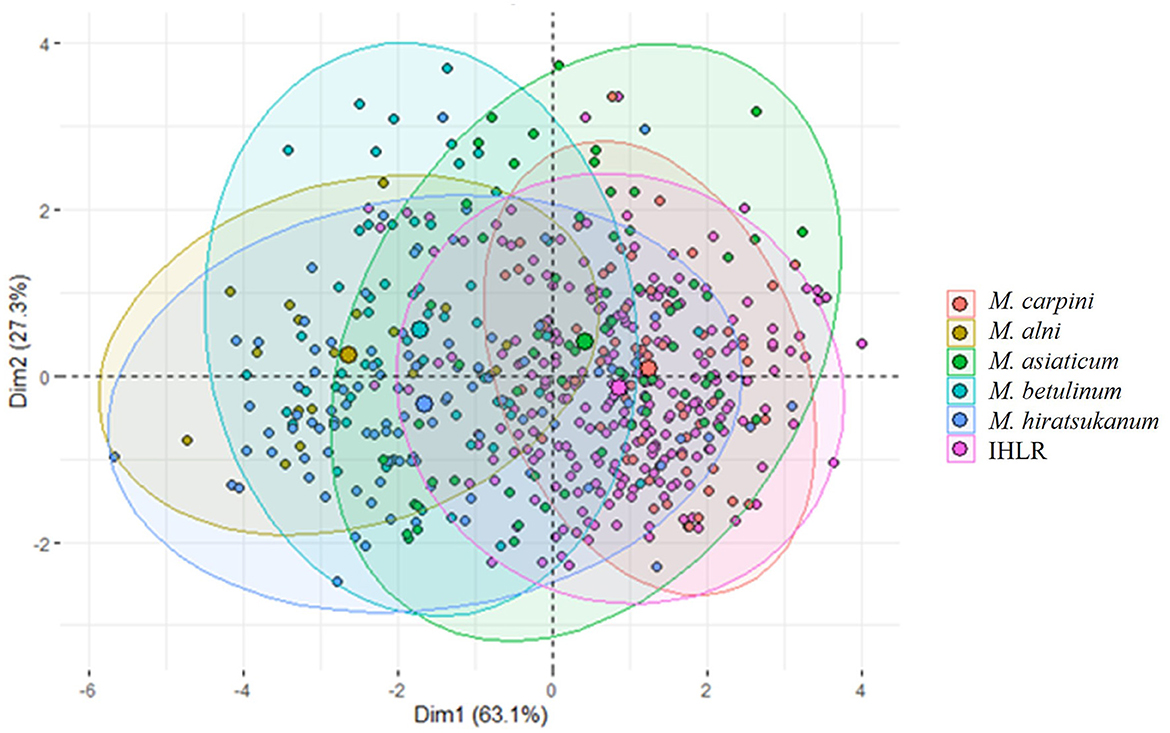
Figure 4. Principal component analysis of Melampsoridium species and IHLR specimens based on urediniospore morphology. Each point projection is based on urediniospore length, width, perimeter, area, and roundness measurement. Ellipses have 95% interval confidence; their respective centroid corresponds to the barycenter belonging to the intervals.
3.2.1.3. IHLR morphological description
The IHLR taxon across the southeastern United States and El Salvador presents the following morphological characteristics: spermogonia and aecia unknown, uredinia hypophyllous, scattered or aggregate, round (0.05–0.30 mm), yellow to orange, ostiolar cells extending in an acute apex (Figure 5A), urediniospores are obovate, (15–)17–27(−31) × (8–)9–15 μm wide, walls 1–3 μm thick, echinulate, colorless. Cytoplasm is orange (Figure 5B), germ pores are inconsistently observed (Figures 5C, D), mostly subequatorial. Telia was not observed.
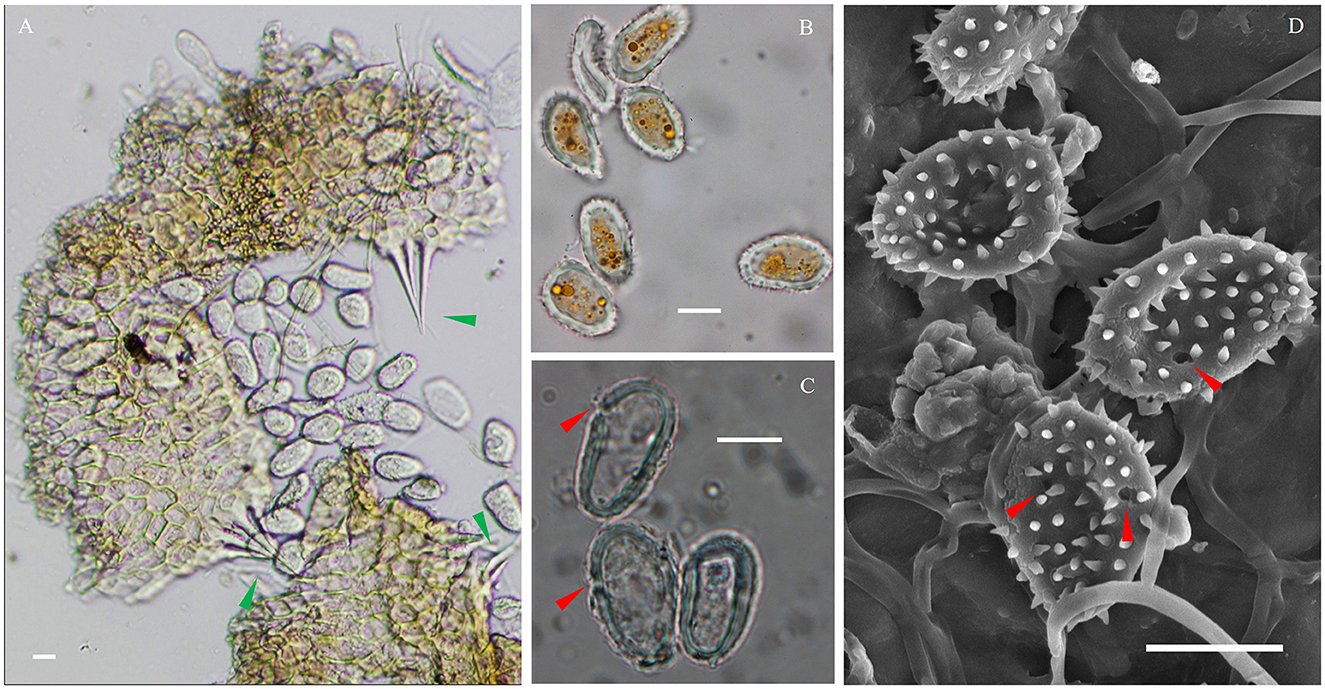
Figure 5. Microscopic observations of the IHLR FLCc1 specimen. (A) Urediniospores released from uredinia through ostioles. (B) Urediniospores general aspect and orange cytoplasm. (C, D) Germ pores in a subequatorial position. Green arrows indicate ostiolar cells, and red arrows indicate germ pores. Bars are 10 μm.
3.2.2. Scanning electron microscopy
Micrographs were obtained from 13 specimens to assess urediniospore echinulation patterns (smooth apex vs. uniformly echinulate). First, specimens FLCc1 and SalOv1 collected from Florida on Carpinus caroliniana and from El Salvador on Ostrya virginiana var. guatemalensis consistently had a homogeneous echinulation pattern, supporting their conspecificity (Figure 6). Similarly, M. alni (MICH 255953), M. asiaticum (IBAR 6599, IBAR 10328, and IBAR 10339), and M. hiratsukanum (PUR 006499, PUR 006500, PUR 006506, PUR 006509, and PUR 006511) specimens consistently show urediniospores with a homogeneous echinulation pattern. On the contrary, M. betulinum (MICH 255975, MICH 255977, and PUR 006358) and M. carpini (BPI 910402) specimens consistently have urediniospores with a bold apex. These observations (Figures 7, 8) agree with the previously published literature. Additionally, specimen PUR006358, labeled M. carpini and collected by Kauffman in 1914 in New York on Ostrya virginiana, was identified as M. betulinum by Kaneko and Hiratsuka (1981) based on the size of the urediniospores. Similarly, several specimens collected by Kauffman in 1914 at the MICH herbarium (e.g., MICH 255975 and MICH 255977) named M. betulae should be considered as M. betulinum. Close observations of the leaves included in these specimens indicate that the host is likely Betula alleghaniensis (yellow birch), which supports the species determination of M. betulinum. Interspine distance was also determined for every taxon and was diagnostic in most taxa combinations except M. alni/M. hiratsukanum (Welch t-test p-value on log10 transformed data = 0.0664), M. alni/IHLR (Welch t-test p-value = 0.6022), and M. hiratsukanum/IHLR (Welch t-test p-value on square root transformed data = 0.3557).
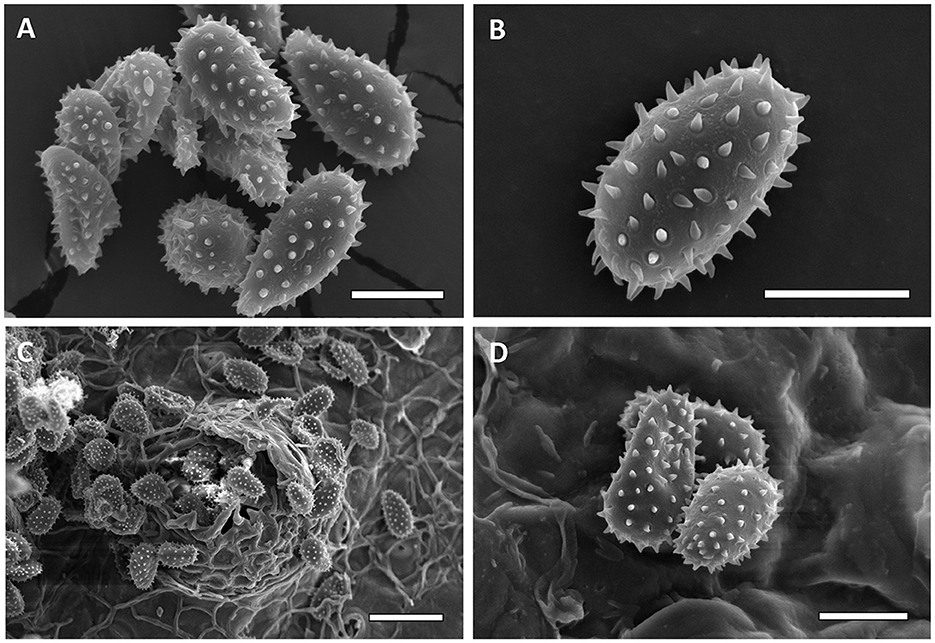
Figure 6. Scanning electron micrographs of urediniospores from FLCc1 IHLR sample [(A, B)—Bar = 10 μm] and SALOv1 IHLR sample [(C)—bar = 25 μm, (D)—bar = 10 μm].
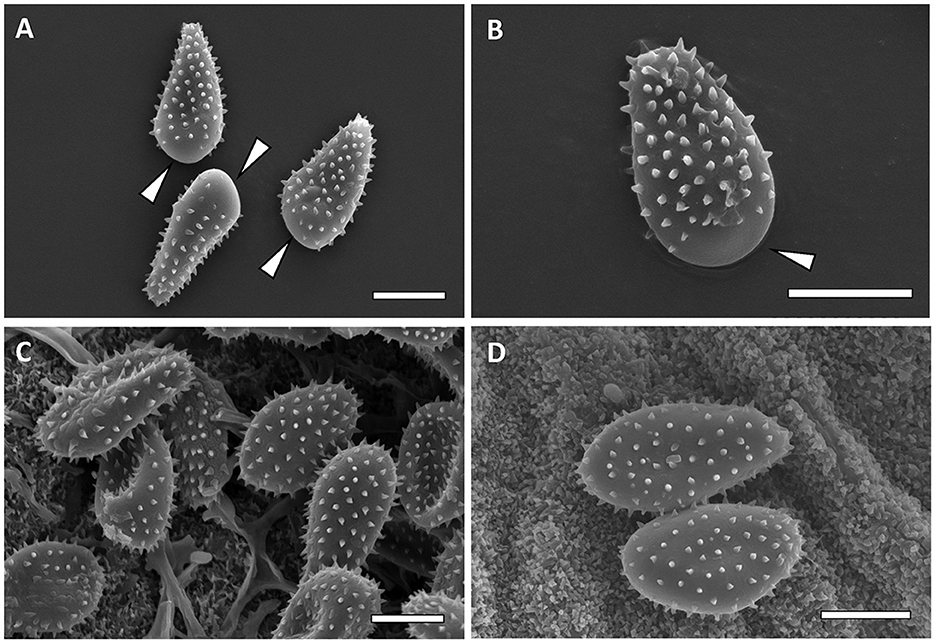
Figure 7. Scanning electron micrographs of urediniospores from M. carpini [(A, B)—Bar = 10 μm] and M. hiratsukanum [(C, D)—Bar = 10 μm]. Bald apex of the spores is indicated by white arrows.
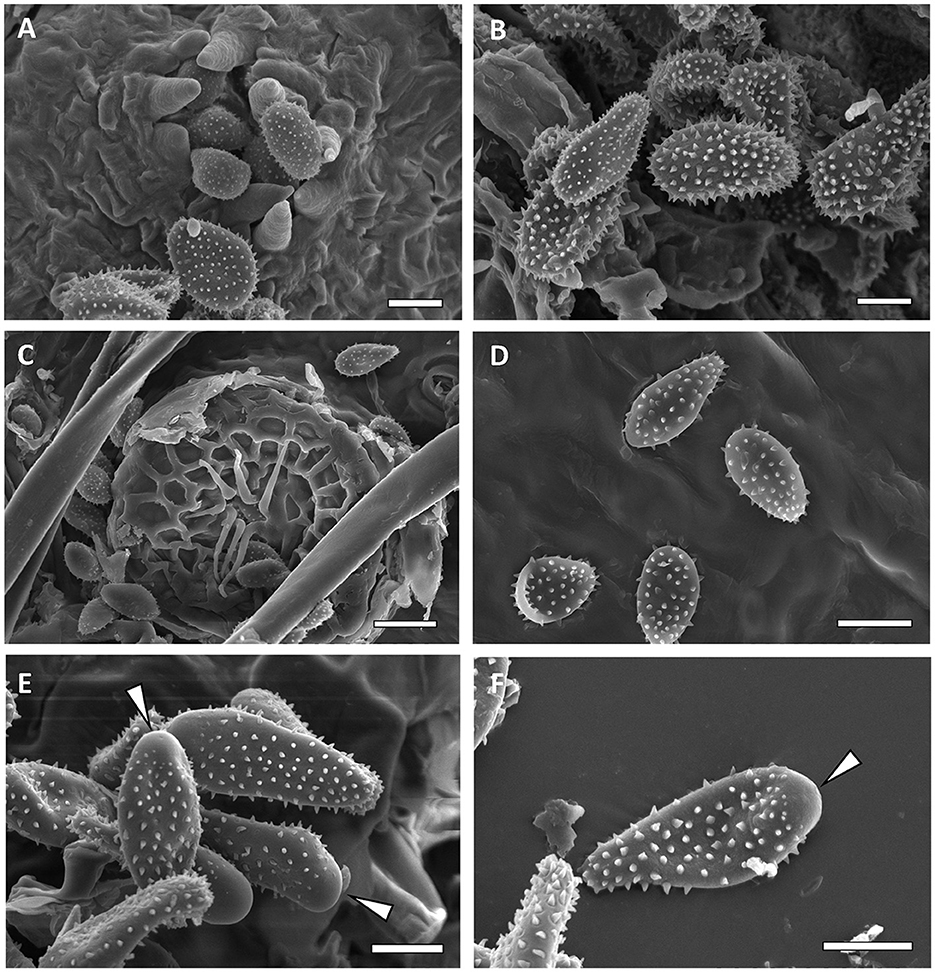
Figure 8. Scanning electron micrographs of urediniospores from M. alni [(A, B)—Bar = 10 μm], M. asiaticum [(C)—bar = 10 μm, (D)—bar 20 = μm], and M. betulinum [(E, F)—Bar = 10 μm]. Bald apex of the spores is indicated by white arrows.
3.3. Sanger sequencing and phylogenetic analysis
In total, 35 sequences were generated in this study, including 24 sequences for the emergent IHLR taxon for each of the 11 locations in the southeastern United States, as well as for the sample obtained from El Salvador. In addition, sequences used in this study were downloaded from GenBank and obtained from published studies (Table 1). DNA extraction was attempted on all requested herbarium specimens, but only the relatively recently collected specimen PUR 006500 (1977, M. hiratsukanum from El Salvador) resulted in the amplification of a DNA sequence from the LSU locus (GenBank accession number OQ371317). However, a partial LSU sequence was obtained from the M. asiaticum specimen TMI 7277 collected in 1941 from Japan but was not included in the phylogenetic analysis due to its short length (223 bp). Only one locus was available for M. hiratsukanum specimen PUR 006500 (LSU) and for M. alni strain J0525, M. asiaticum IBAR 10339 (GenBank accession number OQ366471), and M. carpini KR-M-0048587 (ITS).
ITS and LSU sequences obtained from samples of the IHLR taxon across the southeastern United States and from the El Salvador sample are 100% similar, supporting the hypothesis of conspecificity. The sequences were deposited in GenBank (accession numbers are reported in Table 1) and searched against the NCBI GenBank database using the BLASTn function. The ITS analysis revealed a 93.9% homology with the Melampsoridium hiratsukanum specimen KF031553 (on Alnus incana from Finland), while the nuLSU analysis revealed 97.5% homology with Melampsoridium hiratsukanum (accession number KC 313888) sampled from white alder (Alnus rhombifolia) in California. Bayesian and maximum likelihood analysis yielded comparable topologies with six supported terminal clades corresponding to the five described species: M. betulinum (ML–BS = 100%, PP = 1), M. alni (ML–BS = 94%, PP = 0.83), M. asiaticum (ML–BS = 99%, PP = 0.99), M. carpini (ML–BS = 100%, PP = 0.99), and IHLR specimens. The clade including M. hiratsukanum specimens is weakly supported by the maximum likelihood analysis (BS value = 39%) and moderately supported using the Bayesian inference method (PP = 0.78). The only discrepancy between the two methods corresponds to M. hiratsukanum specimen PUR 006500 falling between M. alni and the two other M. hiratsukanum specimens with the Bayesian inference method. All M. alni, M. betulinum, and M. hiratsukanum specimens fall into a highly supported subclade (ML–BS = 98%, PP = 1), hereafter called Clade A. IHLR specimens are grouped with M. asiaticum specimens in a second subclade (ML–BS = 90%, PP = 1) called Clade B. Finally, M. carpini specimens are clustering into their own group (ML–BS = 100%, PP = 1) called Clade C. Both analyses display M. betulinum, M. hiratsukanum, M. asiaticum, and IHLR specimens grouped in one clade, while M. carpini specimens fall into another one (Clade C). However, that aspect is not statistically supported. The topology obtained from the maximum likelihood analysis is presented in Figure 9.
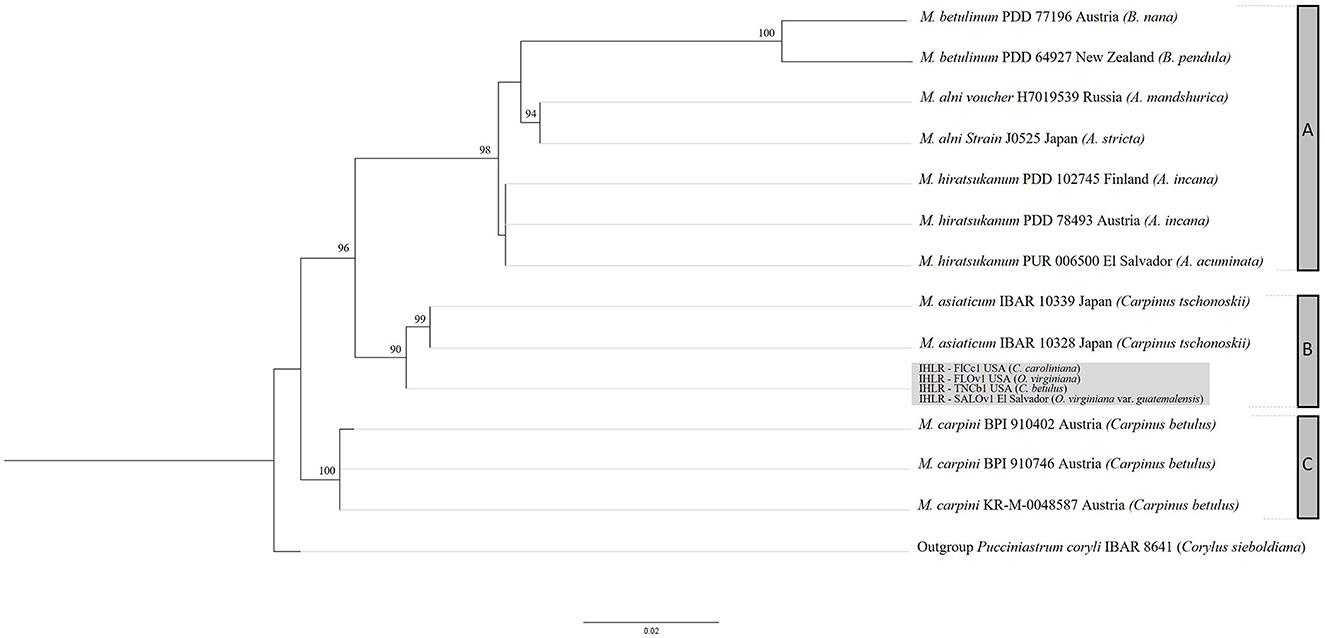
Figure 9. RAxML topology obtained from a 960 nucleotide multilocus alignment (LSU + ITS) under a GTR model with 1,000 bootstrap replicates. Bootstrap values below 75% are not shown. IHLR specimens are in the light gray box. Melampsoridium tree hosts are mentioned in parenthesis. Clade A includes M. betulinum, M. alni, and M. hiratsukanum specimens. Clade B includes M. asiaticum and IHLR specimens, and clade C includes M. carpini specimens. Specimen IBAR 8641 (Pucciniastrum coryli) is used as an outgroup.
4. Discussion
Prior to the report in this study, there was no record of rust disease on Carpinus and Ostrya in the southeastern United States. Typically, rust epidemics in this tree host family are caused by Melampsoridium species. Simple morphological investigations, notably through observations of urediniospore echinulation patterns, allow for differentiation of IHLR from M. betulinum and M. carpini (homogeneously echinulated vs. bald apex, respectively). The ecology of M. hiratsukanum, M. alni, and M. betulinum, with known macrocyclic life cycles, i.e., alternating Larix spp. as gametophyte hosts and alder or birch as sporophyte host species, and other morphological differences, such as greater urediniospore size (length, perimeter, or area), support being non-conspecific with IHLR. The diagnostic features used in this study are summarized in Table 3. These discrepancies are also supported by the molecular analysis, where M. hiratsukanum, M. alni, and M. betulinum are clustered in Clade A. The reconstruction of the genus phylogeny also confirms the IHLR assignment to Melampsoridium. More precisely, IHLR specimens form a well-supported subclade with M. asiaticum specimens. In addition, the dichotomy within the genus between clades B and C (M. carpini, M. asiaticum, and IHLR) and clade A (M. alni, M. betulinum, and M. hiratsukanum) indicates that some taxonomic adjustments might be needed to better accommodate these discrepancies. Taxa from clades B and C do not have a known gametophyte host; their sporophyte hosts belong to both Ostrya and Carpinus and have overall smaller urediniospores. On the contrary, the taxa belonging to Clade C have known gametophyte hosts (Larix spp.), alder and/or birch species as sporophyte hosts, and present bigger urediniospores.
In this context, we propose the designation Melampsoridium cf. asiaticum to refer to IHLR specimens. The two groups (IHLR and M. asiaticum specimens) have Ostrya and Carpinus species as hosts; quantitative morphological traits (urediniospore width, perimeter, and area) are statistically similar; and no gametophyte host has been observed for IHLR and M. asiaticum specimens. However, a few discrepancies are noted (e.g., urediniospore length feature), and nuclear ITS and LSU sequences differ as well (13 polymorphic sites total), resulting in IHLR and M. asiaticum specimens being sister subclades in the topology of the reconstructed phylogeny. Additional DNA information and sequences at the genus level would allow the better phylogenetic signal resolution needed to confirm IHLR identity. The denomination Melampsoridium cf. asiaticum is justified by these discrepancies, which may be due to local adaptation following introduction from Asia or correspond to the characteristics specific to an emergent, novel taxon in the genus.
IHLR specimens from El Salvador and the ones isolated across the southeastern United States share the exact same ITS and LSU sequences. The conspecificity of these isolates raises the question of whether an introduction was first made into Central America or there was a second introduction into the southeastern United States, and therefore the current geographical range of the epidemics needs further study. Overwintering is likely to occur in tropical or subtropical regions (Florida and/or Central America) through repetitive uredinial infections. The observed coexistence of new leaves and infected leaves from previous growth year (Figures 1G, H) support that statement. While a survey with several time points would be necessary and informative, the IHLR geographical distribution area and severity in the southeastern United States likely increase as temperature and humidity, especially in spring and summer, increase as well. Despite the isolated sites of this survey across the southeastern United States, our data suggests that the IHLR pathogen is successfully established in this area. The fitness of the host species in situ can be significantly impacted given the observed incidence and severity data, as well as the ability of the rust to infect seedlings and samaras (Figure 1I). Uredinial sporulation on Carpinus caroliniana samaras represents a novel aspect of the infection biology of a taxon in the Melampsoridium genus on its host.
Data availability statement
The datasets presented in this study can be found in online repositories. The names of the repository/repositories and accession number(s) can be found in the article/Supplementary material.
Author contributions
NA and JS designed the research. NA, JS, YO, and CA performed the sampling. NA, BH, and JS performed the molecular analyses. NA, BH, YO, and RB performed the microscopic analyses. NA analyzed the data and wrote the manuscript with inputs from JS, YO, CA, RB, and BH. All authors contributed to the article and approved the submitted version.
Conflict of interest
The authors declare that the research was conducted in the absence of any commercial or financial relationships that could be construed as a potential conflict of interest.
Publisher's note
All claims expressed in this article are solely those of the authors and do not necessarily represent those of their affiliated organizations, or those of the publisher, the editors and the reviewers. Any product that may be evaluated in this article, or claim that may be made by its manufacturer, is not guaranteed or endorsed by the publisher.
Supplementary material
The Supplementary Material for this article can be found online at: https://www.frontiersin.org/articles/10.3389/ffgc.2023.1212192/full#supplementary-material
References
Aime, M. C. (2006). Toward resolving family-level relationships in rust fungi (Uredinales). Mycoscience 47, 112–122. doi: 10.1007/S10267-006-0281-0
Aime, M. C., Bell, C. D., and Wilson, A. W. (2018). Deconstructing the evolutionary complexity between rust fungi (Pucciniales) and their plant hosts. Stud. Mycol. Lead. Women Fungal Biol. 89, 143–152. doi: 10.1016/j.simyco.2018.02.002
Aime, M. C., McTaggart, A. R., Mondo, S. J., and Duplessis, S. (2017). “Chapter seven - phylogenetics and phylogenomics of rust fungi,” in Advances in Genetics, Fungal Phylogenetics and Phylogenomics, eds. J. P., Townsend, Z., Wang (New York, NY: Academic Press) 267–307. doi: 10.1016/bs.adgen.2017.09.011
Bubner, B., Buchheit, R., Friedrich, F., Kummer, V., and Scholler, M. (2019). Species identification of European forest pathogens of the genus Milesina (Pucciniales) using urediniospore morphology and molecular barcoding including M. woodwardiana sp. nov. MycoKeys 48, 1–40. doi: 10.3897/mycokeys.48.30350
Burns, R. M, and Honkala, B. H. (1990). Silvics of North America. Volume 2. Harwoods., Agriculture Handbook 654. Washington, DC: U.S. Department of Agriculture, Forest Service.
Edgar, R. C. (2004). MUSCLE: a multiple sequence alignment method with reduced time and space complexity. BMC Bioinf. 5, 113. doi: 10.1186/1471-2105-5-113
Engler, A., and Prantl, K. (1899). “Uredinales (Nachträge),” in Die Natürlichen Pflanzenfamilien 546–553.
Gardes, M., and Bruns, T. (1993). ITS primers with enhanced specificity for basidiomycetes-application to the identification of mycorrhizae and rusts. Molec. Ecol. 2, 113–118. doi: 10.1111/j.1365-294X.1993.tb00005.x
Hantula, J., Kurkela, T., Hendry, S., and Yamaguchi, T. (2009). Morphological measurements and ITS sequences show that the new alder rust in Europe is conspecific with Melampsoridium hiratsukanum in eastern Asia. Mycologia 101, 622–631. doi: 10.3852/07-164
Hantula, J., Stringer, R. N., Lilja, A., and Kurkela, T. (2012). Alder rust, Melampsoridium hiratsukanum Ito, identified from Wales, UK and British Columbia, Canada. Forest Pathol. 42, 348–350. doi: 10.1111/j.1439-0329.2012.00761.x
Hiratsuka, N., Sato, S., Katsuya, K., Kakishima, M., Hiratsuka, Y., Kaneko, S., et al. (1992). “Melampsoridium Klebahn 1899,” in The Rust Flora of Japan (Ibaraki, Japan: Sukuba Shuppankai Takezono).
Huelsenbeck, J. P., and Ronquist, F. (2001). MRBAYES: Bayesian inference of phylogenetic trees. Bioinformatics 17, 754–755. doi: 10.1093/bioinformatics/17.8.754
Kaneko, S., and Hiratsuka, N. (1981). Classification of the Melampsoridium species based on the position of urediniospore germ pores. Trans. Mycol. Soc. Japan 22, 463–473.
Kaneko, S., and Hiratsuka, N. (1983). A new species of Melampsoridium on Carpinus and Ostrya. Mycotaxon 18, 1–4.
Kearse, M., Moir, R., Wilson, A., Stones-Havas, S., Cheung, M., Sturrock, S., et al. (2012). Geneious Basic: An integrated and extendable desktop software platform for the organization and analysis of sequence data. Bioinformatics 28, 1647–1649. doi: 10.1093/bioinformatics/bts199
Kurkela, T., Hanso, M., and Hantula, J. (1999). Differentiating characteristics between melampsoridium rusts infecting birch and alder leaves. Mycologia 91, 987–992. doi: 10.1080/00275514.1999.12061108
Liang, Y.-M., Tian, C.-M., and Kakishima, M. (2006). Phylogenetic relationships on 14 morphologically similar species of Pucciniastrum in Japan based on rDNA sequence data. Mycoscience 47, 137–144. doi: 10.1007/S10267-006-0284-X
Mamet, S. D., Brown, C. D., Trant, A. J., and Laroque, C. P. (2019). Shifting global Larix distributions: Northern expansion and southern retraction as species respond to changing climate. J. Biogeogr. 46, 30–44. doi: 10.1111/jbi.13465
McKenzie, E. H. C., Padamsee, M., and Dick, M. (2013). First report of rust on Alnus in New Zealand is Melampsoridium betulinum, not M. hiratsukanum. Plant Pathol. Quarant. 3, 59–65. doi: 10.5943/ppq/3/2/1
Nesom, G., Briggs, R., and Moore, L. (2003). “American hornbeam,” in Plant Fact Sheet/Guide Coordination 2.
Pethybridge, S. J., and Nelson, S. C. (2015). Leaf doctor: a new portable application for quantifying plant disease severity. Plant Dis. 99, 1310–1316. doi: 10.1094/PDIS-03-15-0319-RE
R Core Team (2023). R: A Language and Environment for Statistical Computing. Vienna: R Foundation for Statistical Computing. Available online at: https://www.R-project.org
Stamatakis, A. (2014). RAxML version 8: a tool for phylogenetic analysis and post-analysis of large phylogenies. Bioinformatics 30, 1312–1313. doi: 10.1093/bioinformatics/btu033
Vilgalys, R., and Hester, M. (1990). Rapid genetic identification and mapping of enzymatically amplified ribosomal DNA from several Cryptococcus species. J. Bacteriol. 172, 4238–4246. doi: 10.1128/jb.172.8.4238-4246.1990
Keywords: Melampsoridium, Carpinus, Ostrya, urediniospore, emergence
Citation: Anger N, Held BW, Blanchette RA, Ono Y, Aime CM and Smith JA (2023) Ironwood/hophornbeam leaf rust, an emergent disease across the southeastern United States affiliated to Melampsoridium asiaticum. Front. For. Glob. Change 6:1212192. doi: 10.3389/ffgc.2023.1212192
Received: 25 April 2023; Accepted: 28 August 2023;
Published: 21 September 2023.
Edited by:
Isabel Alvarez Munck, Forest Service (USDA), United StatesReviewed by:
Angus J. Carnegie, NSW Government, AustraliaOscar Santamaria, University of Valladolid, Spain
Copyright © 2023 Anger, Held, Blanchette, Ono, Aime and Smith. This is an open-access article distributed under the terms of the Creative Commons Attribution License (CC BY). The use, distribution or reproduction in other forums is permitted, provided the original author(s) and the copyright owner(s) are credited and that the original publication in this journal is cited, in accordance with accepted academic practice. No use, distribution or reproduction is permitted which does not comply with these terms.
*Correspondence: Nicolas Anger, bmFuZ2VyJiN4MDAwNDA7dWZsLmVkdQ==
†Present address: Jason A. Smith, Department of Biological and Environmental Sciences, College of Natural and Health Sciences, University of Mount Union, Alliance, OH, United States