- Nanyang Technological University, Asian School of the Environment, Singapore, Singapore
Many ecosystem processes revolve around plant roots and the rhizosphere, but root trait knowledge has generally lagged behind aboveground plant traits from leaves and stems. In particular, root trait representation of Paleotropical species and tropical communities in modern trait databases remains poor, constraining our understanding of belowground processes in these regions. Therefore, we sought to examine the current state of root trait representation and associated topics in the Paleotropics to identify gaps and biases in the existing literature. Using an exhaustive literature scan, we compiled a list of publications that contained various belowground trait information. Subsequently, we utilized a statistical topic modeling method to analyze abstracts of publications in order to identify topics studied alongside root trait documentation. Finally, we consolidated trends in root trait coverage and topics across five geographical regions and four time periods to illustrate shifts in literature and knowledge of ecological processes revolving around roots. Root trait representation was heavily biased toward root biomass but other aspects of root systems such as physiology, architecture and anatomical traits remain underrepresented. We categorized 23 unique topics around root trait literature across eight categories: ecosystem productivity and biomass stocks, plant functional traits, resource availability, ecosystem processes and dynamics, mycorrhizal colonization, edaphic processes, seedling experiments, and global change and variation. Traits and topic coverage were unequally distributed across the Paletropics and exhibited a notable shift in focus from resource limitation and mycorrhizae research to root trait variation at large spatial and temporal scales over the last 50 years of root trait literature. Given these trends and the heterogenous effects global change exerts on the tropics at a regional scale, we provided several recommendations to facilitate inter-study comparisons of traits to advance the field’s understanding of belowground ecosystem processes in pantropical communities.
1. Introduction
Advancements in trait-based ecology have provided plant ecologists powerful new avenues to understand how plant communities interact with their associated environmental pressures to drive ecosystem processes. Specifically, the study of functional traits has developed novel insights into the resource acquisition strategies, life histories, growth and performance of plants (Grime et al., 1997; Lavorel and Garnier, 2002; Violle et al., 2007) under the influence of multiple resource constraints (Lambers et al., 2008; Poorter and Markesteijn, 2008; Pérez-Ramos et al., 2012) and defoliation pressures (Archibald et al., 2019; Boonman et al., 2020). Furthermore, as plant communities respond to multiple environmental constraints by expressing various trait syndromes, these traits may simultaneously shape broader ecosystem processes such as carbon sequestration and net primary productivity (Funk et al., 2017; Freschet et al., 2021).
Currently, the field of plant functional ecology is skewed toward aboveground organs (i.e., leaves and stems) while neglecting belowground components such as the roots and the rhizosphere. This bias is likely due to the difficulty in accessing and observing plant organs beneath the soil and the complex interactions between root, mycorrhizae and soil microbes that govern plant–soil feedbacks (Finzi et al., 2015; Fujii et al., 2018). Leaves contain the photosynthetic machinery of plants; thus, their traits directly determine plant growth rate and carbon sequestration capacity (Poorter and Bongers, 2006). However, the photosynthetic capacity of leaves is sustained by water and nutrient availability (Riddoch et al., 1991; Tezara et al., 1999), implying that root traits indirectly control plant growth and performance by mediating belowground resource acquisition. In tropical ecosystems where either water or nutrients are often limited in availability, belowground resource acquisition becomes a primary determinant of plant productivity shaped by root traits and plant–soil interactions in the rhizosphere (Finzi et al., 2015; Nasto et al., 2019; Lugli et al., 2021; Reichert et al., 2022). Furthermore, recent studies have supported the importance of belowground resource acquisition by examining how droughts (Guan et al., 2015; Corlett, 2016) and nutrient limitation (Wieder et al., 2015; Cunha et al., 2022) control plant productivity and performance in the tropics. However, compared to leaf traits, much less is known about root traits and how these traits control resource uptake across climatic and edaphic gradients.
In addition to belowground resource acquisition, roots participate in many other ecosystem processes. For example, a significant proportion of carbon in terrestrial ecosystems is stored in the roots (Jackson et al., 1997), making plant root systems a significant carbon sink worldwide. Roots also mediate belowground processes by hosting communities of mycorrhizal symbionts and microbes in the rhizosphere that influence the decomposition of plant litter and the exchange of nutrients (Gadgil and Gadgil, 1971; Finzi et al., 2015; Taylor et al., 2016), all of which maintain the nutrient cycling process that sustains ecosystem productivity. Several taxonomic clades within the Fabaceae family house important N-fixing bacteria within their root nodules, contributing to nitrogen availability within the soil (Tedersoo et al., 2018). Hence, a holistic understanding of root traits and the rhizosphere may aid us in capturing essential aspects of ecosystem processes hidden belowground to improve current global vegetation models to predict future ecosystem productivity and forest demographics.
Most of our current knowledge of root traits and rhizosphere communities originates from empirical studies in temperate regions from the global north. However, tropical communities possess significantly different abiotic conditions and far greater plant diversity (Kier et al., 2005) than temperate regions. Even within the tropics, there is significant variation in species composition and edaphic conditions (e.g., Townsend et al., 2008; Menge et al., 2019); yet most tropical empirical observations are concentrated in Neotropical sites from the Amazon and Central Americas. For example, many Paleotropics forests in Africa and Southeast Asia are uniquely characterized by ectomycorrhizal-associating species as opposed to Neotropical forest communities (Newbery et al., 1997; Slik et al., 2003), whereas N-fixing species are abundant in the Neotropics but relatively rare elsewhere (Menge et al., 2019). Despite these differences in species composition, root traits from Paleotropical communities represent only 3% of total observations in global databases such as FRED (~2000 out of 57,190 observations; see Iversen and McCormack, 2021) and 2% in GRooT (~2000 out of 114,223 observations; see Guerrero-Ramírez et al., 2021). Achieving a comprehensive characterization of root traits and generalizing their impacts on ecosystem processes will require expansive empirical observations from these underrepresented tropical systems.
Here, we attempted to identify the current state of root trait knowledge within the Paleotropics. Using a wide literature scan, we compiled a list of publications that contained various belowground trait information to address the following research questions and goals:
1. Which categories of root traits should researchers focus on in the Paleotropics? By examining root trait coverage in the existing literature, we make a case that the current trait coverage is missing root traits that address important ecological topics related to global environment change and ecosystem-specific dynamics. In addition, we also demonstrate that “hard” traits that precisely capture aspects of belowground biological function are largely absent from the current literature, which future researchers should focus more on.
2. What are the associated topics studied alongside root traits? Root traits contribute to multiple ecosystem processes simultaneously, including but not limited to carbon storage, nutrient and water acquisition and associations with symbionts (Jackson et al., 1997; Nasto et al., 2019; Reichert et al., 2022). More specifically, we seek to identify differences in topic coverage across studies, reflecting how different research groups prioritize certain topics regarding the role of root traits in ecosystem functioning over others.
3. How are these studies distributed geographically across the Paleotropics? By identifying where root trait information has been reported, we seek to reveal trends in root trait observations across geographical areas and ecosystems which may hinder our understanding of trends in root traits across environmental gradients across the tropics.
4. How have root trait studies changed over time? We examined how trait coverage and publication topics changed over time to capture temporal shifts in research foci. We hypothesize that as techniques to measure root traits and the sharing of trait data through global databases evolve, more recent studies focus on examining broad trends in root trait variation across environmental gradients and how root traits vary with respect to global change.
5. How do these studies differ in their methodologies? Field studies can provide realistic observations of root traits in natural communities, while experimental studies allow us to manipulate environmental variables in a controlled setting. Therefore, we sought to determine differences in the methodologies within both study types to highlight inconsistencies in how root traits are sampled, studied, and reported. Subsequently, we provide recommendations on how studies can be more consistent in the reporting of root trait data across studies.
2. Materials and methods
2.1. Search criteria
We conducted an extensive literature search on Web of Science and Scopus to compile studies containing root trait information from the tropics. Our search was restricted to observational and ex situ studies conducted on natural systems, agroforests, mangroves and their associated plant species while excluding pastures, plantations, agriculture fields, other benthic communities and artificial ecosystems. For a publication to be included in our dataset, it must fulfill two additional criteria: (i) the publication should contain observational or experimental data on at least one root trait (Table 1), and (ii) the publication should be based in tropical regions, but outside the Neotropics (defined as all parts of the tropics outside Hawaii and the Americas). A study was listed to be based in the tropics if it contained at least one study site classified as a tropical climate under the Köeppen-Geiger climatic zone classification or reported as experiencing a “tropical” climate by the authors of the publication themselves. Studies on cosmopolitan species were accepted if the study site or source of plant material was located within the abovementioned geographical boundaries. We excluded studies that did not provide sufficient information to confirm its geographical origin. For meta-analyzes, we examined the geographical origin of their original datasets to verify that at least one tropical region was represented. We conducted the search on 23 Jun 2023, accepting all publications listed up till the search date.
We adopted a modified PRISMA framework (Page et al., 2021) to consolidate relevant documents for this study (Figure 1). Firstly, we searched Web of Science and Scopus using the query (“root*” OR “belowground trait*” or “nutrient uptake” OR “nutrient acquisition” OR “mycorrhiza*” OR “plant functional trait*” OR “BGB” OR “belowground allocation*” OR “P*limit*” OR “N*limit*” OR “nutrient limit*” OR “fine-root*” OR “coarse-root*” OR “soil*” OR “carbon” OR “belowground biomass”) AND (“tropic*”) AND (“*forest*” OR “peatland*” OR “peat swamp*” OR “*montane*” OR “kerangas” OR “agroforest*” OR “mangrove*” OR “grass*” OR “savanna*”) AND NOT (“agri*” OR “arabidopsis” OR “cultivat*” OR “ornamental” OR “education” OR “tourism” OR “learning” OR “toxicology” OR “ecotoxicology” OR “phytoremediation” OR “toxic*” OR “allelopath*” OR “genet*” OR “subtropical” OR “Mediterranean” OR “Middle east*” OR “politic*” OR “plantation*” OR “fish*” OR “aquaculture” OR “seafood” OR “benthic”). Both queries returned over 10,000 entries on both search platforms, but only a minority contained relevant information on root traits. To further reduce the number of publications to examine, we removed publications that did not contain any of the terms (“root” OR “belowground biomass” OR “belowground-biomass” OR “BGB” OR “mycorrhiza” OR “fungi” OR “fungal”) in their abstracts and titles. This substantially reduced the number of relevant publications to ~4,000 unique entries from both search platforms. Subsequently, the titles and abstracts of these publications were examined manually to remove irrelevant publications. Ultimately, we were left with 421 publications for further analysis (Figure 1).
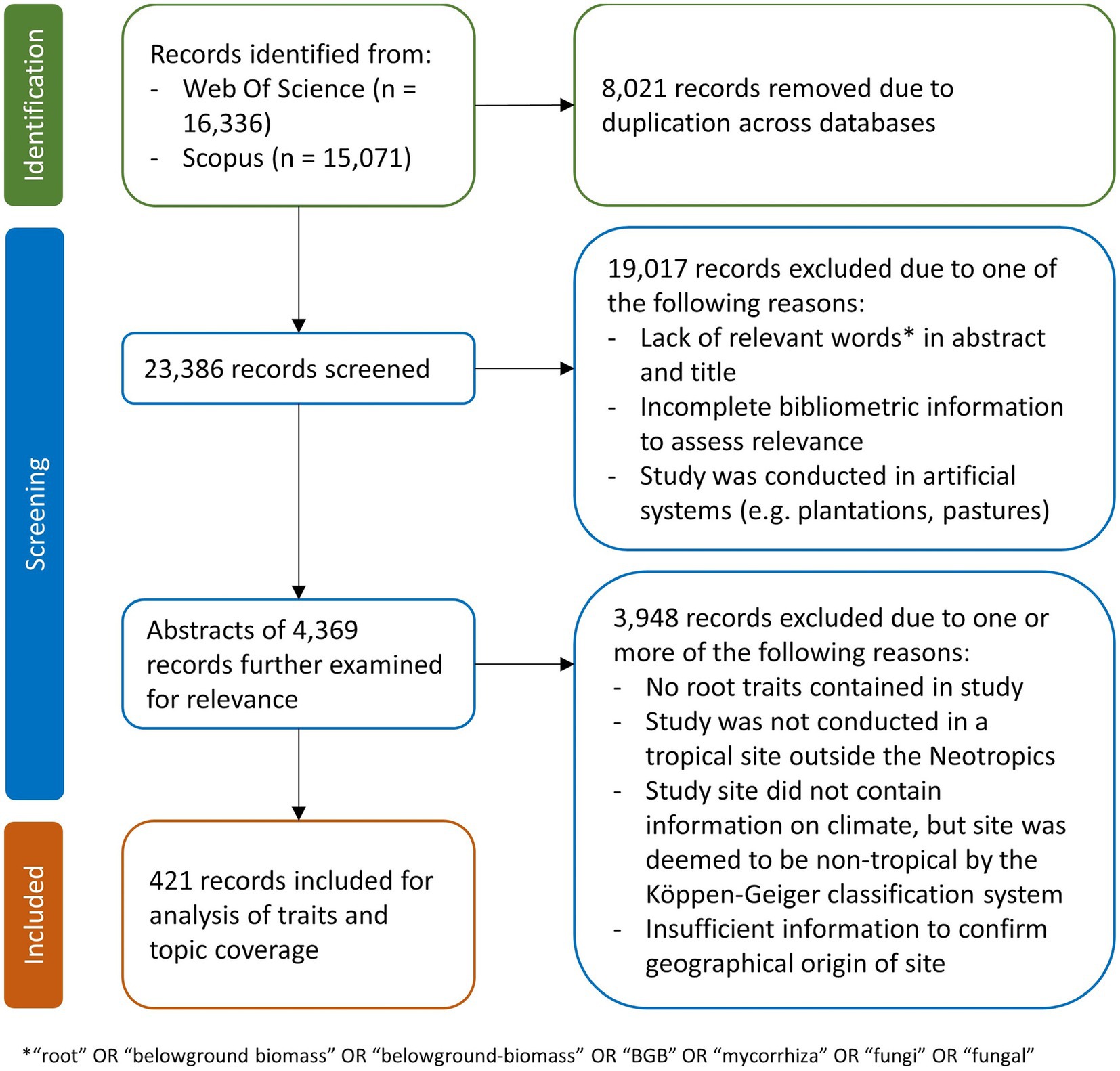
Figure 1. Modified PRISMA framework (Page et al., 2021) used to consolidate relevant root trait publications for this study.
2.2. Bibliometric and site information of publications
For each of the publications remaining, we manually examined each publication to extract its abstract, keywords, site location and coordinates, geographical location, climate, site environment, traits studied and other ancillary information (Supplementary Table S1). For studies that did not report the climatic conditions of their sites, we used the R package “kgc” to verify if the site’s geographical location was based in a tropical climate (Bryant et al., 2017). For this study, we defined the paleotropics as all regions with a tropical climate (Köppen Geiger climate: Af, Am, As and Aw) from longitude 30°W to 170°E. Several publications lacked abstracts due to the formatting of their respective journals. For such publications, we constructed a placeholder abstract based on relevant paragraphs copied from the main text.
2.3. Root trait information of publications
We examined the methodologies of each publication to identify the traits studied. For traits that require one or more additional traits to compute (e.g., calculating specific root length requires root biomass and root length), we only listed the traits relevant to the study’s main findings. Furthermore, we also examined if the authors classified fine or coarse roots in their study and the corresponding classification method (using root orders/root diameters etc.). These traits were then classified into nine categories of root traits (Table 1) for further analysis of trait coverage across publications. Subsequently, we constructed an upset diagram to identify differences in coverage of subsets of trait categories across publications using the “upset” function in the R package “UpSetR” (Gehlenborg, 2019).
2.4. Topic modeling of abstracts
We utilized a topic modeling approach to identify topics covered across the abstracts of publications. Firstly, we processed the corpus of the raw text of the abstracts by transforming all words to lower-case, removing numbers, punctuations and stopwords and stemming the remaining terms to generate a document-term matrix. In addition, we also removed conjunctions, words that possessed no connections to any topics on their own (e.g., “increase,” “positive,” “however”) and only included tokens that appeared at least 10 times across abstracts. Subsequently, the Latent-Dirichlet Allocation (LDA) model using Gibbs sampling method (Blei, 2012) over 1,000 iterations was used to construct topic models using the “LDA” function in the “topicmodels” R package (Grün and Hornik, 2022).
In order to construct topic models using LDA, two key parameters had to be decided prior – the number of topics (k) and the alpha hyperparameter (α). To identify the optimal k that produces the model that best fits our data, we constructed topic models for 2 ≤ k ≤ 50 and diagnosed each model using AIC values, coherence scores and topic exclusivity using the “AIC” and “topic_diagnostics” functions from the R packages “stats” and “topicdoc” respectively (Friedman, 2022). The hyperparameter α controls the distribution of topics within each document, with higher values of α producing greater evenness in topic distribution. We assumed each publication should contain up to three topics and set α = 0.05, producing strong “peaky” topic distributions within each publication. The best topic model was selected by evaluating AIC scores and topic coherence and exclusivity metrics using the “topic_coherence” and “topic_exclusivity” functions from the “topicdoc” package (Friedman, 2022). For our abstracts, mean topic coherence and exclusivity did not produce any discernible trends or converge to a maximum value as k increased. Thus, we conducted 500 iterations of topic models and selected the value of k = 23 that gave the lowest AIC score most frequently (Supplementary Figure S1).
We classified each publication under its top three topics, each representing at least 15% of the abstract. Each topic was given descriptive names to match its respective contents based on the top 15 most probable words within each topic set. Through the topics and the trait information captured within each publication, we constructed a network chord diagram to depict the links between topics covered and traits studied across publications using the R package “circlize” (Gu et al., 2014).
2.5. Geographical distribution of in situ studies
Based on the methodologies of each study, we classified each publication as an in situ, ex situ or meta-analyzes study (see Supplementary Table S1 for precise definitions of each type of study). As a reference, we defined “in situ” as studies conducted under field conditions regardless of manipulation of experimental variables, “ex situ” studies as studies conducted outside field conditions (e.g., shadehouses and potted experiments) and “meta-analyzes” studies as any study that analyzed data from multiple past studies. We plotted in situ study sites on a world map and quantified the number of in situ studies conducted within each country in the Paleotropics. Based on the geographical distribution of the study sites, we classified each publication into one out of five geographical hotspots. Subsequently, we visualized trait and topic coverage within each geographical hotspot using balloon plots from the R package “ggpubr” (Kassambara, 2022).
2.6. Temporal evolution of trait coverage and topics
Using the year of publication of each study, we mapped out the temporal trends of the traits and topics covered within the literature compiled. Publications were split into four periods from before 2000, 2001–2009, 2010–2016 and 2017–2023. These periods were chosen to capture major shifts in the scientific literature corresponding to the formalization of the field of functional ecology in the early 2000s (Diaz et al., 1998; Díaz and Cabido, 2001), the rise in prominence of root trait research in the late 2000s (Lambers et al., 2006; Guo et al., 2008) and recent research trends from 2016 onwards (McCormack et al., 2015; Valverde-Barrantes et al., 2017; Bergmann et al., 2020). We reported the most prominent traits and topics within each period and analyzed how research interests have shifted over time.
2.7. Ancillary information of root traits data
We assessed whether root traits were presented across the publications in a standardized format to facilitate cross-study comparisons. Specifically, we examined whether publications collected root trait data at the species or community level. Subsequently, we checked whether fine or coarse roots were studied and the criteria used to distinguish between the two groups.
For in situ and ex situ studies involving the manipulation of abiotic and biotic factors (e.g., experimental plots and growth-chamber experiments), we identified the environmental factors manipulated in each study (e.g., light, temperature, nutrients). Finally, for in situ studies and meta-analyzes studies, we classified publications according to the biome of their root trait data (e.g., forests, savannas). The breakdown of this ancillary information across publications was visualized in a composite infographic to identify trends in how publications presented root trait data and the environmental variables studied alongside said data.
3. Results
3.1. Trait coverage across publications
Our literature search yielded 421 publications capturing a wide range of traits and topics. We recorded 67 unique traits examined across publications, split into 9 distinct categories. However, trait representation was highly skewed toward biomass-related traits, with two out of three studies measuring traits related to root biomass (Supplementary Figure S2). About one in four studies examined subsets of traits related to mycorrhizal colonization, root nutrient content (chemistry) and root dynamics. On the other hand, root physiology (47 studies, 11%), morphology (53 studies, 12%) and anatomical traits (8 studies, 2%) remain heavily underrepresented across publications. Although about half of all publications (194 studies, 46%) covered more than one trait category, very few publications (41 studies, 10%) examined a combination of traits beyond root biomass (Supplementary Figure S2).
3.2. Topics associated with root traits
Our LDA topic model uncovered 23 distinct topics across all publications (see Supplementary Table S2 for topic interpretation) ranging from resource availability, ecosystem productivity, plant functional traits and ecosystem dynamics from multiple biomes (Figure 2). The most studied individual topics were light limitation (69 studies, 16%), ectomycorrhizae (63 studies, 15%) and seedling experiments (63 studies, 15%) while the least studied topics were savanna/woodland dynamics (22 studies, 5%), forest gap dynamics (24 studies, 6%), litter decomposition (29 studies, 7%) and water limitation (30 studies, 7%). Publications covered these 23 topics evenly, each captured by 5–20% of all publications. When these topics were classified according to eight distinct categories (Figure 2; Supplementary Table S2), topics associated with plant functional traits and ecosystem dynamics were the most studied (145 and 143 studies respectively), while topics related to biomass and productivity and edaphic processes were the least frequently studied (90 and 102 studies respectively), barring the topic of seedling experiments (63 studies).
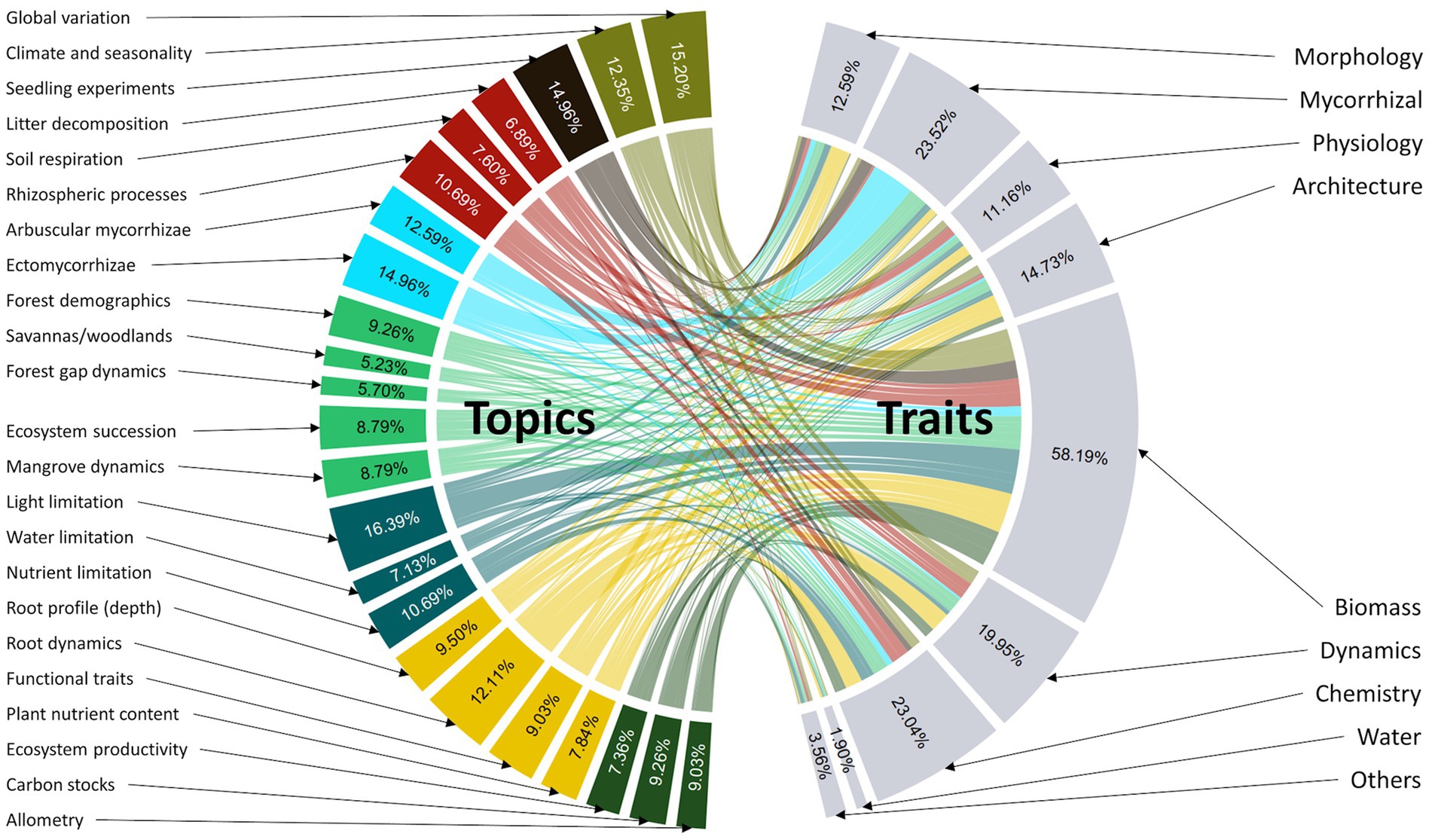
Figure 2. Network chord diagram depicting the topics across publications and the traits studied in conjunction with each topic. Percentages in each sector show the percentage of studies that contain each topic or trait. Percentages do not add up to 100% because each study can contain multiple topics or traits. Colors of subsets of topics depict common topic categories across publications. Dark green topics reflect a category associated with biomass and productivity; orange topics reflect a category of plant functional traits; cyan topics reflect a category of resource availability; green topics reflect a category of ecosystem-specific dynamics; light-blue topics reflect a category of mycorrhizal-related studies; maroon topics reflect a category of soil flux and edaphic processes; black topics reflect a category of seedling experiments and khaki topics reflect a category of global-scale studies or spatial and temporal variation of root traits.
When analyzed in conjunction with trait coverage, many of these topics were studied alongside root biomass. For example, although there is no direct relationship between light availability and root traits, many studies often include root biomass to quantify the biomass allocation patterns and growth of plants under varying light availability (e.g., Brearley et al., 2007; Saner et al., 2011). As expected, studies with topics on plant biomass and productivity largely contained traits from the root biomass category. Similarly, studies with topics on mycorrhizae also primarily included mycorrhizal-related traits. However, studies concentrating on functional traits and rooting depth (orange sectors in Figure 2) were still mainly restricted to quantifying biomass allocation instead of root morphology, physiology and architecture.
3.3. Geographical distribution of traits and topics from in situ studies
Two-thirds of the studies captured by our literature search contained observational data on root traits (i.e., studies that collected root trait information of plant species and communities under field conditions). However, these studies were unevenly distributed across the Paleotropics. Among the 282 in situ studies captured by our literature search, 53 studies were based in Africa, 56 from India and Nepal (henceforth “India”), 42 from China, 100 from Southeast Asia (SE Asia) and 29 from Australia and Oceania (henceforth “Oceania”; Figure 3). These 282 studies were conducted across 444 research sites across the Paleotropics, covering several biomes ranging from forests to grasslands and mangroves.
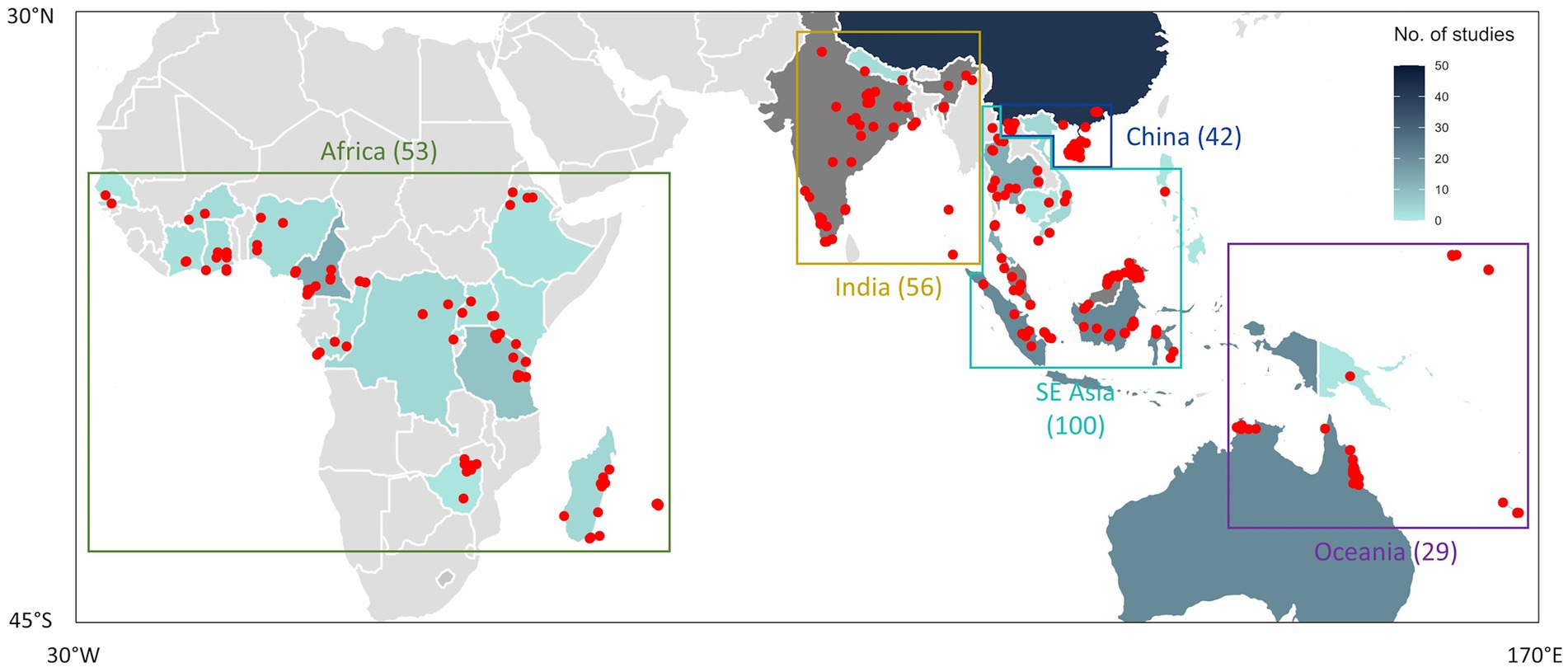
Figure 3. Geographical distribution of 282 in situ studies conducted across the Paleotropics, spanning a total of 444 research sites (denoted as red points). Each study may contain one or more research sites. The shaded boundaries represent the number of publications produced by each country. These 282 in situ studies and their corresponding research sites were subdivided into 5 biogeographic regions, denoted by the enclosed regions and labeled accordingly. Brackets indicate the number of studies within each biogeographic region. Each region is uniquely color-coded to facilitate visualization. See Supplementary Figures S3, S4 for the trait and topic coverage within each biogeographic region, respectively.
Examining the number of studies in each trait category within each geographical region revealed variations in research priorities of different research groups distributed across the tropics (Supplementary Figure S3). Within all five regions, root biomass was the most commonly studied trait among studies. However, most of the root architectural and mycorrhizal traits data was produced from Africa and SE Asia, whereas traits pertaining to root chemistry and dynamics were mainly from India and SE Asia. In contrast, anatomical traits were sparsely represented by three studies in China and SE Asia each, suggesting a minimal understanding of root anatomy and hydraulic-related traits throughout Paleotropical plant communities. Although India and Africa produced a similar number of studies (56 and 53 studies respectively), studies from India focused more heavily on root chemistry (19 in India; 12 in Africa) and dynamics (17 in India; 13 in Africa) while studies from Africa focused on root mycorrhizae (17 in Africa; 10 in India) and architecture (10 in Africa; 4 in India). Studies from China focused slightly more on root chemistry and morphology (17 and 12 studies respectively) compared to other trait categories. In SE Asia and Oceania, studies tended to focus on root dynamics (22 in SE Asia; 9 in Oceania), mycorrhizal-related traits (21 in SE Asia; 7 in Oceania) and root architecture instead (19 in SE Asia; 8 in Oceania).
Topic coverage varied significantly across all five biogeographic regions (Supplementary Figure S4). In Africa, climate and seasonality (12 studies) and ectomycorrhizae (14 studies) were the most common topics studied. In India, rhizospheric processes (16 studies), climate and seasonality (15 studies) and root dynamics (13 studies) were the most frequently studied topics. In China, studies mostly addressed topics such as plant functional traits (13 studies), nutrient limitation (12 studies) and ecosystem succession (12 studies). Studies from SE Asia focused on topics such as ectomycorrhizae (19 studies), carbon stocks (15 studies), root profiles (14 studies) and functional traits (14 studies). Lastly, studies from Australia and the Pacific regions (Oceania) focused on topics revolving around climate and seasonality (7 studies), mangroves (6 studies), ectomycorrhizae (6 studies) and root dynamics (6 studies).
3.4. Temporal trends in trait and topic coverage
The number of studies containing root traits remained stable before 2016 but has significantly increased from 2017 to 2023 (Figure 4A). Correspondingly, the number of studies examining each trait category has either remained stable or gradually increased over time, except for mycorrhizal-related studies which experienced a temporary dip from 2010 to 2016. Traits related to root biomass and chemistry were consistently well-represented across the four periods. Traits related to mycorrhizal colonization and root architecture were also commonly examined in studies before 2009. However, since then, studies examining root dynamics have become more prominent in the recent literature.
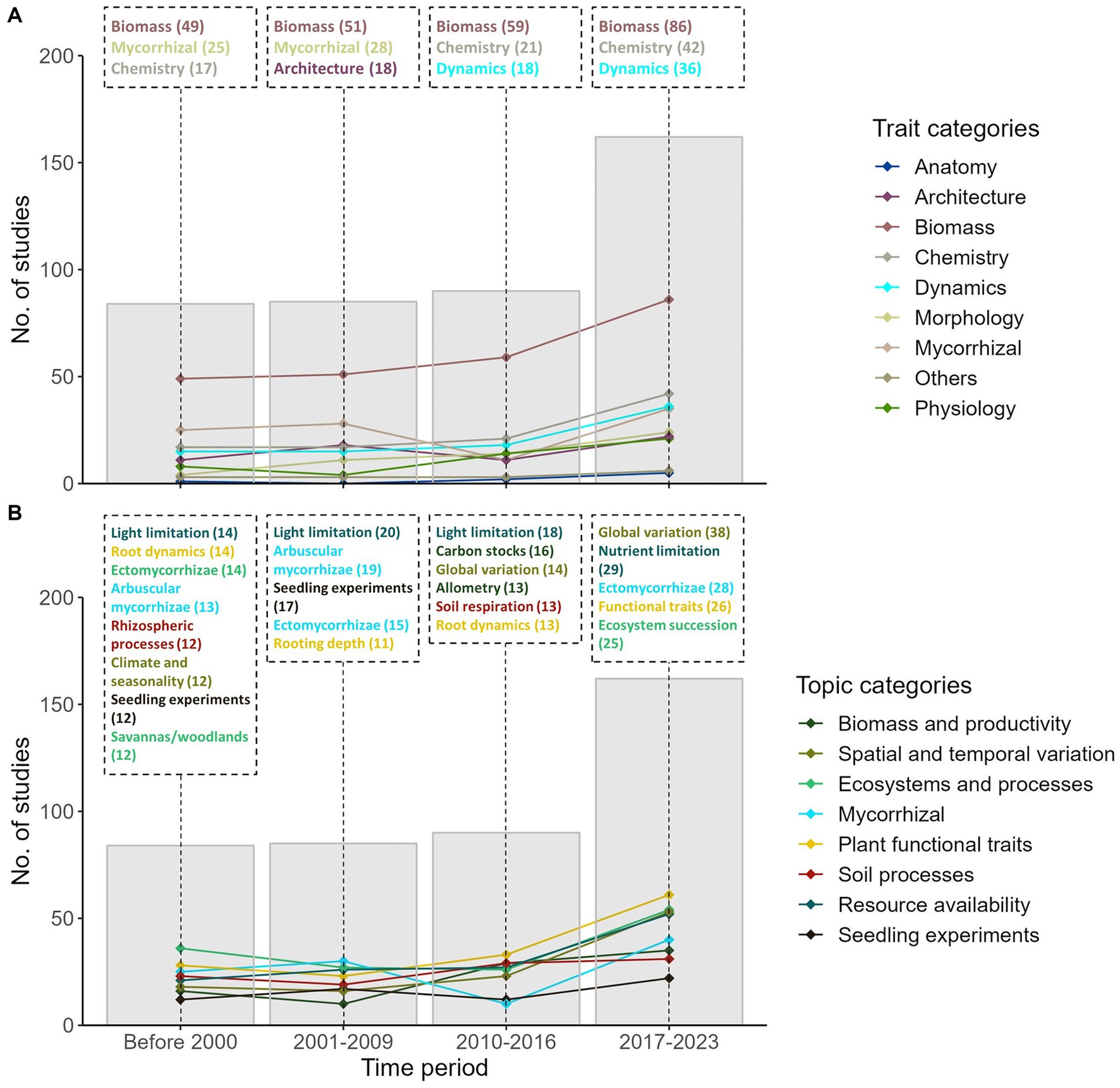
Figure 4. Temporal trends of trait (A) and topic (B) representation over time. In 4A, trait categories listed in the boxes represent the top three categories with the greatest number of studies within each period. In 4B, the topics listed in the boxes represent the top five topics with the greatest number of studies within each period. The number of studies within each trait category or topic is shown in brackets. Colors denote the various trait and topic categories represented across all 421 publications.
Similarly, the number of studies within most topic categories has increased in parallel with the prominence of root trait research over time (Figure 4B). This trend is accompanied by a notable dip in the number of studies examining topics related to mycorrhizae from 2010–2016. Root dynamics and light availability were popular topics examined across the literature across most of the periods. Before 2010, topics related to light limitation, mycorrhizal colonization and root dynamics dominated the field of root trait research in the Paleotropics. However, more recent publications have shifted their focus onto other topics such as global variations in root traits, nutrient limitation and ecosystem succession from 2017 onwards. Similarly, there has been a paralleled increase in the number of studies examining the functional significance of root traits (in 2017–2023) and the inclusion of root traits in allometric models to account for carbon stocks in various ecosystems (in 2010–2016).
3.5. Ancillary information in root trait studies
A large majority of our publications were in situ studies (282 studies), followed by ex situ studies (91 studies) and meta-analyzes (54 studies). Six publications produced both in situ and ex situ studies (Supplementary Figure S5). Most publications across in situ studies (161 studies, 57% of in situ studies) and meta-analyzes (48 studies, 89% of meta-analyzes) produced community-level root traits instead of collecting trait information at the species level (125 studies and 8 studies respectively). Such a trend likely reflects the preferred method of root collection through soil cores instead of tracing roots to specific species.
Among the in situ studies that examined species-level root traits, mycorrhizal colonization was the most frequently reported trait by a large margin (56 out of 125 studies = 45%), followed by root morphology and root chemical traits (23 and 24 studies respectively). Among the ex situ studies, a similar trend was observed, with 28 out of 91 studies reporting mycorrhizal data (31%), 16 studies reporting root morphological trait data (18%) and 15 studies reporting on root chemical traits (16%). The few studies that reported a large number (>50) of species-level trait data typically involved regional-scale examinations of root morphology, chemistry and mycorrhizal associations in parts of China and India (e.g., Muthukumar et al., 2003; Hogan et al., 2020). However, no equivalent studies exist for other traits, nor in other biodiverse areas such as Southeast Asia and Australia.
In situ trait information was highly skewed with respect to ecosystems, as most traits from the field were collected from forests (206 studies, 73%). The rest of the studies were sparsely distributed across disturbed landscapes (29 studies, 10%), savannas (22 studies, 8%), mangroves (17 studies, 6%) and other ecosystems (Supplementary Figure S5). Similarly, over half of all root trait data from meta-analysis publications were also collected from forests (32 studies). As ex situ studies involved the collection of root traits under experimental settings rather than field conditions, we collated the experimental variables manipulated across these publications to understand the environmental context of ex situ root trait data. We found that most ex situ studies examined how root traits covaried with respect to varying levels of nutrients (25 studies), light (23 studies) and mycorrhizal inoculation treatments (20 studies). However, we also found a notable number of multi-factorial studies (32 studies), as well as studies including a diversity of other environmental variables within their experimental design such as competition, water availability and aboveground disturbances.
The breakdown of publications’ ancillary information also revealed that most publications did not sufficiently distinguish between the fine roots and coarse root fractions (Supplementary Figure S6). Among the 282 in situ publications, 135 studies (48%) presented sufficient information on their criteria for distinguishing between fine and coarse roots, with <2 mm and < 5 mm root diameter being the most frequently cited criteria. However, only 10 studies (4%) utilized an order-based classification to distinguish between absorptive or transport fine roots. Similarly, among the 54 meta-analyzes, 20 studies (37%) presented sufficient information on their criteria for distinguishing between fine and coarse roots, with <2 mm root diameter being the most frequently cited criteria. However, only 2 studies (4%) utilized a functional or order-based classification to distinguish between absorptive or transport fine roots. Finally, only 9 out of 78 studies (12%) distinguished between fine and coarse roots among ex situ publications, suggesting a lack of focus on fine root fractions among experimental studies.
4. Summary and recommendations
Recent advancements in methods in root trait measurements and global databases have greatly expanded root trait coverage across the tropics. However, our literature survey and topic models revealed limited coverage of several root traits and associated topics across the Paleotropics. In particular, we observed a heavy bias toward biomass-related root traits and a lack of information on traits on root hydraulics (anatomy), root architecture and root physiology (Figure 2; Supplementary Figure S2). Such a bias is consistent with the low number of observations of these traits found in global root trait databases such as FRED (Iversen and McCormack, 2021) and GRooT (Guerrero-Ramírez et al., 2021), suggesting that our survey is representative of the trait coverage found in the general literature.
Further segregation of publications’ traits and topics by periods and geographical locations revealed shifts in research interests across the tropics over time and highlighted gaps in the current trait coverage with respect to ecosystems and geographical hotspots (Supplementary Figures S3, S4). In addition, the breakdown of publications revealed a lack of trait data at the species level (Supplementary Figure S5) and inconsistencies in how publications distinguish fine roots from coarse roots (Supplementary Figure S6). Based on these points, we propose the following recommendations for future researchers to focus on resolving critical knowledge gaps regarding the role of root traits in ecosystem functioning, with a strong emphasis on understanding root trait variation with respect to environmental stressors and global change.
4.1. Topics and traits relevant to global environmental changes remain understudied
Beyond the overemphasis on root biomass as a functional trait, global change is expected to induce a diversity of physiological responses in plants aboveground and belowground. For example, several potentially strong drivers such as climate change and atmospheric nitrogen deposition may enhance the occurrence of droughts and fires and enhance phosphorus limitation in tropical systems, respectively (Zuidema et al., 2013). However, many belowground responses to these drivers remain understudied in the tropics. For example, water limitation and fires regulate dynamics in many tropical savannas (Beckage et al., 2019). Yet, there exists a lack of coverage of root traits such as rooting depth and non-structural carbohydrates – both of which contribute to acquiring water in deep soil layers and post-fire regrowth in savanna species, respectively (e.g., Boonman et al., 2020; Issifu et al., 2021). Similarly, despite increasing aridity in many parts of the tropics, coverage of traits and topics related to water uptake remains very low with only 8 studies containing root anatomical traits (associated with plant hydraulics) and 30 studies examining the topic of water limitation (Figure 2). To overcome such knowledge gaps, we recommend a targeted approach to expand root trait coverage across the Paleotropics, based on the projected regional changes each geographical region will experience in the near future (Figure 5).
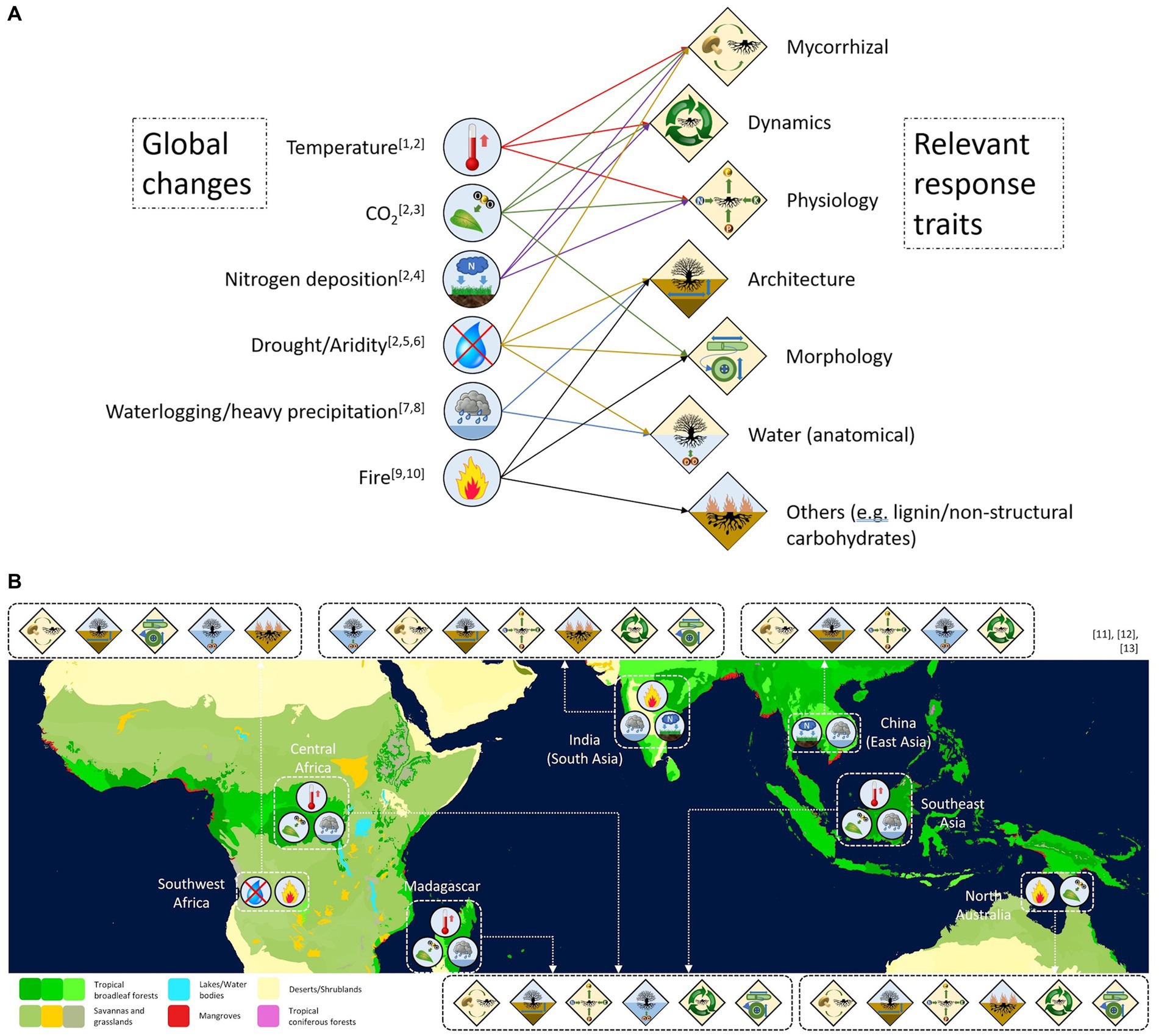
Figure 5. Conceptual network of six global changes impacting current ecosystems worldwide and corresponding underrepresented root trait categories involved in the adaptation against said changes (A), based on the following studies: [1]Wang et al. (2021), [2]Mohan et al. (2014), [3]Nie et al. (2013), [4]Li et al. (2015), [5,6]Zhou et al. (2018, 2020), [7]Yamauchi et al. (2018), [8]Pedersen et al. (2021), [9]Boonman et al. (2020), and [10]Le Stradic et al. (2021). Trait-driver linkages were juxtaposed onto 7 geographical regions across the Paleotropics (B) to identify root trait categories that should be focused on in different geographical regions (in surrounding boxes) that are critical for understanding plant adaptations against global changes. Basemap of Figure 5B was obtained from the [11]World Wildlife Fund Global 200 Ecoregions (Olson and Dinerstein, 2002). Regional global changes were derived from the [12]IPCC WGI Interactive Atlas: Regional Synthesis (all drivers except nitrogen deposition, Gutiérrez et al., 2021) and [13]Phoenix et al. (2006) (for nitrogen deposition hotspots).
Projected changes in temperature, CO2 levels, nutrient deposition and precipitation patterns across the tropics are likely to result in substantial changes in plant physiology beyond biomass allocation. While enhancing root biomass allocation is one way that plants can adapt to belowground resource limitations and aboveground disturbances (e.g., Shipley and Meziane, 2002; Boonman et al., 2020; Weemstra et al., 2020), it remains unclear how other root traits may aid in adapting plant species to these changes in environmental factors. For example, recent studies outside our literature search have suggested how mycorrhizal associations can aid in improving plants’ resilience in several climate change scenarios, including reducing plant stress under drought conditions (Mohan et al., 2014). However, there remains no consensus on whether rising CO2 levels are increasing the amount of photosynthate allocated to mycorrhizae to aid in belowground resource acquisition or induce plant species to expand root systems to enhance direct resource uptake instead (Mohan et al., 2014). In addition, global nitrogen and phosphorus deposition may increase nutrient availability, which could induce various responses across species and ecosystems, such as changes to root morphology, root respiration and plant productivity (Li et al., 2015; Lugli et al., 2021; Cunha et al., 2022). However, it remains unknown how these responses may influence plants’ investments into other belowground acquisition strategies revolving around root physiological traits and plant hydraulic traits. Hence, future studies should examine a broad range of traits to quantify the diversity in responses and strategies of terrestrial vegetation to global changes. While we note the increase in studies that examined topics related to global change (Figure 4B) in recent years, this trend needs to be accompanied by an additional focus on previously understudied traits to understand terrestrial vegetation responses to global change comprehensively.
4.2. Emphasis on “hard” root traits to better represent plant physiological functions and responses
Root biomass allocation is a proxy for plant belowground investment into roots, mycorrhizal symbionts and exudates needed to acquire water and nutrients. Nonetheless, focusing on root biomass allocation alone is not informative of other aspects of resource acquisition strategies among tropical species as species may undergo plastic adjustments in root morphology and physiology given the same unit of biomass allocated (Weemstra et al., 2020). Furthermore, a recent study on global root trait variation has identified a fungal collaboration gradient that dominates the root economic space, agreeing with other studies that showed “outsourcing” resource uptake to mycorrhizae (Bergmann et al., 2020) remains a central strategy in the belowground acquisition strategies of up to 80% of terrestrial plant species worldwide (Wang and Qiu, 2006). As a result, the large majority of root biomass observations observed in our literature survey do not inform us of the extent plants pursue other key strategies – mycorrhizal symbiosis and exudate production – to acquire belowground resources, as well as the yields and costs involved with each of these strategies (Braghiere et al., 2022).
In addition to root biomass, other root traits may simultaneously contribute to multiple physiological functions and ecosystem effects, constraining our ability to infer how observed changes in root traits influence precise belowground strategies and associated ecosystem effects among species (Freschet et al., 2021). For example, are increases in root diameter among species a strategy to enhance root lifespan as part of a resource-conservative strategy (McCormack et al., 2012), to increase root cortex space for mycorrhizal colonization (Bergmann et al., 2020) or both? Similarly, do increases in root nitrogen content translate to an increase in direct nutrient uptake through the root system (i.e., a “do-it-yourself” strategy, see Han et al., 2022), enhanced exudate production (Sun et al., 2021), or simply a reflection of an increase in soil nitrogen availability (Li et al., 2015)? Many of such uncertainties may be resolved by examining “hard” traits that relate to specific physiological functions instead. For example, root longevity can be measured directly using in situ methods instead of relying on root diameter or tissue density to predict root lifespan (see McCormack et al., 2012; Weemstra et al., 2016). Similarly, the extent to which plants “outsource” resource acquisition to mycorrhizal can be evaluated by measuring mycorrhizal colonization intensity directly rather than relying on root diameter to approximate root cortex fraction to estimate site availability for arbuscular mycorrhizae. Thus, we advocate that future researchers consider examining lesser-studied, “hard” morphological, architectural and physiological traits whenever possible to better capture variations in species’ specific belowground strategies, especially concerning environmental variation and global change factors.
4.3. Standardizing trait measuring protocols to facilitate cross-study comparisons of root traits
For effective inter-study comparisons of root trait responses to environmental factors, traits should be reported consistently such that ambiguity within the sampling and measuring processes of root systems is minimized. In particular, our survey revealed that most studies from the Paleotropics are still reliant on the traditional classification of “fine” roots as ≤2 mm in diameter. However, such a classification system is likely insufficient to capture precise physiological functions regarding root exudation, mycorrhizal colonization and morphological adjustment within root systems (McCormack et al., 2015). Generally, absorptive fine roots are highly metabolically active and are the primary sites for resource acquisition and mycorrhizal colonization (Guo et al., 2008; McCormack et al., 2015). In contrast, transport fine roots contain a greater proportion of biomass among the fine root system (Valenzuela-Estrada et al., 2008; Mucha et al., 2020) and are primarily responsible for the reallocation of captured resources to other parts of the plant with a lower turnover rate than absorptive fine roots (Valenzuela-Estrada et al., 2008; Xia et al., 2010). These physiological differences suggest that both classes of fine roots govern fundamentally distinct ecological processes regarding nutrient dynamics and carbon stocks and should be distinguished from one another when examining the relationship between fine roots and ecosystem functioning (McCormack et al., 2015).
Given the focus on belowground biomass allocation and plant growth among publications, distinguishing between fine and coarse roots is likely not a priority for many studies focusing on woody species. Our survey supports such a proposition, as most studies did not produce any criteria to distinguish between fine and coarse roots (Supplementary Figure S6). Yet, plants can exhibit great plasticity in their biomass allocation into fine and coarse roots within their root systems (Freschet et al., 2015; Blume-Werry et al., 2018). Such studies suggest that a theoretical increase in root biomass among plant species may not always translate into a proportionate increase in root absorptive capacity or soil exploration capacity unless more precise traits such as absorptive root surface area or resource uptake capacity are examined in tandem. Hence, we propose that by distinguishing between coarse roots, absorptive fine roots and transport fine roots, future studies will be able to draw insights regarding plant investments toward resource acquisition (Guo et al., 2008; Kong et al., 2016), soil exploration (Eissenstat et al., 2015), carbon storage and anchorage (Hertel et al., 2009; Waring and Powers, 2017) at a greater resolution than previously documented. At the same time, changes in how the field measures and reports root biomass allocations by order and root classes can also better reflect more precise belowground plant strategies and ecosystem functions.
4.4. Examination of a wide array of root traits across ecosystem types and environmental factors using a mix of in situ and ex situ experimental designs
As root traits can vary significantly across multiple ecosystem types and environmental factors, a desirable goal in the field of belowground ecology is to generalize how root traits vary with respect to simultaneous changes in climate, edaphic factors, ecosystem type and local disturbances (Lavorel et al., 2007; Funk et al., 2017). We propose that both in situ and ex situ experimental designs have a unique role in contributing to knowledge gaps in global root trait variation and trait-function relationships. For example, root trait observations in the field provide large-scale insights into how root traits covary across multiple environmental factors simultaneously to form a holistic belowground resource acquisition strategy in plants (Weemstra et al., 2016; Bergmann et al., 2020; Weigelt et al., 2021). However, ex situ studies allow for more precise manipulation of temperature, light and edaphic conditions which can be useful to test how subsets of traits enhance the adaptive capacity of plants to changes in resource availability in natural settings (Freschet et al., 2021). Due to the prevalence of “soft” traits documented in the literature (Freschet et al., 2021; this study), we suggest that there is currently a great need for more ex situ experiments to verify the relationship between soft traits and more precise physiological functions among plant species. However, it is also possible to adopt both in situ and ex situ elements in future experimental designs to reconcile the need for “hard” traits and overcome methodological limitations in the field (e.g., Steidinger et al., 2015; Liu et al., 2017). An alternative but equally promising avenue could be found in more large-scale CO2 and warming manipulation experiments such as FACE (Norby et al., 2016) and TRACE (Kimball et al., 2018) to gather insights into shifts in community-level root traits as environmental conditions vary across ecosystems and over time.
Lastly, a majority of root trait observations from the field are documented at the community level; yet traits from ex situ studies are, by default, conducted at the species level (Supplementary Figure S5). This presents several methodological challenges when comparing species-level root traits from ex situ studies and community-weighed mean trait values from in situ studies. Firstly, species-level root traits from ex situ studies may not be realized in field settings due to the inability to perfectly replicate biotic factors (Valverde-Barrantes et al., 2013), edaphic conditions and rhizosphere communities that may be associated with root morphological and physiological traits (Chen et al., 2016; Spitzer et al., 2021; Sweeney et al., 2021). Secondly, changes in community-level root traits may be due to species turnover across environmental gradients (e.g., Holdaway et al., 2011; Prieto et al., 2015) rather than intraspecific plasticity of root traits (Siefert et al., 2015; Weemstra et al., 2021). Reconciling the multiple levels of root trait variation will require a better understanding of the mechanisms that drive root trait plasticity, which is likely to be best examined under controlled settings (i.e., ex situ studies). As such, we recommend that future ex situ studies be designed to test specific mechanisms of plant physiology that drive trait variation among species, including the effects of abiotic factors, competition and rhizosphere communities (mycorrhizae and soil microbial communities; Valverde-Barrantes et al., 2013, 2017). On the other hand, community-level root traits observed under field conditions should always be accompanied by soil edaphic variables and the species composition of communities sampled. Subsequently, mechanistic findings from ex situ studies can then be used to support and predict trends underlying root traits observed from known environmental gradients across natural communities. While we recognize that obtaining in-situ root trait data from all species in hyperdiverse tropical ecosystems is likely unrealistic, the combination of mechanistic findings from ex situ studies (e.g., Fisher et al., 2010), novel trait imputation methods (e.g., Vleminckx et al., 2021) and a better understanding of trends in root trait plasticity (e.g., Kramer-Walter and Laughlin, 2017) may improve approximations in species and community-level root traits across ecosystems.
5. Conclusion
Roots participate in many plant physiological and ecosystem processes simultaneously. However, our survey has revealed that root trait coverage has been relatively poor across the Paleotropics, with a strong bias toward biomass-related traits. While measuring biomass-related traits tends to be convenient, these measurements fail to provide insights into precise belowground physiological functions including, but not limited to resource acquisition, carbon storage and biomass allocation patterns. Thus, such a knowledge gap constrains our ability to generalize belowground responses to multiple environmental gradients.
Ideally, root trait collection should be maximized from all ecosystems and species across the tropics to quantify belowground responses to global change and environmental gradients. However, as this is unlikely to be a realistic endeavor, we propose that future studies focus on diverse traits beyond root biomass that contribute to plant adaptation to multiple global changes at a regional scale (Figure 5). In addition, we also recommend the standardization of root trait measurements and report site-specific environmental variables in future studies. This is in order to facilitate the compilation and sharing of novel trait data to better quantify the effects of global changes on belowground ecosystem processes in the years to come.
Data availability statement
The original contributions presented in the study are included in the article/Supplementary material, further inquiries can be directed to the corresponding author.
Author contributions
MYL wrote the first draft and conducted the literature search and data analysis. KMA suggested the initial idea which was later further refined into the current manuscript. All authors contributed to the article and approved the submitted version.
Conflict of interest
The authors declare that the research was conducted in the absence of any commercial or financial relationships that could be construed as a potential conflict of interest.
Publisher’s note
All claims expressed in this article are solely those of the authors and do not necessarily represent those of their affiliated organizations, or those of the publisher, the editors and the reviewers. Any product that may be evaluated in this article, or claim that may be made by its manufacturer, is not guaranteed or endorsed by the publisher.
Supplementary material
The Supplementary material for this article can be found online at: https://www.frontiersin.org/articles/10.3389/ffgc.2023.1206225/full#supplementary-material
References
Archibald, S., Hempson, G. P., and Lehmann, C. (2019). A unified framework for plant life-history strategies shaped by fire and herbivory. New Phytol. 224, 1490–1503. doi: 10.1111/nph.15986
Beckage, B., Bucini, G., Gross, L. J., Platt, W. J., Higgins, S. I., Fowler, N. L., et al. (2019). “Water limitation, fire, and savanna persistence” in Savanna Woody plants and large herbivores. eds. P. F. Scogings and M. Sankaran (New York: John Wiley and Sons), 643–659.
Bergmann, J., Weigelt, A., van der Plas, F., Laughlin, D. C., Kuyper, T. W., Guerrero-Ramirez, N., et al. (2020). The fungal collaboration gradient dominates the root economics space in plants. Sci. Adv. 6:eaba3756. doi: 10.1126/sciadv.aba3756
Blume-Werry, G., Lindén, E., Andresen, L., Classen, A. T., Sanders, N. J., Von Oppen, J., et al. (2018). Proportion of fine roots, but not plant biomass allocation below ground, increases with elevation in arctic tundra. J. Veg. Sci. 29, 226–235. doi: 10.1111/jvs.12605
Boonman, C. C. F., van Langevelde, F., Oliveras, I., Couédon, J., Luijken, N., Martini, D., et al. (2020). On the importance of root traits in seedlings of tropical tree species. New Phytol. 227, 156–167. doi: 10.1111/nph.16370
Braghiere, R. K., Fisher, J. B., Allen, K., Brzostek, E., Shi, M., Yang, X., et al. (2022). Modeling global carbon costs of plant nitrogen and phosphorus acquisition. J. Adv. Model. Earth Syst. 14:e2022MS003204. doi: 10.1029/2022MS003204
Brearley, F. Q., Scholes, J. D., Press, M. C., and Palfner, G. (2007). How does light and phosphorus fertilisation affect the growth and ectomycorrhizal community of two contrasting dipterocarp species? Plant Ecol. 192, 237–249. doi: 10.1007/s11258-007-9325-6
Bryant, C., Wheeler, N., Rubel, F., and French, R. (2017). kgc: Koeppen-Geiger climatic zones. R package version 1.0.0.2. Available at: https://CRAN.R-project.org/package=kgc.
Chen, W., Koide, R. T., Adams, T. S., DeForest, J. L., Cheng, L., and Eissenstat, D. M. (2016). Root morphology and mycorrhizal symbioses together shape nutrient foraging strategies of temperate trees. Proc. Natl. Acad. Sci. U. S. A. 113, 8741–8746. doi: 10.1073/pnas.1601006113
Corlett, R. T. (2016). The impacts of droughts in tropical forests. Trends Plant Sci. 21, 584–593. doi: 10.1016/j.tplants.2016.02.003
Cunha, H. F. V., Andersen, K. M., Lugli, L. F., Santana, F. D., Aleixo, I. F., Moraes, A. M., et al. (2022). Direct evidence for phosphorus limitation on Amazon forest productivity. Nature 608, 558–562. doi: 10.1038/s41586-022-05085-2
Díaz, S., and Cabido, M. (2001). Vive la différence: plant functional diversity matters to ecosystem processes. Trends Ecol. Evol. 16, 646–655. doi: 10.1016/S0169-5347(01)02283-2
Diaz, S., Cabido, M., and Casanoves, F. (1998). Plant functional traits and environmental filters at a regional scale. J. Veg. Sci. 9, 113–122. doi: 10.2307/3237229
Eissenstat, D. M., Kucharski, J. M., Zadworny, M., Adams, T. S., and Koide, R. T. (2015). Linking root traits to nutrient foraging in arbuscular mycorrhizal trees in a temperate forest. New Phytol. 208, 114–124. doi: 10.1111/nph.13451
Finzi, A. C., Abramoff, R. Z., Spiller, K. S., Brzostek, E. R., Darby, B. A., Kramer, M. A., et al. (2015). Rhizosphere processes are quantitatively important components of terrestrial carbon and nutrient cycles. Glob. Chang. Biol. 21, 2082–2094. doi: 10.1111/gcb.12816
Fisher, J. B., Sitch, S., Malhi, Y., Fisher, R. A., Huntingford, C., and Tan, S.-Y. (2010). Carbon cost of plant nitrogen acquisition: a mechanistic, globally applicable model of plant nitrogen uptake, retranslocation, and fixation. Global Biogeochem. Cycles 24:GB1014. doi: 10.1029/2009gb003621
Freschet, G. T., Kichenin, E., and Wardle, D. A. (2015). Explaining within-community variation in plant biomass allocation: a balance between organ biomass and morphology above vs below ground? J. Veg. Sci. 26, 431–440. doi: 10.1111/jvs.12259
Freschet, G. T., Roumet, C., Comas, L. H., Weemstra, M., Bengough, A. G., Rewald, B., et al. (2021). Root traits as drivers of plant and ecosystem functioning: current understanding, pitfalls and future research needs. New Phytol. 232, 1123–1158. doi: 10.1111/nph.17072
Friedman, D. (2022). Topicdoc: Topic-specific diagnostics for LDA and CTM topic models. R package version 0.1.1. Available at: https://CRAN.R-project.org/package=topicdoc.
Fujii, K., Shibata, M., Kitajima, K., Ichie, T., Kitayama, K., and Turner, B. L. (2018). Plant–soil interactions maintain biodiversity and functions of tropical forest ecosystems. Ecol. Res. 33, 149–160. doi: 10.1007/s11284-017-1511-y
Funk, J. L., Larson, J. E., Ames, G. M., Butterfield, B. J., Cavender-Bares, J., Firn, J., et al. (2017). Revisiting the holy grail: using plant functional traits to understand ecological processes. Biol. Rev. 92, 1156–1173. doi: 10.1111/brv.12275
Gadgil, R. L., and Gadgil, P. D. (1971). Mycorrhiza and litter decomposition. Nature 233:133. doi: 10.1038/233133a0
Gehlenborg, N. (2019). UpSetR: A more scalable alternative to Venn and Euler diagrams for visualising intersecting sets. R package version 1.4.0. Available at: https://CRAN.R-project.org/package=UpSetR.
Grime, J. P., Thompson, K., Hunt, R., Hodgson, J. G., Cornelissen, J. H. C., Hendry, G. A. F., et al. (1997). Integrated screening validates primary axes of specialisation in plants. Oikos 79, 259–281. doi: 10.2307/3546011
Grün, B., and Hornik, K. (2022). Topicmodels: Topic models. R package version 0.2–13. Available at: https://CRAN.R-project.org/package=topoicmodels.
Gu, Z., Gu, L., Eils, R., Schlesner, M., and Brors, B. (2014). Circlize implements and enhances circular visualisation in R. Bioinformatics 30, 2811–2812. doi: 10.1093/bioinformatics/btu393
Guan, K., Pan, M., Li, H., Wolf, A., Wu, J., Medvigy, D., et al. (2015). Photosynthetic seasonality of global tropical forests constrained by hydroclimate. Nat. Geosci. 8, 284–289. doi: 10.1038/ngeo2382
Guerrero-Ramírez, N. R., Mommer, L., Freschet, G. T., Iversen, C. M., McCormack, M. L., Kattge, J., et al. (2021). Global root traits (GRooT) database. Glob. Ecol. Biogeogr. 30, 25–37. doi: 10.1111/geb.13179
Guo, D., Xia, M., Wei, X., Chang, W., Liu, Y., and Wang, Z. (2008). Anatomical traits associated with absorption and mycorrhizal colonisation are linked to root branch order in twenty-three Chinese temperate tree species. New Phytol. 180, 673–683. doi: 10.1111/j.1469-8137.2008.02573.x
Gutiérrez, J. M., Jones, R. G., Narisma, G. T., Alves, L. M., Amjad, M., Gorodetskaya, I. V., et al. (2021). Atlas. In Climate change 2021: The physical science basis. Contribution of working group I to the sixth assessment report of the intergovernmental panel on climate change. Available at: http://interactive-atlas.ipcc.ch/.
Han, M., Chen, Y., Li, R., Yu, M., Fu, L., Li, S., et al. (2022). Root phosphatase activity aligns with the collaboration gradient of the root economics space. New Phytol. 234, 837–849. doi: 10.1111/nph.17906
Hertel, D., Moser, G., Culmsee, H., Erasmi, S., Horna, V., Schuldt, B., et al. (2009). Below-and aboveground biomass and net primary production in a Paleotropical natural forest (Sulawesi, Indonesia) as compared to neotropical forests. For. Ecol. Manag. 258, 1904–1912. doi: 10.1016/j.foreco.2009.07.019
Hogan, J. A., Valverde-Barrantes, O. J., Ding, Q., Xu, H., and Baraloto, C. (2020). Morphological variation of fine root systems and leaves in primary and secondary tropical forests of Hainan Island, China. Ann. For. Sci. 77, 1–21. doi: 10.1007/s13595-020-00977-7
Holdaway, R. J., Richardson, S. J., Dickie, I. A., Peltzer, D. A., and Coomes, D. A. (2011). Species-and community-level patterns in fine root traits along a 120000-year soil chronosequence in temperate rain forest. J. Ecol. 99, 954–963. doi: 10.1111/j.1365-2745.2011.01821.x
Issifu, H., Vergeer, P., Ametsitsi, G. K. D., Klijn, J., Sartorelli, P., Tanson, M., et al. (2021). Responses of tropical tree seedlings in the forest–savanna boundary to combined effects of grass competition and fire. Biotropica 53, 1082–1095. doi: 10.1111/btp.12948
Iversen, C. M., and McCormack, M. L. (2021). Filling gaps in our understanding of belowground plant traits across the world: an introduction to a virtual issue. New Phytol. 231, 2097–2103. doi: 10.1111/nph.17326
Jackson, R. B., Mooney, H. A., and Schulze, E. D. (1997). A global budget for fine root biomass, surface area, and nutrient contents. Proc. Natl. Acad. Sci. U. S. A. 94, 7362–7366. doi: 10.1073/pnas.94.14.7362
Kassambara, A. (2022). Ggpubr: “ggplot2” based publication ready plots. R package 0.5.0. Available at: https://CRAN.R-project.org/package=ggpubr.
Kier, G., Mutke, J., Dinerstein, E., Ricketts, T. H., Küper, W., Kreft, H., et al. (2005). Global patterns of plant diversity and floristic knowledge. J. Biogeogr. 32, 1107–1116. doi: 10.1111/j.1365-2699.2005.01272.x
Kimball, B. A., Alonso-Rodríguez, A. M., Cavaleri, M. A., Reed, S. C., González, G., and Wood, T. E. (2018). Infrared heater system for warming tropical forest understory plants and soils. Ecol. Evol. 8, 1932–1944. doi: 10.1002/ece3.3780
Kong, D. L., Wang, J. J., Kardol, P., Wu, H. F., Zeng, H., Deng, X. B., et al. (2016). Economic strategies of plant absorptive roots vary with root diameter. Biogeosciences 13, 415–424. doi: 10.5194/bg-13-415-2016
Kramer-Walter, K. R., and Laughlin, D. C. (2017). Root nutrient concentration and biomass allocation are more plastic than morphological traits in response to nutrient limitation. Plant Soil 416, 539–550. doi: 10.1007/s11104-017-3234-9
Lambers, H., Raven, J. A., Shaver, G. R., and Smith, S. E. (2008). Plant nutrient-acquisition strategies change with soil age. Trends Ecol. Evol. 23, 95–103. doi: 10.1016/j.tree.2007.10.008
Lambers, H., Shane, M. W., Cramer, M. D., Pearse, S. J., and Veneklaas, E. J. (2006). Root structure and functioning for efficient acquisition of phosphorus: matching morphological and physiological traits. Ann. Bot. 98, 693–713. doi: 10.1093/aob/mcl114
Lavorel, S., Díaz, S., Cornelissen, J. H. C., Garnier, E., Harrison, S. P., McIntyre, S., et al. (2007). Plant functional types: are we getting any closer to the holy grail?. In Canadell, J. G., Pataki, D. E., and Pitelka, L. F., Terrestrial Ecosystems in a Changing World. Berlin: Springer, 149–164.
Lavorel, S., and Garnier, E. (2002). Predicting changes in community composition and ecosystem functioning from plant traits: revisiting the holy grail. Funct. Ecol. 16, 545–556. doi: 10.1046/j.1365-2435.2002.00664.x
Le Stradic, S., Roumet, C., Durigan, G., Cancian, L., and Fidelis, A. (2021). Variation in biomass allocation and root functional parameters in response to fire history in Brazilian savannas. J. Ecol. 109, 4143–4157. doi: 10.1111/1365-2745.13786
Li, W., Jin, C., Guan, D., Wang, Q., Wang, A., Yuan, F., et al. (2015). The effects of simulated nitrogen deposition on plant root traits: a meta-analysis. Soil Biol. Biochem. 82, 112–118. doi: 10.1016/j.soilbio.2015.01.001
Liu, M., Li, C., Xu, X., Wanek, W., Jiang, N., Wang, H., et al. (2017). Organic and inorganic nitrogen uptake by 21 dominant tree species in temperate and tropical forests. Tree Physiol. 37, 1515–1526. doi: 10.1093/treephys/tpx046
Lugli, L. F., Rosa, J. S., Andersen, K. M., Di Ponzio, R., Almeida, R. V., Pires, M., et al. (2021). Rapid responses of root traits and productivity to phosphorus and cation additions in a tropical lowland forest in Amazonia. New Phytol 230, 116–128. doi: 10.1111/nph.17154
McCormack, L. M., Adams, T. S., Smithwick, E. A. H., and Eissenstat, D. M. (2012). Predicting fine root lifespan from plant functional traits in temperate trees. New Phytol. 195, 823–831. doi: 10.1111/j.1469-8137.2012.04198.x
McCormack, M. L., Dickie, I. A., Eissenstat, D. M., Fahey, T. J., Fernandez, C. W., Guo, D., et al. (2015). Redefining fine roots improves understanding of belowground contributions to terrestrial biosphere processes. New Phytol. 207, 505–518. doi: 10.1111/nph.13363
Menge, D. N. L., Chisholm, R. A., Davies, S. J., Abu Salim, K., Allen, D., Alvarez, M., et al. (2019). Patterns of nitrogen-fixing tree abundance in forests across Asia and America. J. Ecol. 107, 2598–2610. doi: 10.1111/1365-2745.13199
Mohan, J. E., Cowden, C. C., Baas, P., Dawadi, A., Frankson, P. T., Helmick, K., et al. (2014). Mycorrhizal fungi mediation of terrestrial ecosystem responses to global change: mini-review. Fungal Ecol. 10, 3–19. doi: 10.1016/j.funeco.2014.01.005
Mucha, J., Zadworny, M., Helmisaari, H. S., Nihlgård, B., Repo, T., Żytkowiak, M., et al. (2020). Fine root classification matters: nutrient levels in different functional categories, orders and diameters of roots in boreal Pinus sylvestris across a latitudinal gradient. Plant Soil 447, 507–520. doi: 10.1007/s11104-019-04395-1
Muthukumar, T., Sha, L., Yang, X., Cao, M., Tang, J., and Zheng, Z. (2003). Mycorrhiza of plants in different vegetation types in tropical ecosystems of Xishuangbanna, Southwest China. Mycorrhiza 13, 289–297. doi: 10.1007/s00572-003-0234-9
Nasto, M. K., Winter, K., Turner, B. L., and Cleveland, C. C. (2019). Nutrient acquisition strategies augment growth in tropical N2-fixing trees in nutrient-poor soil and under elevated CO2. Ecology 100:e02646. doi: 10.1002/ecy.2646
Newbery, D. M., Alexander, I. J., and Rother, J. A. (1997). Phosphorus dynamics in a lowland African rain forest: the influence of ectomycorrhizal trees. Ecol. Monogr. 67, 367–409. doi: 10.1890/0012-9615(1997)067[0367:PDIALA]2.0.CO;2
Nie, M., Lu, M., Bell, J., Raut, S., and Pendall, E. (2013). Altered root traits due to elevated CO2: a meta-analysis. Glob. Ecol. Biogeogr. 22, 1095–1105. doi: 10.1111/geb.12062
Norby, R. J., De Kauwe, M. G., Domingues, T. F., Duursma, R. A., Ellsworth, D. S., Goll, D. S., et al. (2016). Model-data synthesis for the next generation of forest free-air CO2 enrichment (FACE) experiments. New Phytol. 209, 17–28. doi: 10.1111/nph.13593
Olson, D. M., and Dinerstein, E. (2002). The global 200: priority ecoregions for global conservation. Ann. Missouri Bot. Gard. 89, 199–224. doi: 10.2307/3298564
Page, M. J., McKenzie, J. E., Bossuyt, P. M., Boutron, I., Hoffmann, T. C., Mulrow, C. D., et al. (2021). The PRISMA 2020 statement: an updated guideline for reporting systematic reviews. J. Clin. Epidemiol. 134, 178–189. doi: 10.1016/j.jclinepi.2021.03.001
Pedersen, O., Sauter, M., Colmer, T. D., and Nakazono, M. (2021). Regulation of root adaptive anatomical and morphological traits during low soil oxygen. New Phytol. 229, 42–49. doi: 10.1111/nph.16375
Pérez-Ramos, I. M., Roumet, C., Cruz, P., Blanchard, A., Autran, P., and Garnier, E. (2012). Evidence for a “plant community economics spectrum” driven by nutrient and water limitations in a Mediterranean rangeland of southern France. J. Ecol. 100, 1315–1327. doi: 10.1111/1365-2745.12000
Phoenix, G. K., Hicks, W. K., Cinderby, S., Kuylenstierna, J. C. I., Stock, W. D., Dentener, F. J., et al. (2006). Atmospheric nitrogen deposition in world biodiversity hotspots: the need for a greater global perspective in assessing N deposition impacts. Glob. Chang. Biol. 12, 470–476. doi: 10.1111/j.1365-2486.2006.01104.x
Poorter, L., and Bongers, F. (2006). Leaf traits are good predictors of plant performance across 53 rain forest species. Ecology 87, 1733–1743. doi: 10.1890/0012-9658(2006)87[1733:LTAGPO]2.0.CO;2
Poorter, L., and Markesteijn, L. (2008). Seedling traits determine drought tolerance of tropical tree species. Biotropica 40, 321–331. doi: 10.1111/j.1744-7429.2007.00380.x
Prieto, I., Roumet, C., Cardinael, R., Dupraz, C., Jourdan, C., Kim, J. H., et al. (2015). Root functional parameters along a land-use gradient: evidence of a community-level economics spectrum. J. Ecol. 103, 361–373. doi: 10.1111/1365-2745.12351
Reichert, T., Rammig, A., Fuchslueger, L., Lugli, L. F., Quesada, C. A., and Fleischer, K. (2022). Plant phosphorus-use and -acquisition strategies in Amazonia. New Phytol. 234, 1126–1143. doi: 10.1111/nph.17985
Riddoch, I., Lehto, T., and Grace, J. (1991). Photosynthesis of tropical tree seedlings in relation to light and nutrient supply. New Phytol. 119, 137–147. doi: 10.1111/j.1469-8137.1991.tb01017.x
Saner, P., Philipson, C., Ong, R. C., Majalap, N., Egli, S., and Hector, A. (2011). Positive effects of ectomycorrhizal colonisation on growth of seedlings of a tropical tree across a range of forest floor light conditions. Plant Soil 338, 411–421. doi: 10.1007/s11104-010-0555-3
Shipley, B., and Meziane, D. (2002). The balanced-growth hypothesis and the allometry of leaf and root biomass allocation. Funct. Ecol. 16, 326–331. doi: 10.1046/j.1365-2435.2002.00626.x
Siefert, A., Violle, C., Chalmandrier, L., Albert, C. H., Taudiere, A., Fajardo, A., et al. (2015). A global meta-analysis of the relative extent of intraspecific trait variation in plant communities. Ecol. Lett. 18, 1406–1419. doi: 10.1111/ele.12508
Slik, J. W. F., Poulsen, A. D., Ashton, P. S., Cannon, C. H., Eichhorn, K. A. O., Kartawinata, K., et al. (2003). A floristic analysis of the lowland dipterocarp forests of Borneo. J. Biogeogr. 30, 1517–1531. doi: 10.1046/j.1365-2699.2003.00967.x
Spitzer, C. M., Lindahl, B., Wardle, D. A., Sundqvist, M. K., Gundale, M. J., Fanin, N., et al. (2021). Root trait–microbial relationships across tundra plant species. New Phytol. 229, 1508–1520. doi: 10.1111/nph.16982
Steidinger, B. S., Turner, B. L., Corrales, A., and Dalling, J. W. (2015). Variability in potential to exploit different soil organic phosphorus compounds among tropical montane tree species. Funct. Ecol. 29, 121–130. doi: 10.1111/1365-2435.12325
Sun, L., Ataka, M., Han, M., Han, Y., Gan, D., Xu, T., et al. (2021). Root exudation as a major competitive fine-root functional trait of 18 coexisting species in a subtropical forest. New Phytol. 229, 259–271. doi: 10.1111/nph.16865
Sweeney, C. J., De Vries, F. T., Van Dongen, B. E., and Bardgett, R. D. (2021). Root traits explain rhizosphere fungal community composition among temperate grassland plant species. New Phytol. 229, 1492–1507. doi: 10.1111/nph.16976
Taylor, M. K., Lankau, R. A., and Wurzburger, N. (2016). Mycorrhizal associations of trees have different indirect effects on organic matter decomposition. J. Ecol. 104, 1576–1584. doi: 10.1111/1365-2745.12629
Tedersoo, L., Laanisto, L., Rahimlou, S., Toussaint, A., Hallikma, T., and Pärtel, M. (2018). Global database of plants with root-symbiotic nitrogen fixation: NodDB. J. Veg. Sci. 29, 560–568. doi: 10.1111/jvs.12627
Tezara, W., Mitchell, V. J., Driscoll, S. D., and Lawlor, D. W. (1999). Water stress inhibits plant photosynthesis by decreasing coupling factor and ATP. Nature 401, 914–917. doi: 10.1038/44842
Townsend, A. R., Asner, G. P., and Cleveland, C. C. (2008). The biogeochemical heterogeneity of tropical forests. Trends Ecol. Evol. 23, 424–431. doi: 10.1016/j.tree.2008.04.009
Valenzuela-Estrada, L. R., Vera-Caraballo, V., Ruth, L. E., and Eissenstat, D. M. (2008). Root anatomy, morphology, and longevity among root orders in Vaccinium corymbosum (Ericaceae). Am. J. Bot. 95, 1506–1514. doi: 10.3732/ajb.0800092
Valverde-Barrantes, O. J., Freschet, G. T., Roumet, C., and Blackwood, C. B. (2017). A worldview of root traits: the influence of ancestry, growth form, climate and mycorrhizal association on the functional trait variation of fine-root tissues in seed plants. New Phytol. 215, 1562–1573. doi: 10.1111/nph.14571
Valverde-Barrantes, O. J., Smemo, K. A., Feinstein, L. M., Kershner, M. W., and Blackwood, C. B. (2013). The distribution of belowground traits is explained by intrinsic species differences and intraspecific plasticity in response to root neighbours. J. Ecol. 101, 933–942. doi: 10.1111/1365-2745.12087
Violle, C., Navas, M.-L., Vile, D., Kazakou, E., Fortunel, C., Hummel, I., et al. (2007). Let the concept of trait be functional! Oikos 116, 882–892. doi: 10.1111/j.2007.0030-1299.15559.x
Vleminckx, J., Fortunel, C., Valverde-Barrantes, O., Timothy Paine, C. E., Engel, J., Petronelli, P., et al. (2021). Resolving whole-plant economics from leaf, stem and root traits of 1467 Amazonian tree species. Oikos 130, 1193–1208. doi: 10.1111/oik.08284
Wang, J., Defrenne, C., McCormack, M. L., Yang, L., Tian, D., Luo, Y., et al. (2021). Fine-root functional trait responses to experimental warming: a global meta-analysis. New Phytol. 230, 1856–1867. doi: 10.1111/nph.17279
Wang, B., and Qiu, Y.-L. (2006). Phylogenetic distribution and evolution of mycorrhizas in land plants. Mycorrhiza 16, 299–363. doi: 10.1007/s00572-005-0033-6
Waring, B. G., and Powers, J. S. (2017). Overlooking what is underground: root:shoot ratios and coarse root allometric equations for tropical forests. For. Ecol. Manag. 385, 10–15. doi: 10.1016/j.foreco.2016.11.007
Weemstra, M., Freschet, G. T., Stokes, A., and Roumet, C. (2021). Patterns in intraspecific variation in root traits are species-specific along an elevation gradient. Funct. Ecol. 35, 342–356. doi: 10.1111/1365-2435.13723
Weemstra, M., Kiorapostolou, N., van Ruijven, J., Mommer, L., de Vries, J., and Sterck, F. (2020). The role of fine-root mass, specific root length and life span in tree performance: a whole-tree exploration. Funct. Ecol. 34, 575–585. doi: 10.1111/1365-2435.13520
Weemstra, M., Mommer, L., Visser, E. J. W., Van Ruijven, J., Kuyper, T. W., Mohren, G. M. J., et al. (2016). Towards a multidimensional root trait framework: a tree root review. New Phytol. 211, 1159–1169. doi: 10.1111/nph.14003
Weigelt, A., Mommer, L., Andraczek, K., Iversen, C. M., Bergmann, J., Bruelheide, H., et al. (2021). An integrated framework of plant form and function: the belowground perspective. New Phytol. 232, 42–59. doi: 10.1111/nph.17590
Wieder, W. R., Cleveland, C. C., Smith, W. K., and Todd-Brown, K. (2015). Future productivity and carbon storage limited by terrestrial nutrient availability. Nat. Geosci. 8, 441–444. doi: 10.1038/NGEO2413
Xia, M., Guo, D., and Pregitzer, K. S. (2010). Ephemeral root modules in Fraxinus mandshurica. New Phytol. 188, 1065–1074. doi: 10.1111/j.1469-8137.2010.03423.x
Yamauchi, T., Colmer, T. D., Pedersen, O., and Nakazono, M. (2018). Regulation of root traits for internal aeration and tolerance to soil waterlogging-flooding stress. Plant Physiol. 176, 1118–1130. doi: 10.1104/pp.17.01157
Zhou, Y., Wigley, B. J., Case, M. F., Coetsee, C., and Staver, A. C. (2020). Rooting depth as a key woody functional trait in savannas. New Phytol. 227, 1350–1361. doi: 10.1111/nph.16613
Zhou, G., Zhou, X., Nie, Y., Bai, S. H., Zhou, L., Shao, J., et al. (2018). Drought-induced changes in root biomass largely result from altered root morphological traits: evidence from a synthesis of global field trials. Plant Cell Environ. 41, 2589–2599. doi: 10.1111/pce.13356
Keywords: belowground processes, global change, Paleotropical communities, root traits, trait representation
Citation: Lee MY and Andersen KM (2023) Global root trait underrepresentation in Paleotropical communities: a qualitative analysis. Front. For. Glob. Change. 6:1206225. doi: 10.3389/ffgc.2023.1206225
Edited by:
Camille Emilie Defrenne, Michigan Technological University, United StatesReviewed by:
Joshua S. Caplan, Temple University, United StatesNannan An, Fujian Normal University, China
Copyright © 2023 Lee and Andersen. This is an open-access article distributed under the terms of the Creative Commons Attribution License (CC BY). The use, distribution or reproduction in other forums is permitted, provided the original author(s) and the copyright owner(s) are credited and that the original publication in this journal is cited, in accordance with accepted academic practice. No use, distribution or reproduction is permitted which does not comply with these terms.
*Correspondence: Ming Yang Lee, bGVlbXkwOTk1QGdtYWlsLmNvbQ==