- 1Ecophysiology of Plants, Professorship for Land Surface-Atmosphere Interactions, Research Department Life Science Systems, TUM School of Life Sciences, Technical University of Munich, Freising, Germany
- 2Helmholtz Zentrum München–German Research Center for Environmental Health (GmbH), Research Unit Environmental Simulation, Neuherberg, Germany
- 3Institute of Forest Ecology, Department of Forest and Soil Sciences, University of Natural Resources and Life Sciences (BOKU), Vienna, Austria
- 4Department of Forest Entomology and Pathology, Faculty of Forestry and Wood Technology, Poznań University of Life Sciences, Poznań, Poland
With climate change, the frequency of severe droughts is predicted to increase globally, resulting in increased forest dieback. Although fine-root systems and their associated fungi are considered crucial for tree nutrient exchange after a drought period and consequently for tree recovery, post-drought dynamics remain poorly understood. We rewatered mature European beech and Norway spruce after a 5-year experimental summer drought to shed light on belowground recovery processes. Therefore, we tracked the fine-root parameters growth, vitality, and mycorrhization in monospecific rooting zones with intraspecific root contact and mixed rooting zones with interspecific root contact of both tree species during the first 3 months of recovery, and we analyzed compositions of their root-associated fungal communities by DNA- and RNA-ITS2 sequencing. During recovery, the fine-root parameters differed between both tree species, with only minor effects of the tree rooting zone. Root-associated fungal communities showed no significant response to irrigation within 3 months after drought release. The rooting zone was the dominating factor affecting the root-associated fungal diversity, the abundance of trophic modes, and the response of individual saprotrophic and ectomycorrhizal (ECM) species. Furthermore, an analysis of the most abundant fungal species revealed that for ECM fungi, drought tolerance was common and for saprotrophs, a facultative, root-associated lifestyle. These results suggest that tree species-specific fungal communities are stable despite previous long-term drought and are closely associated with tree species-specific response patterns related to root survival and recovery. Moreover, an association between saprotrophic fungi and roots might be a strategy to support fungal drought survival.
Introduction
Climate change is predicted to increase the frequency and duration of severe droughts worldwide (IPCC, 2021), causing an alarming expansion of forest dieback (Allen et al., 2010, 2015; Anderegg et al., 2013).
Some tree species such as Norway spruce [Picea abies (L.) Karst.] and European beech (Fagus sylvatica L.) often grow better in mixed stands compared to monospecific stands (Pretzsch et al., 2015; Pretzsch, 2022). Therefore, mixing tree species has been suggested as a strategy of forest management to counteract the damages of climate change (Zhang et al., 2012; Forrester, 2015; Pretzsch et al., 2015). Thus, beech trees growing in mixture with conifers have been shown to exploit water of deeper soil layers and to have an improved water use in dry stands (Grossiord et al., 2014; González de Andrés et al., 2018). Reciprocally, spruce growth may profit from being mixed with beech, particularly at dry sites (Rukh et al., 2020) due to hydraulic redistribution (Hafner et al., 2017).
While studies are adding increasingly more details on the drought reactions of aboveground tree organs (e.g., McDowell et al., 2008; Brunner et al., 2015), the belowground system is much less studied, despite access to soil water being crucial for tree survival (Körner, 2019). It is well established that European beech and Norway spruce react differently to drought at the fine-root level (Nikolova et al., 2020). While beech continuously produces fine roots with low biomass, high metabolic activity, and short life span under drought (e.g., Reich, 2014; Nikolova et al., 2020; Zwetsloot and Bauerle, 2021), spruce preserves its long-living fine roots of larger diameter by increased suberisation (e.g., Gaul et al., 2008; Nikolova et al., 2020; Zwetsloot and Bauerle, 2021). The longevity of beech fine roots with interspecific contact with spruce fine roots was increased under drought compared to fine roots with only intraspecific contact, while the survival of spruce fine roots was not related to inter- or intraspecific root contact (Zwetsloot and Bauerle, 2021). However, the interaction of fine roots with mycorrhizal fungi forms the interface for water and nutrients between a tree and the surrounding soil (Lehto and Zwiazek, 2011), with pioneer roots exploring the soil and short roots being colonized primarily by symbiotrophic ectomycorrhizal (ECM) fungi (Kottke and Oberwinkler, 1986; Zadworny and Eissenstat, 2011). Therefore, it is necessary to look at both the root system and the root-associated fungi together to better understand how they interact under changing soil water availability.
Root-associated fungal communities comprise fungi of different trophic modes [saprotrophic, pathotrophic, and symbiotrophic (sensu Nguyen et al., 2016)] and fungi with unknown ecological role (e.g., Tedersoo and Smith, 2013; Nguyen et al., 2016; Unuk et al., 2019). Some saprotrophic species were reported to be able to colonize fine roots and even form mantle and Hartig net-like structures (Smith et al., 2017). As dark septate endophytes, they can accumulate high concentrations of melanin in their hyphae within the roots, which is considered a response to environmental pressure such as drought (Berthelot et al., 2019). Nowadays, they are considered as an important evolutionary step between free-living saprotrophs and mycorrhizal fungi (Ruotsalainen et al., 2022). Furthermore, ECM fungi were shown to play a crucial role in the evolutionary adaptation of land plants to drought and thus can promote both the gain and loss of plant drought resistance to the same extent (Cosme, 2023). Tree mixture increases the local heterogeneity in the soil, thus creating a diverse habitat for fungi (Conn and Dighton, 2000; Aponte et al., 2010). ECM fungi can buffer drought effects on trees (Mohan et al., 2014; Bennett and Classen, 2020) by, e.g., improved water uptake (Maurel et al., 2015; Bennett and Classen, 2020) and improved nutrient status (Köhler et al., 2018). A strong link between plant drought response and ECM fungi has been found, resulting in considerable changes of the root fungal community composition through drought and watering (Veach et al., 2020). Moreover, mycorrhizal fungi can be less affected by drought than free-living soil fungi (Castaño et al., 2018). The ECM community composition shifted during drought in most cases (Shi et al., 2002; Swaty et al., 2004; Richard et al., 2011; Nickel et al., 2018). The drought tolerance of ECM fungi differs between species (Lehto and Zwiazek, 2011), with drought-sensitive species such as Hygrophorus sp. declining (Taniguchi et al., 2018) and tolerant species such as Cenococcum geophilum being highly abundant during desiccation (Querejeta et al., 2009; Peter et al., 2016; Gehring et al., 2020).
After drought release, both beech and spruce prioritize resource allocation to the recovery of the fine roots (Hagedorn et al., 2016; Hikino et al., 2022). A study on black cottonwood seedlings suggested that after drought release, roots of smaller diameter were produced that had a better water uptake capacity than old roots but a lower total surface area (Dhiman et al., 2018). Nevertheless, it remains unclear how individual fine-root parameters such as vitality, growth, and mycorrhization of individual tree species as well as the composition of root-associated fungal communities change after rewatering and how they are influenced by tree species mixture. Furthermore, field studies that control soil moisture are rare in mature stands but necessary to understand changes in root-associated fungal communities and their interplay with roots in terms of drought tolerance and ability to recover in a natural environment. Therefore, we asked if previous droughts would affect the response patterns of fine-root-associated fungal communities upon rewatering.
The Kranzberg roof experiment (KROOF) was initiated in Southern Germany to study the effects of experimental drought by excluded precipitation (throughfall exclusion) during the vegetation period from April to November for five consecutive years on mature beech and spruce trees. The trees were growing in plots each with areas of interspecific and intraspecific fine-root contact (rooting zone, RZ) [details in Grams et al. (2021)]. To better assess the trajectories of forests under future climates, it is necessary to understand to what extent the forest can recover. Therefore, the experimental drought phase ended with controlled watering in 2019. In this study on belowground recovery, we focused on the fungal community composition of regenerating fine roots of mature beech and spruce trees within 3 months after drought release. To do this, we used DNA and RNA metabarcoding to analyze the root-associated fungal communities and examined the vitality, growth, and mycorrhization of the fine roots.
Based on the different drought reactions of both tree species on the fine-root level and an increased beech fine-root longevity in mixture during drought, we anticipate that after drought release, both species follow different fine-root regeneration patterns, with beech reacting faster than spruce while profiting more from the tree mixture. The close interaction between fungi and trees leads us to hypothesize that the root-associated fungal communities change differently in the RZ, with ECM fungi being more abundant and less affected on beech roots after watering due to a continuous fine-root production during drought and a higher heterogeneity in the mixture having a positive effect on fungal diversity [H1]. Furthermore, we hypothesize that changes in soil moisture favor the abundance of fungal species tolerating high variations in soil moisture and saprotrophic fungal species with optional root-associated lifestyle [H2].
Materials and methods
Sampling site and experimental design
The KROOF experimental site is a 0.5 ha mature European beech and Norway spruce stand (c. 90 and 70 years old) located in Kranzberg Forest in Southern Germany (11° 39′ 42″ E, 48° 25′ 12″ N, 490 m a.s.l.) (Pretzsch et al., 2014; Goisser et al., 2016). From May to September, the mean rainfall in the 1971–2000 period was 460–500 mm, and the mean air temperature was 13.8°C (Pretzsch et al., 2020). The soil is a nutrient-rich Luvisol from Tertiary sediments and has good water supply (Pretzsch et al., 1998). In 2010, 12 experimental plots of 110–200 m2 were trenched to a depth of 1 m to inhibit water transport from outside the plots, while a clay layer at approximately 1 m depth limited vertical water transport (Grams et al., 2021). Each plot depicts three RZs: beech neighboring beech (BB), spruce neighboring spruce (SS)—both with mainly intraspecific fine-root contact—and beech neighboring spruce (MIX) with interspecific fine-root contact (Figure 1A; Goisser et al., 2016; Nickel et al., 2018). Retractable roofs were installed on six throughfall exclusion (TE) plots in 2013, and the drought experiment started in 2014. Rain was excluded over five whole vegetation periods (March to November) from 2014 to 2018. In 2015, two TE plots and their corresponding control (CO) plots were excluded due to bark beetle infestation in order to keep a balanced experimental design of 4 CO and 4 TE plots (Figure 1A). In 2019, the drought was released by rewetting of the TE plots using controlled drop irrigation within 40 h (total water supply of 12,849 ± 2,802 l) to reach a similar soil water content to their corresponding CO plots, as described in detail by Grams et al. (2021). After watering, the soil water contents were 14 ± 1% in CO and 15 ± 2% in TE (9 ± 1% before watering). The watering took place in three campaigns over 3 weeks to allow for sampling, measurements, and sample preparation. Therefore, plots 7 and 8 were watered on 25th of June 2019, plots 3, 4, 11, and 12 on 4th of July 2019, and plots 5 and 6 on 10th of July 2019. To reduce the watering effects between the treatments regarding soil temperature or nutrient availability, a low amount of water was added to the CO plots in parallel (average 2,035 ± 537 l). From day 15 after watering, the roofs of the TE plots were kept open.
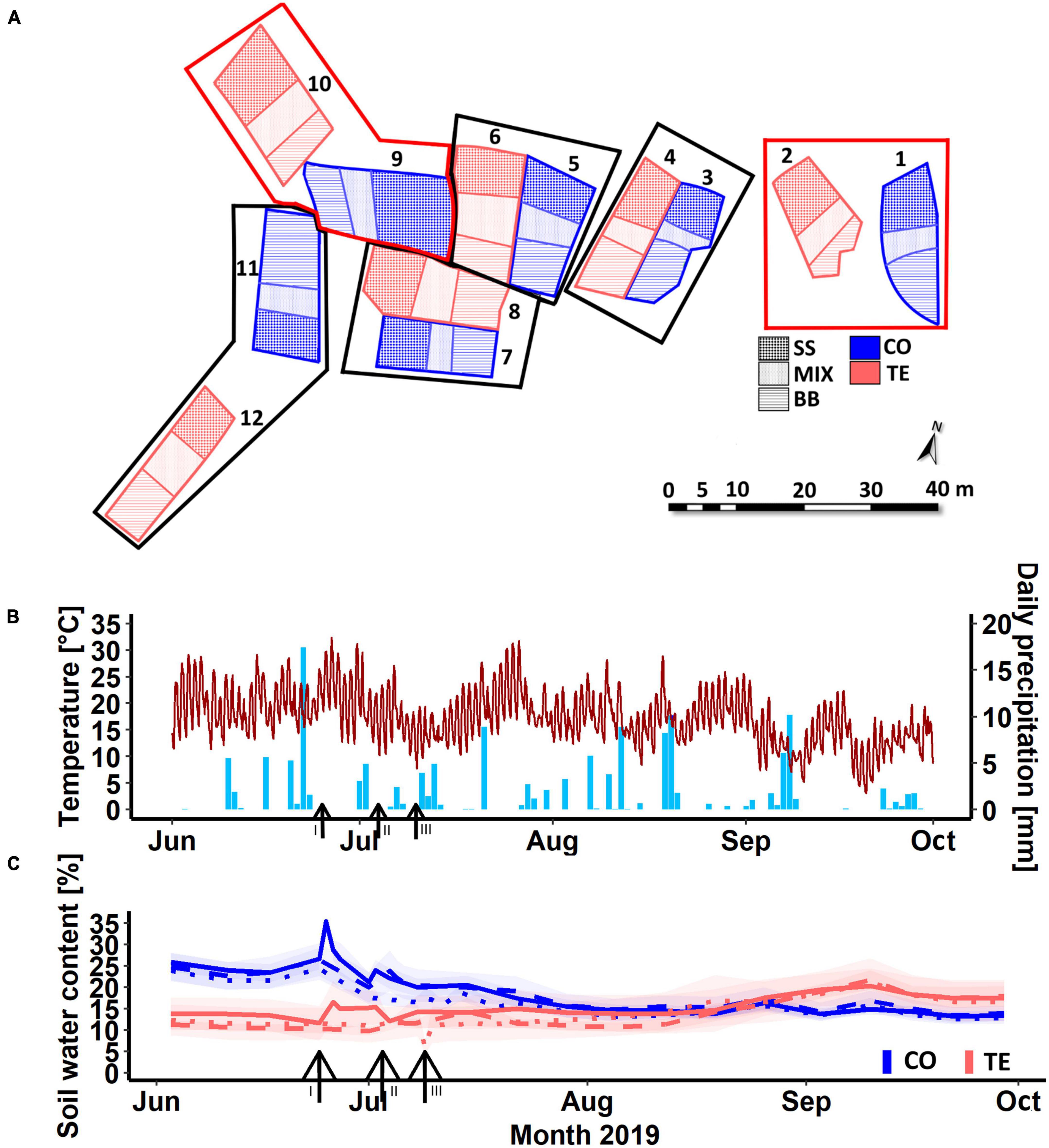
Figure 1. Arrangement of the experimental plots 1–12 (A). CO refers to controls and TE to rewetted former drought plots, SS indicates the spruce monospecific area, BB the beech monospecific area, and MIX the mixture. Boxes display corresponding pairs. Plots in red boxes have not been sampled. Panel (B) illustrates the weather data, including temperature (line) and precipitation (bars) within the sampling period. (C) Illustrates the soil water content in 0–7 cm of control (CO) and former drought plots (TE); different line types indicate the different watering campaigns: solid = first (I, plots 7, 8), dashed = second (II, plots 3, 4, 11, 12), and dotted = third (III, plots 5, 6). Black arrows and Roman numbers mark the starting day of the respective watering campaign: I = 25.06.2019, II = 04.07.2019, III = 10.07.2019. Ribbons = standard errors.
Temperature, precipitation, and soil moisture
Temperature at 2 m height and precipitation (Figure 1B) were continuously measured every 10 min by a climate station on site, including the experimental period from June to October 2019. Volumetric soil water contents at different depths (0–7 cm, 10–30 cm, 30–50 cm, and 50–70 cm) were assessed by time-domain reflectometers (TDR 100, Campbell Scientific Inc., Logan, Utah, USA) (Figure 1C). Within each treatment, the soil water contents did not significantly differ between the CO or TE plots of the different watering campaigns at each sampling day, thus justifying a combination of the data from the staggered watering campaigns (Supplementary Figure 1).
Fine-root sampling
Soil samples were taken on days (d) −7, 6, 18, 41, and 84 with regard to the respective irrigation event on each of the eight plots from each RZ (Figure 2). During sampling, ten drill cores (diameter 1.4 cm each, 25 cm depth) were taken, which corresponded to a surface area of 15.4 cm2, and the dark-colored organic topsoil layer (2–12 cm, average 9.4 cm) of the ten soil cores was pooled, resulting in 120 samples for further processing (8 plots × 3 RZ × 5 sampling dates). Fine roots (diameter < 2 mm) were separated from the soil on site on the sampling day by careful manual sorting with sterile forceps, put on dry ice, and frozen at −80°C until nucleic acid extractions and biomass analysis.
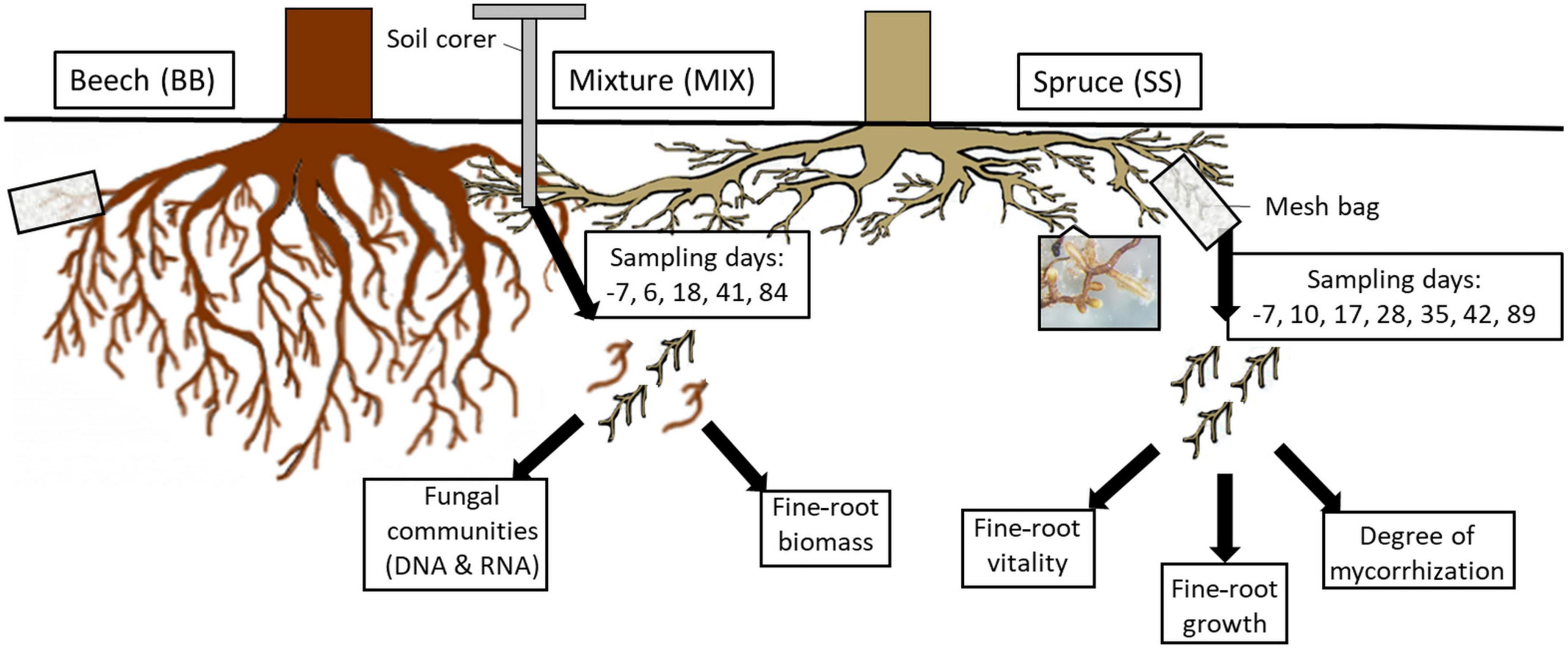
Figure 2. Overview of fine-root sampling methods (mesh bags and soil cores), sampling days (in boxes), and further processing of the respective fine roots. Each sampling was performed on beech and spruce trees on control (CO) and former drought (TE) plots in each rooting zone (SS; MIX, BB; mesh bags: beech–beech, beech–spruce, spruce–beech, spruce–spruce; soil cores: beech monospecific, mixture, spruce monospecific) starting at day −7 before rewatering until day 89 post rewatering. The fine-root picture shows an example of an ectomycorrhizal fungus on the root tips.
In April 2019, individual, tree-connected fine roots from depths of 0–5 cm (CO) and 0–10 cm (TE) were photographed, put into 1/3 soil-filled mesh bags (mesh width 80 μm, open area 29%), moisturized to improve root–soil contact, and covered with soil. Roots growing in MIX were classified as beech in mixture (Bmix) and spruce in mixture (Smix). A total of 608 mesh bags (2 tree species × 2 RZ per species × 4 replicates × 38 samplings; Supplementary Table 1) were thus prepared. Mesh bag roots were harvested and photographed on graph paper 7 days before and 10, 17, 28, 35, 42, and 89 days after watering (Figure 2).
Root vitality
For vitality assays, the method of Qian et al. (1998) was used. In brief, a 10% (w/v) stock solution of fluorescein diacetate (FDA) in acetone was 1:10 diluted with Sörensen phosphate buffer KH2PO4/Na2HPO4 pH 7.5 and used for staining fine-root sections. From each mesh bag, ten representative fine-root tips were cut longitudinally into three slices with a razor blade, whereby the middle slice was stained in FDA–phosphate buffer solution for 15 min in the dark, washed twice in phosphate buffer, and analyzed by fluorescent microscopy (Axioplan, Zeiss, Oberkochen, Germany). The fluorescence intensity of meristem, Hartig net (if mycorrhized) or root cortex, central cylinder, and hyphal mantle (if mycorrhized) was evaluated by eye and categorized in numbers from 0 (no fluorescence/dead) to 3 (bright green/very vital). Missing parts were excluded from the calculation. The vitality score per root tip is described by the mean of the root compartment’s vitality scores, and the vitality of one fine-root sample is reflected by the mean of their 10 accompanying tips’ vitality scores.
Root growth and degree of mycorrhization
Root growth was calculated by measuring the total root length based on the root pictures taken on graph paper before enclosure into mesh bags and at harvest using ImageJ (version 1.53a, National Institute of Health, USA) by subtracting the initial length from the length at harvest. To exclude growth between the mesh bag placement and first sampling day, the average growth at day −7 (per RZ and treatment) was subtracted from the growth values of all the other days. Growth at day −7 thus corresponded to 0. The degree of mycorrhization per root (given in % of ECM and non-ECM root tips relative to the total number of root tips) was determined by visual inspection of the root pictures and assignments based on growth form, tip shape, color, and presence of a hyphal mantle and external hyphae.
Fine-root biomass
Fine-root dry weights were calculated from the fresh weight of each mixed fine-root sample from soil cores using a subsample of 30 mg fine-cut root material dried for 24 h at 75°C. The dry root mass per soil volume was then calculated, accounting for the soil volume of the respective cores from which the samples were taken.
DNA/RNA extraction and high-throughput sequencing
The analysis of rapidly degrading fungal RNA allows to trace sudden changes in the community composition of metabolically active fungi, while DNA analysis can include residual DNA of dead fungi. To validate if the DNA-based data captures the dynamics of root-associated fungal communities in a changing environment correctly, we analyzed RNA-based data in parallel.
Frozen roots were ground in liquid nitrogen with sterile instruments and treated with RNase AWAY (Sigma-Aldrich, St. Louis, MO, USA). In all, 100–250 mg of root powder from each root was collected in 2 ml screw cap vials for DNA and RNA analysis. DNA of 120 root samples (and 5 negative extraction controls without root powder) was extracted using the DNeasy Power soil Kit (Qiagen, Hilden, Germany) with modifications, as described in Nickel et al. (2018). Due to the limited sample material, only 87 samples and 3 negative controls were processed for RNA extraction using the ZymoBIOMICS DNA/RNA Miniprep Kit (Zymo Research, Irvine, CA, USA) following the manufacturer’s protocol. RNA was transcribed to cDNA by applying the High-Capacity cDNA Reverse Transcription Kit (Applied Biosystems, Waltham, MA, USA). DNA and cDNA were treated equally during the library preparation. For high-throughput sequencing, the fungal ITS2 rDNA was amplified by PCR using primer mixes, as described in Tedersoo et al. (2015), which held overhangs with Illumina adapter sequences (Supplementary Table 2) for Miseq sequencing (protocol Part # 15044223; Illumina, San Diego, CA, USA). The reaction mix per sample included 10 μl NEBNext High-Fidelity 2X PCR MasterMix (New England Biolabs, Frankfurt, Germany), 0.5 μl 10 pmol ITS3 tagmix forward primer (equally mixed ITS3-Mix1 – 5), 0.5 μl 10 pmol ITS4 tagmix reverse primer (equally mixed ITS4-Mix 1–4), 8 μl ultra-pure H2O, and 1 μl (c)DNA (5 ng DNA, 10 ng cDNA). The PCR conditions were 5 min at 95°C, 28 cycles (30 s 95°C, 30 s 55°C, 60 s 72°C), and 10 min at 72°C. PCRs were performed in triplicates, which were pooled after quality verification on a 2% agarose gel. The samples were cleaned with the Agencourt AMPure XP DNA purification kit (Beckman Coulter, Krefeld, Germany) using a bead:sample ratio of 1:1. Success was tested on agarose gels. (c)DNA concentration was determined using AccuClear Ultra High Sensitivity dsDNA Quantification (Biotium, Fremont, CA, USA). Individual combinations of Illumina indexing primers (Nextera XT Index Kit v2 Sets A-D) were attached to each sample by indexing the PCR containing 1 μl (c)DNA (5 ng), 2.5 μl Nextera i7 primer, 2.5 μl Nextera i5 primer, 12.5 μl NEBNext High-Fidelity 2X PCR MasterMix, and 6.5 μl ultra-pure H2O. Conditions were 3 min at 95°C, 8 cycles (30 s 95°C, 30 s 55°C, 30 s 72°C), and 10 min at 72°C. Indexing products were purified and size- and quantity-checked as before, and additionally, a Fragment Analyzer (Advanced Analytical, Heidelberg, Germany) (protocol DNF-473 Standard Sensitivity NGS-Fragment Analysis Kit 1–6,000 bp) was used with randomly picked sample pairs before and after indexing. Diluted amplicons (10 nM) were pooled, quantity checked using Qubit 3 (Invitrogen, Carlsbad, CA, USA), and diluted to 4 nM. The final library preparation was handled following the Illumina protocol for the 16S Metagenomic Sequencing Library Preparation (protocol Part # 15044223 Rev. B) and sequenced on Miseq v3chemistry, 600 cycles flow cell (Illumina, San Diego, CA, USA).
Bioinformatics
Output FASTQ files from the Illumina Miseq system were prepared for analysis using the fungal analysis pipeline PIPITS v2.7 (Gweon et al., 2015). Briefly, in the first step, read pairs were joined and sequences filtered by quality corresponding to the pipeline’s default settings. In the second step, the fungal ITS2 was extracted with ITSx (Bengtsson-Palme et al., 2013). In the last step, small sequences (<100 bp) were erased, remaining sequences assigned to operational taxonomic units (OTUs) with a sequence identity of 97 % using VSEARCH (Rognes et al., 2016), and chimeras removed using the UNITE v8.2 (Abarenkov et al., 2020) UCHIME database. The taxonomic classification was achieved with RDP Classifier (Wang et al., 2007), comparing the sequences against the UNITE fungal ITS database (Kõljalg et al., 2013). The database FungalTraits (Põlme et al., 2020) was used to assign the functional traits “fungal trophic mode,” ECM “exploration types” (Agerer, 2001), and saprotrophic “primary” and “secondary” lifestyle to the fungi by combining the taxonomic output file with correlating information on the genus level.
Statistical analysis
Data were analyzed using R (version 4.0.2, R Core Team, 2021) and RStudio (version 1.4.1717, RStudio Inc.), with significances being defined as p < 0.05. Sequencing data were rarefied with 1,000 repetitions using the “rarefy” function in GNuniFrac (Chen et al., 2012) to a depth of 10,000 sequences per sample. Taxa that occurred less than 10 times in the whole experiment were removed. To investigate the dissimilarity of the individual samples, the Bray–Curtis dissimilarity was calculated (“vegdist” function in R package vegan). The Shannon–Wiener Diversity Index (“Shannon Index”) and Simpson’s Index of Diversity (“Simpson Index”) were determined using the function “diversity” in vegan (version 2.5-7; Oksanen et al., 2019) and species richness by using the function “specnumber” in the same package. Evenness was then assessed with the Shannon Index/log (species count). To analyze both the diversity parameters described above and the fine-root parameters’ “vitality,” “growth,” and “mycorrhization,” normal distribution was tested by the Shapiro–Wilk test (“shapiro.test” in package stats). Subsequently, normally distributed data were examined using an analysis of variance (ANOVA) (“aov” in package stats) and non-normally distributed data using a Kruskal–Wallis test (“kruskal.test” in package stats). Significant differences between levels of one factor were assessed using appropriate post-hoc tests with Bonferroni p-value correction. In the case of an ANOVA, a Tukey’s honest significance test (“TukeyHSD” in package stats) was subsequently used, and in the case of a Kruskal–Wallis test, a Dunn’s test for multiple comparisons (“dunn.test” in package dunn.test). After dispersion heterogeneity was tested using the function “betadisper” in vegan, community data were analyzed comparatively with a permutational multivariate analysis of variance (PERMANOVA) (“adonis2” in vegan) to determine the effects of treatment, RZ, and time after watering, with nested plot location and time being a random effect after testing its significance as a factor. All models were run with 9,999 permutations. Significant differences between individual levels of a factor were tested by multilevel pairwise comparisons of permutational multivariate analysis of variance (pairwise PERMANOVA) (pairwise.adonis; Martinez Arbizu, 2020) with Bonferroni p-value correction. The fungal community composition was represented by non-metric multi-dimensional scaling (NMDS) with Bray–Curtis dissimilarity using the “ordinate” function in phyloseq. Correlations between fine-root parameters and between ECM fungal community composition, represented by NMDS axis 1 (NMDS1), and fine-root growth, as well as vitality, were determined by Pearson correlation using the function “cor.test” (stats). Relative abundances of fungal trophic modes and exploration types were assessed using the phyloseq (version 1.36.0; McMurdie and Holmes, 2013) functions “prune_taxa” for trimming and “transform_sample_counts” for sample-by-sample abundance data transformation. Graphs were created using ggplot2 (Wickham, 2016). Relative proportions of fungal trophic modes on TE compared to CO (averaged “by day relative to watering”), as portrayed in Figure 5B, were created in phyloseq. The respective standard errors of the difference between the means (σM1−M2) were calculated according to Foster et al. (2018):
where and describe the variances of the CO and TE samples and n1 and n2 the respective number of replicates.
Results
Recovery of the fine-root system
Fine-root vitality was significantly lower in TE than CO before watering in all RZs except for Bmix (Figure 3A). Neither beech nor spruce generally showed significant differences in time course or treatment between RZs. However, the dynamics in fine-root vitality after watering were species specific. Root vitality in BB and Bmix TE increased from low levels (0–0.2) until d 42 (0.6) to reach CO levels but, in contrast to CO, declined to zero on d 89. For spruce, fine-root vitality in TE increased after rewatering and resembled controls already on d 17 with a faster increase when growing in mixture (Smix).
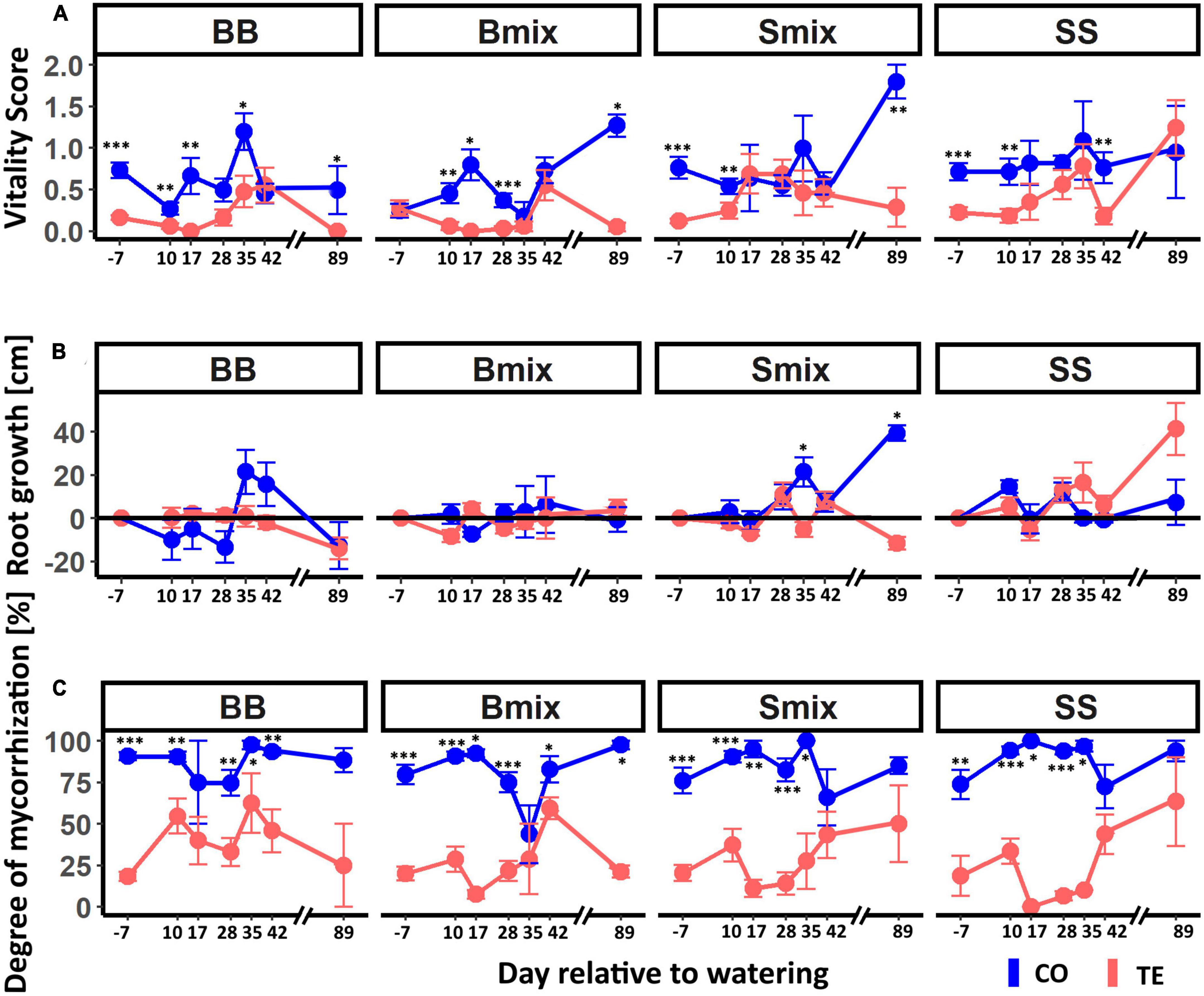
Figure 3. Vitality, growth, and mycorrhization of beech and spruce fine roots in different rooting zones after drought release. CO lines represent control roots and TE lines roots in recovering plots. (A) displays the dynamics in average fine-root vitality on a score from 0 (dead) to 3 (maximal vitality). Panel (B) shows the average root growth (cm) after watering in which the black horizontal line indicates equality between growth (positive) and degradation (negative). Panel (C) illustrates the average mycorrhization of the roots. All mean values are given with standard error. Asterisks indicate significances between the treatments with *p < 0.05, **p < 0.01, and ***p < 0.001.
Similarly, fine-root growth after rewatering followed different dynamics in the two tree species (Figure 3B). In beech, growth did not significantly differ between the CO and TE or RZ and remained around zero except in BB CO on d 35 and d 42. Growth of spruce roots was more dynamic, with significant differences between SS and Smix [CO: p = 0.02, z = (−2.08); TE: p = 0.03, z = (−1.82); Dunn-Test], and responded faster to watering in SS than in Smix. Growth in Smix CO was significantly higher than in TE on d 35 and d 89, while it did not significantly differ between treatments in SS.
The degree of mycorrhization (Figure 3C) was slightly below 25% in all TEs and RZs and significantly lower compared to CO until day 35. In beech, the degree of mycorrhization increased (earlier in BB than in Bmix), while it decreased in spruce RZ until d 17 and then reached the CO level from d 42.
The fine-root biomass was highly variable (Supplementary Figure 2), and thus the roughly two-fold increase of dry mass in all TEs and RZs between d −7 and d 7 was not significant.
Fine-root vitality positively correlated with fine-root growth for both treatments and both tree species (Supplementary Table 3). Fine-root growth and degree of mycorrhization correlated in CO but not TE for both tree species, while vitality and degree of mycorrhization correlated in CO for both species and in TE only for beech.
Taken together, these results indicate differences in the recovery of the fine-root system between beech and spruce, with no clear effect of species mixture.
Dynamics of root-associated fungal communities
Sequencing of 120 rDNA samples resulted in 3,028,498 quality-filtered ITS2 sequences, which could be assigned to 2,233 OTUs and 989 taxa, whereby the 100 most abundant OTUs represented 86% of all sequences. Four samples were excluded from analysis due to their low sequencing depth (<10,000). For the 87 rRNA samples, we obtained 1,443,868 quality-filtered ITS2 sequences assigned to 2,303 OTUs and 1,002 taxa, whereby the top 100 OTUs made up 80% of all sequences. After excluding ten samples of low sequencing depth (<8,000), 77 samples remained for further analysis. Community compositions did not significantly change within 84 days after the watering event (Figure 4) (DNA: p = 0.87, R2< 0.01; RNA: p = 0.82, R2 = 0.01; PERMANOVA). Significant differences in community composition were found between treatments (DNA: p < 0.001, R2 = 0.04; RNA: p < 0.001, R2 = 0.03; PERMANOVA), plots (DNA: p < 0.001, R2 = 0.19; RNA: p < 0.001, R2 = 0.23; PERMANOVA), and RZ (DNA: p < 0.001, R2 = 0.21; RNA: p < 0.001, R2 = 0.13; PERMANOVA).
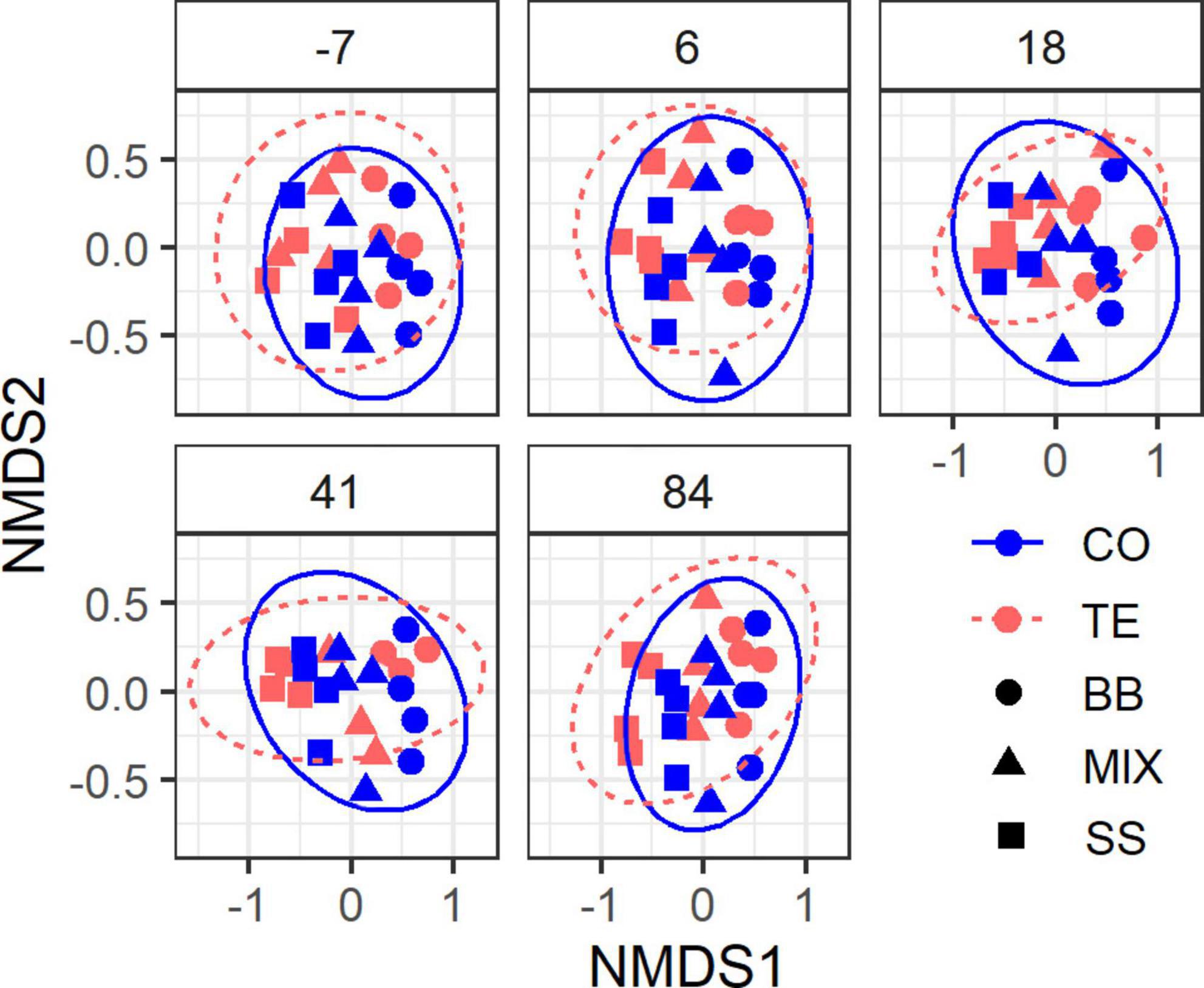
Figure 4. Non-metric multidimensional scaling (NMDS) plot of root-associated fungal community composition. Each plot shows samples from one sampling date relative to the day of rewatering in a shared coordinate system (i.e., the same ordination). Ellipses represent 95% confidence intervals. Stress 0.18.
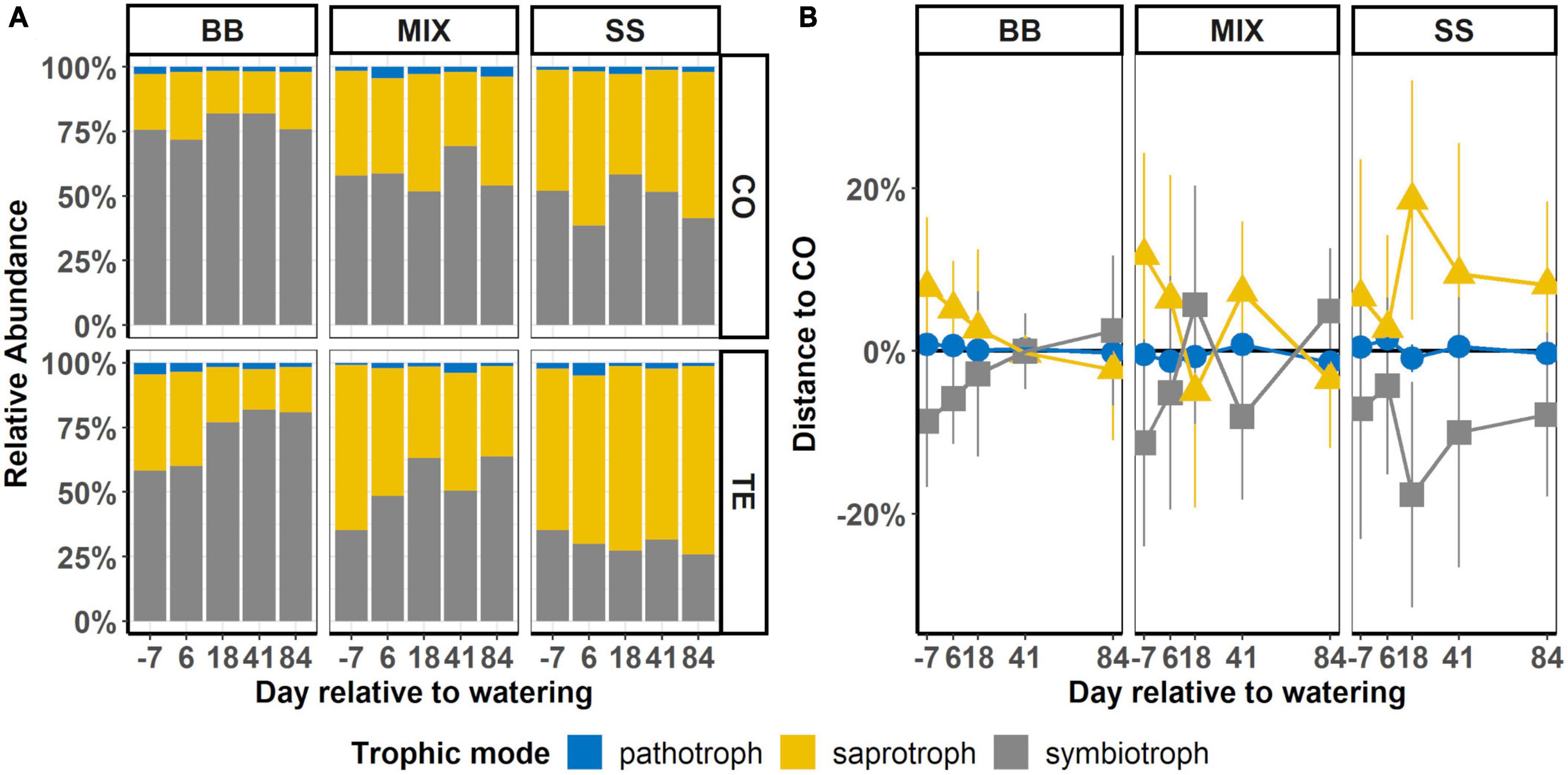
Figure 5. Time course of abundances of pathotrophic, saprotrophic, and symbiotrophic root-associated fungi of rDNA-based communities. (A) Summed-up relative abundance of trophic modes after watering in control (CO) and former drought (TE) plots in each of the three tree rooting zones: beech monospecific (BB), spruce monospecific (SS), and mixture (MIX). (B) Difference between the average abundances of trophic modes on TE and CO (TE-CO), where CO is represented by a horizontal black line at 0%. Error bars display the standard error of the difference between means.
The factor RZ had a highly significant effect on all alpha diversity metrics of root-associated fungi (Table 1). The factor treatment had significant effects on Shannon diversity and OTU richness. The factor time after watering did not significantly affect any diversity metric. Shannon diversity was generally higher in CO than in TE without differences in evenness. Shannon diversity and evenness were higher in SS than in BB. OTU richness was highest in the mixture (Table 1). Fungal diversity (Shannon, Simpson) and evenness significantly differed between all three RZs, and OTU richness differed significantly between the beech monospecific and mixture zone (Supplementary Table 4).
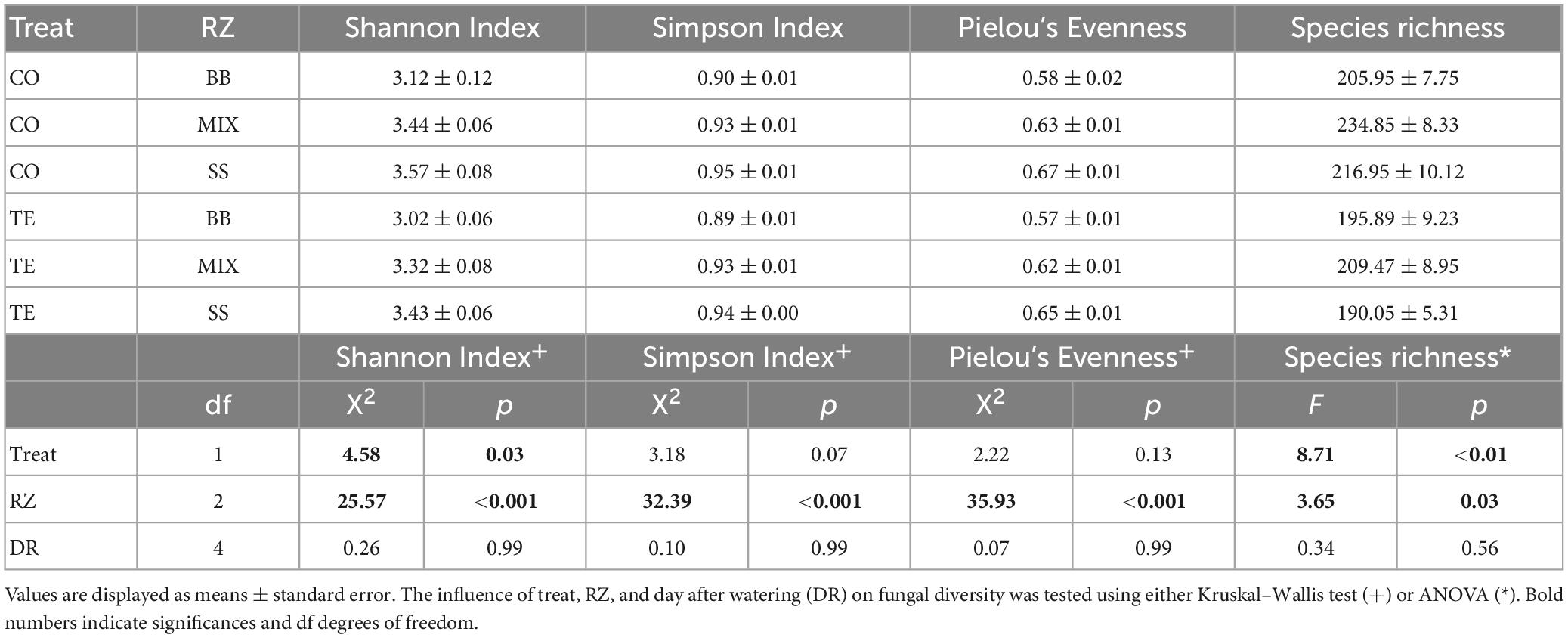
Table 1. Root-associated fungal alpha-diversity based on rDNA displayed by Shannon Index, Simpson Index, Pielou’s Evenness, and Species richness summarized by treatment (treat; CO = controls, TE = drought recovering) and tree rooting zones (RZ; BB = beech monospecific, SS = spruce monospecific, MIX = mixture).
Overall, there was no significant correlation between the growth and fine-root vitality or community composition of all the root-associated fungi or ECM fungi (Supplementary Table 5).
In summary, the fungal community composition did not significantly change after rewatering, and RZ formed fungal diversity the most.
Dynamics of functional traits of root-associated fungi
In all, 53 ± 0.9% (Supplementary Figures 3, 4) of the fungi were of unknown trophic mode irrespective of treatment and RZ and were excluded from further analysis on trophic modes. The fungal community composition according to trophic modes differed significantly between the treatments (Symbiotrophs: p < 0.001, R2 = 0.05, Saprotrophs: p < 0.001, R2 = 0.04, Pathotrophs: p < 0.001, R2 = 0.02; PERMANOVA) and tree RZ for both treatments (Table 2). The factor day after watering only had a significant effect in TE on pathotrophic fungi, which accounted only for c. 1% of the fungal community (Supplementary Figure 3).
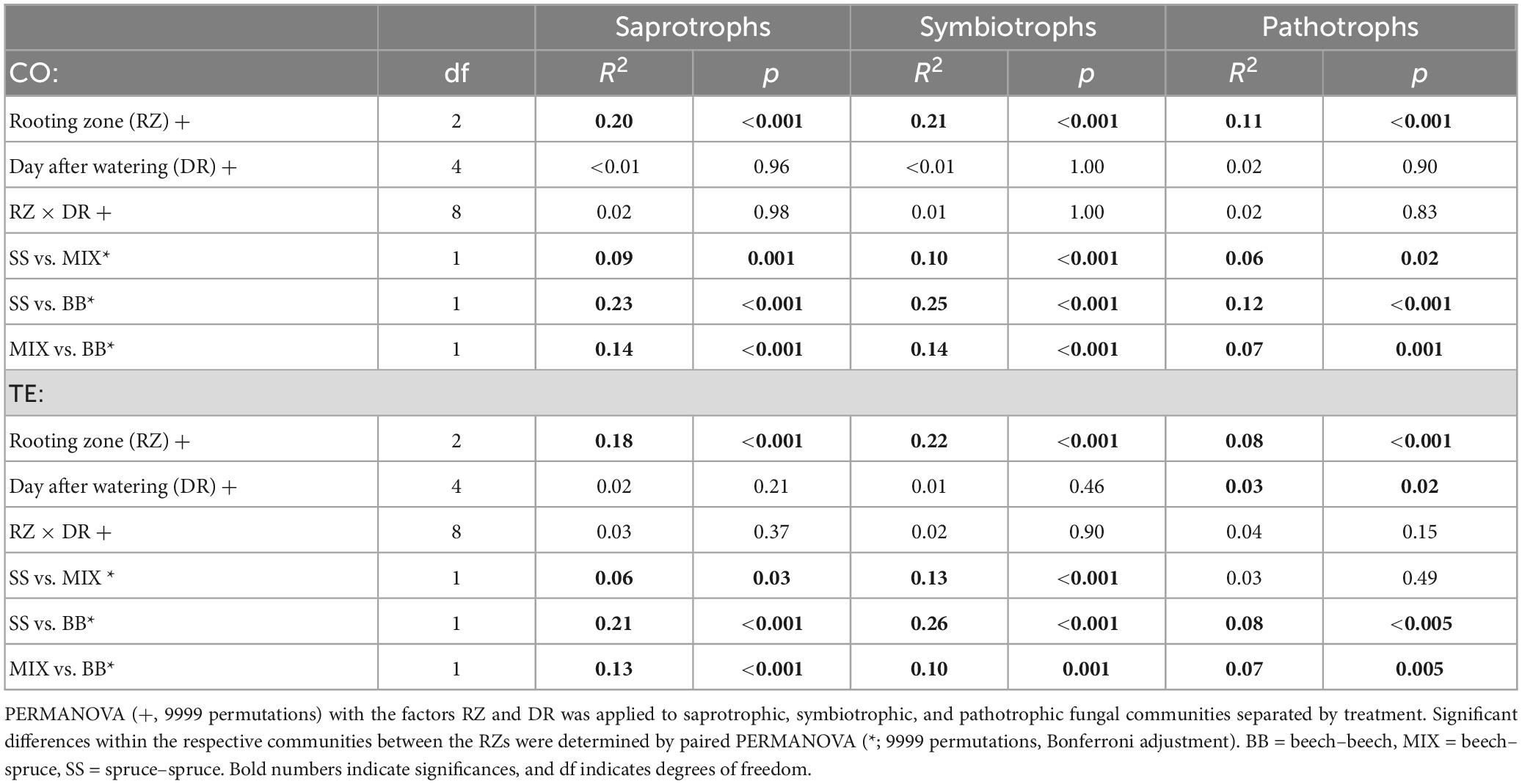
Table 2. The effect of rooting zone (RZ) and day after watering (DR) on root-associated fungal community composition based on rDNA sequences.
Within the tree RZ, relative abundances (Figure 5A) of symbiotrophic fungi were highest in BB with 77 ± 2.0% in CO and 72 ± 5.2% in TE, compared to SS with 48 ± 3.7% and 30 ± 1.6%, respectively (Figure 5A; Table 2). Accordingly, saprotrophs showed the opposite share, with lower abundances in BB and higher abundances in SS, while MIX was in between (Figure 5A; Table 2).
Temporal dynamics after rewatering showed a trend of decreasing saprotrophs in BB TE and increasing in SS CO, with MIX RZ again in between BB and SS, with a tendency to decrease in TE after watering to CO levels (Figure 5B). A similar pattern was observed in the RNA-based data (Supplementary Figure 5).
The OTU richness of saprotrophic fungi (57.1 ± 1.2) did not significantly differ between treatments and RZ. OTU richness of ECM fungi differed significantly between the treatments (p < 0.001, F = 31.7; ANOVA) and RZ (p < 0.001, F = 15.1; ANOVA), with SS being different from the other RZs (SS–BB: padj = 0.002; SS–MIX: padj < 0.001; Tukey HSD). Thus, fewer ECM OTUs were found in TE than in CO in all RZs. The highest number of OTUs appeared in MIX (average richness CO: 27.6 ± 1.4, TE: 23.2 ± 1.0), followed by BB (average richness CO: 25.2 ± 1.0, TE: 20.8 ± 0.7) and SS (average richness CO: 22.2 ± 1.0, TE: 15.5 ± 0.8).
The ten most abundant saprotrophic OTUs in BB were classified as litter saprotrophs and six with a facultative root-associated or root-endophytic lifestyle, while in SS, most saprotrophs were soil saprotrophs with facultative root-endophytic abilities (Table 3; Supplementary Table 6). Among the top 10 most abundant OTUs of saprotrophic fungi (Table 3; Supplementary Table 6), Oidiodendron sp. was overall highly abundant. Phialocephala sp. was also present in all RZs and treatments, mostly reaching between 13 and 20% of the respective top 10 OTUs. In SS TE, Acephala applanata was the second most identified saprotrophic species.
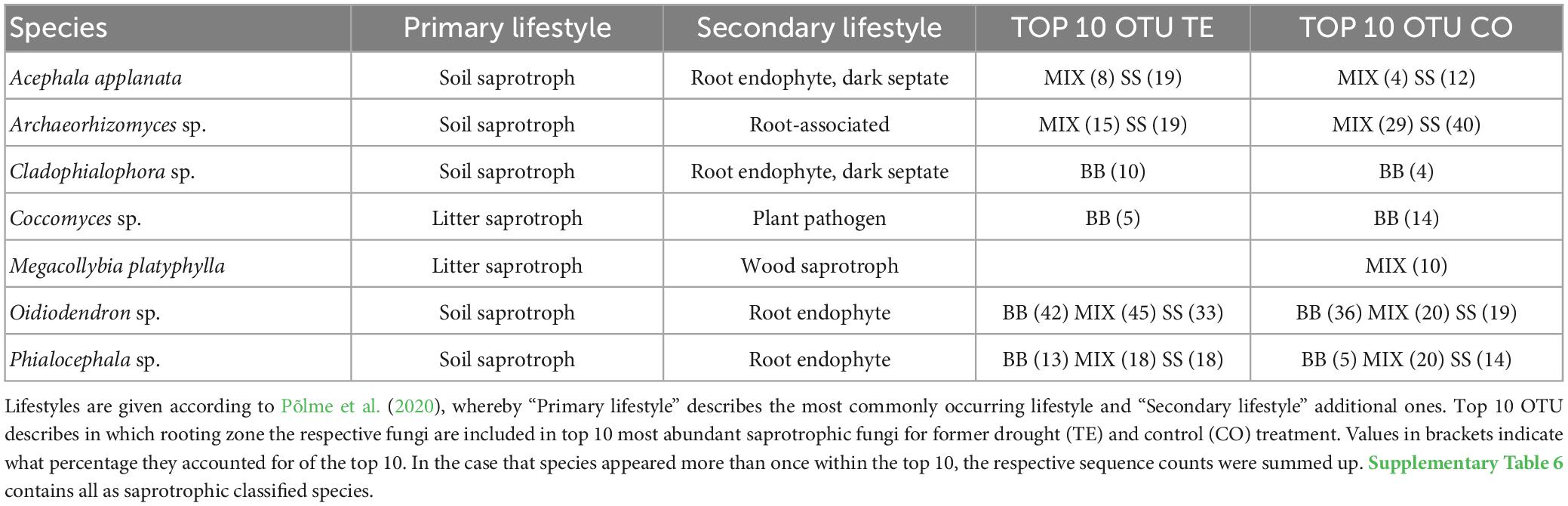
Table 3. Saprotrophic fungal species, which account for ≥10 % of the top 10 species after watering, distinguished by tree rooting zone.
Regarding pathotrophic fungi, Trichoderma sp. was by far the most abundant species in all RZs and treatments, accounting for, on average, 68% ± 4% of the top ten OTUs, mostly followed by Ilyonectria sp. (Table 4; Supplementary Table 7).
The top 10 ECM fungi (Table 5; Supplementary Table 8) varied more than saprotrophs between the RZs. While Lactarius subdulcis and Russula fellea were the most abundant species in both treatments of BB, they did not appear in SS, which was dominated by Elaphomyces granulatus and Russula sp. in both treatments. MIX contained species of BB and SS, but MIX TE was dominated by Russula sp. and Lactarius subdulcis (48%) within the top 10, whereas Lactarius sp., Piloderma sp. and Tomentella botryoides dominated MIX CO (21%, 12%, 11%).
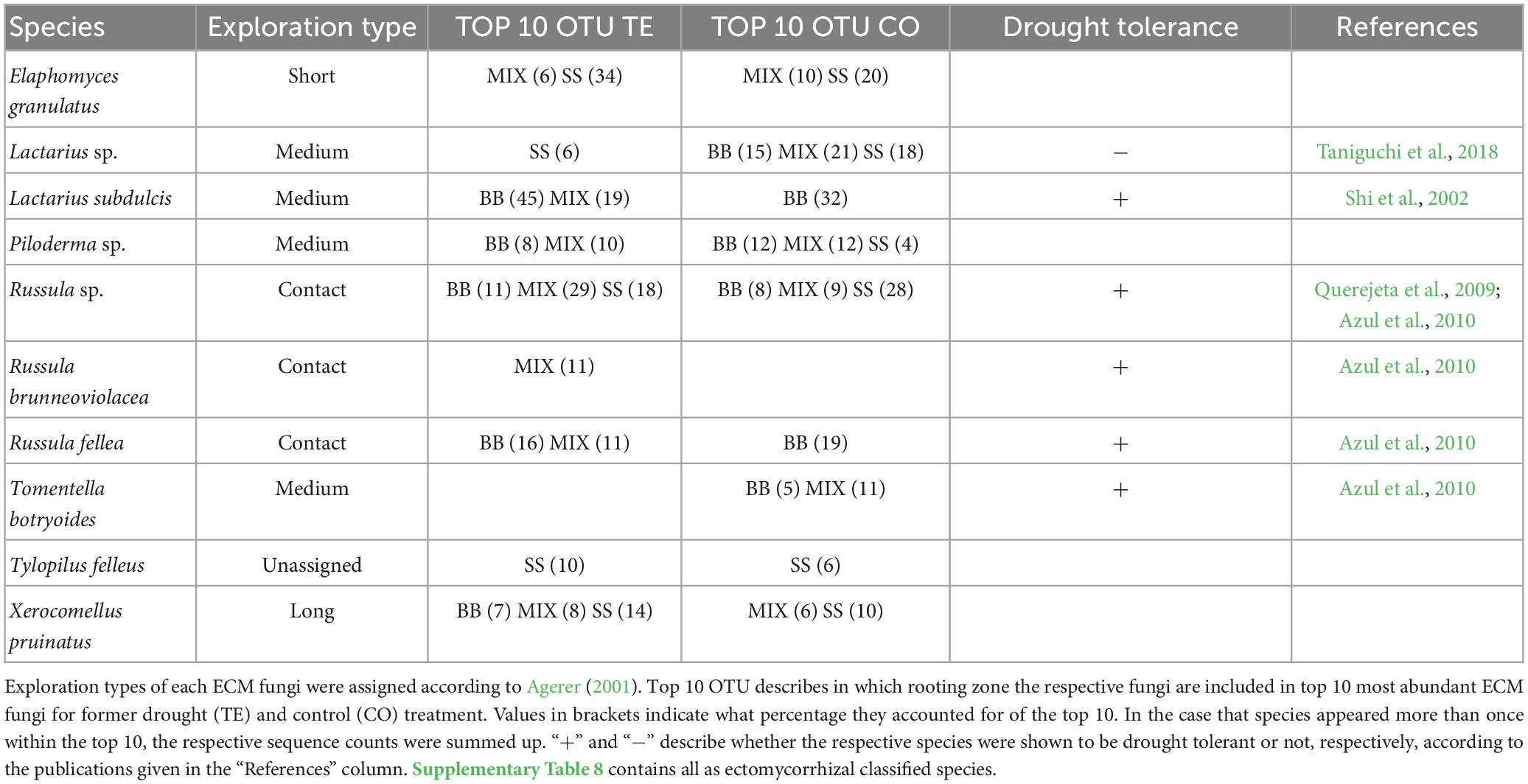
Table 5. ECM fungal species, which account for ≥10% of the top 10 species after watering, distinguished by tree rooting zone.
Regarding ECM exploration types (Table 5; Supplementary Table 8; Supplementary Figure 6A), BB CO was dominated by medium-distance types at all time points (averaged over all sampling times: 56.6 ± 2.7%), while in TE, the abundance of both contact and medium-distance types was around 43 % at all time points. In SS roots, differences between CO and TE were more distinct. While in CO the abundances of short-distance, medium-distance, and contact types were evenly distributed with minor changes over time, contact types were the most dominant exploration type in TE before and shortly after watering (53 ± 0.6%) but tended to decrease after drought release (24 ± 0.9%) in favor of short- and long-distance types, with the latter even exceeding CO levels at later sampling dates (Supplementary Figure 6A). In mixture, contact types were most abundant in TE at all time points (60 ± 4.2%), while it was medium-distance types in CO. The abundance patterns of BB CO in RNA strongly resembled DNA communities, with medium-distance types being dominant in CO at all time points (≥50%), while contact types were more abundant (51 ± 3%) in TE (Supplementary Figure 6B).
In summary, the abundance of fungal species with different fungal traits differed between the RZs, with drought-tolerating ECM fungal species of contact and medium-distance exploration types being more abundant in BB and saprotrophic fungi. with an additional root-associated lifestyle being more abundant in SS.
Discussion
Drought and recovery reactions of the fine-root system are tree species specific
Spruce fine roots formed before drought release (represented by mesh bag roots) showed positive growth and an increasing vitality after rewatering, which indicates a reactivation of dormant fine roots. Beech fine roots enclosed in mesh bags, however, did not grow after rewatering, and their vitality was almost without exceptions below the controls. Nevertheless, a consistent mixture effect could not be determined.
Fine-root biomass in the RZ did not significantly differ between the treatments in both species shortly before watering, similar to complementary results on fine-root surface area on the same experimental site (Brunn et al., 2022). Already within the first week after watering, fine-root biomass in all three tree RZs of both treatments increased, which could indicate a fast response of the fine-root growth of both species and corresponds to findings that trees may respond even to very small changes in soil water content (Joseph et al., 2020).
The vitality of TE spruce roots in mesh bags increased within days after watering and reached a growth rate similar to those of CO, indicating a fast reactivation of existing dormant fine roots after drought, very similar to Scots pine (Joseph et al., 2020). Nevertheless, those reactivated TE fine roots were more prone to death than CO roots, which is in line with earlier findings of increased fine-root mortality after drought release (Gaul et al., 2008). In contrast, beech fine roots in mesh bags did not show any growth after watering and only a short peak in vitality in early autumn (d 35 and d 41), a season where the fine-root length tends to increase in beech (Montagnoli et al., 2014). While the results from the beech fine roots within mesh bags suggest that existing fine roots were not reactivated after rewatering, increasing the fine-root biomass of beech outside the mesh bags suggested that beech trees rapidly formed new fine roots. A likely explanation is the short life span of beech fine roots and their high C turnover (Nikolova et al., 2020; Mariën et al., 2021).
The different fine-root recovery patterns in spruce and beech complement the findings of fine-root preservation in spruce and the continuous renewal of low-biomass short life span fine roots in beech during drought (Meier and Leuschner, 2008; Nikolova et al., 2020). For both tree species, the degree of mycorrhization in TE was below CO during the whole period, which corresponds to the findings of Feil et al. (1988), who showed a decreased growth of mycorrhizae in mature spruce during drought periods. In beech, we observed a parallel increase of vitality and degree of mycorrhization after drought, implying that ECM fungi, to some degree, depended more on host root vitality than on abiotic environmental factors. This was also suggested by Shi et al. (2002), who observed that neither the number of ECM types on young beech roots nor the degree of fungal colonization of the roots were affected by a drought treatment. In spruce, though, the degree of mycorrhization decreased after watering and rose 4 weeks later, suggesting that suberisation of fine roots during drought (Nikolova et al., 2020) could contribute to preventing ECM colonization (e.g., Brundrett, 2002; Sharda and Koide, 2008). Consequently, outgrowing fine roots needed to be colonized anew. Reflecting this and the time of full mycorrhizae formation (Ineichen and Wiemken, 1992), we saw increasing mycorrhization from d 28 onward. The sudden and strong variations in the degree of mycorrhization confirmed earlier observed patterns caused by changes in soil temperature and soil moisture (Swaty et al., 1998).
During our 3-month measuring period, only few significant differences were observed with respect to fine-root growth and vitality between monospecific and mixture zones. However, on specific days, growth and vitality were significantly higher in Bmix compared to BB, corresponding to the findings of Zwetsloot et al. (2019) that beech fine roots had lower mortality when growing in mixture zones with spruce. In spruce, growth and vitality were lower in Smix than in SS on some days, possibly reflecting the lower competitive ability of spruce fine roots in mixed stands with beech (Schmid, 2002; Zwetsloot et al., 2019).
Lasting effects of precedent drought and tree rooting zone on fungal communities after drought release
We found a high agreement between DNA and RNA data regarding the fungal trophic modes, indicating a good representation of the active fungal community by the DNA data and a minor role of inactive fungi and the spores and residues of dead fungi (Pedersen et al., 2015; Carini et al., 2016). According to our data, the root-associated community composition remained stable within 3 months after drought release but differed between the tree species, with ECM fungi being more abundant in beech and saprotrophs in spruce. A higher species richness and increase of ECM fungi after drought indicate a positive effect of tree species mixture. Consequently, H1 can be partially confirmed, which means that the structure of the fungal community after rewatering was lastingly influenced by the previous drought, but its composition highly depends on the development of tree-specific root systems and habitat heterogeneity. A high number of the most abundantly observed ECM fungal species are considered drought resistant, and most saprotrophic species have a facultative root-associated lifestyle, thus confirming H2.
As expected from a previous study on ECM diversity on the KROOF site, the diversity of root-associated fungi was lower in TE than in CO (Nickel et al., 2018). However, alpha diversity and community composition did not change significantly for both tree species within 3 months after drought release. This either indicates that a stable community had established on the treatment plots during drought, which tolerated the changes in soil moisture, or that the drought-surviving fungal communities could not profit from the moisture increase. The majority of the highly abundant fungal species in this study are considered drought tolerant (Table 5; Supplementary Table 8) or have a facultative root-associated lifestyle (Table 3; Supplementary Table 6) that may favor drought survival by easier water access (Querejeta et al., 2003; Hafner et al., 2017). This suggests the emergence of a stable community during drought. Those adapted fungi were closest to newly grown fine roots after rewetting and thus likely the first colonizers (Bruns, 1995), which could explain an unchanged fungal community after drought release.
The tree species RZ was a major driver of the fungal community composition during drought (Nickel et al., 2018) and after watering (our study), which underlines the major influence of tree species on fungal community composition found in many studies (Dickie, 2007; Ishida et al., 2007; Tedersoo et al., 2012; Bogar and Kennedy, 2013; Nacke et al., 2016; Otsing et al., 2021). Furthermore, we observed a higher fungal diversity on spruce compared to beech fine roots irrespective of treatment, which may reflect the higher fungal species richness in the litter layer of spruce compared to beech (Asplund et al., 2019).
In beech, ECM fungi were the most abundant fungal trophic modes in both treatments over time and irrespective of RZ. This suggests that the ECM fungi of beech were better retained through the continuous root growth found in beech under drought as compared to Norway spruce (Nikolova et al., 2020). Moreover, the abundance of ECM fungi increased in mixture after watering but not in the TE SS RZ. Therefore, spruce ECM in mixture may benefit from the continuous renewal of beech fine roots as a more favorable niche to colonize than suberised spruce fine roots, possibly being one aspect of why ECM fungal community compositions of spruce were influenced by neighboring tree species in mixture in other studies, too (Hubert and Gehring, 2008; Otsing et al., 2021). While giving support to the majority of studies that found a positive tree mixture effect on ECM diversity (Gao et al., 2013; Tedersoo et al., 2016), our study adds the aspect of a positive effect of mixtures on fungal diversity under drought. Addressing H1, we further looked at ECM exploration types and found ECM species with contact exploration type were more common in TE compared to CO. Additionally, in spruce, relative abundances shifted with time after watering from dominating contact types to more short- and long-distance types. ECM fungi with rhizomorphs that support water uptake (Duddridge et al., 1980; Cairney, 1992) increased during the first 3 years of experimental drought (Nickel et al., 2018). However, there may be a trade-off between water uptake and carbon costs in which, with the duration of drought, low-cost contact types may become dominant (Castaño et al., 2018). Recently, shifts in ECM exploration types were proposed to rather reflect the fungal C supply by the host (Wasyliw and Karst, 2020). With drought release, spruce in our experiment preferably invested C in fine-root growth within the first month (Hikino et al., 2022), possibly to restore the functions essential for resource uptake (Bardgett et al., 2014; Solly et al., 2018; Germon et al., 2020), which was not reflected in a higher C sink activity of ectomycorrhizae during this time (Hikino et al., 2022). In beech, the photosynthesis rate was constantly higher during drought and after watering compared to spruce (Goisser et al., 2016), which suggests a better supply of photoassimilates to beech-associated ECM fungi during drought, allowing the maintenance of C-costly medium-distance exploration types (Weigt et al., 2012).
The relatively high shares of saprotrophs in the TE SS RZ remained unchanged over the 3 months. Spruce fine roots become suberised during drought (Nikolova et al., 2020), creating a barrier for ectomycorrhizal colonization (e.g., Brundrett, 2002; Sharda and Koide, 2008), and in addition, a severe root dieback (Nickel et al., 2018) may have increased root necromass. The majority of saprotrophic fungal species found in spruce roots within this study, including the highly abundant Oidiodendron sp. and Phialocephala sp., were found to have the ability to associate with roots, which corroborates recent studies showing that fungi can occupy multiple ecological niches (Selosse et al., 2018). Thus, some saprotrophic fungi may reveal a facultative root-endophytic lifestyle (Tedersoo and Smith, 2013; Smith et al., 2017) and even show mycorrhizal traits (Jumpponen, 2001; Kohler et al., 2015; Almario et al., 2017). Dark septate endophytes, for example, are shown to better tolerate environmental stresses such as drought (Berthelot et al., 2019), which is why an increased abundance of saprotrophic species with root-associated lifestyles could be an indication of adaptation to a changing climate (Ruotsalainen et al., 2022). Furthermore, the stable shift toward the predominance of drought-resistant ECM species during the 5 years of summer drought, together with a good recovery of the tree fine-root and carbon transport system (Hikino et al., 2021, 2022), may reflect ectomycorrhiza-assisted plant adaptation to drought, as suggested by Cosme (2023). A high litter accumulation in our TE plots (pers. observation), together with a more humid surrounding due to hydraulic redistribution in beech during drought (Querejeta et al., 2003; Hafner et al., 2017), may explain the detection of several litter-degrading saprotrophs (e.g., different species of Hyaloscypha and Mycena) among the most abundant saprotrophs in BB and MIX RZ. Unlike ECM and saprotrophic fungi, pathotrophs accounted for only a small percentage, highly dominated by Trichoderma sp. This genus comprised mycoparasites (Harman, 2006 and literature therein) but also saprotrophic species that produce a plethora of phytohormones, increasing plant and even ECM hyphal growth (Harman et al., 2004; Shoresh et al., 2010; Oh et al., 2018). Thus, the root-associated unclassified fungal species await further, more detailed studies.
Conclusion
The present study gives insights into the drought recovery of root-associated fungal communities and the fine-root systems of beech and spruce, suggesting that the composition of root fungal communities is strongly influenced by tree species-specific drought reactions and the restoration of their fine-root systems upon drought release. A positive tree mixture effect of increased total fungal OTU richness underlines the role of diversification in forests from a belowground perspective. Moreover, a comparison between fungi identified from soil and roots also provides further evidence that an association between saprotrophic fungi and roots may not be an exception but rather a survival strategy of the fungi. However, this study only considered a period of 3 months. Thus, the resilience of fungal communities and fine-root systems needs to be explored at a longer timescale to clarify whether the whole forest system retains its full functional capacity in terms of root recovery, tree growth, root-associated and soil fungi, and other soil biota.
Data availability statement
The datasets presented in this study can be found in online repositories. The names of the repository/repositories and accession number(s) can be found below: https://www.ncbi.nlm.nih.gov/, PRJNA950082.
Author contributions
FW and KP designed the experiment. JD and RW processed the samples and analyzed and interpreted the data with support from FW. JM provided and compiled data for soil fungi from a parallel study. JD wrote the manuscript with contributions from all the authors. All authors contributed to the article and approved the submitted version.
Funding
This project was funded by the German Research Foundation (DFG) through grants GR 1881/5-1, MA1763/10-1, PR555/2-1, and PR292/22-1 and by the Bavarian State Ministries of the Environment and Consumer Protection and of Food, Agriculture and Forestry (W047/Kroof II). JM was founded by a scholarship from the Deutscher Akademischer Austauschdienst (DAAD) Grant No. 57507437. Open access publication was supported by the Technical University of Munich (TUM) Publishing Fund.
Acknowledgments
We thank our ecology volunteers Valentin Kugler and Sarah Kristen, student helper Laura Pohlenz, and apprentice Isabella Pitzen for their help during the mesh bag preparation, filling, and harvest, as well as their support in the root length analysis. We also thank Susanne Kublik for sequencing, Patrick and Christian Schwaferts for their statistical advice and discussion, Elke Gerstner and Barbara Gross for their reliable lab supply during the COVID pandemic and helping hand for the mesh bag preparation, and Dr. Hans Sanden for taking the time to read this manuscript and providing helpful comments to improve it.
Conflict of interest
The authors declare that the research was conducted in the absence of any commercial or financial relationships that could be construed as a potential conflict of interest.
Publisher’s note
All claims expressed in this article are solely those of the authors and do not necessarily represent those of their affiliated organizations, or those of the publisher, the editors and the reviewers. Any product that may be evaluated in this article, or claim that may be made by its manufacturer, is not guaranteed or endorsed by the publisher.
Supplementary material
The Supplementary Material for this article can be found online at: https://www.frontiersin.org/articles/10.3389/ffgc.2023.1197791/full#supplementary-material
References
Abarenkov, K., Zirk, A., Piirmann, T., Pöhönen, R., Ivanov, F., Nilsson, R. H., et al. (2020). Full UNITE+INSD dataset for Fungi. Version 04.02.2020. Tartu: University of Tartu. doi: 10.15156/BIO/786372
Agerer, R. (2001). Exploration types of ectomycorrhizae. Mycorrhiza 11, 107–114. doi: 10.1007/s005720100108
Allen, C. D., Breshears, D. D., and McDowell, N. G. (2015). On underestimation of global vulnerability to tree mortality and forest die-off from hotter drought in the anthropocene. Ecosphere 6, 1–55. doi: 10.1890/ES15-00203.1
Allen, C. D., Macalady, A. K., Chenchouni, H., Bachelet, D., McDowell, N., Vennetier, M., et al. (2010). A global overview of drought and heat-induced tree mortality reveals emerging climate change risks for forests. For. Ecol. Manag. 259, 660–684. doi: 10.1016/j.foreco.2009.09.001
Almario, J., Jeena, G., Wunder, J., Langen, G., Zuccaro, A., Coupland, G., et al. (2017). Root-associated fungal microbiota of nonmycorrhizal Arabis alpina and its contribution to plant phosphorus nutrition. Proc. Natl. Acad. Sci. U.S.A. 114, E9403–E9412. doi: 10.1073/pnas.1710455114
Anderegg, W. R. L., Kane, J. M., and Anderegg, L. D. L. (2013). Consequences of widespread tree mortality triggered by drought and temperature stress. Nat. Clim. Change 3, 30–36. doi: 10.1038/nclimate1635
Aponte, C., García, L. V., Marañón, T., and Gardes, M. (2010). Indirect host effect on ectomycorrhizal fungi: Leaf fall and litter quality explain changes in fungal communities on the roots of co-occurring Mediterranean oaks. Soil Biol. Biochem. 42, 788–796. doi: 10.1016/j.soilbio.2010.01.014
Asplund, J., Kauserud, H., Ohlson, M., and Nybakken, L. (2019). Spruce and beech as local determinants of forest fungal community structure in litter, humus and mineral soil. FEMS Microbiol. Ecol. 95, 1–11. doi: 10.1093/femsec/fiy232
Azul, A. M., Sousa, J. P., Agerer, R., Martín, M. P., and Freitas, H. (2010). Land use practices and ectomycorrhizal fungal communities from oak woodlands dominated by Quercus suber L. considering drought scenarios. Mycorrhiza 20, 73–88. doi: 10.1007/s00572-009-0261-2
Bardgett, R. D., Mommer, L., and De Vries, F. T. (2014). Going underground: Root traits as drivers of ecosystem processes. Trends Ecol. Evol. 29, 692–699. doi: 10.1016/j.tree.2014.10.006
Bengtsson-Palme, J., Ryberg, M., Hartmann, M., Branco, S., Wang, Z., Godhe, A., et al. (2013). Improved software detection and extraction of ITS1 and ITS2 from ribosomal ITS sequences of fungi and other Eukaryotes for analysis of environmental sequencing data. Methods Ecol. Evol. 4, 914–919. doi: 10.1111/2041-210X.12073
Bennett, A. E., and Classen, A. T. (2020). Climate change influences mycorrhizal fungal–plant interactions, but conclusions are limited by geographical study bias. Ecology 101:e02978. doi: 10.1002/ecy.2978
Berthelot, C., Chalot, M., Leyval, C., and Blaudez, D. (2019). “From darkness to light: Emergence of the mysterious dark septate Endophytes in plant growth promotion and stress alleviation,” in Endophytes for a growing world, eds T. Hodkinson, F. Doohan, M. Saunders, and B. Murphy (Cambridge: Cambridge University Press), 143–164. doi: 10.1017/9781108607667.008
Bogar, L. M., and Kennedy, P. G. (2013). New wrinkles in an old paradigm: Neighborhood effects can modify the structure and specificity of Alnus-associated ectomycorrhizal fungal communities. FEMS Microbiol. Ecol. 83, 767–777. doi: 10.1111/1574-6941.12032
Brundrett, M. C. (2002). Coevolution of roots and mycorrhizas of land plants. New Phytol. 154, 275–304. doi: 10.1046/j.1469-8137.2002.00397.x
Brunn, M., Hafner, B. D., Zwetsloot, M. J., Weikl, F., Pritsch, K., Hikino, K., et al. (2022). Carbon allocation to root exudates is maintained in mature temperate tree species under drought. New Phytol. 235, 965–977. doi: 10.1111/nph.18157
Brunner, I., Herzog, C., Dawes, M. A., Arend, M., and Sperisen, C. (2015). How tree roots respond to drought. Front. Plant Sci. 6:547. doi: 10.3389/fpls.2015.00547
Bruns, T. D. (1995). Thoughts on the processes that maintain local species diversity of ectomycorrhizal fungi. Plant Soil 170, 63–73. doi: 10.1007/BF02183055
Cairney, J. W. G. (1992). Translocation of solutes in ectomycorrhizal and saprotrophic rhizomorphs. Mycol. Res. 96, 135–141. doi: 10.1016/S0953-7562(09)80928-3
Carini, P., Marsden, P. J., Leff, J. W., Morgan, E. E., Strickland, M. S., and Fierer, N. (2016). Relic DNA is abundant in soil and obscures estimates of soil microbial diversity. Nat. Microbiol. 2:16242. doi: 10.1038/nmicrobiol.2016.242
Castaño, C., Lindahl, B. D., Alday, J. G., Hagenbo, A., Martínez de Aragón, J., Parladé, J., et al. (2018). Soil microclimate changes affect soil fungal communities in a mediterranean pine forest. New Phytol. 220, 1211–1221. doi: 10.1111/nph.15205
Chen, J., Bittinger, K., Charlson, E. S., Hoffmann, C., Lewis, J., Wu, G. D., et al. (2012). Associating microbiome composition with environmental covariates using generalized UniFrac distances. Bioinformatics 28, 2106–2113. doi: 10.1093/bioinformatics/bts342
Conn, C., and Dighton, J. (2000). Litter quality influences on decomposition, ectomycorrhizal community structure and mycorrhizal root surface acid phosphatase activity. Soil Biol. Biochem. 32, 489–496. doi: 10.1016/S0038-0717(99)00178-9
Cosme, M. (2023). Mycorrhizas drive the evolution of plant adaptation to drought. Commun. Biol. 6:346. doi: 10.1038/s42003-023-04722-4
Dhiman, I., Bilheux, H., DeCarlo, K., Painter, S. L., Santodonato, L., and Warren, J. M. (2018). Quantifying root water extraction after drought recovery using sub-mm in situ empirical data. Plant Soil 424, 73–89. doi: 10.1007/s11104-017-3408-5
Dickie, I. A. (2007). Host preference, niches and fungal diversity. New Phytol. 174, 230–233. doi: 10.1111/j.1469-8137.2007.02055.x
Duddridge, J. A., Malibari, A., and Read, D. J. (1980). Structure and function of mycorrhizal rhizomorphs with special reference to their role in water transport. Nature 287, 834–836. doi: 10.1038/287834a0
Feil, W., Kottke, I., and Oberwinkler, F. (1988). The effect of drought on mycorrhizal production and very fine root system development of norway spruce under natural and experimental conditions. Plant Soil 108, 221–231. doi: 10.1007/BF02375652
Forrester, D. I. (2015). Transpiration and water-use efficiency in mixed-species forests versus monocultures: Effects of tree size, stand density and season. Tree Physiol. 35, 289–304. doi: 10.1093/treephys/tpv011
Foster, G. C., Lane, D., Scott, D., Hebl, M., Guerra, R., Osherson, D., et al. (2018). An introduction to psychological statistics. St. Louis, MO: University of Missouri.
Gao, C., Shi, N. N., Liu, Y. X., Peay, K. G., Zheng, Y., Ding, Q., et al. (2013). Host plant genus-level diversity is the best predictor of ectomycorrhizal fungal diversity in a chinese subtropical forest. Mol. Ecol. 22, 3403–3414. doi: 10.1111/mec.12297
Gaul, D., Hertel, D., Borken, W., Matzner, E., and Leuschner, C. (2008). Effects of experimental drought on the fine root system of mature norway spruce. For. Ecol. Manag. 256, 1151–1159. doi: 10.1016/j.foreco.2008.06.016
Gehring, C., Sevanto, S., Patterson, A., Ulrich, D. E. M., and Kuske, C. R. (2020). Ectomycorrhizal and dark septate fungal associations of Pinyon pine are differentially affected by experimental drought and warming. Front. Plant Sci. 11:582574. doi: 10.3389/fpls.2020.582574
Germon, A., Laclau, J. P., Robin, A., and Jourdan, C. (2020). Tamm review: Deep fine roots in forest ecosystems: Why dig deeper?. For. Ecol. Manag. 466:118135. doi: 10.1016/j.foreco.2020.118135
Goisser, M., Geppert, U., Rötzer, T., Paya, A., Huber, A., Kerner, R., et al. (2016). Does belowground interaction with Fagus sylvatica increase drought susceptibility of photosynthesis and stem growth in Picea abies?. For. Ecol. Manag. 375, 268–278. doi: 10.1016/j.foreco.2016.05.032
González de Andrés, E., Camarero, J. J., Blanco, J. A., Imbert, J. B., Lo, Y. H., Sangüesa-Barreda, G., et al. (2018). Tree-to-tree competition in mixed european beech–scots pine forests has different impacts on growth and water-use efficiency depending on site conditions. J. Ecol. 106, 59–75. doi: 10.1111/1365-2745.12813
Grams, T. E. E., Hesse, B. D., Gebhardt, T., Weikl, F., Rötzer, T., Kovacs, B., et al. (2021). The Kroof experiment: Realization and efficacy of a recurrent drought experiment plus recovery in a beech/spruce forest. Ecosphere 12:e03399. doi: 10.1002/ecs2.3399
Grossiord, C., Granier, A., Gessler, A., Jucker, T., and Bonal, D. (2014). Does drought influence the relationship between biodiversity and ecosystem functioning in boreal forests?. Ecosystems 17, 394–404. doi: 10.1007/s10021-013-9729-1
Gweon, H. S., Oliver, A., Taylor, J., Booth, T., Gibbs, M., Read, D. S., et al. (2015). PIPITS: An automated pipeline for analyses of fungal internal transcribed spacer sequences from the illumina sequencing platform. Methods Ecol. Evol. 6, 973–980. doi: 10.1111/2041-210X.12399
Hafner, B. D., Tomasella, M., Häberle, K. H., Goebel, M., Matyssek, R., and Grams, T. E. E. (2017). Hydraulic redistribution under moderate drought among English Oak, European beech and Norway spruce determined by deuterium isotope labeling in a split-root experiment. Tree Physiol. 37, 950–960. doi: 10.1093/treephys/tpx050
Hagedorn, F., Joseph, J., Peter, M., Luster, J., Pritsch, K., Geppert, U., et al. (2016). Recovery of trees from drought depends on belowground sink control. Nat. Plants 2:16111. doi: 10.1038/nplants.2016.111
Harman, G. E. (2006). Overview of mechanisms and uses of Trichoderma Spp. Phytopathology 96, 190–194. doi: 10.1094/PHYTO-96-0190
Harman, G. E., Howell, C. R., Viterbo, A., Chet, I., and Lorito, M. (2004). Trichoderma species–opportunistic, avirulent plant symbionts. Nat. Rev. Microbiol. 2, 43–56. doi: 10.1038/nrmicro797
Hikino, K., Danzberger, J., Riedel, V. P., Hesse, B. D., Hafner, B. D., Gebhardt, T., et al. (2022). Dynamics of initial C allocation after drought release in mature norway spruce - increased belowground allocation of current Photoassimilates covers only half of the C used for fine-root growth. Glob. Chang. Biol. 28, 6889–6905. doi: 10.1111/gcb.16388
Hikino, K., Danzberger, J., Riedel, V. P., Rehschuh, R., Ruehr, N. K., Hesse, B. D., et al. (2021). High resilience of carbon transport in long-term drought-stressed mature Norway spruce trees within 2 weeks after drought release. Glob. Change Biol. 28, 2095–2110. doi: 10.1111/gcb.16051
Hubert, N. A., and Gehring, C. A. (2008). Neighboring trees affect ectomycorrhizal fungal community composition in a woodland-forest ecotone. Mycorrhiza 18, 363–374. doi: 10.1007/s00572-008-0185-2
Ineichen, K., and Wiemken, V. (1992). Changes in the fungus-specific, soluble-carbohydrate pool during rapid and synchronous ectomycorrhiza formation of Picea abies with Pisolithus tinctorius. Mycorrhiza 2, 1–7.
IPCC (2021). Climate change 2021 The physical science basis summary for policymakers working group I contribution to the sixth assessment report of the intergovernmental panel on climate change. Climate change 2021: The physical science basis. Geneva: IPCC.
Ishida, T. A., Nara, K., and Hogetsu, T. (2007). Host effects on ectomycorrhizal fungal communities: Insight from eight host species in mixed conifer-broadleaf forests. New Phytol. 174, 430–440. doi: 10.1111/j.1469-8137.2007.02016.x
Joseph, J., Gao, D., Backes, B., Bloch, C., Brunner, I., Gleixner, G., et al. (2020). Rhizosphere activity in an old-growth forest reacts rapidly to changes in soil moisture and shapes whole-tree carbon allocation. Proc. Natl. Acad. Sci. U.S.A. 117, 24885–24892. doi: 10.1073/pnas.2014084117
Jumpponen, A. (2001). Dark septate endophytes–are they mycorrhizal?. Mycorrhiza 11, 207–211. doi: 10.1007/s005720100112
Kohler, A., Kuo, A., Nagy, L. G., Morin, E., Barry, K. W., Buscot, F., et al. (2015). Convergent losses of decay mechanisms and rapid turnover of symbiosis genes in mycorrhizal mutualists. Nat. Genet. 47, 410–415. doi: 10.1038/ng.3223
Köhler, J., Yang, N., Pena, R., Raghavan, V., Polle, A., and Meier, I. C. (2018). Ectomycorrhizal fungal diversity increases phosphorus uptake efficiency of European beech. New Phytol. 220, 1200–1210. doi: 10.1111/nph.15208
Kõljalg, U., Nilsson, R. H., Abarenkov, K., Tedersoo, L., Taylor, A. F. S., Bahram, M., et al. (2013). Towards a unified paradigm for sequence-based identification of fungi. Mol. Ecol. 22, 5271–5277. doi: 10.1111/mec.12481
Körner, C. (2019). No need for pipes when the well is dry—A comment on hydraulic failure in trees. Tree Physiol. 39, 695–700. doi: 10.1093/treephys/tpz030
Kottke, I., and Oberwinkler, F. (1986). Mycorrhiza of forest trees–structure and function. Trees 1, 1–24. doi: 10.1007/BF00197021
Lehto, T., and Zwiazek, J. J. (2011). Ectomycorrhizas and water relations of trees: A review. Mycorrhiza 21, 71–90. doi: 10.1007/s00572-010-0348-9
Mariën, B., Ostonen, I., Penanhoat, A., Fang, C., Nguyen, H. X., Ghisi, T., et al. (2021). On the below-and aboveground phenology in deciduous trees: Observing the fine-root lifespan, turnover rate, and phenology of Fagus sylvatica L., Quercus robur L., and Betula pendula roth for two growing seasons. Forests 12, 1–27. doi: 10.3390/f12121680
Martinez Arbizu, P. (2020). pairwiseAdonis: Pairwise multilevel comparison using adonis. R package version 0.4.
Maurel, C., Boursiac, Y., Luu, D. T., Santoni, V., Shahzad, Z., and Verdoucq, L. (2015). Aquaporins in plants. Physiol. Rev. 95, 1321–1358. doi: 10.1152/physrev.00008.2015
McDowell, N., Pockman, W. T., Allen, C. D., Breshears, D. D., Cobb, N., Kolb, T., et al. (2008). Mechanisms of plant survival and mortality during drought: Why do some plants survive while others succumb to drought?. New Phytol. 178, 719–739. doi: 10.1111/j.1469-8137.2008.02436.x
McMurdie, P. J., and Holmes, S. (2013). Phyloseq: An R package for reproducible interactive analysis and graphics of microbiome census data. PLoS One 8:e61217. doi: 10.1371/journal.pone.0061217
Meier, I. C., and Leuschner, C. (2008). Belowground drought response of European beech: Fine root biomass and carbon partitioning in 14 mature stands across a precipitation gradient. Glob. Change Biol. 14, 2081–2095. doi: 10.1111/j.1365-2486.2008.01634.x
Mohan, J. E., Cowden, C. C., Baas, P., Dawadi, A., Frankson, P. T., Helmick, K., et al. (2014). Mycorrhizal fungi mediation of terrestrial ecosystem responses to global change: Mini-review. Fungal Ecol. 10, 3–19. doi: 10.1016/j.funeco.2014.01.005
Montagnoli, A., Di Iorio, A., Terzaghi, M., Trupiano, D., Scippa, G. S., and Chiatante, D. (2014). Influence of soil temperature and water content on fine-root seasonal growth of european beech natural forest in Southern Alps, Italy. Eur. J. For. Res. 133, 957–968. doi: 10.1007/s10342-014-0814-6
Nacke, H., Goldmann, K., Schöning, I., Pfeiffer, B., Kaiser, K., Villamizar, G. A. C., et al. (2016). Fine spatial scale variation of soil microbial communities under european beech and Norway Spruce. Front. Microbiol. 7:2067. doi: 10.3389/fmicb.2016.02067
Nguyen, N. H., Song, Z., Bates, S. T., Branco, S., Tedersoo, L., Menke, J., et al. (2016). FUNGuild: An open annotation tool for parsing fungal community datasets by ecological guild. Fungal Ecol. 20, 241–248. doi: 10.1016/j.funeco.2015.06.006
Nickel, U. T., Weikl, F., Kerner, R., Schäfer, C., Kallenbach, C., Munch, J. C., et al. (2018). Quantitative losses vs. qualitative stability of ectomycorrhizal community responses to 3 years of experimental summer drought in a beech–spruce forest. Glob. Change Biol. 24, e560–e576. doi: 10.1111/gcb.13957
Nikolova, P. S., Bauerle, T. L., Häberle, K. H., Blaschke, H., Brunner, I., and Matyssek, R. (2020). Fine-root traits reveal contrasting ecological strategies in European beech and Norway Spruce during extreme drought. Front. Plant Sci. 11:1211. doi: 10.3389/fpls.2020.01211
Oh, S. Y., Park, M. S., Cho, H. J., and Lim, Y. W. (2018). Diversity and effect of trichoderma isolated from the roots of Pinus densiflora within the fairy ring of pine mushroom (Tricholoma matsutake). PLoS One 13:e0205900. doi: 10.1371/journal.pone.0205900
Oksanen, A. J., Blanchet, F. G., Friendly, M., Kindt, R., Legendre, P., Mcglinn, D., et al. (2019). “Vegan,” in Encyclopedia of food and agricultural ethics, ed. D. M. Kaplan (Dordrecht: Springer Netherlands), 2395–2396. doi: 10.1007/978-94-024-1179-9_301576
Otsing, E., Anslan, S., Ambrosio, E., Koricheva, J., and Tedersoo, L. (2021). Tree species richness and neighborhood effects on ectomycorrhizal fungal richness and community structure in boreal forest. Front. Microbiol. 12:567961. doi: 10.3389/fmicb.2021.567961
Pedersen, M. W., Overballe-Petersen, S., Ermini, L., Sarkissian, C. D., Haile, J., Hellstrom, M., et al. (2015). Ancient and modern environmental DNA. Philos. Trans. R. Soc. Lond. B Biol. Sci. 370:20130383. doi: 10.1098/rstb.2013.0383
Peter, M., Kohler, A., Ohm, R. A., Kuo, A., Krützmann, J., Morin, E., et al. (2016). Ectomycorrhizal ecology is imprinted in the genome of the dominant symbiotic fungus Cenococcum geophilum. Nat. Commun. 7:12662. doi: 10.1038/ncomms12662
Põlme, S., Abarenkov, K., Nilsson, R. H., Lindahl, B. D., Clemmensen, K. E., Kauserud, H., et al. (2020). FungalTraits: A user-friendly traits database of fungi and fungus-like stramenopiles. Fungal Divers. 105, 1–16. doi: 10.1007/s13225-020-00466-2
Pretzsch, H. (2022). Mixing degree, stand density, and water supply can increase the overyielding of mixed versus monospecific stands in Central Europe. For. Ecol. Manag. 503:119741. doi: 10.1016/j.foreco.2021.119741
Pretzsch, H., Biber, P., Schütze, G., Uhl, E., and Rötzer, T. (2014). Forest stand growth dynamics in Central Europe have accelerated since 1870. Nat. Commun. 5:4967. doi: 10.1038/ncomms5967
Pretzsch, H., del Río, M., Ammer, C., Avdagic, A., Barbeito, I., Bielak, K., et al. (2015). Growth and yield of mixed versus pure stands of scots pine (Pinus sylvestris L.) and European beech (Fagus sylvatica L.) analysed along a productivity gradient through Europe. Eur. J. For. Res. 134, 927–947. doi: 10.1007/s10342-015-0900-4
Pretzsch, H., Grams, T., Häberle, K. H., Pritsch, K., Bauerle, T., and Rötzer, T. (2020). Growth and mortality of Norway Spruce and European Beech in monospecific and mixed-species stands under natural episodic and experimentally extended drought. Results of the KROOF throughfall exclusion experiment. Trees Struct. Funct. 34, 957–970. doi: 10.1007/s00468-020-01973-0
Pretzsch, H., Kahn, M., and Grote, R. (1998). The mixed spruce-beech forest stands of the ‘sonderforschungsbereich’ ‘growth or parasite defence?’ in the forest district Kranzberger forst. Forstwissenschaftliches Centralblatt 117, 241–257. doi: 10.1007/bf02832979
Qian, X. M., Kottke, I., and Oberwinkler, F. (1998). Activity of different ectomycorrhizal types studied by vital fluorescence. Plant Soil 199, 91–98. doi: 10.1023/A:1004226220283
Querejeta, J. I., Egerton-Warburton, L. M., and Allen, M. F. (2009). Topographic position modulates the mycorrhizal response of oak trees to interannual rainfall variability. Ecology 90, 649–662. doi: 10.1890/07-1696.1
Querejeta, J. I., Egerton-Warburton, L. M., and Allen, M. F. (2003). Direct nocturnal water transfer from oaks to their mycorrhizal symbionts during severe soil drying. Oecologia 134, 55–64. doi: 10.1007/s00442-002-1078-2
R Core Team (2021). R: A language and environment for statistical computing. Vienna: R Foundation for Statistical Computing.
Reich, P. B. (2014). The world-wide ‘fast-slow’ plant economics spectrum: A traits manifesto. J. Ecol. 102, 275–301. doi: 10.1111/1365-2745.12211
Richard, F., Roy, M., Shahin, O., Sthultz, C., Duchemin, M., Joffre, R., et al. (2011). Ectomycorrhizal communities in a mediterranean forest ecosystem dominated by Quercus ilex: Seasonal dynamics and response to drought in the surface organic horizon. Ann. For. Sci. 68, 57–68. doi: 10.1007/s13595-010-0007-5
Rognes, T., Flouri, T., Nichols, B., Quince, C., and Mahé, F. (2016). VSEARCH: A versatile open source tool for metagenomics. PeerJ 2016, 1–22. doi: 10.7717/peerj.2584
Rukh, S., Poschenrieder, W., Heym, M., and Pretzsch, H. (2020). Drought resistance of Norway spruce (Picea abies [L.] Karst) and European beech (Fagus sylvatica [L.]) in mixed vs. monospecific stands and on dry vs. wet sites. From evidence at the tree level to relevance at the stand level. Forests 11:639. doi: 10.3390/f11060639
Ruotsalainen, A. L., Kauppinen, M., Wäli, P. R., Saikkonen, K., Helander, M., and Tuomi, J. (2022). Dark septate endophytes: Mutualism from by-products?. Trends Plant Sci. 27, 247–254. doi: 10.1016/j.tplants.2021.10.001
Schmid, I. (2002). The influence of soil type and interspecific competition on the fine root system of Norway Spruce and European beech. Basic Appl. Ecol. 3, 339–346. doi: 10.1078/1439-1791-00116
Selosse, M. A., Schneider-Maunoury, L., and Martos, F. (2018). Time to re-think fungal ecology? fungal ecological niches are often prejudged. New Phytol. 217, 968–972. doi: 10.1111/nph.14983
Sharda, J. N., and Koide, R. T. (2008). Can hypodermal passage cell distribution limit root penetration by mycorrhizal fungi?. New Phytol. 180, 696–701. doi: 10.1111/j.1469-8137.2008.02600.x
Shi, L., Guttenberger, M., Kottke, I., and Hampp, R. (2002). The effect of drought on mycorrhizas of beech (Fagus sylvatica L.): Changes in community structure, and the content of carbohydrates and nitrogen storage bodies of the fungi. Mycorrhiza 12, 303–311. doi: 10.1007/s00572-002-0197-2
Shoresh, M., Harman, G. E., and Mastouri, F. (2010). Induced systemic resistance and plant responses to fungal biocontrol agents. Annu. Rev. Phytopathol. 48, 21–43. doi: 10.1146/annurev-phyto-073009-114450
Smith, G. R., Finlay, R. D., Stenlid, J., Vasaitis, R., and Menkis, A. (2017). Growing evidence for facultative biotrophy in saprotrophic fungi: Data from microcosm tests with 201 species of wood-decay basidiomycetes. New Phytol. 215, 747–755. doi: 10.1111/nph.14551
Solly, E. F., Brunner, I., Helmisaari, H. S., Herzog, C., Leppälammi-Kujansuu, J., Schöning, I., et al. (2018). Unravelling the age of fine roots of temperate and boreal forests. Nat. Commun. 9:3006. doi: 10.1038/s41467-018-05460-6
Swaty, R. L., Deckert, R. J., Whitham, T. G., and Gehring, C. A. (2004). Ectomycorrhizal abundance and community composition shifts with drought: Predictions from tree rings. Ecology 85, 1072–1084. doi: 10.1890/03-0224
Swaty, R. L., Gehring, C. A., Van Ert, M., Theimer, T. C., Keim, P., and Whitham, T. G. (1998). Temporal variation in temperature and rainfall differentially affects ectomycorrhizal colonization at two contrasting sites. New Phytol. 139, 733–739. doi: 10.1046/j.1469-8137.1998.00234.x
Taniguchi, T., Kitajima, K., Douhan, G. W., Yamanaka, N., and Allen, M. F. (2018). A pulse of summer precipitation after the dry season triggers changes in ectomycorrhizal formation, Diversity, and community composition in a mediterranean forest in California, USA. Mycorrhiza 28, 665–677. doi: 10.1007/s00572-018-0859-3
Tedersoo, L., and Smith, M. E. (2013). Lineages of ectomycorrhizal fungi revisited: Foraging strategies and novel lineages revealed by sequences from belowground. Fungal Biol. Rev. 27, 83–99. doi: 10.1016/j.fbr.2013.09.001
Tedersoo, L., Anslan, S., Bahram, M., Põlme, S., Riit, T., Liiv, I., et al. (2015). Shotgun metagenomes and multiple primer pair-barcode combinations of amplicons reveal biases in metabarcoding analyses of fungi. MycoKeys 10, 1–43. doi: 10.3897/mycokeys.10.4852
Tedersoo, L., Bahram, M., Cajthaml, T., Põlme, S., Hiiesalu, I., Anslan, S., et al. (2016). Tree diversity and species identity effects on soil fungi, protists and animals are context dependent. ISME J. 10, 346–362. doi: 10.1038/ismej.2015.116
Tedersoo, L., Bahram, M., Toots, M., Diédhiou, A. G., Henkel, T. W., Kjoller, R., et al. (2012). Towards global patterns in the diversity and community structure of ectomycorrhizal fungi. Mol. Ecol. 21, 4160–4170. doi: 10.1111/j.1365-294X.2012.05602.x
Unuk, T., Martinović, T., Finžgar, D., Šibanc, N., Grebenc, T., and Kraigher, H. (2019). Root-associated fungal communities from two phenologically contrasting silver fir (Abies alba Mill.) groups of trees. Front. Plant Sci. 10:214. doi: 10.3389/fpls.2019.00214
Veach, A. M., Chen, H., Yang, Z. K., Labbe, A. D., Engle, N. L., Tschaplinski, T. J., et al. (2020). Plant hosts modify belowground microbial community response to extreme drought. mSystems 5:e00092-e20. doi: 10.1128/msystems.00092-20
Wang, Q., Garrity, G. M., Tiedje, J. M., and Cole, J. R. (2007). Naïve bayesian classifier for rapid assignment of RRNA sequences into the new bacterial taxonomy. Appl. Environ. Microbiol. 73, 5261–5267. doi: 10.1128/AEM.00062-07
Wasyliw, J., and Karst, J. (2020). Shifts in ectomycorrhizal exploration types parallel leaf and fine root area with forest age. J. Ecol. 108, 2270–2282. doi: 10.1111/1365-2745.13484
Weigt, R. B., Raidl, S., Verma, R., and Agerer, R. (2012). Exploration type-specific standard values of extramatrical mycelium–a step towards quantifying ectomycorrhizal space occupation and biomass in natural soil. Mycol. Prog. 11, 287–297. doi: 10.1007/s11557-011-0750-5
Wickham, H. (2016). Ggplot2: Elegant graphics for data analysis. Use R!. Cham: Springer International Publishing. doi: 10.1007/978-3-319-24277-4
Zadworny, M., and Eissenstat, D. M. (2011). Contrasting the morphology, anatomy and fungal colonization of new pioneer and fibrous roots. New Phytol. 190, 213–221. doi: 10.1111/j.1469-8137.2010.03598.x
Zhang, Y., Chen, H. Y. H., and Reich, P. B. (2012). Forest productivity increases with evenness, species richness and trait variation: A global meta-analysis. J. Ecol. 100, 742–749. doi: 10.1111/j.1365-2745.2011.01944.x
Zwetsloot, M. J., and Bauerle, T. L. (2021). Repetitive seasonal drought causes substantial species-specific shifts in fine-root longevity and spatio-temporal production patterns in mature temperate forest trees. New Phytol. 231, 974–986. doi: 10.1111/nph.17432
Keywords: drought recovery, European beech, fine roots, Norway spruce, root-associated fungi, tree mixture
Citation: Danzberger J, Werner R, Mucha J, Pritsch K and Weikl F (2023) Drought legacy effects on fine-root-associated fungal communities are modulated by root interactions between tree species. Front. For. Glob. Change 6:1197791. doi: 10.3389/ffgc.2023.1197791
Received: 31 March 2023; Accepted: 19 July 2023;
Published: 03 August 2023.
Edited by:
Camille Emilie Defrenne, Michigan Technological University, United StatesReviewed by:
Marco Cosme, University of Antwerp, BelgiumJose G. Maciá-Vicente, Wageningen University and Research, Netherlands
Copyright © 2023 Danzberger, Werner, Mucha, Pritsch and Weikl. This is an open-access article distributed under the terms of the Creative Commons Attribution License (CC BY). The use, distribution or reproduction in other forums is permitted, provided the original author(s) and the copyright owner(s) are credited and that the original publication in this journal is cited, in accordance with accepted academic practice. No use, distribution or reproduction is permitted which does not comply with these terms.
*Correspondence: Jasmin Danzberger, amFzbWluLmRhbnpiZXJnZUBmcmVlbmV0LmRl
†ORCID: Jasmin Danzberger, orcid.org/0000-0002-1683-7345; Ramona Werner, orcid.org/0000-0003-0075-1720; Joanna Mucha, orcid.org/0000-0002-1290-9639; Karin Pritsch, orcid.org/0000-0001-6384-2473; Fabian Weikl, orcid.org/0000-0003-3973-6341