- Faculty of Forestry and Wood Sciences, Czech University of Life Sciences Prague, Prague, Czechia
Intoduction: The bark beetle Ips typographus currently represents the primary pest of Norway spruce (Picea abies) in Central Europe. Early detection and timely salvage cutting of bark beetle-infested trees are functional management strategies for controlling bark beetle outbreaks. However, alternative detection methods are currently being developed, and possible indicators of bark beetle infestation can be assessed through changes in the physiological, biochemical, and beetle-acceptance characteristics of trees.
Method: This study monitored infested and non-infested Norway spruce trees before and 3 weeks after Ips typographus natural attack. Permanently installed sensors recorded physiological features, such as sap flow, tree stem increment, bark surface temperature, and soil water potential, to monitor water availability. Defensive metabolism characteristics, beetle host acceptance, and attractiveness to trees were monitored discretely several times per season. The forest stand that was later attacked by bark beetles had lower water availability during the 2018–2020 seasons compared to the non-attacked stands.
Results: After the attack, sap flow and tree stem increment were significantly lower in infested trees than in intact ones, and bark surface temperature moderately increased, even when measured in the inner forest stand from the shadowed side. Infested trees respond to attacks with a surge in monoterpene emissions. In addition, freshly infested trees were more accepted by males in the no-choice bioassays, and a significantly higher number of beetles were caught in passive traps in the first week of infestation.
Conclusion: The most promising characteristics for early detection methods of bark beetle-infested trees include tree bark temperature measured only in certain meteorological conditions, elevated monoterpene emissions, and significantly high catches in passive traps.
1. Introduction
The Eurasian Spruce Bark Beetle Ips typographus (Linnaeus, 1758) (Coleoptera: Scolytinae) is the most devastating pest of Norway spruce [Picea abies (L.) Karst.] and forests in the Palearctic region (Christiansen and Bakke, 1988). When its population is in the endemic phase, it attacks weakened trees, contributing to the ecological balance in natural forests. However, the incidence of disturbance agents, such as strong winds or drought periods, lead to the transition of the I. typographus population to the epidemic phase (Kausrud et al., 2012). Ongoing climate change, the occurrence of spruce stands outside their natural range, and economically oriented silviculture practices have led to intense bark beetle outbreaks over the last decade (Seidl et al., 2016; Marini et al., 2017; Biedermann et al., 2019). The Central European region has been seriously affected by I. typographus outbreaks that started after severe drought events in 2015 and 2018 (Hlásny et al., 2021a). The scenario resulted in an exponentially growing volume of salvage logging from 2017 to 2020 (approximately 5.9 mil m3 in 2017 to 26.2 mil m3 in 2020 in the Czech Republic) (Hlásny et al., 2021b,2022).
A traditional method for managing bark beetle outbreaks is the early detection of infested trees prior to the emergence of offspring generation (Hlásny et al., 2019). The presence of boring dust at the base of the trunk is considered the most reliable symptom of bark beetle infestation (Kautz et al., 2023), but its use requires personal inspection of each trunk, which is difficult to achieve. The extent of the forest stands, the intensity of the outbreaks, and the ability to distinguish infested trees were the main limits for applying this procedure. The possibility of including modern tools and procedures in the search for bark beetle-attacked trees is currently being intensively researched. Currently, the most promising and advanced methods are remote sensing methods, which include a wide range of approaches (Huo et al., 2021). Despite the large number of areas that they are able to scan, there remain problems with the demands of time and expertise involved in processing the captured records and the inaccuracy of detection owing to the considerable variability of the attack signs. A different method for detecting infested trees is based on the chemical communication between bark beetles (Raffa et al., 2016). For this purpose, specially trained dogs have recently been used (Johansson et al., 2019), which can detect trees more successfully and efficiently than human experts (Vošvrdová et al., 2023). The first study using an artificial nose to detect substances in the forest environment is currently underway (Hüttnerová et al., 2023). However, for the purpose of early detection of bark beetles, the sap flow, tree increment and content of terpenes in phloem and catches to passive traps in infested trees have not been evaluated.
Like all plants, trees infested by bark beetles rouse defense mechanisms against herbivores that have evolved over a million years (Berini et al., 2018). In conifers, it manifests itself with an immediate response as resin exudations and leads to changes in basic tree physiology, such as transpiration (Wang, 1983) and resource allocation (Franceschi et al., 2005; Boone et al., 2011). The metabolism in trees is based on the fundamental process of photosynthesis, when atmospheric carbon is sequestered, and carbohydrates are synthesized (Lawlor and Cornic, 2002). These carbon resources are distributed between primary tree metabolism (tree growth or reproduction) and secondary metabolism (constitutive defense compounds as phenolics and terpenes important in conifers) (Huang et al., 2020). Physiological characteristics that describe these processes can be measured using specific techniques. These characteristics have been previously recorded in various contexts related to tree stress and susceptibility to bark beetle attacks.
The sap flow value, which expresses a deficit in tree transpiration measured in short time intervals, is often used as a quantitative characteristic of drought stress in trees (Střelcová et al., 2013; Gebhardt et al., 2023) or stress from sudden sun radiation in fragmented forests (Özçelik et al., 2022). The acute transpiration deficit positively correlates with the lowering of the defense ability of trees against bark beetle infestation (Netherer et al., 2015; Matthews et al., 2018).
Another physiological characteristic of Norway spruce discussed in the article as an indicator of infestation is the tree stem diameter. Measuring stem increment has been reported in the literature to indicate reductions in growth rates related to drought (Ježík et al., 2015). It has also been used to evaluate wood production in different tree species or genotypes (Cocozza et al., 2016). Fluctuations in stem circumference are influenced by the dynamics of plant tissue water balance on a daily and seasonal basis, with radial growth increments depending on these fluctuations (Offenthaler et al., 2001). The stem increment is also influenced by carbohydrate distribution. In healthy trees, there is a balance between growth and defense, with carbon resources allocated to both. However, during herbivore or pathogen attacks, the allocation of carbon resources shifts toward the production of defense metabolites, limiting investment in growth, including stem increment (Huang et al., 2020).
A previous study (Majdák et al., 2021) reported a measurable increase in bark surface temperature following bark beetle infestation in sun-exposed trees on the forest edge. These trees were weakened by infestation and could not keep the optimal temperature. Generally, the temperature of the cambium and phloem in a healthy tree with sufficient water content is well-regulated. Healthy trees can maintain their internal thermal environment and prevent excessive temperature increase (Leuzinger and Korner, 2007) through evaporative cooling facilitated by the water movement within the sap flow. However, the bark surface temperature is influenced by direct radiation and air temperature (Hietz et al., 2005), and there exists some correlation between phloem temperature and surface temperature (Powell, 1967).
The most noticeable changes in tree characteristics following bark beetle infestation are chemical defense reactions. These reactions occur in two stages. The first stage is the immediate defense response, where conifers exude stored resin. The second stage, known as the induced defense response, involves the formation of traumatic resin ducts (Franceschi et al., 2005) and is triggered within the first few weeks following a bark beetle attack (Celedon and Bohlmann, 2019). The overall resin exudation of trees measured as resin flow was in several studies suggested as a marker of resistance of Norway spruce trees against the bark beetles and characteristics of conifer defense (Netherer et al., 2015). Resin flow varies from tree to tree owing to genetic variation and age (Christiansen and Horntvedt, 1983; Schroeder, 1990) and strongly depends on temperature and meteorological conditions (Baier et al., 2002; Stříbrská et al., 2022) and on phenotypic variables, and location (Zas et al., 2020).
Resin is a mixture of terpene compounds with toxic and immobilizing effects on bark beetles; however, it also has a communication function for them (Erbilgin et al., 2007). In spruce, the predominant volatile monoterpenes are alpha-pinene, beta-pinene, Δ-carene, limonene, β-phellandrene, camphene and myrcene (Borg-Karlson et al., 1993). Resin also contains sesquiterpenes in smaller quantities and higher content of diterpenes (Netherer et al., 2021). Oxidized forms of all terpenes are also present at a low level, and the content of oxygenated monoterpenes is modified by a stress reaction and tree decay (Schiebe et al., 2019) caused alternatively by inoculation by beetle’s symbiotic ophiostomatoid fungi (Kandasamy et al., 2023). Even non-infested conifers emit large amounts of terpenes, mainly from the needles (Juráò et al., 2017). The emission has a diurnal rhythm and depends on the actual meteorological and physiological conditions of trees (Kopaczyk et al., 2020) as well as on genetic origin (Kännaste et al., 2013). These phytochemicals play their role in the selection of suitable bark beetle habitat (Erbilgin, 2019). When Norway spruce is attacked by bark beetles, either due to constitutive resin storage opening or induction of defense terpene biosynthesis, the content of emitted terpenes increases 10–100 fold (Ghimire et al., 2016; Jaakkola et al., 2022).
The attractivity of the freshly attacked trees for additional beetle conspecifics is modified based on olfactometric cues perceived by beetles, predominantly on aggregation pheromones (Schlyter et al., 1987a) and host volatiles (Erbilgin et al., 2007). The acceptance of host tree by attacking beetles is a function of the defense ability of trees and stadia of beetle attacks. The I. typographus infestation begins with the selection of a suitable host tree by pioneer males (Byers, 1989; Lehmanski et al., 2023). When males successfully overcome tree defenses, they produce potent aggregation pheromones to attract conspecifics and start mass aggregation. Pheromone consists of oxidized terpenes 2-methyl-3-buten-2-ol and cis-verbenol 10:1 (Birgersson et al., 1984; Ramakrishnan et al., 2022). Bark beetles can detect these highly biologically active compounds from a complex mixture of other compounds in the forest owing to their specific antennal receptors. However, pheromones are only a minor component of the total volatile emissions of infested trees.
This study aimed to identify alternative tools for the early detection of I. typographus attacks based on modifications in physiology, defense biochemistry, and insect-tree interaction levels. In particular, we compared changes in Norway spruce trees in the first stadia of I. typographus attack in terms of (i) physiological and physical parameters (sap flow, stem increment, and surface temperature); (ii) spruce defense reaction (resin flow, emission, and phloem content of defensive terpenes, including selection of compounds specific for infested trees); and (iii) beetles attraction to infested and non-infested stands by monitoring beetles using non-baited passive traps and (iv) beetle acceptance of the host tree. Furthermore, measurable characteristics that displayed significant differences in infested trees compared to non-infested trees were evaluated as potential tools for developing early attack detection methods for more efficient bark beetle management.
2. Materials and methods
2.1. Study area and sampling setup and conditions
The study was conducted from May 6th to July 2nd, 2020 at the property of the Forests CZU in Kostelec nad Černými lesy in central Czech Republic (Figure 1). The weather during the growing season of 2020 was humid and warm. The detailed recording of the meteorological conditions has been previously published (Stříbrská et al., 2022) and is attached as a supplement to this article (Supplementary Table 1).
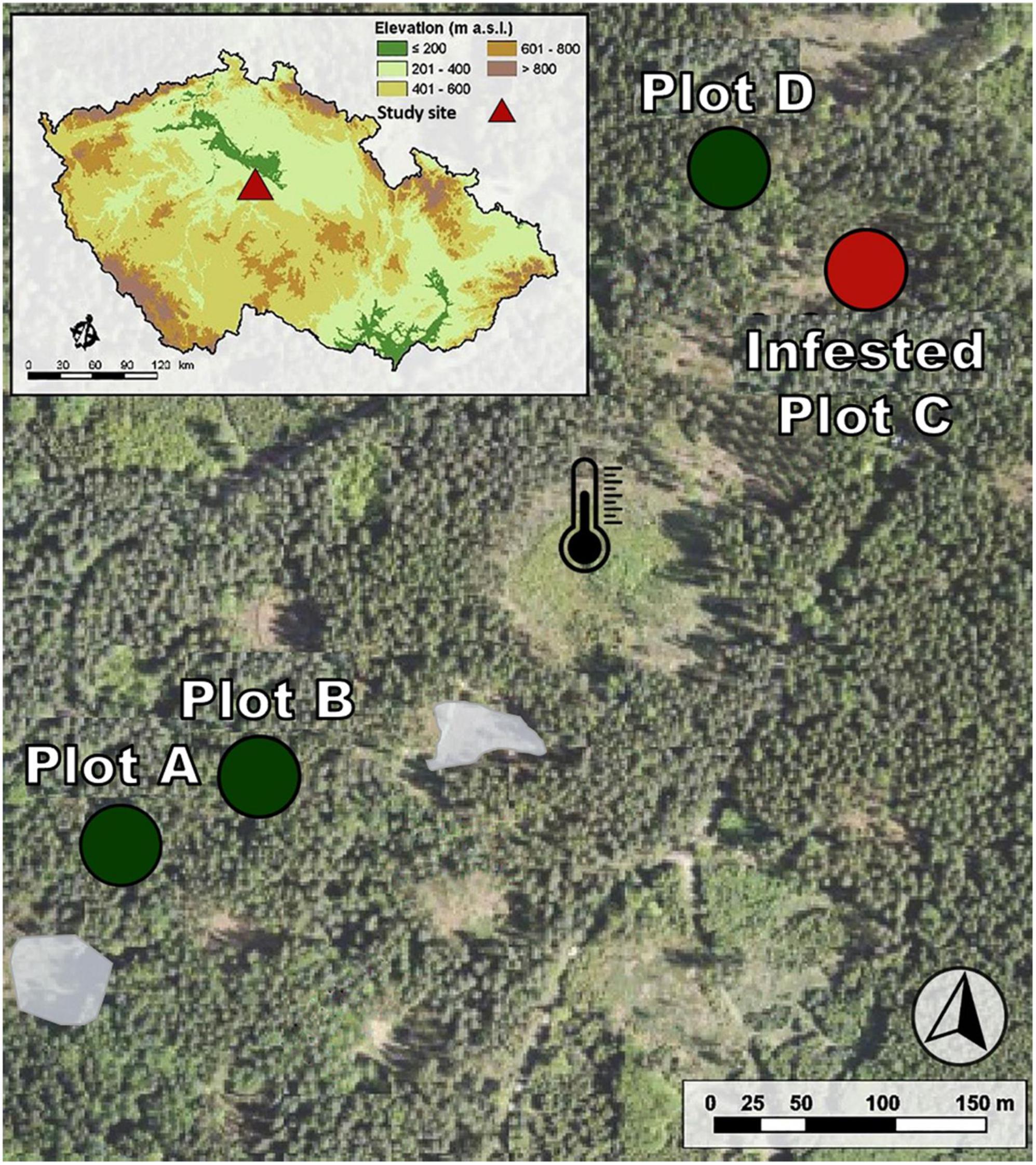
Figure 1. Arrangement of study plots with the naturally attacked plot. Green circles represent the inner forest plots (non-attacked trees), corresponding to plots A, B, and D. Plot C was infested by I. typographus on June 16th and salvaged in July (red circle). The gray spots are areas of previous natural bark beetle attack. The black thermometer marks the meteorological station.
As part of the Extemit-K project, a large study area was established in 2018 to measure physiological and dendrological characteristics in trees exposed to stress conditions such as drought and forest fragmentation in the context of bark beetle attack. Originally, eight plots with different treatments were monitored (Stříbrská et al., 2022). However, the study reported in this article focuses on four non-treated plots established within a closed, undisturbed area in Norway spruce stands (Table 1).
From 2018 to 2020, the soil water potential was monitored for all plots. In 2020, sensors for sap flow, tree stem increment, and bark surface temperature collected data from a total of eighteen trees. Four trees were monitored in plots B and D, while five trees were monitored in plots A and C. Additionally, monoterpene sampling and non-choice beetle bioassays were conducted six times per growing season in 2020 on three selected trees in each of the four studied plots (12 trees in total).
Throughout the study period, we checked the entire area within a 500 m distance from the monitored trees for bark infestation at approximately weekly intervals. However, on June 16th, 2020, a fresh infestation was detected in all five trees in plot C at the stage of the nuptial chamber building by male beetles. In next 3 weeks, from June 16th to July 2nd, these five infested trees were further monitored with sensors, along with the remaining thirteen uninfested trees. On July 2nd, the infested trees had to be cut down to prevent the emergence of new beetle generations, and the observation was concluded.
In 2020, an outbreak of I. typographus occurred in the Norway spruce forests of the Forests CZU. Extreme droughts in the years 2015 and 2018 were the cause of the exponential increase in infested trees, which started in 2017 with 284 m3 and culminated in 2020 with 76,113 m3 of salvaged cut wood (Klinovský, 2021). In 2018 and 2019, the local centra (gray spots in Figure 1) of bark beetle infestation was detected in the studied area and cut down. None of them were closer than 50 m from the monitored trees (Stříbrská et al., 2022).
In each plot, the soil water potential was recorded to check the water availability of trees using five sensors (Teros 21, Meter Group, München, Germany) distributed in each plot and placed 20 cm below the surface. The whole dataset (Supplementary Figure 1) was taken from the 2018 to 2020 seasons to examine water stress on the trees.
2.2. Physiological characteristics of trees
The methodology for data sampling was modified from Stříbrská et al. (2022).
Briefly, sap flow, tree stem increment, and bark surface temperature were measured using sensors installed on individual trees. Data were stored in a single data logger (GreyBox N2N 3P; EMS Brno, Brno, Czech Republic) and connected to a cloud system via the GSM. To enable statistical analysis, we express the continuously recorded values of physiological characteristics as means per collection day or a specific period, which allowed us to process them together with discretely measured characteristics and show changes in the same time points.
Sap flow was measured based on the thermodynamic principle by heating the wood around stainless-steel electrodes (EMS 81; EMS Brno) using the trunk heat balance method. Data were recorded at 10-min intervals (Čermák et al., 2004; Stříbrská et al., 2022). The data were subjected to post-processing, including baseline correction, and sap flow rates were recomputed as kg/h as the sum for each sampling date.
Tree stem increment was recorded using a sensor (DR26E Band dendrometer for sap flow system; EMS 81 DR26E; EMS Brno), which was installed 3.5 m above the ground. The change in trunk circumference was measured every 5 min and stored in the data logger as a 10-min average. After cleaning up the errors caused by various influences, the data were converted to 1 h averages, and then divided by two times 3,14 (pi). The beginning of the season is determined according to the growth and the start of sap flow as the zero point of tree increment increases in a given year, for this season, it was determined on April 1st.
Bark surface temperature was measured on the north side of the tree stem to eliminate the influence of sun radiation. Infrared thermometers (Apogee Instruments, Logan, UT) were installed at a height of 3 m. Data were collected at hourly intervals, and averages for days (24 h) for the collection period were calculated (Stříbrská et al., 2022).
2.3. Defense characteristics of trees
Resin flow was measured using glass tubes (inner diameter: 3 mm; outer diameter: 5 mm; length: 12 cm). One glass tube per tree/repetition was inserted into holes (6 mm) drilled into the bark and phloem at breast height (1.3 m) from the north and south exposed sides of the trunks. The resin was collected for 24 h (start and end of collection between 3 and 5 pm), and the level of exudated resin in glass tubes was measured (Netherer et al., 2015; Stříbrská et al., 2022).
The main monoterpenes in the close vicinity of the spruce stem were collected using SPME (Solid Phase Microextraction) fiber (PDMS/CAR/DVB; Supelco, USA), which was placed in an aluminum box (25 cm × 5 cm × 5 cm) loosely fixed by rope on the tree stem surface at 3.5 m height. When collected from infested trees, the boxes were attached out of the beetle’s entrance hole and frass. The collection took 1 h (from 1 pm to 2 pm) on the day of the sampling. Immediately after sampling, the fibers were sealed, stored on dry ice, and transported to the laboratory. Desorption and compound measurements by gas chromatography-electron impact-time of flight-mass spectrometry (GC-EI-TOF-MS) were performed within 3 days after collection (Stříbrská et al., 2022).
Bark samples for analysis of compounds extractable from phloem were collected on May 27th, June 16th, and June 30th. Three sections (8 mm diameter) were pinched out using a cork borer at a distance of 15 cm from each other at a height of 2 m on the south side of the tree trunks. Samples were stored in liquid N2 and transported to the laboratory. Three sections from each tree were pooled and ground in liquid nitrogen to obtain a fine powder. The powder (200 mg) was extracted for 10 min in 2 mL of hexane (containing 5 μg/mL of the internal standard 1-bromododecane) in an ultrasonic bath. Extracts were filtered into 2-mL vials for GC-EI-TOF-MS (Stříbrská et al., 2022).
The collected monoterpenes and compounds from the phloem were analyzed using a gas chromatograph (Agilent 7890 B; Agilent, USA) coupled to a mass spectrometer with a time-of-flight mass analyzer GC-EI-TOF-MS Pegasus 4D (LECO, St. Joseph, MI, USA). The instrument was equipped with a capillary column HP-5MS UI (30 m, 0.25 mm i.d., 0.25 μm film thickness; Agilent). The analysis setups were as follows. For analysis of SPME, a hot PTV inlet (265°C) was used in a 2 min spitless period. The temperature program for the GC oven was 40°C (1 min) - 15°C/min to 210°C - 20°C/min to 280°C. To analyze phloem extracts, 1 μL of hexane extract was injected in spitless mode into the PTV inlet (programmed from 20°C–8°C/s to 265°C). The GC oven was programmed: 40°C (1 min) - 5°C/min to 210°C - 20°C/min to 320°C (6 min). The mass spectrometer setup was as follows: ionization energy in electron impact mode was 70 eV, ensuring spectra compatibility with NIST library. Full spectra were collected in mass range, 35–500 Da with speed of 10 spectra per second.
The ChromaTOF software (LECO) was used for chromatographic data processing. Compound identification was performed using comparison of measured mass spectra and spectra in mass spectral library NIST (2017), using comparison of measured retention indexes (counted on C8-C40 saturated alkane scale) with retention indexes published in NIST. For main monoterpenes, retention times of analytical standards measured by the same methods as samples were used.
The main monoterpenes detected in Norway spruce were tricyclene, α-pinene, and β-pinene in coelution with myrcene, β-phellandrene, Δ-carene, camphene (quantification mass m/z 93) and limonene (quantification mass m/z 68). To statistically evaluate the monoterpenes collected from air by solid-phase microextraction (SPME) close by infested and non-infested trees, the sum of peak areas of quantification masses of these compounds were summed and treated as a single value per tree per collection. The concentration of the sum of the same monoterpenes extracted from the phloem was quantified as μg/mg of the dry weight of material using calibration curves constructed for α-pinene, β-pinene, Δ-carene, camphene, and limonene. Peak areas were normalized by internal standard (1-bromodecane) to correct injection volume fluctuation. Furthermore, differences in the content of these eight monoterpenes individually were compared in air collected by SPME as peak areas and in the phloem extract as concentration (μg/mg of dry weight).
The peak areas of quantification masses of all detectable compounds with a sufficient threshold in GC-EI-TOF-MS chromatograms, which were recorded from the collection by SPME on June 24th, were preprocessed by aligning software and analyzed using principal component analysis (PCA) and the discriminant analysis model (PLS-DA).
2.4. Beetle catches in passive traps and beetle acceptance bioassays
Catches of beetles in passive traps made from transparent plastic (40 × 60 cm) indicated the attractivity of trees. Traps were mounted on the southern side of the tree trunk at breast height (Schlyter et al., 1987b; Stříbrská et al., 2022). Ten passive traps were mounted on trees, which were infested on June 16th, and ten passive traps on trees within the control plots. The checking of the number of beetles caught in passive traps without any additional pheromone attraction was conducted from May 1st, and the last catch collection was on June 16th.
No-choice bioassay to monitor beetle acceptance was performed by encapsulating males of I. typographus to Eppendorf tubes (one male per tube). Ten tubes were attached to the south-exposed side of the tree trunk at a height of 2 m and fixed with tape. The beetles were left to feed for 24 h (Turčáni and Nakládal, 2007; Stříbrská et al., 2022). Active beetles used in the statistical evaluation were assigned into three behavioral categories: beetles that bored inside the bark and stood inside the pitch tubes, beetles that bored inside but were expelled by resin, and beetles that started to feed but were interrupted. The Eppendorf bioassay was performed three times per season (June 16th, June 24th, and June 30th).
2.5. Statistical analyses
The R statistical software (R Core Team, 2022) was used for statistical analyses.
For testing the hypothesis that infested and non-infested trees differ, we utilized a general linear mixed model (glmer() function from the lme4 package; Bates et al., 2015). We split measurements into the pre-attack period and post-attack period to assess differences between those periods separately. Repeated measures model was fit with the fixed effect of measurement time and bark beetle attack and random effect of the plot. We used the gamma distribution with log link; in cases of count data in response (number of beetles), we used the Poisson distribution. Post hoc Tukey analyses between infested and non-infested trees in overall repeated measures model and inside each measurement separately were performed using lsmeans() function from the lsmeans package (Lenth, 2016). Histograms of residuals and residuals vs predicted values plots were inspected.
The total profile of compounds collected on SPME fiber was normalized (constant raw sum), central log transformed and pareto scaled for PCA and subsequent partial least square-discriminant analysis (PLS-DA) created in the SIMCA 17 software (Sartorius Stedim Data Analytics AB, Malmö, Sweden).
3. Results
3.1. Soil water potential
Within the vegetation season of 2020, the soil water potential did not decrease below -200 kPa in all monitored plots A, B, C, and D, which suggests sufficient water supply for all studied trees; however, in plot C, infested in June 16th, soil water potential was significantly lower than on non-infested plots (p = 0.04; Figure 2). The history of water supply in the study site revealed mild water stress in the trees in 2018. Soil water potential values ranged between -800 and -1400 kPa, with a short decrease below the wilting point of -1500 kPa in August 2018. In the growing season of 2019, the lowest values of soil water potential were from -400 kPa to -900 kPa. In both years preceding the study, the soil water potential in plot C was lower than that in plots A, B, and D (Supplementary Figure 1).
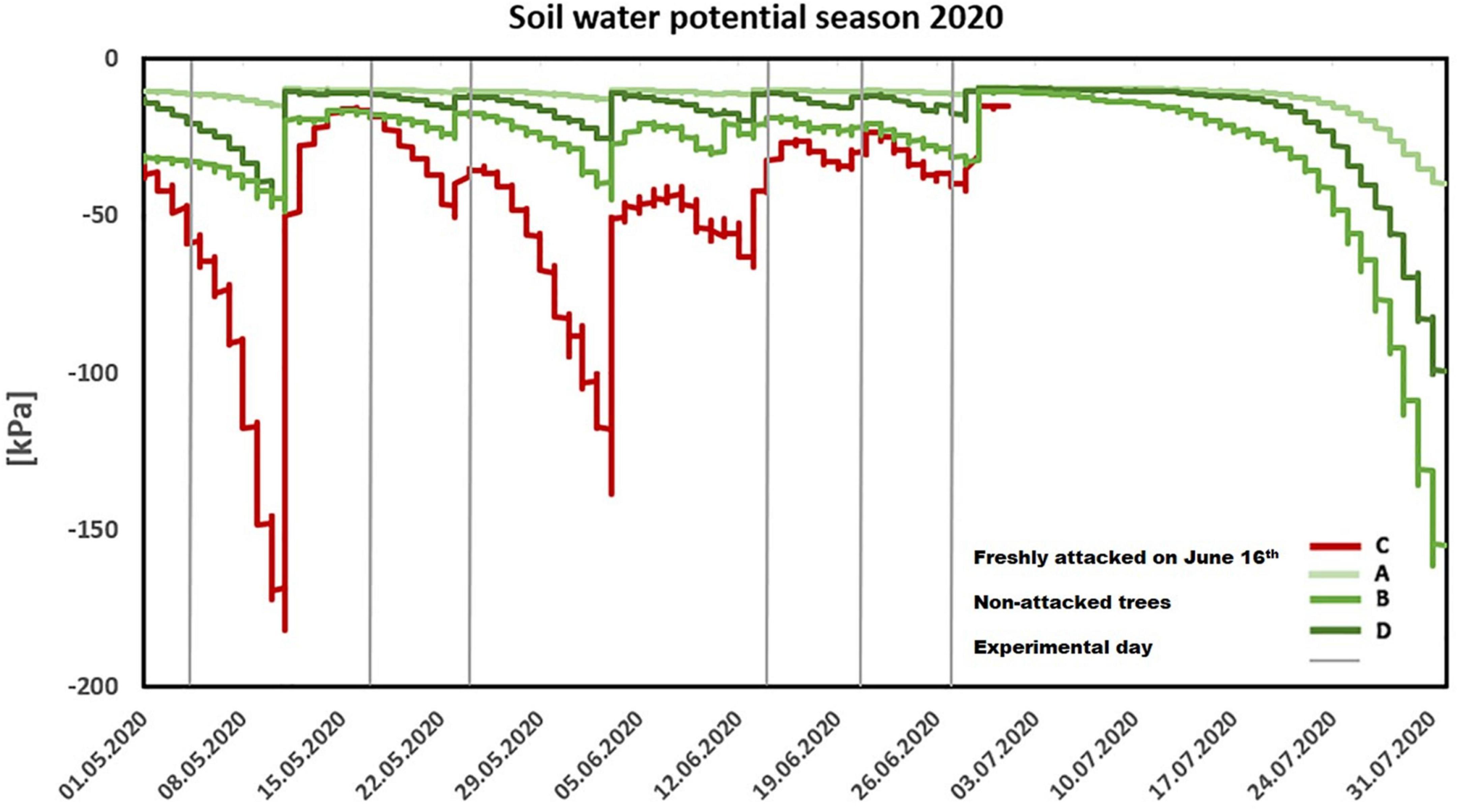
Figure 2. Seasonal trend of soil water potential (2020). Individual lines represent measurements through the season in freshly attacked plot C (red color)/the non-attacked plots A, B, and D (green color). The gray color (vertical line) represents individual experiments.
3.2. Physiological characteristics
The sap flow of trees in the monitored area (quantified as the sum of sap flow in kg per data sampling day (Figure 3A) did not show a significant difference in data sampling days from May to the date of beetle attack detection in plot C. On June 16th, the first week of infestation in plot C, sap flow significantly decreased in attacked trees (p = 0.035), and this difference was even more prominent in the next 2 weeks of advance infestation (p = 0.019 on June 24th and p = 0.010 on June 30th).
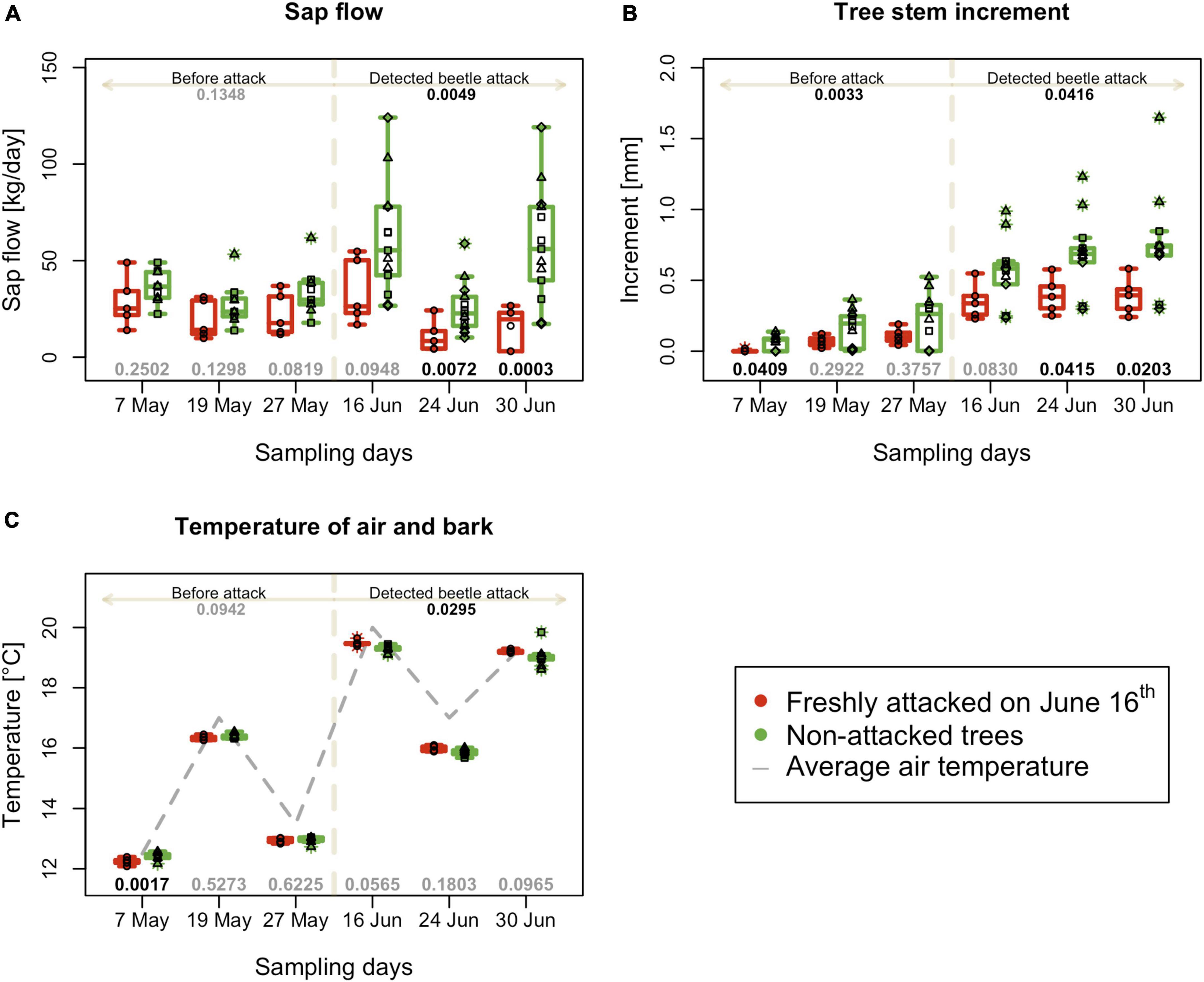
Figure 3. Physiological and physical characteristics of individual trees during the experimental period. (A) Mean daily sap flow. (B) Mean tree increment 1 week before sampling. (C) Mean bark surface temperatures on the north side of individual trees; boxplots summarize measurements on respective experimental days at the freshly attacked subplot C (red color) and control subplots A, B, and D (green color). Individual measurements are represented by circles (subplot C) and squares, triangles and diamonds for subplot A, B and C. Numbers on the top are p-values of differences between attacked and non-attacked plots calculated by post hoc Tukey analyses based on repeated measures GLMM separately for pre-attack and attack period; bottom numbers are p-values of differences between attacked and non-attacked plots estimated by post hoc Tukey analyses in the given sampling time. The dashed line represents the period when the bark beetle attack was detected (from June 16th).
Tree stem increment counted for the week prior to the data sampling days was lower for trees in plot C from the beginning of the season. After the second week of the bark beetle attack on June 24th, there was a significantly lower increment in infested trees than in non-infested, with a continuing trend in the following weeks (Figure 3B).
The bark surface temperatures measured on the north side of tree stems before the attack was detected, were equal on all monitored plots on May 19th and May 27th. Only on May 7th were temperature higher for plots A, B, and D, which later remained non-infested. On the monitoring day of June 16th, in the first week of the attack, the temperature of infested trees in plot C significantly increased (but the difference between infested and non-infested trees was only + 0.4°C) (Figure 3C). The average temperature of plot C in the period after infestation was 18.2°C, whereas that of the non-infested control was 17.8°C.
All the statistical details are listed in Supplementary Table 2.
3.3. Tree defense characteristics
The abundance of the sum of eight main spruce monoterpenes in the headspace close to the tree was similar in all monitored plots at the beginning of the season; however, after the bark beetle infestation of plot C on June 16th, their emission significantly increased in the vicinity of the affected trees and was significantly higher than in the non-infested trees for all three sampling days (Figure 4A). Nevertheless, monoterpene emissions declined during the third week of the attack. The progress of individual monoterpene emissions was similar to the progress of their sum (Supplementary Figure 2).
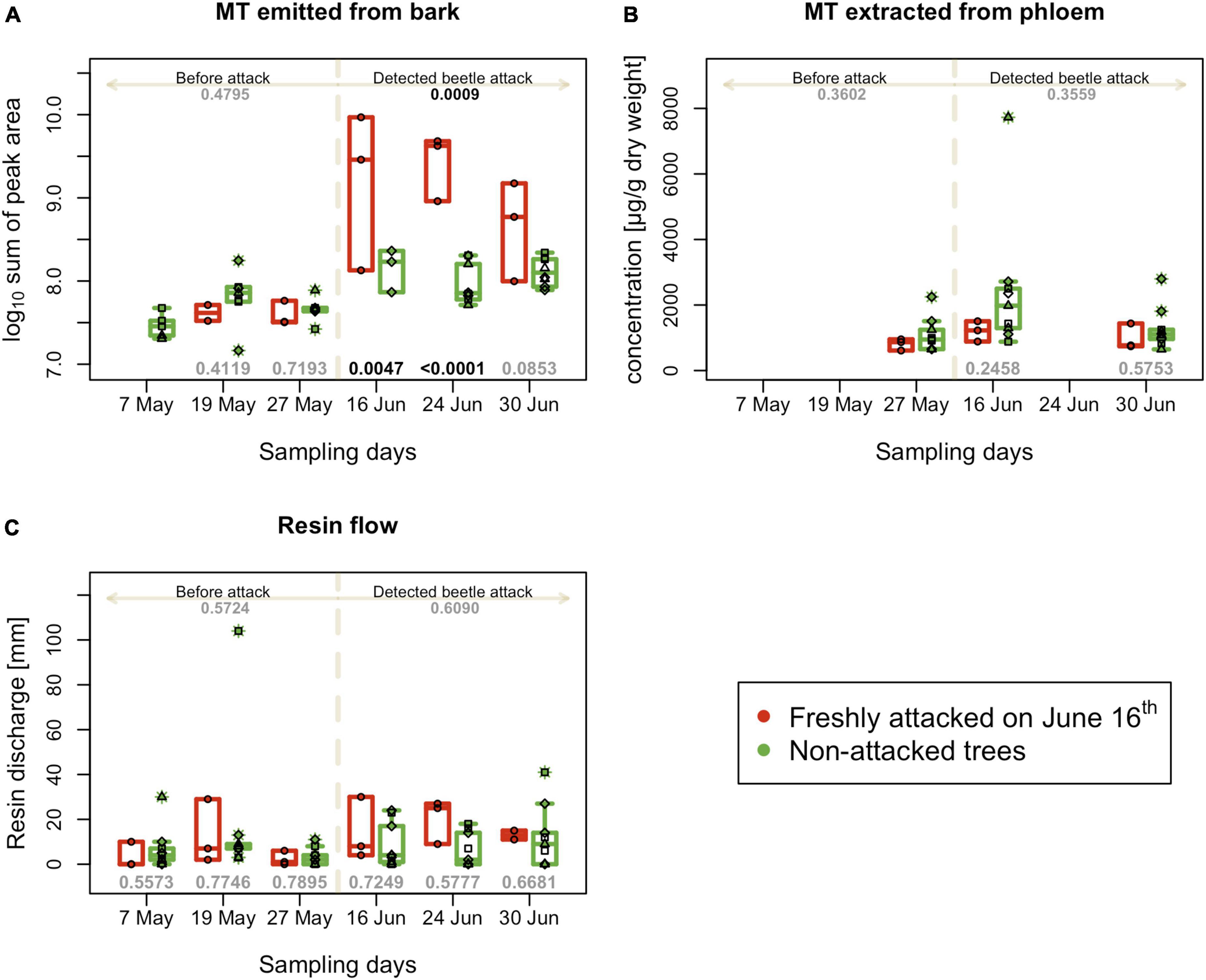
Figure 4. Tree defense characteristics recorded on individual trees on different experimental days. (A) Sum of peak areas of selected monoterpenes emitted by bark (B) Sum of peak selected monoterpenes extracted from phloem - performed three times per season; (C) resin flow per 24 h; boxplots summarize measurements on respective experimental days at the freshly attacked subplot C (red color) and control subplots A, B, and D (green color). Individual measurements are represented by circles (subplot C) and squares, triangles and diamonds for subplot A, B and C. Numbers on the top are p-values for differences between attacked and non-attacked plots calculated by post hoc Tukey analyses based on repeated measures GLMM separately for pre-attack and attack period; bottom numbers are p-values for differences between attacked and non-attacked plots estimated by post hoc Tukey analyses in the given sampling time. The dashed line represents the period when the bark beetle attack was detected (from June 16th).
The total content of monoterpenes extracted from the phloem of infested and non-infested trees did not differ significantly during the first and third weeks of bark beetle attack on June 16th and June 30th (Figure 4B). When focusing on individual monoterpenes, in the third week of the attack, statistically significant differences were found only in the content of Δ-carene, which increased, and camphene, which decreased in infested trees. The other monitored monoterpenes were less abundant in plot C, even before bark beetle infestation, and maintained the same trend after infestation on June 16th, but not significantly lower in infested plot compared to non-infested (Supplementary Figure 3).
The resin flow values recorded for individual trees showed a large variability; therefore, after the bark beetle attack, no significant differences were observed between infested and non-infested trees (Figure 4C). In the second week after attack detection (June 24th) was resin flow higher in infested trees, but this difference was not significant.
PCA score plots were created from metabolomic profiles recorded via GC-EI-TOF-MS from SPME-sampled volatiles on June 24th. The PCA (Figure 5A) explained 51% of the variance in data. A clear separation of two (from three) infested trees is shown. Following PLS-DA (Figure 5B) had parameters R2X(cum) = 0.48, R2Y(cum) = 0.97, Q2(cum) = 0.74, and connected variable importance plot revealed a higher abundance of terpinolene, α- and β-pinene, campholenal, limonene, sabinol, pinocamphone, and myrtenal, which had the highest importance for separation between the two classes of samples. Compounds of I. typographus aggregation pheromone, cis-verbenol, and 2-methyl-3-but-2-enol were not detected under the setup conditions on any experimental day.
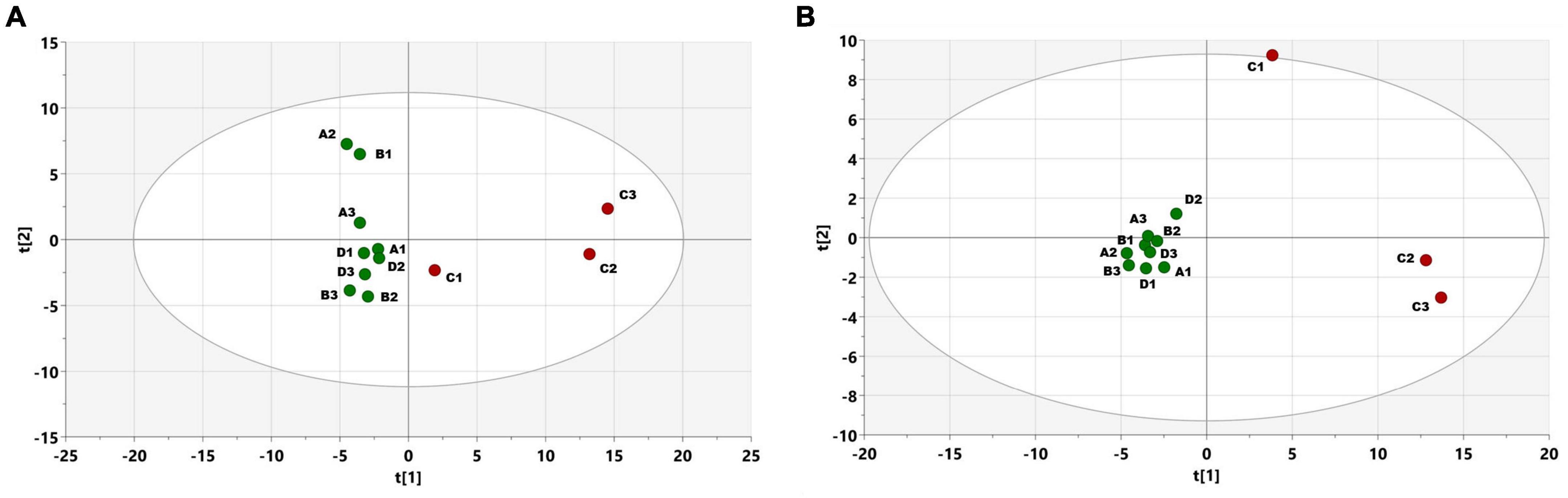
Figure 5. (A) PCA scores plot, showing the distribution of samples, according to the whole collected profile of volatiles, measured using GC-EI-TOF-MS. Individual points represent measurements of trees on June 24th in freshly attacked plot C (red color) with three trees/Non-attacked plots A- three trees, B-three trees, D-three trees (green color). Hoteling ellipse with significance level 0.05, t1 and t2 stand for the two most important components, together explaining 51% of the variance in data (B) PLS-DA scores plot showing the distribution of samples in the discriminant analysis model, using attacked vs. non-attacked as classes.
All the statistical details are listed in Supplementary Table 2.
3.4. Beetle catches in passive traps and beetle acceptance bioassays
The total number of beetles caught in passive traps was low for most of the observational period. Before the detection of the beetle attack, none or only few beetles (no more than one) were recorded. In the first week of the attack (June 16th), the number of beetles caught in traps mounted in the infested plot C increased steeply. The difference in the number of beetles caught in traps in the non-infested control plots A, B, and D was significant (p = 0.001) (Figure 6A).
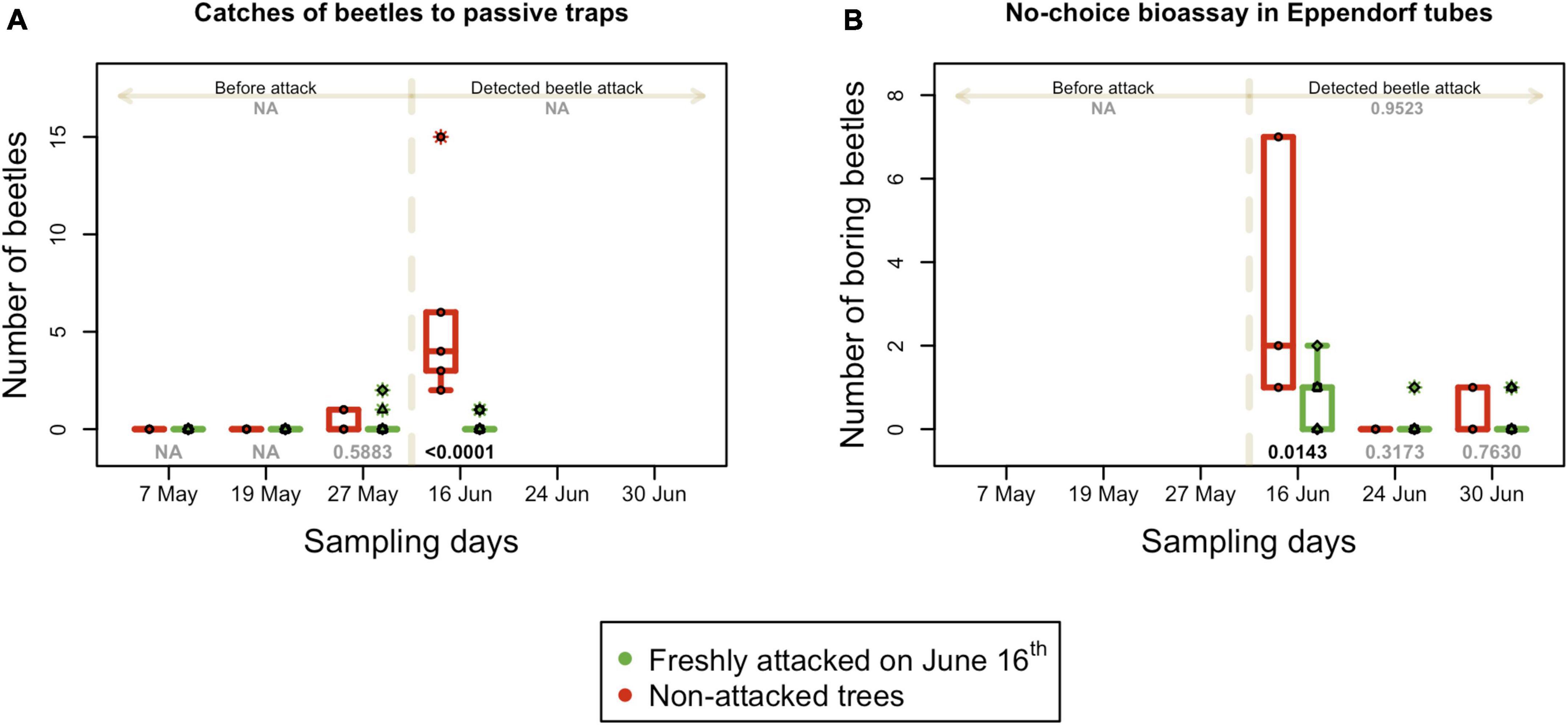
Figure 6. Absolute numbers of active I. typographus in the field. (A) Number of beetles caught in passive traps in the long-distance attraction bioassay. (B) Number of beetles that attacked trees in Eppendorf tubes in the no-choice bioassay; boxplots summarize measurements on respective experimental days at the freshly attacked subplot C (red color) and control subplots A, B, and D (green color). Individual measurements are represented by circles (subplot C) and squares, triangles and diamonds for subplot A, B, and C. Numbers on the top are p-values of differences between attacked and non-attacked plots calculated by post hoc Tukey analyses based on repeated measures GLMM separately for pre-attack and attack period; bottom numbers are p-values of differences between attacked and non-attacked plots estimated by post hoc Tukey analyses in the given sampling time. The dashed line represents the period when the bark beetle attack was detected (from June 16th).
The non-choice bioassay in Eppendorf tubes was performed three times per season after the detection of a beetle attack on June 16th. Therefore, all data from plot C were collected from infested trees and compared with non-infested trees in control plots A, B, and D. The number of beetles actively boring in the infested trees was significantly higher (p = 0.002) than that in non-infested trees in the first week of attack (Figure 6B). In the second and third weeks, bark beetle behavior was similar in both studied groups of trees. All the statistical details are listed in Supplementary Table 2.
4. Discussion
Previous studies have explored the connection between transpiration deficit (expressed as changes in sap flow) and tree defense ability in relation to bark beetle attack (Kirisits and Offenthaler, 2002; Matthews et al., 2018). Our study focuses on the characteristics of infested Norway spruce stands and reports a significant decrease in sap flow during the development of I. typographus infestation, consistent with previous findings (Wang, 1983). However, sap flow changes are variable and influenced by factors such as diurnal or seasonal rhythm (Nehemy et al., 2023), selective tree cutting (Özçelik et al., 2022), terrain shape, and soil properties affecting water supply (Netherer et al., 2015). Direct measurement of sap flow changes using the heat balance method (Čermák et al., 2004) for early detection of beetle attack is impractical due to technical, cost, and knowledge requirements. Further research is needed to develop more easily measurable indicators of sap flow changes.
Before infestation, the tree stem increment measured in plot C was lower compared to other plots, likely due to lower soil water potential recorded not only in the study season 2020 but also in the two previous seasons, 2018-2019. This relationship between water availability and stem increment in conifers has been previously reported (Ježík et al., 2015). However, we did not conclude that lower water availability in plot C caused the later bark beetle attack since the trees were not exposed to real drought stress because the soil water potential did not decrease below −1500 kPa (Lopushinsky and Klock, 1974; Brodribb et al., 2014). After infestation was detected, the growth of infested trees stopped while the healthy trees continued to grow, as the affected trees saved carbon resources for defense by limiting growth investment (Hartmann et al., 2013). Although tree stems increment changed significantly in infested trees, it is challenging to measure such small alterations in diameter (less than 1 mm) using basic dendrometry methods on the scale of a large forest area on the individual trees. Terrestrial laser scanning could provide a solution but is currently capable of monitoring seasonal increments greater than 1 mm in diameter (Yrttimaa et al., 2023).
The higher bark temperature recorded in plots A, B, and D before infestation most likely indicates that these plots were located in areas within the inner forests that received more sunlight. The bark surface temperature correlated with the air temperature overall (Stříbrská et al., 2022), but it increased significantly in affected trees after infestation. However, the average difference compared to the intact trees was only about 0.4°C, which is below the sensitivity threshold of the sensors. A study by Majdák et al. (2021) reported using an infrared-based thermo-camera to distinguish infested trees on forest edges. They found a significant difference in bark surface temperature (reaching tens of°C) only on the sun-exposed side of infested trees on days when air temperature reached 34°C and bark surface temperature was nearly 60°C. The temperature difference on the shaded side was lower, and on colder days (maximum air temperature of 24°C), it was not significant, which corresponds with our findings. Still, scanning the bark surface temperature from a distance using a Thermo camera can be considered a promising method for detecting infested trees. However, the methodology needs optimization, including using cameras with sufficient resolution, and measurements should be taken only in certain conditions on the sunny sides of stems on warm days with high sun radiation.
Resin exudation in the conifers, expressed as a measurement of resin flow, has been reported several times as a factor of the defense ability of trees against wood-boring insects (Turtola et al., 2003; Boone et al., 2011). However, resin flow varies significantly within a tree, limiting its usefulness as a defense marker. In our study, plot C had insignificantly higher resin flow after infestation, suggesting induced tree defense. However, the differences in resin flow between infested and intact trees were non-significant, making it an unreliable early attack detection marker. These findings align with the low incidence of resin flow as a visual symptom of I. typographus infestation (Kautz et al., 2023).
In volatiles collected close by infested and non-infested Norway spruce were the most abundant monoterpene fraction of resin, which was likely due to opening of constitutive resin storage. The main monoterpenes were α- and β-pinene in coelution with myrcene, Δ-carene, limonene, β-phellandrene and camphene, The summary emission of these compounds steeply increased in 1 week after infestation and remained higher till the end of the study similarly to previous findings by Ghimire et al. (2016) and Jaakkola et al. (2022). Notably, I. typographus pheromone 2-methyl-3-buten-2-ol and cis-verbenol were not detected in the overall headspace, probably because its content was below the detection limit of the used technique.
A detailed metabolomic study of the GC-EI-TOF-MS chromatograms of volatiles collected during the second week after infestation (June 24th) was conducted to identify compounds that may be significant for infestation. In addition to the major spruce monoterpenes mentioned earlier (namely terpinolene, α- and β-pinene, campholenal, and limonene), the infested trees were clustered based on a higher abundance of minor oxygenated monoterpenes (here sabinol, pinocamphone, and myrtenal) in two of the three infested trees. The increase of these compounds is a consequence of stress-related oxidations of spruce monoterpenes caused by the beetle attack and ophiostomatoid fungi infestation (Schiebe et al., 2019; Kandasamy et al., 2023). Even though internal cross-validation of the PLS-DA model provided a predictive power of 74%, owing to the lack of natural replication, the relative abundance of these compounds is only mentioned as a possible marker of tree infestation.
Despite the significant increase in the amount of emitted monoterpenes by infested trees, the content of the same monoterpenes extracted from the phloem does not increase significantly after infestation. This impairing has been previously reported by Hietz et al. (2005) and Stříbrská et al. (2022). It can be explained by the fact that while the immediate emission after tree infestation is due to the opening of constitutive resin storage, the tree response by the biosynthesis of defense compounds against herbivore attack in phloem requires a longer time. The content of major monoterpenes in the phloem, except Δ-carene, was found to be higher in intact trees even before the attack when these compounds were individually analyzed. This corresponds with the theory that higher content of toxic monoterpenes can make trees more resistant in certain circumstances (Raffa et al., 2016).
Based on these findings, there are two possible approaches for using volatile compound emissions as markers for early detection of tree infestation. The first approach involves using non-specific sensors that react to the concentration gradient of the most abundant compounds emitted by an infested tree. Pilot studies have already been carried out using an electronic nose with sensors based on metal oxidation or a change in electrical conductivity, showing promising potential for application in broad-scale volatile organic compound (VOC) scanning, including mounting on unmanned aerial vehicle (UAV) carriers (Paczkowski et al., 2021; Hüttnerová et al., 2023).
The second principle involves focusing on compounds specific to infested trees, such as oxygenated monoterpenes and I. typographus pheromones. However, the detection of these compounds requires sensitive and specific methods, such as a special mass detector (Juráò et al., 2017) or a special sampling device (Křůmal et al., 2016), which are not available for field testing yet.
Our observations from the non-choice assay align with research on bark beetle attack dynamics (Byers, 1989). According to this theory, male beetles show a preference for boring into trees that have already been attacked, particularly in the initial week of infestation when their conspecifics have already overcome the trees‘ defense abilities. However, as the infestation progresses and the resources of the trees are depleted, and the previously attacking beetles enter the mating and egg-laying stages, the beetles no longer prefer the previously infested trees. In the context of bark beetle management, the acceptance of trees determined in a non-choice assay is not suitable. However, it can still provide insights into the dynamics of bark beetle attacks.
On the other hand, passive traps have been shown to be potentially useful for early attack detection, as catching beetles significantly increases when trees are infested. The use of unbaited traps for bark beetle research is rather uncommon. Changes in the biodiversity of saproxylic beetles (including bark beetles) with respect to the age of the windblown trees were evaluated using a window trap by Wermelinger et al. (2002). The same type of trap as in our research was used by Schlyter et al. (1987a) for a behavioral study on I. typographus. The sensitivity of the trap and the fast-signaling of changes in the aggregation activity of I. typographus evidenced the suitability of this method for early attack detection research.
The present study had a limitation due to the small number of I. typographus attacked Norway spruce trees, which were monitored. However, despite this limitation, this study observed significant differences in physiological, defense biochemistry, and bark beetle acceptance characteristics between infested and non-infested trees.
We evaluated the potential use of the measured characteristics as a supporting research tool for developing early bark beetle attack detection methods through the following three approaches. The first is scanning the bark temperature of infested trees using a high-resolution thermal camera when used on days with high temperatures and sun irradiation. The second is monitoring the abrupt increase in the emission of defense monoterpenes using non-specific sensors, such as an electronic nose, with the possibility of carrying it on the UAV. Specific detection of spruce stress and I. typographus aggregation pheromone compounds can also be considered. The third is installing passive traps for the automatic detection of trapped or attacking beetles.
The determination of certain measurable characteristics of freshly infested trees can provide opportunities for developing alternative methods of early attack detection as a complement to the traditional and functional approach of early detection of infested trees through personal inspection of boring dust and entrance holes. Follow-up studies should focus on a deeper understanding of the physiological and defense mechanisms in relation to the detailed stage of bark beetle infestation. Future research is required to obtain practical outcomes that would lead to improved management of bark beetle outbreaks, especially the technical development of the solutions suggested in the present study.
Data availability statement
The raw data supporting the conclusions of this article will be made available by the authors, without undue reservation.
Author contributions
BS: experimental work, data curation, and writing draft. JH: GC-EI-TOF-MS analysis, statistical data processing, and editing. JČ: formal data analysis and statistical data processing. IT: physiological data collecting and handling. RM: conceptualization and investigation. AJ: conceptualization, data sampling, formal analysis, writing, editing, and supervision. All authors contributed to the article and approved the submitted version.
Funding
The project was funded by “EXTEMIT-K,” No. CZ.02.1.01/0.0/0.0/15_003/0000433, Ministry of Education, Youth and Sport, Operational Programme Research, Development and Education, Internal Grant Commission BS [IGA A_21_01] at the Faculty of Forestry and Wood Sciences, Czech University of Life sciences, Prague, Czech Republic, and EU project “UGC,” No. CZ.02.2.69/0.0/0.0/19_073/0016944 project number 75/2021 financed by OP RDE at the Czech University of Life Sciences, Prague, Czech Republic.
Acknowledgments
We acknowledge Jan Bílý, Jiří Synek, Ondřej Kalina, Rajarajan Ramakrishnan, Nicole Vošvrdová, Aleksei Trubin, Khodabakhsh Zabihi Afratakhti, Kristýna Římanová, Jaromír Bláha, Zdenĕk Rouč, Mallikarjuna Reddy JOGA, and Byrappa Ammagarahalli Munishamappa for their assistance in field experiments. We also acknowledge Daniel Tyšer for helping with the figure graphics. We thank management and foresters from Forests CZU in Kostelec nad Černými lesy for assistance and support.
Conflict of interest
The authors declare that the research was conducted in the absence of any commercial or financial relationships that could be construed as a potential conflict of interest.
Publisher’s note
All claims expressed in this article are solely those of the authors and do not necessarily represent those of their affiliated organizations, or those of the publisher, the editors and the reviewers. Any product that may be evaluated in this article, or claim that may be made by its manufacturer, is not guaranteed or endorsed by the publisher.
Supplementary material
The Supplementary Material for this article can be found online at: https://www.frontiersin.org/articles/10.3389/ffgc.2023.1197229/full#supplementary-material
References
Baier, P., Führer, E., Kirisits, T., and Rosner, S. (2002). Defence reactions of Norway spruce against bark beetles and the associated fungus Ceratocystis polonica in secondary pure and mixed species stands. For. Ecol. Manage. 159, 73–86. doi: 10.1016/S0378-1127(01)00711-3
Bates, D., Mächler, M., Bolker, B., and Walker, S. (2015). Fitting linear mixed-Effects models using lme4. J. Stat. Softw. 67, 1–48. doi: 10.18637/jss.v067.i01
Berini, J. L., Brockman, S. A., Hegeman, A. D., Reich, P. B., Muthukrishnan, R., Montgomery, R. A., et al. (2018). Combinations of abiotic factors differentially alter production of plant secondary metabolites in five woody plant species in the boreal-temperate transition zone. Front. Plant Sci. 9:1257. doi: 10.3389/fpls.2018.01257
Biedermann, P. H., Müller, J., Grégoire, J. C., Gruppe, A., Hagge, J., Hammerbacher, A., et al. (2019). Bark beetle population dynamics in the Anthropocene: Challenges and solutions. Trends Ecol. Evol. 34, 914–924. doi: 10.1016/j.tree.2019.06.002
Birgersson, G., Schlyter, F., Löfqvist, J., and Bergström, G. (1984). Quantitative variation of pheromone components in the spruce bark beetle Ips typographus from different attack phases. J. Chem. Ecol. 10, 1029–1055. doi: 10.1007/BF00987511
Boone, C. K., Aukema, B. H., Bohlmann, J., Carroll, A. L., and Raffa, K. F. (2011). Efficacy of tree defense physiology varies with bark beetle population density: A basis for positive feedback in eruptive species. Can. J. For. Res. 41, 1174–1188. doi: 10.1139/x11-041
Borg-Karlson, A. K., Lindström, M., Norin, T., Persson, M., and Valterová, I. (1993). Enantiomeric composition of monoterpene hydrocarbons in different tissues of Norway spruce, Picea abies (L) Karst. A multi-dimensional gas chromatography study. Acta Chem. Scand. 47, 138–144. doi: 10.3891/acta.chem.scand.47-0138
Brodribb, T. J., McAdam, S. A., Jordan, G. J., and Martins, S. C. (2014). Conifer species adapt to low-rainfall climates by following one of two divergent pathways. Proc. Natl. Acad. Sci. U.S.A. 111, 14489–14493. doi: 10.1073/pnas.1407930111
Byers, J. A. (1989). Chemical ecology of bark beetles. Experientia 45, 271–283. doi: 10.1007/BF01951813
Celedon, J. M., and Bohlmann, J. (2019). Oleoresin defenses in conifers: Chemical diversity, terpene synthases and limitations of oleoresin defense under climate change. New Phytol. 224, 1444–1463. doi: 10.1111/nph.15984
Čermák, J., Kučera, J., and Nadezhdina, N. (2004). Sap flow measurements with some thermodynamic methods, flow integration within trees and scaling up from sample trees to entire forest stands. Trees 18, 529–546. doi: 10.1007/s00468-004-0339-6
Christiansen, E., and Bakke, A. (1988). “The spruce bark beetle of Eurasia,” in Dynamics of forest insect populations: Patterns, causes, implications. Population Ecology, ed. A. A. Berryman Boston, MA: Springer, 479–503. doi: 10.1007/978-1-4899-0789-9_23
Christiansen, E., and Horntvedt, R. (1983). Combined Ips/Ceratocystis attack on Norway spruce, and defensive mechanisms of the trees. Zeitschrift Angew. Entomol. 96, 110–118. doi: 10.1111/j.1439-0418.1983.tb03650.x
Cocozza, C., Palombo, C., Tognetti, R., La Porta, N., Anichini, M., Giovannelli, A., et al. (2016). Research paper Monitoring intra-annual dynamics of wood formation with microcores and dendrometers in Picea abies at two different altitudes. Tree Physiol. 36, 832–846. doi: 10.1093/treephys/tpw009
Erbilgin, N. (2019). Phytochemicals as mediators for host range expansion of a native invasive forest insect herbivore. New Phytol. 221, 1268–1278. doi: 10.1111/nph.15467
Erbilgin, N., Krokene, P., Kvamme, T., and Christiansen, E. (2007). A host monoterpene influences Ips typographus (Coleoptera: Curculionidae, Scolytinae) responses to its aggregation pheromone. Agric. For. Entomol. 9, 135–140. doi: 10.1111/j.1461-9563.2007.00329.x
Franceschi, V. R., Krokene, P., Christiansen, E., and Krekling, T. (2005). Anatomical and chemical defenses of conifer bark against bark beetles and other pests. New Phytol. 167, 353–376. doi: 10.1111/j.1469-8137.2005.01436.x
Gebhardt, T., Hesse, B. D., Hikino, K., Kolovrat, K., Hafner, B. D., Grams, T. E. E., et al. (2023). Agricultural and forest meteorology repeated summer drought changes the radial xylem sap flow profile in mature Norway spruce but not in European beech. Agric. For. Meteorol. 329:109285. doi: 10.1016/j.agrformet.2022.109285
Ghimire, R. P., Kivimäenpää, M., Blomqvist, M., Holopainen, T., Lyytikäinen-Saarenmaa, P., and Holopainen, J. K. (2016). Effect of bark beetle (Ips typographus L.) attack on bark VOC emissions of Norway spruce (Picea abies Karst.) trees. Atmos. Environ. 126, 145–152. doi: 10.1016/j.atmosenv.2015.11.049
Hartmann, H., Ziegler, W., Kolle, O., and Trumbore, S. (2013). Thirst beats hunger–declining hydration during drought prevents carbon starvation in Norway spruce saplings. New Phytol. 200, 340–349. doi: 10.1111/nph.12331
Hietz, P., Baier, P., Offenthaler, I., Führer, E., Rosner, S., and Richter, H. (2005). Tree temperatures, volatile organic emissions, and primary attraction of bark beetles. Phyton Ann. Rei Bot. 45, 341–354.
Hlásny, T., Barka, I., Merganičová, K., Křístek, Š, Modlinger, R., Turčáni, M., et al. (2022). A new framework for prognosing forest resources under intensified disturbance impacts: Case of the Czech Republic. For. Ecol. Manage. 523:120483. doi: 10.1016/j.foreco.2022.120483
Hlásny, T., Krokene, P., Liebhold, A., Montagné-Huck, C., Müller, J., Qin, H., et al. (2019). Living with bark beetles: Impacts, outlook and management options. Sci. Policy. 8:53. doi: 10.36333/fs08
Hlásny, T., Zimová, S., Merganičová, K., Štĕpánek, P., Modlinger, R., and Turčáni, M. (2021a). Devastating outbreak of bark beetles in the Czech Republic: Drivers, impacts, and management implications. For. Ecol. Manage. 490:119075. doi: 10.1016/j.foreco.2021.119075
Hlásny, T., König, L., Krokene, P., Lindner, M., Montagné-Huck, C., Müller, J., et al. (2021b). Bark beetle outbreaks in Europe: State of knowledge and ways forward for management. Curr. For. Rep. 7, 138–165. doi: 10.1007/s40725-021-00142-x
Huang, J., Kautz, M., Trowbridge, A. M., Hammerbacher, A., Raffa, K. F., Adams, H. D., et al. (2020). Tree defence and bark beetles in a drying world: Carbon partitioning, functioning and modelling. New Phytol. 225, 26–36. doi: 10.1111/nph.16173
Huo, L., Persson, H. J., and Lindberg, E. (2021). Remote Sensing of Environment Early detection of forest stress from European spruce bark beetle attack, and a new vegetation index: Normalized distance red & SWIR (NDRS). Remote Sensing Environ. 255:112240. doi: 10.1016/j.rse.2020.112240
Hüttnerová, T., Paczkowski, S., Neubert, T., Jirošová, A., and Surový, P. (2023). Comparison of individual sensors in the electronic nose for stress detection in forest stands. Sensors 23:2001. doi: 10.3390/s23042001
Jaakkola, E., Gärtner, A., Jönsson, A. M., Olsson, K. L. P., and Holst, T. (2022). Spruce bark beetle (Ips typographus) infestation cause up to 700 times higher bark BVOC emission rates from Norway spruce (Picea abies). Biogeosciences 20, 803–826. doi: 10.5194/bg-2022-125
Ježík, M., Blaženec, M., Letts, M. G., Ditmarová, L’, Sitková, Z., and Střelcová, K. (2015). Assessing seasonal drought stress response in Norway spruce (Picea abies (L.) Karst.) by monitoring stem circumference and sap flow. Ecohydrology 8, 378–386. doi: 10.1002/eco.1536
Johansson, A., Birgersson, G., and Schlyter, F. (2019). Using synthetic semiochemicals to train canines to detect bark beetle–infested trees. Ann. For. Sci. 76:58. doi: 10.1007/s13595-019-0841-z
Jurán̆, S., Pallozzi, E., Guidolotti, G., Fares, S., Šigut, L., Calfapietra, C., et al. (2017). Fluxes of biogenic volatile organic compounds above temperate Norway spruce forest of the Czech Republic. Agric. For. Meteorol. 232, 500–513. doi: 10.1016/j.agrformet.2016.10.005
Kandasamy, D., Zaman, R., Nakamura, Y., Zhao, T., Hartmann, H., Andersson, M. N., et al. (2023). Conifer-killing bark beetles locate fungal symbionts by detecting volatile fungal metabolites of host tree resin monoterpenes. PLoS Biol. 21:e3001887. doi: 10.1371/journal.pbio.3001887
Kännaste, A., Zhao, T., Lindström, A., Stattin, E., Långström, B., and Borg-Karlson, A. K. (2013). Odors of Norway spruce (Picea abies L.) seedlings: Differences due to age and chemotype. Trees 27, 149–159. doi: 10.1007/s00468-012-0783-7
Kausrud, K., Økland, B., Skarpaas, O., Grégoire, J. C., Erbilgin, N., and Stenseth, N. C. (2012). Population dynamics in changing environments: The case of an eruptive forest pest species. Biol. Rev. 87, 34–51. doi: 10.1111/j.1469-185X.2011.00183.x
Kautz, M., Peter, F. J., Harms, L., Kammen, S., and Delb, H. (2023). Patterns, drivers and detectability of infestation symptoms following attacks by the European spruce bark beetle. J. Pest Sci. 96, 403–414. doi: 10.1007/s10340-022-01490-8
Kirisits, T., and Offenthaler, I. (2002). Xylem sap flow of Norway spruce after inoculation with the blue-stain fungus Ceratocystis polonica. Plant Pathol. 51, 359–364. doi: 10.1046/j.1365-3059.2002.00722.x
Klinovský, M. (2021). Letová aktivita kalamitních druhù kùrovcù (Coleoptera: Scolytinae) v závislosti na sumì efektivních teplot. Ph.D. thesis. Prague: Czech University of Life Sciences Prague.
Kopaczyk, J. M., Warguła, J., and Jelonek, T. (2020). The variability of terpenes in conifers under developmental and environmental stimuli. Environ. Exp. Bot. 180:104197. doi: 10.1016/j.envexpbot.2020.104197
Křůmal, K., Mikuška, P., Večeřová, K., Urban, O., Pallozzi, E., and Večeřa, Z. (2016). Wet effluent diffusion denuder: The tool for determination of monoterpenes in forest. Talanta 153, 260–267. doi: 10.1016/j.talanta.2016.03.032
Lawlor, D. W., and Cornic, G. (2002). Photosynthetic carbon assimilation and associated metabolism in relation to water deficits in higher plants. Plant Cell Environ. 25, 275–294. doi: 10.1046/j.0016-8025.2001.00814.x
Lehmanski, L. M., Kandasamy, D., Andersson, M. N., Netherer, S., Alves, E. G., Huang, J., et al. (2023). Addressing a century old hypothesis–do pioneer beetles of Ips typographus use volatile cues to find suitable host trees? New Phytol. 238, 1762–1770. doi: 10.1111/nph.18865
Lenth, R. V. (2016). Least-squares means: The R package lsmeans. J. Stat. Softw. 69, 1–33. doi: 10.18637/jss.v069.i01
Leuzinger, S., and Korner, C. (2007). Tree species diversity affects canopy leaf temperatures in a mature temperate forest. Agric. For. 146, 29–37. doi: 10.1016/j.agrformet.2007.05.007
Lopushinsky, W., and Klock, G. O. (1974). Transpiration of conifer seedlings in relation to soil water potential. For. Sci. 20, 181–186. doi: 10.1093/forestscience/20.2.181
Majdák, A., Jakuš, R., and Blaženec, M. (2021). Determination of differences in temperature regimes on healthy and bark-beetle colonised spruce trees using a handheld thermal camera. iForest-Biogeosci. For. 14, 203–211. doi: 10.3832/ifor3531-014
Marini, L., Økland, B., Jönsson, A. M., Bentz, B., Carroll, A., Forster, B., et al. (2017). Climate drivers of bark beetle outbreak dynamics in Norway spruce forests. Ecography 40, 1426–1435. doi: 10.1111/ecog.02769
Matthews, B., Netherer, S., Katzensteiner, K., Pennerstorfer, J., Blackwell, E., Henschke, P., et al. (2018). Transpiration deficits increase host susceptibility to bark beetle attack: Experimental observations and practical outcomes for Ips typographus hazard assessment. Agric. For. Meteorol. 263, 69–89. doi: 10.1016/j.agrformet.2018.08.004
Nehemy, M. F., Pierrat, Z., Maillet, J., Richardson, A. D., Stutz, J., Johnson, B., et al. (2023). Phenological assessment of transpiration: The stem-temp approach for determining start and end of season. Agric. For. Meteorol. 331:109319. doi: 10.1016/j.agrformet.2023.109319
Netherer, S., Kandasamy, D., Jirosová, A., Kalinová, B., Schebeck, M., and Schlyter, F. (2021). Interactions among Norway spruce, the bark beetle Ips typographus and its fungal symbionts in times of drought. J. Pest Sci. 94, 591–614. doi: 10.1007/s10340-021-01341-y
Netherer, S., Matthews, B., Katzensteiner, K., Blackwell, E., Henschke, P., Hietz, P., et al. (2015). Do water-limiting conditions predispose Norway spruce to bark beetle attack? New Phytol. 205, 1128–1141. doi: 10.1111/nph.13166
Offenthaler, I., Hietz, P., and Richter, H. (2001). Wood diameter indicates diurnal and long-term patterns of xylem water potential in Norway spruce. Trees 15, 215–221. doi: 10.1007/s004680100090
Özçelik, M. S., Tomášková, I., Surový, P., and Modlinger, R. (2022). Effect of forest edge cutting on transpiration rate in Picea. Forests 13:1238. doi: 10.3390/f13081238
Paczkowski, S., Datta, P., Irion, H., Paczkowska, M., Habert, T., Pelz, S., et al. (2021). Evaluation of early bark beetle infestation localization by drone-based monoterpene detection. Forests 12:228. doi: 10.3390/f12020228
Powell, J. M. (1967). A study of habitat temperatures of the bark beetle Dendroctonus ponderosae Hopkins in lodgepole pine. Agric. Meteorol. 4, 189–201.
R Core Team (2022). R: A language and environment for statistical computing. Vienna: R Foundation for Statistical Computing.
Raffa, K. F., Andersson, M. N., and Schlyter, F. (2016). Host selection by Bark Beetles: Playing the odds in a high-stakes game. Insect Physiol. 50, 1–74. doi: 10.1016/bs.aiip.2016.02.001
Ramakrishnan, R., Hradecký, J., Roy, A., Kalinová, B., Mendezes, R. C., Synek, J., et al. (2022). Metabolomics and transcriptomics of pheromone biosynthesis in an aggressive forest pest Ips typographus. Insect Biochem. Mol. Biol. 140:103680. doi: 10.1016/j.ibmb.2021.103680
Schiebe, C., Unelius, C. R., Ganji, S., Binyameen, M., Birgersson, G., and Schlyter, F. (2019). Styrene, (+)-trans-(1R,4S,5S)-4-Thujanol and oxygenated monoterpenes related to host stress elicit strong electrophysiological responses in the bark beetle Ips typographus. J. Chem. Ecol. 45, 474–489. doi: 10.1007/s10886-019-01070-8
Schlyter, F., Birgersson, G., Byers, J. A., Löfqvist, J., and Bergström, G. (1987a). Field response of spruce bark beetle, Ips typographus, to aggregation pheromone candidates. J. Chem. Ecol. 13, 701–716.
Schlyter, F., Lofqvist, J. A. N., and Byers, J. A. (1987b). Behavioural sequence in the attraction of the bark beetle Ips typographus to pheromone sources. Physiol. Entomol. 12, 185–196.
Schroeder, L. M. (1990). Duct resin flow in Scots pine in relation to the attack of the bark beetle Tomicus piniperda (L.) (Col., Scolytidae). J. Appl. Entomol. 109, 105–112. doi: 10.1111/j.1439-0418.1990.tb00026.x
Seidl, R., Müller, J., Hothorn, T., Bässler, C., Heurich, M., and Kautz, M. (2016). Small beetle, large-scale drivers: How regional and landscape factors affect outbreaks of the European spruce bark beetle. J. Appl. Ecol. 53, 530–540. doi: 10.1111/1365-2664.12540
Střelcová, K., Kurjak, D., Leštianska, A., Kovalčíková, D., Ditmarová, L´, Škvarenina, J., et al. (2013). Differences in transpiration of Norway spruce drought stressed trees and trees well supplied with water. Biologia 68, 1118–1122. doi: 10.2478/s11756-013-0257-4
Stříbrská, B., Hradecký, J., Čepl, J., Tomášková, I., Jakuš, R., Modlinger, R., et al. (2022). Forest margins provide favourable microclimatic niches to swarming bark beetles, but Norway spruce trees were not attacked by Ips typographus shortly after edge creation in a field experiment. For. Ecol. Manage. 506:119950. doi: 10.1016/j.foreco.2021.119950
Turčáni, M., and Nakládal, O. (2007). The results of manipulated experiments with inoculation of Ips typographus (L., 1758) to spruce trees under various levels of water stress. J. For. Sci. 53, 25–30. doi: 10.17221/2159-jfs
Turtola, S., Manninen, A. M., Rikala, R., and Kainulainen, P. (2003). Drought stress alters the concentration of wood terpenoids in Scots pine and Norway spruce seedlings. J. Chem. Ecol. 29, 1981–1995. doi: 10.1023/A:1025674116183
Vošvrdová, N., Johansson, A., Turčáni, M., Jakuš, R., Tyšer, D., Schlyter, F., et al. (2023). Dogs trained to recognise a bark beetle pheromone locate recently attacked spruces better than human experts. For. Ecol. Manage. 528:120626. doi: 10.1016/j.foreco.2022.120626
Wang, S. (1983). Transpiration stream velocity in Norway spruce trees attacked by bark beetles. Rep. Norwegian For. Res. Inst. 38:8.
Wermelinger, B., Duelli, P., and Obrist, M. K. (2002). Dynamics of saproxylic beetles (Coleoptera) in windthrow areas in alpine spruce forests. For. Snow Landscape Res. 77, 133–148.
Yrttimaa, T., Junttila, S., Luoma, V., Calders, K., Kankare, V., Saarinen, N., et al. (2023). Capturing seasonal radial growth of boreal trees with terrestrial laser scanning. For. Ecol. Manage. 529:120733. doi: 10.1016/j.foreco.2022.120733
Keywords: green attack, tree defence, bark beetles, Picea abies, tree physiology, VOC, sap flow, dendrometer
Citation: Stříbrská B, Hradecký J, Čepl J, Modlinger R, Tomášková I and Jirošová A (2023) Physiological and biochemical indicators in Norway spruces freshly infested by Ips typographus: potential for early detection methods. Front. For. Glob. Change 6:1197229. doi: 10.3389/ffgc.2023.1197229
Received: 30 March 2023; Accepted: 28 June 2023;
Published: 12 July 2023.
Edited by:
Barry Alan Gardiner, Institut Européen de la Forêt Cultivée (IEFC), FranceReviewed by:
Jennifer Gene Klutsch, University of Alberta, CanadaLuis Sampedro, Spanish National Research Council (CSIC), Spain
Copyright © 2023 Stříbrská, Hradecký, Čepl, Modlinger, Tomášková and Jirošová. This is an open-access article distributed under the terms of the Creative Commons Attribution License (CC BY). The use, distribution or reproduction in other forums is permitted, provided the original author(s) and the copyright owner(s) are credited and that the original publication in this journal is cited, in accordance with accepted academic practice. No use, distribution or reproduction is permitted which does not comply with these terms.
*Correspondence: Anna Jirošová, amlyb3NvdmFhQGZsZC5jenUuY3o=
†These authors have contributed equally to this work