- 1Institute of Pure and Applied Biology, Bahauddin Zakariya University, Multan, Pakistan
- 2Department of Environmental Sciences, Kohsar University Murree, Murree, Pakistan
- 3School of Plant Sciences, University of Arizona, Tucson, AZ, United States
- 4Department of Botany and Microbiology, College of Science, King Saud University, Riyadh, Saudi Arabia
- 5Bangor College China, A Joint Unit of Bangor University and Central South University of Forestry and Technology, Changsha, Hunan, China
- 6Key Laboratory for Sustainable Forest Ecosystem Management-Ministry of Education, College of Forestry, Northeast Forestry University, Harbin, China
- 7Division of Science and Technology, Department of Botany, University of Education, Lahore, Pakistan
The moist temperate forests extend along the whole length of outer ranges of Himalaya between the subtropical pine forests and sub-alpine timberline formation with a rainfall from 400 mm to 800 mm. The altitudinal range is from 1500 m to 3000 m. The floristic variation in these Himalayan forests is poorly understood especially in the study area. Species composition may be either unpredictable or it may correspond to environmental heterogeneity. Vegetation from 144 stands in between 2000 – 2700 m altitude was sampled. Soil samples were collected to document edaphic conditions. Soils were physically and chemically analyzed. Ordination (DECORANA) and classificatory techniques were used to analyze the vegetation data. No clear disjunct vegetation patterns emerged from these analyses. The major axes brought out by the ordination were related to altitude and although it is possible to relate the units of classification to broad soil types. Soil chemical properties, litter cover and rockiness were significantly associated with the vegetation variation along an altitudinal gradient. The application of classification to the ordination allowed the interpretations of the vegetation variation in terms of topography and predictable climatic factors such as rainfall, wind speed and extent of snow accumulation. The vegetation patterns revealed have been discussed in relation to the general problem of plant community definition in continuous forest type. Our results demonstrate the overlapping rather than clearly discrete boundaries between the vegetation types and species distributions. This overlapping nature of the vegetation types are discussed in terms of overlapping environmental preferences of the species. Our results are consistent with notion that species separate edaphically and landscape scale within the uniform looking forest. This view maintains that differences in soil within the forest are distinct enough to favor different species, and thus create numerous floristically differentiated forest patches along the altitudinal gradients. The findings that non obvious but distinct floristic and edaphic variation exist within the Himalayan moist temperate forests at the landscape scale have important practical implications for forest management. In biodiversity conservation a high degree of habitat heterogeneity implies an increased need for wide-scale information on species distribution and endemism patterns to better assess where the different habitats are, which species they harbor, and where conservation efforts should be concentrated. The overlapping nature of vegetation types and hazy boundaries of the plant communities implies that plant ecologists must continue to attempt the difficult definition of hazy boundaries.
1. Introduction
In recent decades, vegetation ecologists have become increasingly interested in recording and understanding mountain vegetation species composition and spatial structure. Owing to the interference of several influences in the high mountain vegetation pattern, the driving factors of the vegetation pattern always remain a topic of debate (Wazir et al., 2008; Saima et al., 2009; Khan et al., 2011, 2012).
In mountain landscapes, altitude is the most important factor that influences habitats, climate, and flora contrasts. Altitude represents a complex combination of related variables such as topography, aspect, soil types, and other environmental features that further affect vegetation composition (Dasti et al., 2007; Wazir et al., 2008; Saima et al., 2009). Ellu and Obua (2005) described how different altitude and slope aspects encounter species diversity. Kharkwal et al. (2005) pointed out that the elevation gradient and its linked geo-climatic variables like precipitation and temperature determine species diversity and community composition. Topographic heterogeneity is also considered an important factor in temperate mountain landscapes that shape phytodiversity, as they strongly influence the length of the growing season related to temperature (Dasti et al., 2007; Wazir et al., 2008; Saima et al., 2009). Downslope movement and distribution of soil water contents depend on the ratio of a rocky surface area to soil volume and the spatial variation in runoff pattern. Redistribution of rainfall water by runoff from some areas depends on rainfall intensity and the surface's infiltration rate.
In most Himalayan forests, runoff accumulation occurs at various scales (Yair and Shahak, 1982; Wazir et al., 2008). Thus, niches and habitats of various kinds and sizes are formed, which determine the structure and composition of vegetation (Orshan, 1986). The distribution patterns of runoff determine the differences in vegetation type and influence plant assemblage (Dasti et al., 2007; Wazir et al., 2008). Besides the runoff pattern, strong winds and snowpack distribution at high altitudes significantly shape plant communities (Tanner et al., 1990; Ahmad et al., 2006; Wazir et al., 2008). The results of previous studies (Tanner et al., 1998; Wazir et al., 2008; Saima et al., 2009; Khan et al., 2012) showed continuous variations in composition across a broad range from lower blue pine canopied forest to upper, relatively open Abies pindrow formation.
We used modern data analysis techniques to assess the compositional patterns of plant communities in such a complex landscape. These approaches help summarize the gradients in data sets, assisting in formulating hypotheses and testing their validity (Odgaard and Rasmussen, 2000). Many studies have explored variations in species diversity and soil nutrient concentrations along altitudinal gradients using numerical techniques (Khan et al., 2011; Ping et al., 2013; Saima et al., 2018). Sharma et al. (2009) described the species distribution pattern in India's Garhwal forest of the Himalayas. Bhattarai et al. (2014) investigated species diversity in Nepal's Karnali River Valley in the Himalayas. Shaheen et al. (2011) studied species diversity, community structure, and distribution patterns in Kashmir, Pakistan's Western Himalayan Alpine pasture. Ummara et al. (2013) quantitatively analyzed understory vegetation from only 22 different Shogran Valley reserve forest sites. Raja et al. (2014) described the floristic composition of Ayubia National Park from 27 sampling stands (sites). Jadoon et al. (2017) described ecological gradient analyses of plant associations in the Thandiani reserve forests of the Western Himalayas, Pakistan, using 50 sampling stands. Saima et al. (2018) conducted research to quantify the diversity of species and boundaries of plant communities along the altitudinal gradient in the Shogran Valley reserve forest, while Rahman et al. (2019) explored the floristic diversity of Manoor Valley (Naran). Qadir et al. (2020) researched the anthropogenic effects on natural plant biodiversity distribution and diversity patterns, particularly along the sides of the road in pine forests. Noor et al. (2021) explored the structure and vegetation composition of the forest located in Murree Hills, Punjab, Pakistan. Ahmad et al. (2021) studied the structure and vegetation composition of the Himalayans' moist temperate forest, Kaghan Valley. In other parts of the world, Wani et al. (2023) conducted research to quantify the diversity and compositional patterns in the Kashmir Himalaya.
Although all these studies add significant literature to the topic, studies related to species diversity and richness using a multivariate analysis of the study area are missing and confined to the single forest type due to its remote location and inaccessibility of tracks. Moreover, no information is available regarding a long transect along the elevation gradient from low-level blue pine forests to upper timberline fir forests in these temperate mountain areas. Besides these, we have attempted to elucidate how vegetation changes along the pronounced environmental gradient across the three reserved forest types covering an area of 3,500 Km2. We aimed to relate vegetation distribution to topography and soils along an altitudinal gradient and examine some environmental factors for their correlation with the vegetation pattern in our study area.
2. Materials and methods
2.1. Study area
The valley of Kaghan (34.5417° N, 73.3500° E) is located in the mountains of the Western Himalayas, Pakistan with an elevation range of 650–4,170 m a.s.l (Figure 1). The valley is enveloped by the lower Himalayan mountain range, resulting in an alpine climate and the prevalence of pine forests and alpine meadows. The natural range of temperate forests covers an extensive area of the northwestern Himalayas in Pakistan, India, China, Nepal, and Bhutan. The area is located on gneisses and schists, extending over granites, limestones, and quartzite of the Precambrian age (Champion et al., 1965). The region's topography is a mosaic of rocky hills, rock outcrops, wetlands, and glaciated lakes of varying sizes (Hussain and Illahi, 1991).
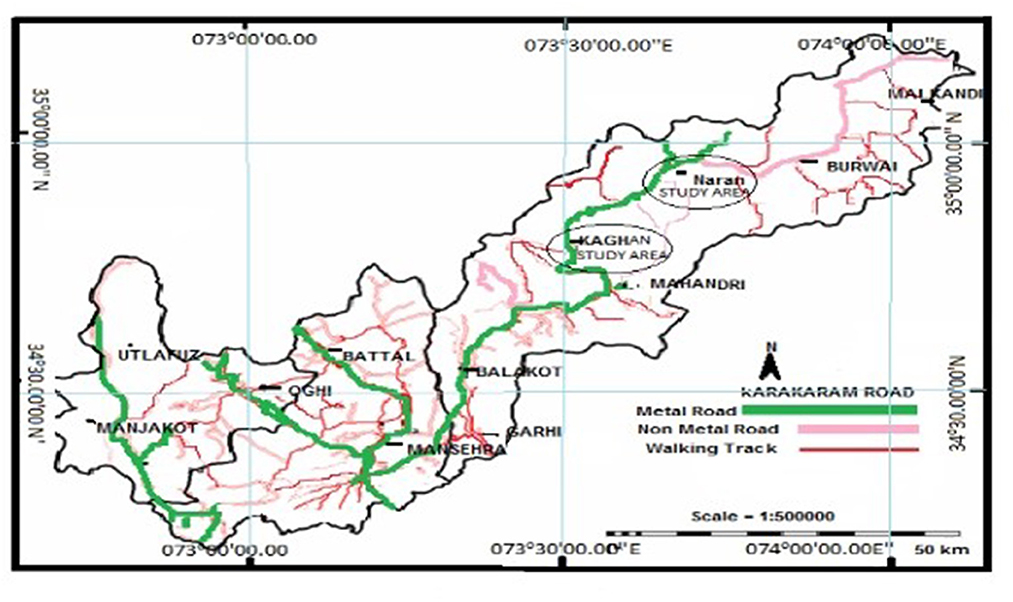
Figure 1. A map of the study area depicting sampling localities in circles. Sampling sites for lower Western Himalaya temperate forests (low-level blue pine forests), mid-Cedrus deodara forests, Kaghan, and upper-west Himalayan fir forests (upper Abies pindrow forests, Naran) are shown in circles.
The environment varies widely along the altitudinal gradient (Table 1). There is a considerable year-to-year climatic variation, especially in rainfall and winter snow accumulation. The overall climate of the study area is classified as temperate continental, with an average frost-free growing period of 204 days from April to October. The average annual precipitation for the area is 1,200 mm, with 65% falling as rain from May to August. However, an appreciable amount is brought in during the winter and spring. On average, precipitation is greater at low altitudes than at high altitudes, while temperatures show the opposite trend (Figures 2, 3).
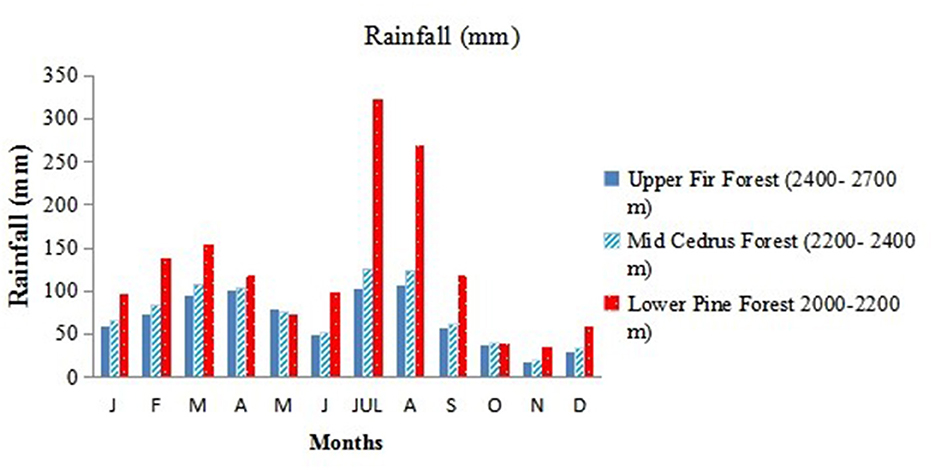
Figure 2. Mean monthly rainfall distribution of three forest types: low-level blue pine forests (lower pine forests), mid-Cedrus deodara forests (mid-Cedrus forests), and upper Abies pindrow forest (upper fir forests) along an altitudinal gradient.
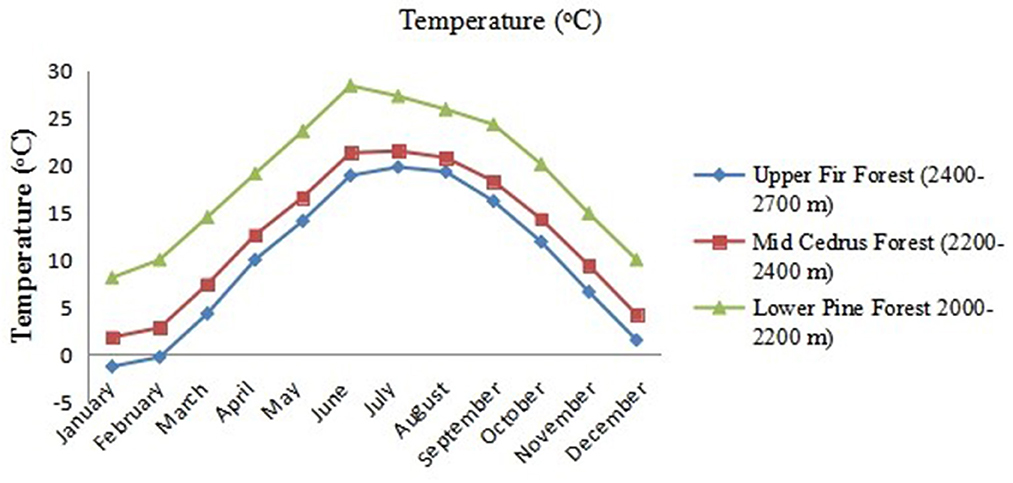
Figure 3. The mean monthly temperature of three forest types: low-level blue pine forests (lower pine forests), mid-Cedrus deodara forests (mid-Cedrus forests), and upper Abies pindrow forests (upper fir forests) along an altitudinal gradient.
In the study area, there are certain dry inner valleys that remain unaffected by the monsoon, leading to the transition to dry temperate forests. In these areas, the snowmelt provides sufficient moisture to sustain the soil during the summer months. Most of the trees and shrubs found in the outer regions of the moist temperate forests do not extend into the inner dry valleys. Overall, the vegetation in these areas undergoes significant changes, warranting a distinct classification of dry and wet temperate forests separately. However, it is important to note that there is no definitive boundary between the moist and dry temperate forests as they gradually transition into one another.
The moist temperate conifer forests in Pakistan (N 34° 38.38′ latitudes and E 73° 33.11′ longitudes) lie within an elevation range of 1,500 to 3,000 m above sea level and receive 400–800 mm of annual rainfall. The topmost characteristic of these forests is the extensive development of coniferous species, which follows a typical structural sequence along the altitudinal gradients. Continuous canopy prevails at lower elevations (close forest zone). At an altitude of 3,000 m, the forest canopy becomes fragmented, and the crowns of individual trees generally do not overlap forming woodland zone. Noticeably, this woodland zone was followed by herbaceous pastures (Alpine zone). Commonly, these forests consist of different plant species that exhibits considerable amount of overlap, because these species have specific site preferences like temperature regime and edaphic attributes.
The altitudinal range is from ~1,371 to 2,743 m (a.s.l), with tree limits varying markedly with aspect and configuration. The transition from moist to dry is gradual, and many forests are intermediate, between the two (transitional/ecotone). It is considered that the abundance of tall shrub Parrotiopsis jacquemontiana marks the transition from the moist to the dry type, where Artemisia brevifolea tends to replace grass with distinctive grayish foliage (Parker, 1924). The moist temperate forests lie between 2,000 and 2,700 m and are located in the same geographical region (N 34° 38.38′ latitudes and E 73° 33.11′ longitudes). At ~1,800 m, the tropical pine (Pinus roxburgii) forest ends, giving way to a belt of moist temperate forests followed by the subalpine Krummholz formation, above which alpine vegetation takes over until the beginning of the permanent snowline (Arnfield, 2023).
2.2. Selection of sites
The study area was subdivided into two major forest zones based on physiognomy and environmental conditions along an elevation gradient. These forest types are designated as lower Western Himalayan temperate forests, which include (a) low-level blue pine (Pinus wallichiana) forests (2,000 m a.s.l.), (b) mid-level moist deodar (Cedrus deodara) forests (2,200 m a.s.l.), and (c) upper West Himalayan fir (Abies pindrow) forests (2,400 m a.s.l.). After repeated surveys, study sites were marked within each forest located in the Kaghan Valley. Low-level blue pine forest at Paprung (N 34° 37.511′, E 73° 18.311′), mid-level moist deodar forest at Kamal Ban (N 34° 42. 034′, E 073° 31. 232′), and upper Himalayan mixed fir forests at Naran (N 34° 55. 003′) were chosen for investigation. The chosen forests encompass a broad range of altitudes, from the lower close forest (2,000–2,200 m a.s.l) to the upper timberline forest (2,400–2,700 m a.s.l) with a relatively open canopy. The three forests selected during the survey exhibited contrasting environmental conditions. In addition, natural disturbances such as fire, insect infestations, and avalanches are relatively infrequent, as they have been protected from human interference. Nevertheless, illegal collection of litter and grazing by livestock had taken place at most of the sites. The exact magnitude of this influence is difficult to estimate.
2.3. Vegetation sampling
Based on elevation, physiognomy, and principal plant species, the coniferous forest was stratified into the blue pine zone (200 m), the cedar zone (2,200 m), and the Abies zone (2,400 m). Fieldwork was carried out from mid-July to mid-August, a time of highest cover for most flowering species. Vegetation sampling was conducted during the peak growing season, July–August 2021–2022. Sampling sites were visited regularly throughout the growing season to sample flowering individuals for identification. Vegetation data were obtained from three forest types, with two sites in each forest [the difference in altitude between the upper and lower sites was ~100 m (Figure 4)].
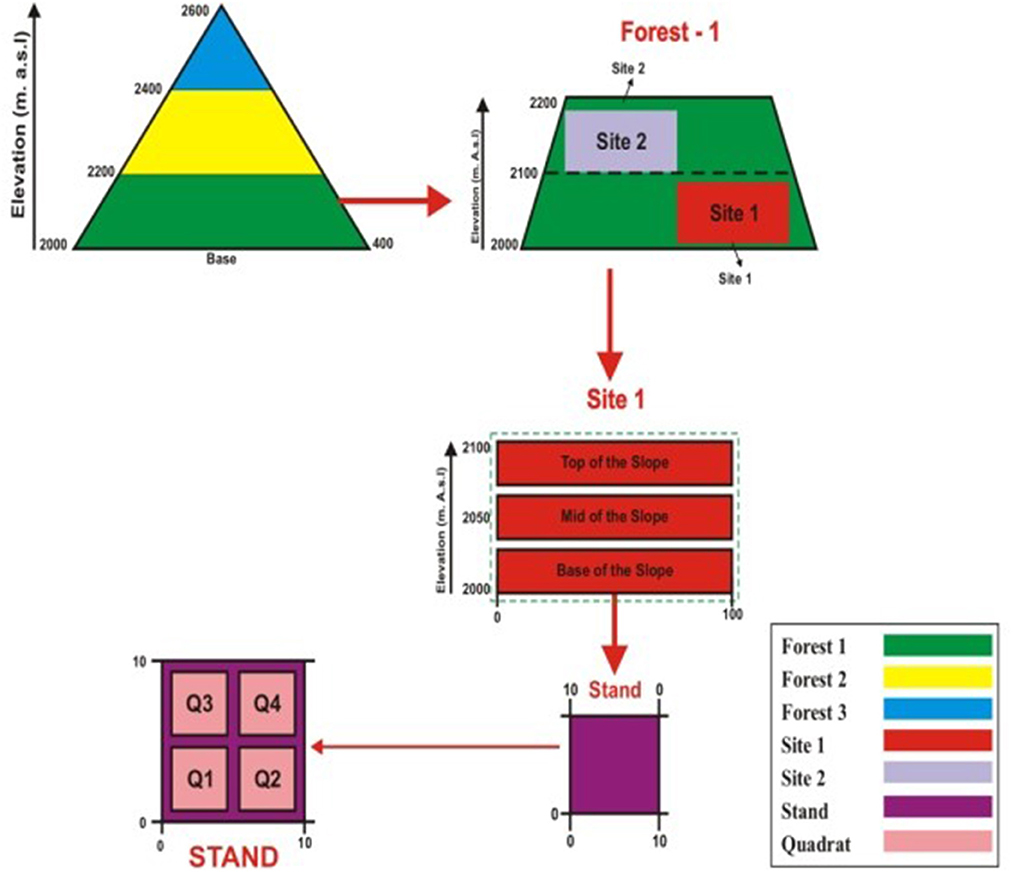
Figure 4. Depiction of sampling techniques. Forest 1 = low-level blue pine forest; Forest 2 = mid-Cedrus deodara forest; Forest 3 = upper Abies Pindrow forest, stand (10 × 10 m) and quadrate (4 × 4 m).
Two sites (200 m) and three stands with an interval distance of 100 m were positioned in each forest type. In each stand, four quadrates were sampled. Three topographic positions and eight sampling stands (10 × 10 m) positioned perpendicular to the base of the slope minimized altitude and soil variations within each stand (Figure 4). A total of 144 stands were monitored. The stands were geo-referenced using GPS technology and listed. A total of 288 quadrates were sampled from 144 stands. The size of quadrate was 1 × 1 m2 for herbs, 5 × 5 m2 for shrubs and 10 × 10 m2 for trees. Quantitative phytosociological techniques were used to record each species' density, frequency, and cover. The soil and the presence/absence of herbs and shrubs rooted in the 4 × 4 m quadrat were recorded and identified in the field. Voucher specimens of critical species were collected and identified later by matching the specimens in the Bokhari Memorial Herbarium, BZ University, Multan, Pakistan. Identifications and nomenclature were mainly based on the Flora of Pakistan (Nasir and Ali, 1972).
2.4. Soil sampling
Soil samples (0–15 cm) were randomly collected from each stand at three points and mixed into a composite sample. Pieces of roots, stones, and plant debris, if any, were carefully removed by sieving through a 2-mm mesh. Three subsamples were drawn from this composite sample. The collected 144 samples were oven dried at 105°C for 48 h before analysis. Soil pH was determined in 1 M CaCl2 (7 gm of soil in 0.35 ml) using an HM Digital 10 K pH meter (Allen, 1989; Cramer, 2012). Soil electrical conductivity (EC) was measured on a water extract (7 gm of soil in 0.35 ml) using a CM-30 ET digital EC meter (Cramer, 2012). Soil organic matter (OM) was determined in 1 g of milled soil following the method of Walkley and Black (1934). Available phosphorus (AP) was determined following the Bray II method. Total nitrogen (TN) content was determined using the Kjeldahl method [BUCHI Kjeldahl Line B-324 (Cramer, 2012)], and the concentrations of exchangeable cations were analyzed after extraction with 1 N ammonium acetate at pH 7. Total sodium (TNa) and total potassium (TK) were measured using a flame photometer (Jenway - 500 701- 230 V). Mg++ and Ca++ were determined using an atomic absorption spectrophotometer, Model- GD-320 N. CO−3 and HCO−3 were measured using the titration method (Richards, 1954). Ordinal scales assessed the percentage rock cover, litter cover, and canopy openness. The point scale for rock cover ranged from 1 to 4, with 1 = (<75%), 2 = (25–75%), 3 = (>25%), and 4 = absence of rocks or stones. Point scales for litter cover per stand were 1–4, with 1 = (25%), 2 = (25–50%), 3 = (51–75%), and 4 = (>75%). The point scales for forest canopy openness structure also ranged from 1 to 3, with 1 = separated, 2 = side by side, and 3 = overlapped (Suarez et al., 2004).
2.5. Environmental data
We used several environmental variables as explanatory variables that are important for shaping the plant communities in a complex mountain landscape based on previous studies (Hall and Swaine, 1976; Tanner et al., 1992, 1998; Dasti and Angew, 1994; Herbert and Fownes, 1995; Reich et al., 1996; Vitousek and Farrington, 1997; Dasti et al., 2007; Wazir et al., 2008; Saima et al., 2009). Stands were geo-referenced (Supplementary Table S1) using the GPS Navigator (Garmin e Trex Legend H, Lenexa, Kansas). Climatic parameters such as temperature (°C), relative humidity (%), heat index (°C), and wind speed (m/s) were recorded at the time of sampling by using Kestral, Japan (4500NV Applied Ballistics Meter). The annual data for all the climatic variables were obtained from the Pakistan Metrological Department.
2.6. Statistical analysis
We used cluster analyses to classify the sampling units based on their similarity. Presence-absence data were used for this analysis using the default option “Farthest neighbor,” incorporating the Pearson coefficient. Ordination analyses were conducted to visualize the floristic pattern of vegetation composition in relation to environmental factors. The main emphasis was on classification ordination, which was used in part to check whether the classification results adequately reflect the main floristically gradient in the data set and to detect the relation between some environmental factors and the composition and structure of vegetation. Species and stand data were ordinated using detrended correspondence analysis (DCA) using the program DECORANA (Dasti and Angew, 1994; Wazir et al., 2008). Ordination axes 1 and 2 were used for data interpretation; scatters of the classification groups from both procedures were plotted on overlays of the ordination to assess the compatibility of the two methods of data simplification (Dasti and Angew, 1994; Wazir et al., 2008). The relationships between altitude, soil characteristics, and DCA axes were determined using the Pearson correlation (Saima et al., 2009). The calculations were made with the help of statistical computer software (MVSP Version 3.1 and MINITAB, Version 15.0). The variation between soil heterogeneity and plant communities was estimated using variance analyses (ANOVA). Duncan multiple range tests were used to detect and compare any significant difference between the means of soil parameters in different communities at the 5% significance level.
3. Results
3.1. Floristic structure and species diversity
A total of 154 plant species belonging to 50 families and 131 genera were recorded. Asteraceae (14), Lamiaceae (12), Rosaceae (09 species), Fabaceae (08 species), Ranunculaceae (06 species), and Polygonaceae (05 species) were found to be the dominant families in these forests (Table 1). The remaining families were represented by less than three genera and three species and thus contributed little to the floristic richness.
3.2. Vegetation structure and composition
Based on the numerical classification, six vegetation types were identified in Himalayan moist temperate forests: Senecio chrysanthemoides – Clinopodium piperitum; Artemisia brevifolea – Fragaria nubicola; Parrotiopsis jacquemontiana – Indigofera gerardiana; Micromeria – Viburnum grandiflorum; Siline viscosa – Arisaema propinquum; and Adiantum venustum – Ajuga parviflora (Table 2). The indicator values for each association are shown in Table 3.
3.2.1. Association A. Senecio chrysanthemoides – Clinopodium piperitum (Group I)
This association is generally found between 2,500 and 2,600 m (a.s.l.) on the mountain slopes, which receive ~800 mm of average annual rainfall. The dominant Senecio chrysanthemoides and Clinopodium piperitum are perennial herbs. Spiraea canescens, Rosa microphylla, Rubus biflorus, Lonicera quinquelocularis, and Jasminum humile are important shrubs. Various ferns such as Dryopteris remosa, Athyrium rupicola, and Adiantum venustum are among the first to develop in late spring after the snow melts. The important climbers include Clematis montana and Hedera nepalensis (Table 3).
3.2.2. Association B. Artemisia brevifolea – Fragaria nubicola (Group II)
This association colony occupies the altitudinal range between 2,400 and 2,500 m (a.s.l.) and is distinctive in the combination of a high coverage of strongly aromatic, shrubby Artemisia brevifolea and procumbent, white-flowered Fragaria nubicola along with erect, shiny blue-flowered Myosotus alpestris. The former species dominate the open, dry stony, while the latter is widely distributed in forests and shrubberies. The dominance of the species can be ranked in the order Artemisia brevifolea > Fragaria nubicola > Myosotus alpestris (Table 3).
3.2.3. Association C. Parrotiopsis jacquemontiana – Indigofera gerardiana (Group III)
This association occurs at an altitude range of 2,300–2,400 m (a.s.l), and the diagnostic species of this association is Parrotiopsis jacquemontiana. The abundance of this species marks the transition from moist to dry forests, where Artemisia tends to dominate. The dominance of Indigofera gerardiana and Sambucus wightiana and the presence of Sorbaria tomentosa and Spiraea vaccinifolia indicate an ample moisture regime in this vegetation type (Table 3).
3.2.4. Association D. Micromeria – Viburnum grandiflorum (Group IV)
The component species of this association is distributed over the altitudinal ranges of 2,100–2,200 m (a.s.l.), corresponding to the lower part of the altitudinal range of Cedrus deodara. The dominant species of this association are Vibernum grandiflorum, Micromeria biflora, and blue-flowered Delphinium roylei. Among the component shrubs, Vibernum grandiflorum and Indigofera gerardiana are most common on stony pavements with shallow soils. Aster falconeri, Geranium lucidium, and Trifolium repens are common herbs found on the level of gently sloping wet meadows and pastures (Table 3).
3.2.5. Association E. Siline viscosa – Arisaema propinquum (Group V)
This association occupies the wetland sites of the valley highlands ranging from 2,200 to 2,300 m (a.s.l.) and is characterized by small white-flowered Silene viscosa and Arisaema propinquum. Geranium wallichianum assumes the leading role in the flora dominating the association. Among the shrubs, Barbaris lyceum, Indigofera gerardiana, and Vibernum grandiflorum contributes the most to this association. Among the ferns, Dryopteris remosa and Adiantum venustum occupy the micro-relives distributed along the wet, stony slopes. Among the component species, Fragaria indica, Geranium wallichiana, and Micromeria biflora are dominant on lower slopes, and Silene viscosa and Viola biflora are frequent on mid-slopes. At the same time, Vibernum grandiflorum is recorded at its maximum at the top of the slopes (Table 3).
3.2.6. Association F. Adiantum venustum – Ajuga parviflora (Group VI)
This association is distributed in the low-level blue pine forest zone from 2,000 to 2,100 m (a.s.l.) and is characterized by Pimpinella diversifolia and Pteracanthus urticifolia, which were altogether absent from the rest of the study area. Adiantum venustum, Ajuga parviflora, and Geranium tuberaria are the conspicuous species, while Primula rosea and Sambucus wightiana are significantly frequent. Among shrubs, Indigofera gerardiana, Lavatera kashimiriana, and Lonicera quinquelocularis contribute sufficiently to community composition (Table 3).
3.3. Environmental determinants
In addition to the overriding importance of altitude in determining species distribution and shaping plant communities in mountain landscapes, most of the edaphic factors included have significant correlations with the first axis (Table 4). The importance of soil EC, K, Ca, Mg, Na, and N was notable. All these cations were negatively correlated with DCA axis 1 but were mostly much lower in values except EC, Na, and P, whose r- values exceeded 0.4. Regarding axis 2, the r-values of these factors were considerably low (r < 0.3). Most of the soil cations and soil EC exhibited a positive correlation, with axis 2 suggesting cation-rich soil conditions from the top to the bottom of the gradient. All chemical variables measured in the soil samples showed broad variations at the landscape scale (Tables 4, 5). There was a general increase in the concentration of all the solutes in the sequence from the upper Abies pindrow forest to the mid-Cedrus deodara forest to the low-level Pinus wallichiana forest.
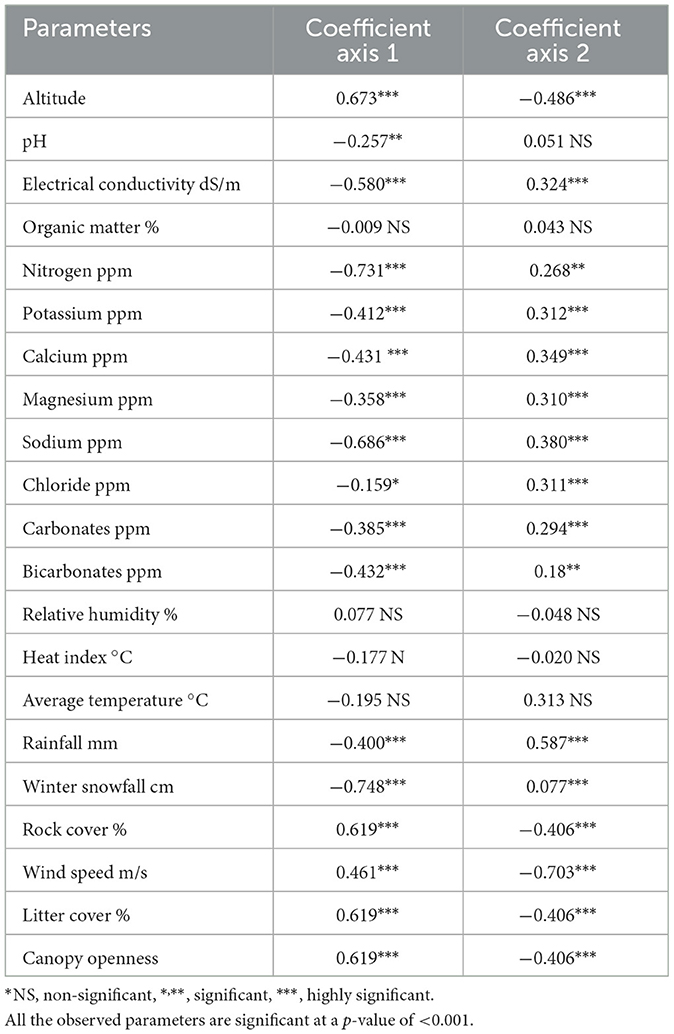
Table 4. Pearson correlation coefficient between DCA axis scores of soil parameters and altitudes of three forest types (located along the altitudinal range 2,000–2,700-m a.s.l.).
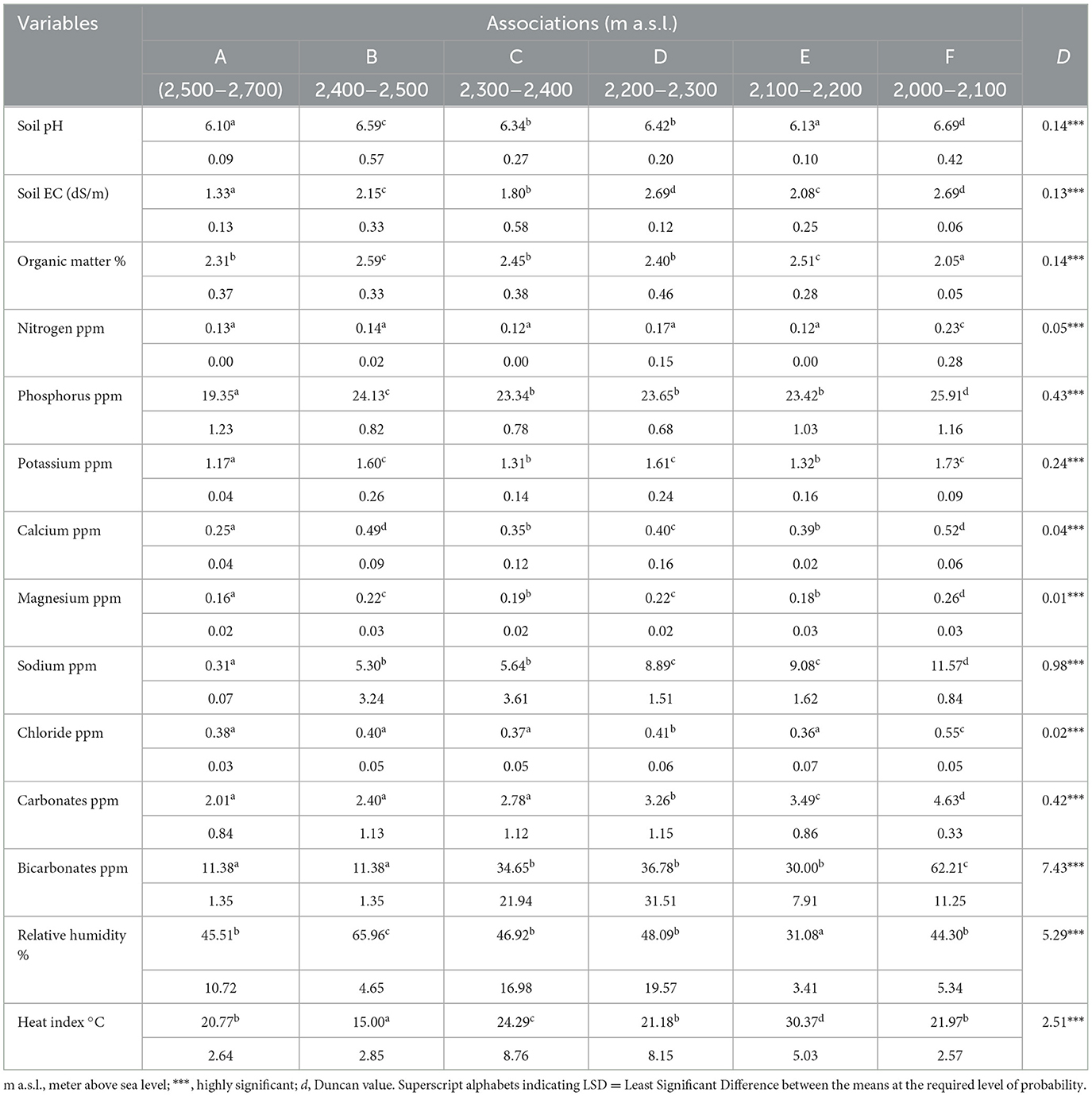
Table 5. The mean values and standard deviation for all the soil variables for identified six associations.
3.4. Ordination
To identify a minimal set of geo-climatic variables and to know the relative importance of these factors, stands and species ordination (DCA) was performed, using geo-climatic parameters as response variables. The DECORANA (DCA) ordination results were used to plot the scatters of classificatory results, as shown in Figures 5, 6. DECORANA (axes 1 and 2) showed a plot of 144 stands from three forest types. Stands are plotted individually, and zones are shown where the NCA segregates each of the six associations, as indicated in Figure 5. The eigenvalues of axes 1 and 2 were very large compared to the lower-order axis, and the latter were ignored (Figure 6).
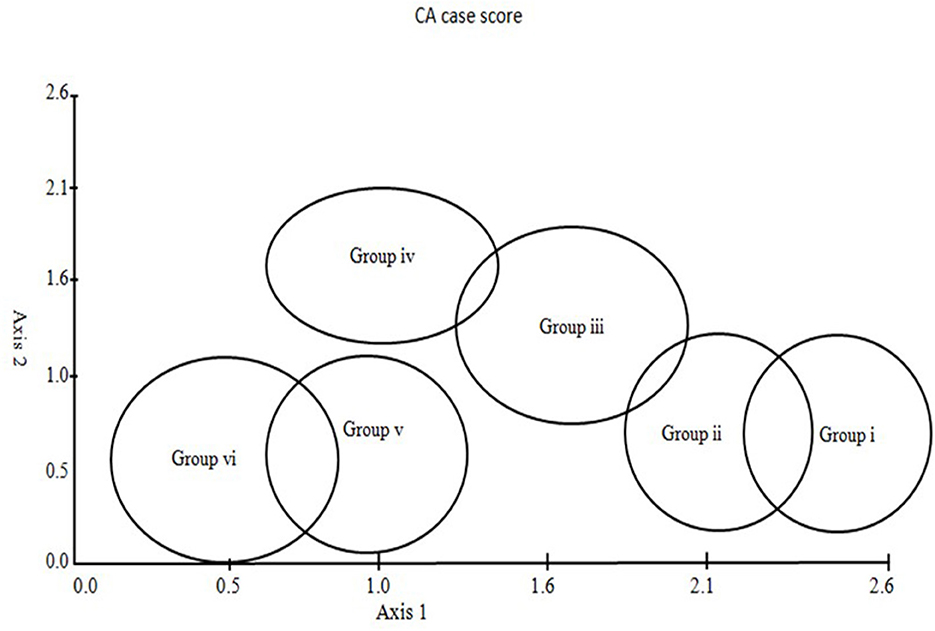
Figure 5. Sampling stand distribution in different communities exhibiting association delineated by ordination.
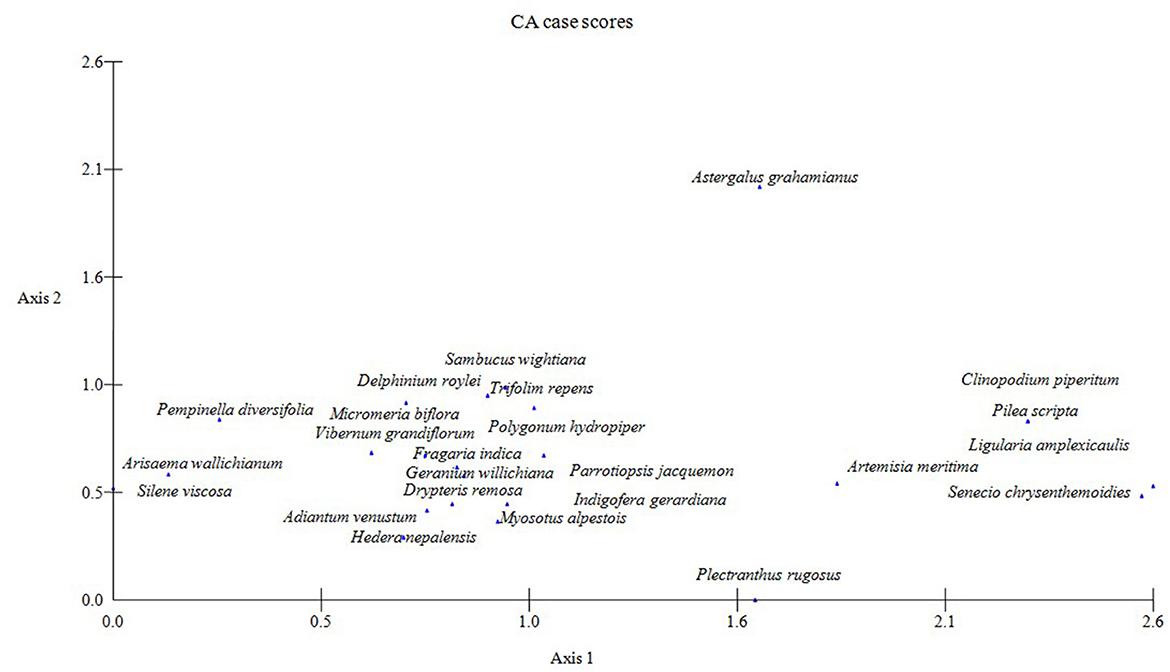
Figure 6. Detrended correspondence analysis (DCA) species ordination of the qualitative data of three temperate forests regarding ordination indicates species distribution along the elevation gradient (Abies Pindrow forest, Cedrus Deodara forest, and low-level Pinus Wallichiana forest).
3.5. Correlation of the ordination axes
The stands belonging to associations (A and B) delineated from the upper Abies pindrow forest were grouped at one end (high scores) and those of low-level Pinus wallichiana forest (associations E and F) on the other end (low scores) of the DCA axis 1. Stands belonging to the associations C and D identified in the mid-Cedrus deodara forest occupy the intermediate position along axis 1, reflecting the mid-elevations of the study area (Figure 5). The results depicted in Figure 6 confirm the overlapping nature of the associations in space defined by the ordination axes. The first ordination axis represents an elevation gradient, with the highest elevation on the right (high scores) and the lowest on the left (low scores). Within the first axes, species such as Senecio chrysanthemoides, Ligularia amplexicaulis, Clinopodium piperitum, and Artemisia brevifolea lie at the most elevated end of axis 1. At the same time, Pimpinella diversifolia, Arisaema propinquum, and Silene viscosa are confined to the lowest elevation end of the gradient. The diagram's midpoint is occupied by Parrotiopsis jacquemontiana, Sambucus wightiana, Micromeria biflora, Trifolium repens, and Delphinium roylei. Similarly, on axis 2, Plectranthus rugosus and Hedera nepalensis are at the lower end of the axis, while Sambucus wightiana and Trifolium repens are at the opposite end. The second axis represents increasing soil cations from the top to the bottom.
4. Discussion
Low-level Pinus wallichiana forest is located between 2,000 and 2,200 m (a.s.l); in this forest type, there is no definite evidence of basic changes through human activities. The forest under consideration is exclusive of blue pine with a small admixture of spruce (Picea smithiana), deodar (Cedrus Deodara), and fir (Abies Pindrow). The top canopy is more or less continuous. The mid-level moist deodar (Cedrus deodara) forest is nearly pure, but some spruce is also present in patches. Deodar extends into the inner drier ranges of the Himalayas, ultimately becoming sufficiently different to be described as dry deodar. Upper-west Himalayan fir and mixed broad-leaved forest represent the subalpine forests where regeneration of Abies pindrow is usually fairly abundant. The general climate of this forest is dry and temperate; however, soil moisture is adequate, which makes it difficult to distinguish it from most temperate forests.
The extensive growth of Artemisia brevifolea distinguishes the upper fir forests from the lower coniferous belt. Champion et al. (1965) and Hussain and Illahi (1991) described these forest types completely. The temperature, relative humidity, and wind speed were significantly related to DCA axes 1 and 2. Both the ordination axes are significantly influenced by altitude and the environmental queues generated by it, which indicated some important implications for these factors in determining vegetation attributes. For example, low species richness in associations belonging to upper forests may be attributed to increasingly strong winds, reduced precipitation, reduced temperature, increased soil acidity, and a reduced supply of nutrients with increasing altitude. These results confirm those of Pendry (1994).
Soil pH usually falls with altitude, and the most acidic soils are generally found in upper fir forests. These findings are consistent with those of Pendry and Proctor (1996) but contradict the results of Veneklaas (1991), who reported a higher pH of soils in upper forests than soils in nearby lower forests. Soil organic matter increased with altitude, partly because of reduced decomposition at lower temperatures but often due to temporary waterlogged conditions created by melting snow in the upper mountains. Extractable phosphorus is also low at higher altitudes. The elevational reduction in phosphorus might be partially due to a high concentration of organic matter, which reduced the potential of phosphorus fixation (Tanner et al., 1998). Differences in the concentration of cations among the three forests are noted. These differences may be largely attributed to the parent material (Pendry and Proctor, 1996), but the importance of the downslope movement of water cannot be ignored (Dasti et al., 2007, 2010; Wazir et al., 2008). The study demonstrates significant associations between the landscape-level patterns of environmental heterogeneity and the distribution patterns of plant communities and species across mountain landscapes. Two factors seem to be significant: altitude and substrate. The overriding importance of altitude as an environmental factor affecting plant species association is not surprising, considering its close correlation with temperature and rainfall (Danin et al., 1975; Dasti and Malik, 1998; Dasti et al., 2007; Wazir et al., 2008; Saima et al., 2009). The analysis and assessment of patterns and the zonation along the first axis suggest that the most important environmental gradients and boundaries across the landscape are associated with the downslope movement of water, soil chemistry, and organic matter. It is difficult to assess the relative importance of these factors in comparison with altitude. Still, the consistent negative interaction terms between these factors suggest that some combined effect is significant. The reasons for these correlations might be due to variations in runoff patterns.
The spatial distribution of soil moisture might correlate with rainfall in lower blue pine valleys, which receive an appreciable amount of runoff. However, the situation is quite different in the upper rocky mountains with patches of soil. Here, soil moisture distribution depends on the ratio of rock surface to soil volume (Olsvig-Whittaker et al., 1983; Dasti and Malik, 1998; Wazir et al., 2008). These patterns seem best explained by interactions between soil depth and the downslope redistribution of runoff. The lower forests gain water from run-on, while the upper forests lose water from run-off. This run-off will be distributed along a moisture gradient, along which vegetation change will be gradual. Besides this factor, snow accumulation and its gradual melting are of considerable importance in determining the vegetation type at high elevations. Apart from the differences in moisture regime, the topographically induced variation in soil EC plays a significant role in community composition and plant assemblage.
The species belonging to associations located at lower elevations occur on soil with relatively high EC, which decreases with an increase in altitude. Thus, the altitudinal floristic differences may partially be attributed to soil EC. The decrease in EC with increased altitude is largely due to the downward movement of nutrients, which affects spatial distribution along the altitudinal transect (Pendry and Proctor, 1996; Dasti and Malik, 1998; Raja et al., 2014). The correlation of soil pH with the distribution and association of plant species is not surprising but has already been reported in several studies (Pendry and Proctor, 1996). We have demonstrated that the topographically induced edaphic patchiness governs the distribution of the species across the landscape. A clear relationship between the characteristics of the substrate and associations defined by numerical analysis shows that these groupings of plant species are not arbitrary assemblages.
Differences in soil within the forest are distinct enough to favor different species at different locations and thus create numerous floristically differentiated forest patches. These findings confirm the edaphic patchiness model rather than the random walk or regional homogeneity model (Tuomisto et al., 2003). In this respect, the spatial pattern of environmental conditions along the elevation gradient appears particularly important to plant communities' composition and distribution. Altitude and topography govern the properties of the soil, which are of overriding importance in determining the variations in floristic composition. Sharp species turnover occurs when the environmental conditions change, and different (dominant) species are found at sites with different environmental conditions. These findings are consistent with the findings of Lieberman et al. (1985) and Clark et al. (1998, 1999). The dominance of only one plant species at most sites was obvious in the field, and this is a notable feature of the vegetation of Himalayan moist temperate forests. However, a few associations, defined by cluster analysis and confirmed by DCA, showed that some species were restricted to the upper forests and others to the low or mid forests. Still, several have distribution ranges that extend into all associations.
The visual representation of the results resemble the continuous nature of the vegetation more than the grouping produced by classification. However, describing the salient features of associations between stands and species with ordination is easy and worthwhile. The scatters of the DCA demonstrate the overlapping rather than discrete boundaries between the vegetation types and species distributions. This overlapping nature of the vegetation types might be due to the species overlapping environmental preferences. The substrate of all these communities is found through the interaction of topography and related basic geomorphological processes of surface movement through wind and downslope movement of water, which are expected to bring about arbitrary changes in dominance within the association. Finally, the altitude limits the vegetation observed along an altitudinal gradient. For example, the dominance of Senecio chrysanthemoides and Clinopodium piperitum is restricted to high altitudes (2,400–2,700 m a.s.l). These species were absent from altitudes below 2,400 m a.s.l. Similarly, the species dominating the low forest (Silene viscosa and Arisaema propinquum) were not found in the upper fir forest zone.
5. Conclusion
The present study described the vegetation patterns and community composition in three different forest zones along the altitudinal gradient. Our findings revealed that these forest types significantly differ physiognomically and structurally. The special nature of the mountain landscape, with its variable altitude and topographically induced environmental variations, is the main factor that determines the prominent vegetation zones in these mountain forests. The soil's physical and chemical properties appear to be the most highly influential factors in the distribution and diversity of species along the altitude. The present investigation showed that the distribution of community types, species composition, and diversity are better understood by studying geo-climatic factors along the elevational gradient on the mountain landscape. Based on the comprehensive botanical survey and early descriptive accounts of the study area, the vegetation in the temperate forest zone was stratified into three physiognomic units: (a) low-level Pinus wallichiana forest, (b) mid-Cedrus deodara forest, and (c) upper Abies pindrow forest. As Himalayan moist temperate forests exhibit a rather stable floristic composition and slow species dynamics, the different years in which the vegetation was sampled were not considered a serious methodological problem.
Data availability statement
The original contributions presented in the study are included in the article/Supplementary material, further inquiries can be directed to the corresponding authors.
Author contributions
MH, EB, AD, MK, TF, and AAb contributed to the study's concept, design, and statistical analysis. MK, SM, MH, AAs, and AD contributed to the analysis and interpretation of the data. EB, MK, and HA contributed to the investigation and resources. MH, AAb, HA, and ZA contributed to the drafting of the manuscript. SM, EB, TF, AAs, MK, and AD contributed to this manuscript's review, editing, and proofreading. HA contributed to funding acquisition and study supervision. All authors contributed to the article and approved the submitted version.
Funding
This research was funded by the Researchers Supporting Project number (RSP2023R123), King Saud University, Riyadh, Saudi Arabia.
Acknowledgments
We would like to extend our sincere appreciation to the Researchers Supporting Project number (RSP2023R123), King Saud University, Riyadh, Saudi Arabia.
Conflict of interest
The authors declare that the research was conducted in the absence of any commercial or financial relationships that could be construed as a potential conflict of interest.
Publisher's note
All claims expressed in this article are solely those of the authors and do not necessarily represent those of their affiliated organizations, or those of the publisher, the editors and the reviewers. Any product that may be evaluated in this article, or claim that may be made by its manufacturer, is not guaranteed or endorsed by the publisher.
Supplementary material
The Supplementary Material for this article can be found online at: https://www.frontiersin.org/articles/10.3389/ffgc.2023.1195491/full#supplementary-material
References
Ahmad, I., Altaf, A., Hashim, M., Gulshan, A. B., Hussain, F., Bushra, E., et al. (2021). Species richness and community composition along glaciated Alpine Lake, Pakistan. GU J. Phytosci. 1, 24–40. doi: 10.12692/ijb/18.2.114-129
Ahmad, M., Hussain, T., Sheikh, A. H., Hussain, S. D., and Siddiqui, M. F. (2006). Phytosociology and structure of Himalayan forests from different climatic zones of Pakistan. Pak. J. Bot. 38, 361–383.
Arnfield, A. J. (2023). Köppen Climate Classification. Encyclopedia Britannica. Available online at: https://www.britannica.com/science/Koppen-climate-classification
Bhattarai, P., Bhatta, K. P., Chhetri, R., and Chaudhary, R. P. (2014). Vascular plant species richness along a elevation gradient of the Karnali River Valley, Nepal Himalaya. Int. J. Plant Animal Environ. Sci. 4, 109–114.
Clark, D. B., Clark, D. A., and Read, J. M. (1998). Edaphic variation and the meso-scale distribution of tree species in a neo-tropical rain forest. J. Ecol. 86, 101–112. doi: 10.1046/j.1365-2745.1998.00238.x
Clark, D. B., Clark, D. A., and Read, J. M. (1999). Edaphic factors and the landscape-scale distribution of rain forest trees. Ecology 80, 2662–2675. doi: 10.1890/0012-9658(1999)0802662:EFATLS2.0.CO;2
Cramer, M. D. (2012). Unraveling the limits to tree height: a major role for water and nutrient trade-offs. Oecologia 169, 61–72. doi: 10.1007/s00442-011-2177-8
Danin, A., Orshan, G., and Zohary, M. (1975). The vegetation of the northern Negev and the Judean Desert of Israel. Isra. J. Bot. 24, 118–172.
Dasti, A. A., and Angew, D. Q. (1994). The vegetation of Cholistan and Thal deserts Pakistan. J. A. Envir. 27, 193–208. doi: 10.1006/jare.1994.1058
Dasti, A. A., and Malik, S. A. (1998). A transect of vegetation and soil on the Indus valley scrape slope. Pak. J. Plant Sci. 4, 73–84.
Dasti, A. A., Saima, S., Athar, M., Rehman, A., and Malik, S. A. (2007). Botanical composition and multivariate analysis of vegetation on the Pothowar Plateau, Pakistan. J. Bot. Res. Inst. Texas. 1, 557–568.
Dasti, A. A., Saima, S., Mahmood, Z., Athar, M., and Gohar, S. (2010). Vegetation zonation along the geological and geomorphological gradient at eastern slope of Sulaiman Range, Pakistan. Afr. J. Biotechnol. 9, 6105–6115.
Ellu, G., and Obua, J. (2005). Tree condition and Natural Regeneration in Distributed sites of Bwindi Impenetrable forest national park, Southwest Uganda. Trop. Ecol. 46, 99–111.
Hall, J. B., and Swaine, M. (1976). Classification and ecology of closed-canopy forest in Ghana. J. Ecol. 64, 913–951.
Herbert, D. A., and Fownes, J. H. (1995). Phosphorus limitation of forest leaf area and net primary production on a highly weathered soil. Biogeochemistry. 29, 223–235.
Jadoon, W., Khan, S., Ahmad, H., Shakeel, A., and Page, S. (2017). Ecological gradient analyses of plant associations in the Thandiani forests of the Western Himalayas, Pakistan. Turk. J. Bot. 41, 253–264. doi: 10.3906/bot-1602-22
Khan, S. M., Harper, D. M., Page, S., and Ahmad, H. (2011). Species and community diversity of vascular flora along environmental Gradients in Narran valley: a multivariate approach through indicator species analysis. Pak. J. Bot. 43, 2337–2346.
Khan, S. M., Page, S. S., Ahmad, H., and Harper, D. M. (2012). Identifying plant species and communities across environmental gradients in the western Himalayas: method development and conservation use. J. Ecol. 11, 1–10.
Kharkwal, G., Mehrotra, P., Rawat, Y. S., and Pangtey, Y. P. S. (2005). Phyto-diversity and growth form in relation to altitudinal gradient in central Himalayan (Kumaun) region of India. Curr. Sci. 5, 873–878.
Lieberman, M., Lieberman, D., Hartshorn, G. S., and Peralta, R. (1985). Small-scale altitudinal variation in lowland tropical forest vegetation. J. Ecol. 73, 505–511. doi: 10.2307/2260490
Nasir, E., and Ali, S. I. (1972). Flora of Pakistan. Agricultural Research Council, Islamabad. Nature 163, 780–785.
Noor, S., Hashim, M., Altaf, A., Bushra, E., Bibi, A., Nazir, A., et al. (2021). A numerical analysis of understory plant associations in a Pinus wallichiana forest, Pakistan. J. Biodiversity Environ. Sci. 18, 19–34.
Odgaard, R. V., and Rasmussen, P. (2000). Origin and temporal development of macro-scale vegetation pattern in the cultural landscape of Denmark. J. Ecol. 88, 733–748. doi: 10.1046/j.1365-2745.2000.00490.x
Olsvig-Whittaker, L., Shachak, M., and Yair, A. (1983). Vegetation patterns related to environmental factors in a Negev desert watershed. Vegetation 54, 153–165. doi: 10.1007/BF00047104
Orshan, G. (1986). “The deserts of Middle East,” in Ecosystems of world 12B Hot Deserts and Shrub Lands, eds M. Evenari, I. Noy-Meir, and D. W. Doodall (Ams: Els), 28p.
Parker, R. N. (1924). A Forest Flora for the Punjab With Hazara and Delhi. Punjab: Government Printing.
Pendry, C. A. (1994). Ecological studies on rain forests at three altitudes on Bukit Belalong, Brunei (Ph.D. Thesis) University of Stirling.
Pendry, C. A., and Proctor, J. (1996). The causes of altitudinal zonation of rain forests on Bukit Bel along Brunei. J. Ecol. 84, 407–418. doi: 10.2307/2261202
Ping, C. L., Michaelson, G. J., Stiles, C. A., and Gonzalez, G. (2013). Soil characteristics, carbon stores, and nutrient distribution in eight forest types along an elevation gradient, eastern Puerto Rico. Ecol. Bull. 54, 67–86.
Qadir, S., Altaf, A., Hashim, M., Bushra, E., BiBi, A., Ahmad, N., et al. (2020). Distribution of roadside plant communities along the altitudinal gradient in pine forests, Pakistan. Int. J. Biosci. 17, 96–115. doi: 10.12692/ijb/17.5.96-115
Rahman, I. U., Afzal, A. I., Iqbal, Z., Abd Allah, E. F., Alqarawi, A. A., Calixto, E. S., et al. (2019). Role of multivariate approaches in floristic diversity of Manoor Valley (Himalayan region), Pakistan. Appl. Ecol. Environ. Res. 17, 1475–1498. doi: 10.15666/aeer/1702_14751498
Raja, R., Bukhari, T. Z., Younis, U., and Dasti, A. A. (2014). Multivariate analysis in wet temperate forests of Pakistan. J. Pharm. Biol. Sci. 1, 54–59. doi: 10.9790/3008-09165459
Reich, J. W., Russell, A. R., Crews, T. E., Farringon, H., and Vitousek, P. M. (1996). Both Nitrogen and Phosphorous limit plant production on young Hawaiian lava flows. Biochemistry. 32, 1–14.
Richards, L. A. (1954). Diagnosis and Improvement of Saline and Alkali Soils. Agricultural Hand-Book. - No. 60. Bombay: U. S. Dept. Of Agriculture and J. B. H Publishing, 230 p.
Saima, S., Altaf, A., Faiz, M. H., Shahnaz, F., and Wu, G. (2018). Vegetation patterns and composition of mixed coniferous forests. Aust. J. Fores. Sci. 135, 159–180.
Saima, S., Dasti, A. A., Hussain, F., Wazir, S. M., and Malik, S. A. (2009). Floristic compositions along an 18 - km long transect in Ayubia National Park district Abbottabad, Pakistan. Pak. J. Bot. 41, 2115–2127.
Shaheen, H., Khan, S. M., Harper, D. M., Ullah, Z., and Qureshi, R. A. (2011). Species diversity, community structure and distribution patterns in Western Himalayan alpine pastures of Kashmir, Pakistan. Mount. Res. Develop. 31, 153–159. doi: 10.1659/MRD-JOURNAL-D-10-00091.1
Sharma, C. M., Suyal, S., Gairola, S., and Ghildiyal, S. K. (2009). Species richness and diversity along an altitudinal gradient in moist temperate forest of Garhwal Himalaya. J. Am. Sci. 5, 119–128.
Suarez, M. L., Ghermandi, L., and Kitzberger, T. (2004). Factors predisposing episodic drought-induced tree mortality in Nothofagus- site, climatic sensitivity and growth trends. J. Ecol. 92, 954–966. doi: 10.1111/j.1365-2745.2004.00941.x
Tanner, E. V. J., Kapos, V., and Franco, W. (1992). Nitorgen and phosphorus fertilization effects on Venezuelan montane forest trunk growth and litterfall. Ecology. 73, 78–86.
Tanner, E. V. J., Kapos, V., Freskos, S., Healey, J. R., and Theobald, A. M. (1990). Nitrogen and phosphorus fertilization of Jamaican montane forest trees. J. Trop. Ecol. 6, 231–238. doi: 10.1017/S0266467400004375
Tanner, E. V. J., Virtuosic, P. M., and Cuevas, E. (1998). Experimental investigation of nutrient limitation of forest growth on wet tropical mountains. Ecology 79, 10–22. doi: 10.1890/0012-9658(1998)0790010:EIONLO2.0.CO;2
Tuomisto, H., Kolainen, K. R., and Halla, M. Y. (2003). Dispersal, environment and floristic variation of Western Amazonian Forests. Science 299, 241–244. doi: 10.1126/science.1078037
Ummara, U., Bokhari, T. Z., Altaf, A., Younis, U., and Dasti, A. A. (2013). Quantitative description of understorey vegetation of Shogran valley, Pakistan. FUUAST J. Biol. 5, 63–70.
Veneklaas, E. J. (1991). Litter fall and Nutrient fluxes in two montane tropical rainforests, Colombia. J. Trop. Ecol. 7, 319–336. doi: 10.1017/S0266467400005587
Vitousek, P. M., and Farrington, H. (1997). Nutrient limitation and soil development: experimental test of a biogeochemical theory. Biogeochemistry. 37, 63–75.
Walkley, A., and Black, I. A. (1934). An examination of Degtjareff method for soil organic matter and a proposed modification of the chromic acid titration method. Soil Sci. 37, 29–37. doi: 10.1097/00010694-193401000-00003
Wani, Z. A., Negi, V. S., Bhat, J. A., Satish, K. V., Kumar, A., and Khan, S. (2023) Elevation, aspect, habitat heterogeneity determine plant diversity compositional patterns in the Kashmir Himalaya. Front. Forest. Glob. Chang. 6, 1019277. doi: 10.3389/gc.2023.1019277
Wazir, S. M., Dasti, A. A., Saima, S., Shah, J., and Hussain, F. (2008). Multivariate analysis of vegetation of Chapursan valley: an alpine meadow in Pakistan. Pak. J. Bot. 40, 615–626.
Keywords: mountain ecosystem, species distribution, multivariate analysis, Western Himalayas, distribution pattern, composition
Citation: Hashim M, Bushra E, Dasti AA, Abbasi A, Ali HM, Maqsood S, Farooq TH, Aslam A, Abbas Z and Khan MT (2023) Exploration of species diversity and vegetation pattern in temperate conifer forests along altitudinal gradients in the Western Himalayas. Front. For. Glob. Change 6:1195491. doi: 10.3389/ffgc.2023.1195491
Received: 28 March 2023; Accepted: 13 June 2023;
Published: 21 July 2023.
Edited by:
Gopal Shukla, Uttar Banga Krishi Viswavidyalaya, IndiaReviewed by:
Rajendra Mohan Panda, Mississippi State University, United StatesMuhammad Umar, University of Tasmania, Australia
Copyright © 2023 Hashim, Bushra, Dasti, Abbasi, Ali, Maqsood, Farooq, Aslam, Abbas and Khan. This is an open-access article distributed under the terms of the Creative Commons Attribution License (CC BY). The use, distribution or reproduction in other forums is permitted, provided the original author(s) and the copyright owner(s) are credited and that the original publication in this journal is cited, in accordance with accepted academic practice. No use, distribution or reproduction is permitted which does not comply with these terms.
*Correspondence: Taimoor Hassan Farooq, t.farooq@bangor.ac.uk; Zaheer Abbas, zaheer.abbas@ue.edu.pk