- 1College of Forestry, VCSG Uttarakhand University of Horticulture and Forestry, Ranichauri, New Tehri, Uttarakhand, India
- 2Centre of Excellence on Sustainable Land Management, Indian Council of Forestry Research and Education, Dehradun, Uttarakhand, India
- 3Department of Forestry and Natural Resources, HNB Garhwal University, Srinagar, Uttarakhand, India
The management of forests is essential for the conservation of biodiversity and climate change. Studies on the variation in forest tree diversity and carbon sequestration by the forests along altitudinal gradients are limited for most parts of the Garhwal Himalaya. The present study was carried out in montane forests in a part of district Tehri of the Garhwal Himalaya, India at four different sites between altitudinal ranges of 850 to 2,200 masl (i.e., 850–1,500 masl under sub-tropical region and 1,800–2,200 masl under temperate region) for vegetation, soil, and carbon stock analysis using different parameters including the existing pressure on the sites for fuel, fodder, timber, grazing, and browsing. All the standard methods were used for vegetation and soil analysis. The highest value of tree diversity (H = 0.48) and concentration of dominance (CD = 0.95) was recorded at 850–1,100 masl altitude under sub-tropical forests. The aboveground biomass density (AGBD) and belowground biomass density (BGBD) ranged from 271 to 703.20 and 83.21 to 163.92 Mg ha–1, respectively. The pH value ranged from 5.63 to 6.86 (0–15 cm) and 6.17 to 7.21 (15–30 cm). Similarly, soil organic carbon (SOC) was reported to range from 1.86 to 2.57% and 1.78 to 2.44% at 0–15 cm and 15–30 cm depths, respectively. Available nitrogen (N) under temperate forests was reported to be significantly higher than at other altitudes. Among the studied parameters, a significant positive correlation was reported between the pH of the soil and the Shannon–Wiener and Margalef diversity indices. The study revealed that the sub-tropical region was more diversified in terms of tree species whereas soil properties were reported to be better in the temperate region. Among the species in the study region, Pinus roxburghii, Boswellia serrata, Quercus leucotrichophora, and Cedrus deodara have shown good potential for carbon storage. Thus, based on the dominant and associated species present in the area, forest management activities may be followed for the survival and regeneration of species with the potential of being the next dominant species in the future.
Introduction
The Garhwal Himalaya is a hot spot of biodiversity, located in the western part of Central Himalaya. Forests in the Himalayas have been under various kinds of pressure in the past decades because of changes in socioeconomic status, exponential population growth, and infrastructure development (Singh et al., 2009). The variation in physiography, soil types, and climatic conditions in the Garhwal Himalaya has changed the community structure and its dynamics which has attracted researchers to conduct comprehensive studies in tropical and sub-tropical montane forests to study species richness, biomass, and productivity (Tripathi and Khongjee, 2010). In Uttarakhand, Quercus leucotrichophora forest covers 5.24% of the area (i.e., 1,284 km2) from 1,000 to 2,500 masl, whereas, between 1,000 and 1,500 m, patches with other forests are observed (Singh et al., 2016). The pine-oak forest is believed to be between 1,500 and 1,800 m in altitude, whereas, Pinus roxburghii is widespread from 900 to 1,500 m in altitude (Chaturvedi and Singh, 1987), and sometimes reaches the higher elevations. However, recent alterations as a consequence of climatic and anthropogenic disturbances have also resulted in huge canopy gaps and interference in forest soils. The change in forest species composition is usually expected to affect the properties of soil because soil and vegetation have a complex interrelation. Similarly, the changes also influence carbon accumulation in the forest. Further, soil properties and soil fertility differ significantly based on the tree species grown in it (Augusto et al., 2002).
The disturbance processes in the ecosystems usually increase greenhouse gas emissions (GHGs) and affect the atmospheric gas concentration (Kumar et al., 2019, 2021b). The level of greenhouse gas emissions, especially from rapid anthropogenic disturbances, leads to further disturbances in the carbon cycle (Anderegg et al., 2015; Waltham et al., 2020; Kumar et al., 2021b; Rawat et al., 2021). In the central part of India, disturbances associated with mining have augmented the fragmentation of natural forests and a reduction in the density and diversity of vegetation has been documented. This loss of biomass has reportedly resulted in a net loss of 1.08 Tg C in the past two decades (Mishra et al., 2022). Dong et al. (2022) reported the changes in soil carbon decomposition due to the priming effect caused by an accumulation of more plant-derived substrate on the surface soil as being affected by climate change.
In the western Himalayas, the trees in temperate forests are P. roxburghii, Quercus spp. Rhododendron arboreum, Lyonia ovalifolia, Myrica esculenta, Cedrus deodara, and Pinus wallichiana (Luna, 2005) and are used for quality fodder, fuel, charcoal making, and agricultural implements apart from providing other ecosystem services (Rawat D. et al., 2022). But owing to the increasing human interferences in forests and rapid urbanization in the nascent Himalayan region, the extent of deforestation, loss of biodiversity, and forest degradation is growing rapidly along with crucial alterations in land use patterns. These changes in the terrestrial landscapes are also affecting the tree composition and nutrient dynamics of the system (Mishra et al., 2017a; Kumar et al., 2021c; Rawat S. et al., 2022; Tariyal et al., 2022; Sharma et al., 2023).
Usually, 43–50% of the dry biomass of trees consists of carbon (Malhi et al., 2002; Negi et al., 2003; Rawat S. et al., 2022) and soil carbon plays an important role in the global carbon cycle (Gupta and Sharma, 2011). Also, biogeochemical nutrient cycling is important for the quality of a site. However, the vegetation of the area influences the physicochemical characteristics of soil and these soil characteristics change due to various biotic and abiotic factors. The stoichiometric ratios of nutrients such as carbon, nitrogen, and phosphorus within a forest ecosystem can alter the status of soil nutrients and the future sink of carbon (Kumar et al., 2021a). Further, forest soils impact forest composition, growth rate, regeneration, etc (Sheikh and Kumar, 2010; Rawat S. et al., 2022). Altitude plays an important role in that it changes both climatic and site conditions and has a significant influence on the plant communities (Kharkwal et al., 2005; Gauthier et al., 2011). Moreover, altitude plays an important role in species distribution which also sustains other associated flora and fauna. Various studies on tree diversity and carbon stocks (Gairola et al., 2011a; Sharma et al., 2011), the physical properties of the soil (Sharma et al., 2010a), and the structure and composition of forests (Gairola et al., 2011b) have also been carried out previously. Verma and Garkoti (2019) studied the species composition, soil properties, and carbon stock of the regenerated Q. leucotrichophora forests in the Kumaon region of the Himalaya. Meena et al. (2021) documented the significant influence of the topographical position factor on the concentration of soil organic carbon and carbon management value in the Indian Himalayan ecosystem. However, the investigations that bring together the cumulative information about vegetation composition, tree diversity, soil properties, and carbon density of forests of Garhwal Himalaya are meager. Therefore, this study was conducted with the following objectives: (i) to understand how vegetation composition, structure, and carbon storage are influenced by altitude, (ii) to understand the variation in physicochemical characteristics of soil among different forests and altitudes, and (iii) to assess the influence of various anthropogenic disturbances on vegetation patterns at different altitudes.
Materials and methods
This study was conducted in the district of Tehri in the Garhwal Himalaya. Four different sites at different altitudinal ranges were selected for the study (Table 1). The vegetation of the area and soil properties were studied at two sites of sub-tropical montane forests, i.e., Khadi (850–1,100 masl) and Gaja (1,300–1,500 masl), as well as two sites of temperate montane forests, i.e., Kanatal (1,800–2,000 masl) and Dhanolti (2,000–2,200 masl) (Figure 1). The climate of the study area falls under sub-tropical to temperate regions. The soil of the region is developed from granite-ferrous biotite, granite, gneiss, and phyllites. Usually, the soil color in this region ranges from brown to dark grayish and the soils are slightly acidic in reaction. The texture of the soil at Khadi was sandy loam, at Gaja, sandy clay loam, and at Kanatal and Dhanolti, it was loam.
Vegetation analysis
For vegetation analysis, a total of 50 quadrats (10 m2 × 10 m2 size) were laid out randomly in each forest site to study the structure, composition, and biomass carbon. The study area plants were identified by their local names and unidentified species specimens were brought to the laboratory and identified with the help of the Flora of District Garhwal, North West Himalaya (Gaur, 1999). The vegetation data were analyzed for density, frequency, and abundance (Curtis and McIntosh, 1950) and the importance value index (IVI) of species was calculated using the method of Phillips (1959). The Simpson’s index (Cd-concentration of dominance) calculated by Simpson (1949), Shannon–Wiener index (h) calculated by Shannon and Weaver (1963), and Margalef’s index (Margalef, 1968) were assessed using standard methods and the following formulae;
Where D is the Simpson’s index of dominance, Pi is the proportion of importance value of the ith species. Pi is the proportion of importance value of the ith species. Pi was calculated by dividing the importance value index (IVI) of the ith species (ni) by the IVI of all the species. H′ is the Shannon–Weiner index of diversity. The Dmg is Margalef’s index of richness, S is the total number of species, and N is the total number of individuals.
The impact of various anthropogenic pressure on forests and further on local people were assessed, i.e., fuel, fodder, timber, fruit, grazing, browsing, etc. This information was collected from the villagers during the field survey and based on other available information about the study area.
General volume equations were used to estimate standing tree volume for each species (FSI, 1996). Growing stock density (GSD) was estimated for an individual tree within a sample plot. The total volume was multiplied by the digit 100 for converting the growing stock volume density (GSVD) into m3 ha–1.
Some of the species with no volume equations were calculated based on the volume tables/equations of similar species representing similar height, form, taper, and growth rate. Further, the calculated GSVD was converted into aboveground biomass density (AGBD), which was calculated by multiplying it with the appropriate biomass expansion factor (BEF) (Brown and Schroeder, 1999). The BEFs for hardwood, spruce-fir, and pine were estimated by using the following equations:
Hardwood: BEF = exp [1.91–0.34 × In (GSVD)] for GSVD ≤ 200 m3 ha–1, BEF = 1.0 for GSVD > 200 m3 ha–1;
Spruce-fir: BEF = exp [1.77–0.34 × In (GSVD)] for GSVD ≤ 160 m3 ha–1, BEF = 1.0 for GSVD 160 m3 ha–1;
Pine: BEF = 1.68 Mg m for GSVD < 10 m3 ha–1. BEF = 0.95 for GSVD = 10–100 m3 ha–1. BEF = 0.81 (for GSVD > 10 m3 ha–1).
The equation of spruce-fir was used for other conifer species by using the regression equation (Brown and Schroeder, 1999). Using the regression equation (Cairns et al., 1997), the belowground biomass density (BGBD; fine and coarse roots) was also estimated for each forest type using the following equation:
Total biomass density (TBD) was calculated by adding the AGBD and BGBD. For estimating the total carbon density (TCD), the total biomass values were converted to carbon stock (C) by using a default value of 0.50 C fractions (IPCC, 2003).
Soil sampling and analysis
For the determination of soil properties, samples were collected from two different depths i.e., 0–15 cm and 15–30 cm from ten quadrats (10 m2 × 10 m2 size) laid in each site in both the sub-tropical (Khadi, Gaja) and temperate (Kanatal, Dhanolti) regions. The collected soil samples were air-dried, grounded, and sieved using a 2 mm sieve and further analysis was conducted in the Soil Science Laboratory, College of Forestry, Ranichauri, Tehri Garhwal, Uttarakhand. The bulk density (BD) of the soil was calculated using the core tube method. In order to take samples from the surface soil, the ground surface was cleared carefully to remove the entire residue followed by the collection of soil samples by inserting a core tube into the soil. The topsoil was excavated in rectangular volumes using a grub knife to reach the 15 cm and 30 cm soil layers and the sample was collected from the lower depths. The soil bulk density was calculated with the help of the following formula and expressed in g cm−3 (Duan et al., 2019).
The soil moisture content (gravimetric) was measured as the difference between the moist soil and the soil dried at 105°C in an oven, known as the oven-dry weight (Voroney, 2019). The percentage of soil moisture content was calculated as follows:
The particle density (PD) of the soil was determined by pycnometer methods as described by Heiskanen (1992).
The soil pH was determined with a dynamic digital pH meter using 1:2 soil water suspensions. SOC was estimated using Walkley and Black’s (1934) rapid titration method. SOC (%) was converted into soil organic matter (%) by multiplying by a factor of 1.724, i.e., SOC × 1.724. Olsen et al.’s (1954) method was used to determine the available phosphorus (P) in the soil. Available nitrogen (N) in soil was determined by using the Kjeldahl procedure (Subbiah and Asija, 1956). Available sulfur was determined using the 0.15% calcium chloride extraction method (Williams and Steinberg, 1959).
Statistical analysis
One-way analysis of variation (ANOVA) was performed for the analysis of soil data and the mean values were compared by Duncan’s multiple range test at p < 0.05 level of significance using SPSS 16.0 statistical software. The Pearson correlation coefficient was calculated among the different physical and chemical parameters of the soil and various vegetation parameters using SPSS-16 software.
Results
In this study, a total of 79 species belonging to different types of vegetation, i.e., trees, shrubs, and herbs, were found. At different altitudinal ranges, out of 79 species, 16 trees (belong to 16 genera and 14 families), 30 shrubs (belong to 28 genera and 26 families), and 33 herbs (belong to 30 genera and 18 families). The survey of the study area revealed that at sub-tropical forest sites (850–1,100 masl and 1,300–1,500 masl) trees are specifically being used for fuel, fodder, and minor timber, and forests also serve as grazing sites close to the villages. Thus in the sub-tropical forests, these services imparted a high pressure at 850–1,100 masl and medium pressure at 1,300–1,500 masl. On the other hand, the temperate forests (1,800–2,000 and 2,000–2,200 masl) were used for the collection of herbal medicines and wild fruits including fuel, fodder, and minor timbers (Table 2). However, the disturbance caused by grazing was reported as low pressure on the forests.
In the sub-tropical region, species diversity (H) parameters indicated that the maximum value of tree diversity, H′ = 0.48, was recorded at 850–1,100 masl, followed by H′ = 0.27 at 1,100–1,300 masl and the minimum value, H′ = 0.13, was recorded at 1,300–1,500 masl. In the temperate region, the highest tree diversity (H′ = 0.44) was at 1,800–2,000 masl, and the lowest was at 2,000–2,200 masl (H′ = 0.09). For the Simpson’s dominance index of the tree, the maximum (0.95) value was observed at 850–1,100 masl, and the minimum (0.18) at 1,300–1,500 masl in the sub-tropical region. For Margalef’s index of richness, the maximum (2.68) value was at 1,800–2,000 masl and the minimum (0.52) was noted at 1,300–1,500 masl in the sub-tropical montane forest (Table 3).
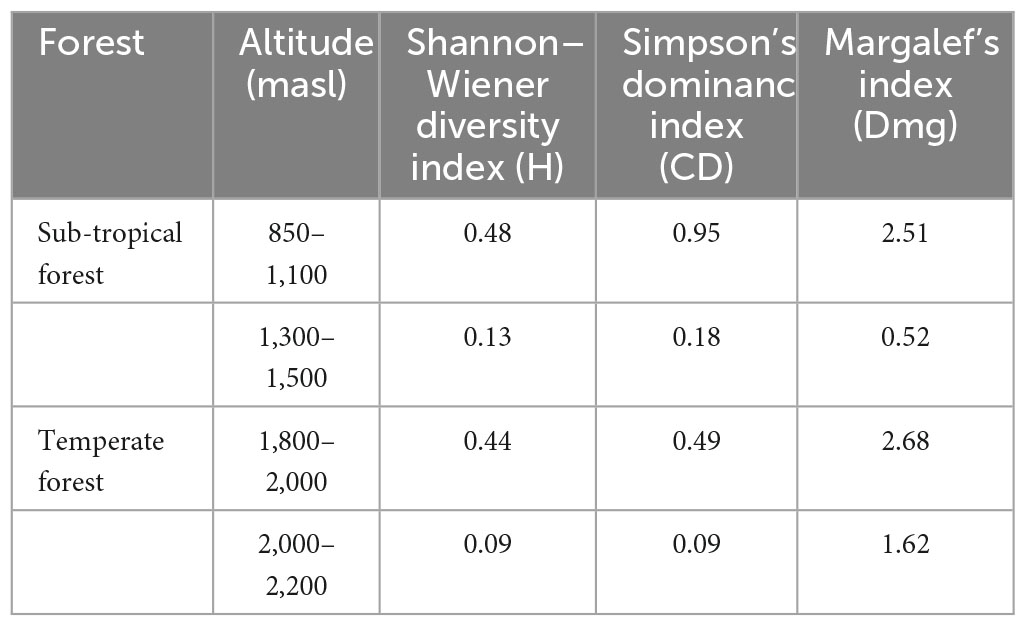
Table 3. Shannon–Wiener diversity, Simpson’s diversity, and Margalef diversity indices at different altitudes of sub-tropical and temperate forests.
At lower altitudes (850 and 1,100 masl), 10 trees were recorded with the dominance of P. roxburghii with maximum density and IVI. At the altitudinal range of 1,300–1,500 masl, the trees reported were P. roxburghii and Q. leucotrichophora, where P. roxburghii was dominant with the highest density (730 ind./ha) and IVI (265.56). At the altitude of 1,800–2,000 masl, a total of six trees were recorded and Q. leucotrichophora was dominant with the highest density (520 ind./ha) and IVI (172.31). At the altitudinal range of 2,000–2,200 masl, only four trees were recorded and C. deodara was dominant (IVI-272.54) with a density value of 690 ind./ha (Table 4).
At the different altitudinal ranges for sub-tropical forests, overall, the highest AGBD (596.31 Mg/ha), BGBD (133.46 Mg/ha), TBD (729.78 Mg/ha), and TCD (364.89 Mg/ha) were recorded at 1,300–1,500 masl where P. roxburghii was reported to be the dominant and Q. leucotrichophora the co-dominant tree species. However, the lowest values for AGBD, BGBD, TBD, and TCD were 270.94, 83.17, 354.18, and 177.06 Mg/ha, respectively, at 850–1,100 masl. In the temperate region, the highest values for AGBD, TBD, and TCD were reported as 338.25, 421.53, and 210.76 Mg/ha, respectively, at 2,000–2,200 masl, which was dominated by C. deodara while the maximum BGBD (89.18 Mg/ha) was recorded at 1,800–2,200 masl (Table 4).
Amongst the soil properties, a significant difference was recorded in soil BD at the 15–30 cm depth while a statistically non-significant difference was observed at the 0–15 cm depth. The difference in the values of PD of various sites and depths was also statistically non-significant. For the 0–15 cm depth, the moisture percentage of soil at 2,000–2,200 masl was reported as 14.83% which was significantly higher than the soil moisture at 850–1,100 masl (8.10%), 1,300–1,500 masl (8.24%), and 1,800–2,000 masl (10.62 %). Similarly, the soil pH in both depths varied significantly. The maximum value of pH in both the soil depths, i.e., 6.97 (0–15 cm) and 7.28 (15–30 cm) was observed at the lower altitudinal range of 850–1,100 masl. Significant variations in soil organic carbon and soil organic matter at both the soil depths were also observed and the higher values were recorded at higher altitudes ranging between 2,000 and 2,200 masl. The value of available N was recorded to be maximum at the altitude of 2,000–2,200 masl at both the soil depths and was significantly higher compared to the available N at other altitudes considered in the present study. The available P at 850–1,100 masl was significantly higher than the P at 1,800–2,000 masl and 2,000–2,200 masl. The values of available S also varied significantly (p < 0.05) among the different altitudes of the study sites. The maximum (29.88 mg/kg) value of available S was recorded at 850–1,100 masl and the lowest at 1,800–2,000 masl at the 0–15 cm soil depth (Table 5).
At the depth of 0–15 cm, a significant negative correlation was observed between BD and PD. A significant negative correlation was observed between SOC/Soil organic matter (SOM) with Moisture %. Both SOC and SOM were positively correlated with available S. A significant negative correlation between available P and PD was also observed. On the other hand, a significant positive correlation was recorded between TBD and AGBD, TBD and BGBD, and AGBD and BGBD (Table 6). At the 15–30 cm depth, BD showed a significant positive correlation with TBD, AGBD, and BGBD. The SOC had a significant positive correlation with SOM and N % and SOM with N %. A significant positive correlation was also recorded between TBD and AGBD, TBD and BGBD, and AGBD and BGBD (Table 7).
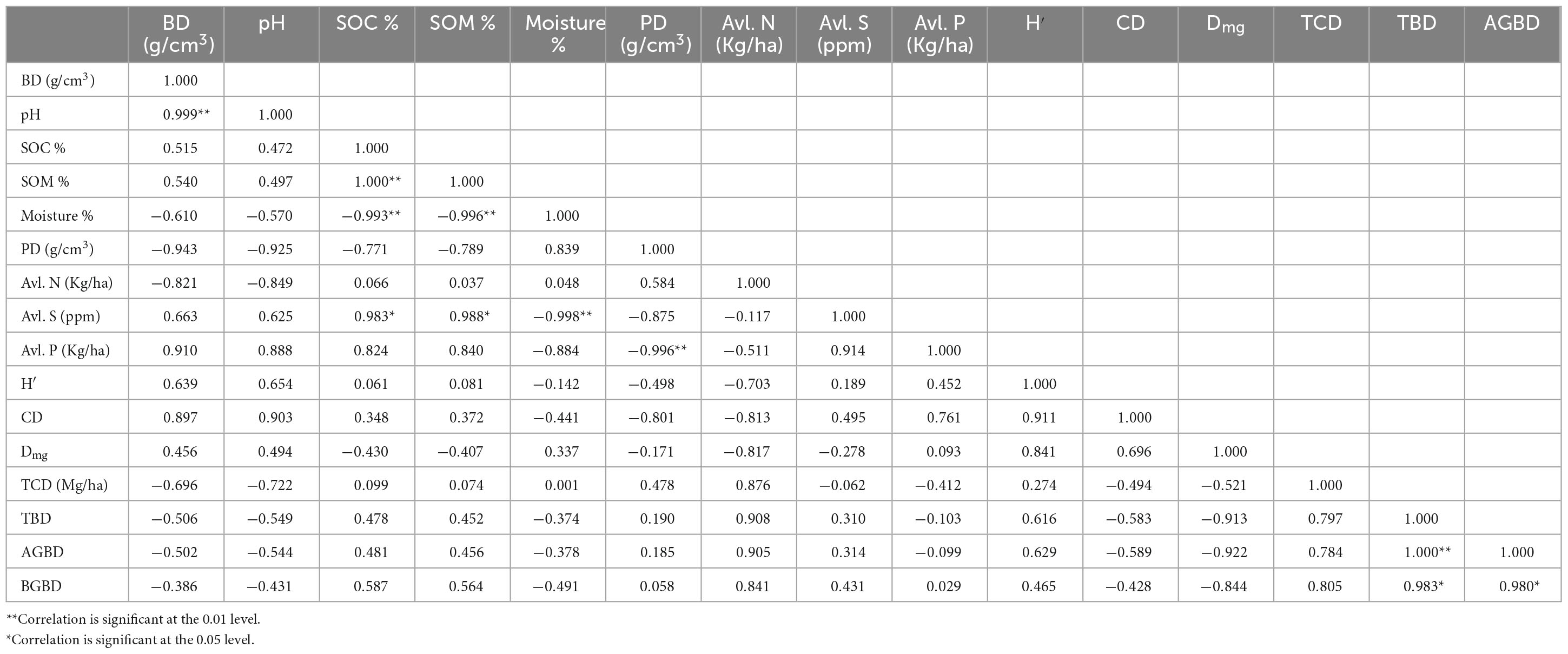
Table 6. Pearson correlation coefficient between different vegetation and soil parameters of the sub-tropical and temperate forest at 0–15 cm soil depth.
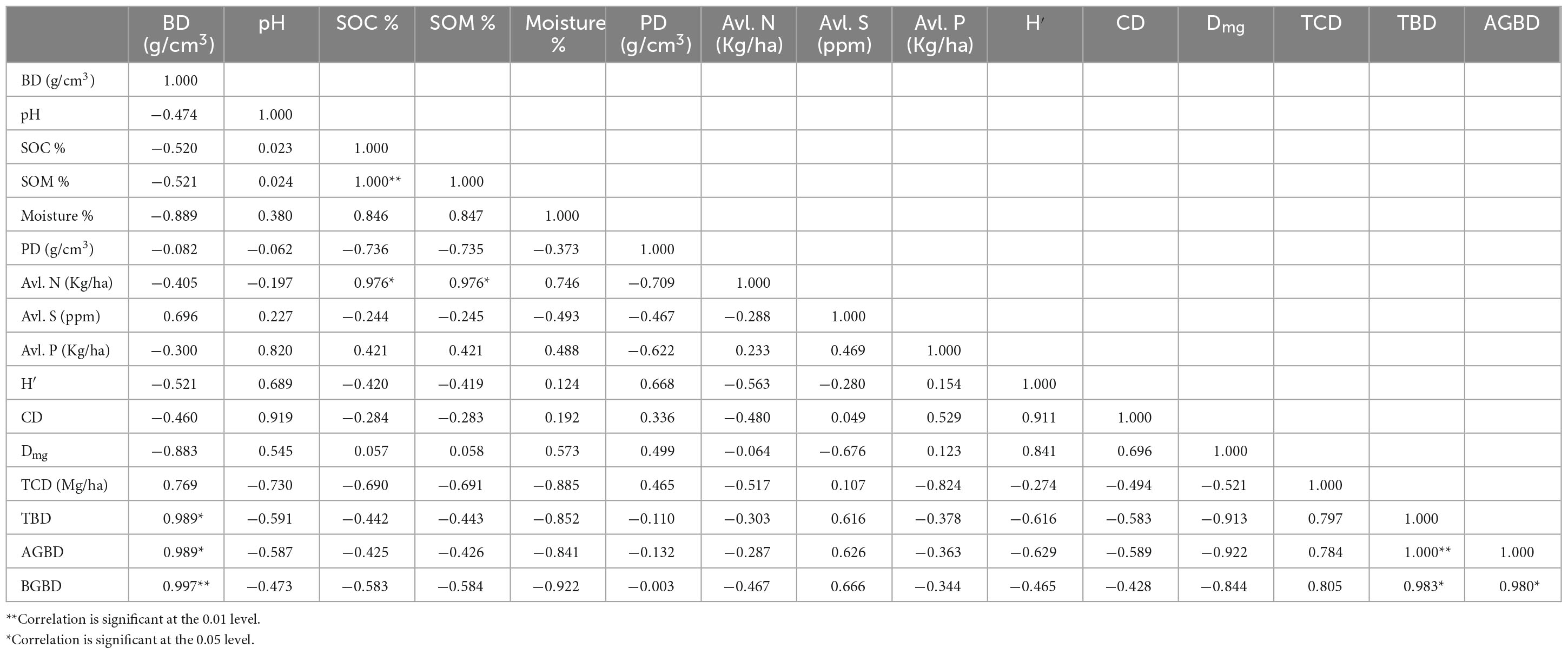
Table 7. Pearson correlation coefficient between different vegetation and soil parameters of the sub-tropical and temperate forest at 15–30 cm soil depth.
Discussion
Time plays an important role in the plant community to occupy an area of a region among many other factors such as altitude, aspect, slope, latitude, rainfall, and humidity which help the vegetation to grow and develop (Kharkwal et al., 2005). A total of 79 species of trees, shrubs, and herbs were recorded in different altitudes. The different species grow together in a community because they require similar environmental conditions for survival (Ter Braak, 1987). Q. leucotrichophora was the dominant tree at 1,800–2,000 masl and was also found co-dominant at 1,300–1,500 masl after P. roxburghii which suggests its ability to tolerate biotic pressures and wider ecological amplitude (Rawat S. et al., 2022).
The Shannon–Wiener index measures the scarcity and frequency of species in a habitat and usually varies from 1.5 to 3.5, not exceeding 4.5 (Kent and Coker, 1992), and the value observed is usually less in temperate forests. The maximum value for tree diversity (H′ = 0.48) at 850–1,100 masl was reported in the sub-tropical region, which could be because of human interference in these low-elevation forest areas which assisted the plantation and survival of exotic species (Rawal and Pangtey, 1994). A higher number of species was reported at lower elevations compared to higher elevations. These observations recorded in our study were in conformity with the findings of Wani et al. (2022). In the present investigation, the richness of non-native species such as Alnus nepalensis, Melia azedarach, and Mallotus philippensis, was more widespread in early succession/pioneer communities. In the temperate region, the highest value for tree diversity (H′ = 0.44) was recorded at 1,800–2,000 masl and a similar trend was also described by Kumar and Ram (2005). Further, the higher availability of nutrients in the soil at higher elevations favored the growth of most trees. Pausas and Austin (2001) suggested that the distribution of species richness in any large region is likely to be governed by two or more environmental factors.
The Simpson’s index exhibited the “concentration” of dominance and a higher value shows the dominance of one or a few species. The concentration of dominance (CD) for different life forms ranges between 0.12 and 0.80 for Himalayan regions (Tiwari and Tiwari, 2012). The CD of trees in the recent study ranged from 0.18 to 0.95 in sub-tropical regions at different altitudes. While in the temperate region, it ranged from 0.09 to 0.49. The higher reported CD values of trees in the sub-tropical region make it clear that the forest has less diversity and is mostly dominated by single species of P. roxburghii. The maximum value of CD of trees (0.49) was reported at 2,000–2,000 masl in the temperate region. Higher community stability indicates higher species richness which means greater species diversity (Swamy et al., 2000). The tree species richness was the highest at 1,800–2,000 masl and the least (1.62) was recorded at 2,000–2,200 masl in the temperate region. Dangwal et al. (2012) showed an increasing trend in the diversity of herbs and shrubs with increasing altitudes, whereas the diversity of tree species declined with increasing altitude in the western Himalayan region. Similar outcomes for species richness were reported in an elevation gradient study conducted by Sharma et al. (2017). The total species richness was found to be higher in warmer sites (lower elevation) as compared to the cooler sites (higher elevation).
The AGBD and BGBD ranged from 270.94 to 596.31 Mg/ha and 83.17 to 133.456 Mg/ha, respectively, while TBD ranged from 354.21 to 729.78 Mg/ha. The variation in the amount of biomass might be due to the differences in species composition at different altitudes. In this study, the average value of TBD was reported to be 541.98 Mg/ha in sub-tropical forests and 414.66 Mg/ha in temperate forests. These values were higher than the values reported by Gairola et al. (2011a) for similar forests in the Uttarakhand region. The average AGBD (381.04 Mg/ha) and BGBD (97.25 Mg/ha) in the present study were on par with the values given for different forests of the Garhwal Himalaya (Sharma et al., 2010). On average, the TCD value was 270.98 Mg/ha in the sub-tropical forests and 207.32 Mg/ha in the temperate forests, which falls within the range (92–640 Mg/ha) reported by Singh et al. (1994). The cites with minimum human or natural disturbance have optimum to higher values of biomass/carbon stock (Lugo and Brown, 1992). Higher capacity to produce high levels of biomass as reported in the mixed stands was generally due to the varying carbon fixing rates of different species as the majority of forest trees have a huge potential for short-term and long-term carbon storage (Houghton, 2005). In any forest’s ecosystems, large storage of carbon is recognized in big, long-lived tree species with dense wood (Bunker et al., 2005). The results of the present study also highlighted the disproportionate contribution of a small number of species viz., Cornus capitata, Ilex dipyrone, Myrica esculenta, and Lyonia ovalifolia to carbon stock as these areas contained a great amount of biomass and carbon storage. These species are slow-growing and survive under trees in these forests.
The soil moisture content in the present study sites was comparable to the reported values for the Himalayan region (Khera et al., 2001; Nazir, 2009). However, variation in moisture content might be because of altitude, rainfall, forest cover, types of vegetation, aspect, slope, water holding capacity, etc., and higher water absorbing characteristics of some trees (i.e., Q. leucotrichophora, Q. floribunda, and Q. semecarpifolia). The soil moisture content was reported higher with increasing altitude. Singh and Singh (1986) have also reported that the soil of oak forests was comparatively rich in moisture. In the present study, the maximum moisture content was reported at 2,000–2,200 masl in the Dhanolti region which was associated with lower temperatures, leading to low evaporation rate and soil moisture loss.
Soil acidity and alkalinity indicate the soil pH. Leskiw (1998) reported that forest soils require slightly acidic pH values for nutrient supply. The pH values of the present study varied from 6.86 to 7.21 at 850–1,500 masl (sub-tropical forests) and 5.63 to 6.24 at 1,800–2,200 masl (temperate forests). All the sites of the temperate region had pH levels below 6.0, at 0–15 cm depth, owing to an uninterrupted layer of high organic matter content. The higher assemblage of SOC and release of weak organic acids due to the slow decomposition rate usually lowers the soil pH (De Hann, 1977; Robertson and Vitousek, 1981; Adams and Sidle, 1987, Mishra et al., 2017a). However, higher pH values than the present study were found in the Kumaun region (Khera et al., 2001). The highest soil organic carbon percentage was found in Dhanolti (2,000–2,200 masl) in temperate forests and the lowest in Khadi in sub-tropical forests (850–1,100 masl). The SOM-enriched soil of the higher altitude zone of the Dhanolti site has been ascribed to low temperature and slower decomposition rate (Dimri et al., 1997). The lower SOM content in the Khadi has been attributed to the meager status of understory vegetation in the region. Furthermore, lesser understory vegetation facilitates the loss of topsoil and SOM sediments through erosion (Li et al., 2015; Song et al., 2020). Among the essential nutrients for plants, N is an essential primary nutrient that is responsible for the vegetative growth of plants (Rawat D. et al., 2022). The deficiency of N in plants may be seen as the yellowing of leaves and stunted growth. The values of N at the present study sites were comparable to the values observed in various previous works (Khera et al., 2001, Srivastava et al., 2005; Semwal, 2006; Sharma et al., 2010b) in the Uttarakhand Himalaya. The maximum value of soil N in the study sites was in the upper layers because of the large amount of litter and humus in the upper layer and possible leaching of N in lower altitudes due to the presence of a coarser fraction in the soil (Saha et al., 2018). The available P in the soil was recorded to be significantly higher at 850–1,100 masl compared to the temperate areas at both depths. Similar findings were recorded by Rawat et al. (2020) in the montane forests of the Garhwal Himalaya. This trend could be associated with the pH of the soil which is near neutral in case of 850–1,100 m because P remains in plant-available forms in neutral pH whereas low pH leads to its fixation in insoluble forms in the soil (Murphy, 1958; Mishra et al., 2017b). The available S decreased with an increase in soil depths due to greater inputs of organic matter on the surface soil (Amanuel et al., 2018).
The present study area has shown that pressure from the local people on the forests existed. Singh et al. (2022) observed that, on average, 15.01% of the forests in the Garhwal Himalaya were disturbed annually from 2005 to 2018. However, the pressure is based on the requirements of the local people and the availability of resources in the different altitudinal ranges. It has been noticed that lower altitudes are rich in species used by the local villagers and thus anthropogenic activities are reported dominant. Similar findings were documented by Singh et al. (2018) as the unmanaged extraction of trees through the collection of fuel, fodder, and overgrazing was found to be the major factor of forest disturbance along an altitudinal range of < 1,700 to > 1,900 masl in the Garhwal Himalaya. The field surveys in our investigation also confirmed the fact that at lower altitudes, the villagers were residing closer to the forest environment, and the dependence on a thick population has increased the level of pressure on forest resources as compared to the temperate region. The resources availed of by the villagers in the study area were fuel, fodder, fiber, timber, fruits, etc., along with other associated requirements such as grazing and browsing. Anthropogenic activities such as tree cutting and lopping were also seen prominently at lower altitudes of temperate forests in the Tehri and Uttarkashi districts of the Garhwal Himalaya (Tewari and Sharma, 2023). Overall, this study presented an insight into the condition of the sub-tropical forests in the study area which are under high pressure due to the large-level needs of the surrounding population as compared to the forests at high altitudinal ranges.
Conclusion
The intervention of human activities in the lower altitude forests of the sub-tropical region with the introduction of non-native tree species and the probable climatic gradient developed in the altitude ranges of temperate forests accounted for the diversity of tree species in these forests. Amongst the species, P. roxburghii dominated the altitudinal ranges of 1,100–1,300 masl and 1,300–1,500 masl in sub-tropical forests, with higher values of AGBD, BGBD, TBD, and total carbon stock signifying its superior carbon sequestration potential. The contribution of species such as Q. leucotrichophora, Q. floribunda, R. arboreum, and C. deodara, improved the soil moisture, SOC, and available N in the soil in the temperate forests. However, the lower availability of P and S was influenced by the low pH of the soil. The present study indicated that coniferous species such as P. roxburghii, in the sub-tropical forests and Q. leucotrichophora, C. deodara in the temperate forests of the Garhwal Himalaya have high carbon storage potential and require conservation and carbon management attention through plantation and natural regeneration because regular pressure on the forests for various purposes and resources by the villagers may affect the species survival and further carbon sequestration potential. Although, this study was limited to four sites in the Garhwal Himalaya, the findings contribute to an important understanding of species distribution and soil health in sub-tropical and temperate montane forests which may further be helpful for long-term monitoring of and assessment studies on ongoing anthropogenic disturbances. The framework of management activities focusing on forest conservation may also be developed with the help of information gathered from this investigation.
Data availability statement
The raw data supporting the conclusions of this article will be made available by the authors, without undue reservation.
Author contributions
AT experimented, data collection, and prepared the first draft. DR and BS helped in the analysis of data and preparation of the first manuscript. VK and DR designed the research and supervised the research. VK, DR, GM, and MK reviewed and modified the manuscript. MR analyzed the data and software. All authors contributed to the manuscript writing, revised and approved the submitted version.
Acknowledgments
We are thankful to the College of Forestry, VCSG Uttarakhand University of Horticulture and Forestry, Ranichauri, Tehri Garhwal, Uttarakhand for providing all the facilities.
Conflict of interest
The authors declare that the research was conducted in the absence of any commercial or financial relationships that could be construed as a potential conflict of interest.
Publisher’s note
All claims expressed in this article are solely those of the authors and do not necessarily represent those of their affiliated organizations, or those of the publisher, the editors and the reviewers. Any product that may be evaluated in this article, or claim that may be made by its manufacturer, is not guaranteed or endorsed by the publisher.
References
Adams, P. W., and Sidle, R. C. (1987). Soil conditions in three recent landslides in southeast Alaska. For. Ecol. Manag. 18, 93–102. doi: 10.1016/0378-1127(87)90136-8
Amanuel, W., Yimer, F., and Karltun, E. (2018). Soil organic carbon variation in relation to land use changes: The case of birr watershed, upper Blue Nile River Basin, Ethiopia. J. Ecol. Environ. 42:16. doi: 10.1186/s41610-018-0076-1
Anderegg, W. R., Schwalm, C., Biondi, F., Camarero, J. J., Koch, G., Litvak, M., et al. (2015). Pervasive drought legacies in forest ecosystems and their implications for carbon cycle models. Science 349, 528–532.
Augusto, L., Ranger, J., Binkley, D., and Rothe, A. (2002). Impact of several common tree species of European temperate forests on soil fertility. Ann. Sci. 59, 233–253. doi: 10.1051/forest:2002020
Brown, S., and Schroeder, P. E. (1999). Spatial patterns of aboveground production and mortality of woody biomass for eastern US forests. Ecol. Applic. 9, 968–980. doi: 10.2307/2641343
Bunker, D. E., DeClerck, F., Bradford, J. C., Colwell, R. K., Perfecto, I., Phillips, O. L., et al. (2005). Species loss and aboveground carbon storage in a tropical forest. Science 310, 1029–1031. doi: 10.1126/science.1117682
Cairns, M. A., Brown, S., Helmer, E. N., and Baumgardener, G. A. (1997). Root biomass allocation in the world’s upland forests. Oecologia 111, 1–11. doi: 10.1007/s004420050201
Chaturvedi, O. P., and Singh, J. S. (1987). The structure and function of pine forest in Central Himalaya. I. dry matter dynamics. Ann. Bot. 60, 237–252. doi: 10.1093/aob/mcf004
Curtis, J. T., and McIntosh, R. (1950). The interrelations of certain analytic and synthetic phytosociological characters. Ecology 31, 434–455. doi: 10.2307/1931497
Dangwal, L. R., Singh, T., Singh, A., and Sharma, A. (2012). Plant diversity assessment in relation to disturbances in subtropical chir pine forest of the Western Himalaya of district Rajouri. J and K, India. Int. J. Plant Anim. Environ. Sci. 2, 206–213.
De Hann, S. (1977). Humus, its formation, its relation with the mineral part of the soil and its significance for soil productivity. Vienna: International Atomic Energy Agency.
Dimri, B. M., Jha, M. N., and Gupta, M. K. (1997). Status of soil nitrogen at different altitudes in Garhwal Himalaya. Van Vigyan 359, 77–84.
Dong, H., Lin, J., Lu, J., Li, L., Yu, Z., Kumar, A., et al. (2022). Priming effects of surface soil organic carbon decreased with warming: A global meta-analysis. Plant Soil doi: 10.1007/s11104-022-05851-1 [Epub ahead of print].
Duan, A., Lei, J., Hu, X., Zhang, J., Du, H., Zhang, X., et al. (2019). Effects of planting density on soil bulk density, pH and nutrients of un-thinned Chinese fir mature stands in south subtropical region of China. Forests 10:351. doi: 10.3390/f10040351
FSI (1996). Volume equations for forests of India, Nepal and Bhutan. Dehradun: Forest Survey of India.
Gairola, S., Sharma, C. M., Ghildiyal, S. K., and Suyal, S. (2011a). Live tree biomass and carbon variation along an altitudinal gradient in moist temperate valley slopes of the Garhwal Himalaya (India). Curr. Sci. 100, 1862–1870.
Gairola, S., Sharma, C. M., Ghildiyal, S. K., and Suyal, S. (2011b). Tree species composition and diversity along an altitudinal gradient in moist tropical montane valley slopes of the Garhwal Himalaya, India. For. Sci. Technol. 7, 91–102. doi: 10.1080/21580103.2011.597109
Gaur, R. D. (1999). Flora of the District Garhwal North West Himalaya (with ethnobotanical notes). Srinagar: Transmedia Publication.
Gauthier, G., Berteaux, D., Bêty, J., Tarroux, A., Therrien, J. F., McKinnon, L., et al. (2011). The tundra food web of Bylot Island in a changing climate and the role of exchanges between ecosystems. Ecoscience 18, 223–235.
Gupta, M. K., and Sharma, S. D. (2011). Sequestrated carbon: Organic carbon pool in the soils under different forest covers and land uses in Garhwal Himalayan region of India. Int. J. Agric. For. 1, 14–20.
Heiskanen, J. (1992). Comparison of three methods for determining the particle density of soil with liquid pycnometers. Soil Sci. Plant Anal. 23, 841–846. doi: 10.1080/00103629209368633
Houghton, R. A. (2005). Aboveground forest biomass and the global carbon balance. Glob. Change Biol. 11, 945–958. doi: 10.1111/j.1365-2486.2005.00955.x
IPCC (2003). Good practice guidance for land use, land-use change, and forestry. Hayama: IPCC National Greenhouse Gas Inventories Programme.
Kent, M., and Coker, P. (1992). Vegetation description and analysis a practical approach. Boca Raton, FL: CRC Press, 363.
Kharkwal, G., Mehrotra, P., Rawat, Y. S., and Pangtey, Y. P. S. (2005). Phytodiversity and growth form in relation to altitudinal gradient in the Central Himalayan (Kumaun) region of India. Curr. Sci. 89, 873–878.
Khera, A. N., Kumar, A., and Ram, J. (2001). Plant biodiversity assessment in relation to disturbances in mid-elevation forest of Central Himalaya, India. Trop. Ecol. 42, 83–95.
Kumar, A., Yu, Z., Klemeš, J. J., and Bokhari, A. (2021b). A state-of-the-art review of greenhouse gas emissions from Indian hydropower reservoirs. J. Clean. Prod. 320:128806. doi: 10.1016/j.jclepro.2021.128806
Kumar, S., Bijalwan, A., Singh, B., Rawat, D., Yewale, A. G., Riyal, M. K., et al. (2021c). Comparison of carbon sequestration potential of Quercus leucotrichophora–based agroforestry systems and natural forest in central Himalaya, India. Water Air Soil Pollut. 232:350.
Kumar, A., Kumar, M., Pandey, R., ZhiGuo, Y., and Cabra-Pinto, M. (2021a). Forest soil nutrient stocks along altitudinal range of Uttarakhand Himalayas: An aid to nature based climate solutions. Catena 207:105667. doi: 10.1016/j.catena.2021.105667
Kumar, A., and Ram, J. (2005). Anthropogenic disturbances and plant biodiversity in forests of Uttaranchal, Central Himalaya. Biodivers. Conserv. 14, 309–331.
Kumar, A., Yang, T., and Sharma, M. P. (2019). Long-term prediction of greenhouse gas risk to the Chinese hydropower reservoirs. Sci. Total Environ. 646, 300–308. doi: 10.1016/j.scitotenv.2018.07.314
Leskiw, L. A. (1998). Land capability classification for forest ecosystem in the oil stands region. Edmonton: Alberia Environmental Protection.
Li, Y., Yu, H. Q., Zhou, N., Tian, G., Poesen, J., and Zhang, Z. D. (2015). Linking fine root and understory vegetation to channel erosion in forested hillslopes of southwestern China. Plant Soil 389, 323–334. doi: 10.1007/s11104-014-2362-8
Lugo, A. E., and Brown, S. (1992). Tropical forests as sinks of atmospheric carbon. For. Ecol. For. Manage. 54, 239–255.
Malhi, Y., Meir, P., and Brown, S. (2002). Forests, carbon and global climate. Philos. Trans. R. Soc. Lond. Ser. A 360, 1567–1591.
Meena, V. S., Ghosh, B. N., Singh, R. J., Bhattacharyya, R., Sharma, N. K., Alam, N. M., et al. (2021). Land use types and topographic position affect soil aggregation and carbon management in the mountain agroecosystems of the Indian Himalayas. Land Degrad. Dev. 32, 3992–4003. doi: 10.1002/ldr.3864
Mishra, A., Swamy, S. L., Thakur, T. K., Kumar, A., and Pandey, M. (2022). Impact of coal mining on land use changes, deforestation, biomass, and C losses in Central India: Implications for offsetting CO2 emissions. Land Degrad. Dev. 33, 3374–3731. doi: 10.1002/ldr.4419
Mishra, G., Das, P. K., Borah, R., and Dutta, A. (2017a). Investigation of phytosociological parameters and physicochemical properties in northern tropical semi-evergreen Forests of Eastern Himalaya. J. For. Res. 28, 513–520.
Mishra, G., Debnath, S., and Rawat, D. (2017b). Managing phosphorus in terrestrial ecosystem: A review. Eur. J. Biol. Res. 7, 255–270.
Murphy, H. P. (1958). The fertility status of some soils of Ethiopia. Alemaya: College of Agriculture and Mechanical Arts.
Nazir, T. (2009). Estimation of site quality of important temperate forest covers on the basis of soil nutrients and growing stock in Garhwal Himalaya. Ph.D. thesis. Srinagar: HNB Garhwal University.
Negi, J. D. S., Manhas, R. K., and Chauhan, P. S. (2003). Carbon allocation in different components of some tree species of India: A new approach for carbon estimation. Curr. Sci. 85, 1528–1531.
Olsen, S. R., Cole, C. L., Watanabe, F. S., and Dean, D. A. (1954). Estimation of available phosphorus in soil by extraction with sodium bicarbonate. Washington, DC: US Government Printing Office.
Pausas, J. G., and Austin, M. P. (2001). Patterns of plant species richness in relation to different environments: An appraisal. J. Veg. Sci. 12, 153–166.
Rawal, R. S., and Pangtey, Y. P. S. (1994). High altitude forests in a part of Kumaun, Central Himalaya. Proc. Ind. Natl. Sci. Acad. 60, 557–564.
Rawat, D., Mukhopadhyay, D., Mishra, G., and Bijalwan, A. (2022). “Soil nitrogen dynamics and management in agroforestry systems for ecological sustainability,” in Land degradation neutrality: Achieving SDG 15 by forest management, eds P. Panwar, G. Shukla, J. A. Bhat, and S. Chakravarty (Singapore: Springer), doi: 10.1007/978-981-19-5478-8_20
Rawat, S., Khanduri, V. P., Singh, B., Riyal, M. K., Thakur, T. K., Kumar, M., et al. (2022). Variation in carbon stock and soil properties in different Quercus leucotrichophora forests of Garhwal Himalaya. Catena 213:106210. doi: 10.1016/j.catena.2022.106210
Rawat, D., Sati, S. P., Khanduri, V. P., Riyal, M., and Mishra, G. (2021). “Carbon sequestration potential of different land use sectors of Western Himalaya,” in Advances in carbon capture and utilization. energy, environment, and sustainability, eds D. Pant, A. Kumar Nadda, K. K. Pant, and A. K. Agarwal (Singapore: Springer), doi: 10.1007/978-981-16-0638-0_12
Rawat, D. S., Tiwari, P., Das, S. K., and Tiwari, J. K. (2020). Tree species composition and diversity in montane forests of Garhwal Himalaya in relation to environmental and soil properties. J. Mt. Sci. 17, 3097–3111. doi: 10.1007/s11629-019-5761-8
Robertson, G. P., and Vitousek, P. M. (1981). Nitrification in primary and secondary succession. Ecology 1981, 376–386. doi: 10.1007/BF00396734
Saha, S., Rajwar, G. S., and Kumar, M. (2018). Soil properties along altitudinal gradient in Himalayan temperate forest of Garhwal region. Acta Ecol. Sin. 38, 1–8. doi: 10.1016/j.chnaes.2017.02.003
Semwal, S. (2006). Studies on phytosociology, diversity patterns and competition along an altitudinal gradient in a part of lesser Himalaya in Garhwal, Uttaranchal. Ph.D. Thesis. Srinagar: HNB Garhwal University.
Shannon, C. E., and Weaver, W. (1963). The mathematical theory of communication. Urbana, IL: University of Illinois Press.
Sharma, C. M., Baduni, N. P., Gairola, S., Ghildiyal, S. K., and Suyal, S. (2010a). Tree diversity and carbon stocks of some major forest types of Garhwal Himalaya, India. For. Ecol. Manage. 260, 2170–2179.
Sharma, C. M., Gairola, S., Ghildiyal, S. K., and Suyal, S. (2010b). Physical properties of soils in relation to forest composition in moist temperate valley slopes of the Central Western Himalaya. J. For. Sci. 26, 117–129.
Sharma, C. M., Gairola, S., Baduni, N. P., Ghildiyal, S. K., and Suyal, S. (2011). Variation in carbon stocks on different slope aspects in seven major forest types of temperate region of Garhwal Himalaya, India. J Biosci. 36, 701–708. doi: 10.1007/s12038-011-9103-4
Sharma, C. M., Mishra, A. K., Tiwari, O. P., Krishan, R., and Rana, Y. S. (2017). Effect of altitudinal gradients on forest structure and composition on ridge tops in Garhwal Himalaya. Energ. Ecol. Environ. 2, 404–417. doi: 10.1007/s40974-017-0067-6
Sharma, H., Pant, K. S., Bishist, R., Gautam, L., Ludarmani, D. R., Kumar, M., et al. (2023). Estimation of biomass and carbon storage potential in agroforestry systems of north western Himalayas, India. Catena 225:107009. doi: 10.1016/j.catena.2023.107009
Sheikh, M. A., and Kumar, M. (2010). Nutrient status and economic analysis of soils in oak and pine forests in Garhwal Himalaya. J. Am. Sci. 6, 117–122.
Singh, G., Padalia, H., Rai, I. D., Bharti, R. R., and Rawat, G. S. (2016). Spatial extent and conservation status of Banj oak (Quercus leucotrichophora A. Camus) forests in Uttarakhand, Western Himalaya. Trop. Ecol. 57, 255–262.
Singh, H., Kumar, M., and Sheikh, M. A. (2009). Distribution pattern of oak and pine along altitudinal gradients in Garhwal Himalaya. Nat. Sci. 7, 81–85.
Singh, J. S., and Singh, S. P. (1986). Structure and function of central Himalaya oak forests. Proc. Ind. Natl. Acad. 96, 156–189.
Singh, R., Kumar, P., Pandey, R., and Bala, N. (2022). Forest disturbance detection in Garhwal Himalayas using MODIS NDVI time-series and BFAST model. Geocarto Int. 37, 12689–12708. doi: 10.1080/10106049.2022.2071472
Singh, S. P., Adhikari, B. S., and Zobel, D. B. (1994). Biomass productivity, leaf longevity and forest structure in the Central Himalaya. Ecol. Monogr. 64, 401–421.
Singh, V., Singh, D. S., and Dasgupta, S. (2018). Effect of stand structure and aspect on the regeneration of banj oak (Quercus leucotrichophora A. Camus) forest along disturbance in Garhwal Himalaya, Uttarakhand, India. For. Stud. 68, 33–39. doi: 10.2478/fsmu-2018-0003
Song, L., Boithias, L., Sengtaheuanghoung, O., Oeurng, C., Valentin, C., Souksavath, B., et al. (2020). Understory limits surface runoff and soil loss in teak tree plantations of Northern Lao PDR. Water 12:2327. doi: 10.3390/w12092327
Srivastava, R. K., Khanduri, V. P., Sharma, C. M., and Kumar, P. (2005). Structure, diversity and regeneration potential of oak-dominant conifer mixed forest along an altitudinal gradient in Garhwal Himalaya. Indian For. 131, 1537–1553.
Subbiah, B. V., and Asija, G. L. (1956). A rapid method for estimation of available N in soil. Curr. Sci. 25, 259–260.
Swamy, P. S., Sundarapandian, S. M., Chandrasekar, P., and Chandrasekaran, S. (2000). Plant species diversity and tree population structure of a humid tropical forest in Tamil Nadu, India. Biodivers. Conserv. 9, 1643–1669.
Tariyal, N., Bijalwan, A., Chaudhary, S., Singh, B., Dhanai, C. S., Tewari, S., et al. (2022). Crop production and carbon sequestration potential of Grewia oppositifolia-based traditional agroforestry systems in Indian Himalayan Region. Land 11:839.
Ter Braak, C. J. E. (1987). The analysis of vegetation-environment relationships by canonical correspondence analysis. Vegetatio 69, 69–77.
Tewari, O. P., and Sharma, C. M. (2023). Anthropogenic disturbance impact on forest composition and dominance-diversity: A case of an ecosensitive region of Garhwal Himalaya, India. Acta Ecol. Sin. 43, 662–673. doi: 10.1016/j.chnaes.2022.08.003
Tiwari, P. K., and Tiwari, S. C. (2012). Environmental regeneration of woody species: A case study of forest ecosystems of submontane and montane zones of Garhwal Himalaya, India. Saarbrücken: LAP LAMBERT Academic Publishing.
Tripathi, O. P., and Khongjee, R. (2010). Effect of forest fragment size on tree diversity and population structure of humid subtropical forest of Meghalaya. Biodivers. Sci. 18, 208–214.
Verma, A. K., and Garkoti, S. K. (2019). Population structure, soil characteristics and carbon stock of the regenerating banj oak forests in Almora, Central Himalaya. For. Sci. Technol. 15, 117–127. doi: 10.1080/21580103.2019.1620135
Voroney, P. (2019). “Soils for horse pasture management,” in Horse pasture management, ed. S. Paul (Cambridge, MA: Academic Press).
Walkley, A., and Black, J. A. (1934). Estimation of soil organic carbon by chromic acid filtration method. Soil Sci. 37, 38–39.
Waltham, N. J., Elliott, M., Lee, S. Y., Lovelock, C., Duarte, C. M., Buelow, C., et al. (2020). UN decade on ecosystem restoration 2021–2030—what chance for success in restoring coastal ecosystems? Front. Mar. Sci. 7:71. doi: 10.3389/fmars.2020.00071
Wani, Z. A., Khan, S., Bhat, J. A., Malik, A. H., Alyas, T., Pant, S., et al. (2022). Pattern of β-diversity and plant species richness along vertical gradient in Northwest Himalaya, India. Biology 11:1064. doi: 10.3390/biology11071064
Keywords: montane sub-tropical, montane temperate, carbon stock, tree diversity, SOC range, Garhwal Himalayas
Citation: Tiwari A, Khanduri VP, Rawat D, Singh B, Riyal MK, Mishra G and Kumar M (2023) Vegetation composition, soil properties, and carbon stock of montane forests along a disturbance in the Garhwal Himalaya, India. Front. For. Glob. Change 6:1193792. doi: 10.3389/ffgc.2023.1193792
Received: 25 March 2023; Accepted: 14 July 2023;
Published: 04 August 2023.
Edited by:
Mehebub Sahana, The University of Manchester, United KingdomReviewed by:
Amit Kumar, Nanjing University of Information Science and Technology, ChinaPravin Rawat, ICFRE-Himalayan Forest Research Institute, Shimla, India
Shashi Sekhar, Banaras Hindu University, India
Copyright © 2023 Tiwari, Khanduri, Rawat, Singh, Riyal, Mishra and Kumar. This is an open-access article distributed under the terms of the Creative Commons Attribution License (CC BY). The use, distribution or reproduction in other forums is permitted, provided the original author(s) and the copyright owner(s) are credited and that the original publication in this journal is cited, in accordance with accepted academic practice. No use, distribution or reproduction is permitted which does not comply with these terms.
*Correspondence: Vinod Prasad Khanduri, a2hhbmR1cml2cEB5YWhvby5jb20=; Deepa Rawat, cmF3YXRkZWVwYTI5MUBnbWFpbC5jb20=; Bhupendra Singh, YnV0b2xhX2JzQHlhaG9vLmNvLmlu