- 1Division of Silviculture and Agroforestry, Faculty of Forestry SKUAST-K, Malangpora, Jammu and Kashmir, India
- 2KVK, Directorate of Research, Malangpora, Jammu and Kashmir, India
- 3Directorate of Research, Malangpora, Jammu and Kashmir, India
- 4Division of FPU, Malangpora, Jammu and Kashmir, India
- 5Division of NRM-SKUAST-Kashmir, Srinagar, Jammu and Kashmir, India
Agroforestry is an age-old practise of integrating farming with practises, preferably on the same unit of land on sustainable basis. There is tremendous scope for Agroforestry because India has achieved self-sufficiency in food production. Now its attention is becoming more focused on the ecological problems and shortage of fuel, fodder and other outputs as well as unemployment. So, agroforestry land use systems offer a better option by serving as carbon sinks and contributing in attaining food safety, subsistence income, and habitat amelioration. The current study was conducted in this context in the south Kashmir area of India with the objective of recognising the capability of various agroforestry systems for biomass estimation and carbon sequestration assessment for mitigating climate variation. The present study entitled “Biomass production, carbon stock and sequestration potential of prominent agroforestry systems in North-western Himalaya, India” was carried out, during 2018–2019. The study site is located between 33° 72’N and 75° 14’E at an altitude ranging from 2,900 to 5,900 M (amsl). Multistage random sampling technique was used for selection of sampled tehsils, blocks, villages and farmers in Anantnag district. The first stage was the random sampling of six tehsils namely Anantnag, Shangus, Kokernag, Dooru, Sallar, and Larnu and blocks per tehsil namely, viz.; Anantnag, Shangus, Sagam, Shahabad, Pahalgam and Larnu. Then four villages within each block like Anantnag (Ruhoo, Dialgam, Wanihama and Donipawa), Shangus (Shangus, Nowgam, Kutheir and Andoora), Sagam (Hiller, Khalhar, Sagam and Bidder), Shahabad (Larkipora, Shankerpora, Nathipora and Bragam), Pahalgam (Yanner, Charigam, Movra and Wahdan) and Larnu (Leesu, Dandipora, Bidhard and Larnu). Ten farmers within each village were selected, thus making a total of 240 farmers, which accounts 25% sampling intensity. Four agroforestry systems including horti-agriculture, agrisilviculture, (boundary planting), agri-horti-silviculture and horti-silvipasture were chosen for the study as the experimental configuration. Maximum biomass was found in horti-silvipastoral contributing 66.55 t ha−1 followed by agri-horti-silviculture as 50.18 t ha−1, horti-agriculture as 36.22 t ha−1 and agri-silviculture as 34.87 t ha−1. The maximum mean biomass of fruit trees (30.54 t ha−1), forest trees (25.19 t ha−1), grass (7.18 t ha−1) during the first cut and 3.64 t ha−1 during the second cut, was calculated in horti-silvipasture system. The overall biomass output of diverse agroforestry systems goes along with the sequence: horti-silvipasture > agri-horti-silviculture > horti-agriculture > boundary plantation, respectively. As a result, estimates of carbon stocks and carbon sequestration for all systems showed the same trend as values for biomass since they are both directly related to the capacity for biomass generation of various plant components. By providing for rural livelihoods and serving as an efficient carbon sink through carbon sequestration, these agroforestry systems in the Himalayas can significantly increase total production and productivity. The results of the current study could have a big impact on how alternative agroforestry systems and tree combinations are chosen for efficient carbon budget management in delicate Himalayan ecosystems.
1. Introduction
In the modern era of global warming, interconnected climate indicators provide a comprehensive view of climate change and the rising threats to the Sustainable Development Goals (SDGs) affecting environmental, social, and economic systems. Carbon dioxide (CO2) is the principal greenhouse gas (GHG) produced as a result of anthropogenic activity and the cumulative impact of humans during the past, along with industrialisation (Malhi et al., 2021; Zandalinas et al., 2021). The well-known “greenhouse effect” is the link between CO2 and global warming that leads to climate change (Anderson et al., 2016). According to the WMO (2021), the amount of CO2 concentration in the earth’s atmosphere is around 413.20 0.2 ppm, and 55% of it is absorbed by oceans and land vegetation.
Since the middle of the 20th century, anthropogenic landscape activity has been a significant contributor to climate change, which accelerated in 2020 and 2021 despite a 5.6% decrease in fossil fuel CO2 emissions as a result of COVID-19 pandemic restrictions (WMO, 2021). Climate change and the rise in land-use conflicts necessitate the creation of sustainable land-use systems that balance agricultural production with the provisioning of several ecological services. According to global estimates, land use interventions help reduce emissions by roughly 30% through the process of carbon sequestration and are crucial for achieving the carbon reduction goal set by the COP-25 summit (Aynekulu et al., 2020). According to Mangalassery et al. (2014), carbon capture has been considered as a way to help minimise the rising CO2 accumulation in the environment. The soil and plant biomass that make up the large terrestrial carbon pool have the ability to take in and store atmospheric carbon through photosynthesis (Kaul et al., 2010).
It is well known that forestry can improve carbon sinks and lower CO2 emissions, thus playing a crucial role in carbon cycling and aids in mitigating climate change (Besar et al., 2020; Sarkar et al., 2021). However, the dynamics of nutrients (carbon and nitrogen) in the terrestrial environment were affected by rapid increases in urbanisation and anthropogenic activity (deforestation, land use change, biodiversity loss, and forest degradation; Dhyani et al., 2021; Mey and Gore, 2021; Kumar et al., 2022). However, it is impossible to ignore the role that trees outside forests (TOFs) play in enhancing human well-being and offering ecosystem services. In addition to supplying the needs for timber, industry, and livelihoods in both agricultural and human-dominated landscapes, the TOFs will successfully and effectively enable carbon dioxide (CO2) emissions to be offset by the forest ecosystems (Chauhan et al., 2020). Since they constitute one of the major C pools that contribute to global carbon (C) cycling, tree-based land use systems, particularly those in non-forested environments like agricultural land, have a important impact on process. Productivity in plantation is higher (3.2 Mg ha/1 year) than productivity of natural forest (1.1 Mg ha/1 year), so it can benefit from better silvicultural techniques than a wild forest (Lal and Singh, 2000). Additionally, resource management through agroforestry may offer a natural way to synchronise the implementation of adaptation and mitigation measures with the satisfaction of forest-based needs (Komal et al., 2022). Agroforestry has been acknowledged as a GHG mitigation strategy under the Kyoto Protocol because it is an integrated approach (Birhane et al., 2020; Das et al., 2020; Sarkar et al., 2021). This recognition is based on carbon capture and utilisation efficiency leading to greater carbon sequestration (Das et al., 2020; Semere et al., 2022).
Agroforestry is the deliberate coexistence of woody vegetation with agricultural crops, grasses, or farm animals on the same plot of land to benefit the environment, the economy, and society (Rapidel et al., 2015; Armengot et al., 2020). Agroforestry systems have different structural and functional compositions depending upon the temperature, elevation, soil structure, and rainfall pattern (Rajput et al., 2017; Chisanga et al., 2018; Singh et al., 2019; Birhane et al., 2020; Salve and Bhardwaj, 2020; Nath et al., 2022; Sharma et al., 2022). Given the significance of this activity, India developed a national agroforestry plan in 2014 to promote the full spread of agroforestry practises. The Green India Mission has also noticed agroforestry interferences. The promise of agroforestry to mitigate climate and assist farmers in adapting to its effects is a key driving force behind India’s agroforestry programme. The Green India Mission has also noticed agroforestry interferences. The potential of agroforestry to slow climate change and help farmers adapt to its impacts is a major impetus behind India’s agroforestry plan. As evidenced by the multiple-purpose trees (such as fodder, fruit, fuelwood, and timber trees) purposefully retained on farm bunds (Semere, 2019; Chavan et al., 2020), agroforestry has been practised historically for as long as anyone can remember. It also plays a significant role in preserving sustainability in farming systems (Chandra and Singh, 2018). Agroforestry has been identified as having the greatest potential for carbon sequestration among all the land uses that were the subject of the Land-Use, Land-Use Change, and Forestry (LULUCF) report’s research (Nair and Garrity, 2012). Agroforestry is receiving increased attention in global initiatives like Reducing Emissions from Deforestation and Forest Degradation (REDD) because of its implication in improving and regulating climate variability (Jose and Bardhan, 2012).
Current study focused on the accurate estimation of carbon stock in the prominent agroforestry systems in the eco-sensitive Himalayan region in order to understand the carbon sequestration potential of agroforestry systems in the region. Keeping this in view, the present investigation was carried out with the objective of quantifying the biomass, carbon stock and carbon sequestration potential of different agroforestry systems in district Anantnag of Kashmir division. The study might be helpful in giving information to decision-framers and agriculturists for efficient and long-term carbon balancing and climate variability mitigation in the temperate region of the Kashmir Himalayan ecosystem.
2. Materials and methods
2.1. Study site
The study area is situated in the southern region of Kashmir covering Anantnag district of Jammu and Kashmir, India. The district is 33 miles (53 km) away from Srinagar (the capital city), and is situated between 33o 20′ and 34o15’ N latitude and 74o30’ and 75o35’ E longitude. There is a mild climate in Anantnag (Koppen climate classification). As per Koppen climate classification, district Anantnag is having a mild climate compared to other regions of Kashmir valley Geographical factors like the Karakoram range to its east and the Pir Panjal range to its south greatly influence its climate. A typical description of the climate is that it is cool in the spring and the autumn season, warm in the summer, and frigid in the winter. The region has a temperate climate, with substantial winter snowfall occurring in the higher elevations. The entire southern sector of the study area, which borders the Jammu province tehsils of Reasi, Banihal, and Kishtwar, as well as the eastern sector, which borders the Ladakh division tehsil of Kargil. Pulwama district borders its northern and western sides, and Kulgam district is located on its western side. Anantnag has the most streams (Nallas) in Kashmir valley including Sandran, Brengi, Arpath, and Lidder,. Lidder, which emerges from Sheshnag Lake and irrigates the largest territory in the district, is the most significant of these. The Anantnag district has a wide intermountain valley and is hilly and mountainous in the north-east and south-west. Productivity is vulnerable in higher ranges and fertile in central regions because soil is poor in steep locations and rich in plain areas. Hapludalf is the name given to the medium- to fine-textured soils that have grown on Karewa tops and upland locations. Clay loam soils, which are dark brown in colour, are typical in plains.
In district Anantnag land use land cover category, there is a total of 341 ha dense built-up, 337 ha mixed built-up, 2,176 ha agriculture, 480 ha plantation, 118 ha water bodies, 60 ha wastelands, 196 ha sparse forests and 95 ha dense forests forming a total of 3,803 ha land (Malik, 2012). Nowadays, agroforestry is considered as the primary route to success for farmers and rural residents in the region, thereby generating work and income, ensuring the security of food and nutrition, satisfying other fundamental human requirements and protecting farmers from the effects of climate change (Figure 1).
2.2. Experimental setup
The current research was performed in year 2018–2019 in district Anantnag of Kashmir division. For conducting this study regarding different land use patterns, field survey of all the agroforestry systems prevalent in selected representative areas was carried. Six tehsils, namely Anantnag, Shangus, Kokernag, Dooru, Sallar, and Larnu, were chosen out of a total of twelve. The blocks were chosen using a multistage stratified random selection procedure viz.; Anantnag, Shangus, Sagam, Shahabad, Pahalgam and Larnu. Then four villages within each block like Anantnag (Ruhoo, Dialgam, Wanihama and Donipawa), Shangus (Shangus, Nowgam, Kutheir and Andoora), Sagam (Hiller, Khalhar, Sagam and Bidder), Shahabad (Larkipora, Shankerpora, Nathipora and Bragam), Pahalgam (Yanner, Charigam, Movra and Wahdan) and Larnu (Leesu, Dandipora, Bidhard and Larnu). Ten farmers within each village were selected, thus making a total of 240 farmers to document the land use pattern of district Anantnag. A total of 240 households were selected for the field study from the sample villages with a 25% sampling intensity using a basic random sampling technique. Household heads/eldest members were the respondents interviewed during survey programme.
For different agroforestry systems at different sites, one 20 m × 20 m quadrant was laid for measuring tree biomass and three 1 m × 1 m quadrant were laid within 20 m × 20 m quadrant for measuring crop biomass thus making a total of 240 quadrats for trees and 720 quadrats for crops. During survey across the field, in horti-agriculture system, a total number of 555 trees/ha in combination C1(Apple + radish-kale), 555 trees/ha in combination C2(Apple + kale-chilli), 555 trees/ha in combination C3(Apple + kale-beans) and 80 trees/ha in combination C4(Walnut + oats-maize) were recorded. In agri-silviculture system (boundary plantation), a total of 138 trees /ha in combination C1(Poplar + salix + oats-paddy) and 160 trees /ha in combination C2(Poplar + mustard-paddy) were documented (Table 1).
Also, in agri-horti-silviculture system, a total number of 336 trees/ha (156 fruit trees and 180 forest trees), 380 trees/ha (180 fruit trees and 200 forest trees) and 444 trees/ha (204 fruit trees and 240 forest trees) were recorded in combination C1(Poplar + apple + kale-beans), C2(Poplar + apple + kale-chilli) and C3(Poplar + apple + turnip-kale) respectively. Similarly, in horti-silvipasture system, a total of 753 trees/ha (625 fruit trees and 128 forest trees) and 756 trees/ha (625 fruit trees and 131 forest trees) were recorded in combination C1(Apple + poplar + grasses) and C2(Apple + poplar + salix + grasses) respectively (Table 1). All the above-mentioned trees (forest and fruit trees) and crops were further evaluated for biomass, carbon stock and carbon sequestration potential.
3. Methodology adopted
3.1. Biomass production
The study calculated both above- ground and below-ground biomass in trees and crops in all agroforestry systems. The above-ground biomass of several plant components, including stems, branches, leaves, and roots, was estimated. Biomass of forest trees, fruit trees and agricultural crops were calculated separately as below:
3.1.1. Forest tree
Forstem biomass with a diameter tape and a Ravi’s multimeter, the diameter of trees at breast height (dbh) that fell in the 20 × 20 m quadrat were measured. In order to get the volume using the Pressler (1854) and Bitterlich (1984) formula, the form factor was computed using the Spiegel relascope. Specific gravity was calculated by using formula proposed by Smith (1954). Belowground biomass was measured by using a factor of 0.25 for forest trees (Schulze, 1983). Thus, biomass of stem wood = specific gravity × volume. For branch biomass on each sample tree, the aggregate number of branches were calculated regardless of size. Based on the basal diameter, the branches were categorised into three groups: small, medium, and large. Each group’s two sampled branches weight was recorded individually. The formula given by Chidumaya (1990) was used to calculate the branch biomass in trees. Leaf biomass by dividing the sample tree’s branches into three groups based on basal diameter, small, medium, and large, the leaf biomass was calculated. Fresh weight of two sampled branches from each group was recorded separately. Immediately after being severed from the branch, the leaf biomass of each representative branch of the sample trees in each diameter class was counted in 20× 20 m quadrat. The leaf samples from two sampled branches of each group were put in individual bags and oven-dried at 65°C for 72 h, or until the consistent weight is obtained (Chapman, 1964).
3.1.2. Fruit tree
For stem biomass it was calculated by means of the regression equation, prescribed by Brown et al. (1989) and the formula is given as: Y = exp. {−2.4090 + 0.9522 ln (D2HS)}, where, Y = Biomass/tree (kg), D = Tree collar diameter, H = Height of tree (cm), S = Density of wood (g cm-3). Canopy biomass Canopy biomass was estimated by multiplying crown volume by specific gravity. Crown volume was estimated by using formula given by Avery and Burkhart (2002) {Crown volume = (db2L)/12, where, db = Crown base diameter (m), L = Length of crown}.
For the purpose of calculating specific gravity, Smith’s (1954) maximum moisture method was applied. Fruit samples weighing 1 kg were sun dried followed by oven dried at 60°C until their weight remained constant. Belowground biomass was determined by using formula given by Singh (2010). Then total biomass was computed by summing up of the total above-ground biomass and belowground biomass of the fruit trees.
3.1.3. Crop biomass
Crop (rabi and kharif) and grass biomass were measured by means of (1 × 1) m quadrates. At the time of harvest, the total biomass was determined by uprooting, which was then evaluated to calculate green/fresh weight. The samples were put in individual bags and dried in an oven at 65°C for 72 h, or until the weight remained consistent (Chapman, 1964). Estimation of carbon. The calculation of carbon stock was determined by multiplying total biomass by a conversion factor that represents the average carbon content in biomass. The default value of 0.45 for the conversion of biomass to carbon was used (Woomer, 1999). Therefore, the total carbon stock (C) present in the calculated total biomass was equal to C = 0.45 × Biomass (total). The mitigation potential of the system was worked out by calculating equivalent carbon dioxide (eCO2) as per formula: CO2e = C × 3.67.
3.2. Data analysis
The data obtained were subjected to the statistical analysis as per procedure given by Gomez and Gomez (1984) using R-software and SAS statistical package. Also, one-way ANOVA was used for analyzing the data of different systems in the present study.
4. Results
Among four different systems under study, the horti-silvi-pasture system recorded maximum aboveground tree biomass (43.11 t/ha), belowground tree biomass (12.58 t/ha) and total tree biomass (55.73 t/ha) and was significantly greater than all other agroforestry systems. The agri-silvipasture (homegardens) however was found next to horti-silvipasture recording 30.80 t/ha, 8.16 t/ha and 32.76 t/ha as aboveground tree biomass, belowground tree biomass and total tree biomass respectively, while as significantly lowest aboveground tree biomass (15.44 t ha −1), belowground tree biomass (3.84 t ha − 1) and total tree biomass (19.3 t ha −1) were recorded in agri-silviculture system (boundary plantation). Also, maximum total crop biomass was found in agri-silviculture (boundary plantation) as 15.54 t ha−1, which was significantly higher than all other agroforestry systems. The horti-agriculture system was found next to agri-silviculture (boundary plantation) recording 12.52 t/ha as total crop biomass, while as lowest total crop biomass (10.82 t ha−1) was recorded in horti-silvipasture system. Total biomass production (tree+ crop component) followed a similar pattern as that seen in above and below ground tree biomass, with horti-silvi-pastoral system contributing maximum as 66.55 t ha−1 which was significantly higher than all other systems and was followed by agri-horti-silviculture as 50.18 t ha−1 and horti-agriculture as 36.22 t ha−1. Minimum value was recorded under agri-silviculture (boundary plantation) as 34.87 t ha−1 (Table 2).
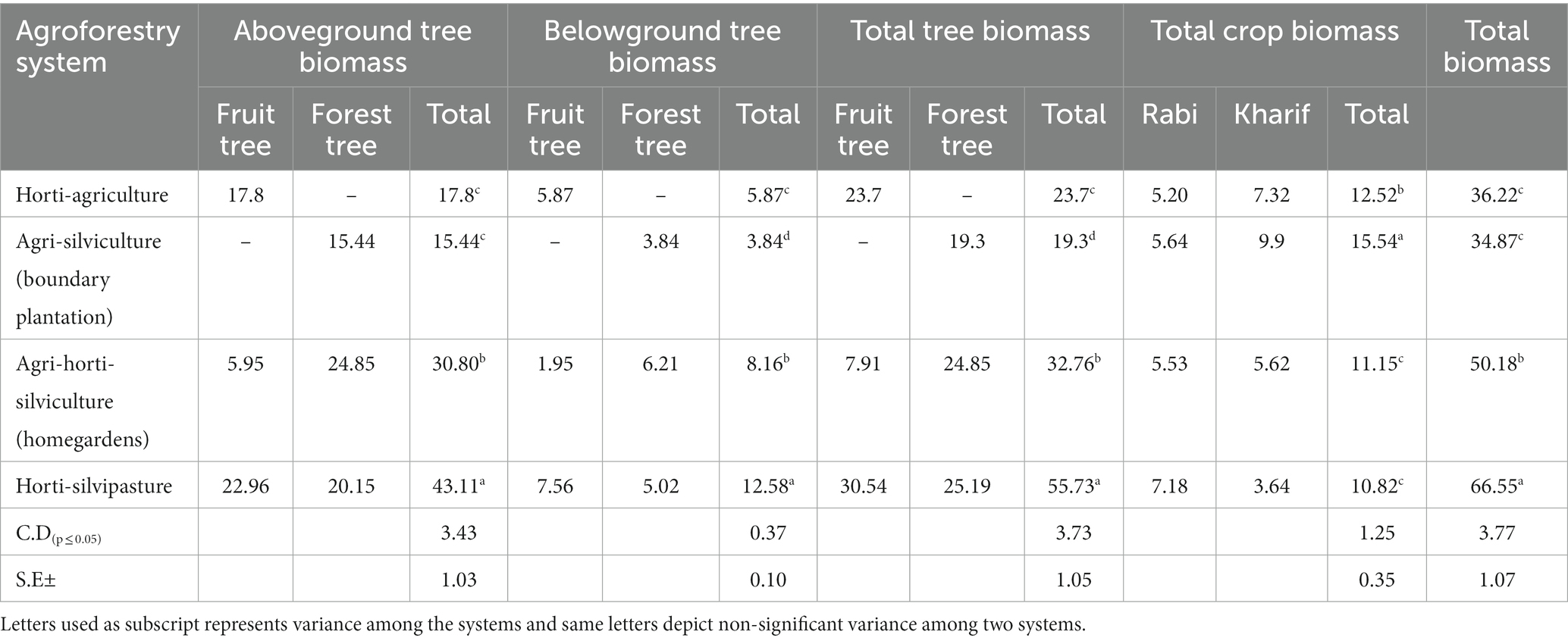
Table 2. Aboveground, belowground and total biomass (t ha−1) production in prominent agroforestry systems.
Since both carbon storage and carbon capture capability of all the systems are directly linked to the biomass production of the various plant components, so they both match their capacity for biomass production. Plant biomass can vary significantly in terms of carbon content in an intricate agroforestry system, and this variation affects production. Productivity is determined by a variety of elements, including age, kind of soil, crop type, growing habits, and management intensity. So, in our study, a similar trend was observed in values of carbon stock and carbon sequestration potential as that of above-mentioned total biomass estimations in agroforestry systems. Horti-silvipasture was having highest values for tree carbon stock (25.07 t ha−1) and tree carbon sequestration potential (92.04 t ha−1) and were significantly higher than all other systems. The agri-silvipasture (homegardens) however was found next to horti-silvipasture recording 38.82 t ha−1 and 64.33 t ha−1 as tree carbon stock and carbon sequestration potential respectively, while as significantly lowest tree carbon stock and sequestration potential as 8.68 t ha−1and 31.85 t ha−1, respectively, were recorded in agri-silviculture system (boundary plantation).Also, maximum total crop carbon stock and carbon sequestration potential was found in agri-silviculture (boundary plantation) as 7.98 t ha−1 and 25.65 t ha−1 respectively, which was significantly higher than all other agroforestry systems. The horti-agriculture system was found next to agri-silviculture (boundary plantation) recording 5.63 t ha−1and 20.62 t ha−1as total crop carbon stock and sequestration potential, while as lowest total crop carbon stock and sequestration potential (4.86 t ha−1 and 17.93 t ha−1 respectively) were recorded in horti-silvipasture system (Tables 3, 4).
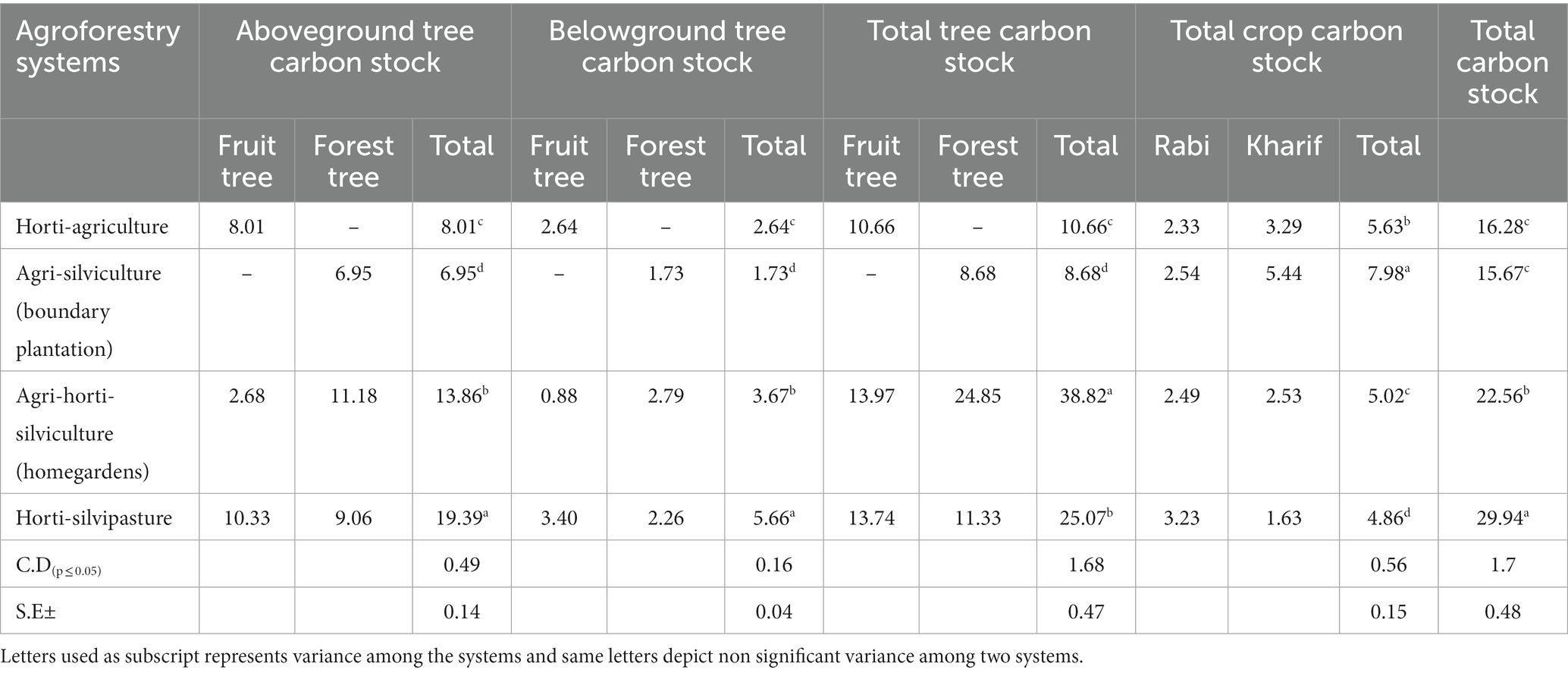
Table 3. Aboveground, belowground and total carbon stock (t ha−1) in prominent agroforestry systems.
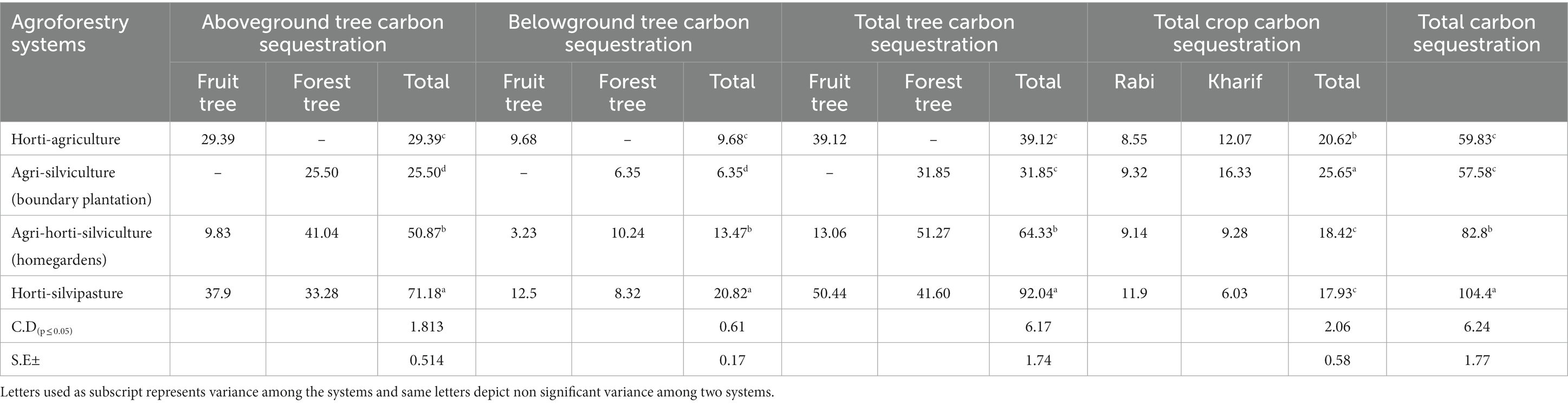
Table 4. Aboveground, belowground and total carbon sequestration (t ha−1) potential in prominent agroforestry systems.
The overall comparison of agroforestry systems practised by the farmers of Anantnag district clearly shows that the horti-silvipastoral system outperforms the other agroforestry systems and are most productive in terms of total biomass accumulation (66.55 t ha−1), carbon stock (29.94 t ha−1) and carbon dioxide sequestration potential of 104.4 t ha−1 (Tables 2–4). The involvement of forest trees in the horti-silvipastoral system was 37.83%, fruit trees as 45.86% and crop (rabi and kharif) contributed 16.29% in biomass, carbon stock and carbon sequestration potential. Agri-silviculture, on the other hand, was least productive in terms of total biomass (34.87 t ha−1), carbon stock (15.67 t ha−1) and CO2 sequestration potential (57.58 t ha−1). The contribution of forest trees in agri-silviculture system was 55.36% and crops contribute 44.61%.
5. Discussion
The horti-silvi-pasture system recorded maximum aboveground tree biomass (43.11 t/ha), belowground tree biomass (12.58 t/ha) and total tree biomass (55.73 t/ha) and was significantly greater than all other agroforestry systems. Also, lowest aboveground tree biomass (15.44 t ha −1), belowground tree biomass (3.84 t ha − 1) and total tree biomass (19.3 t ha −1) was recorded in agri-silviculture system (boundary plantation). In horti-silvi-pasture systems, the density of trees being high and greater average height and dbh values of individual trees will contribute to the higher values of total tree biomass and vice-versa (fewer trees per hectare in agri-silviculture). Also, aboveground biomass is a valuable resource in most land use regimes, so more biomass is allocated to aboveground components as it includes biomass from tree’s trunk, stump, branch, twig, and leaves, as opposed to the tree’s underground biomass, which only includes biomass from its fine and coarse roots. The number of woody plants in a specific agro-forestry system, as well as management strategies, play a vital impact in affecting biomass in a particular agro forestry system (Dar et al., 2019; Siarudin et al., 2021; Kumar et al., 2022). Agroforestry systems are complex and heterogeneous and, the more the heterogeneity, the more efficiently the carbon is sequestered compared to simpler systems (Rai et al., 2021; Tamang et al., 2021). However, the efficiency of Agroforestry systems as carbon sinks is governed by their size, natural site qualities, choice of species and management practises followed, i.e., carbon sequestered by an AFS depends on its structure and composition modified by environmental and socio-economic factors. Moreover, inter- and intra-specific variation in tree diameter, stand age, stand structure and diversity of the system also affect variation in biomass and its carbon (Bajigo et al., 2015; Panwar et al., 2022). Similarly, study conducted by Devagiri et al. (2020) in western Ghats region of peninsular India found that coffee agroforests resembled natural forest and mixed species plantations in terms of biomass production due to presence of tree diversity in this system. Also, study conducted by Panwar et al. (2022) reported that AGB was highest in block plantations (mean = 109.8 Mg ha − 1), followed by plantation crop systems (mean = 88.49 Mg ha − 1), with the lowest AGB observed in boundary plantations (mean = 17.14 Mg ha − 1). BGB was highest in homegardens (mean = 34.68 Mg ha − 1), followed by block plantations (mean = 26.19 Mg ha − 1), and lowest in boundary plantations (mean = 2.67 Mg ha − 1).
Current study also witnessed that agri-silviculture (boundary plantation) contributed maximum crop biomass as 15.54 t ha−1, which was significantly higher than all other agroforestry systems and minimum was recorded in horti-silvipasture system (10.82 t ha−1; Table 2). Greater crop biomass observed in case of agri-silviculture system might be due to the consistent and enough solar incidence in open agricultural fields as a result of larger open photosynthetic area, and the absence of competition between woody perennials and agricultural crops for moisture, light, nutrients, and space, among other factors. Minimum biomass of grass species in case of horti-silvipasture might be because trees and grasses compete for the same resources, such as sunlight, water, and nutrients. Our results are corroborated with Nandal and Singh (2001), Handa et al. (2004), Yadav (2010), and Chauhan et al. (2008) who found that increasing the distance between trees and crops, improved crop performance and production. Chavan et al. (2022) also, reported that the performance of sorghum and wheat crops is strongly influenced by the distance from tree lines and the age of the poplar trees and might be due to significant interaction between distance and aspect that was attributed to small differences in the intensity and duration of shadows cast by the boundary stands. Such study also observed a significant reduction of fodder biomass of sorghum and wheat yield was observed up to 9 m distant from the tree line. The reduction was 10–60% for the kharif season and 7.2–29.5% for rabi crops from the 2nd year to 8 years after planting, respectively. Similarly, Honfy et al., 2023 investigated the tree planting pattern of black locust (Robinia pseudoacacia L.) when intercropped with triticale (x Triticosecale W.) and observed that more the trees planted on a hectare, the higher the volume of the stand, and the less yield of triticale. Studies carried out by various researchers observed that a significant yield reduction of more than 70% was observed near the tree base (0–3 m) and about a 10–35% reduction up to 9 m away from the tree (Yang et al., 2015).
Total biomass (tree + crop component) followed a similar pattern as in above and below ground tree biomass, with horti-silvi-pastoral system contributing maximum as 66.55 t ha−1 which was significantly higher than all other agroforestry systems and was followed by agri-horti-silviculture as 50.18 t ha−1 and horti-agriculture as 36.22 t ha−1. Also, minimum total biomass was recorded under agri-silviculture as 34.87 t ha−1 (Table 2). This could be attributed to higher tree density and difference in management regimes; for example, in horti-silvipasture system, farmers retain native trees in large numbers to provide them fruit on a large scale and forest trees serve as live fence, thus overcomes fodder scarcity.
Similary, carbon stock and carbon sequestration values of different agroforestry systems follows same trend as in above mentioned biomass production being significantly more in horti-silvipasture followed by agri-horti-silviculture (homegardens) and horti-agriculture and minimum values in agri-silviculture (boundary plantation). The inclusion of forest trees in the agroforestry system, which results in continuous carbon locking in woody plants, and the natural accumulation of fallen leaf debris to soil, which assists in the build-up of carbon in soil beds and, consecutively, aids in considerable production of structure, may be the primary causes of the maximum carbon reserve in horti-silvipasture and agri-horti-silviculture (homegardens). However, most of the biomass in horti-agriculture and agri-silviculture (boundary planation) is taken yearly by harvesting, trimming, and felling. The ability to capture carbon dioxide rely on variety of characteristics, involving species type, genetic make-up, maturity, structure, functional components, and number. Tree plantations are a better option for reducing atmospheric carbon since they store more carbon, but due to population pressure and the rising demands for agricultural land, these plantations fail to extend. As a result, the agroforestry system appears to be a better alternative for preserving biodiversity while still providing economic advantages to society. Nair et al. (2009) reported that the capacity for carbon capture in agroforestry land use systems is based on the woody element. Farmlands has greater capacity to sequester carbon with highest woody component than other land use systems (lesser woody components; Sureshbhai et al., 2017; Dar et al., 2019). The ability of different agroforestry systems to store carbon varies greatly, and the amount of carbon stored is mostly influenced by the kind of agroforestry system utilised, its arrangement and role, as well as the surrounding environment and socioeconomic factors. Tree species and system management are two further elements that may influence carbon storage in agroforestry system (Nair et al., 2010).
6. Conclusion
Agroforestry systems has a greater capacity to influence the source or sink role of the trees. It could play an important role in mitigating climate change as it sequesters more atmospheric carbon in plant parts and soil than conventional farming. The present study recognised the capability of various agroforestry systems for biomass estimation and carbon sequestration potential for mitigating climate variation in north-western Himalayas. Agroforestry systems differed in terms of biomass output and carbon sequestration potential. As seen from the data, the horti-silvipasture system recorded maximum values for biomass and carbon sequestration potential, agri-silviculture system (boundary plantation) recorded minimum values. So, the study concluded that horti-silvipasture system outperforms the other agroforestry systems and are most productive in terms of carbon sequestration potential. Thus, adopting these agroforestry systems not only contributes to environmental stability by reducing carbon dioxide emissions, but it also increases farming income, so improving farmer livelihood. As a result, if the carbon trapped by these systems is traded to rich countries, there is a compelling financial incentive for farmers to use them thus, by development of suitable agroforestry systems in diverse agroclimatic zones, not only the area of greenery in the country be increased, but the proportion of greenhouse gases in the environment may also be significantly reduced. Therefore, agroforestry will be a workable method for reducing climate crises if it is encouraged for greater adoption by cultivators in the nation.
Data availability statement
The original contributions presented in the study are included in the article/supplementary material, further inquiries can be directed to the corresponding author.
Author contributions
IS and JAM framed the concept. IS, BM, and AA did the field work. IS, JAM, NAP, GMB, AHM, and THM did the analysis and drafted the manuscript. IS and NAP finalised the manuscript. All authors contributed to the article and approved the submitted version.
Conflict of interest
The authors declare that the research was conducted in the absence of any commercial or financial relationships that could be construed as a potential conflict of interest.
Publisher’s note
All claims expressed in this article are solely those of the authors and do not necessarily represent those of their affiliated organizations, or those of the publisher, the editors and the reviewers. Any product that may be evaluated in this article, or claim that may be made by its manufacturer, is not guaranteed or endorsed by the publisher.
References
Anderson, T. R., Hawkins, E., and Jones, P. D. (2016). CO2, the greenhouse effect and global warming: from the pioneering work of Arrhenius and Callendar to today’s earth system models. Endeavour 40, 178–187. doi: 10.1016/j.endeavour.2016.07.002
Armengot, L., Ferrari, L., Milz, J., Velasquez, F., Hohmann, P., and Schneider, M. (2020). Cacao agroforestry systems do not increase pest and disease incidence compared with monocultures under good cultural management practices. Crop Prot. 130:105047. doi: 10.1016/j.cropro.2019.105047
Avery, T.E., and Burkhart, H.E. (2002). Forest Measurements, 5th. MCGraw-Hill Series in Forest Resources, New York, USA.
Aynekulu, E., Suber, M., Noordwijk, M., Arango, J., Roshetko, J. M., and Rosenstock, T. S. (2020). Carbon storage potential of silvopastoral systems of Colombia. Land 9, 1–12. doi: 10.3390/land9090309
Bajigo, A., Tadesse, M., Moges, Y., and Anjulo, A. (2015). Monitoring of seasonal variation in physicochemical water parameters in Nalasopara region. J. Ecosyst. Ecography 5:157.
Besar, N. A., Suaedi, H., Phua, M. H., James, D., Mokhtar, M. B., and Ahmed, M. F. (2020). Carbon stock and carbon sequestration potential of an agroforestry system in Sabah. Malaysia. Forests 11, 1–16. doi: 10.3390/f11020210
Birhane, E., Ahmed, S., Hailemariam, M., Negash, M., Rannestad, M. M., and Norgrove, L. (2020). Carbon stock and woody species diversity in homegarden agroforestry along an elevation gradient in southern Ethiopia. Agrofor. Syst. 94, 1099–1110. doi: 10.1007/s10457-019-00475-4
Bitterlich, W. (1984). The Relaskop Idea Slough: Commonwealth Agricultural Bureaux. Farnham Royal, England pp.242.
Brown, S., Gillespie, A. J. R., and Lugo, A. E. (1989). Biomass estimation methods for tropical forests with application to forest inventory data. For. Sci. 35, 881–902.
Chandra, K. K., and Singh, A. K. (2018). Carbon stock appraisal of naturally growing trees on farmlands in plain zone districts of Chhattisgarh. India. Trop. Ecol. 59, 679–689.
Chapman, H. D. (1964). Suggested foliar sampling and handling techniques for determining the nutrient status of some field, horticultural and plantation crops. Indian J Hortic. 21, 94–105.
Chauhan, V. K., Joshi, A. K., and Dholta, V. K. (2008). Performance of maize (Zea maize L.) varieties under different spacings of poplar (Populus deltoids M.) in lower western Himalayas. Indian Forester 134, 1603–1611.
Chauhan, M., Kumar, M., and Kumar, A. (2020). Impact of carbon stocks of Anogeissus latifolia on climate change and socio-economic development: a case study of Garhwal Himalaya, India. Water Air Soil Pollut. 231:436. doi: 10.1007/s11270-020-04803-8
Chavan, S. B., Dhillon, R. S., Sirohi, C., Keerthika, A., Kumari, S., Bharadwaj, K. K., et al. (2022). Enhancing farm income through boundary plantation of poplar (Populus deltoides): an economic analysis. Sustainability 14:8663. doi: 10.3390/su14148663
Chavan, S. B., Newaj, R., Rizvi, R. H., Ajit, P. R., Alam, B., Handa, A. K., et al. (2020). Reduction of global warming potential Vis-a-Vis greenhouse gases through traditional agroforestry systems in Rajasthan, India. Environ. Dev. Sustain. 23, 4573–4593. doi: 10.1007/s10668-020-00788-w
Chidumaya, E. N. (1990). Aboveground woody biomass structure and productivity in Zambezian woodland. For. Ecol. Manag. 36, 33–46. doi: 10.1016/0378-1127(90)90062-G
Chisanga, K., Bhardwaj, D. R., Pala, N. A., and Thakur, C. L. (2018). Biomass production and carbon stock inventory of high-altitude dry temperate land use systems in North Western Himalayas. Ecol. Process. 7, 1–13. doi: 10.1186/s13717-018-0134-8
Dar, M. D., Qaisar, K. N., Masoodi, T. H., Mughal, A. H., and Khan, P. A. (2019). Biomass production and carbon stock potential under home gardens of Kashmir Himalaya. J. Plant Dev. Sci. 11, 265–271.
Das, M., Nath, P. C., Reang, D., Nath, A. J., and Das, A. K. (2020). Tree diversity and the improved estimate of the carbon storage for traditional agroforestry systems in north-East India. Appl Ecol Environ Sci 8, 154–159.
Devagiri, G. M., Khaple, A. K., Anithraj, H. B., Kushalappa, C. G., Amaresh Kumar, K., and Shashi Bhushan, M. (2020). Assessment of tree diversity and above-ground biomass in coffee agroforest dominated tropical landscape of India’s Central Western Ghats. J For Res 31, 1005–1015. doi: 10.1007/s11676-019-00885-1
Dhyani, S., Murthy, I. K., Kadaverugu, R., Dasgupta, R., Kumar, M., and Gadpayle, K. A. (2021). Agroforestry to achieve global climate adaptation and mitigation targets: are south Asian countries sufficiently prepared? Forests 12:303. doi: 10.3390/f12030303
Gomez, K.A., and Gomez, A.A. (1984). Statistical Procedure for Agricultural Research. 2nd, Willey, Hoboken, 28–192.
Handa, D. P., Jaggis, S., Gill, A. S., and Singh, N. P. (2004). Impact of fruit trees of the yield of wheat crop. Indian J. For. 27, 141–144.
Honfy, V., Pödör, Z., Keser, U. Z., Rásó, J., Ábri, T., and Borovics, A. (2023). The effect of tree spacing on yields of alley cropping systems—a case study from Hungary Veronika. Plan. Theory 12:595. doi: 10.3390/plants12030595
Jose, S., and Bardhan, S. (2012). Agroforestry for biomass production and carbon sequestration: an overview. Agrofor. Syst. 86, 105–111. doi: 10.1007/s10457-012-9573-x
Kaul, M., Mohren, G. M. J., and Dadhwal, V. K. (2010). Carbon storage and sequestration potential of selected tree species in India. Mitig. Adapt. Strateg. Glob. Chang. 15, 489–510. doi: 10.1007/s11027-010-9230-5
Komal, N., Zaman, Q., Yasin, G., Nazir, S., Ashraf, K., Waqas, M., et al. (2022). Carbon storage potential of agroforestry systems near brick kilns in irrigated agro-ecosystem. Agriculture 12, 1–13. doi: 10.3390/agriculture12020295
Kumar, A., Mishra, S., Pandey, R., Yu, Z., Kumar, M., Khoo, K. S., et al. (2022). Microplastics in terrestrial ecosystems: un-ignorable impacts on soil characterises, nutrient storage and its cycling. TrAC Trends Anal. Chem. 158:116869. doi: 10.1016/j.trac.2022.116869
Lal, M., and Singh, R. (2000). Carbon sequestration potential of Indian forests. Environ Monit Assess 60, 315–327. doi: 10.1023/A:1006139418804
Malhi, G. S., Kaur, M., and Kaushik, P. (2021). Impact of climate change on agriculture and its mitigation strategies: a review. Sustainability 13:1318. doi: 10.3390/su13031318
Malik, M. I. (2012). Analysis of population growth and land use change in Anantnag town of South Kashmir using remote sensing and geographical information system. J. Exp. Sci. 3, 23–27.
Mangalassery, S., Dayal, D., Meena, S. L., and Ram, B. (2014). Carbon sequestration in agroforestry and pasture systems in arid northwestern India. Curr. Sci. 107, 1290–1293.
Mey, C. B. J., and Gore, M. L. (2021). Biodiversity conservation and carbon sequestration in agroforestry systems of the Mbalmayo Forest reserve. J For Environ Sci 37, 91–103.
Nair, P. K. R., and Garrity, D. (2012). “Agroforestry research and development: the way forward” in Agroforestry – The Future of Global Land Use. eds. N. PKR and D. Garrity (Dordrecht: Springer), 515–531.
Nair, P. K. R., Kumar, B. M., and Nair, V. D. (2009). Agroforestry as a strategy for carbon sequestration. J. Plant Nutr. Soil Sci. 172, 10–23. doi: 10.1002/jpln.200800030
Nair, P. K. R., Nair, V. D., Mohan Kumar, B., and Showalter, J. M. (2010). “Chapter five - carbon sequestration in agroforestry systems” in Advances in Agronomy. ed. D. L. Sparks , vol. 108 (Academic Press), 237–307.
Nandal, D. P. S., and Singh, R. R. (2001). Productivity of different cropping sequences in Dalbergia sissoo Roxb. Based agroforestry silviculture system. Indian J. Agroforestry 24, 433–436.
Nath, P. C., Thangjam, U., Kalita, S. S., Sahoo, U. K., Giri, K., and Nath, A. J. (2022). Tree diversity and carbon important species vary with traditional agroforestry managers in the Indian eastern Himalayan region. Environ. Sci. Pollut. Res. 29, 64732–64744. doi: 10.1007/s11356-022-20329-4
Panwar, P., Mahalingappa, D. G., Kaushal, R., Bhardwaj, D. R., Chakravarty, S., Shukla, G., et al. (2022). Biomass production and carbon sequestration potential of different agroforestry systems in India: a critical review. Forests 13, 1–15. doi: 10.3390/f13081274
Rai, P., Vineeta,, Shukla, G., Manohar, K. A., Bhat, J. A., Kumar, A., et al. (2021). Carbon storage of single tree and mixed tree dominant species stands in a reserve Forest—case study of the eastern sub-Himalayan region of India. Land 10:435. doi: 10.3390/land10040435
Rajput, B. S., Bhardwaj, D. R., and Pala, N. A. (2017). Factors influencing biomass and carbon storage potential of different landuse systems along an altitudinal gradient in temperate northwestern Himalayas. Agrofor. Syst. 91, 479–486. doi: 10.1007/s10457-016-9948-5
Rapidel, B., Ripoche, A., Allinne, C., Metay, A., Deheuvels, O., Lamanda, N., et al. (2015). Analysis of ecosystem services trade-offs to design agroecosystems with perennial crops. Agron. Sustain. Dev. 35, 1373–1390. doi: 10.1007/s13593-015-0317-y
Salve, A., and Bhardwaj, D. R. (2020). Soil carbon stock and nutrient study in different agroforestry systems at Kinnaur district, Himachal Pradesh. Plant Arch. 20, 4251–4260.
Sarkar, P. K., Sarkar, P., and Kumar, A. (2021). Carbon storage potential of a waterlogged agroforestry system of Tripura. India. Water Air Soil Pollut 232, 232–151. doi: 10.1007/s11270-021-05098-z
Semere, M. (2019). Biomass and soil carbon stocks assessment of agroforestry systems and adjacent cultivated land, in Chehawereda, Gurage zone, Ethiopia. Int. J. Res. Agric. For. 6, 17–24.
Semere, M., Cherinet, A., and Gebreyesus, M. (2022). Climate resilient traditional agroforestry systems in Silite district, southern Ethiopia. J. For. Sci. 68, 136–144. doi: 10.17221/151/2021-JFS
Sharma, P., Bhardwaj, D. R., and Singh, M. K. (2022). Geospatial technology in agroforestry: status, prospects, and constraints. Environ. Sci. Pollut. Res. doi: 10.1007/s11356-022-20305-y
Siarudin, M., Rahman, S. A., Artati, Y., Indrajaya, Y., Narulita, S., Ardha, M. A., et al. (2021). Carbon sequestration potential of agroforestry systems in degraded landscapes in West Java, Indonesia. Forests 12:714. doi: 10.3390/f12060714
Singh, B. (2010). Bioeconomic Appraisal and Carbon Sequestration Potential of Different Land Use Systems in Temperate North-Western Himalayas. Ph.D Thesis Dr. Y S Parmar University of Horticulture and Forestry, Nauni, Solan.
Singh, R., Bhardwaj, D. R., Pala, N. A., and Rajput, B. S. (2019). Biomass production and carbon stock potential of natural vegetation, agroforestry and cultivated land use systems along altitudinal gradient in north western Himalayas. Range Manage. Agroforestry 40, 94–103.
Smith, D. M. (1954). Maximum Moisture Content for Determining Specific Gravity of Small Wood Samples. Forest Product Laboratory. USDA Forest Service Report pp. 2014.
Sureshbhai, P. J., Thakur, N. S., Jha, S. K., and Kumar, V. (2017). Productivity and carbon sequestration under prevalent agroforestry systems in Navsari District, Gujarat, India. Int. J. Curr. Microbiol. App. Sci. 6, 3405–3422. doi: 10.20546/ijcmas.2017.609.419
Tamang, M., Chettri, R., Vineeta,, Shukla, G., Bhat, J. A., Kumar, A., et al. (2021). Stand structure, biomass and carbon storage in Gmelina arborea plantation at agricultural landscape in foothills of eastern Himalayas. Land 10:387. doi: 10.3390/land10040387
WMO , (2021). State of the Global Climate. 2021. WMO Provisional Report. World Meteorological Organization, Geneva, Switzerland, p. 47.
Woomer, P. L. (1999). Impact of cultivation of carbon fluxes in woody savannahs of southern Africa. Water Air Soil Pollut. 70, 403–412.
Yadav, A. K. (2010). Carbon sequestration: underexploited environmental benefits of Tarai agroforestry systems. Indian J. Soil Conserv 38, 125–131.
Yang, Z. C., Zhao, N., Huang, F., and Lv, Y. Z. (2015). Long-term effects of different organic and inorganic fertilizer treatments on soil organic carbon sequestration and crop yields on the North China plain. Soil Tillage Res. 146, 47–52. doi: 10.1016/j.still.2014.06.011
Keywords: Himalaya, climate, landuse, energy, greenhouse
Citation: Saleem I, Mugloo JA, Pala NA, Bhat GM, Masoodi TH, Mughal AH, Baba AA and Mehraj B (2023) Biomass production, carbon stock and sequestration potential of prominent agroforestry systems in north-western Himalaya, India. Front. For. Glob. Change. 6:1192382. doi: 10.3389/ffgc.2023.1192382
Edited by:
Donald Mlambo, National University of Science and Technology, ZimbabweReviewed by:
Gopal Shukla, Uttar Banga Krishi Viswavidyalaya, IndiaBhupendra Singh, VCSG Uttarakhand University, India
Copyright © 2023 Saleem, Mugloo, Pala, Bhat, Masoodi, Mughal, Baba and Mehraj. This is an open-access article distributed under the terms of the Creative Commons Attribution License (CC BY). The use, distribution or reproduction in other forums is permitted, provided the original author(s) and the copyright owner(s) are credited and that the original publication in this journal is cited, in accordance with accepted academic practice. No use, distribution or reproduction is permitted which does not comply with these terms.
*Correspondence: Nazir A. Pala, bmF6aXJwYXVsQGdtYWlsLmNvbQ==