- 1Department of Biotechnology, School of Biosciences and Biotechnology, Baba Ghulam Shah Badshah University, Rajouri, Jammu and Kashmir, India
- 2Department of Botany, University of Delhi, New Delhi, India
- 3Centre for Biodiversity and Taxonomy, Department of Botany, University of Kashmir, Srinagar, Jammu and Kashmir, India
- 4Pharmacology Division, Council of Scientific and Industrial Research-National Botanical Research Institute, Lucknow, India
The best natural laboratories for studying the ecological responses of plants are high-altitude areas like the Himalaya, where many gradients coexist on a relatively small spatial scale. Here, we investigate the effect of soil physico-chemical and eco-physiological properties on the broadleaf evergreen woody shrub Rhododendron anthopogon D.Don along an altitudinal gradient in the Kashmir Himalaya. We collected leaf and soil samples for eco-physiological and soil physico-chemical analysis, respectively, at five different sites along an altitudinal gradient (3,220–3,908 m). Our results demonstrate a significant difference between soil physico-chemical and eco-physiological parameters along the altitudinal gradient. A significant correlation was observed between different studied parameters and altitude. Principal component analysis of studied soil physico-chemical and eco-physiological parameters revealed that all the sites were grouped into four clusters according to altitude, suggesting a more pronounced effect of altitude. Our findings showed that soil infiltration capacity increased with altitude, which would be helpful for the growth and development of R. anthopogon. We also observed significant variations in eco-physiological parameters, demonstrating their critical involvement in adapting R. anthopogon to the harsh environment of the alpine regions of Kashmir Himalaya. Therefore, the present study adds to our understanding that R. anthopogon has sufficient soil physico-chemical and eco-physiological plasticity, which should be favorable for its survival in future climates, offering an adaptive advantage and expanding its range shortly.
1. Introduction
Mountainous ecosystems support unique biodiversity with distinct vegetation zones along an altitudinal gradient. They exhibit considerable variation in microclimate even with a slight elevation difference, which affects the distribution and structure of plant communities (Maclean et al., 2017; Antonelli et al., 2018; Fatima et al., 2022). Distributional ranges for plant species are anticipated to move to higher elevations with rise in temperature (Alberto et al., 2013; Freeman et al., 2018; Wani and Pant, 2021). Substantial differences in environments can occur over short distances; hence, altitude gradients in alpines are studied to understand the consequences of climate change on plant distribution and soil properties (Adamczyk et al., 2019).
Variation in elevation gradients significantly impacts biotic and abiotic components, which are closely related to ongoing human progressive processes (Gupta et al., 2019). The change in vegetation along altitude reveals that temperature, wind, UV radiation, nutrient availability and precipitation are the main drivers (Piao et al., 2011; Wani et al., 2023). Due to different climatic conditions, soil physico-chemical properties and vegetation also vary significantly, which are related to lower levels of nitrification, pH, and organic carbon (Decker and Boerner, 2003; Kewlani et al., 2021). A major factor that decreases with increasing elevation is temperature; altitudinal gradients act as thermoclines to trigger long-term environmental conditions (Mayor et al., 2017). Plants with more phenotypic flexibility have been observed at higher elevations more frequently (Stöcklin et al., 2009; Pluess et al., 2016), allowing them to adapt to the changing environment. In several alpine and subalpine species, phenotypic plasticity compensates for the short growing season by permitting fast reproduction (Wani et al., 2022). In reaction to climate fluctuations, plants adjust critical physiological, reproductive, and physical features (Körner, 2007; Wani et al., 2022). The flexibility of plants allows them to inhabit various habitats across altitudinal gradients. Therefore, understanding the restrictions imposed by altitudinal gradients on plant distribution can be aided by studying these adaptive features and relating them to environmental factors (Fatima et al., 2022).
Altitudinal changes in soil characteristics significantly impact the composition of plant communities. Soil qualities can be affected by changes in vegetation along an elevational gradient, and the reverse is also true (Ahmad et al., 2018; Hou et al., 2019). Plant communities in alpine habitats can be better understood by examining the interaction between soil conditions and vegetation along an altitudinal gradient (Anic et al., 2010). Alpine mountains have a significant topographic role in soil physicochemical characteristics, such as slope aspects (Bardelli et al., 2017). Differences in air temperature with elevation are assumed to be the common cause driving changes in nutritional status across diverse locales (Sundqvist et al., 2013). Other variables, including soil temperature, slope exposure, vegetation, and altitude, can also impact soil qualities (Måren et al., 2015; Bardelli et al., 2017). Many studies have established the differential influence of variations in climate along an elevational gradient on soil attributes (Adamczyk et al., 2019; Hou et al., 2019; Hamid et al., 2020a; Zhang et al., 2021; Trujillo-González et al., 2022). Total organic carbon and nitrogen have been found to increase with altitude and were found to be positively correlated with annual mean temperature and precipitation (Zhang et al., 2021). Accordingly, soil temperature, pH, electrical conductivity and C:N ratio were found to show increased trend toward altitudinal gradient (Hamid et al., 2020a). A temperature reduction and precipitation increase is generally noticed with the rise in altitude (Körner, 2007; Hassan and Nile, 2021). Simon et al. (2018) reported linear correlation between soil organic carbon stock and pH with altitude. Climate change impacts the composition and biomass of plants, which is reflected in differences in soil characteristics and taxa (Zhu et al., 2019; Hamid et al., 2020a).
The eco-physiological characteristics of a plant species determine how it reacts to environmental changes (Rathore et al., 2018; Li H. et al., 2022; Visakorpi et al., 2022). The ability to forecast species mortality, distributions of species, and ecosystem-level processes can be improved with the knowledge of a species physiology (Fyllas et al., 2014; Anderegg et al., 2016; Visakorpi et al., 2022). However, due to their time-consuming testing requirements and frequent absences from trait databases, plant eco-physiological traits are rarely used to predict changes in the composition of plant communities. Instead, most research forecasting how plant communities will respond to climate change relies on morphological characteristics, such as height and leaf mass per area, which are thought to characterize species physiology implicitly and nutrient uptake (Shipley et al., 2016; Visakorpi et al., 2022). The primary goal of plant ecophysiology is to explain or comprehend ecological patterns through studies on the physiological mechanisms behind these ecological patterns. For instance, understanding the climatic effects of elevation on physiological processes is necessary to learn why plants in alpine ecosystems have distinct morphological and anatomical features from equivalent species in lowland habitats. Many authors have reported the effects of rising elevation on the eco-physiology of plants (Takahashi and Matsuki, 2017; Wang et al., 2017; Zhou et al., 2017; Rathore et al., 2018). In a study conducted by Rathore et al. (2018), a significant variation in leaf size, relative water content (RWC), chlorophyll and proline content (PR) were observed along an altitudinal gradient. Molina-Montenegro et al. (2012) studied the adaptability of Taraxacum officinale’s invasive photoprotective approaches and performance along altitudinal gradient. They observed greater plasticity in individuals from higher altitudes compared to those from lower altitudes. Similar to this, individuals exposed to high light levels produced more photoprotective pigments, biomass, and flowers (Molina-Montenegro et al., 2012).
Due to the restructuring of populations or species as a result of climatic change along the altitudinal gradient, plants have evolved numerous resistance traits (Lenoir et al., 2008; Bellard et al., 2012). According to Rapp et al. (2012), plant species with comparatively more genotypic or phenotypic variety may be better able to tolerate these changes. Studying these characteristics and connecting them to environmental gradients might provide insightful data on the ability of plants to adapt to changing conditions or explain how they are distributed within a specific geographic range (Ahrens et al., 2020). At higher altitudes, lower resource availability and harsh environmental conditions encourage stress-tolerant species that establish xeric characteristics such as diminished leaf area (LA) and heavily pubescent leaf surface, more prostrate habit, water conservation-related increases in lignin deposition or possibly succulence (Körner, 2012; Fatima et al., 2022).
One of the best natural laboratories for studying how plants respond ecologically is high-altitude areas like the Himalaya, where numerous gradients are present on a very small spatial scale (Enquist et al., 2017; Rathore et al., 2018). The primary elements affecting plant functioning along these gradients include temperature, pH, UV radiation, atmospheric humidity, and wind speed (Rathore et al., 2018). Several studies have been conducted on the effect of eco-physiological and soil parameters on an altitudinal gradient (Rathore et al., 2018; Qi et al., 2019; Hamid et al., 2020a; Han et al., 2020; Fatima et al., 2022). However, most of these studies focused on herbaceous plants. In the present study, we focused on Rhododendron anthopogon D.Don, a broadleaf evergreen woody plant that belongs to the family Ericaceae. It is endemic to Himalaya to S. Tibet, and can be found at elevations ranging from treeline to 4,800 m.a.s.l. The plant is in high demand as herbal medicines. Leaf decoction is used to treat cough, cold, asthma, chronic bronchitis, and mucus in the nose or throat (Kumar and Srivastava, 2002; Popescu and Kopp, 2013). In post-delivery problems, dried and powdered flowers mixed with oil are used as a body massage (Bhattacharjee, 1998). Rhododendron species are great examples of investigating the functional significance of adaptive characteristics having a long history of research on trait variation among species and its importance to physiology and ecology (Nilsen, 1992). Drought tolerance, cold adaptation, nutrient use efficiency, and habitat selection of Rhododendron species have been shown to represent a condition that implies vascular system adaptive changes (Taneda and Noshiro, 2019). Plants in high-light environments have a shorter leaf survival span than plants growing in low-light conditions.
In light of this, the current study was conducted in the Kashmir Himalaya. Global warming has considerably impacted this Himalayan region during the last few decades (Murtaza and Romshoo, 2017). The climate of the area has experienced significant change, particularly in the irregular behavior of precipitation patterns and seasonal temperature (Hamid et al., 2020b; Dad et al., 2021). In this study, we hypothesized that (a) soil physico-chemical and eco-physiological parameters change along an altitudinal gradient, (b) there exist a strong correlation between soil physico-chemical, eco-physiological properties and altitude, (c) the overall variation of soil physico-chemical and eco-physiological properties affect R. anthopogon along an altitudinal gradient.
2. Materials and methods
2.1. Study area and sampling sites
The present study was conducted at Apharwat mountain in the Gulmarg region of Jammu and Kashmir, located in India’s North-Western Himalaya. The study area is located in the Kashmir Himalaya between 34° 05′ N latitude and 74° 38′ E longitude. The altitude varies between 2,800 m (at Gulmarg) and 4,200 m (at Apharwat) above sea level. With 1,049 mm of annual precipitation on average, this area has a continental temperate climate. The hottest month of the year is July, with an average temperature of 20°C; the coldest month is January, with a decline in temperature up to −6°C (Hamid et al., 2020b). The sampling locations were built along an altitudinal gradient at five different sites: Site-1 is at 3,220 m, followed by Site-2 (3,351), Site-3 (3,500), Site-4 (3,716), and Site-5 (3,908 m) (Figure 1).
2.2. Soil and plant sampling
The soil samples were collected from randomly located three populations at each site. From each population, soil samples were collected from the surface (0–10 cm) and subsurface (10–20 cm) layers per site (Hamid et al., 2020a). The soil samples collected from 0 to 10 cm and 10 to 20 cm depths from each population were homogenized (appropriately mixed). At the same time, the soil samples were also air-dried, stored at room temperature, and sieved through a 2 mm sieve before the physicochemical examination. Fresh soil samples from each site were used to analyze soil pH, soil moisture content (MC) and temperature.
For the eco-physiological analysis, a total of 10 different parameters were measured as: RWC, LA, leaf dry mass content (LDMC), specific leaf area (SLA), proline content (PR), total chlorophyll (TCH), carotenoid content (CRT), total phenolics content (TPC), total flavonoids (TFV), and total soluble sugars (TSS).
2.3. Soil physico-chemical analysis
Soil temperature was measured using a field soil thermometer (TP 3001 Digital Thermometer). To determine soil moisture, we dried 5 g of fresh soil by keeping it overnight at 105°C. The percentage of soil MC was estimated as per Allen et al. (1974). Soil pH, electrical conductivity (CD), bulk density (BD), and particle density (PD) were measured by taking a soil: solution in distilled water at a ratio 1:2.5 at 24°C (Allen and Hajek, 1989). For the estimation of soil sodium (Na), magnesium (Mg), calcium (Ca) and sulfur (S), standard procedure of Allen et al. (1974) was followed. Total soil nitrogen (N) content was analyzed using the standard method of Kjeldahl (Bremner et al., 1965). The soil available phosphorous (P) was estimated by Olsen’s sodium bicarbonate method (Olsen et al., 1954). Available potassium (K) was estimated using the ammonium acetate and flame photometric detection method (Hanway and Heidel, 1952). Total soil organic carbon (TOC) was analyzed using chromic acid titration (Walkley and Black, 1934). In contrast, soil organic matter (SOM) was estimated by following the method of Hoogsteen et al. (2015).
2.4. Eco-physiological analysis
2.4.1. Functional traits
The functional traits (RWC, LDMC, LA, and SLA) that reflect plant ecological strategies were measured. Thirty mature, sun-exposed, healthy, fresh leaves were randomly selected from different plants at each sampling site to measure RWC, LA, LDMC, and SLA. The traits were estimated using the method followed by Rathore et al. (2018).
2.4.2. Determination of total phenolic and flavonoid content
The Folin–Ciocalteu’s test was used to determine the total phenolic content using gallic acid as a reference (Idris et al., 2017). The results were expressed as milligram gallic acid equivalent (mg GAE) per gram of dry sample using the equation based on the calibration curve of gallic acid: y = 0.0111x + 0.0456, R2 = 0.9965. The TFV content was measured using the aluminum chloride colorimetric technique with quercetin as a reference (Olajire and Azeez, 2011). The equation based on the quercetin calibration curve was used to translate the results into milligrams of quercetin equivalent (mg QE) per gram of dry sample: y = 0.0209x + 0.0503, R2 = 0.9984.
2.4.3. Determination of total chlorophyll and carotenoid content
We used the Arnon (1949) method to estimate the TCH and CRT. The following methods were used to calculate the chlorophyll concentrations: TCH (a + b) (mg.g–1) was calculated as (8.02 × A663 + 20.20 × A645) × A663 × V/1,000 × W, where V is the volume of the extract (ml) and W is the weight of the leaf tissue (g). The following calculation was used to calculate the amount of carotenoid present: A480 + (0.114 × A663–0.638 × A645).
2.4.4. Determination of total soluble sugars and proline assay
With minor changes, the anthrone method proposed by Yemm and Willis (1954) was used to estimate TSSs. The TSSs were estimated as mg.g–1 fresh weight using a calibration curve for a standard glucose solution. According to Bates et al. (1973), the calorimetric approach was utilized to quantify proline by generating a proline-acid ninhydrin complex. L-proline was employed as a control. The PR was determined and expressed using a standard curve as mol g–1 DW.
2.5. Data analysis
Data generated had three replicates per variable. A Two-way analysis of variance (ANOVA) was employed to evaluate the effects of altitude and soil depth (fixed effects) on soil physico-chemical parameters (response variable) by performing multiple pairwise-comparison between the means of sites and to identify the values with significant differences (p ≤ 0.05). Also, One-way ANOVA was performed to evaluate the effects of altitude on the eco-physiological parameters as a response variable to identify the values with significant differences (p ≤ 0.05). Principal component analysis (PCA) was performed to illustrate the commonalities between the different soil physico-chemical parameters, eco-physiological parameters and altitude using the FactoMineR (Husson et al., 2020) and factoextra (Kassambara and Mundt, 2020) packages. We employed Pearson’s pairwise multiple correlation analysis between soil and eco-physiological parameters, using the Performance Analytics package (Peterson and Carl, 2020) to visualize the relationship between different parameters. To study the effect of altitude on the soil’s physicochemical properties and eco-physiological parameters, we also used a linear regression model with the basicTrendline R package (Weiping and Guangchuang, 2020). R statistical software version 4.0.2 was used for all analyses (R Core Team, 2020).
3. Results
3.1. Variation in soil physico-chemical parameters of Rhododendron anthopogon along an altitudinal gradient
We observed a significant difference between soil physico-chemical properties and soil depth among five sites along the altitudinal gradient. The results of ANOVA pertaining to altitude and soil depth are given in Table 1 and Supplementary Table 1. The soil at all altitudes was slightly acidic 0–10 cm (5.54–4.52) and 10–20 cm (5.67–4.64). Soil pH varied significantly, decreasing with increasing altitude at 0–10 cm and 10–20 cm depths (p < 0.001). In addition, with 10–20 cm depth increase in soil pH was recorded (p < 0.001). We also observed a slight rise in Mg, C, Na (p < 0.001) and S (p < 0.01) with the increasing altitude at both 0–10 cm and 10–20 cm depths, while a decline in Mg and C was observed at 10–20 cm depth. Similarly, Na and S increase with altitude at 10–20 cm depth. BD was slightly decreased with the increase in altitude at both 0–10 cm and 10–20 cm depths (p < 0.001), and a rise in 10–20 cm depth was also recorded (p < 0.001). Soil MC, OM, SL, CD, N, K, and Ca show an increase with altitude at 0–10 cm and 10–20 cm depths (p < 0.001). Interestingly, all these parameters were significantly reduced with soil at 10–20 cm depth (Table 1 and Supplementary Table 1).
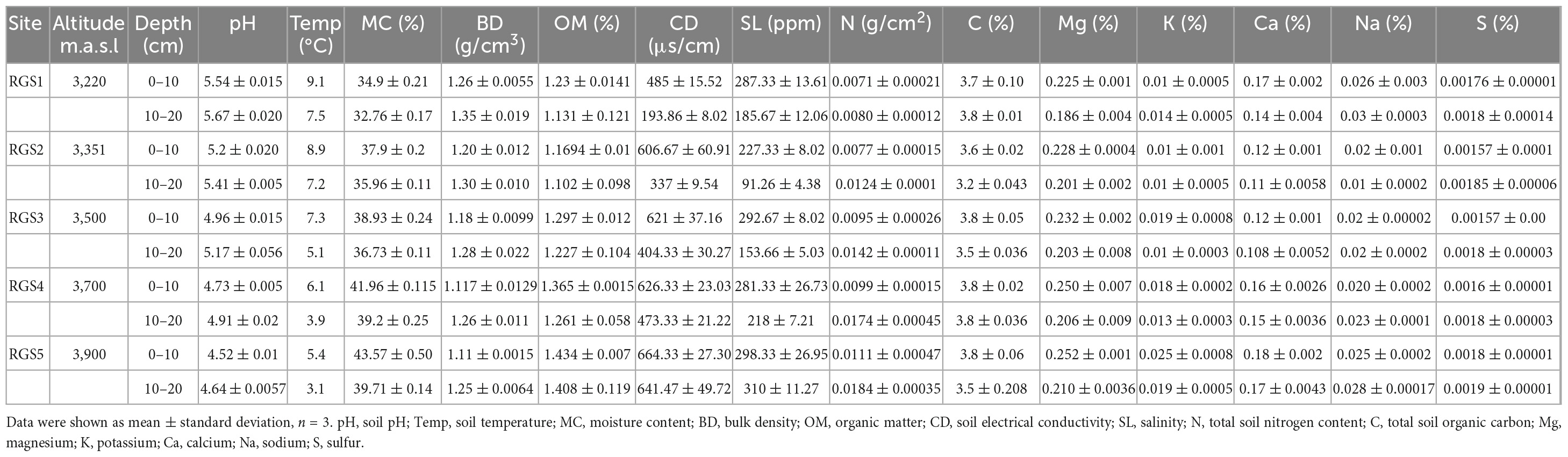
Table 1. Physico-chemical properties of soil at five sites along the altitudinal gradient in Gulmarg area of Kashmir Himalaya.
The linear regression analysis of soil physico-chemical parameters along the altitude gradient reveals a significant decreasing trend in pH and temp (p < 0.0001) with increasing altitude (Figure 2). With increasing altitude, the data also shows a significant increasing trend in MC, OM, K (p = 0.0001), SL (p = 0.00778), CD (p = 0.00806), N (p = 0.000442), and Ca (p = 0.00586). In contrast, BD (p = 0.747), C (p = 0.408), Na (p = 0.475), Mg (p = 0.768) and S (p = 0.343) do not show any trend with the altitude.
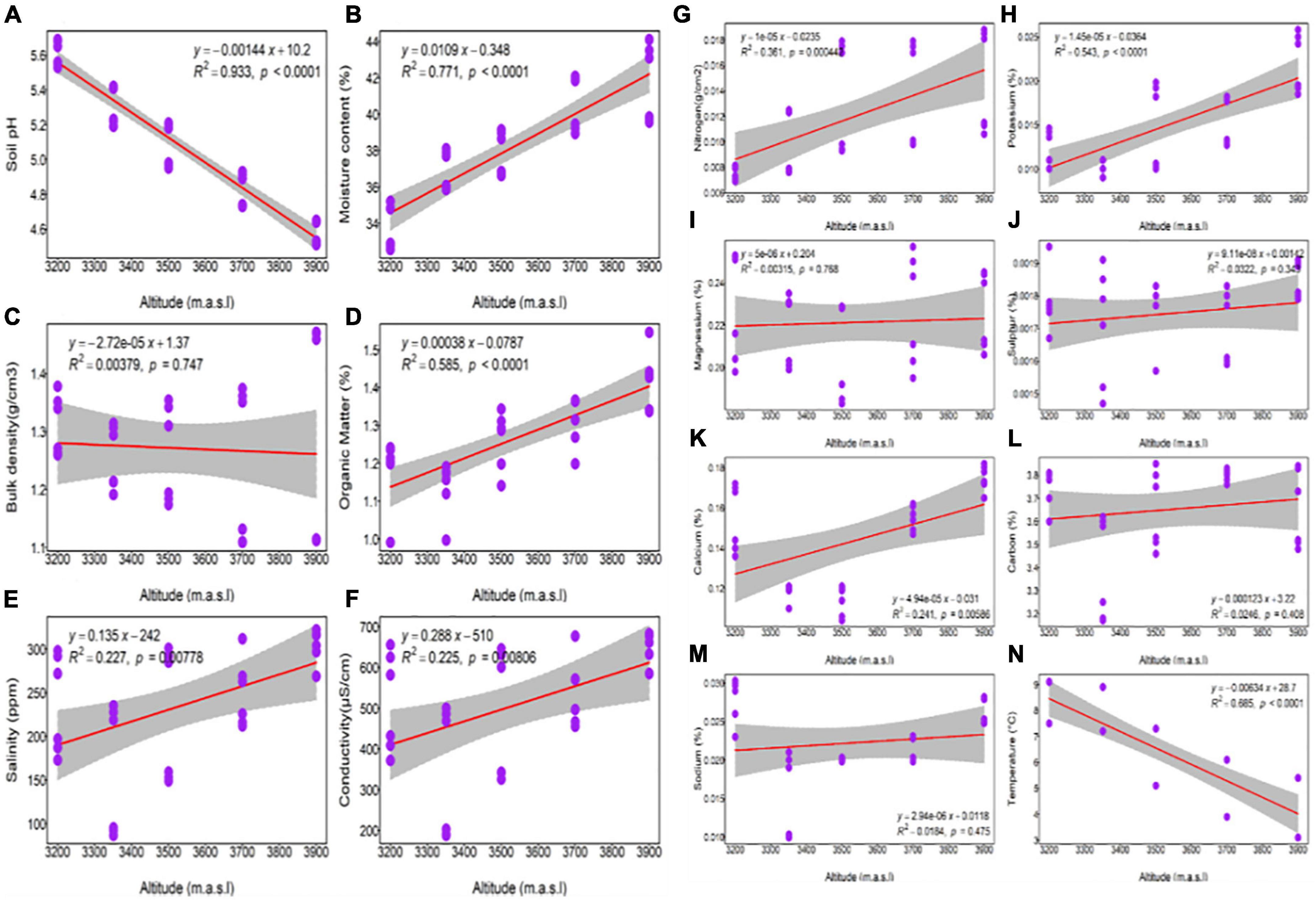
Figure 2. Linear regression representing a change in soil physico-chemical parameters of Rhododendron anthopogon along the altitude gradient. Relationship between altitude and (A) soil pH; (B) moisture content; (C) bulk density; (D) organic matter; (E) salinity; (F) soil electrical conductivity; (G) nitrogen; (H) potassium; (I) magnesium; (J) sulfur; (K) calcium; (L) total soil organic carbon; (M) sodium; (N) soil temperature. Each purple dot in the plot represents the mean value of the soil physico-chemical parameters, and the gray areas represent a 95% confidence interval.
3.2. Relationship between soil physico-chemical parameters of Rhododendron anthopogon along an altitudinal gradient
The PCA analysis is shown in Figure 3 wherein the first two principal components (PCs) show about 71.7% of the total variation. The plot grouped sites into four classes based on soil parameters. Site 1 and Site 2 (RGS1 and RGS2) were not differentiated from each other and made a clear group, whereas the other three sites differed well from this group by pH and temperature. The Site 3 (RGS3) was found to be dominated by BD, Mg and C. Site 4 (RGS4) revealed dominance of Na, Ca, MC, SL, N and S. Site 5 (RGS5) was dominated by OM, K, ALT and CD, which significantly separated Site 5 (RGS5) from the rest of the four sites (Figure 3).
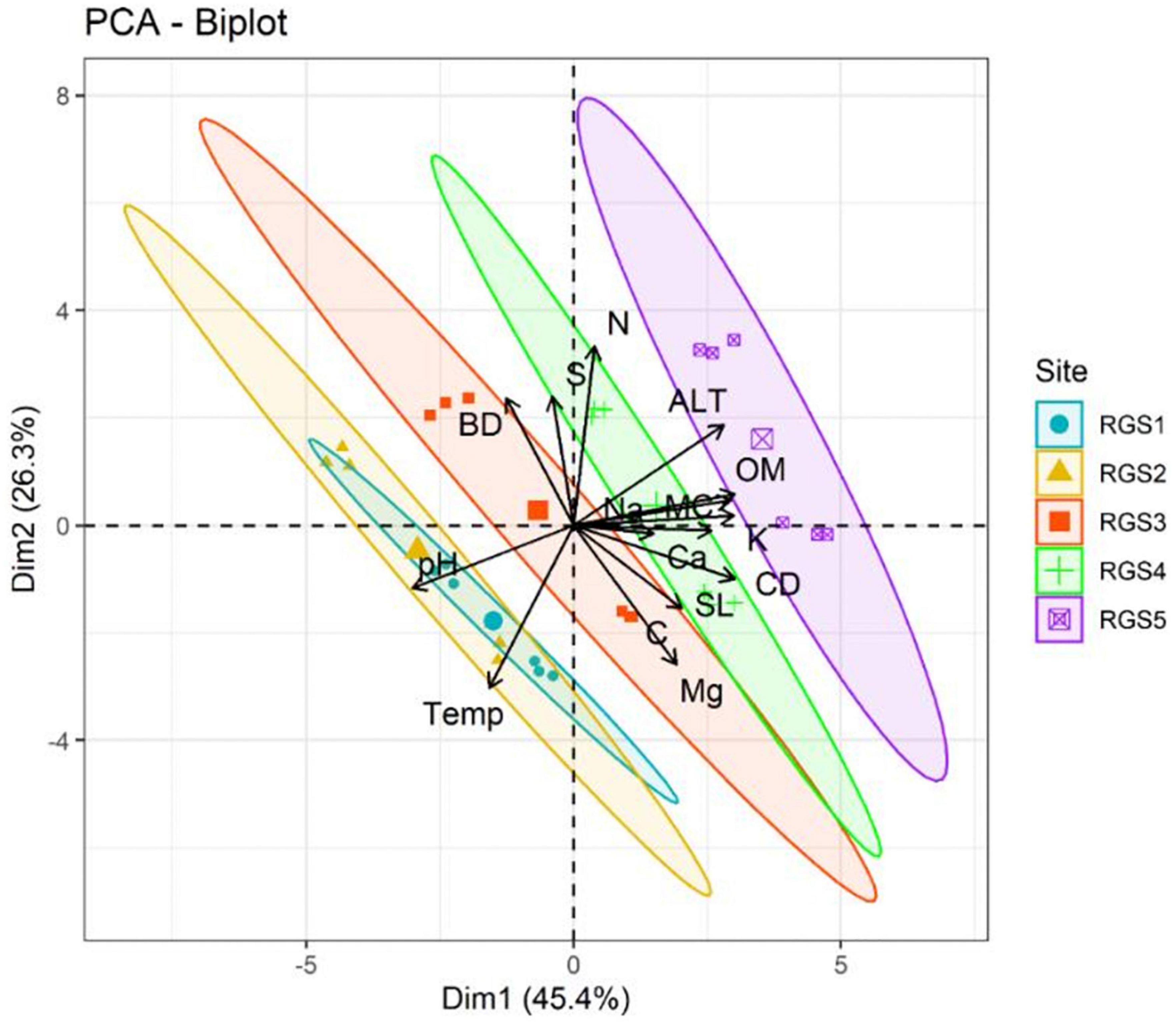
Figure 3. Principal component analysis (PCA) biplot of Rhododendron anthopogon soil physico-chemical parameters across the five sampling sites: Site-1 (RGS1), Site-2 (RGS2), Site-3 (RGS3), Site-4 (RGS4), and Site-5 (RGS5) along an altitudinal gradient in the Gulmarg area of Kashmir Himalaya. N, total soil nitrogen content; pH, soil pH; Temp, soil temperature; MC, moisture content; BD, bulk density; OM, organic matter; CD, soil electrical conductivity; SL, salinity; C, total soil organic carbon; Mg, magnesium; K, potassium; Ca, calcium; Na, sodium; S, sulfur; ALT, altitude.
Pearson’s correlation analysis indicated a relationship among studied soil parameters. Soil pH showed a significant negative correlation with ALT, MC, OM, K, SL, and CD, whereas there was a positive correlation with temp (p < 0.001). Soil K, MC, OM (p < 0.001), SL, CD, Ca and (p < 0.01) show a significant positive correlation with altitude. Temperature showed a negative correlation with N, ALT (p < 0.001), MC, OM and S (p < 0.01), whereas positive with Mg p < 0.05. C showed only a positive correlation with SL, CD, Na (p < 0.001), and Mg (p < 0.01), while CD (p < 0.001) shows a significant negative correlation with the pH and a positive correlation with the soil SL, K, OM, C, Mg, Ca (p < 0.001), ALT, MC and Na (p < 0.01) (Figure 4).
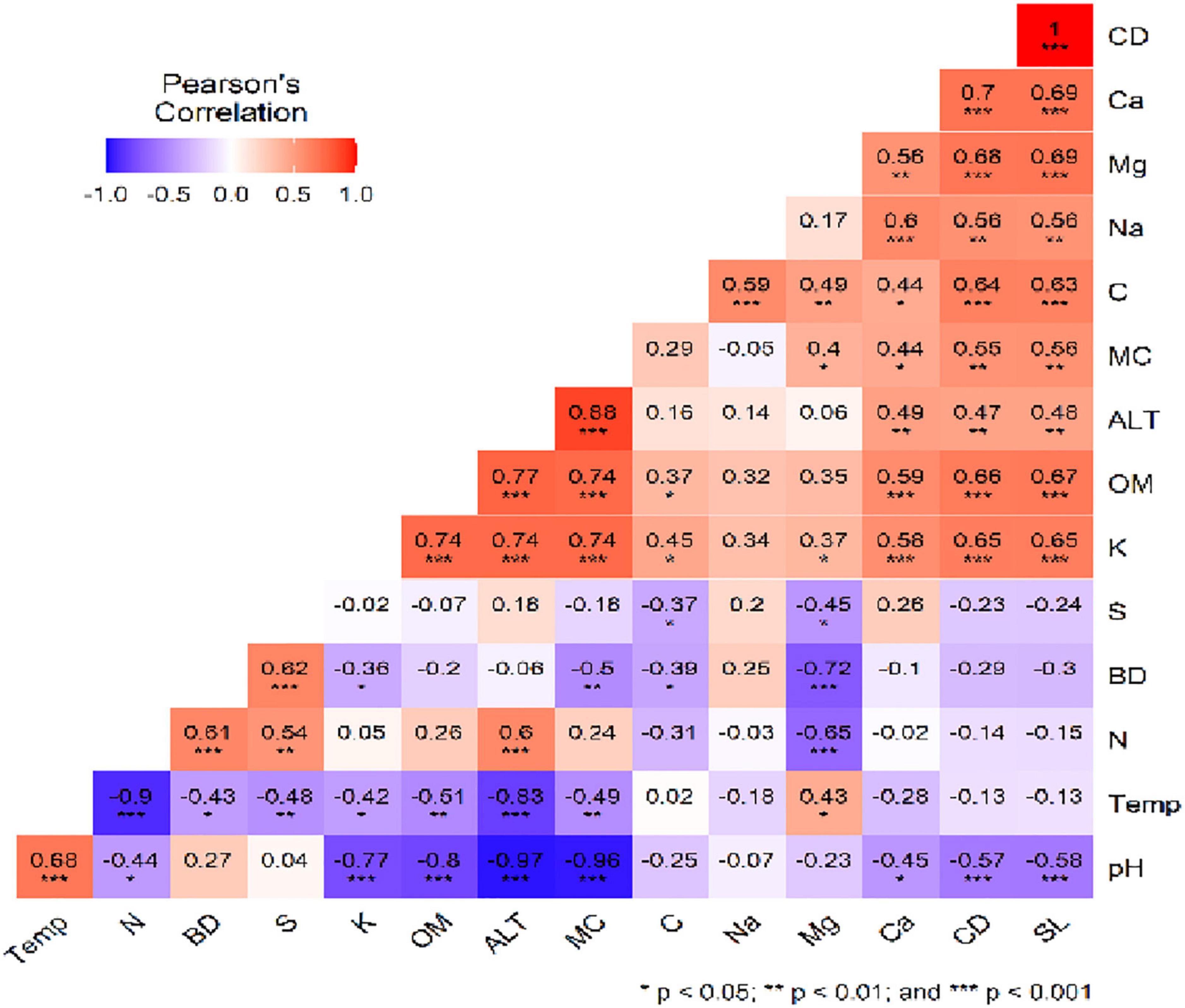
Figure 4. Pearson’s correlation plot among studied soil physico-chemical parameters. The distribution of each variable and correlation coefficient plus the significance level is given in the figure. Full forms of abbreviations are presented in Figure 2.
3.3. Variation in eco-physiological parameters of Rhododendron anthopogon along an altitudinal gradient
Eco-physiological parameters in the current study reveal a significant variation among different sites along altitude (Table 2 and Supplementary Table 2). One-way ANOVA shows a significant effect of altitude on eco-physiological parameters (Supplementary Table 2). We observed that RWC varied significantly, showing an increasing trend with the increasing altitude (p < 0.001). In contrast, LA and SLA significantly show a decreasing trend with the rising altitude (p < 0.001), and LDMC does not show any significant trend with the altitude. Similarly, PR, CRT, TPC, TFV and TSS varied significantly, showing an increasing trend with the increase in altitude (p < 0.001). On the other hand, TCH significantly decreased with the increase in altitude (p < 0.001) (Table 2 and Supplementary Table 2).

Table 2. Altitudinal variations in eco-physiological traits of Rhododendron anthopogon at five sites along the altitudinal gradient in Gulmarg area of Kashmir Himalaya.
The linear regression analysis of eco-physiological parameters concerning altitude shows a positive increasing trend of RWC, PR CRT, TSS, TPC (p < 0.0001) and TFV (p = 0.000194). Similarly, we also found a negative trend in LA, SLA and TCH (p < 0.0001) along altitude, while LDMC (p = 0.167) does not show any significant trend with increasing altitude (Figure 5).
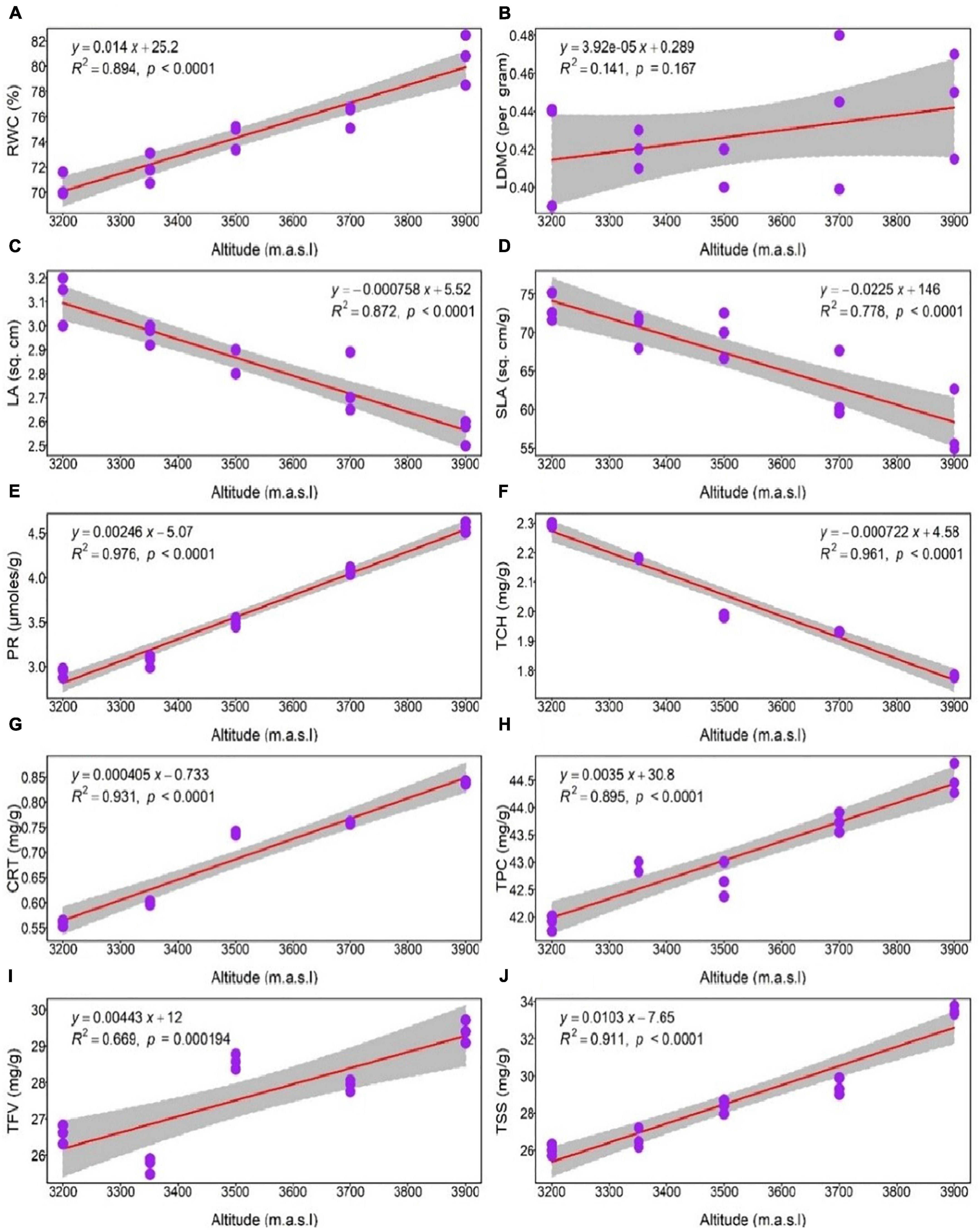
Figure 5. Linear regression representing a change in eco-physiological parameters of Rhododendron anthopogon along the altitude gradient. Relationship between altitude and (A) RWC, relative water content; (B) LDMC, leaf dry mass content; (C) LA, leaf area; (D) SLA, specific leaf area; (E) PR, proline content; (F) TCH, total chlorophyll; (G) CRT, carotenoid content; (H) TPC, total phenolics content; (I) TFV, total flavonoids; (J) TSS, total soluble sugars. Each purple dot in the plot represents the mean value of the eco-physiological parameters, and the gray areas represent a 95% confidence interval.
3.4. Relationship between eco-physiological traits of Rhododendron anthopogon along an altitudinal gradient
In the PCA analysis, the first two PCs show about 93.9% of total variation (Figure 6). Site 1 and Site 2 (RGS1 and RGS2) formed a separate group based on TCH, and LA from the rest of the sites. Similarly, Site 4 (RGS4) and Site 5 (RGS5) were separated by the most significant variables ALT, PR, RWC, TPC, CRT, TSS and LDMC, while Site 3 (RGS3) was different by SLA and TFV from other sites.
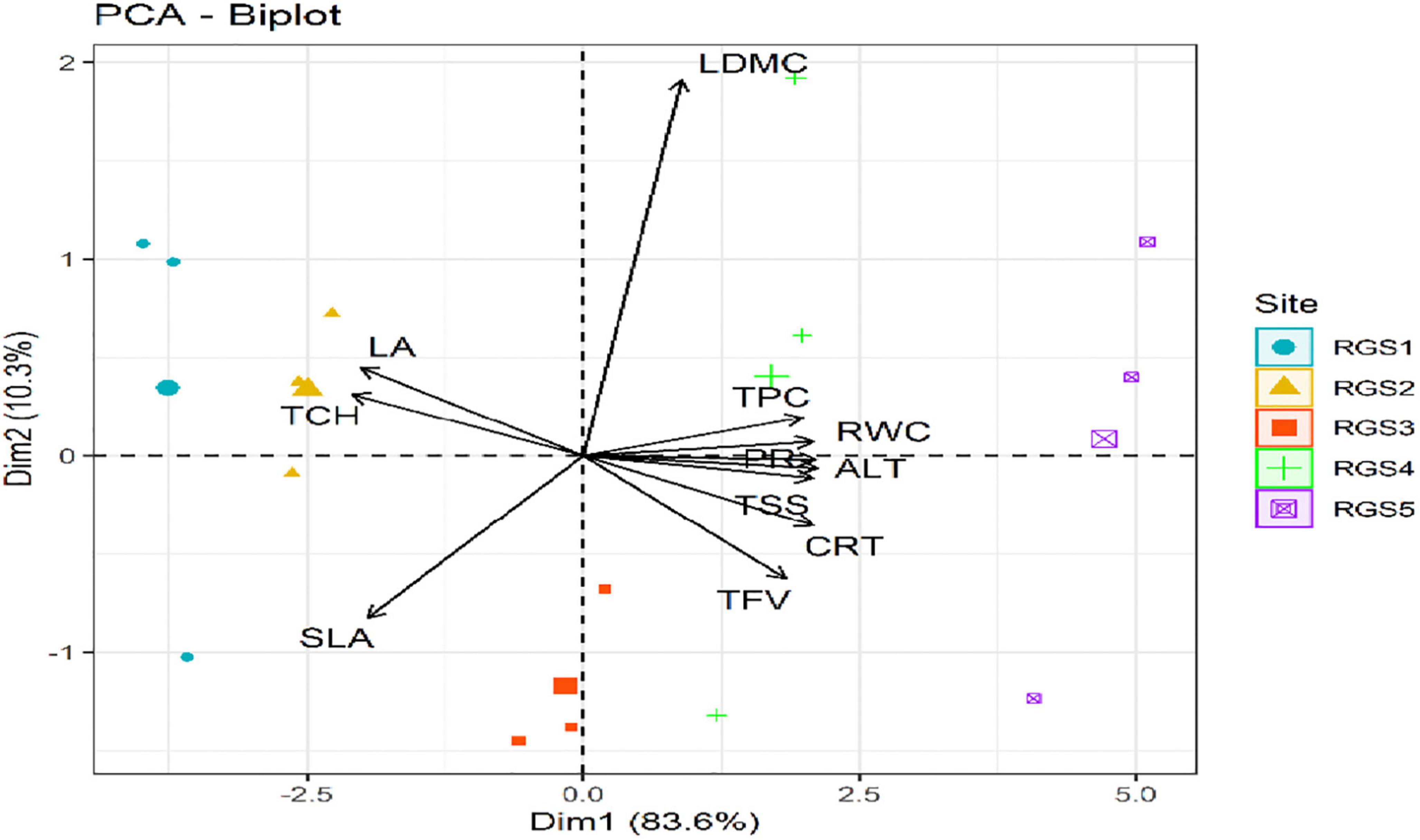
Figure 6. Principal component analysis (PCA) biplot of Rhododendron anthopogon eco-physiological traits across the five sampling sites: Site-1 (RGS1), Site-2 (RGS2), Site-3 (RGS3), Site-4 (RGS4), and Site-5 (RGS5) along an altitudinal gradient in the Gulmarg area of Kashmir Himalaya. RWC, relative water content; LDMC, leaf dry mass content; LA, leaf area; SLA, specific leaf area; PR, proline content; TCH, total chlorophyll; CRT, carotenoid content; TPC, total phenolics content; TFV, total flavonoids; TSS, total soluble sugars; ALT, altitude.
Pearson’s correlation analysis shows the relationship among different studied eco-physiological parameters (Figure 7). The result reveals that the SLA, LA and TCH show a significant negative correlation with the PR, ALT, CRT, TSS, RWC, TPC, and TFV. In contrast, SLA positively correlates with LA and TCH with p < 0.001. In comparison, TFV shows a significant positive correlation with PR, ALT, CRT, TSS and RWC with p < 0.001. Similarly, TPC, RWC, TSS, CRT and PR show a significant positive correlation with the ALT (p < 0.001).
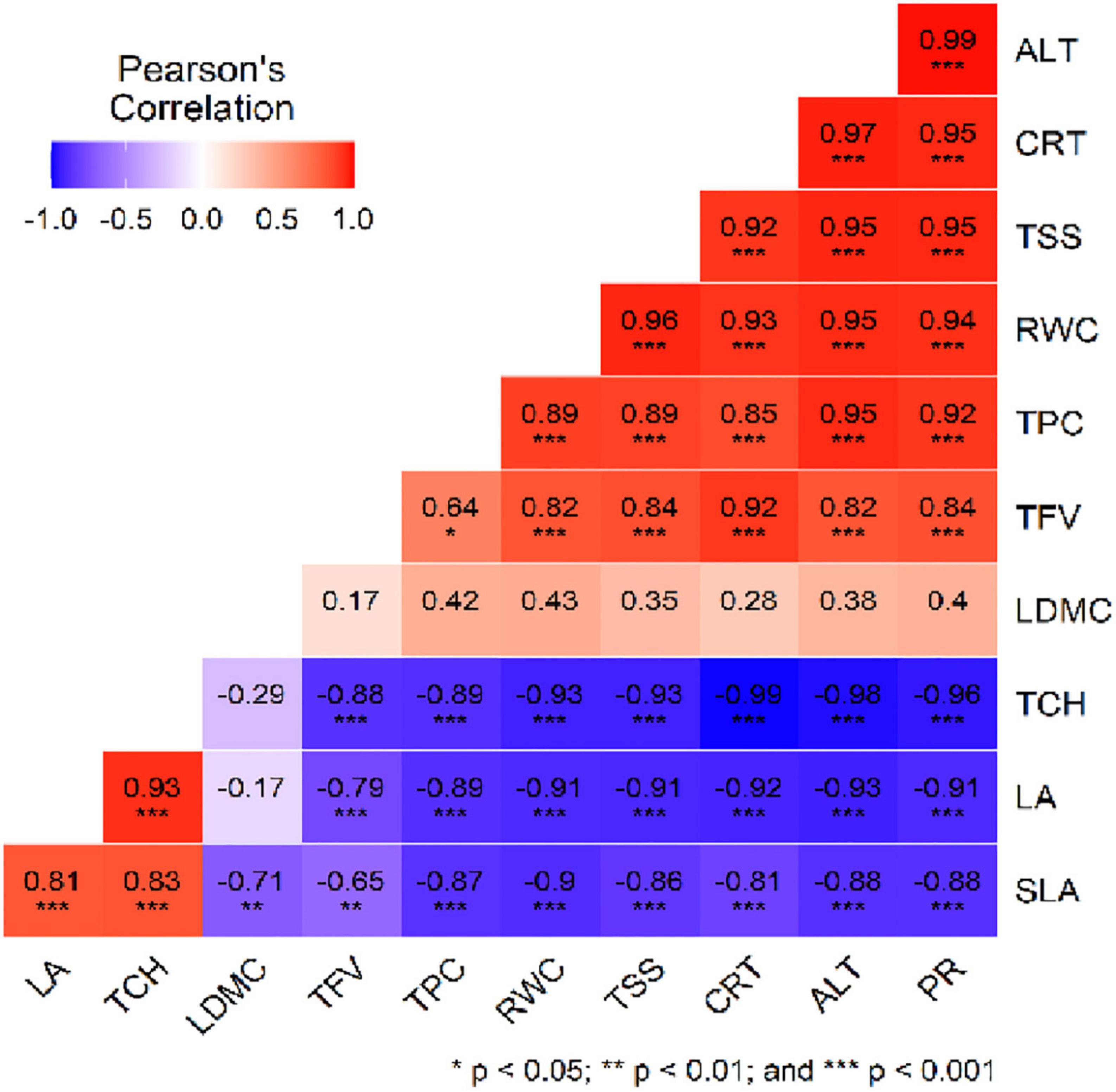
Figure 7. Pearson’s correlation plot of the eco-physiological parameters and altitude. The distribution of each variable and correlation coefficient plus the significance level is given in the figure. Full forms of abbreviations are presented in Figure 6.
4. Discussion
4.1. Variation in soil physico-chemical parameters of Rhododendron anthopogon along an altitudinal gradient
A significant difference was observed between the soil physico-chemical properties of Rhododendron anthopogon among all the sites along the altitudinal gradient (Table 1 and Figure 2). Soil pH varied significantly, showing decreasing trend with increasing altitude. This can be attributed to the differences in soil temperature along the altitudinal gradient, as we also observed a decline in temperature with an increase in altitude. Variations in species composition and SOM along altitudinal gradients can also influence soil acidity by accumulating various organic acids (Egli et al., 2009). Furthermore, environmental factors such as slope and aspect substantially influence pH in alpine regions. A fall in pH at higher elevations may be attributable to the leaching of base cations caused by increased precipitation and a steeper slope (Tsui et al., 2004). The acidic nature of soil found in all altitudes confirms that R. anthopogon flourishes in acidic soil. The lower temperature at higher altitudes results in a slower decomposition rate and accumulates more organic matter (Northcott et al., 2009; Liang et al., 2016; Kumar et al., 2019). This is consistent with our findings as organic matter (OM) shows an increasing trend with an increase in altitude. Soil bulk density (BD) is an important parameter influencing root development, soil aeration, and water infiltration (Basso et al., 2013). The results of our study revealed that the average soil bulk density slightly decreased with the increase in altitude at both 0–10 cm and 10–20 cm depths, and a rise in 10–20 cm depth was also recorded. The observed pattern is consistent with findings from other research (Griffiths et al., 2009; Yang et al., 2018). The decrease in bulk density with increasing altitude might be due to soil porosity (Li et al., 2017), increased soil organic carbon content (Haynes and Naidu, 1998), increased ratio of silt and clay content in the soil (Wen et al., 2005) and freezing and thawing process (Chen et al., 1998). The present findings revealed that with an increase in altitude in the study area, soil infiltration capacity increased, which would be helpful for the growth and development of R. anthopogon.
Soil MC significantly affects the growth and distribution of vegetation (Porporato et al., 2004; Paschalis et al., 2015). In the present study, we observed a significant increase in MC with altitude. The presence of high precipitation and low temperature at higher altitudes could explain this trend. Optimum MC in the soil increases the decomposition of organic matter and microbial movement, which controls nutrient availability (Zhou et al., 2002). This might have an impact on R. anthopogon’s ecophysiology. Like soil MC, the CD also showed an increased trend with altitude. Low temperature at higher altitudes is responsible for the slow decomposition of organic matter, leading to higher soil cation exchange capacity and increasing CD values (Bardelli et al., 2017). Similarly, we observed a slight rise in Mg, C, Na and S with the increasing altitude; thus, they are not limiting factors of alpine regions. A similar trend in micronutrient dynamics with respect to altitudinal variation was found (Wang et al., 2011; Kidanemariam et al., 2012; Charan et al., 2013; Du et al., 2014; Yang et al., 2018; Mishra and Francaviglia, 2021).
In the case of N and K, increasing trends along the altitudinal gradient were observed (Figure 2). These results align with several other studies (Göransson et al., 2014; Bardelli et al., 2017; Zhu et al., 2019). Differences in soil temperature along the altitudinal gradient in our study area could be attributed to the availability of different levels of soil N. Furthermore, an increased K concentration with altitude could be related to better rock and mineral dissolution in slightly higher acidic conditions at higher altitudes. Usually, the topsoil layer is more vulnerable to changing environments, such as changes in temperature, precipitation, and organic matter input (Hamid et al., 2020a). In all elevations of the study area, topsoil (0–10 cm) revealed a significantly higher concentration of soil parameters than the subsoil (10–20 cm). These results also conform to other studies (Huang et al., 2011; Liu et al., 2013; He et al., 2016; Qasba et al., 2017; Yang et al., 2018). The present study also indicates that leaching, commonly associated with alpines, did not occur in the study area, which plays an essential role in transporting the subsurface’s extractable nutrients away from the surface layer (Hamid et al., 2020a).
4.2. Variation in eco-physiological parameters of Rhododendron anthopogon along an altitudinal gradient
We found a significant variation in eco-physiological parameters of R. anthopogon along altitude (Table 2 and Figure 5). An increasing trend of RWC observed in the present study could be explained by high precipitation and low temperature at higher altitudes. However, Rathore et al. (2018) reported a negative relationship between RWC with altitude, while Schob et al. (2013) reported a positive correlation with altitude. Photosynthetic pigments which absorb and transform light directly impact plant photosynthetic capability and are susceptible to environmental challenges. Water stress, intense sunlight, and high temperature can diminish chlorophyll concentration by slowing chlorophyll synthesis, hastening decomposition, or causing damage to chloroplast structures (Ashraf and Harris, 2013; Hazrati et al., 2016; Cui et al., 2018). In the present study, TCH, LA and SLA decreased with increasing elevation, which could be an adaptive response of R. anthopogon to avoid oxidative damage by limiting light absorption and preventing reactive oxygen species (ROS) generation (Cui et al., 2018). Thus, plants have developed efficient ways to eliminate surplus energy to avoid damage to the photosynthetic mechanism at high altitudes where radiation is more potent (Li H. et al., 2022). This indicates that the efficient defense mechanism against damage from high radiation in R. anthopogon was primarily implemented through heat dissipation at higher altitudes. There was no discernible variation in LDMC throughout the altitudinal gradient; therefore, it is likely not a limiting factor in alpine areas.
Carotenoids are versatile compounds that play an essential role in photosynthesis and the construction of light-harvesting complexes, as well as can remove accumulated ROS efficiently to prevent photooxidation-related damage to chloroplasts (Armstrong and Hearst, 1996; Li T. X. et al., 2022). High altitudes are known to cause a decrease in carotenoid concentration, while the contrary has also been reported (Ahmad et al., 2016). Our study showed an increasing trend of CRT along the altitudinal gradient, indicating the possibility that environmental elements at a higher altitude may have encouraged the biosynthesis of CRT. These findings are consistent with other studies (Cui et al., 2018; Rathore et al., 2018). The significant decrease in TCH and the increase in CRT in high-altitude zones could be attributed to a reduction in photosynthesis and photoinhibition (Demmig-Adams and Adams, 1992).
Phenolic compounds protect plants from structural damage by shielding harmful UV-B radiations. Because of more intense UV-B radiation stress, plants in alpine regions are likely to include more phenolics than in low altitudes (Bernal et al., 2013). Similarly, we observe an increasing trend of TPC and TFV in R. anthopogon along an altitudinal gradient (Figure 5). These phenolic and flavonoid chemicals significantly improve the adaptation to abiotic oxidative stress (Hashim et al., 2020). Increasing phenolic concentrations have been reported as a herbivore defense system for plant attacks (Chadwick et al., 2013; Rathore et al., 2018). Herbivore attacks decrease with increasing altitude, which may account for the higher TPC at higher altitudes (Carey and Wink, 1994; Hodkinson, 2005; Rathore et al., 2018).
Proline and TSS are the two most significant osmotic regulators under abiotic stress conditions, allowing plants to tolerate harsh environments, such as cold temperatures (Bartels and Sunkar, 2005; Zhang et al., 2012; Adnan et al., 2016). Accumulation of proline with increasing altitude observed in the present study could effectively lower the freezing temperature and shield R. anthopogon from frozen injury, highlighting the significance of proline accumulation in plant adaptation. This concurs with previous related studies showing an increasing trend in proline (Ma et al., 2015; Cui et al., 2018; Rathore et al., 2018; Li T. X. et al., 2022). A significant increase in the TSS observed in the present study could be the ability of carbohydrates to withstand low temperatures and boost the supercooling capability of long-lasting R. anthopogon leaves (Rada et al., 2001). As described in earlier studies, the ability to accumulate soluble sugars on high mountains may also be the primary reason for the persistence of R. anthopogon in future (Rathore et al., 2018).
Overall, we observed significant variations in eco-physiological parameters, demonstrating their critical involvement in adapting R. anthopogon to the harsh environment of the alpine regions of Kashmir Himalaya. The observed patterns revealed how important trait flexibility is for the survival and function of this species. Additionally, it is believed that variation in traits brought on by plasticity in functional features will impact the expansion of this species’ niche breadth, leading to more comprehensive ranges (Bolnick et al., 2011). The Himalayan region is warming considerably more quickly than the rest of the world; hence the projected climate change scenario will not face limitations imposed by low temperatures (Salinger et al., 2014). In alpine regions, this will cause shrubs to move upward. A prolonged growing season brought on by climate change will help R. anthopogon to spread to higher elevations (He et al., 2016; Rathore et al., 2018).
4.3. Relationship between soil physico-chemical parameters of Rhododendron anthopogon along an altitudinal gradient
Various environmental variables, such as temperature, moisture, oxygen, photoperiod, UV intensity, and light intensity, fluctuate along the altitudinal gradient (Chen et al., 2014). As the altitude rises, precipitation, light, oxygen pressure, and radiation intensity all rise while gravity and temperature decrease concurrently. Soil nutrients also significantly influence species distribution along an altitudinal gradient. Consequently, the impact of altitude on plant growth and development results from several elements functioning in concert (Ahmad et al., 2016). In the present study, the PCA analysis of studied soil physico-chemical parameters of R. anthopogon revealed that all the sites were grouped into four clusters according to altitude, suggesting a more pronounced effect of altitude (Figure 3). The most significant variables differentiating Site 1 and Site 2 from the rest were pH and temperature. Soil pH and Temperature are important factors affecting plant diversity in alpine regions. As soil pH directly affects nutrient availability, microbial activity, immobilization and mineralization (Curtin et al., 1998; Evans et al., 2012). At the same time, soil temperature affects alpine plants by providing a fundamental niche and is essential to withstand extreme climatic conditions (Hamid et al., 2020a). Accordingly, Site 5 was dominated by OM, K, ALT, and CD variables, significantly separating Site 5 from the rest of the four sites. These variables can be considered helpful for the growth and development of R. anthopogon at higher altitudes. This is consistent with the notion that changes in soil variables account for variation in vegetation in higher altitudes and the species-specific diversity of alpine plants (Arnesen et al., 2007; Hamid et al., 2020a).
The current investigation results showed that most soil variables had strong correlations with one another (Figure 4). This also conforms with results from other studies (Ahmad et al., 2016; Yang et al., 2018; Yuan et al., 2019; Hamid et al., 2020a). Litter decomposition and pH variations in the soil impact the overall availability of nutrients in the soil (Curtin et al., 1998). Soil pH is an essential factor regulating nutrient availability by favoring conditions that accelerate oxidation, mineralization, and immobilization (Mathew et al., 2016). In the present study, we noticed a substantial negative correlation of soil pH with ALT, MC, OM, K, SL and CD. This is in line with the fact that acidic solid conditions and decomposition rates at higher altitudes favored the availability of these soil variables. The present study also observed a significant positive correlation between soil pH with temperature. The increased temperature significantly increases soil pH. Guoju et al. (2012) reported that 0.5°C–2.0°C increases in winter temperature increased soil pH by 0.42–0.67 compared to no temperature increase. Similarly, soil K, MC, OM, SL, CD, and Ca show a significant positive correlation with altitude, suggesting their relationship enhances their availability. Therefore, the present study adds to our understanding that R. anthopogon have effective metabolic separation and resource preferences, even over short distances, due to their variable reactions to soil physico-chemical parameters, which may aid the conservation of this endemic species.
4.4. Relationship between eco-physiological traits of Rhododendron anthopogon along an altitudinal gradient
Efficient physiological modifications are essential for developing and growing high-altitude plants, as their seasonal changes are caused by environmental variables (Magaña Ugarte et al., 2019). In the present study, PCA analysis of studied eco-physiological traits of R. anthopogon revealed that Site 1 and Site 2 formed a separate group based on TCH and LA from the rest of the sites (Figure 6). TCH and LA are considered critical factors in promoting photosynthesis and growth (Takashima et al., 2004; Han et al., 2020). A higher concentration of these traits in Site 1 and Site 2 suggests an apparent disparity among altitudes. Similarly, Site 4 and Site 5 were separated by the most significant variables ALT, PR, RWC TPC, CRT, TSS and LDMC. Therefore, these variables might have a more dramatic impact on the eco-physiology of R. anthopogon. In a related study on R. anthopogon by Rathore et al. (2018), the PCA biplot showed distinct clusters based on the season compared to altitude. In another study, the eco-physiological traits of four Rhododendrons had similar adaptive features to warm and cold seasonal adversity in subalpine regions (Li H. et al., 2022). The present study’s correlation analysis revealed a significant relationship among eco-physiological parameters (Figure 7). Our results indicated that the SLA, LA and TCH showed a significant negative correlation with the PR, ALT, CRT, TSS, RWC, TPC and TFV. This suggests that higher altitude with a low concentration of SLA, LA, and TCH favors the availability of PR, ALT, CRT, TSS, RWC, TPC, and TFV parameters. Galiba (1994) found a positive correlation between proline synthesis and plant adaptability. Other researchers have unambiguously stated that, in addition to other solutes, the proline level increase substantially during cold hardening (Galiba, 1994; Mahajan and Tuteja, 2005).
Furthermore, proline, which has been demonstrated to be an excellent cryoprotectant, is one of the critical variables associated with freezing tolerance (Mahajan and Tuteja, 2005). According to Kandler and Hopf (1982), cold stress caused TSS to accumulate in various plant species. For example, Bano and Fatima (2009) noted that higher-altitude alpine herbs in Pakistan’s Hunza Valley had the highest level of TSS. To protect plant components such as cell membranes from cold stress, TSS buildup along the altitudinal gradient is an excellent method. The present study also observed a significant positive correlation of TPC, RWC, TSS, CRT and PR with the ALT. This indicates that these parameters play an important role in adapting R. anthopogon in the alpine regions of Kashmir Himalaya.
5. Conclusions
Understanding the variation in soil physico-chemical and eco-physiological attributes along altitudinal gradient is essential to predict indirectly how climate change will affect R. anthopogon. The present study revealed that soil physico-chemical and eco-physiological parameters along an altitudinal gradient significantly affect R. anthopogon in Kashmir Himalaya. We observed that with an increase in altitude in the study area, soil in filtration capacity increased, which would be helpful for the growth and development of R. anthopogon. A significant variation observed in eco-physiological parameters, demonstrating their critical involvement in adapting R. anthopogon to the harsh environment of the alpine regions of Kashmir Himalaya. Our results indicate how soil physico-chemical and eco-physiological properties help R. anthopogon spread widely and withstand various stresses. Additionally, by repeatedly observing the plasticity of soil physico-chemical and eco-physiological characteristics across different altitudes, we will be able to extrapolate the effects of climate change on these geographical gradients. The results generated during the present study also revealed that R. anthopogon has a significant potential for conducting studies on cold tolerance in the Himalaya’s fragile ecology. Therefore, from the present work, we deduce that R. anthopogon has sufficient soil physico-chemical and eco-physiological plasticity, which should be favorable for its survival in future climates, offering an adaptive advantage and expanding its range shortly.
Data availability statement
The original contributions presented in this study are included in the article/Supplementary material, further inquiries can be directed to the corresponding author.
Author contributions
TD conceptualized, designed, and supervised the study. ZM carried out the field and laboratory work. SI, LT, SK, and AM, supported in field and laboratory work. RA, SG, RB, and SB analyzed the data. TD and ZM wrote the manuscript. All authors reviewed the manuscript and approved it for publication.
Funding
This work was supported by funding from Department of Biotechnology, Ministry of Science and Technology, Government of India, New Delhi, under grant no.: BT/PR29259/FCB/125/2/2018.
Acknowledgments
We are thankful to Dr. A. A. Shah, Associate Dean, School of Biosciences and Biotechnology, Baba Ghulam Shah Badshah University, Rajouri, for facilitating this work. During this work, financial support received from the Department of Biotechnology, Government of India, New Delhi to TD under grant no.: BT/PR29259/FCB/125/2/2018 is highly acknowledged.
Conflict of interest
The authors declare that the research was conducted in the absence of any commercial or financial relationships that could be construed as a potential conflict of interest.
Publisher’s note
All claims expressed in this article are solely those of the authors and do not necessarily represent those of their affiliated organizations, or those of the publisher, the editors and the reviewers. Any product that may be evaluated in this article, or claim that may be made by its manufacturer, is not guaranteed or endorsed by the publisher.
Supplementary material
The Supplementary Material for this article can be found online at: https://www.frontiersin.org/articles/10.3389/ffgc.2023.1181299/full#supplementary-material
References
Adamczyk, M., Hagedorn, F., Wipf, S., Donhauser, J., Vittoz, P., Rixen, C., et al. (2019). The soil microbiome of Gloria Mountain summits in the Swiss Alps. Front. Microbiol. 10:1080. doi: 10.3389/fmicb.2019.01080
Adnan, M. Y., Hussain, T., Asrar, H., Hameed, A., Gul, B., Nielsen, B. L., et al. (2016). Desmostachya bipinnata manages photosynthesis and oxidative stress at moderatesalinity. Flora Morphol. Dist. Func. Ecol. Plants 225, 1–9. doi: 10.1016/j.flora.2016.09.006
Ahmad, K. S., Hameed, M., Fatima, S., Ashraf, M., Ahmad, F., and Naseer, M. (2016). Morpho-anatomical and physiological adaptations to high altitude in some Aveneae grasses from NeelumValley, Western Himalayan Kashmir. Acta Physiol. Plantar. 38:93. doi: 10.1007/s11738-016-2114-x
Ahmad, K. S., Hameed, M., Hamid, A., Nawaz, F., Kiani, B. H., Ahmad, M. S. A., et al. (2018). Beating cold by being tough: impact of elevationon leaf characteristics in Phleum himalaicum Mez. endemic to Himalaya. Acta Physiol. Plantar. 40, 1–17. doi: 10.1007/s11738-018-2637-4
Ahrens, C. W., Andrew, M. E., Mazanec, R. A., Ruthrof, K. X., Challis, A., Hardy, G., et al. (2020). Plant functional traits differ in adaptability and arepredicted to be differentially affected by climate change. Ecol. Evol. 10, 232–248. doi: 10.1002/ece3.5890
Alberto, F. J., Aitken, S. N., Alía, R., González-Martínez, S. C., Hänninen, H., Kremer, A., et al. (2013). Potential forevolutionary responses to climate change–evidence from tree populations. Glob. Change Biol. 19, 1645–1661. doi: 10.1111/gcb.12181
Allen, B. L., and Hajek, B. F. (1989). Mineral occurrence in soil environments. Min. Soil. Environ. 1, 199–278. 2ed.c5 doi: 10.2136/sssabookser1
Allen, S. E., Grimshaw, H. M., Parkinson, J. A., Quarmby, C., and Roberts, J. D. (1974). Chemical analysis of ecological materials. London: Blackwell Scientific Publications.
Anderegg, W. R., Klein, T., Bartlett, M., Sack, L., Pellegrini, A. F., Choat, B., et al. (2016). Meta-analysis reveals that hydraulic traits explain cross-species patterns of drought-induced tree mortality across the globe. Proc. Natl. Acad. Sci. U.S.A. 113, 5024–5029. doi: 10.1073/pnas.1525678113
Anic, V., Hinojosa, L. F., Díaz-Forester, J., Bustamante, E., de la Fuente, L. M., Casale, J. F., et al. (2010). Influence of soil chemical variables and altitude on the distribution ofhigh-alpine plants: the case of the Andes of Central Chile. Arctic Antarctic Alpine Res. 42, 152–163. doi: 10.1657/1938-4246-42.2.152
Antonelli, A., Kissling, W. D., Flantua, S. G. A., Bermúdez, M. A., Mulch, A., MuellnerRiehl, A. N., et al. (2018). Geological and climatic influences onmountain biodiversity. Nat. Geosci. 11, 718–725. doi: 10.1038/s41561-018-0236-z
Armstrong, G. A., and Hearst, J. E. (1996). Carotenoids 2: genetics and molecular biology ofcarotenoid pigment biosynthesis. FASEB J. 10, 228–237. doi: 10.1096/fasebj.10.2.8641556
Arnesen, G., Beck, P. S., and Engelskjøn, T. (2007). Soil acidity, content of carbonates, and available phosphorus are the soil factors best correlated with alpine vegetation: evidence from Troms, North Norway. Arctic Antarctic Alpine Res. 39, 189–199. doi: 10.1657/1523-0430200739[189:sacoca]2.0.co;2
Arnon, D. I. (1949). Copper enzymes in isolated chloroplasts. Polyphenol oxidase in Beta vulgaris. Plant Physiol. 24, 1–15. doi: 10.1104/pp.24.1.1
Ashraf, M., and Harris, P. J. C. (2013). Photosynthesis under stressful environments: an overview. Photosynthetica 51, 163–190. doi: 10.1007/s11099-013-0021-6
Bano, A., and Fatima, M. (2009). Salt tolerance in Zea mays (L.) following inoculation withRhizobium and Pseudomonas. Biol. Fertil. Soils 45, 405–413. doi: 10.1007/s00374-008-0344-9
Bardelli, T., Gómez-Brandón, M., Ascher-Jenull, J., Fornasier, F., Arfaioli, P., and Francioli, D. (2017). Effects of slope exposure on soil physico-chemical and microbiological properties along an altitudinal climosequence in the Italian Alps. Sci. Total Environ. 575, 1041–1055. doi: 10.1016/j.scitotenv.2016.09.176
Bartels, D., and Sunkar, R. (2005). Drought and salt tolerance in plants. Crit. Rev. Plant Sci. 24, 23–58. doi: 10.1080/07352680590910410
Basso, A. S., Miguez, F. E., Laird, D. A., Horton, R., and Westgate, M. (2013). Assessing potential of biochar for increasing water-holding capacity of sandy soils. Glob. Change Biol. Bioenergy 5, 132–143. doi: 10.1111/gcbb.12026
Bates, L. S., Waldren, R. P., and Teare, I. D. (1973). Rapid determination of free proline for water-stress studies. Plant Soil 39, 205–207.
Bellard, C., Bertelsmeier, C., Leadley, P., Thuiller, W., and Courchamp, F. (2012). Impacts of climate change on the future of biodiversity. Ecol. Lett. 15, 365–377. doi: 10.1111/j.1461-0248.2011.01736.x
Bernal, M., Llorens, L., Julkunen-Tiitto, R., Badosa, J., and Verdaguer, D. (2013). Altitudinaland seasonal changes of phenolic compounds in Buxus sempervirens leaves and cuticles. Plant Physiol. Biochem. 70, 471–482. doi: 10.1016/j.plaphy.2013.06.012
Bolnick, D. I, Amarasekare, P., Araújo, M. S., Bürger, R., Levine, J. M., Novak, M., et al. (2011). Why intraspecific trait variation matters in community ecology. Trends Ecol. Evol. 26, 183–192. doi: 10.1016/j.tree.2011.01.009
Bremner, J. M. (1965). “Total nitrogen,” in Methods of soil analysis: Part 2 chemical and microbiological properties, Vol. 9, ed. A. G. Norman (Madison, WI: American Society of Agronomy), 1149–1178.
Carey, D., and Wink, M. (1994). Elevational variation of quinolizidine alkaloid contents in alupine (Lupinus argentus) of the rocky mountains. J. Chem. Ecol. 20, 849–857. doi: 10.1007/BF02059582
Chadwick, M., Trewin, H., Gawthrop, F., and Wagstaff, C. (2013). Sesquiterpenoids Lactones: benefits to plants and people. Int. J. Mol. Sci. 14, 12780–12805. doi: 10.3390/ijms140612780
Charan, G., Bharti, V. K., Jadhav, S. E., Kumar, S., Acharya, S., Kumar, P., et al. (2013). Altitudinal variations in soil physico-chemical properties at cold deserthigh altitude. J. Soil Sci. Plant Nutr. 13, 267–277. doi: 10.4067/S0718-95162013005000023
Chen, B. X., Zhang, X. Z., Tao, J., Wu, J. S., Wang, J. S., Shi, P. L., et al. (2014). The impact of climate change and anthropogenic activities on alpine grassland over the Qinghai-Tibet Plateau. Agr. For. Meteorol. 189, 11–18. doi: 10.1016/j.agrformet.2014.01.002
Chen, Y., Tessier, S., and Rouffignat, J. (1998). Soil bulk density estimation for soil tillagesystem and soil texture. Trans. ASAE. 41, 1601–1610. doi: 10.13031/2013.17328
Cui, G., Li, B., He, W., Yin, X., Liu, S., Lian, L., et al. (2018). Physiological analysis ofthe effect of altitudinal gradients on Leymus secalinus on the Qinghai-Tibetan Plateau. PLoS One 13:e0202881. doi: 10.1371/journal.pone.0202881
Curtin, D., Campbell, C. A., and Jalil, A. (1998). Effects of acidity on mineralization: dependence of organic matter mineralization in weakly acidic soils. Soil Biol. Biochem. 30:5764. doi: 10.1016/S0038-0717(97)00094-1
Dad, J. M., Muslim, M., Rashid, I., and Reshi, Z. A. (2021). Time series analysis of climatevariability and trends in Kashmir Himalaya. Ecol. Indicat. 126:107690. doi: 10.1016/j.ecolind.2021.107690
Decker, K. L., and Boerner, R. E. J. (2003). Elevation and vegetation influences on soil properties in Chilean Nothofagus forests. Rev. Chil. Hist. Nat. 76, 371–381.
Demmig-Adams, B., and Adams, W. W. (1992). Carotenoid composition in sun and shade leaves of plants with different life forms. Plant Cell Environ. 15, 411–419. doi: 10.1111/j.1365-3040.1992.tb00991.x
Du, B., Kang, H., Pumpanen, J., Zhu, P., Yin, S., Zou, Q., et al. (2014). Soil organiccarbon stock and chemical composition along an altitude gradient in the Lushan Mountain, subtropical China. Ecol. Res. 29, 433–439. doi: 10.1007/s11284-014-1135-4
Egli, M., Sartori, G., Mirabella, A., Favilli, F., Giaccai, D., and Delbos, E. (2009). Effect of northand south exposure on organic matter in high alpine soils. Geoderma 149, 124–136. doi: 10.1016/j.geoderma.2008.11.027
Enquist, B. J., Bentley, L. P., Shenkin, A., Maitner, B., Savage, V., Michaletz, S., et al. (2017). Assessingtrait-based scaling theory in tropical forests spanning a broad temperature gradient. Glob. Ecol. Biogeogr. 26, 1357–1373. doi: 10.1111/geb.12645
Evans, C. D., Jones, T. G., Burden, A., Ostle, N., Zielinski, P., Cooper, M. D., et al. (2012). Acidity controls on dissolved organic carbon mobility in organic soils. Glob. Change Biol. 18, 3317–3331. doi: 10.1111/j.1365-2486.2012.02794.x
Fatima, S., Hameed, M., Ahmad, F., Ahmad, M. S. A., Khalil, S., Munir, M., et al. (2022). Structural and functional responses in widespread distribution of some dominant grasses along climatic elevation gradients. Flora 289:152034. doi: 10.1016/j.flora.2022.152034
Freeman, B. G., Scholer, M. N., Ruiz-Gutierrez, V., and Fitzpatrick, J. W. (2018). Climatechange causes upslope shifts and mountaintop extirpations in a tropical bird community. Proc. Natl. Acad. Sci. U.S.A 115, 11982–11987. doi: 10.1073/pnas.1804224115
Fyllas, N. M., Gloor, E., Mercado, L. M., Sitch, S., Quesada, C. A., Domingues, T. F., et al. (2014). Analysing Amazonian Forest productivity using a new individual and trait-based model (TFS v. 1). Geosci. Model Dev. 7, 1251–1269. doi: 10.5194/gmd-7-1251-2014
Galiba, G. (1994). In-vitro adaptation for drought and cold hardiness in wheat. Plant Breeding Rev. 12, 115–162. doi: 10.1002/9780470650493.ch5
Göransson, H., Edwards, P. J., Perreijn, K., Smittenberg, R. H., and Venterink, H. O. (2014). Rocks create nitrogen hotspots and N: P heterogeneity by funnelling rain. Biogeochemistry 121, 329–338. doi: 10.1007/s11104-016-2821-5
Griffiths, R. P., Madritch, M. D., and Swanson, A. K. (2009). The effects of topography on forestsoil characteristics in the Oregon Cascade Mountains (USA): implications for the effects ofclimate change on soil properties. Forest Ecol. Manage. 257, 1–7. doi: 10.1016/j.foreco.2008.08.010
Guoju, X., Qiang, Z., Jiangtao, B., Fengju, Z., and Chengke, L. (2012). The relationship between winter temperature rise and soil fertility properties. Air Soil Water Res. 5:8599. doi: 10.4137/ASWR.S8599
Gupta, A. K., Negi, M., Nandy, S., Alatalo, J. M., Singh, V., and Pandey, R. (2019). Assessing thevulnerability of socio-environmental systems to climate change along an altitude gradient in theIndian Himalayas. Ecol. Indic. 106:105512. doi: 10.1016/j.ecolind.2019.105512
Hamid, M., Khuroo, A. A., Malik, A. H., Ahmad, R., and Singh, C. P. (2020a). Elevation and aspect determine the differences in soil properties and plant species diversity on Himalayan Mountain summits. Ecol. Res. 36, 340–352. doi: 10.1111/1440-1703.12202
Hamid, M., Khuroo, A. A., Malik, A. H., Ahmad, R., Singh, C. P., Dolezal, J., et al. (2020b). Early evidence of shifts in alpine summit vegetation: a case study from KashmirHimalaya. Front. Plant Sci. 11:421. doi: 10.3389/fpls.2020.00421
Han, Y. J., Gharibeshghi, A., Mewis, I., Foerster, N., Beck, W., and Ulrichs, C. (2020). Plant responses to ozone: effects of different ozone exposure durations on plant growth and biochemical quality of Brassica campestris L. spp. chinensis. Sci. Horticul. 262:108921. doi: 10.1016/j.scienta.2019.108921
Hanway, J. J., and Heidel, H. (1952). Soil analysis methods as used in Iowa State College Soil Testing Laboratory. Iowa Agric. 57, 187–193.
Hashim, A. M., Alharbi, B. M., Abdul Majeed, A. M., Awatif, M., Elkelish, A., Hozzein, W. N., et al. (2020). Oxidative stress responses of some endemic plants to high altitudes byintensifying antioxidants and secondary metabolites content. Plants 9:869. doi: 10.3390/plants9070869
Hassan, W. H., and Nile, B. K. (2021). Climate change and predicting future temperature in Iraqusing CanESM2 and HadCM3 modeling. Model. Earth Syst. Environ. 7, 737–748. doi: 10.1007/s40808-020-01034-y
Haynes, R. J., and Naidu, R. (1998). Influence of lime, fertilizer and manure applications on soilorganic matter content and soil physical conditions: a review. Nutr. Cycling Agroecosyst. 51, 123–137. doi: 10.1023/A:1009738307837
Hazrati, S., Tahmasebi-Sarvestani, Z., Modarres-Sanavy, S. A. M., Mokhtassi-Bidgoli, A., and Nicola, S. (2016). Effects of water stress and light intensity on chlorophyll fluorescence parameters and pigments of Aloe vera L. Plant Physiol. Biochem. 106, 141–148. doi: 10.1016/j.plaphy.2016.04.046
He, X., Hou, E., Liu, Y., and Wen, D. (2016). Altitudinal patterns and controls of plant and soilnutrient concentrations and stoichiometry in subtropical China. Sci. Rep. 6:24261. doi: 10.1038/srep24261
Hodkinson, I. D. (2005). Terrestrial insects along elevation gradients: species and community responses to altitude. Biol. Rev. Camb. Philos. Soc. 80, 489–513. doi: 10.1017/s1464793105006767
Hoogsteen, M. J., Lantinga, E. A., Bakker, E. J., Groot, J. C., and Tittonell, P. A. (2015). Estimatingsoil organic carbon through loss on ignition: effects of ignition conditions and structural waterloss. Eur. J. Soil Sci. 66, 320–328. doi: 10.1111/ejss.12224
Hou, X., Han, H., Tigabu, M., Cai, L., Meng, F., Liu, A., et al. (2019). Changes in soilphysicochemical properties following vegetation restoration mediate bacterial community composition and diversity in Changting. China. Ecol. Eng. 138, 171–179. doi: 10.1016/j.ecoleng.2019.07.031
Huang, D., Yu, L., Zhang, Y., and Zhao, X. (2011). Belowground biomass and its relationship toenvironmental factors of natural grassland on the northern slopes of the Qilian mountains. Acta Prataculturae Sinica. 20, 1–10.
Husson, F., Josse, J., Le, S., Mazet, J., and Husson, M. F. (2020). Package “FactoMineR”. Available online at: https://github.com/husson/FactoMineR
Idris, O. A., Wintola, O. A., and Afolayan, A. J. (2017). Phytochemical and antioxidant activitiesof Rumex crispus L. in treatment of gastrointestinal helminths in Eastern Cape Province, SouthAfrica. Asian Pac. J. Trop. Biomed. 7, 1071–1078. doi: 10.1016/j.apjtb.2017.10.008
Kandler, O., and Hopf, H. (1982). Oligo saccharides based on sucrose. Encycl. Plant Physiol. N. Ser. 13, 348–382.
Kassambara, A., and Mundt, F. (2020). Factoextra: Extract and visualize the results of multivariate data analyses (R package version 1.0.7).
Kewlani, P., Negi, V. S., Bhatt, I. D., Rawal, R. S., and Nandi, S. K. (2021). Soil nutrients concentration along altitudinal gradients in Indian Western Himalaya. Scand. J. For. Res. 36, 98–104. doi: 10.1080/02827581.2020.1871065
Kidanemariam, A., Gebrekidan, H., Mamo, T., and Kibret, K. (2012). Impact of altitude and land use type on some physical and chemical properties of acidic soils in Tsegede Highlands, Northern Ethiopia. Open J. Soil Sci. 2, 223–233. doi: 10.4236/ojss.2012.23027
Körner, C. (2007). The use of ‘altitude’ in ecological research. Trends Ecol. Evol. 22, 569–574. doi: 10.1016/j.tree.2007.09.006
Körner, C. (2012). Treelines will be understood once the functional difference between a tree and a shrub is. Ambio. 41, 197–206. doi: 10.1007/s13280-012-0313-2
Kumar, S., and Srivastava, N. (2002). Herbal research in Garhwal Himalayas: Retrospects & amp. Prospect. Ann. For. 10, 99–118.
Kumar, S., Suyal, D. C., Yadav, A., Shouche, Y., and Goel, R. (2019). Microbial diversity andsoil physicochemical characteristic of higher altitude. PLoS One. 14:e0213844. doi: 10.1371/journal.pone.0213844
Lenoir, J., Gégout, J. C., Marquet, P. A., de Ruffray, P., and Brisse, H. (2008). A significant upward shift in plant species optimum elevation during the 20th century. Science 320, 1768–1771. doi: 10.1126/science.1156831
Li, H., Guo, Q., Yang, L., Quan, H., and Wang, S. (2022). Seasonal eco-physiologycharacteristics of four evergreen rhododendron species to the subalpine habitats. Forests 13:653. doi: 10.3390/f13050653
Li, Q., Liu, G. B., Zhang, Z., Tuo, D. F., Bai, R. R., and Qiao, F. F. (2017). Relative contribution of root physical enlacing and biochemistrical exudates to soil erosion resistance in the Loesssoil. Catena 153, 61–65. doi: 10.1016/j.catena.2017.01.037
Li, T. X., Shen-Tu, X. L., Xu, L., Zhang, W. J., Duan, J. P., Song, Y. B., et al. (2022). Intraspecific and sex-dependent variation of leaf traits along altitude gradient in the endangered dioecious tree Taxus fuana Nan Li & RR Mill. Front. Plant Sci. 13:996750. doi: 10.3389/fpls.2022.996750
Liang, J., Reynolds, T., Wassie, A., Collins, C., and Wubalem, A. (2016). Effects of exoticEucalyptus spp. plantations on soil properties in and around sacred natural sites in the northernEthiopian highlands. AIMS Agric. Food 1, 175–193. doi: 10.3934/agrfood.2016.2.175
Liu, X. D., Zhao, W. J., Zhang, X. L., Jing, W. M., and Fan, L. M. (2013). Variation of soilnutrient content and pH value under Picea crassifolia forest in the Pailugou drainage basin in the Qilian mountains. Arid Zone Res. 30, 1013–1020.
Ma, L., Sun, X., Kong, X., Galvan, J. V., Li, X., Yang, S., et al. (2015). Physiological, biochemical and proteomics analysis reveals the adaptation strategies of the alpine plant Potentilla saundersiana at altitude gradient of the Northwestern Tibetan Plateau. J. Prot. 112, 63–82. doi: 10.1016/j.jprot.2014.08.009
Maclean, J. E., Mitchell, R. J., Burslem, D. F., Genney, D., Hall, J., and Pakeman, R. J. (2017). Understorey plant community composition reflects invasion history decades after invasive Rhododendron has been removed. J. Appl. Ecol. 55, 874–884. doi: 10.1111/1365-2664.12973
Magaña Ugarte, R., Escudero, A., and Gavilán, R. G. (2019). Metabolic and physiological responsesof Mediterranean high-mountain and alpine plants to combined abiotic stresses. Physiol. Plant 165, 403–412. doi: 10.1111/ppl.12898
Mahajan, S., and Tuteja, N. (2005). Cold, salinity, and drought Stresses: an overview. Arch. Biochem. Biophys. 444, 139–158. doi: 10.1002/9783527628964.ch7
Måren, I. E., Karki, S., Prajapati, C., Yadav, R. K., and Shrestha, B. B. (2015). Facing north orsouth: does slope aspect impact forest stand characteristics and soil properties in semiarid trans Himalayan valley? J. Arid Environ. 121, 112–123. doi: 10.1016/j.jaridenv.2015.06.004
Mathew, M. M., Majule, A. E., Marchant, R., and Sinclair, F. (2016). Variability of Soilmicronutrients concentration along the slopes of Mount Kilimanjaro, Tanzania. Appl. Environ. Soil Sci. 2016:9814316. doi: 10.1155/2016/9814316
Mayor, J. R., Sanders, N. J., Classen, A. T., Bardgett, R. D., Clement, J. C., Fajardo, A., et al. (2017). Elevation alters ecosystem properties across temperate treelinesglobally. Nature 542, 91–95. doi: 10.1038/nature21027
Mishra, G., and Francaviglia, R. (2021). Land uses, altitude and texture effects on soilparameters. A comparative study in two districts of Nagaland, northeast India. Agriculture 11:171. doi: 10.3390/agriculture11020171
Molina-Montenegro, M. A., Peñuelas, J., Munné-Bosch, S., and Sardans, J. S. (2012). Higher plasticity in ecophysiological traits enhances the performance and invasion success of Taraxacum officinale (dandelion) in alpine environments. Biol. Invasions 14, 21–33. doi: 10.1007/s10530-011-0055-2
Murtaza, K. O., and Romshoo, S. A. (2017). Recent glacier changes in the Kashmir alpine Himalayas, India. Geocarto Int. 32, 188–205. doi: 10.1080/10106049.2015.1132482
Nilsen, E. T. (1992). Thermonastic leaf movements: a synthesis of research with Rhododendron. Bot. J. Linn. Soc. 110, 205–233. doi: 10.1111/j.1095-8339.1992.tb00291.x
Northcott, M. L., Gooseff, M. N., Barrett, J. E., Zeglin, L. H., Takacs-Vesbach, C. D., and Humphrey, J. (2009). Hydrologic characteristics of lake and stream-side riparian margins in theMcMurdo Dry Valleys, Antarctica. Hydrol. Process. 23, 1255–1267. doi: 10.1002/hyp.7262
Olajire, A. A., and Azeez, L. (2011). Total antioxidant activity, phenolic, flavonoid and ascorbicacid contents of Nigerian vegetables. Afr. J. Food Sci. Technol. 2, 22–29.
Olsen, S. R., Cole, C. V., Watanabe, F. S., and Dean, L. A. (1954). Estimation of availablephosphorus in soils by extraction with sodium bicarbonate circular No. 939. Washington, DC: U.S.Department of Agriculture.
Paschalis, A., Fatichi, S., Katul, G. G., and Ivanov, V. Y. (2015). Cross-scale impact of climatetemporal variability on ecosystem water and carbon fluxes. J. Geophy. Res. Biogeosci. 120, 1716–1740. doi: 10.1002/2015JG003002
Peterson, B. G., and Carl, P. (2020). Performance analytics: econometric tools for performance and risk analysis. R package version 2.0.4.
Piao, S., Wang, X., Ciais, P., Zhu, B., Wang, T. A. O., and Liu, J. I. E. (2011). Changes insatellite-derived vegetation growth trend in temperate and boreal Eurasia from 1982 to 2006. Glob. Change Biol. 17, 3228–3239. doi: 10.1111/j.1365-2486.2011.02419.x
Pluess, A. R., Frank, A., Heiri, C., Lalagüe, H., Vendramin, G. G., and Oddou-Muratorio, S. (2016). Genome–environment association study suggests local adaptation to climate at the regional scale in Fagus sylvatica. N. Phytol. 210, 589–601. doi: 10.1111/nph.13809
Popescu, R., and Kopp, B. (2013). The genus Rhododendron: an ethnopharmacological and toxicological review. J. Ethnopharmacol. 147, 42–62. doi: 10.1016/j.jep.2013.02.022
Porporato, A., Daly, E., and Rodriguez-Iturbe, I. (2004). Soil water balance and ecosystem response to climate change. The Amer. Nat. 164, 625–632. doi: 10.1086/424970
Qasba, S., Masoodi, T. H., Bhat, S. J. A., Paray, P. A., Bhat, A., and Khanday, M. U. D. (2017). Effect of altitude and aspect on soil physicochemical characteristics in Shankaracharya Reserved Forest. Int. J. Pure Appl. Biosci. 5, 585–596.
Qi, X., Jia, J., Liu, H., and Lin, Z. (2019). Relative importance of climate change and humanactivities for vegetation changes on China & 39;s silk road economic belt over multipletimescales. Catena 180, 224–237. doi: 10.1016/j.catena.2019.04.027
R Core Team. (2020). R: a language and environment for statistical computing. Vienna: R Foundation for Statistical Computing.
Rada, F., García-Núñez, C., Boero, C., Gallardo, M., Hilal, M., González, J., et al. (2001). Low-temperature resistance in Polylepis tarapacana, a tree growing at the highest altitudes in the world. Plant Cell Environ. 24, 377–381. doi: 10.1046/j.1365-3040.2001.00685.x
Rapp, J. M., Silman, M. R., Clark, J. S., Girardin, C. A., Galiano, D., and Tito, R. (2012). Intra-and interspecific tree growth across a long altitudinal gradient in the Peruvian Andes. Ecology 93, 2061–2072. doi: 10.1890/11-1725.1
Rathore, N., Thakur, D., and Chawla, A. (2018). Seasonal variations coupled with elevationgradient drives significant changes in eco-physiological and biogeochemical traits of a high-altitude evergreen broadleaf shrub, Rhododendron anthopogon. Plant Physiol. Biochem. 132, 708–719. doi: 10.1016/j.plaphy.2018.08.009
Salinger, M. J., Shrestha, M. L., Ailikun Dong, W., McGregor, J. L., and Wang, S. (2014). “Climate in Asia and the Pacific: climate variability and change,” in Climate in Asia and the Pacific, Vol. 56, eds M. Manton and L. Stevenson (Dordrecht: Springer), doi: 10.1007/978-94-007-7338-7_2
Schob, C., Armas, C., Guler, M., Prieto, I., and Pugnaire, F. I. (2013). Variability in functional traits mediates plant interactions along stress gradients. J. Ecol. 101, 753–762. doi: 10.1111/1365-2745.12062
Shipley, B., De Bello, F., Cornelissen, J. H. C., Laliberté, E., Laughlin, D. C., and Reich, P. B. (2016). Reinforcing loose foundation stones in trait-based plant ecology. Oecologia 180, 923–931. doi: 10.1007/s00442-016-3549-x
Simon, A., Dhendup, K., Rai, P. B., and Gratzer, G. (2018). Soil carbon stocks along elevational gradients in Eastern Himalayan Mountain forests. Geoder. Reg. 12, 28–38. doi: 10.1016/j.geodrs.2017.11.004
Stöcklin, J., Kuss, P., and Pluess, A. R. (2009). Genetic diversity, phenotypic variation and local adaptation in the alpine landscape: case studies with alpine plant species. Bot. Helv. 119, 125–133. doi: 10.1007/s00035-009-0065-1
Sundqvist, M. K., Sanders, N. J., and Wardle, D. A. (2013). Community and ecosystem response to elevational gradients: processes, mechanisms, and insights for global change. Annu. Rev. Ecol. Evol. Syst. 44, 261–280. doi: 10.1146/annurev-ecolsys-110512-135750
Takahashi, K., and Matsuki, S. (2017). Morphological variations of the Solidago virgaurea L. complex along an elevational gradient on Mt Norikura, Central Japan. Plant Species Biol. 32, 238–246. doi: 10.1111/1442-1984.12148
Takashima, T., Hikosaka, K., and Hirose, T. (2004). Photosynthesis or persistence: nitrogen allocation in leaves of evergreen and deciduous Quercus species. Plant Cell Environ. 27, 1047–1054. doi: 10.1111/j.1365-3040.2004.01209.x
Taneda, H., and Noshiro, S. (2019). Altitudinal trends of efficiency and stability of water transport in the stems and leaves of Nepalese Rhododendron species, based on morphological traits. Rhododendr. Int. 3, 43–65.
Trujillo-González, J. M., Torres-Mora, M. A., Ballesta, R. J., and Brevik, E. C. (2022). Spatial variability of the physicochemical properties of acidic soils along an altitudinal gradient in Colombia. Environ. Earth Sci. 81:108. doi: 10.1007/s12665-022-10235-w
Tsui, C. C., Chen, Z. S., and Hsieh, C. F. (2004). Relationships between soil properties and slopeposition in a lowland rain forest of southern Taiwan. Geoderma 123, 131–142. doi: 10.1016/j.geoderma.2004.01.031
Visakorpi, K., Block, S., Pellissier, L., Levine, J. M., and Alexander, J. (2022). Eco-physiological and morphological traits explain alpine plant species' response to warming. Funct. Ecol. 37, 287–301. doi: 10.1111/1365-2435.14228
Walkley, A., and Black, I. A. (1934). An examination of the Degtjareff method for determining soil organic matter, and a proposed modification of the chromic acid titration method. Soil Sci. 37, 29–38. doi: 10.1097/00010694-193401000-00003
Wang, H., Prentice, I. C., Davis, T. W., Keenan, T. F., Wright, I. J., and Peng, C. (2017). Photosynthetic responses to altitude: an explanation based on optimality principles. N. Phytol. 213, 976–982. doi: 10.1111/nph.14332
Wang, W. J., Qiu, L., Zu, Y. G., Dong-Xue, S., Jing, A., Hong-Yan, W., et al. (2011). Changes in soil organic carbon, nitrogen, pH and bulk density with the development of larch (Larix gmelinii) plantations in China. Glob. Change Biol. 17, 2657–2676. doi: 10.1111/j.1365-2486.201102447.x
Wani, I. A., Khan, S., Verma, S., Al-Misned, F. A., Shafik, H. M., and El-Serehy, H. A. (2022). Predicting habitat suitability and niche dynamics of Dactylorhiza hatagirea and Rheum webbianum in the Himalaya under projected climate change. Sci. Rep. 12:13205. doi: 10.1038/s41598-022-16837-5
Wani, Z. A., and Pant, S. (2021). Betula utilis D. Don: an ecologically and economically important timberline species of himalayan region in jeopardy. Bot. Rev. 87, 377–391.
Wani, Z. A., Negi, V. S., Bhat, J. A., Satish, K. V., Kumar, A., Khan, S., et al. (2023). Elevation, aspect, and habitat heterogeneity determine plant diversity and compositional patterns in the Kashmir Himalaya. Front. For. Glob. Change 6:1019277. doi: 10.3389/ffgc.2023.1019277
Weiping, M., and Guangchuang, Y. (2020). Basic trendline: add trendline and confidence interval of basic regression models to plot. R package version 2.0.5.
Wen, H. Y., Zhao, H. L., and Fu, H. (2005). Effects of years for reclamation and enclosure yearson soil properties of degraded sandy grassland. Acta Pratacul. Sin. 14, 31–37.
Yang, Y., Wu, J., Zhao, S., Han, X., He, F., and Chen, C. (2018). Assessment of the responses of soil pore properties to combined soil structure amendments using X-ray computed tomography. Sci. Rep. 8:695. doi: 10.1038/s41598-017-18997-1
Yemm, E. W., and Willis, A. J. (1954). The estimation of carbohydrates in plant extracts by anthrone. Biochem. J. 57, 508–514. doi: 10.1042/bj0570508
Yuan, Z. Q., Jiang, X. J., Liu, G. J., Jin, H. J., Chen, J., and Wu, Q. B. (2019). Responses of soilorganic carbon and nutrient stocks to human-induced grassland degradation in a Tibetan alpinemeadow. Catena 178, 40–48. doi: 10.1016/j.catena2019.03.001
Zhang, S. B., Zhang, J. L., Slik, J. W. F., and Cao, K. F. (2012). Leaf element concentrations of terrestrial plants across China are influenced by taxonomy and the environment. Glob. Ecol. Biogeogr. 21, 809–818. doi: 10.1111/j.1466-8238.2011.00729.x
Zhang, Y., Ai, J., Sun, Q., Li, Z., Hou, L., Song, L., et al. (2021). Soil organic carbon and total nitrogen stocks as affected by vegetation types and altitude across the mountainous regions in the Yunnan Province, south-western China. Catena 196:104872. doi: 10.1016/j.catena.2020.104872
Zhou, J., Xia, B., Treves, D. S., Wu, L. Y., Marsh, T. L., O’Neill, R. V., et al. (2002). Spatial and resource factors influencing high microbial diversity insoil. Appl. Environ. Microbiol. 68, 326–334. doi: 10.1128/AEM.68.1.326-334.2002
Zhou, X., Chen, S., Wu, H., Yang, Y., and Xu, H. (2017). Biochemical and proteomics analyses of antioxidant enzymes reveal the potential stress tolerance in Rhododendron chrysanthum. Pall. Biol. Direct 12, 1–9. doi: 10.1186/s13062-017-0181-6
Keywords: Rhododendron anthopogon, eco-physiology, soil property, temperature, Kashmir Himalaya
Citation: Mangral ZA, Islam SU, Tariq L, Kaur S, Ahmad R, Malik AH, Goel S, Baishya R, Barik SK and Dar TUH (2023) Altitudinal gradient drives significant changes in soil physico-chemical and eco-physiological properties of Rhododendron anthopogon: a case study from Himalaya. Front. For. Glob. Change 6:1181299. doi: 10.3389/ffgc.2023.1181299
Received: 07 March 2023; Accepted: 10 April 2023;
Published: 28 April 2023.
Edited by:
Jahangeer A. Bhat, Fiji National University, FijiReviewed by:
Muhammad Majeed Majeed, University of Gujrat, PakistanRupesh Dhyani, Govind Ballabh Pant National Institute of Himalayan Environment and Sustainable Development, India
Copyright © 2023 Mangral, Islam, Tariq, Kaur, Ahmad, Malik, Goel, Baishya, Barik and Dar. This is an open-access article distributed under the terms of the Creative Commons Attribution License (CC BY). The use, distribution or reproduction in other forums is permitted, provided the original author(s) and the copyright owner(s) are credited and that the original publication in this journal is cited, in accordance with accepted academic practice. No use, distribution or reproduction is permitted which does not comply with these terms.
*Correspondence: Tanvir Ul Hassan Dar, dGFudmlydWxoYXNhbkBnbWFpbC5jb20=