- 1College of Forestry, Fujian Agriculture and Forestry University, Fuzhou, China
- 2Key Laboratory of Ecology and Resources Statistics of Fujian Colleges, Fuzhou, China
- 3Entomology and Nematology Department, University of Florida, Gainesville, FL, United States
- 4College of Finance, Fujian Jiangxia University, Fuzhou, Fujian, China
Cellulose and lignin are the principal constituents of plant litter, and their degradation plays an important role in the maintaining the soil carbon balance in terrestrial ecosystems. Yet, our knowledge of the responses of litter mass loss and cellulose and lignin degradation to litter quality, environmental factors, and taxonomic diversity indices along an elevational gradient in subtropical forests remains sparse. To gain insight into litter decomposition dynamics and its influencing factors, we investigated the cellulose and lignin degradation of foliar litter that from the two most dominant tree species along an elevational gradient (900–1,600 m) in a subtropical forest on Daiyun Mountain, China. Our results showed the following. (1) The degradation rate of cellulose was higher than that of lignin for litter collected at each elevation. The mass loss for foliar litter at different elevations was greater in the early period (0–180 days) than later period (180–270 days). (2) Litter quality, as well as environmental factors and taxonomic diversity indices, together influenced the foliar litter mass loss rate in addition to the rates of cellulose and lignin degradation. The key environmental factors included air and soil temperature, which had significant correlations with litter decomposition positively. The litter quality, namely is N (nitrogen) and P (phosphorus) contents, had significant positive correlations with degradation rates of cellulose and lignin in litter, and vice versa for lignin/N and C/N ratios. Some of the taxonomic diversity indices had positive correlations with litter decomposition, indicating that the input of highly diver litter material leads to a non-additive effect. (3) The litter decomposition was affected by the combination of litter quality, environmental factors, and taxonomic diversity indices. Of these, however, the environmental factors are the main factors that controlled the litter decomposition along elevational gradients in this subtropical forest. The results could provide a theoretical basis for understanding foliar litter nutrient release for the subtropical forest ecosystem of China.
1. Introduction
Plant litter decomposition is crucial for the cycling of soil organic matter in terrestrial ecosystems, and is influenced by litter quality, environmental factors, and taxonomic diversity indices (Yin et al., 2019; Hong et al., 2021). Cellulose and lignin are the primary components of leaf litter, accounting for over 50% of the carbon stored in plants (Bradford et al., 2016). Several studies have highlighted the importance of cellulose and lignin degradation in litter decomposition and nutrient cycling (Bradford et al., 2016; Yin et al., 2019; Yang et al., 2021). Berg and McClaugherty (2020) proposed a hypothesis that the rate of cellulose degradation increases in the later stages of litter decomposition due to the presence of lignin surrounding labile litter components in plant cell walls (Berg and McClaugherty, 2020). Additionally, microclimate conditions can impact litter decomposition, leading to further degradation of litter cellulose in response to environmental changes (He et al., 2019). However, climate warming can disrupt the original cellulose and lignin degradation processes during litter decomposition (Yin et al., 2019). Therefore, a comprehensive understanding of how cellulose and lignin respond to climate-induced degradation is crucial for better understanding soil carbon and nutrient cycling.
The initial quality of plant litter is determined by the plant species composition, which has a significant impact on the substrate’s quality (García-Palacios et al., 2016; Krishna and Mohan, 2017). Litter quality is a critical factor that regulates litter decomposition (Veen et al., 2015). Several studies have confirmed that litter quality is a reliable indicator for predicting nutrient release from subsequent litter (Cornwell et al., 2008; Bradford et al., 2014; Veen et al., 2015). Bokhorst et al. (2013) demonstrated that the initial quality of litter significantly affects the rate of litter mass loss. In another study, Yang et al. (2019) found that litter with higher initial nitrogen and phosphorus concentrations is more conducive to degradation. Additionally, the species composition of plants affects the degradation of cellulose in litter, which is negatively correlated with initial litter lignin and cellulose concentrations, as well as the C/N ratio (Zhang et al., 2016). Furthermore, the variability in microclimate conditions can affect cellulose and lignin degradation in litter more than litter quality (Aerts, 1997; He et al., 2019). Thus, it is essential to determine the relative contributions of litter quality and environmental factors to the degradation of cellulose and lignin in litter.
Litter decomposition is a crucial ecosystem process influenced by environmental factors (Gavazov, 2010; He et al., 2019). Elevation plays a crucial role in shaping ecosystem properties and processes in mountainous regions, providing a unique opportunity to study terrestrial ecosystems. Microclimate heterogeneity at different elevations (Suseela and Tharayil, 2018; Wang et al., 2021) can lead to various taxonomic diversity patterns, including a monotonous decline and an intermediate height expansion pattern (Lomolino, 2001). The latter suggests that taxonomic diversity at mid-elevation is higher than at high and low elevations (Lomolino, 2001). The environmental energy hypothesis states that species richness is mainly influenced by the direct control of energy on species’ physiological activities, while the hydrothermal dynamic hypothesis posits that taxonomic diversity on a large scale is explained by the combination of moisture and energy (Wang et al., 2009; Huang et al., 2017). Recent studies have shown that the taxonomic and phylogenetic diversity of tree communities follows an intermediate height expansion pattern along elevations in subtropical forests (Li et al., 2021). Furthermore, in areas with high taxonomic diversity, mixed litter decomposition is faster than single litter due to a mixed non-additive effect (Duan et al., 2013; Porre et al., 2020). However, little is known about litter decomposition dynamics as shaped by litter quality, environmental factors, and taxonomic diversity indices along elevational gradients in forests.
In this study, we investigated how litter quality, environmental factors, and taxonomic diversity indices affect litter decomposition along an elevation gradient of subtropical forest on Daiyun Mountain. This mountain is home to the largest Pinus taiwanensis Hayata community in southeastern China and has a clear hierarchical structure of forest vegetation, offering a suitable platform to explore how microclimate heterogeneity affects litter decomposition (Su et al., 2015). Previous studies have shown that coniferous litter generally decomposes more slowly than broad-leaved litter due to its higher concentration of cellulose and lignin. At elevations of 900–1,300 m, there is a mixed forest of coniferous and broad-leaved species, while at 1,400–1,600 m, the forest is predominantly coniferous. Studies by Xu et al. (2017) and Chen et al. (2022) have shown that temperature, rainfall, and the C, N, and P contents of the litter vary along the elevation gradient of Daiyun Mountain. However, the degradation of cellulose and lignin in litter in response to litter quality, environmental factors, and taxonomic diversity has not been studied for Daiyun Mountain. Our study addressed three main questions: (i) What are the patterns of litter decomposition, including its litter mass loss and cellulose and lignin degradation rates, along the elevational gradient? (ii) What are the correlations between litter decomposition and litter quality, environmental factors, and taxonomic diversity indices? (iii) What is the influence of litter quality, environmental factors and taxonomic diversity indices upon litter decomposition dynamics?
2. Materials and methods
2.1. Study site
Daiyun Mountain National Nature Reserve (25°38’∼25°43’N, 118°05’∼118°20’E) is located in Dehua County, Fujian Province, China. The reserve has a peak elevation of 1,856 m and a lowest elevation of 650 m. It is well-known for its biodiversity and serves as a transitional zone between the south and middle subtropical regions. The climate in the reserve is characterized by a subtropical marine monsoon, with an annual average temperature ranging from 15.6 to 19.5°C. The coldest month, January, has an average temperature of 6.5–10.5°C, while the hottest month, July, has an average temperature of 23–27.5°C. The annual average sunshine duration is 1875.4 h, and the reserve experiences 220 foggy days per year. The main soil type found in the reserve is ferric acrisols, as reported in previous studies (Xu et al., 2017; Jiang et al., 2020; Chen et al., 2022).
2.2. Sampling plots’ set-up
On the southern slope of Daiyun Mountain, we established an elevational gradient spanning from 900 to 1,600 m, with eight sampling locations at intervals of 100 m. Following the methodology of CTFS (Tropical Forestry Science Center; Condit, 1998), we set up fixed plots to sample the plant community at each elevation. Each sampling plot was 20 m × 30 m and divided into three 10 m × 20 m quadrats, selecting representative forest communities with well-defined forest patterns, and the general information of sample plots along elevations in Daiyun Mountain were refer to Li et al. (2021). We recorded the longitude, latitude, elevation, slope, aspect, and other topographic factors of each quadrat using a GPS unit [the detailed information were recorded in reference of Jiang et al. (2019)].
2.3. Data collection
We recorded species identity, diameter at breast height (DBH), and tree height information for all trees with a DBH greater than 1 cm in each sampling plot, using the China Flora database1 for species identification (Supplementary Table 1). To quantify taxonomic diversity and explore its correlation with litter decomposition, we selected four common indexes of species Margalef, Shannon-Wiener, Simpson, and Pielou, based on survey data of the arbor layer in the sample plots of the comprehensive observation site of typical forest ecosystems in China (Fang et al., 2004). According to the survey data of arbor layer in the sample plots of the comprehensive observation site of typical forest ecosystems in China, four common indexes of species Margalef, Shannon-Wiener, Simpson and Pielou (Supplementary Table 4) were selected to use to quantify taxonomic diversity and explore their correlation with litter decomposition in this study (Fang et al., 2004). Margalef index: This index measures the richness of species in a community relative to the number of individuals present. It calculates the species diversity as the natural logarithm of the number of species divided by the total number of individuals in a community. The higher the value of Margalef index, the more diverse the community is. Shannon-Wiener index: This index measures the diversity and evenness of species in a community. It calculates the diversity as the negative sum of the product of the natural logarithm of the proportion of each species and the proportion itself. The higher the value of Shannon-Wiener index, the more diverse and even the community is. Simpson index: This index measures the dominance of species in a community. It calculates the diversity as the inverse sum of the squared proportion of each species. The higher the value of Simpson index, the more dominated the community is by a few species. Pielou index: This index measures the evenness of species in a community relative to the maximum possible evenness. It calculates the evenness as the diversity divided by the natural logarithm of the number of species in a community. The higher the value of Pielou index, the more even the community is Jost (2006); Gamito (2010).
Based on the survey results, we selected the two most important tree species to conduct litter decomposition experiments, and three pairs of each of these species were used in each quadrat (see Supplementary Table 1). During the peak of autumn defoliation in 2018, we collected fresh fallen leaves that showed clear signs of aging but had not yet decomposed. We weighed 40 g of litter (20 g of leaves of each dominant species) for each decomposition period and placed them into a nylon decomposition bag (20 cm × 20 cm) with 0.1 mm holes on both sides. We used the residual amount of each sample to determine the initial litter quality. At each elevation, we placed 36 bags, for a total of 288 bags. On March 5, 2019, we randomly distributed the litter decomposition bags in the sampling plot, separating them by more than 2 cm and distributing them up and down the slope. We connected the bags of each square with a rope and fixed them with nails. The bags remained in place for the duration of the recovery time from June 5, 2019 to March 7, 2020, with sampling intervals of approximately 90, 180, 270, and 360 days for measuring decomposition. At each time interval, we randomly retrieved three bags from each site, shook off any dirt, fine roots, and impurities, and placed them in a sterilized, sealed polyethylene bag. We transported the samples to the laboratory in sealed bags. Then, we washed away impurities such as soil with pure water, picked out any visible roots of plants, to avoid losing tiny pieces of organic material, we used a series of sieves with progressively smaller mesh size. First, we used a larger mesh size to remove larger pieces of organic material and soil. Then, used a smaller mesh size to remove smaller particles. Repeat this process with progressively smaller mesh sizes until we have removed as much impurities as possible without losing the tiny pieces of organic material that we want to keep, and we have been modified in the paper, and oven-dried the bag content at 70°C to a constant weight, then weighed and recorded the dry weight to calculate the litter decomposition rate.
For the soil sampling, we first removed fallen leaves from the litter layer at the sampling point. We used an auger to collect soil samples from 0 to 20 cm depth at each point, and homogenized the samples to form a composite soil sample for each soil layer in each plot at each elevation. The soil and litter samples were transported to the laboratory and dried at 70°C until reaching a constant weight for further analysis. Litter cellulose concentration was determined with a cellulose kit from Comin (CLL-1-Y, SuZhou, China)2; The litter lignin concentration was determined with a lignin kit, also from Comin (MZS-1-G, SuZhou, China; see text footnote 2). Air temperature data were collected using an American MAXIM iButton recorder (MAXIM Integrated, iButton, San Jose, CA, USA) (model DS1923-F5) at the center of each elevation’s sample point, placed at a height of approximately 1.5 m from the soil surface. Soil temperature data were collected using an iButton temperature recorder (DS1922L-F50) buried 10 cm below the ground surface. Temperature data were automatically recorded every 2 h from 12:00 a.m. onward on a daily basis, and the experimental average values for each month were used as the soil or air temperature data for each of the eight elevations forming the gradient. Total carbon (TC) and total nitrogen (TN) were measured using a nitrogen analyzer (VARIOMAX CN, Elementar, Hanau, Germany) as a proxy for litter quality, and total phosphorus (TP) was measured using an inductively coupled plasma emission spectrometer (PE OPTIMA 8000, PerkinElmer, Waltham, MA, USA). The soil C, N, and P data are presented in Supplementary Table 2, and details regarding the litter quality results can be found in Supplementary Table 3.
2.4. Data analysis
The calculation formula used for the litter decomposition rate (Mr) was as follows: Mr = (M0–Mt)/M0/T × 100%, where M0 is the initial dry mass sample (g); Mt is the litter dry mass (g) at time T, with T being the number of elapsed litter decomposition days. The calculation formula for litter cellulose or lignin degradation rate is as follows: E = [(C0 × M0–Ct × Mt)/C0 × M0] × 100, where E is the degradation rate of material (%); Ct is the concentration of cellulose or lignin (g/kg) in the litter at time T; C0 is the initial concentration of litter (g/kg); M0 and Mt are the same as above. And exponential models for 50% (T50%) and 95% (T95%) litter decomposing time referred to Olson models (Olson, 1963).
Prior to analysis, normality and linearity checks were performed on the data, which included cellulose and lignin concentrations, litter quality, environmental factors, and taxonomic diversity indices corresponding to each period. We used one-way analysis of variance (ANOVA) to analyze the litter mass loss, cellulose and lignin concentration and degradation rate at different elevations in different time periods. Duncan’s test was used for multiple comparisons. For a general linear mixed model (GLMM), Y = Xβ + Zμ + e, where Y represents the measured value vector of the response variable, X is the design matrix of the fixed effect independent variable, β is the fixed effect parameter vector corresponding to X, Z is the design matrix of the random effect independent variable, μ is the fixed effect parameter vector corresponding to Z, and e is the vector matrix of the residual. The homogeneity of the variances we used a Levene’s Test, and the residuals for normality with a Shapiro test. We used the GLMM to test how elevation and time factors (fixed factors) affected litter mass loss, and litter cellulose and lignin degradation rates and their concentrations. Block was set as a random factor, with M = 3 blocks. Tukey’s honestly significant difference (HSD) was used to explore differences between means when the results were significant at P < 0.05. P-values were calculated using the “lmerTest” package in R v4.1.03 (R Core team, 2020) and random factor significance are presented in Supplementary Table 5. Pearson correlation analysis was performed using the “corrplot” package in R to examine the relationship between data collected on the 90th, 180th, 270th, and 360th day of field sampling.
The Structural Equation Model (SEM) is a powerful statistical approach for examining the relationships among multiple variables, based on their correlation coefficients or covariance matrix. In light of the fact that plant litter decomposition can be influenced by litter quality (e.g., litter TC, litter TN, litter TP, litter C/N, and litter lignin/N), environmental factors (e.g., air temperature, soil temperature, soil water content, slope, and soil pH), and taxonomic diversity (e.g., Margalef, Simpson, Shannon-Wiener, and Pielou indices) (Veen et al., 2015; García-Palacios et al., 2016), we constructed an SEM that incorporated these potential variables. We assessed the collinearity of all driving factors to eliminate any collinear relationships and removed factors with VIF > 10. VIF value between litter decomposition factors are presented in Supplementary Table 6. Subsequently, we established an initial SEM model based on known knowledge and relationships among the main driving factors. The initial SEM model was then parameterized using natural logarithm transformed and standardized data to assess its goodness of fit using the Chi-square (χ2) test, goodness-of-fit index (GFI), comparative fit indexes (CFI), and standardized root mean square residual (SRMR), in order to determine the optimal model for our study (Grace et al., 2016). The fit indices yielded a χ2 test insignificant statistic (P > 0.05), and GFI, CFI, and SRMR values that ranged from 0 to 1. Higher values indicated better model fit, with values >0.9 considered acceptable (Bentler, 1999; Shipley, 2000). The SEM structure was implemented using the “lavaan” package in R (Rosseel, 2012).
3. Results
3.1. Litter mass loss at different elevations
The GLMM analysis showed that litter mass loss was significantly influenced by elevation, time, and their interaction (Figure 1). Generally, the rate of litter mass loss decreased over time at different elevations (900–1,600 m), with the highest litter mass loss occurring at 900 m after 90 and 180 days of decomposition. At 180 days, the litter mass loss at elevations of 1,200 and 1,300 m was higher than that at 1,100 m and 1400–1600 m, and at 270 days, the litter mass loss at 1,300 m was higher than at other elevations (Figure 1). From the four time periods at each elevation, the litter mass loss was higher in the first 0–180 days than the 180–360 days of decomposition at each elevation (Figure 1). The correlation coefficient R2 of litter decomposition at different elevations indicated that the Olson index model fits the decomposition of leaves (Table 1). The decomposition time for 50% and 95% at 1,200 m was lower than that at other elevations, except for 900 m. Additionally, the decomposition time for 50 and 95% at 1,300 m was lower than that at 1,100 m and 1,400–1,600 m.
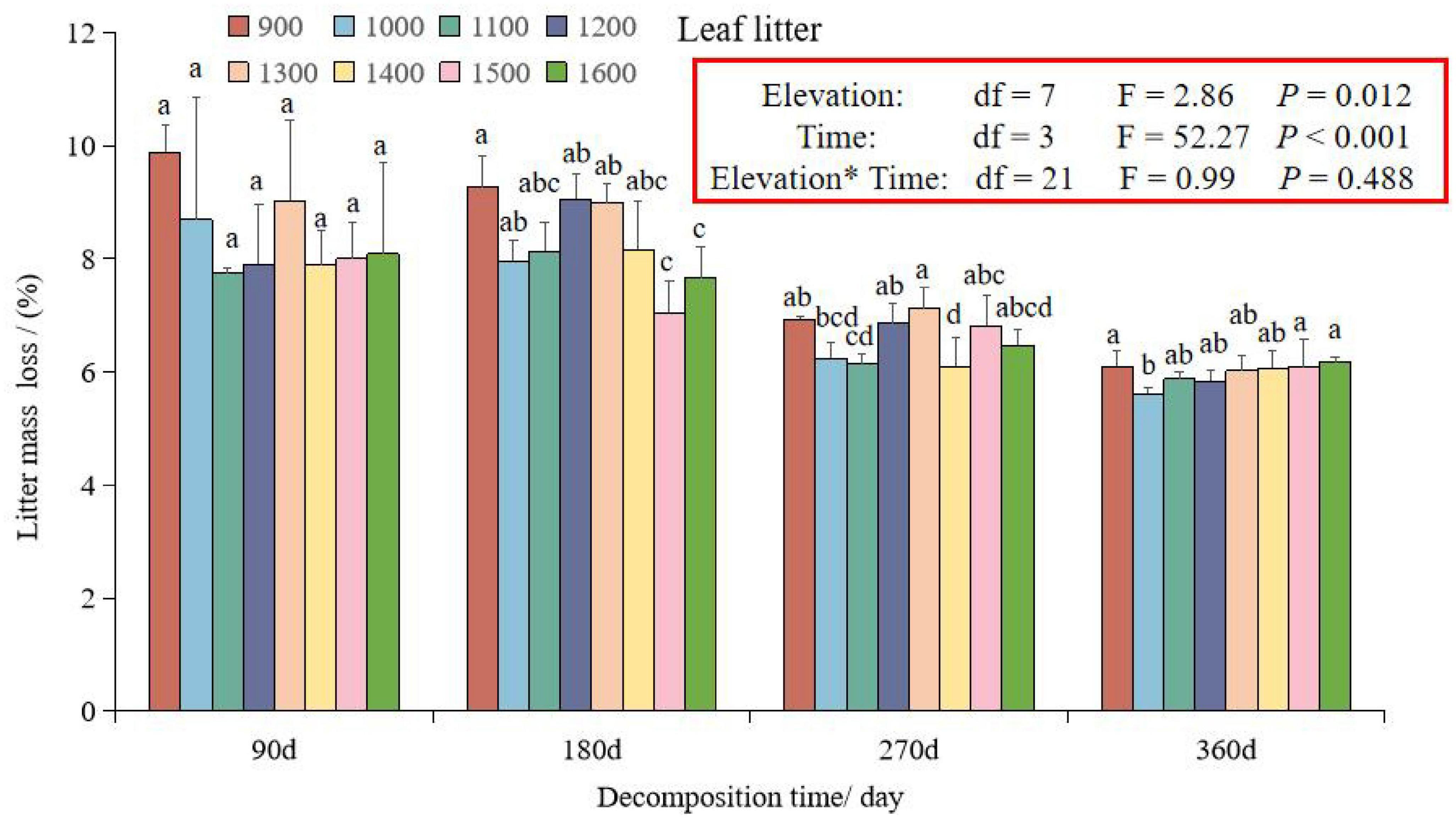
Figure 1. Dynamics of mass loss of foliar litter at different elevations along Daiyun Mountain. Each bar represents the mean litter mass loss of different elevation (900–1,600 m) in that incubation site (N = 3). Sample size, N = 96, F-values from the effects of elevation, time and interactions of litter mass loss in a general linear mixed model (GLMM). Different lowercase letters indicate the difference among different elevations during the same decomposition period at a significance level of 0.05.
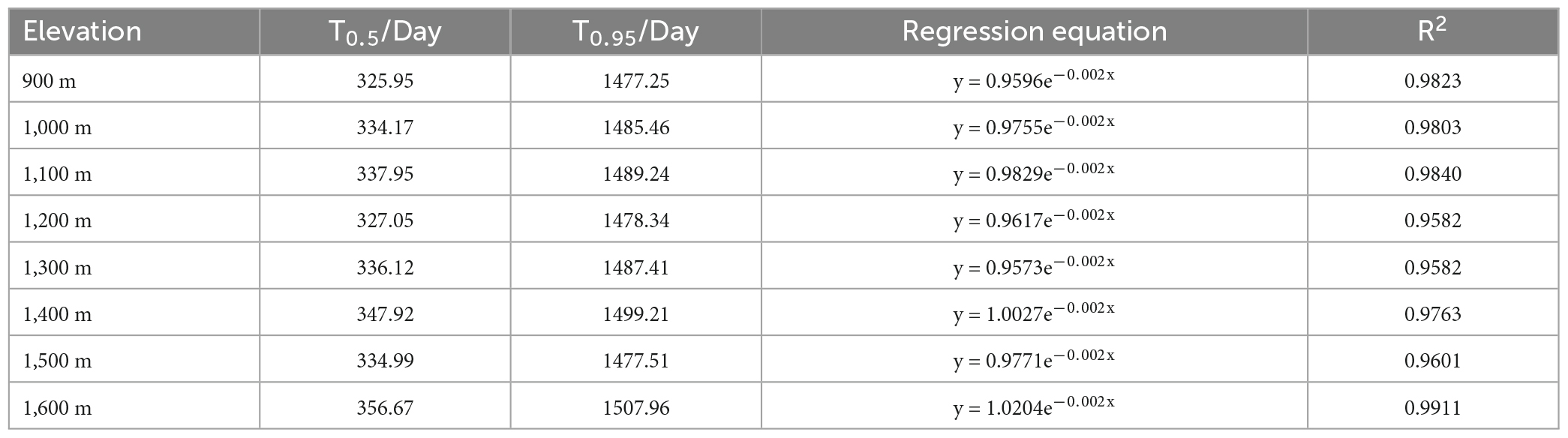
Table 1. Regression equation of residual mass in the process of litter decomposition in Daiyun Mountain.
3.2. Litter cellulose and lignin concentration at different elevations
The concentrations of cellulose and lignin in litter were significantly affected by elevation, time, and their interaction, and both decreased over time at different elevations (Figures 2A, B). The average cellulose content in litter increased from 900 to 1,400 m at 0, 180, and 270 days. However, at 90 and 360 days, the cellulose concentration at low elevations was relatively lower than that at high elevations (Figure 2A). The cellulose concentration at different elevations showed a net release state at 360 days elevation 90, 180, and 360 days (Figure 2B). The lignin content of 1,300–1,600 m was higher than that of 900–1,200 m during the experimental stage. Additionally, it showed a net release state at 360 days (Figure 2B).
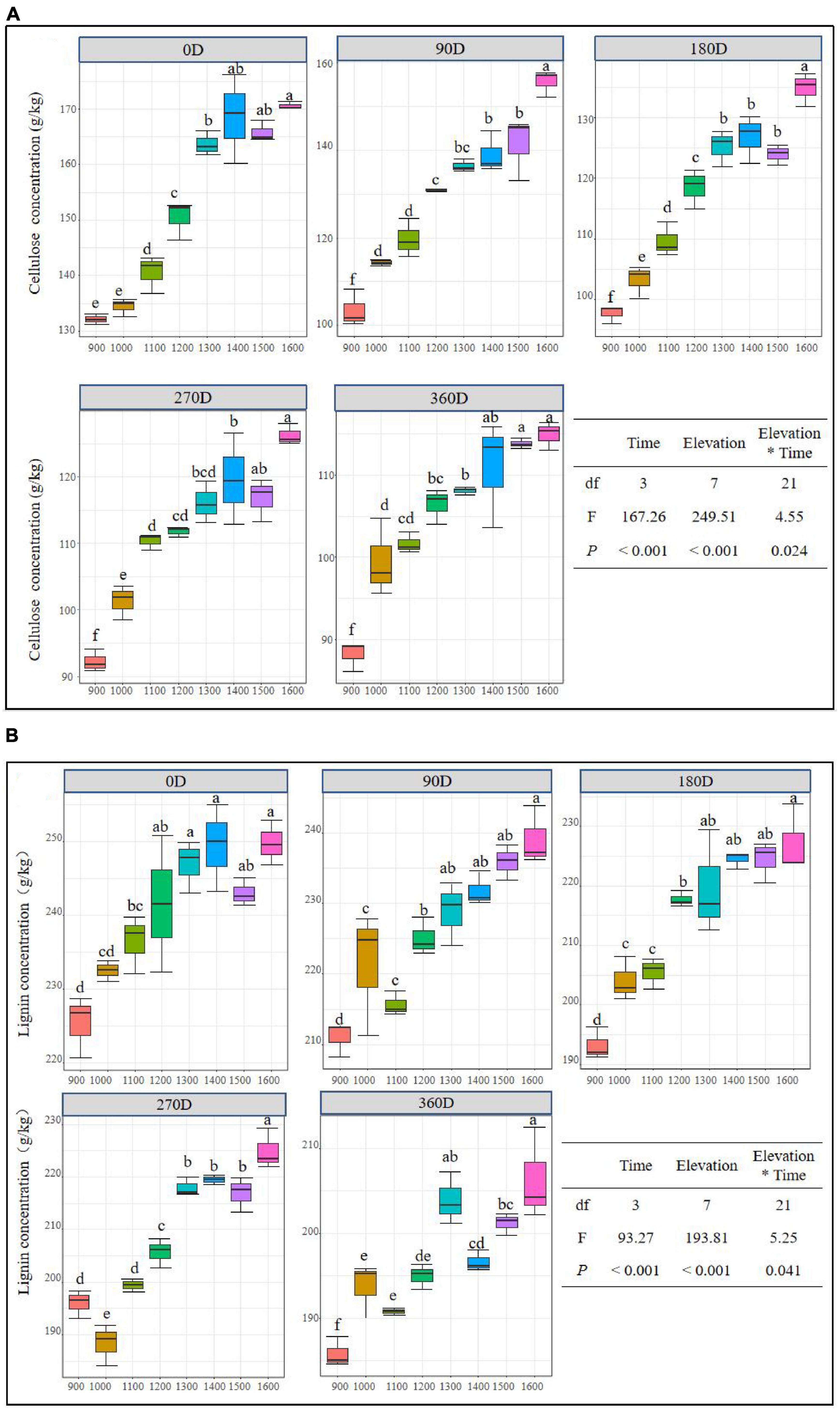
Figure 2. Litter cellulose (A) and lignin (B) concentration in different decomposition stages at different elevations along Daiyun Mountain. Each box represents the mean litter cellulose and lignin concentration of different elevation (900–1,600 m) in that incubation site (N = 3). Sample size, N = 120, F-values from the effects of elevation, time and interactions of litter mass loss in a general linear mixed model (GLMM). Different lowercase letters indicate the difference among different elevations during the same decomposition period at a significance level of 0.05.
3.3. Litter cellulose and lignin degradation rate at different elevations
The degradation rates of cellulose and lignin were significantly influenced by elevation, time, and their interaction (Figures 3A, B). The cellulose degradation rate of litter at the 900 m elevation was higher than at other elevations at 90, 180, and 360 days, and at 270 days, the cellulose degradation rate at 1,300 m was higher than other elevations (Figure 3A). The litter lignin degradation rate of 1,500–1,600 m was lower than at other elevations, but at 90 and 180 days, the rate was higher at 900 m than at other elevations (Figure 3B). Generally, the cellulose and lignin degradation rates were faster at lower elevations than at higher elevations. During the experimental stage, the cellulose degradation rate continued to increase at different elevations. Moreover, the rate of cellulose degradation was higher than that of lignin at the same elevation and during the same time periods throughout the year-long litter decomposition process (Figures 3A, B).
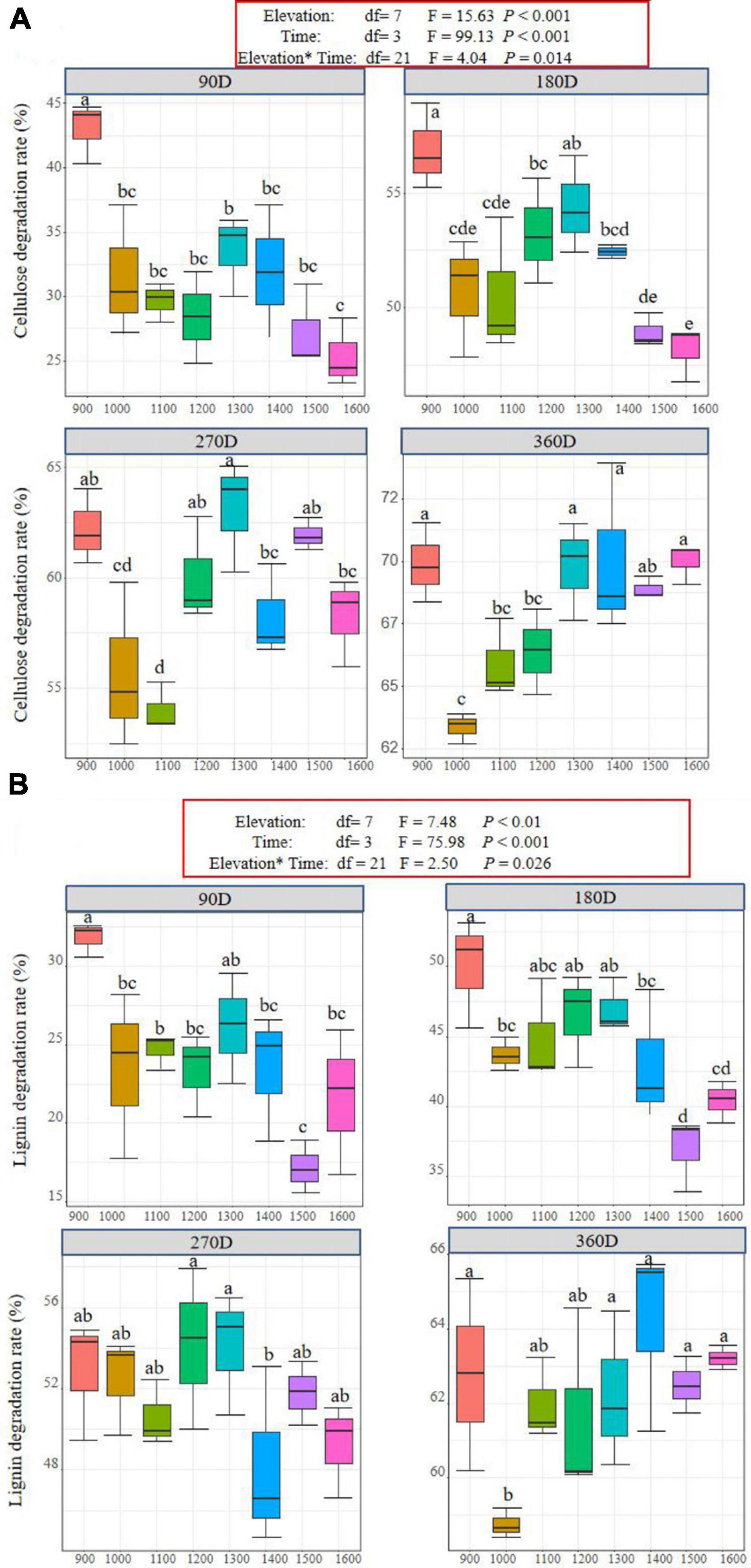
Figure 3. Litter cellulose (A) and lignin (B) degradation rate in different decomposition stages at different elevations along Daiyun Mountain. Each box represents the mean litter cellulose and lignin degradation rate of different elevation (900–1,600 m) in that incubation site (N = 3). Sample size, N = 96, F-values from the effects of elevation, time and interactions of litter mass loss in a general linear mixed model (GLMM). Different lowercase letters indicate the difference among different elevations during the same decomposition period at a significance level of 0.05.
3.4. Correlations between litter decomposition and litter quality, environmental factors, and taxonomic diversity indices
These correlation results are presented in Table 2. Litter samples’ cellulose and lignin degradation rates were significantly correlated with litter quality including both litter TN and TP (positive, P < 0.05). Litter decomposition (litter mass loss, lignin degradation rate, and cellulose degradation rate) was significantly correlated with litter quality expressed as by the litter C/N ratio and lignin/N ratio (negative, P < 0.05).
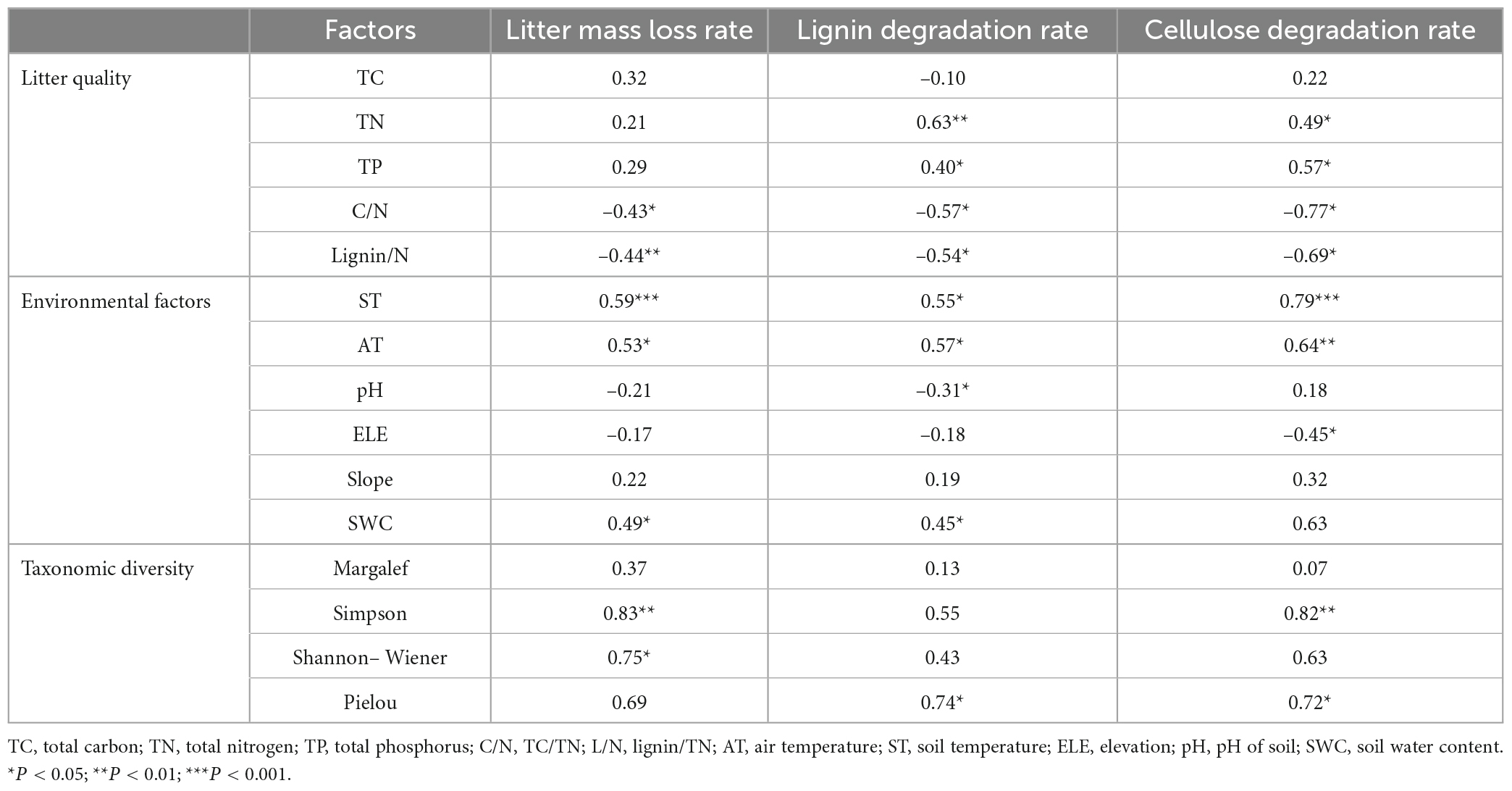
Table 2. Correlation between litter degradation rate and litter quality, environmental factors and taxonomic diversity indices.
Litter mass loss and lignin degradation rate were each significantly correlated with environmental factors, including soil temperature, air temperature, and soil water content (positive, P < 0.05). Litter mass loss and lignin degradation rate were also significantly correlated with soil pH, but negatively (P < 0.05). Litter cellulose degradation rate was significantly correlated with environmental factors including both soil and air temperature (positive, P < 0.05). Litter cellulose degradation rate was significantly correlated with elevation (negative, P < 0.05).
Litter mass loss was correlated with the taxonomic diversity indices, significantly so for the Simpson and Shannon–Wiener indices significantly (positive, P < 0.05). Litter cellulose degradation rate was correlated with Simpson index significantly (positive, P < 0.01). Litter lignin degradation rate was correlated with Pielou index significantly (positive, P < 0.05).
3.5. Interpretation of litter quality, environmental factors, and taxonomic diversity indices with respect to litter decomposition
The latent variables of litter quality, environmental factors, and taxonomic diversity indices all had a positive impact on litter mass loss and both cellulose and lignin degradation rates (Figures 4–6). The influence coefficients of litter mass loss, cellulose and lignin degradation rates as follows: environmental factors > litter quality > taxonomic diversity indices. Litter TN and TP, as well as air and soil temperatures, Shannon- Wiener and Simpson indices had indirect effects on litter mass loss and degradation of lignin positively (Figures 4, 6). Litter TN and TP, as well as air and soil temperatures, Shannon- Wiener and Simpson indices also had indirect effects on litter degradation of cellulose positively, but litter TC had indirect effects on litter degradation of cellulose negatively (Figure 5).
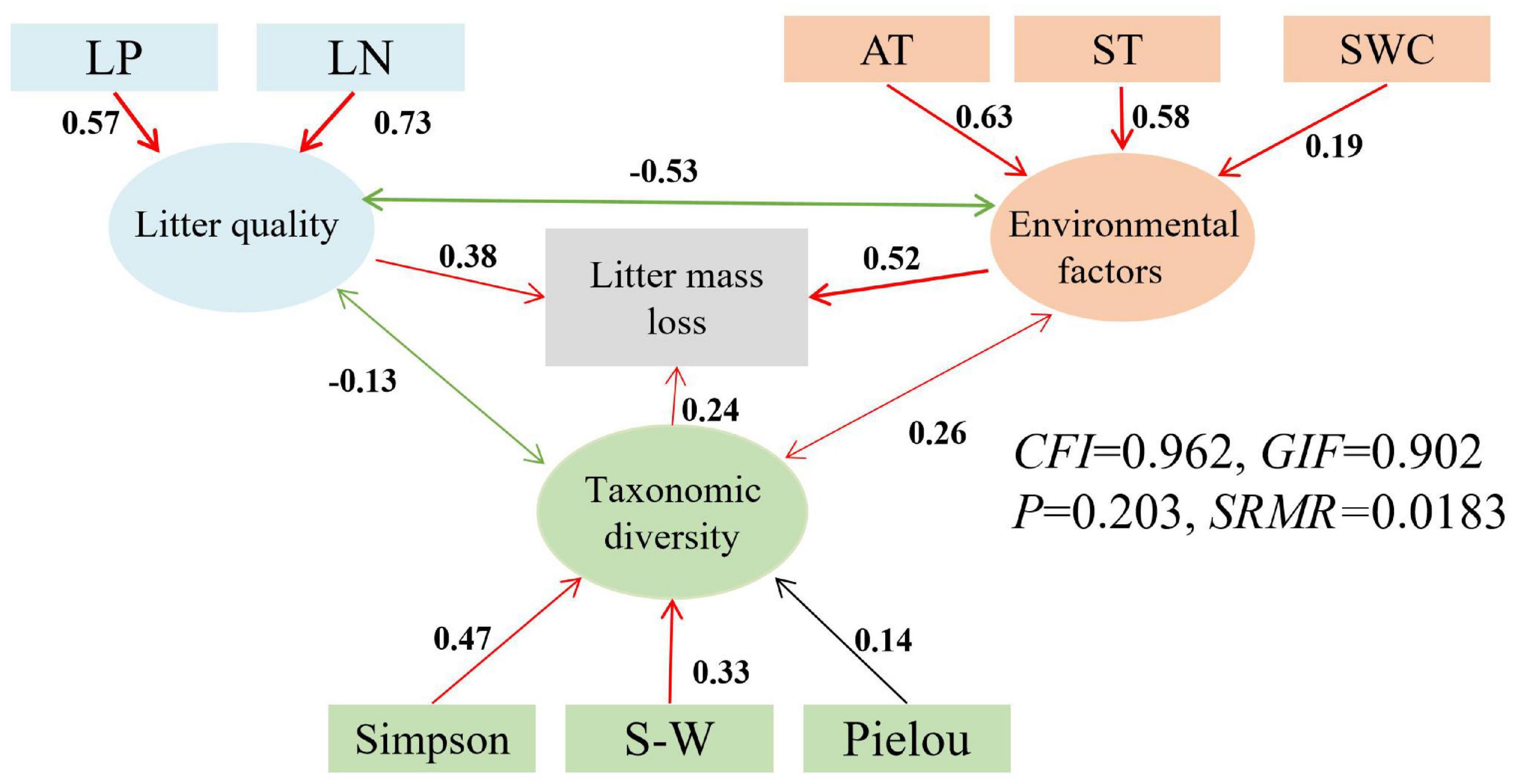
Figure 4. Structural equation models (SEMs) for the direct and indirect relationships between environmental factors (air and soil temperature, soil water content), litter quality (N, P), taxonomic diversity (Shannon-Wiener, Simpson and Pielou indices) and litter mass loss. AT, air temperature; ST, soil temperature; SWC, soil water content; LN, initial total nitrogen of litter; LP, initial total phosphorus of litter; SW, Shannon- Wiener, red represents positive correlation, green represents negative correlation, black represents non-correlation.
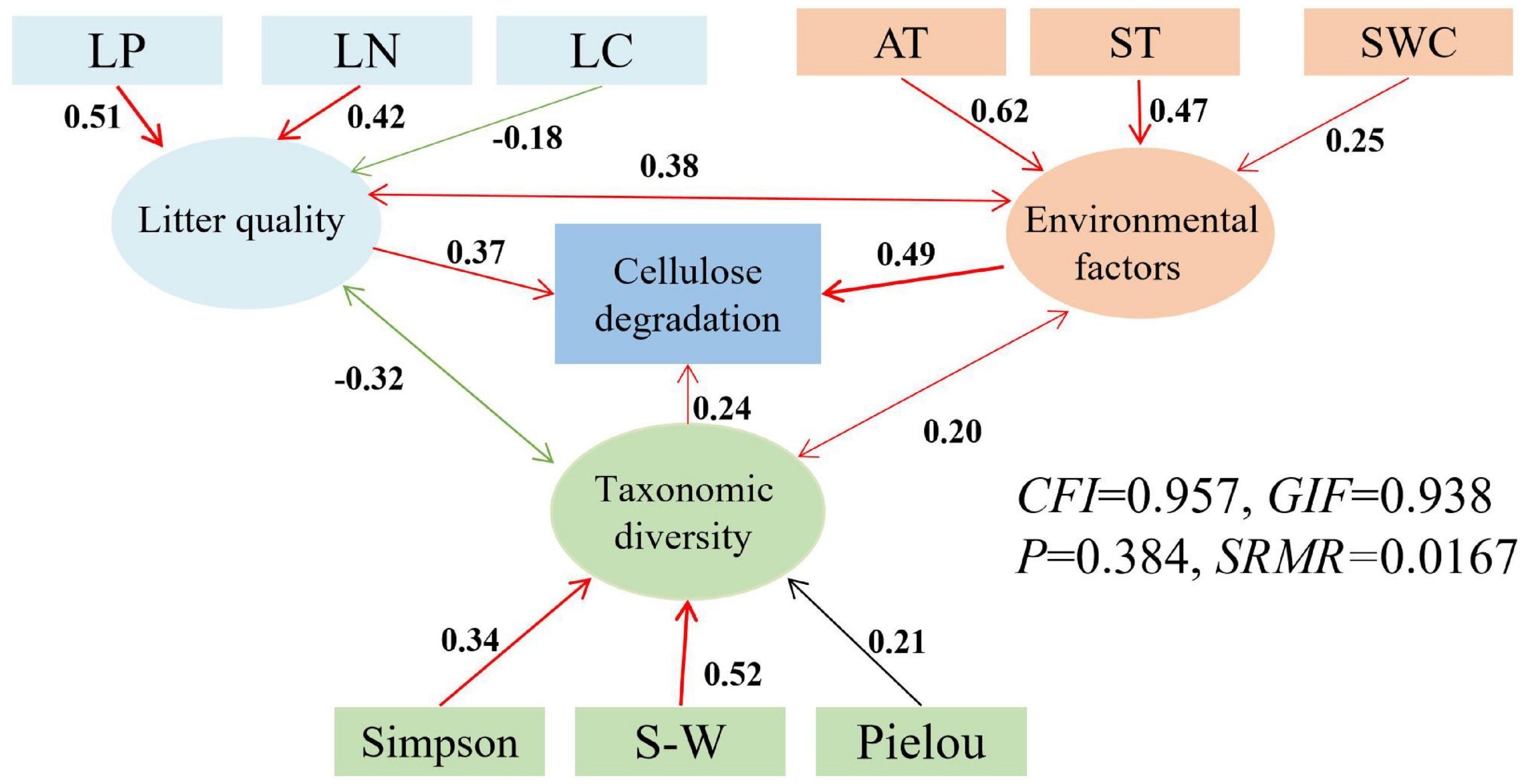
Figure 5. Structural equation models (SEMs) for the direct and indirect relationships between environmental factors (air and soil temperature, and soil water content), litter quality (C, N, P), taxonomic diversity (Shannon-Wiener, Simpson and Pielou indices) and litter cellulose degradation. AT, air temperature; ST, soil temperature; SWC, soil water content; LN, initial total nitrogen of litter; LP, initial total phosphorus of litter; SW, Shannon- Wiener, red represents positive correlation, green represents negative correlation, black represents non-correlation.
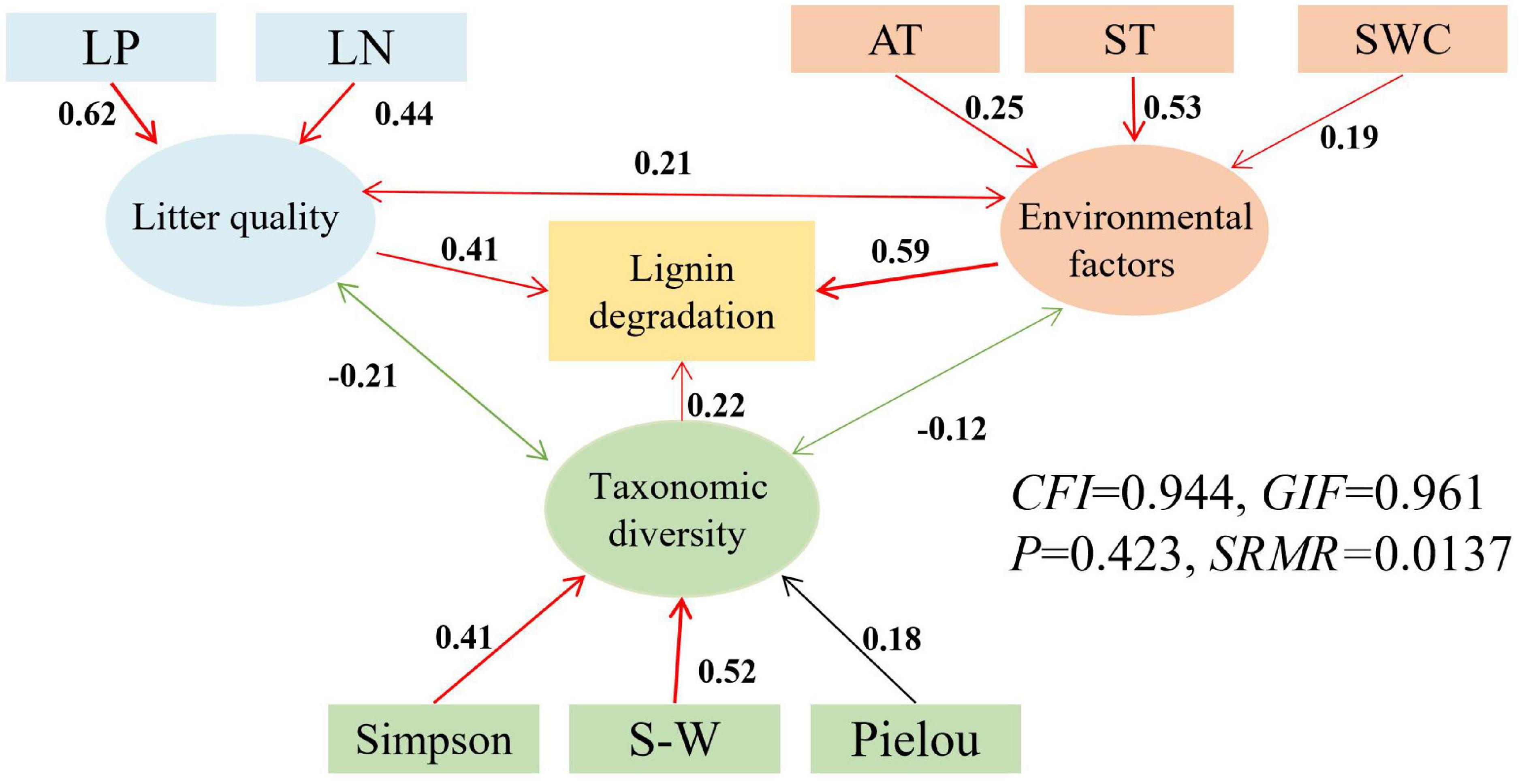
Figure 6. Structural equation models (SEMs) for the direct and indirect relationships between environmental factors (air and soil temperature and soil water content), litter quality (N, P), taxonomic diversity (Shannon-Wiener, Simpson and Pielou indices) and litter lignin degradation. AT, air temperature; ST, soil temperature; SWC, soil water content; LN, initial total nitrogen of litter; LP, initial total phosphorus of litter; SW, Shannon- Wiener, red represents positive correlation, green represents negative correlation, black represents non-correlation.
4. Discussion
4.1. Analysis of the decomposition rate of litter and its cellulose and lignin concentrations and degradation rates at different elevations
The litter mass loss on Daiyun Mountain showed a decreasing trend with time at different elevations (900–1,600 m) (Figure 1). The mass loss is a cumulative value, meaning that as decomposition time increases, more litter is lost, resulting in a higher loss rate. The decomposition rate in the first two time periods (0–180 days) was generally faster than in the latter two time periods (180–360 days) at the same elevation and different periods (Figure 1). This result is consistent with previous studies (Wang et al., 2021) on litter decomposition in other subtropical regions. At the initial decomposition stage, soluble components in the litter are leached out, resulting in a higher decomposition rate. As decomposition progresses, recalcitrant substances become relatively more abundant, and the decomposition rate becomes controlled by microorganisms. Seasonal factors may also play a role in the observed trend. For instance, the first two sampling times were in June and September, when temperatures and rainfall were high, and microbial activity correspondingly increased, resulting in a higher decomposition rate. The last two sampling times were in December and March, during the winter when temperatures were lower, and microbial activity decreased, resulting in a slower decomposition rate. Therefore, the litter decomposition rate in the first two time periods was generally higher than that in the latter two time periods.
The concentrations of cellulose and lignin in litter at different elevations decrease over time, as shown in Figures 2A, B. At 90 days, the concentrations of cellulose and lignin at 900 m were lower than those at 1,100 m or other elevations. This trend continued until 360 days, where the concentrations of cellulose and lignin in litter at 900 m were still lower than those at 1,100 m or other elevations. These results suggest that the release of litter cellulose and lignin concentrations is faster at low elevations than at high elevations, which is consistent with the findings of studies on forest litter decomposition and nutrient dynamics in different regions of Canada (Moore et al., 2011). This may be because the concentrations of cellulose and lignin in the later stage of litter decomposition depend on the initial amounts of these compounds in the litter (Ma et al., 2019).
During the experimental stage, the cellulose degradation rate at different elevations continued to increase in Daiyun Mountain (Figure 3A). This result is in line with findings of He et al. (2019), who studied the degradation characteristics of cellulose and lignin during the decomposition process of dominant species in Three Gorges Reservoir, and of Fioretto et al. (2005), who studied cellulose and lignin degradation characteristics in three types of litter pools in the Mediterranean ecosystem. This result supports the hypothesis of Berg and McClaugherty (2020) that labile components in plant cell walls are surrounded by lignin (Berg and McClaugherty, 2020). Additionally, cellulose degradation rates were higher than lignin degradation rates at the same elevation and during the same decomposition period (Figures 2A, B), consistent with the findings of Ma et al. (2015, 2019). Soil microorganisms prefer to decompose cellulose and lignin, and their digestion and excretion in the intestines change the fungal and bacterial community structure in the leaf residue and increase the cellulase activity, affecting the degradation (Ma et al., 2019). Furthermore, cellulose leaching plays a role in the greater degradation rate of cellulose than lignin due to the stronger leaching effect of cellulose, aided by the high annual precipitation and relative humidity of Daiyun Mountain (Fioretto et al., 2005; Brandt et al., 2010).
4.2. Analysis of correlations among litter quality, environmental factors, taxonomic diversity indices, and litter decomposition
The higher the plant taxonomic diversity, the more litter types that can be easily mixed and non-additive litter (Duan et al., 2013). Significant correlations were found between mass loss and taxonomic diversity according to the Simpson and Shannon–Wiener indices (positive, P < 0.05, Table 2). At 90 days, the litter mass loss was higher at 1,300 m than either 1,000–1,200 or 1,400–1,600 m, while at the 180 and 270 days, the litter mass loss at 1,200–1,300 m surpassed those at 1,000–1,100 and 1,400–1,600 m (Figure 1). Both 1,200 and 1,300 m correspond to the middle elevation of Daiyun Mountain. Li et al. (2021) observed an intermediate height expansion pattern of taxonomic diversity along the elevation gradient in Daiyun Mountain, with the central region exhibiting high taxonomic diversity. Our results (Table 2) indicate that the decomposition time of 50 and 95% in 1,200 m is lower than that at other elevations, except for 900 m, and the decomposition time of 50 and 95% in 1,300 m is lower than that at 1,100 m and 1,400–1,600 m, suggesting that mixed decomposition of litter may exert non-additive effects (Duan et al., 2013). The positive correlation between taxonomic diversity and the litter decomposition rate suggests that the decomposition of litter is related to the elevation gradient pattern of biodiversity. The intermediate expansion pattern of taxonomic diversity in the middle elevation may have a certain non-additive effect on litter decomposition, which is consistent with the findings of Ricardo et al. (2019), who studied the effect of taxonomic diversity on litter decomposition in southern Brazil. They found that high taxonomic diversity leads to more types of litter input, enhancing the litter’s mixed non-additive effects. Additionally, the cumulative yield of litter is relatively higher with greater taxonomic diversity, and the ground cover layer formed by litter can increase the litter temperature at the bottom and hasten litter decomposition. Furthermore, Duan et al. (2013) found that taxonomic diversity is an important factor promoting the non-additive effect of litter, which further suggests that litter at the middle elevation in Daiyun Mountain may generate a non-additive effect on litter decomposition.
During the initial stage of decomposition, the chemical characteristics of litter can be affected by its initial carbon (C), nitrogen (N), and phosphorus (P) concentrations, as well as other chemical components (Berg and Meentemeyer, 2002; Alhamd et al., 2004; Yang and Jin, 2009). Our results (Table 2) show that the degradation rates of cellulose and lignin were significantly positively correlated with the initial total N (TN) and total P (TP) concentrations of litter (P < 0.05). This is consistent with the findings of Zhang et al. (2016), who observed that the initial chemical composition of subtropical litter, particularly high concentrations of N and P, can strongly influence the decomposition process. Furthermore, higher concentrations of N and P can stimulate the metabolic activity of microorganisms and enhance their reproductive rates. Therefore, an adequate supply of carbon substrates, N, and P is crucial to support a diverse and abundant microbial community during litter decomposition.
The initial C/N and lignin/N ratios of litter can influence its decomposition rate (Polyakova and Billor, 2007). High C/N and lignin/N ratios are unfavorable for decomposition (Halvorson et al., 2016). Our results showed a significant negative correlation between litter mass loss and C/N (P < 0.05, Table 2). Similarly, the degradation rates of cellulose and lignin were significantly correlated with C/N (negative, P < 0.05), which is consistent with previous studies (Polyakova and Billor, 2007; Halvorson et al., 2016). A high initial C/N ratio of litter hinders its decomposition, resulting in slow nutrient release. We also found significant negative correlations between litter mass loss, cellulose and lignin degradation rates, and lignin/N (P < 0.01), in agreement with Mendonca and Stott (2003), who studied the relationship between nutrient release rate, C/N, and initial lignin/N of litter. Lignin/N is a good predictor of litter decomposition because lignin is an acid-insoluble C component that is difficult to decompose and tends to accumulate in the litter’s later stages, resulting in slower decomposition rates (Herman et al., 2008; Hall et al., 2020).
The degradation rate of litter lignin was found to be significantly correlated with air and soil temperatures as well as soil water content (positive, P < 0.05, Table 2). This result can be attributed to the fact that the study area is situated in the humid subtropical region of China, where microbial activity dominates the degradation of litter lignin. Higher air and soil temperatures and higher soil water content provide an environment conducive to the proliferation of soil microorganisms, enabling them to produce specific extracellular enzymes to degrade lignin (Bugg et al., 2011). Moreover, we found that the litter lignin degradation rate was significantly correlated with soil pH (negative, P < 0.05) and TN (positive, P < 0.05). This may be due to the fact that microbial reproduction requires a large amount of energy in the form of nitrogen. Additionally, lower pH levels are more conducive to microbial attachment, and when combined with suitable temperature and sufficient water content, they can accelerate the consumption and decomposition of litter by various microorganisms.
4.3. Structural equation models (SEMs) between litter quality, environmental factors, taxonomic diversity, and litter decomposition
The SEMs for direct and indirect relationships between influencing factors and litter decomposition (Figures 4–6) revealed that the latter is affected by a combination of litter quality, environmental factors, and taxonomic diversity indices. Moreover, the influence coefficients of litter mass loss, cellulose, and lignin degradation rates always decreased in the following order: litter quality, environmental factors, and taxonomic diversity indices. This pattern is consistent with Joly et al. (2017), who studied taxonomic diversity’s impact on litter decomposition in Europe. Due to the significant changes in lignin and cellulose during the later stages of litter decomposition, environmental factors may exhibit greater sensitivity toward them compared to other factors (Hall et al., 2020). The late stage of litter decomposition is when the degradation of its components (lignin, cellulose, tannin, etc.) mainly occurs. Substances are slowly decomposed into simple small molecules under the action of soil enzyme activities and microbial metabolism, and the degree of degradation mainly depends on their interaction with environmental factors. For example, an increase in temperature enables the extracellular enzymes that degrade cellulose and lignin to maintain higher activity levels, thereby accelerating the degradation of both cellulose and lignin. Work by Tan et al. (2020) and Fravolini et al. (2018) also showed that the degradation process of litter cellulose and lignin is particularly sensitive to temperature. This is because when the temperature rises, it promotes the mineralization of soil nutrients and increases the activity of microorganisms, thereby accelerating the rates of cellulose and lignin degradation. In addition, soil and air temperatures have indirect positive effects on litter mass loss, and the degradation results for cellulose and lignin confirm this conclusion. Based on the correlation results, there was a significant positive correlation between air temperature and soil temperature, as well as between the litter mass loss, lignin degradation rate, and cellulose degradation rate (P < 0.05). Furthermore, there was a significant positive correlation between soil water content and the litter mass loss and lignin degradation rate (P < 0.05). Combined with the results of the structural equation model, temperature and soil water content were found to have positive effects on litter. This suggests that temperature and soil water content are the primary driving factors of litter decomposition in Daiyun Mountain.
5. Conclusion
Litter mass loss showed a downward trend during the yearlong process of decomposition examined, being greater in the early stage (0–180 days) than in late stage (180–360 days) along different elevations in the subtropical forest of Daiyun Mountain. The decomposition pattern of litter cellulose degradation rate supported the hypothesis of Berg and McClaugherty (2020), in that the degradation rate of cellulose was higher than that of lignin in litter at the same elevation in the same period of litter decomposition. The promotion of litter decomposition is facilitated by air and soil temperature, soil water content, and low soil pH, with temperature and water content being the primary drivers of the process. Litter with initially high N and P concentrations could promote the litter cellulose and lignin degradation, while the high initial litter C/N and lignin/N could constrain both cellulose and lignin degradation. Combined with the intermediate expansion pattern of taxonomic diversity found for Daiyun Mountain, the decomposition of litter was in line with the hypothesis of mixed non-additiveness of litter, indicating that a corresponding relationship exists between the elevation gradient pattern of taxonomic biodiversity and litter decomposition dynamics. Litter decomposition at different elevations is driven by the comprehensive combination of litter quality, environmental factors and taxonomic diversity indices, and environmental factors together have a great impact on net litter decomposition. Overall, our findings gained an insight to the driving factors of litter decomposition in subtropical forest along an elevational gradient.
Data availability statement
The original contributions presented in this study are included in the article/Supplementary material, further inquiries can be directed to the corresponding author.
Author contributions
BC: conceptualization and methodology. BC and YH: software. YY: validation. JZ: formal analysis. LJ and KK: investigation. DX and ZH: data curation. BC and ZH: writing—original draft preparation. LC: writing—review and editing. JL: funding acquisition. All authors read and agreed to the published version of the manuscript.
Funding
This research was funded by the National Natural Science Foundation of China (NSFC), grant numbers: 31700550 and 31770678, Science and Technology Promotion of Project Forestry Bureau of Fujian Province, grant number: 2022FKJ11, and Special Fund Project for Science and Technology Innovation of Fujian Agriculture and Forestry University, grant number: KFb22030XA.
Acknowledgments
We wish to express our thanks for the support received from the Daiyun Mountain Nature Reserve in Dehua City, Fujian Province to allow us to collect samples. We thank Cong Xing, Xinguang Gu, Yangdi Li, and Wenwei Chen in the field work. This work is part of the BEST (Biodiversity along Elevational Gradients: Shifts and Transitions) research network (https://best-mountains.org). We also thank all the BEST research network researchers for their great assistance with data analysis, review, and editing of the manuscript. We also record sincere appreciation for helpful and constructive comments made by reviewers of the draft manuscript.
Conflict of interest
The authors declare that the research was conducted in the absence of any commercial or financial relationships that could be construed as a potential conflict of interest.
Publisher’s note
All claims expressed in this article are solely those of the authors and do not necessarily represent those of their affiliated organizations, or those of the publisher, the editors and the reviewers. Any product that may be evaluated in this article, or claim that may be made by its manufacturer, is not guaranteed or endorsed by the publisher.
Supplementary material
The Supplementary Material for this article can be found online at: https://www.frontiersin.org/articles/10.3389/ffgc.2023.1134598/full#supplementary-material
Footnotes
References
Aerts, R. (1997). Climate, leaf litter chemistry and leaf-litter decomposition in terrestrial ecosystems—a triangular relationship. Oikos 79, 439–449. doi: 10.2307/3546886
Alhamd, L., Arakaki, S., and Hagihara, A. (2004). Decomposition of leaf litter of four tree species in a subtropical evergreen broad-leaved forest, Okinawa Island, Japan. For. Ecol. Manage. 202, 1–11. doi: 10.1016/j.foreco.2004.02.062
Bentler, P. M. (1999). Cutoff criteria for fit indexes in covariance structure analysis: Conventional criteria versus new alternatives. Struct. Equ. Model. 6, 1–55. doi: 10.1080/10705519909540118
Berg, B., and McClaugherty, C. (2020). Plant litter. decomposition, humus formation, carbon sequestration, 4th Edn. New York, NY: Springer. doi: 10.1007/978-3-030-59631-6
Berg, B., and Meentemeyer, V. (2002). Litter quality in a north European transect versus carbon storage potential. Plant Soil 242, 83–92. doi: 10.1023/A:1019637807021
Bokhorst, S., Metcalfe, D. B., and Wardle, D. A. (2013). Reduction in snow depth negatively affects decomposers but impact on decomposition rates is substrate dependent. Soil Biol. Biochem. 62, 157–164. doi: 10.1016/j.soilbio.2013.03.016
Bradford, M. A., Berg, B., Maynard, D. S., Wieder, W. R., and Wood, S. A. (2016). Understanding the dominant controls on litter decomposition. J. Ecol. 104, 229–238. doi: 10.1111/1365-2745.12507
Bradford, M. A., Warren, R. J., Baldrian, P., Crowther, T. W., Maynard, D. S., Oldfield, E. E., et al. (2014). Climate fails to predict wood decomposition at regional scales. Nat. Clim. Change 4, 625–630. doi: 10.1038/nclimate2251
Brandt, L. A., King, J. Y., Hobbie, S. E., Milchunas, D. G., and Sinsabaugh, R. L. (2010). The role of photodegradation in surface litter decomposition across a grassland ecosystem precipitation gradient. Ecosystems 13, 765–781. doi: 10.1007/s10021-010-9353-2
Bugg, T. D., Ahmad, M., Hardiman, E. M., and Singh, R. (2011). The emerging role for bacteria in lignin degradation and bio-product formation. Curr. Opin. Biotechnol. 22, 394–400. doi: 10.1016/j.copbio.2010.10.009
Chen, B., Chen, L., Jiang, L., Zhu, J., Chen, J., Huang, Q., et al. (2022). C:N:P Stoichiometry of Plant, Litter and Soil along an Elevational Gradient in Subtropical Forests of China. Forests 13:372. doi: 10.3390/f13030372
Cornwell, W. K., Cornelissen, J. H. C., Amatangelo, K., Dorrepaal, E., Eviner, V. T., Godoy, O., et al. (2008). Plant species traits are the predominant control on litter decomposition rates within biomes worldwide. Ecol. Lett. 11, 1065–1071. doi: 10.1111/j.1461-0248.2008.01219.x
Duan, J. C., Wang, S. P., Zhang, Z. H., Xu, G. P., Luo, C. Y., Chang, X. F., et al. (2013). Non-additive effect of species diversity and temperature sensitivity of mixed litter decomposition in the alpine meadow on Tibetan Plateau. Soil Biol. Biochem. 57, 841–847. doi: 10.1016/j.soilbio.2012.08.009
Fang, J. Y., Shen, Z. H., Tang, Z. Y., and Wang, Z. H. (2004). The Protocol for the Survey Plan for Plant Species Diversity of China’s Mountains. Biodivers. Sci. 12, 5–9. doi: 10.17520/biods.2004002
Fioretto, A., Nardo, C. D., Papa, S., and Fuggi, A. (2005). Lignin and cellulose degradation and nitrogen dynamics during decomposition of three leaf litter species in a Mediterranean ecosystem. Soil Biol. Biochem. 37, 1083–1091. doi: 10.1016/j.soilbio.2004.11.007
Fravolini, G., Tognetti, R., Lombardid, F., Eglie, M., Ascher-Jenullf, J., Arfaiolig, P., et al. (2018). Quantifying decay progression of deadwood in Mediterranean mountain forests. For. Ecol. Manage. 408, 228–237. doi: 10.1016/j.foreco.2017.10.031
Gamito, S. (2010). Caution is needed when applying Margalef diversity index. Ecol. Indic. 10, 550–551. doi: 10.1016/j.ecolind.2009.07.006
García-Palacios, P., McKie, B., Handa, I. T., Frainer, A., and Httenschwiler, S. (2016). The importance of litter traits and decomposers for litter decomposition, a comparison of aquatic and terrestrial ecosystems within and across biomes. Funct. Ecol. 30, 819–829. doi: 10.1111/1365-2435.12589
Gavazov, K. S. (2010). Dynamics of alpine plant litter decomposition in a changing climate. Plant Soil 337, 19–32. doi: 10.1007/s11104-010-0477-0
Grace, J. B., Anderson, T. M., Seabloom, E. W., Borer, E. T., Adler, P. B., and Harpole, W. S. (2016). Integrative modelling reveals mechanisms linking productivity and plant species richness. Nature 529, 390–393. doi: 10.1038/nature16524
Hall, S. J., Huang, W., Timokhin, V. I., and Hammel, K. E. (2020). Lignin lags, leads, or limits the decomposition of litter and soil organic carbon. Ecology 101:e03113. doi: 10.1002/ecy.3113
Halvorson, H. M., Scott, E. E., Entrekin, S. A., Evans, W. M. A., and Scott, J. T. (2016). Light and dissolved phosphorus interactively affect microbial metabolism, stoichiometry and decomposition of leaf litter. Freshw. Biol. 61, 1006–1019. doi: 10.1111/fwb.12763
He, W., Ma, Z. Y., Pei, J., Teng, M., Zeng, L. X., Yan, Z. G., et al. (2019). Effects of predominant tree species mixing on lignin and cellulose degradation during leaf litter decomposition in the Three Gorges Reservoir, China. Forests 10, 360–360. doi: 10.3390/f10040360
Herman, J., Moorhead, D., and Berg, B. (2008). The relationship between rates of lignin and cellulose decay in aboveground forest litter. Soil Biol. Biochem. 40, 2620–2626. doi: 10.1016/j.soilbio.2008.07.003
Hong, J. T., Lu, X. Y., Ma, X. X., and Wang, X. D. (2021). Five-year study on the effects of warming and plant litter quality on litter decomposition rate in a Tibetan alpine grassland. Sci. Total Environ. 750:142306. doi: 10.1016/j.scitotenv.2020.142306
Huang, S. Z., Huang, Q., Leng, G. Y., Zhao, M. L., and Meng, E. H. (2017). Variations in annual water-energy balance and their correlations with vegetation and soil moisture dynamics: a case study in the Wei River Basin, China. J. Hydrol. 546, 515–525. doi: 10.1016/j.jhydrol.2016.12.060
Jiang, L., He, Z. S., Gu, X. G., Liu, J. F., and Liu, Y. H. (2020). Classification and ordination of the Pinus taiwanensis forest on Daiyun Mountain. Fujian Province, China. Taiwania 65, 119–128.
Jiang, L., He, Z. S., Liu, J. F., Xing, C., Gu, X. G., Wei, C. S., et al. (2019). Elevation gradient altered soil C, N, and P stoichiometry of Pinus taiwanensis forest on Daiyun Mountain. Forests 10:1089. doi: 10.3390/f10121089
Joly, F. X., Milcu, A., Scherer-Lorenzen, M., Jean, L. K., Bussotti, F., Dawud, S. M., et al. (2017). Tree species diversity affects decomposition through modified micro-environmental conditions across European forests. New Phytol. 214, 1281–1293. doi: 10.1111/nph.14452
Krishna, M. P., and Mohan, M. (2017). Litter decomposition in forest ecosystems: a review. Energy Ecol. Environ. 2, 236–249. doi: 10.1007/s40974-017-0064-9
Li, M. J., He, Z. S., Jiang, L., Gu, X. G., Jin, M. R., Chen, B., et al. (2021). Distribution pattern and driving factors of species diversity and phylogenetic diversity along altitudinal gradient on the south slope of Daiyun Mountain. Acta Ecol. Sin. 41, 1148–1157. doi: 10.5846/stxb201910082091
Lomolino, M. (2001). Elevation gradients of species-density, historical and prospective views. Glob. Ecol. Biogeogr. 10, 3–13. doi: 10.1046/j.1466-822x.2001.00229.x
Ma, C., Yin, X. Q., Kou, X. C., Wang, Z. H., Li, X. Q., Jiang, Y. F., et al. (2019). Effects of Soil Fauna on Cellulose and Lignin Decomposition of Plant Litter in the Changbai Mountain, China. Environ. Entomol. 48, 592–602. doi: 10.1093/ee/nvz035
Ma, Z. L., Gao, S., Yang, W. Q., and Wu, F. Z. (2015). Degradation characteristics of lignin and cellulose of foliar litter at different rainy stages in subtropical evergreen broadleaved forest. Chin. J. Ecol. 34, 122–129.
Mendonca, E. S., and Stott, D. E. (2003). Characteristics and decomposition rates of pruning residues from a shaded coffee system in Southeastern Brazil. Agrofor. Syst. 57, 117–125. doi: 10.1023/A:1023900822261
Moore, T. R., Trofymow, J. A., Prescott, C. E., and Titus, B. D. (2011). Nature and nurture in the dynamics of C, N and P during litter decomposition in Canadian forests. Plant Soil 339, 163–175. doi: 10.1007/s11104-010-0563-3
Olson, J. S. (1963). Energy storage and the balance of producers and decomposers in ecological systems. Ecology 44, 322–331. doi: 10.2307/1932179
Polyakova, O., and Billor, N. (2007). Impact of deciduous tree species on litterfall quality, decomposition rates and nutrient circulation in pine stands. For. Ecol. Manage. 253, 11–18. doi: 10.1016/j.foreco.2007.06.049
Porre, R. J., Werf, W. V. D., Deyn, G. B. D., Stomph, T. J., and Hoffland, E. (2020). Is litter decomposition enhanced in species mixtures? A meta-analysis. Soil Biol. Biochem. 145:107791. doi: 10.1016/j.soilbio.2020.107791
R Core team (2020). R: A Language and Environment for Statistical Computing. Vienna: R Foundation for Statistical Computing.
Ricardo, A. C. O., Renato, M., and Márcia, C. M. M. (2019). Plant diversity and local environmental conditions indirectly affect litter decomposition in a tropical forest - Science Direct. Appl. Soil Ecol. 134, 45–53. doi: 10.1016/j.apsoil.2018.09.016
Rosseel, Y. (2012). lavaan: An R package for structural equation modeling. J. Stat. Softw. 48, 1–36. doi: 10.18637/jss.v048.i02
Shipley, B. (2000). A New Inferential Test for Path Models Based on Directed Acyclic Graphs. Struct. Equ. Model. 7, 206–218. doi: 10.1207/S15328007SEM0702_4
Su, S. J., Liu, J. F., He, Z. S., Zheng, S. Q., Hong, W., and Xu, D. W. (2015). Ecological species groups and interspecific association of dominant tree species in Daiyun Mountain National Nature Reserve. J. Mountain Sci. 12, 637–646. doi: 10.1007/s11629-013-2935-7
Suseela, V., and Tharayil, N. (2018). Decoupling the direct and indirect effects of climate on plant litter decomposition: Accounting for stress-induced modifications in plant chemistry. Glob. Change Biol. 24, 1428–1451. doi: 10.1111/gcb.13923
Tan, B., Yin, B., Yang, W. Q., Zhang, J., Xu, Z. F., Liu, Y., et al. (2020). Soil fauna show different degradation patterns of lignin and cellulose along an elevational gradient. Appl. Soil Ecol. 155:103673. doi: 10.1016/j.apsoil.2020.103673
Veen, G. F., Sundqvist, M. K., and Wardle, D. A. (2015). Environmental factors and traits that drive plant litter decomposition do not determine home-field advantage effects. Funct. Ecol. 29, 981–991. doi: 10.1111/1365-2435.12421
Wang, L., Chen, Y., Zhou, Y., Zheng, H., and Liu, Y. (2021). Litter chemical traits strongly drove the carbon fractions loss during decomposition across an alpine treeline ecotone. Sci. Total Environ. 753:142287. doi: 10.1016/j.scitotenv.2020.142287
Wang, Z. H., Tang, Z. S., and Fang, J. Y. (2009). The species–energy hypothesis as a mechanism for species richness patterns. Biodivers. Sci. 17, 613–624. doi: 10.3724/SP.J.1003.2009.09161
Xu, D. W., Liu, J. F., Marshall, P., He, Z. S., and Zheng, S. Q. (2017). Leaf litter decomposition dynamics in unmanaged Phyllostachys pubescens stands at high elevations in the Daiyun Mountain National Nature Reserve. J. Mountain Sci. 14, 2246–2256. doi: 10.1007/s11629-017-4426-8
Yang, X., and Jin, C. (2009). Plant litter quality influences the contribution of soil fauna to litter decomposition in humid tropical forests., southwestern China. Soil Biol. Biochem. 41, 910–918. doi: 10.1016/j.soilbio.2008.12.028
Yang, X., Wang, X., Xiao, S., Liu, Z., and Nielsen, U. N. (2021). Dominant plants affect litter decomposition mainly through modifications of the soil microbial community. Soil Biol. Biochem. 161:108399. doi: 10.1016/j.soilbio.2021.108399
Yang, Y., Qu, Y., Yang, N., Wang, J., Zhao, N., and Gao, Y. (2019). Litter species diversity is more important than genotypic diversity of dominant grass species Stipa grandis in influencing litter decomposition in a bare field. Sci. Total Environ. 666, 490–498. doi: 10.1016/j.scitotenv.2019.02.247
Yin, R., Eisenhauer, N., Auge, H., Purahong, W., Schmidt, A., and Schädler, M. (2019). Additive effects of experimental climate change and land use on faunal contribution to litter decomposition. Soil Biol. Biochem. 131, 141–148. doi: 10.1016/j.soilbio.2019.01.009
Keywords: cellulose, elevation, foliar litter, lignin, microclimate
Citation: Chen B, Yang Y, Chen L, Jiang L, Hong Y, Zhu J, Liu J, Xu D, Kuang K and He Z (2023) Microclimate along an elevational gradient controls foliar litter cellulose and lignin degradation in a subtropical forest. Front. For. Glob. Change 6:1134598. doi: 10.3389/ffgc.2023.1134598
Received: 30 December 2022; Accepted: 25 April 2023;
Published: 15 May 2023.
Edited by:
Yanlong Guo, Chinese Academy of Sciences (CAS), ChinaReviewed by:
Ivika Ostonen, University of Tartu, EstoniaTimo Domisch, Natural Resources Institute Finland (Luke), Finland
Yongchuan Yang, Chongqing University, China
Copyright © 2023 Chen, Yang, Chen, Jiang, Hong, Zhu, Liu, Xu, Kuang and He. This is an open-access article distributed under the terms of the Creative Commons Attribution License (CC BY). The use, distribution or reproduction in other forums is permitted, provided the original author(s) and the copyright owner(s) are credited and that the original publication in this journal is cited, in accordance with accepted academic practice. No use, distribution or reproduction is permitted which does not comply with these terms.
*Correspondence: Zhongsheng He, anhoenM4NUBmYWZ1LmVkdS5jbg==