- 1Ethiopian Biodiversity Institute, Addis Ababa, Ethiopia
- 2Department of Plant Biology and Biodiversity Management, College of Natural and Computational Sciences, Addis Ababa University, Addis Ababa, Ethiopia
C. grandiflora has been termed the worst invasive alien plant species. However, its allelopathic potential has not been studied yet. The goal of this research was to study the allelopathic potential of C. grandiflora on the germination and seedling growth of L. usitatissimum and G. abyssinica. Plant specimens and samples of C. grandiflora were collected from the Minjar-Shenkora district, North Shewa Zone, Ethiopia. An experimental investigation was conducted to check the impact of its leaf and root extracts on the growth and germination parameters of the indicated test plant species. The root and leaf samples were extracted with water and methanol (99.85%). Leaf and root samples of 25 g were steeped separately in 250 ml of each of the specified solvents to make the stock solutions. The experiment was conducted in 9-cm-diameter Petri-dishes and laid out in completely random designs, replicated thrice with five blocks, each block representing a different concentration of extract (25, 50, 75, and 100%) and control. Twenty-five seeds of each test plant were placed in a petri-dish along with five ml of each extract. Differences between treatments were evaluated using one-way-ANOVA and Tukey's Honestly Significant Difference (HSD) Test. Results revealed that the inhibitory effect of water extracts of roots and leaves samples was concentration-dependent. With leaf extracts as opposed to root, the inhibitory effect was more pronounced. Hence, when L. usitatissimum seeds were treated with 100% concentration of water extracts from leaf samples of C. grandiflora germination-percentage, seedlings dry-weight, plumule, and radicle length were reduced by 80, 79.3, 73.6, and 79.4% respectively compared to the control. The above parameters also dropped considerably when G. abyssinica seeds were treated with the indicated concentrations. Conversely, the germination of seeds from both test plant species was entirely inhibited in methanol extracts. From this study, it can be deduced that these invasive plant extracts have herbicidal properties. Further phytochemical and molecular study is recommended to identify the active ingredients of C. grandiflora and determine their characteristics. Besides, the integration of identified allelopathic substances as a tool in the management of invasive species is proposed as an environment-friendly option to lessen the deterioration of ecosystem services.
Introduction
Invasive alien species (IASs) are both alien and harmful to their environments, which include plants, animals, and microorganisms. A decline in the production of indigenous capacity or loss of biodiversity has taken place, particularly as a result of invasive alien plant species (IAPS) (Witt and Luke, 2017). IAPSs can have an even greater detrimental effect on economic performance and food security in developing countries where agriculture makes up a larger portion of GDP. IAPS affect almost every type of ecosystem. They are one of the main destroyers of biological diversity globally (Brown et al., 1998; Reddy, 2008). IAPSs are likely to proliferate into various ecosystems and harm biodiversity by establishing dominant and successful populations (Maharjan et al., 2007; Mushtaq and Siddiqui, 2018).
Allelopathy is one of the fundamental mechanisms responsible for the success of IAPS and involves the release of substances that prevent native plant communities from germinating, promoting early seedling growth, developing, and reproducing. It occurs as a result of the release of secondary metabolites. Plants produce a mass of compounds via secondary metabolism. The production of these compounds depends on the presence of precursor molecules and the triggering of specialized genes. The activation of genes required for the biosynthesis of allelochemicals is often dependent on environmental stimuli. These allelochemicals are stored in a single location, multiple locations, or throughout the entire plant. They are often found in the roots, leaves, pollen, shoots, fruit, rhizomes, stems, buds, seeds, and flowers (Javaid et al., 2006; Pisula and Meiners, 2010; Trezzia et al., 2016; Chen et al., 2017; and Hussain et al., 2020). Root exudate, volatilization, leaching from existing plant parts, residue putrefaction, and farming activities all contribute to the release of allelochemicals into the environment. Chemical exudates released from many IAPS parts have inhibitory, competitive, and pathogenic effects on the native flora. Hence, allelopathy serves as an invasion mechanism (Clark, 2017; Moraa et al., 2018; Novak et al., 2018; Scavo et al., 2019).
Allelopathy can be considered a system of communication between plants. Mechanisms of allelopathic inhibition are multifaceted and can also consist of the interaction of different classes of chemical compounds, such as flavonoids, alkaloids, phenolics, terpenoids, and steroids, with combinations of different compounds occasionally having a superior allelopathic effect than distinct compounds alone. Moreover, physi-ological and environmental stresses, such as solar radiation, pests and diseases, herbicides, and less than optimal nutrient, temperature, and moisture levels can affect allelopathic weed suppression. Consequently, allelopathic interactions are an essential factor in determining species distribution and abundance within plant communities and are also thought to be important in the accomplishment of many IAPS (Aguilera et al., 2015; Kalisz et al., 2021). Thus, allelochemicals seem to alter a range of physiological processes, and it is challenging to separate the primary from secondary effects. Allelochemicals also have substantial effects on ion and water uptake, water status, cell division, cell differentiation, respiration, photosynthesis, enzyme function, phytohormone metabolism, signal transduction, and gene expression. Allelochemicals may produce more than one effect on the cellular processes responsible for reduced plant growth. C. grandiflora is one of the IAPS that has been described as the biggest threat to natural ecosystems, biodiversity, the economy, and health (Brown et al., 1998; Chen et al., 2017; Rana, 2018; and Thiébaut et al., 2019). C.grandiflora Roxb. Ex R. Br. (rubber vine), family Asclepiadaceae, is a ferocious woody climbing shrub that can climb over trees up to 30 meters tall and harms native trees by encroaching on their canopy, obstructing their access to the sun, and “choking” them (Brown et al., 1998). Its astonishing seed output, which can result from a single vine bloom, wind-dispersed seeds, or water, is largely responsible for its invasiveness. A fully developed plant can yield more than 8,000 seeds. It can also set seed at least twice annually and form a deep taproot. If moisture is present, more than 90% of seeds will germinate (Rodriguez-Estrella et al., 2010). It has the capacity to spread into new environments and exert detrimental effects on biodiversity, the economy, and the environment. It is toxic to animals and displaces native species, making grazing land less productive (Luizza et al., 2016). The plant contains toxic cardenolides (cardiac glycosides), which can cause heart failure as well as stomach and intestinal disorders in both humans and animals (Prasad and Lavanya, 2017).
C. grandiflora has been related to the extinction of various animal species and poses a threat to several areas with high conservation value (Doak and Deveze, 2004; Burg et al., 2012). All parts of this plant are poisonous (Nelson et al., 2007). Numerous diverse ecosystems, including national parks and places designated as World Heritage sites, are at risk of being completely displaced (Head et al., 2015). It was deliberately introduced in other places as an ornamental plant, but its origin of introduction to Ethiopia is uncertain. In Ethiopia, it is a major threat to biodiversity and local pastoral livelihoods in the Afar region (Luizza et al., 2016) and spreading rapidly to the East and North Shewa zones. In the study areas, C. grandiflora is alarmingly invading the wood and shrub lands in different Kebeles (the lowest administration zone in Ethiopia) at low altitude, such as Gordo Solomon, Akrmit, and Mintamir (Berehet district), Korma (Minjar Shenkora district), Gelgela (Asagirt district), and Abayatir (Kewot district). The allelopathic potential of C. grandiflora has not been investigated yet. Therefore, determining the allelopathic properties of C. grandiflora on the germination and seedling growth of the indigenous plant species, G.abyssinica and L.usitatissimum, was identified as the goal of this study.
Materials and methods
Laboratory experiments were conducted to examine the effect of C. grandiflora leaf and root extracts on the germination and seedling growth of the test plant species (G. abyssinica and L. usitatissimum) at the Ethiopian Biodiversity Institute (EBI). Root and leaf samples were chosen because these parts of the plant species are the most common sources of allelochemicals, though they can be present in almost all parts of plants (Zhang and Fu, 2010; Weston et al., 2012; Ferguson et al., 2016). The root and leaf samples were extracted using water and 99.85% methanol. Thus, two laboratory experiments were conducted from September 2021 to March 2022.
Collection of plant materials
Fresh and mature C. grandiflora leaf and root samples were collected from the North Shewa Zone, Minjar-Shenkora district, Korma Kebele (39°34′01.620″E, 9°01′55.590″N), Amhara region, Ethiopia, which is located ~139 kilometers (km) east of Addis Ababa at an elevation of 1,388 meters above sea level from 15 to 16 September, 2021. To take the root and leaf samples, sharp pruning shears, and hatchets were used. The cutting edge of those tools was sterilized with sanitizers at the beginning and before using them again. The collected root and leaf samples were placed in loosely sealed plastic bags with a few air holes cut in them in a moisture-free atmosphere and shipped soon after collection. Then the soil particles, contaminating, and other foreign materials were removed from root and leaf samples by gentle brushing. The leaf and root samples were chopped by using hatchets into small pieces (2 cm long) and then air dried in the shade at room temperature of 25°C for about a month. Next, the dried root and leaf samples were crushed into smaller pieces using a locally made mortar and pestle. Those tools were also disinfected by means of sterilizers at the beginning and before being used again. The dried and crushed samples were stored in plastic bags before being used for experiments. Finally, with the help of a mechanical grinder (Nicht offnen bei laufendem Motor, BDI-01-15-0010-), the dried and crushed samples were ground in the laboratory, and the powders were stored in plastic bottles at room temperature (25°C) until extraction (Khan et al., 2011; Dar et al., 2017; Hassan et al., 2018).
Aqueous extract preparation
The aqueous extracts of C. grandiflora leaf and root samples were prepared from the ground materials. Ground materials weighing 25 g were soaked separately in 250 ml of distilled water within conical flasks. Then, the flasks were shaken on an orbital shaker at 150-rpm agitation speed for 24 h at room temperature to make the stock solution of the maximum concentration. Following, the extracts were stored in plastic bottles at room temperature until dilution. Subsequently, to obtain a final volume of 250 ml, each crude extract was filtered using Whatman filter paper no.1. Both crude extracts (ml) were diluted with distilled water (ml) in the ratio of 25 ml of extract to 75 ml of distilled water, 50 ml of extract to 50 ml of distilled water, 75 ml of extract to 25 ml of distilled water, and 100 ml of extract to 0 ml of distilled water to obtain different concentrations of 25, 50, 75, and 100%, respectively (by assuming 25ml, 50ml, 75ml, and 100ml extracts equivalent to 25, 50, 75, and 100% concentrations respectively). The diluted extracts were kept in the refrigerator at 4°C. The leaf and root samples were also extracted with methanol (99.85%). The same procedures were conducted for methanol extraction except for the removal of the alcohol by evaporation. Evaporation of methanol was carried out to minimize the effect of the alcohol on the germination of the test seeds. Accordingly, at the end of the filtration process, methanol extracts were evaporated using a rotary evaporator at 40°C using a Buchi R-114 Rota Vapor, and the residues were weighed. A thick mass of coagulated liquid was then collected. From a 25-gram sample of C. grandiflora powder, the average extract was 4.39 g. For the bioassay, each stock extract from C. grandiflora leaf and root samples was diluted in distilled water (in similar procedures to the above extraction process) to provide extract concentrations of 25, 50, 75, and 100%. All extracts were also stored in a refrigerator at 4°C until use. A control (distilled water) was used for comparison (Mehrafarin et al., 2011; Waris et al., 2016; Dar et al., 2017; Laizer et al., 2021; Singh et al., 2021).
Test seed preparation
The test seeds were accessed from the Ethiopian Biodiversity Institute based on the Ethiopian Genetic Resources Access and Benefit Sharing law which were collected from North Shewa Zone, Amhara region. These indigenous plant species were selected because they germinate easily, aside from their high economic significance to the community in the study area.
Different accessions (with accession numbers 15119, 15135, 15767, 202449, 202450, 212492, 214926, 212495, 212497, and 212501 for G. abyssinica, and 13502, 13503, 13506, 13508, 18788, 18793, 18794, 18797, 18803, and 35549 for L. usitatissimum) of test plant species seeds were surface sterilized for 30 minutes in 5% sodium hypochlorite and then rinsed six times with distilled water.
Germination bioassay
We placed 25 seeds of test plant species in 9 cm diameter Petri dishes lined with Whatman no. 1 filter paper. The Petri dishes were moisturized with 5 ml of different concentrations of water extracts of leaf and root samples of C. grandiflora (25, 50, 75, and 100%). They were also humidified with distilled water when necessary. Control Petri dishes were also retained in all experiments using only distilled water. Every single treatment was duplicated three times, making a total of 60 Petri dishes, and each of the three replicates was repeated twice (30 Petri dishes for each test plant species). The germination tests were also performed in Petri dishes on filter paper with methanol extracts. Methanol extracts (5ml) were applied to each individual filter paper after the removal of the alcohol via evaporation. The seeds were considered to have germinated when the radicle grew to 2 mm long (Waris et al., 2016). The number of seeds germinated was recorded every 24 h until 12 and 14 days for L. usitatissimum and G. abyssinica, respectively (Stefanello et al., 2017).
Seedling length (cm) of test plant species
Test plants' plumule and radicle length were measured using a ruler, and the average length was taken (5 seedlings from each treatment were measured for a single test, in total 5*3) (Hussain et al., 2020).
Fresh and dry weight of test plant seedlings (mg)
With the aid of an electronic balance, the fresh and dry weight of L. usitatissimum and G. abyssinica seedlings were measured after twelve and fourteen days of germination, respectively, for the treatment group. On the other hand, the fresh and dry weights of the control group were measured after seven and ten days of germination for L. usitatissimum and G. abyssinica, respectively (IBPGR, 2012). The average fresh and dry biomass were then estimated in mg (5 seedlings from each treatment were measured, for a single treatment, 5*3 in total) (Benyas et al., 2010 and Waris et al., 2016). Dry weights were measured after drying at 70°C for 48 h in an oven (ISTA, 2005).
Experimental design and data analysis
The tests were set up in a Completely Randomized Design (CRD) with five blocks and three replications repeated twice, where each block represents a control and concentration of an extract from leaf and root samples of C. grandiflora (0, 25, 50, 75, and 100%). The R-package (version 3.6.2) was used to analyze the data that were gathered. Using descriptive statistics, growth rate and germination percentage (GP) data were examined. Additionally, an inferential statistical approach was used. The significant impacts of the leaf and root extracts of C. grandiflora on the growth and germination of test plant species were tested with simple linear regression analysis. The leaves and roots extract and the control (0, 25, 50, 75, and 100%) were taken as the independent variables while seedlings growth and number of seeds germinated were taken as the dependent variables.
The recorded data for the experiment were subjected to a one-way ANOVA for each parameter to establish differences among the treatment means. Significance levels among treatment means were compared using Tukey's Honestly Significant Difference (HSD) Test at a 5% level of significance to find out which groups were significantly different from one another (the control to 25, 50, 75, 100%, and each other too). Each of the following germination parameters was calculated using the following formulas:
Mean Germination Time
Mean Germination Time (MGT) is an exact measure of the period taken for the given seeds to germinate, but does not correlate this with the uniformity or time spread of germination.
MGT = ΣTiNi/S, where Ti = number of days after beginning of the trial, Ni = number of seeds germinated on the day, S = total number of seeds germinated (Waris et al., 2016).
Germination Rate Index
Germination Rate Index (GRI) is the percentage of germination on each day of the germination period. Higher GRI values indicate higher and faster germination, subsequently the higher the percentage and the shorter the time to germinate, the higher the GRI. GRI = G1/1 + G2/2 +· · ·+ Gx/x, where G1 = germination percentage × 100 at the first day after sowing, G2 = Germination percentage × 100 at the second day after sowing +, (Kader, 2005).
Coefficient of Velocity of Germination
Coefficient of Velocity of Germination (CVG) is an indication of the rapidity of germination. It increases when the number of germinated seeds increases and the time required for germination decreases.. CVG= (N1 + N2 + · · + Nx) *100/N1T1 + · ·· + NxTx, where N = number of seeds germinated each day, T= number of days from seeding parallel to N. Maximum CVG might exist ideally is 100, if all seeds germinated on the first day (Kader, 2005; Ranal and Santana, 2006).
Results
Effects of aqueous extracts of leaf and root samples of C. grandiflora on germination of the test plant species
The results of this study revealed that there were significant variations in the average number of germinated seeds for each treatment. The quantity of seeds that sprouted for each treatment was observed to depend on concentration gradients (independent variables). The quantity of seeds that germinated per treatment dropped as the concentration of water extracts from leaf and root samples increased. Hence, the effect of water extracts of leaf and root samples of C. grandiflora on germination of L. usitatissimum, liner regression equation can be presented as: Y = −20.556X + 26.156, R2 = 0.9963) (Figure 1A) and Y = −20.667X + 27.267, R2 = 0.9288 respectively. The concentration of water extracts from leaf and root samples of C. grandiflora and the quantity of G. abyssinica seeds that germinated in each treatment also had a very strong negative association. The regression equation for seeds treated with water extracts of leaf and root samples: Y = −19.733X + 26.4, R2 = 0.9756 (Figure 1B) and Y = −11.2X + 26.267, R2 = 0.9093 respectively. Contrary to water extracts no germination was seen for either test plant seed in methanol extracts of C. grandiflora root and leaf samples.
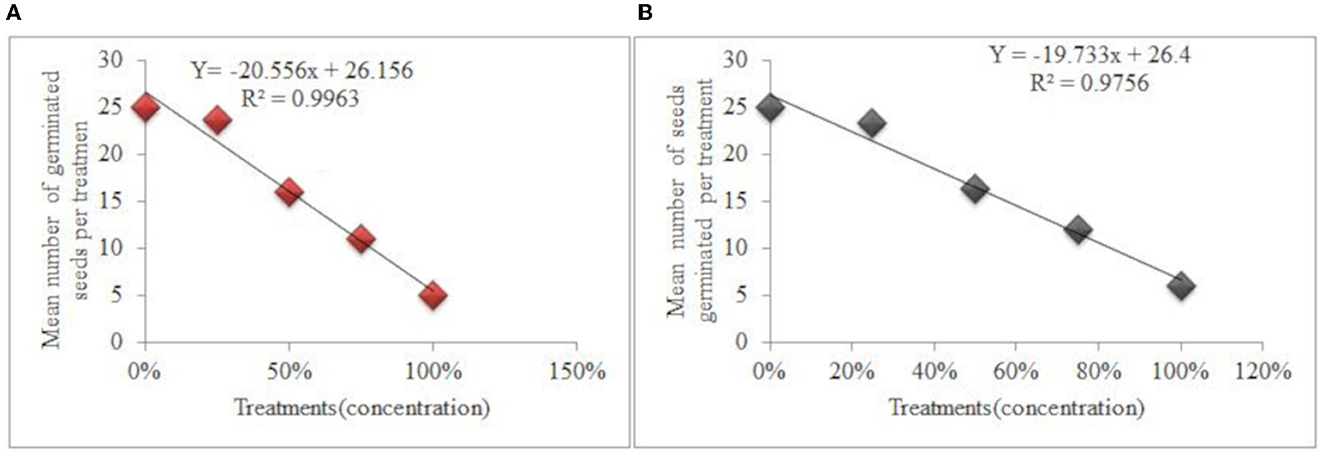
Figure 1. Effects of water extracts of leaf samples of C. grandiflora on the germination of L. usitatissimum (A) and G. abyssinica (B).
Effect of aqueous extracts of leaf and root samples of C. grandiflora on the germination parameters
Germination percentage
The control group had the highest mean GP (100%) in both test plant species. In contrast, the L. usitatissimum seeds treated with 100% concentration of water extracts of leaf sample of C. grandiflora had the lowest mean GP (20%), followed by 24% (G. abyssinica seeds treated with 100% concentration of water extracts of leaf sample). Largely, higher concentrations of water extracts of leaf (≥50%) and root (≥75%) samples in all laboratory experiments were effective in suppressing the germination of both test seeds compared to the control (Tables 2, 3). In general, greater concentrations of water extracts of leaf samples (50, 75, and 100%) reduced germination much more than root extracts and were also more efficient in delaying the germination of L. usitatissimum than G. abyssinica seeds (Figure 2). In comparison to the control, the GP of L. usitatissimum and G. abyssinica seeds treated with 100% concentration of water extracts of leaf samples, dropped by 80 and 76% respectively. The result of one-way ANOVA (Analysis of variance) also showed that both water extracts leaf and root samples had a substantial effect on the germination of the test plant species (Table 1).
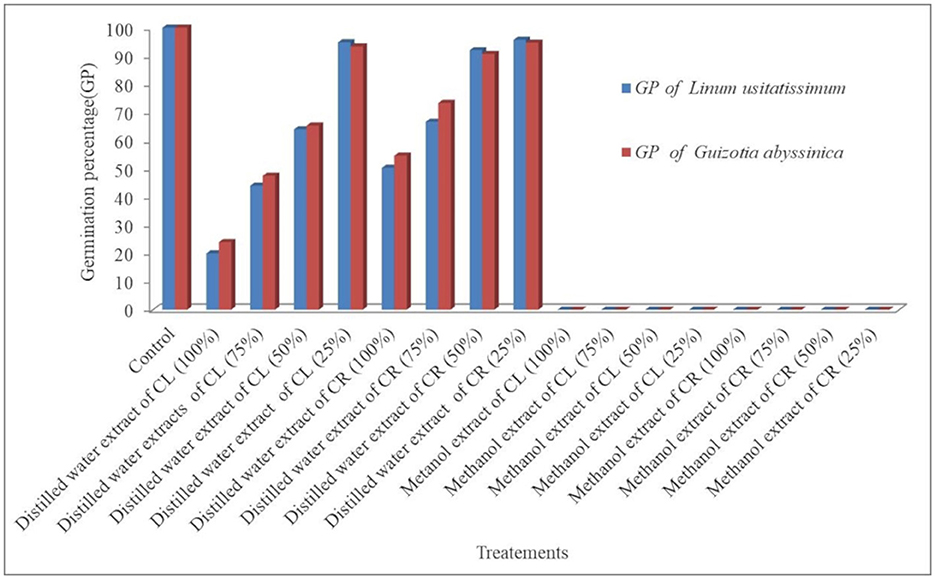
Figure 2. Effect of water and methanol extracts of leaf and root samples of C. grandiflora on the germination Percentage (GP) of test plant seeds: CL: - C. grandiflora leaf extracts, CR: - C. grandiflora root extracts.

Table 1. One-way ANOVA on the effect of water extracts C. grandiflora leaf and root samples on germination percentage (GP) of L. usitatissimum and G. abyssinica seeds.
Tukey's Honestly Significant Difference (HSD) Test (multiple comparisons) revealed that both test plants treated with root extracts of only 75 and 100% concentrations were significantly different from the control as well as from seeds treated with 25% concentration of the indicated extract (P ≤ 0.001). Both test seeds treated with water extracts of 50% concentrations of root samples were not also significantly different from the control. On the other hand, GP of L. usitatissimum and G. abyssinica seeds treated with water extracts of 50, 75, and 100% concentrations of leaf samples were significantly different from the control as well as to the seeds treated with 25% water extracts of the specified samples (P ≤ 0.001). Hence, both test seeds treated with water extracts of 25% concentrations of leaf samples were not significantly different from the control.
Mean germination time
L. usitatissimum seeds treated with 100% concentration water extract of C. grandiflora leaf samples had the greatest MGT (9.98 days), followed by seeds treated with 100% concentration root extract (8.81 days). Seeds treated with 25% concentrations of water extracts of C. grandiflora's root samples had the lowest MGT (3.51days) from the experimental group. The control had the lowest MGT (3.25 days). In comparison to the control, the MGT of L. usitatissimum seeds treated with 100% concentration water extracts of leaf samples increased by 67.43% (Table 2). Regarding the MGT of G. abyssinica seeds, the control group also had the lowest MGT (3.36 days). From the experimental group, seeds treated with 25% concentrations of leaf (3.72%) and root extracts (3.75%) had smaller MGTs. On the other hand, G. abyssinica seeds treated with 100% concentration of water extract from leaf samples had the highest MGT (9.41 days). As a result, the MGT of the G. abyssinica seeds treated with 100% water extracts of leaf samples increased by 64.29% compared to the control (Table 3).
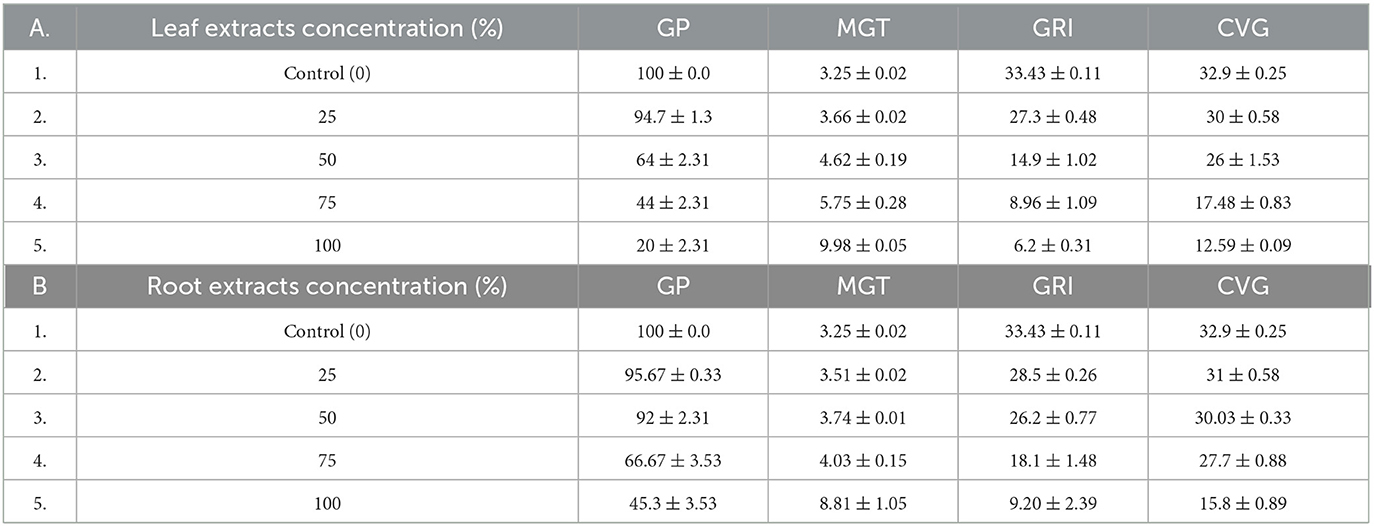
Table 2. Effects of water extracts of leaf (A) and root (B) samples of C. grandiflora on GP, MGT, GRI, and CVG of L. usitatissimum seeds [values, expressed as Mean ± standard error (SE)].
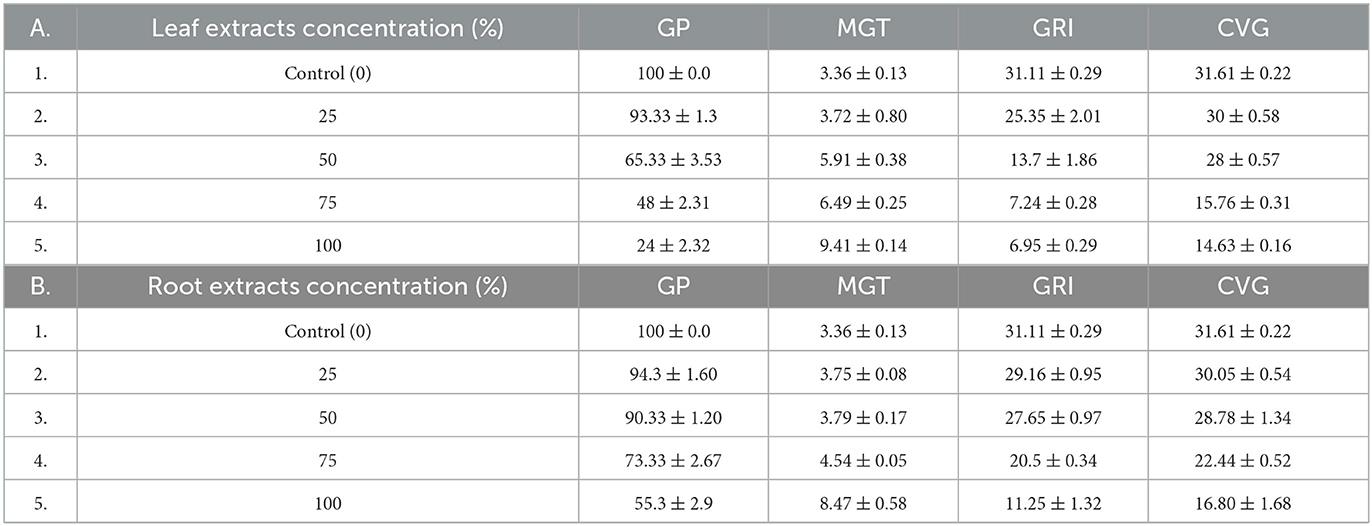
Table 3. Effects of water extracts of leaf (A) and root (B) samples of C. grandiflora on the GP, MGT, GRI, and CVG of G. abyssinica seeds [values, expressed as Mean ± standard error (SE)].
Germination rate index
The L. usitatissimum seeds used as the control had the highest mean GRI (33.43), and G. abyssinica seeds came in second (31.11). From the experimental group, G. abyssinica seeds treated with 25% root extracts had the highest mean GRI (29.16). Conversely, L.usitatissimum seeds (6.2), treated with 100% water extracts of leaf samples, had the lowest mean GRI, followed by G. abyssinica (6.95). As a result, the mean GRI of L. usitatissimum and G. abyssinica seeds treated with a 100% concentration of water extracts from C. grandiflora leaf samples decreased by 81.45, and 77.66 % respectively (Tables 2, 3).
Coefficient of velocity of germination
L.usitatissimum seeds used as controls had the greatest mean CVG (32.9) followed by 31.61 (the control seeds of G. abyssinica). From the experimental group, L. usitatissimum seeds treated with 25% concentration root extracts had the greatest mean CVG (31). L. usitatissimum seeds treated with 100% concentrations of water extracts of leaf samples had the lowest mean CVG (12.59). In comparison to the control, the CVG of L. usitatissimum and G. abyssinica seeds treated with 100% water extracts of leaf samples fell by 61.73 and 53.72%, respectively. Generally, CVG decreased as the concentration of water extracts from leaf and root samples of C. grandiflora increased in both tests (Tables 2, 3).
Effects of aqueous extracts of leaf and root samples of C. grandiflora on growth parameters
Plumule and Radicle length (in cm) of the seedlings
The result of one-way ANOVA indicated water extracts from C. grandiflora root and leaf samples generally had a significant (P ≤ 0.001) impact on the mean plumule and radicle length of both test plant species (Table 4). Tukey's Honestly Significant Difference (HSD) Test on mean plumule length also revealed that seedlings exposed to 50, 75, and 100% concentrations of water extract from leaf and roots samples were substantially different from the control for both tests (P ≤ 0.001) whereas seedlings of both tests exposed to a 25% concentration of each extract were not considerably varied from the control. G. abyssinica seeds in the control group had the tallest mean plumule length (8.98 cm). Conversely, L.usitatissimum and G. abyssinica seedlings treated with 100% concentration of water extracts of leaf samples had mean plumule lengths of 2.19 and 2.39 cm respectively. Therefore, L. usitatissimum seedlings treated with 100% concentration of water extract of leaves samples had the smallest mean plumule length (2.19 cm). Consequently, mean plumule lengths of L. usitatissimum and G. abyssinica seedlings treated with water extracts of C. grandiflora leaf samples (100% concentration) were reduced by 73.6 and 73.3% respectively compared to the control (Tables 6, 7).
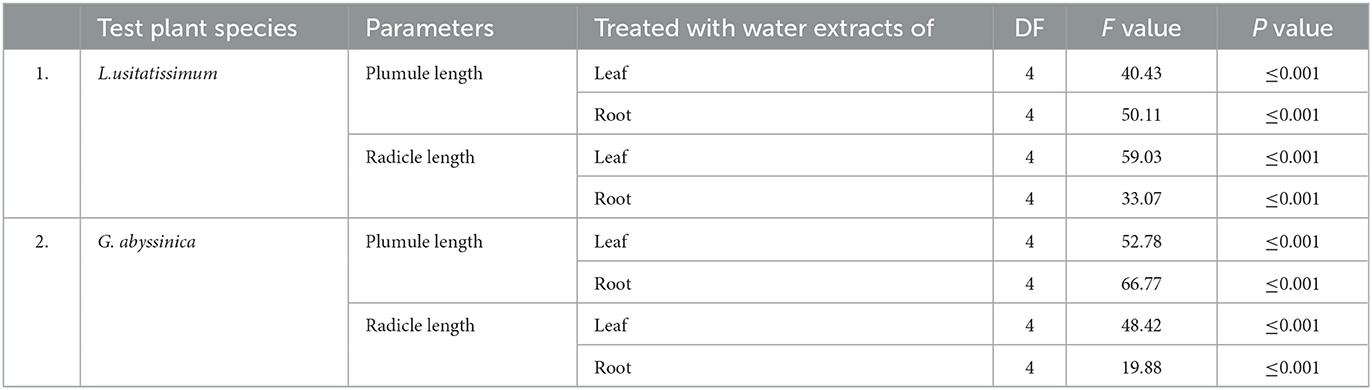
Table 4. One-way ANOVA on the effect of water extracts C. grandiflora leaf and root sample on plumule and radicle length of L. usitatissimum and G. abyssinica.
The concentration of water extracts from leaf samples of C. grandiflora and mean plumule length of L. usitatissimum and G. abyssinica seedlings for each treatment had a very strong negative association [the regression equation for both test species were:-Y = −6.692X + 8.864, R2 = 0.9507 (Figure 3A) and Y = −0.0672X + 8.842, R2 = 0.9483 (Figure 3B) respectively]. Mean plumule length of L. usitatissimum and G. abyssinica seedlings treated with water extracts root samples had also a strong negative association (regression equation Y = −0.0562X + 8.964, R2 = 0.9378, to and Y = −0.0557X + 9.534, R2 = 0.9341 respectively). Hence, as a concentration of water extracts of root and leaf samples of C. grandiflora increased, plumule length declined.
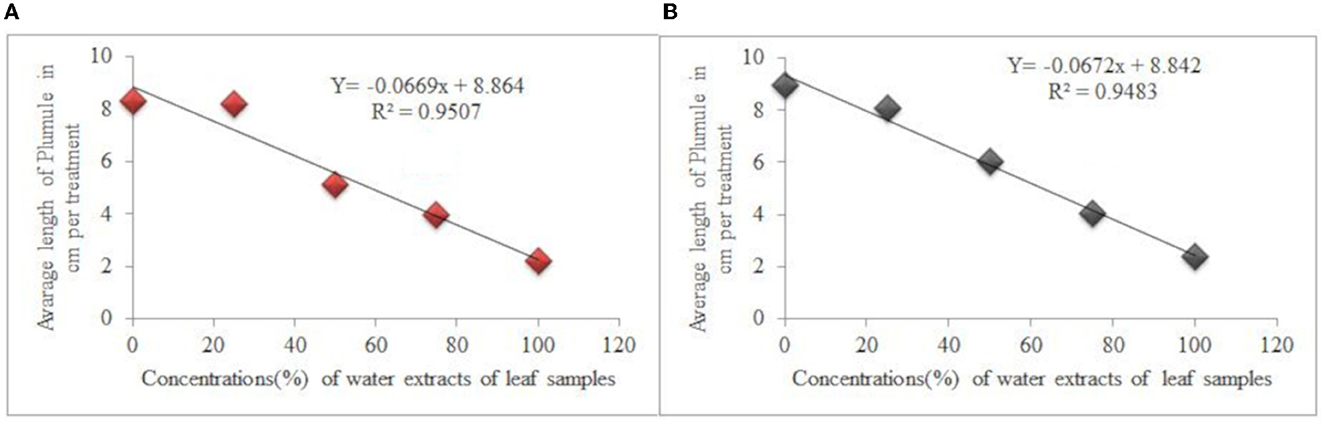
Figure 3. Effects of water extracts of leaf samples of C. grandiflora on plumule length of L. usitatissimum (A) and G. abyssinica (B).
In addition, the outcomes of Tukey's HSD Test revealed that the mean radicle length of seedlings of both test plant species treated with 50, 75, and 100% concentrations of water extracts of leaf samples of C. grandiflora were significantly different from the control (P ≤ 0.001) and to seedlings treated with water extracts of 25% concentration leaves sample (P ≤ 0.05). Moreover, the mean radicle length of seedlings treated with water extract of roots sample of 75 and 100% concentrations were substantially different (P ≤ 0.001) to the control as well as to seedlings treated with water extracts of 25% concentration root sample for both tests. Therefore, radicle lengths of both test plant species seedlings treated with water extracts of root samples of 25 and 50% concentrations were not considerably different from the control. Furthermore, the control seedlings of G. abyssinica were found to have the longest mean radicle length (7.87 cm). In contrast, the L. usitatissimum seedlings treated with 100% concentration of water extracts of leaf samples had the shortest mean radicle length (1.43 cm) succeeding by 2 cm (G. abyssinica). Hence, the mean radicle length of L. usitatissimum and G. abyssinica seedlings treated with 100% concentrations of water extract of leaves sample were reduced by 79.37 and 74.59% respectively compared to the control (Tables 6, 7).
The concentration of water extracts from leaf samples and mean radicle length of L. usitatissimum and G. abyssinica for each treatment also had a very strong negative association [the regression equation: Y = −0.0486X + 6.2, R2 = 0.9991 (Figure 4A) and Y = −0.058X + 7.584, R2 = 0.9876 (Figure 4B) respectively]. Seedlings radicle length of L.usitatissimum and G.abyssinica treated with water extracts of root samples had also a strong negative association (Y = −0.0507X + 6.836, R2 = 0.9087 and Y = −0.0404X + 7.622, R2 = 0.8933 respectively). Generally, as water extract of roots and leaves sample of C. grandiflora increased from 25 to 100% concentrations, radicle length was observed to drop for both tests.
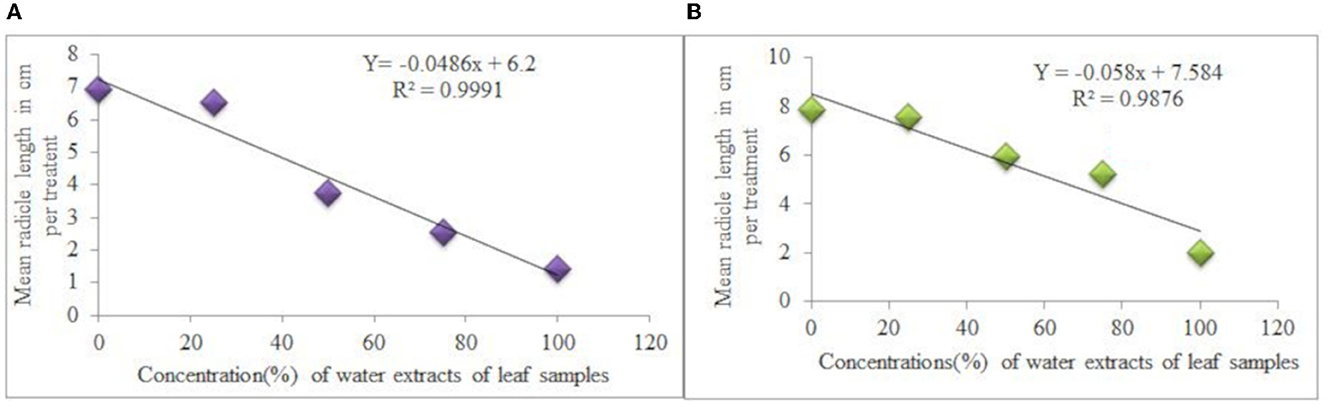
Figure 4. Effects of water extracts of leaf samples of C. grandiflora on radicle length of L. usitatissimum (A) and G.abyssinica (B).
Seedling fresh and dry weight (mg)
One-way ANOVA indicated water extracts of leaf and root samples of C. grandiflora had a significant (P ≤ 0.001) impact on fresh and dry seedling weight (mg) of both test plant species (Table 5). Tukey's Honestly Significant Difference (HSD) test also indicated the mean seedling fresh weight of L. usitatissimum treated with water extracts of leaf and root samples at 50, 75, and 100% concentrations were significantly different from the control as well as to seedlings treated with 25% concentration of water extract of leaves sample (P ≤ 0.001). Whereas the mean seedling fresh weight of G. abyssinica treated with water extracts of leaf and root samples at concentrations of 75 and 100% were significantly different from the control as well as to seedlings treated with 25% concentration of water extracts of leaf and root samples (P ≤ 0.001). However, the mean seedling fresh weights of G. abyssinica treated with 50% concentration of water extracts leaf samples (P ≤ 0.01) and water extracts of root samples (P ≤ 0.05) were significantly different from the control respectively.
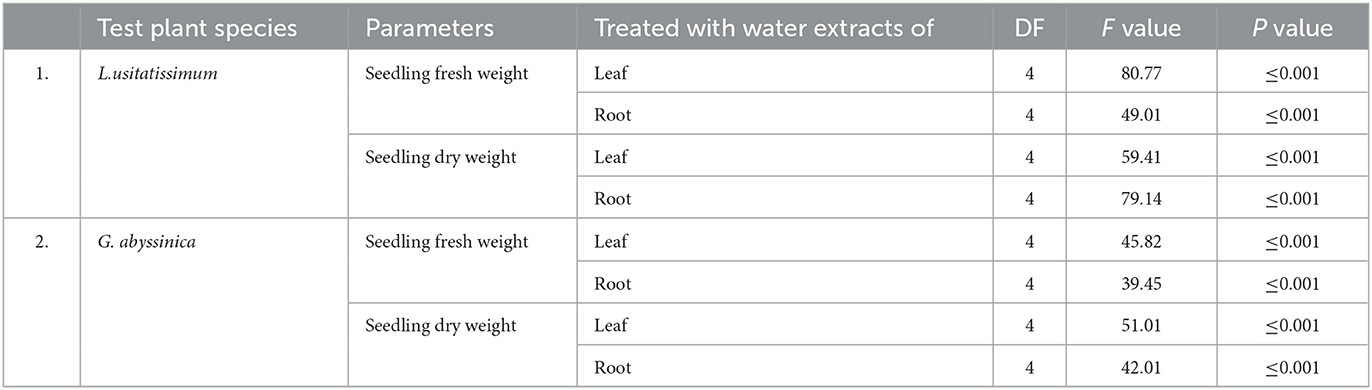
Table 5. One-way ANOVA on the effect of water extracts C. grandiflora leaf and root sample on seedling fresh and dry weight of L. usitatissimum and G. abyssinica.
The control group's seedlings of G. abyssinica had the highest mean fresh weight (67.87 mg). In contrast, L. usitatissimum seedlings treated with water extracts made from a 100% concentration of leaf samples were found to have the lowest mean fresh weight (16.77 mg) followed by 21.93 mg (G. abyssinica). Hence, the seedling fresh weight of L. usitatissimum and G. abyssinica seedlings treated with water extracts of 100% concentration of leaf samples, were reduced by 69.1 and 67.69% correspondingly in comparison to the control (Tables 6, 7). In addition, the mean fresh weight of both test plant species seedlings had a very strong negative correlation with the amount of water extracts of leaf and root samples. The regression equation for seedlings fresh weight of L. usitatissimum seeds treated with water extracts of leaf and root samples [Y = −39.168X + 52.388, R2 = 0.9789 (Figure 5A) and Y = −41.088X + 54.714, R2 = 0.9302 respectively]. The regression equation of seedlings fresh weight of G. abyssinica seeds that were treated with water extracts of leaf and root samples were: Y = −47.072X + 72.562, R2 = 0.9567 (Figure 5B) and Y = −38.7X + 68.696, R2 = 0.899 respectively. Generally, the mean fresh weight of seedlings of both test plant species dropped as the concentration water extract of roots and leaves sample of C. grandiflora raised.
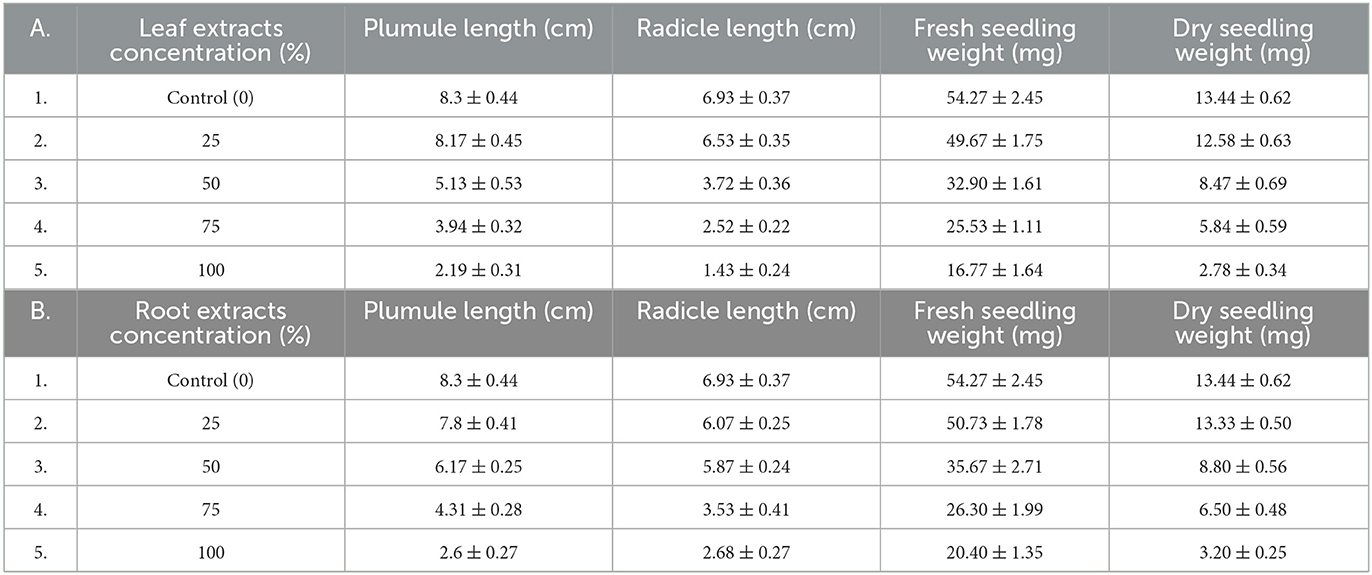
Table 6. Effects of water extracts of leaf (A) and root (B) samples of C. grandiflora on seedlings growth of L.usitatissimum, values expressed as Mean ± standard error (SE).
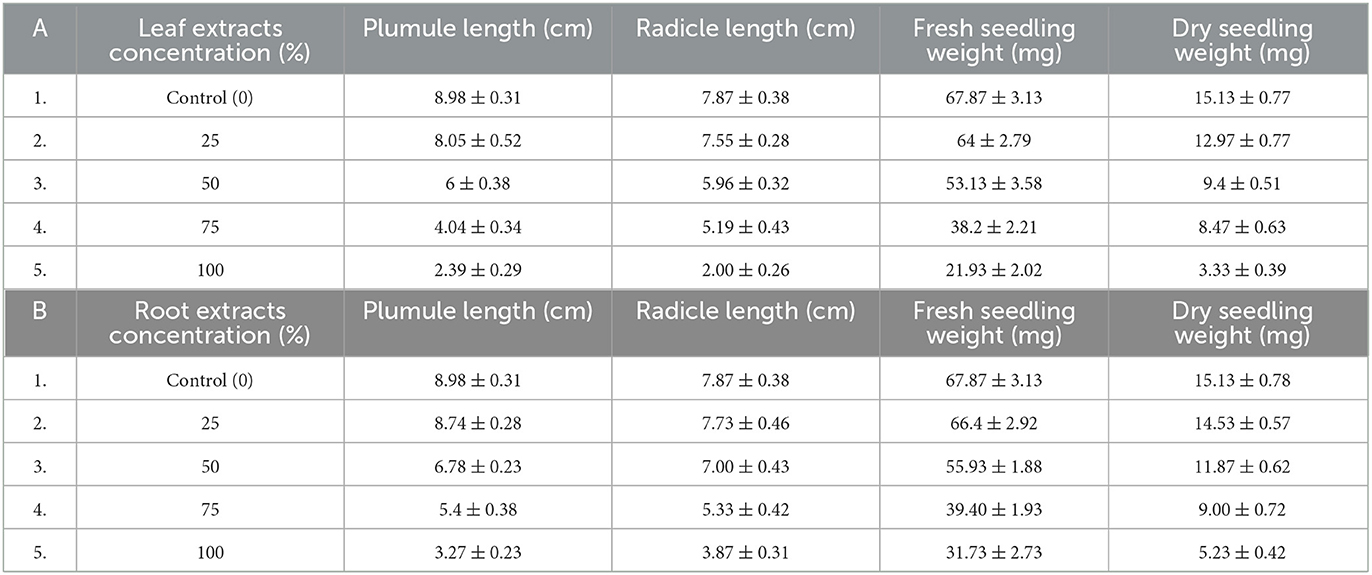
Table 7. Effects of water extracts of leaf (A) and root (B) samples of C. grandiflora on seedling growth of G. abyssinica, values expressed as Mean ± standard error (SE).
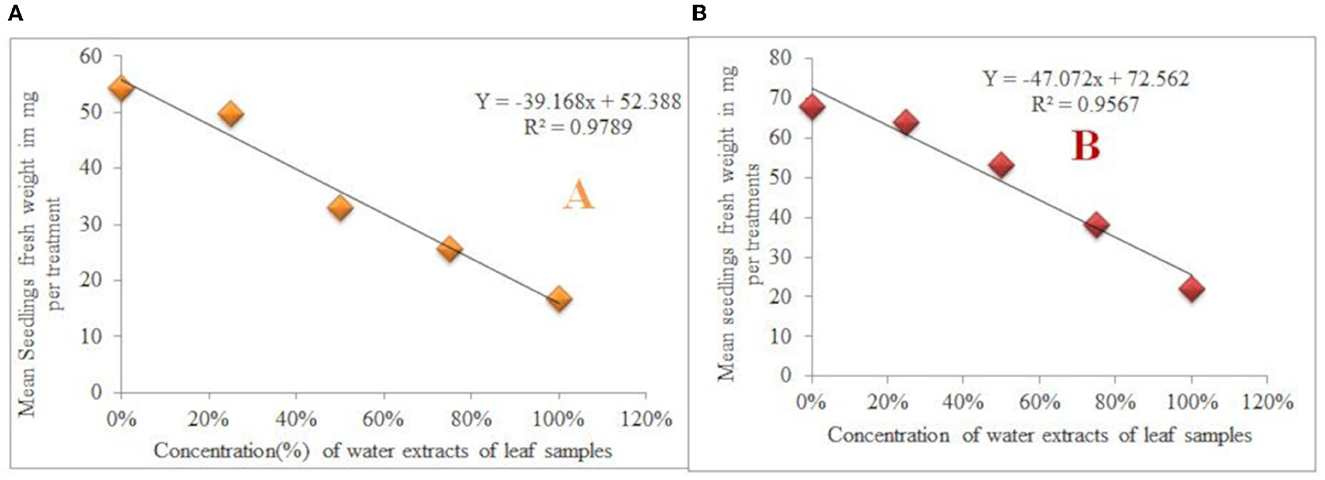
Figure 5. Effects of water extracts of leaf samples on seedling fresh weight of L. usitatissimum (A) and G. abyssinica (B).
Furthermore, Tukey's Honestly Significant Difference (HSD) Test also revealed that the mean seedling dry weight of G. abyssinica seedlings, treated with 75 and 100% concentration of water extracts of root samples were significantly different from the control (P ≤ 0.001) whereas seedlings treated with 50% concentration root extracts were considerably different from the control (P ≤ 0.01). Besides, the mean seedling dry weight of L. usitatissimum seeds treated with water extract of root samples at 50, 75, and 100% concentrations were significantly different from the control (P ≤ 0.001). Likewise, the mean seedling dry weight of both test plant species treated with 50, 75, and 100% concentrations of water extracts of leaf samples were significantly different (P ≤ 0.001) from the control. Hence, the mean desiccated weight of seedlings treated with 25% concentration of water extract of leaves and roots sample were not significantly different from the control of both test plant species.
From the control group, the seedling's maximum mean dry weight was 15.13 mg (G. abyssinica seedlings). In contrast, the L. usitatissimum seedling treated with water extracts of 100% concentrations leaf samples had the lowest mean dry weight (2.78 mg). Thus, seedling mean dry weight of L. usitatissimum and G. abyssinica seeds treated with water extracts of 100% concentrations leaf samples were dropped by 79.32 and 77.99% respectively compared to the control (Tables 6, 7). Thus, water extracts from leaf and root samples had a negative correlation with the mean dry weight of both test plant species seedlings as well. The regression equation of seedlings dry weight of L. usitatissimum seeds treated with water extracts of leaf and root samples were:- Y = −11.224X + 14.138, R2 = 0.9708 (Figure 6A) and Y = −11.452X + 14.482, R2 = 0.9487 respectively. The regression equation for seedlings dry weight of G. abyssinica seeds treated with water extracts of leaf and root samples were:-Y = −9.4X + 13.89, R2 = 0.9579 (Figure 6B) and Y = −7.792X + 14.138, R2 = 0.8754 respectively. From the regression equations, as the concentration of water extracts of root and leaf samples of C. grandiflora raised, the seedling dry weight of G. abyssinica and L. usitatissimum declined.
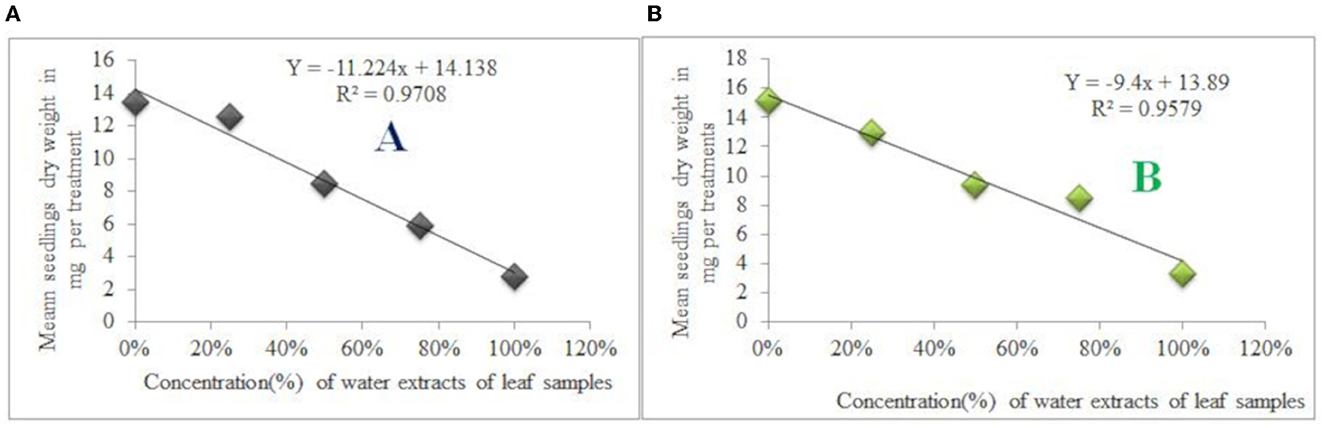
Figure 6. Effects of water extracts of leaf samples on the mean seedling dry weight of L.usitatissimum (A) and G. abyssinica (B).
Discussion
The findings of this research affirmed the assumption that water extracts of leaf and root samples of C. grandiflora greatly inhibited the germination and seedling growth of G. abyssinica and L. usitatissimum. Generally, as the water extracts concentration of root and leaf samples of C. grandiflora increased in comparison to the control, GP, GRI, CVG, plumule, and radicle length, the fresh and dehydrated seedling weights were significantly reduced. The outcomes of this study especially, indicated that treatments with higher concentrations of water extracts from roots (75 and 100%) and leaves (50, 75, and 100%) samples had adverse effects on the means values of these parameters. Hence, the aqueous extracts' inhibitory action was therefore concentration-dependent. Numerous studies undertaken in this field of research, including Elisante et al. (2013); Waris et al. (2016); Mushtaq and Siddiqui (2018); and Kalisz et al. (2021), support our findings.
Another study by Rodriguez-Estrella et al. (2010) indicated C. grandiflora is an extremely IAPSs in dry and semidry ecosystems of the world. It is a stress tolerant and adaptive species that is highly competitive in arid environments. In Ethiopia, C. grandiflora is exotic, and it poses a potentially major threat to biodiversity because it outcompetes native vegetation. It has been described as the biggest threat to natural ecosystems and is capable of destroying upper-story vegetation (Luizza et al., 2016). Additionally, the findings of the study by Pisula and Meiners (2010) showed that IAPSs are frequently more successful in introduced areas than in their natural habitats. Allelopathy has been proposed as a possible means for this achievement (Novak et al., 2018; Negi et al., 2020).
There are many studies that approve the allelopathic effects of various IAPSs on germination and seedling growth of native plant species. The study by Aguilera et al. (2015) on the IAPS, Acacia dealbata, indicated the GP was significantly affected by the aqueous extracts of all parts of this IAPS. Particularly, the decrease in GP ranged from 50 to 60% for Quillaja saponaria treated with the aqueous extracts from flowers and leaves of Acacia dealbata. In the current study, the mean GP of L. usitatissimum and G. abyssinica seeds treated with a 100% concentration of water extracts from C. grandiflora leaf samples were reduced by 80 and 76% respectively. Other research results on IAPSs with allelopathic potential, including Benyas et al. (2010), Dar et al. (2017), Laizer et al. (2021); and Hussain et al. (2020), found that treatments using higher concentrations of aqueous extracts from root and shoot samples had an adverse impact on germination and growth parameters.
GRI reflects the germination percentage (GP) for each day of the germination phase. It exhibited a decrease as concentrations of water extracts of both root and leaf samples increased. Hence, the mean GRI of L. usitatissimum and G. abyssinica seeds treated with 100% concentration of water extracts from leaf samples of C. grandiflora decreased by 81.45 and 77.67% respectively as compared to the control. In addition, the CVG provides a measurement of germination speed. It rises when the number of seeds that germinate increases and the time required for germination decreases. CVG does not center on the final percentage of germination but places emphasis on the time needed for reaching it (Kader, 2005; Ranal and Santana, 2006). Accordingly, in comparison to the control, the mean CVG of L. usitatissimum and G. abyssinica seeds treated with 100% concentration of water extracts of leaf samples fell by 61.73 and 53.72% respectively. As to MGT, it is an accurate measure of the time it takes for the specified seeds to germinate, but it does not relate this to the uniformity of germination (Moosavi et al., 2011). According to the study's findings, MGT increased when concentration rose from 0 to 100%. Hence, in the current investigation the mean MGT of the L.usitatissimum and G. abyssinica seeds tested with 100% concentrations of water extracts of leaf samples increased by 67.43 and 64.29% respectively compared to the control. The more quickly the germination occurs, the lower the MGT value is (Kader, 2005; Moosavi et al., 2011, and Waris et al., 2016).
Water and methanol, when compared as extraction solvents, had varied effects on the germination and seedling growth of the test plant species and had different interactions with the extract concentration in all measured parameters. Methanol extracts of the leaf and root samples of C. grandiflora completely inhibited (100%) seed germination of both test plant species. This might be because methanol has a hybrid bond nature that helps to extract both polar and non-polar allelochemicals from the root and leaf samples (a polar oxygen-hydrogen bond and a non-polar hydrocarbon chain). In some cases, methanol was reported to have the ability to hinder germination at higher concentrations (Mehrafarin et al., 2011; Waris et al., 2016). Besides, Sitthinoi et al. (2017) observed that irrespective of the extract concentration, the methanol extraction solvent severely hindered the seed sprouting of two rice cultivars.
Comparing water extracts of leaf and root samples, 75% of the concentrations of water extracts from C. grandiflora leaf samples significantly decreased the seedling growth and sprouting of both test plant species, especially at concentrations of 50, 75, and 100%. In general, this study's findings indicated that leaf extracts had a more pronounced inhibitory effect than root extracts on the test plant species' germination and growth parameters which were comparable with the findings of numerous other research results. Thus, when L. usitatissimum seeds were tested with higher concentrations (100%) of water extracts of leaf samples of C. grandiflora, GP, plumule and radicle length, seedlings' fresh and dry weight were inhibited by 80, 73.6, 79.37, 69.1, and 79.32% respectively compared to the control. In addition, when G. abyssinica seeds were treated with 100% concentrations of water extracts of leaf samples, GP, plumule, and radicle lengths, and the fresh and desiccated weight of seedlings were reduced by 76, 73.3, 74.59, 67.69, and 77.99%, correspondingly, in comparison to the control. Favaretto et al. (2011), Hu and Zhang (2013) and Negi et al. (2020) are among the studies supporting the present findings. In addition, the findings of research by Javaid et al. (2006) were also concurrent with our findings. Moreover, Turk and Tawaha (2003) study showed that the degree of allelopathic potential of various Brassica nigra plant parts can be graded in the following order of inhibition: leaves > flowers > mixture of all plant parts > stems > roots.
In terms of germination propensity, L. usitatissimum and G. abyssinica were reasonably comparable (at lower concentration ≤ 25%; however, the germination of L. usitatissimum seeds was inhibited more than that of G. abyssinica at higher concentrations of water extracts of leaf (≥ 50%), and root (≥ 75%) samples of C. grandiflora. Therefore, G. abyssinica seeds treated with higher concentrations of water extracts of leaf and root samples of C. grandiflora had a larger germination percentage than L. usitatissimum. This might be indicated the allelopathic potential of G. abyssinica against C. grandiflora. The findings of the studies by Moraa et al. (2018) and Oimbo et al. (2018) confirmed that G.abyssinica has been observed to have allelopathic potential on certain weeds and common beans (Phaseolus vulgaris) respectively. In addition, the result of the study by HabtamuAshagre et al. (2018) also indicated that higher concentrations (> 60%) of G. abyssinica leaf, root, and stem extracts have maximum allelopathic potential on Parthenium hysterophorus seed germination and growth parameters. These are some of the studies supporting the allelopathic potential of G. abyssinica as being resistant to allopathic properties of water extracts of leaf and root samples C. grandiflora).
Conclusion
The outcome of this study indicated that the inhibitory effect of water extracts of both root and leaf samples of C. grandiflora was concentration-dependent. The rate of inhibition rose in both test plant species as the concentration of water extracts of root and leaf samples increased. The seedling growth and germination of both test plant species were generally suppressed by water extracts of C. grandiflora root and leaf samples at varying concentrations. With leaf extracts as opposed to root extracts, this inhibitory impact was more pronounced. As a result of the nature of this solvent, methanol extracts completely inhibited the sprouting of seeds in both test plant species. According to the findings of this investigation, this plant's extracts may contain herbicidal properties. It is recommended that further phytochemical and molecular research be done to determine the herbicidal potential of C. grandiflora because allelopathy plays a major role in invasive alien species management.
Data availability statement
The original contributions presented in the study are included in the article/Supplementary material, further inquiries can be directed to the corresponding author.
Author contributions
AS performed the material preparation, field data collection, laboratory experiments, analysis, and wrote the first draft of the manuscript. EL, SD, and ZW supervised the entire work of this research. All authors have read and approved subsequent versions of the draft manuscript, including the final article, and contributed to the study's formation and design.
Acknowledgments
The corresponding author acknowledges the Ethiopian Biodiversity Institute for providing the opportunity to pursue a Ph.D. study and Addis Ababa University for financial support. The authors also extend remarkable thanks to Ethiopian Biodiversity Institute's, Genetic Resources Access and Benefit Sharing directorate, Germination and Nutrition Laboratory staff members for their assistance during laboratory experiments. AS also extends a special thanks to Mr. Gebeyaw Tilaye for his assistance during the entire laboratory works.
Conflict of interest
The authors declare that the research was conducted in the absence of any commercial or financial relationships that could be construed as a potential conflict of interest.
Publisher's note
All claims expressed in this article are solely those of the authors and do not necessarily represent those of their affiliated organizations, or those of the publisher, the editors and the reviewers. Any product that may be evaluated in this article, or claim that may be made by its manufacturer, is not guaranteed or endorsed by the publisher.
Supplementary material
The Supplementary Material for this article can be found online at: https://www.frontiersin.org/articles/10.3389/ffgc.2023.1131815/full#supplementary-material
References
Aguilera N. Becerra J. Guedes J. M. Villaseñor-parada C. González L. Hernández V. (2015). Allelopathic effect of the invasive Acacia dealbata Link (Fabaceae) on two native plant species in south-central Chile. Gayana Bot. 72, 231–239. doi: 10.4067/S0717-66432015000200007
Benyas, E., Hassanpouraghdam, M. B. S., Salmasi, I. S. Z., and Oskooei, O. S. K. (2010). Allelopathic Effects of Xanthium strumarium L. shoot aqueous extract on germination, seedling growth and chlorophyll content of lentil (Lens culinaris Medic.). Rom. Biotechnol. Lett. 15, 5223–5228.
Brown, J. R., Scanlan, J. C., and McIvor, J. G. (1998). Competition by herbs as a limiting factor in shrub invasion in grassland: a test with different growth forms. J. Veg. Sci. 9, 829–836. doi: 10.2307/3237048
Burg, W. J. V. J., Freitas, J. D., Debrot, A. O., and Lotz, L. A. P. (2012). Naturalized and invasive alien plant species in the Caribbean Netherlands: status, distribution, threats, priorities and recommendations: Plant Research International;part of Wageningen UR PRI report 437 Business Unit Agrosystems Research Imares report C185/11
Chen, R. -M., Liao, H. -X., Chen, W. -B., Wei, H. -J., and Peng, S. (2017). Role of allelopathy in plant invasion and control of invasive plants. Allelopathy J. Allelopathy Foundat. 41, 155–166. doi: 10.26651/2017-41-2-1092
Clark, K. (2017). Allelopathy: plant chemical warfare; Refuge Notebook _ 19(50):101_102; USFWS Kenai National Wildlife Refuge. Available online at: http://www.fws.gov/refuge/kenai/ or http://www.facebook.com/kenainationalwildliferefuge
Dar, B. A., Al-rowaily, S., Assaeed, A. M., El-bana, M. I., Hegazy, A. K., and Malik, J. A. (2017). Allelopathic potential of Argemone ochroleuca from different habitats on seed germination of native species and cultivated crops. Pak. J. Bot. 49, 1841–1848.
Doak, A., and Deveze, M. (2004). “Rubber vine management: control methods and case studies,” Queensland Department of Natural Resources, Mines and Energy, Brisbane.
Elisante, F., Tarimo, M. T., and Ndakidemi, P. A. (2013). Allelopathic effect of seed and leaf aqueous extracts of Datura stramonium on Leaf chlorophyll content, shoot and root elongation of Cenchrus ciliaris and Neonotonia wightii. Am. J. Plant Sci. 4, 2332–2339. doi: 10.4236/ajps.2013.412289
Favaretto, A., Scheffer-Basso, S. M., Felini, V., Neto, A., Zoch, A., and Carneiro, C. M. (2011). Growth of white clover seedlings treated with aqueous extracts of leaf and root of tough love-grass. R. Bras. Zootec. 40, 1168–1172. doi: 10.1590/S1516-35982011000600002
Ferguson, J. J., Rathinasabapathi, B., and Chase, C. A. (2016). Allelopathy: How Plants Suppress Other Plants;UF/IFAS Extension Service, University of Florida, IFAS, Florida A and M University Cooperative Extension Program, HS944. Available online at: http://edis.ifas.ufl.edu
HabtamuAshagre, A., TeferiTolesa, T., and Ibrahim, A. H. (2018). Allelopathic Potential of Niger (Guizotia abyssinica L.) on parthenium hysterophorus seed germination and seedling growth. Adv. J. Seed Sci. Technol. 5, 162–167.
Hassan, G., Rashid, H. U., Amin, A., Khan, I. A., and Shehzad, N. (2018). Allelopathic effect of Parthenium hysterophorus on germination and growth of some important crops and weeds of economic importance. Planta Daninha. 36, 1–11. doi: 10.1590/s0100-83582018360100132
Head, L., Atchison, J., and Phillips, C. (2015). The distinctive capacities of plants: re-thinking difference via invasive species. Transact. Instit. Br. Geograph. 40, 399–413. doi: 10.1111/tran.12077
Hu, G., and Zhang, Z. (2013). Allelopathic effects of Chromolaena odorata on native and non-native invasive herbs. J. Food Agri. Envion. 11, 878–882.
Hussain, F., Rasool, A., Aziz, K., Raisham, S., Aziz, S., Badshah, L., et al. (2020). Allelopathic inhibition of germination, seedling growth and cell division of selected plant species by Calotropis procera (Ait. ) Ait. Plant Sci. Today 7, 1–8. doi: 10.14719/pst2020.7.1.606
IBPGR (2012). International Board for Plant Genetic Resourcesfile:///D|/52/contents. htm[11/27/2012 11:26:47 AM]; Handbook of seed technology for gene bank No. 3.
ISTA (2005). International rules for seed testing, International Seed Testing Association. Zurich, Switzerland.
Javaid, A., Shafique, S., Bajwa, R., and Shafique, S. (2006). Effect of aqueous extracts of allelopathic crops on germination and growth of Parthenium hysterophorus L. South Afric. J. Bot. 72, 609–612. doi: 10.1016/j.sajb.2006.04.006
Kader, M. A. (2005). A comparison of seed germination calculation formulae and the associated interpretation of resulting data. J. Proceed. Royal Soc. NSW. 138, 65–75.
Kalisz, S., Kivlin, S. N., and Bialic-Murphy, L. (2021). Allelopathy is pervasive in invasive plants. Biol. Invas. 23, 367–371. doi: 10.1007/s10530-020-02383-6
Khan, M., Mohammad, I., Musharaf, S., Hussain, F., Ali, S. G., Hameed, I., et al. (2011). Effect of aqueous extracts of Chrozophora obliqua (Del) Juss on germination and seedling growth of Zea mays. Int. J. Biosci. 4, 94–99.
Laizer, H. C., Chacha, M. N., and Ndakidemi, P. A. (2021). Allelopathic effects of Sphaeranthus suaveolens on seed germination and seedling growth of Phaseolus vulgaris and Oryza sativa: Hindawi. Adv. Agric. 2021, 8882824. doi: 10.1155/2021/8882824
Luizza, M. W., Tewodros, W., Evangelista, P. H., and Jarnevich, C. S. (2016). Integrating local pastoral knowledge, participatory mapping, and species distribution modeling for risk assessment of invasive rubber vine (Cryptostegia grandiflora) in Ethiopia's Afar region. Ecol. Soc. 21, 22. doi: 10.5751/ES-07988-210122
Maharjan, S., Shrestha, B. B., and Jha, P. K. (2007). Allelopathic effects of aqueous extract of leaves of Parthenium hysterophorus l. on seed germination and seedling growth of some cultivated and wild herbaceous species. Scientific World 5, 33–39. doi: 10.3126/sw.v5i5.2653
Mehrafarin A. Badi H. N. Noormohammadi G. Zand E. Rezazadeh S. Qaderi A. (2011), Effects of environmental factors methanol on germination emergence of Trigonella foenum-graecum L. Afr. J. Agric. Res. 6, 4631–4641.
Moosavi, A., Afshari, R. T., Asadi, A., and Gharineh, M. H. (2011). Allelopathic Effects of aqueous extract of leaf stem and root of sorghum bicolor on seed germination and seedling growth of Vigna radiata. Not. Sci. Biol. 3, 114–118. doi: 10.15835/nsb325862
Moraa, O. L., Lucas, N., and Elmada, A. (2018). Allelopathic effect of Niger Plant (Guizotia abyssinica L.) on abundance of selected weeds. Emer. Life Sci. Res. 4, 38–44. doi: 10.31783/elsr.2018.423844
Mushtaq, W., and Siddiqui, M. B. (2018). Allelopathy in Solanaceae plants. J. Plant Protect. Res. 58, 1–7.
Negi, B., Bargali, S. S., Bargali, K., and Khatri, K. (2020). Allelopathic interference of Ageratum conyzoides L. against rice varieties. Curr. Agricult. Res. J. 8, 69–76. doi: 10.12944/CARJ.8.2.01
Nelson, L. S., Shih, R. D., and Balick, M. J. (2007). Handbook of poisonous and injurious plants; Institute of Economic Botany; the New York Botanical Garden; Springer Science New York, NY 10013, USA), springer. com, second edition.
Novak, N., Novak, M., Barić, K., Šćepanović, M., and Ivić, D. (2018). Allelopathic potential of segetal and ruderal invasive plants. J. Cent Euro. Agricul. 19, 408–422. doi: 10.5513/JCEA01/19 2. 2116
Oimbo, L. M., Auma, O. A., and Ngode, L. (2018). Influence of Niger plant (Guizotia abyssinica l.) on growth and development of common beans (Phaseolus vulgaris). RUFORUM Working Document Series. 17, 593–599.
Pisula, N. L., and Meiners, S. J. (2010). Relative allelopathic potential of invasive plant species in young disturbed woodland. J. Torrey Bot. Soc. 137, 81–87. doi: 10.3159/09-RA-040.1
Prasad, K. N., and Lavanya, K. (2017). A case of complete heart block due to intentional ingestion of plant extracts. Indian J. Cardiovascul. Dis. J. Women 12, 2.
Rana, S. S. (2018). Allelochemicals; Department of Agronomy, Csk Himachal Pradesh Krishi Vishvavidyalaya, Palampur, 176062, HP, India. Available online at: https://www.researchgate.net/publication/323077104
Ranal, M. A., and Santana, D. G. (2006). How and why to measure the germination process? Revista Brasil. Bot. 29:1–11. doi: 10.1590/S0100-84042006000100002
Rodriguez-Estrella, R., Navarro, J. P., Granados, B., and Rivera, L. (2010). The distribution of an invasive plant in a fragile ecosystem: the rubber vine (Cryptostegia grandiflora) in oases of the Baja California peninsula. Biol. Invas. 12, 3389–3393. doi: 10.1007/s10530-010-9758-z
Scavo, A., Abbate, C., and Mauromicale, G. (2019). Plant allelochemicals: agronomic, nutritional and ecological relevance in the soil system. Plant Soil 442, 23–48. doi: 10.1007/s11104-019-04190-y
Singh, A. A., Rajeswari, G., Nirmal, L. A., and Samuel, J. (2021). Synthesis and extraction routes of allelochemicals from plants and microbes: a review reviews. Analytic. Chemistr. 40, 293–311. doi: 10.1515/revac-2021-0139
Sitthinoi, P., Lertmongkol, S., Chanprasert, W., and Vajrodayab, S. (2017). Allelopathic effects of jungle rice (Echinochloa colona (L.) Link) extract on seed germination and seedling growth of rice. Agricult. Nat. Resour. 51, 74–78. doi: 10.1016/j.anres.2016.09.004
Stefanello, R., Viana, B. B., and Neves, L. A. S. (2017). Germination and vigor of linseed seeds under different conditions of light, temperature and water stress. Semina: Ciências Agrárias, Londrina 38, 1161–1168. doi: 10.5433/1679-0359.2017v38n3p1161
Thiébaut, G., Tarayre, M., and Rodríguez-Pérez, H. (2019). Allelopathic effects of native versus invasive plants on one major invader. Front. Plant Sci. 10, 1–10. doi: 10.3389/fpls 2019. 00854
Trezzia, M. M., Vidalb, R. A., Juniorc, A. A. B., Bittencourtd, H. H., and Filhoe, A. P. S. S. (2016). Allelopathy: driving mechanisms governing its activity in agriculture. J. Plant Interact. 11, 53–60. doi: 10.1080/17429145.2016.1159342
Turk, M. A., and Tawaha, A. M. (2003). Allelopathic Effects of Black Mustard (Brassica nigra) on Germination and Growth of Wild Barley (Hordeum spontaneum). J. Agronom. Crop Sci. 189, 298-−303. doi: 10.1046/j.1439-037X.2003.00047.x
Waris, A., Waris, L., Khan, M. A., and Shad, A. A. (2016). Allelopathic Effect of Methanol and Water Extracts of Camellia sinensis L. on seed germination and growth of Triticum aestivum L. and Zea maysL. J. Bio-resour. Manage. 3, 43. doi: 10.35691/JBM.6102.0043
Weston, L. A., Ryan, P. R., and Michelle Watt, M. (2012). Mechanisms for cellular transport and release of allelochemicals from plant roots into the rhizosphere. J. Experiment. Bot. 63, 3445–3454. doi: 10.1093/jxb/ers054
Witt, A., and Luke, Q. (2017). Guide to the Naturalized and Invasive Plants of Eastern Africa, ISBN-13: 978 1 78639 2145: Printed and bound by Gutenberg Press Ltd., Tarxien, Malta. CABI. Nosworthy Way. Wallingford, Oxford OX10 8DE UK:, JRS-Biodiversity, www.cabi.org
Keywords: concentration, control, inhibition, methanol, water
Citation: Seifu A, Lulekal E, Demissew S and Woldu Z (2023) Allelopathic potential of root and leaf aqueous extracts of invasive alien plant species, Cryptostegia grandiflora, on germination and seedling growth of Linum usitatissimum and Guizotia abyssinica. Front. For. Glob. Change 6:1131815. doi: 10.3389/ffgc.2023.1131815
Received: 26 December 2022; Accepted: 17 April 2023;
Published: 12 May 2023.
Edited by:
Anteneh Tesfaye Tekleyohannes, Ethiopian Environment and Forest Research Institute, EthiopiaReviewed by:
Sisay Asfaw, Ethiopian Institute of Agricultural Research (EIAR), EthiopiaShasho Megersa, Ethiopian Environment and Forest Research Institute, Ethiopia
Copyright © 2023 Seifu, Lulekal, Demissew and Woldu. This is an open-access article distributed under the terms of the Creative Commons Attribution License (CC BY). The use, distribution or reproduction in other forums is permitted, provided the original author(s) and the copyright owner(s) are credited and that the original publication in this journal is cited, in accordance with accepted academic practice. No use, distribution or reproduction is permitted which does not comply with these terms.
*Correspondence: Amare Seifu, YW1lc2VpZnUyMSYjeDAwMDQwO2dtYWlsLmNvbQ==