- 1School of Hydraulic and Ecological Engineering, Nanchang Institute of Technology, Nanchang, China
- 2Key Laboratory of Silviculture, Co-Innovation Center of Jiangxi Typical Trees Cultivation and Utilization, College of Forestry, Jiangxi Agricultural University, Nanchang, China
- 3Lushan Nature Reserve of Jiangxi (Lushan Mountain National Forest Ecological Station), Jiujiang, China
Aims: Wood decomposition plays an important role in ecosystem soil fertility and nutrient cycling, but how different forms of nitrogen (N) affect these biogeochemical processes is still unclear. The effects of N deposition on wood decomposition have been widely studied, but the decomposition process and biotic driver response to different forms of N have rarely been studied.
Methods: In this study, we conducted a two-year field factorial fertilization experiment with different N forms in a subtropical Chinese forest. Glycine and urea were selected as organic N (ON), and ammonium nitrate was selected as inorganic N (IN). Six different ratios (control, 0:10, 3:7, 5:5, 7:3, 10:0) of IN:ON with equal N amounts were uniformly added to the studied wood.
Results: We found that both forms of N deposition, i.e., ON and IN, accelerated the wood decomposition rates across the four studied species, and the magnitude of the increase was species specific. Mixed fertilizer with ON and IN resulted in the highest responses in the wood decomposition rate, which was 1.73- and 1.48-fold higher than that in the control and in response to IN addition alone across species. The ON + IN treatment resulted in the highest faunal and microbial community abundance of the decomposing wood.
Conclusion: In summary, our results indicate that different forms of anthropogenic N enrichment can promote wood decomposition through the modification of microbial and faunal communities in the wood decomposition process. Our results show that future studies need to consider N forms and components when estimating exogenous N deposition effects on the woody material nutrient cycle and terrestrial ecosystem carbon cycles.
1. Introduction
The amount of bioactive nitrogen (N) in the exogenous atmosphere due to anthropogenic activities has increased by three- to fivefold over several centuries (IPCC, 2007). In addition, the increasing rates of global N deposition are predicted to be two times higher over the next century under climate change (Lamarque et al., 2005). Moreover, a previous study found that the experimental estimated N deposition (19.6 Tg yr–1) was higher than that of a mathematical model (16.4 Tg yr–1) for China (Zhao et al., 2017; Yu et al., 2019). Therefore, the significantly increasing rates of N deposition could have profound consequences on ecosystem processes and functions, including wood decomposition (Van Groenigen et al., 2017; Wu et al., 2019a,2022; Wang et al., 2022).
The wood decomposition process plays a key role in nutrient cycling and the regulation of ecosystem biogeochemical cycles (Wu et al., 2019b,2021c; Harmon et al., 2020). Research has been conducted to clarify the effects of high levels of N fertilization on wood decomposition and the ecosystem carbon (C) cycle (Wu et al., 2019a,2022; Harmon et al., 2020; Wang et al., 2022). In addition, a growing body of literature has examined the influences of N fertilization on wood decomposition using only inorganic N, with the main form being ammonium nitrate (NH4NO3) (Wu et al., 2019a,2020). Atmospheric N deposition includes different forms, i.e., organic N [e.g., C5H9NO4, CO (NH2)2, C2H5NO2, ON] and inorganic N (e.g., NH4+, NH3, NO3–, and NOx, IN) (Neff et al., 2002; Cornell et al., 2003; Cornell, 2011). A previous study found that the contribution of organic N to total globally exogenous atmospheric N deposition accounted for approximately 30% (Cornell, 2011). Zhang et al. (2012) found that the average contributions of organic N deposition to total exogenous atmospheric N deposition reached 28% in China. Previous studies reported that ON was readily bioavailable, such as dissolved urea and amino acids, and ON has a higher dissolved bioavailability than IN (Peierls and Paerl, 1997). In addition, decomposer communities have a different and unequal response to IN and ON when equal amounts of N are added (Dong et al., 2020, 2022). To our knowledge, however, how different forms of N deposition and how mixed forms of N addition influence wood decomposition have not yet been studied.
The proportion of ON increases with increasing atmospheric N deposition, but few studies have studied the influence of mixed ON and IN addition on forest ecosystem functions and processes (Hobbie et al., 2012; Du et al., 2014; Dong et al., 2020, 2022). A previous study determined the influences of mixtures of different ON-to-IN ratios on fine root decomposition in grassland (Dong et al., 2020, 2022). However, our understanding of how different ON-to-IN ratios impact wood decomposition is unclear.
Wood decomposition, which plays an important role in nutrient and carbon cycling, is often ignored in forests. In addition, studies have focused on the mechanisms and patterns of wood decomposition in different forest ecosystems, and initial wood traits (tree species, wood diameter, density and decay stage) have been measured as the key factors influencing the decay process (Harmon et al., 1986, 2020; Wu et al., 2015, 2019b,2021c). These results could be explained by the differences in the initial wood quality (i.e., C, N content, lignin content and C/N ratio), colonization of ectomycorrhizae (Langley et al., 2003; See et al., 2019) and the ratio of the woody tissue to the epidermis (Chen et al., 2001). There are large differences in wood morphological traits and chemicals between conifers and broadleaf species; however, how wood decomposition responds to N addition in different forms and how decomposition varies with tree species are still largely unclear.
In this research, we designed a 24-month field fertilization experiment to determine the effects of N addition with different forms on the decomposition of wood and the associated fauna and microbial communities for four tree species. We aimed to test how wood decomposition responds to N deposition in different forms and how N fertilization effects vary with tree species. The specific hypotheses we tested were as follows: (1) N deposition in all forms (organic or inorganic) accelerates the decomposition of wood; (2) the individual effects of N fertilization (either individual organic or inorganic N fertilization) on wood decomposition are significantly lower than the combined effects of mixed ON and IN; (3) mixed N deposition changes the composition of soil organisms and induces more microbes and fauna to decompose the wood; and (4) the responses to different forms of N deposition are species specific.
2. Materials and methods
2.1. Study site
This field research was conducted in a mixed broadleaved forest (BLF) at Lu Mountain in Jiangxi Province, China (29°31′∼29°41′ N, 115°51′∼116°07′ E). The mean temperature and annual precipitation range from 17.1 to 11.6°C and from 1,308 to 2,068 mm, respectively (Wu et al., 2018a,2019b,2021a). The monthly rainfall and air temperature during the experimental period are shown in Figure 1. The soil types on Lu Mountain change from Haplic Alisols at high elevations to Ferric Alisols at low elevations according to the FAO (Food and Agriculture Organization) soil texture classification system (Liu and Wang, 2010; Wu et al., 2018b,2021b). The N deposition rates were predicted to reach a maximum value of 63.53 kg N ha–1 per year by 2030 in subtropical China (Lü and Tian, 2007). Four species, Cryptomeria japonica (L. f.) D. Don, Platycarya strobilacea Sieb. et Zucc, Pinus taiwanensis Hayata, and Lithocarpus glaber (Thunb.) Nakai., were selected. The soil properties (Appendix 1) and initial quality of the wood were measured using the experimental method described in (Section 2.4. “Chemical property measurements”) of this study.
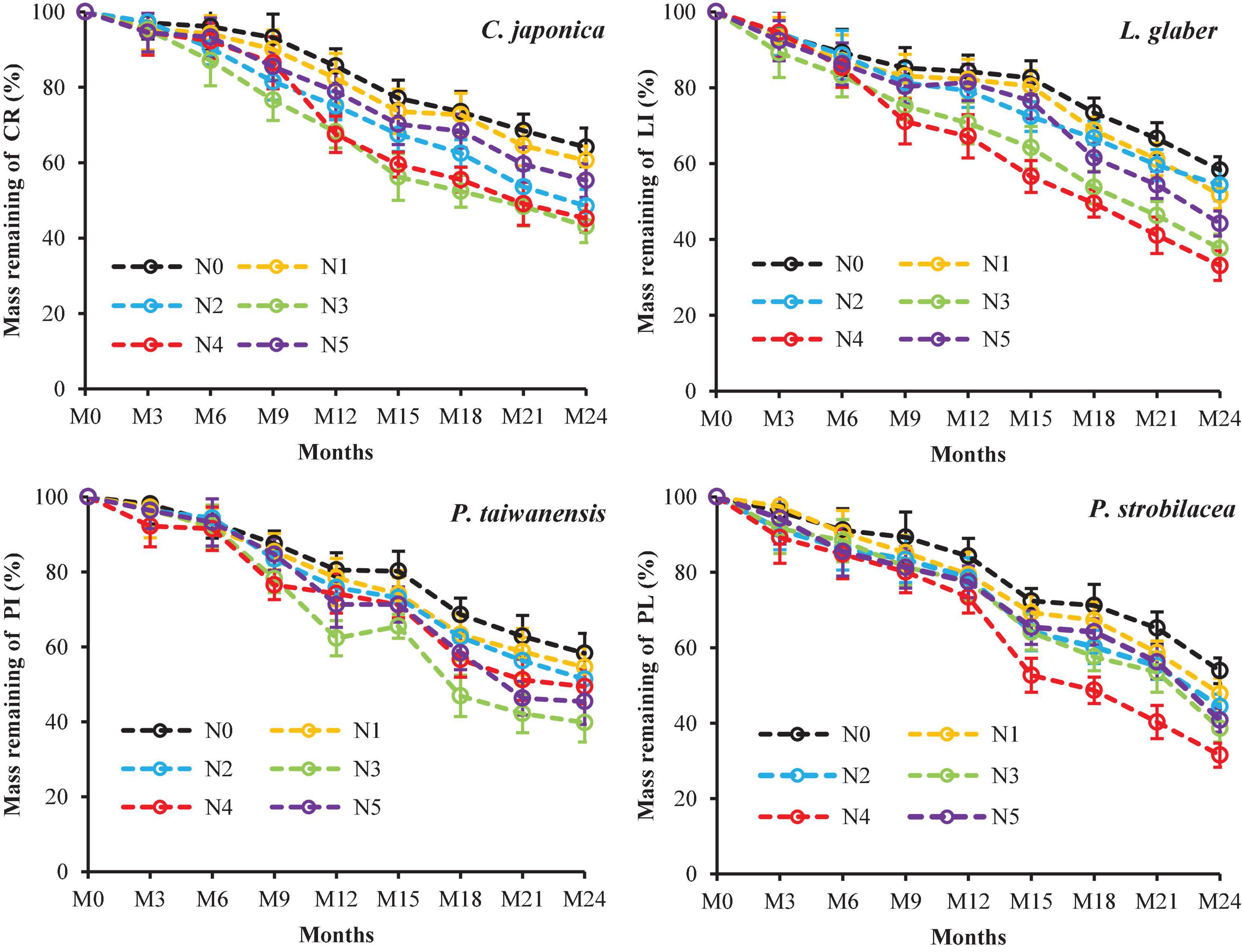
Figure 1. Changes in the mass remaining of the four studied species during 24 months of wood decomposition under different forms of N fertilizer. Error bars indicate the standard error (n = 6). N5, IN:ON = 0:10; N4, IN:ON = 3:7; N3, IN:ON = 5:5; N2, IN:ON = 7:3; N1, IN:ON = 10:0 and N0, control. Cryptomeria japonica (C. japonica), Platycarya strobilacea (P. strobilacea), Pinus taiwanensis (P. taiwanensis), and Lithocarpus glaber (L. glaber).
2.2. Experimental design
In October 2017, we randomly established 36 field plots (e.g., 6 m × 6 m) with six different N treatments (N5, IN:ON = 0:10; N4, IN:ON = 3:7; N3, IN:ON = 5:5; N2, IN:ON = 7:3; N1, IN:ON = 10:0 and N0, control) and six replicates at the field experimental site. Six subplots were set up with a size of 1 m × 1 m approximately 3 m apart in each 6 m × 6 m plot. In this study, the inorganic N source (IN) was NH4NO3, while the organic N source (ON) consisted of a mixture of equal proportions of glycine and urea. The control treatment was only sprayed with groundwater. Starting in January 2018, a total amount of 60 g N m–2 per year was sprayed onto plots using mixed N solutions in each month. For example, a 20 L mixed N solution comprising 1,734 g glycine (equivalent to 9.0 g ON m–2 per year), 696 g urea (equivalent to 9.0 g ON m–2 per year) and 4,320 g NH4NO3 (equivalent to 42 g IN m–2 per year) was used as the N2 treatment (IN:ON = 7:3). The relative contribution of exogenous organic N to the average total exogenous atmospheric N deposition was reported to be 28 and 30%, respectively, in China (Cornell, 2011), which is largely in line with the N2 treatment in this study.
2.3. Sampling design and wood decomposition
In November 2017, fresh woody material from the four dominant tree species in this study, namely, Cryptomeria japonica (C. japonica), Platycarya strobilacea (P. strobilacea), Pinus taiwanensis (P. taiwanensis), and Lithocarpus glaber (L. glaber), was collected from each of the four nonfertilized forest plots. In December 2017, we collected wood and soil [from the top layer (0–5 cm)] samples from each of the four discrete plots for the studied forest types. To achieve a constant weight, all fresh wood samples were air-dried in the laboratory for 1 month.
Wood decomposition in the BLF was determined by the mesh bag method (Verhoef and Brussaard, 1990; Wu et al., 2021a). The contributions of detritivores and microorganisms to the decomposition of wood were measured using nylon mesh bags (15 cm × 20 cm) with two different sizes. The fine 0.2 mm mesh bags allowed access only to microfauna and microorganisms, while the coarse 4 mm mesh bags allowed the passage of most meso- and macrofauna. Dried wood (fifty Grams) of the four studied species was added to the nylon mesh bags with different sizes, and to ensure direct contact between the mineral soil and the mesh bags, the humus and litter layer was completely removed from the field sample plots. The coarse and fine mesh bags were placed in the field plots in December 2017. Wooden screws were used to affix the mesh bags in position. A total of 1,152 mesh bags were used (4 species × 6 different N treatments × 6 replicates × 8 collection times).
The mesh bags of different sizes were collected for analysis every 3 months during the study period (e.g., M3, M6, M9, M12, M15, M18, M21, and M24). One hundred and forty-four mesh bags were analyzed in each collection period, and we also used a soil corer (5 cm diameter) to collect soil samples below the mesh bags. All of the collected samples were separated into two portions. One portion (approximately 10 g) was stored at −20°Cto examine the microbial community. The other portion was used to measure wood fauna and was dried at 60°C for 5 days to measure the moisture content. After the samples were crushed and sieved through a 2 mm mesh, the chemical properties were measured for each sampling time.
2.4. Chemical property measurements
A TOC analyzer (Vario TOC, Elementar, Germany) was used to measure the carbon fractions [e.g., AUR (acid unhydrolyzable residue, formerly referred to as lignin), cellulose and hemicellulose] of the wood and soil samples, and the Kjeldahl method (K-370, Buchi Scientific Instruments, Switzerland) was used to analyze the total N content. The total P content was measured using a molybdate blue reaction (Lu, 1999) with a UV-2450 spectrophotometer (Shimadzu Scientific Instruments, Japan). Soil-available P was extracted using a HCl-NH4F solution and was measured by molybdenum-antimony anti-colorimetric assay (Liu, 1996). Soil pH was measured using a Mettler-S20P-K pH meter (1:2.5, H2O).
2.5. Moisture and temperature measurements
The wood moisture content (Mwood) and wood temperature (Twood) were determined simultaneously over the course of wood decomposition. A hand-held long-stem thermometer (Model SK-250WP, Sato Keiryoki Mfg. Co. Ltd, Tokyo, Japan) was used to measure the wood temperature at a wood depth of approximately 2 cm. The wood moisture content (Mwood) at the time of each experimental measurement (8 collection times) was calculated by weight using equation (1).
where Mwood (%) is the moisture content of each wood sample during each mass loss measurement (8 collection times), and Wwt (g) and Wd (g) are the respective wet and dry weights of the wood during each mass loss measurement.
2.6. Wood PLFA analyses
In the laboratory, the wood samples were stored at −20°C for the analysis of the microbial community using phospholipid fatty acids (PLFAs). Microbial biomass and the relative index of bacteria to fungi were quantified and determined by PLFA analysis. The concentration of the PLFAs relative to the 19:0 internal standard was used to measure the concentration of each PLFA (ng g–1 dry wood samples). The total microbial community biomass was the sum of the PLFAs of all individual wood samples. Microbial groups, i.e., actinomycetes (ACT), Gram-positive bacteria (G+), Gram-negative bacteria (G–), bacteria (B), arbuscular mycorrhizal fungi (AMF), and fungi (F), were used as different biomarkers of the individual characteristic fatty acids (Appendix 2).
2.7. Extraction of wood fauna
Wood fauna were extracted using the dry funnel method (Tullgren funnel method) at each sampling time (Crossley and Blair, 1991; Carrillo et al., 2011; Wang et al., 2021). Alcohol (90%) was used to store all of the animals. The animals were counted using a binocular microscope, and we identified all animals to three subclass levels, Collembola, Termites and Acari (Faber, 1991; Lavelle, 1996; Chomel et al., 2015; Ji et al., 2020; Wang et al., 2021). All other invertebrates were further assigned to faunal functional groups following Moore et al. (2005). The proportion of wood fauna determines the abundance of each taxon to the abundance of all fauna per unit wood dry mass (per gram, g–1) (Ji et al., 2020; Wu et al., 2021a,b).
2.8. Statistical analyses
The wood mass loss data were transformed using the natural logarithm. The effects of different forms of N deposition, measurement month, and their interactions on the wood temperature, moisture content and mass loss of wood were analyzed using repeated-measures ANOVAs. Two-way ANOVAs were used to analyze the effects of tree species and different forms of N deposition on the wood temperature and moisture content, wood mass loss, and wood faunal and microbial communities. This was due to the significant interaction between the tree species and N deposition. One-way ANOVA with Dunnett’s post-hoc test was finally used to determine the effects of N fertilization with different forms on each tree species.
The remaining mass was expressed as a percentage of the initial dry wood mass. We calculated the wood decomposition rates following Olson (1963) with [Equation (2)]:
where the y value is the relative concentration between the remaining mass and the initial wood mass at interval t (measurement month), while the k value is the constant of the wood decay rate.
R version 4.0.3 (R Core Team, 2020) with a significance value of p < 0.05 was used to analyze all mass loss data. We analyzed the effects of different forms of N deposition, mesh sizes and wood species on wood mass loss using multiway ANOVAs. We also determined the effects of different forms of N deposition, experimental times, and tree species on the chemical properties and wood biota using repeated-measures ANOVA. The initial chemical properties of the wood were compared using one-way ANOVA.
The different decay constants (k) show the fertilizer N effects between different forms of N deposition and control treatments (organic N effect: kN5–kControl, inorganic N effect: kN1–kControl, mixed N effect: kaverage of N2,N3, N4–kControl; which is shown in Section “2.2. Experimental design”).
3. Results
3.1. Initial wood substrate quality
The initial wood quality (e.g., nutrients and C fractions) was significantly different between the four tree species before placement into the mesh bags (Table 1). The initial wood N concentration was the highest and lowest in Lithocarpus glaber and C. japonica, respectively; the order was L. glaber > P. strobilacea > P. taiwanensis > C. japonica (Table 1). In contrast, the C/N ratios of the wood were significantly different among the four tree species, with the order L. glaber < P. strobilacea < P. taiwanensis < C. japonica (Table 1).
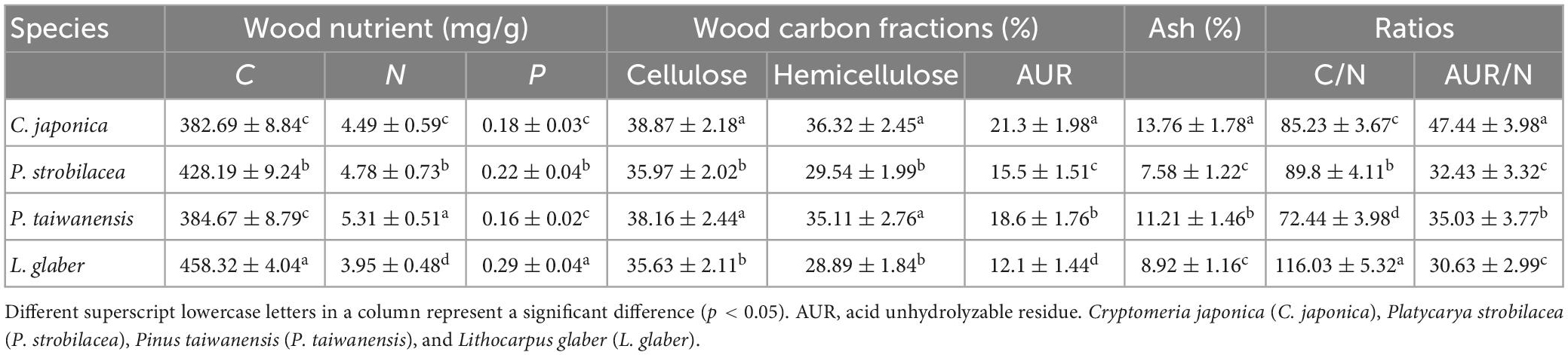
Table 1. Initial physiochemical properties and carbon fractions of the four types of wood (means ± SEs, n = 6).
The AUR, cellulose and hemicellulose concentrations of the wood were significantly different among the four studied species (p < 0.05). Cryptomeria japonica had the highest cellulose and hemicellulose concentrations, which were significantly higher than those in L. glaber and P. strobilacea. There was no significant difference between C. japonica and P. taiwanensis. The initial wood AUR concentrations were significantly different among the four studied species, with the highest and lowest in P. taiwanensis and L. glaber, respectively, with the order P. taiwanensis > C. japonica > P. strobilacea > Lithocarpus glaber (Table 1).
3.2. Wood mass loss and decomposition rates
Regardless of the N treatment, the mass loss of all four studied species of wood increased during the 24-month decomposition period (Figure 1). The wood mass loss was significantly influenced by the sampling time, N treatment, mesh type and wood species (Figure 1). Both the mesh type and wood species significantly affected the wood mass loss during the 24-month experimental period (p < 0.001, Figure 1). Wood decomposition rates were higher in the open mesh bag treatments than in the closed mesh bag treatments (Figure 1). In the open- and closed-mesh bags, the wood decomposition rates of the four study species increased due to N deposition in different forms, and the highest decomposition rate was observed in the N3 or N4 treatment (the mixtures of IN and ON) (Figure 2).
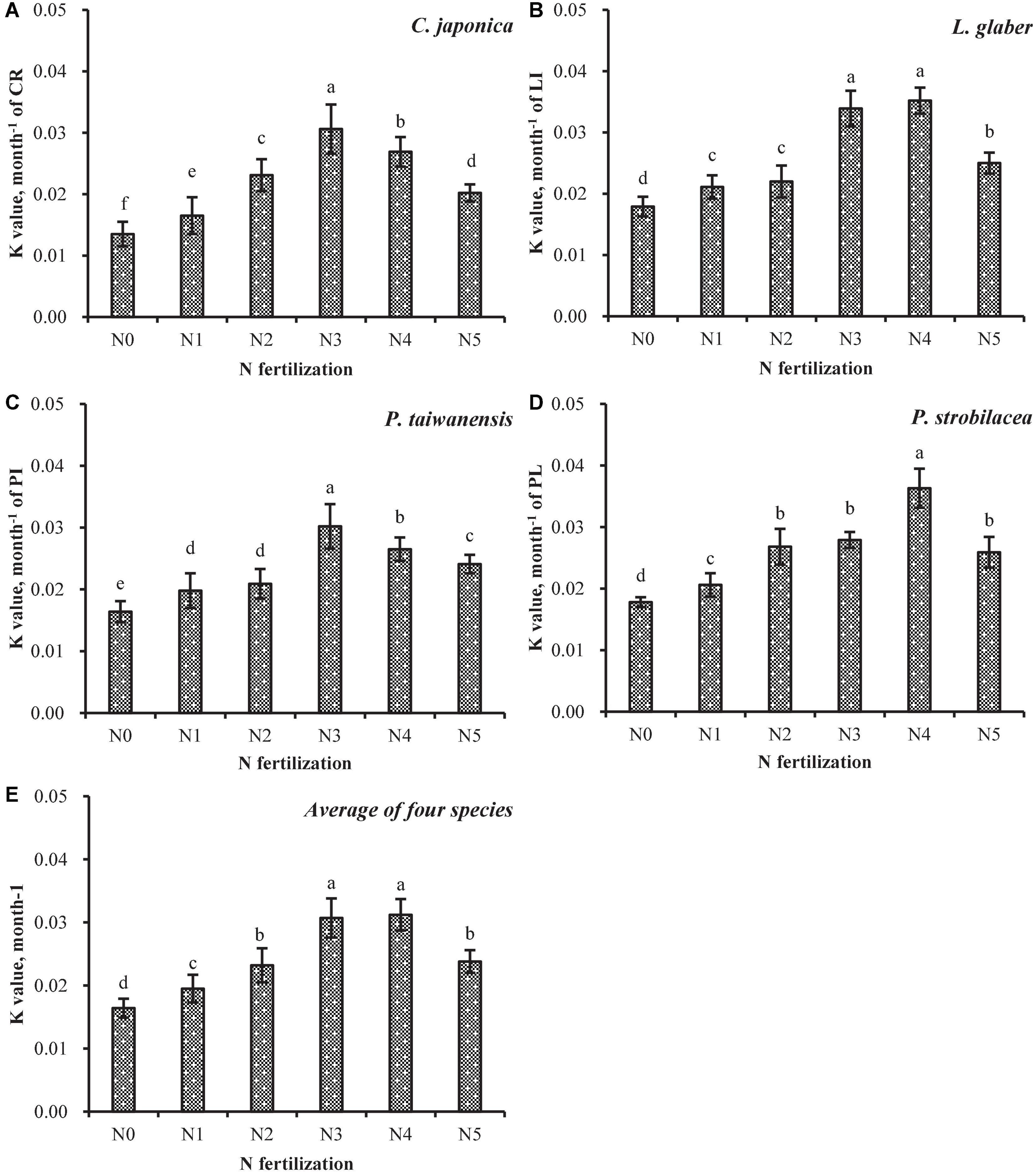
Figure 2. Wood decomposition rates (k) of the four studied species (A–D) and the average of the four species (E) for the different N fertilization treatments. Error bars indicate the standard error (n = 6). Bars with different letters differ significantly among different forms of N fertilizer at p < 0.05. N5, IN:ON = 0:10; N4, IN:ON = 3:7; N3, IN:ON = 5:5; N2, IN:ON = 7:3; N1, IN:ON = 10:0 and N0, Control. Cryptomeria japonica (C. japonica), Platycarya strobilacea (P. strobilacea), Pinus taiwanensis (P. taiwanensis), and Lithocarpus glaber (L. glaber).
The wood decomposition rates were significantly lower in the plots to which a single form of N was added (N5 and N1: ON and IN added individually) compared with the mixed N plots (N4, N3 or N2) (Figures 2A–D). The mixed N plots (N4, N3 or N2) significantly increased the wood decay rates, which were approximately 1. 19-, 1. 48-, and 1.73-fold higher than those in the ON, IN, and control treatments, respectively (average of all wood samples; Figure 2E). In addition, the mixed N treatments most significantly accelerated the wood decomposition rate of the four studied species (Figure 2E). However, the stimulation effects were weak, decreased or disappeared when only single-form N was added as ON to the four studied species (Figures 2A, B).
3.3. Substrate chemistry effects
In the control plots, the higher N concentration in L. glaber and P. strobilacea resulted in a higher wood decomposition rate compared with C. japonica and P. taiwanensis, which had a lower N concentration (Table 2). The wood decomposition rates were significantly positively related to the initial wood N concentration (Table 2) but significantly negatively related to the cellulose and hemicellulose concentrations (Table 2). In addition, the effects of the addition of mixed N (IN and ON) were significantly negatively correlated with the initial wood N concentration (R2 = 0.61, Figure 3G) but were not correlated with the initial wood AUR concentration (Figure 3H) among the four studied species. Meanwhile, compared with the mixed N treatments, the effect of the addition of an individual N source was not related to the initial wood N concentration and was not correlated with the initial wood AUR concentration (Figure 3).
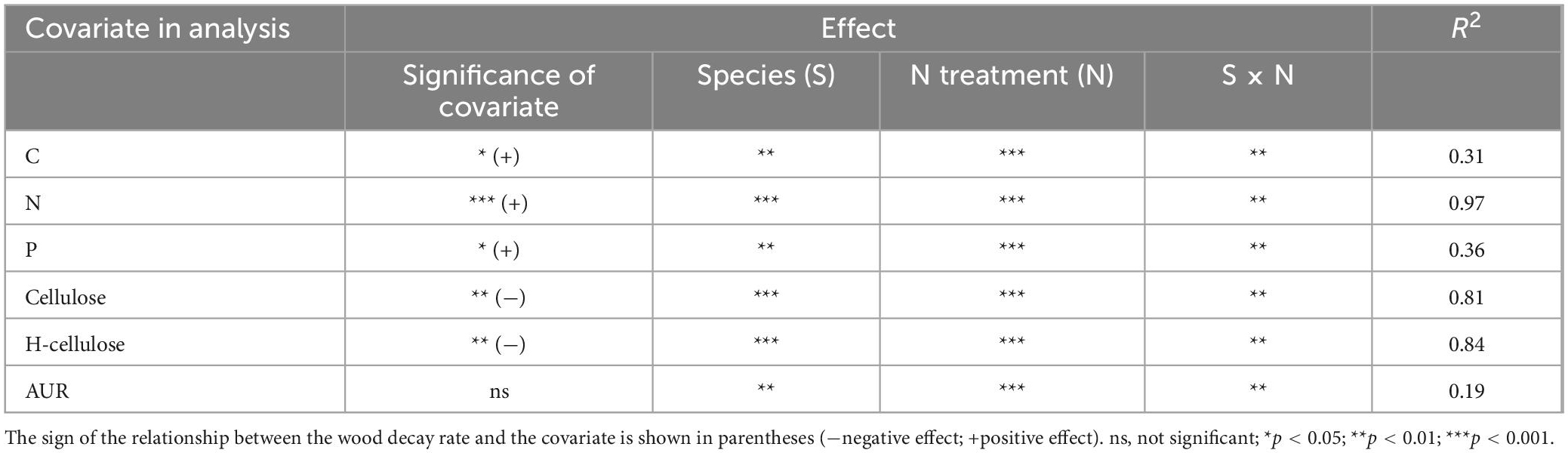
Table 2. The main effects of the N fertilizer treatment, species, and their interaction and the covariate effects of the initial substrate chemistry on the wood decomposition rate (k, month–1).
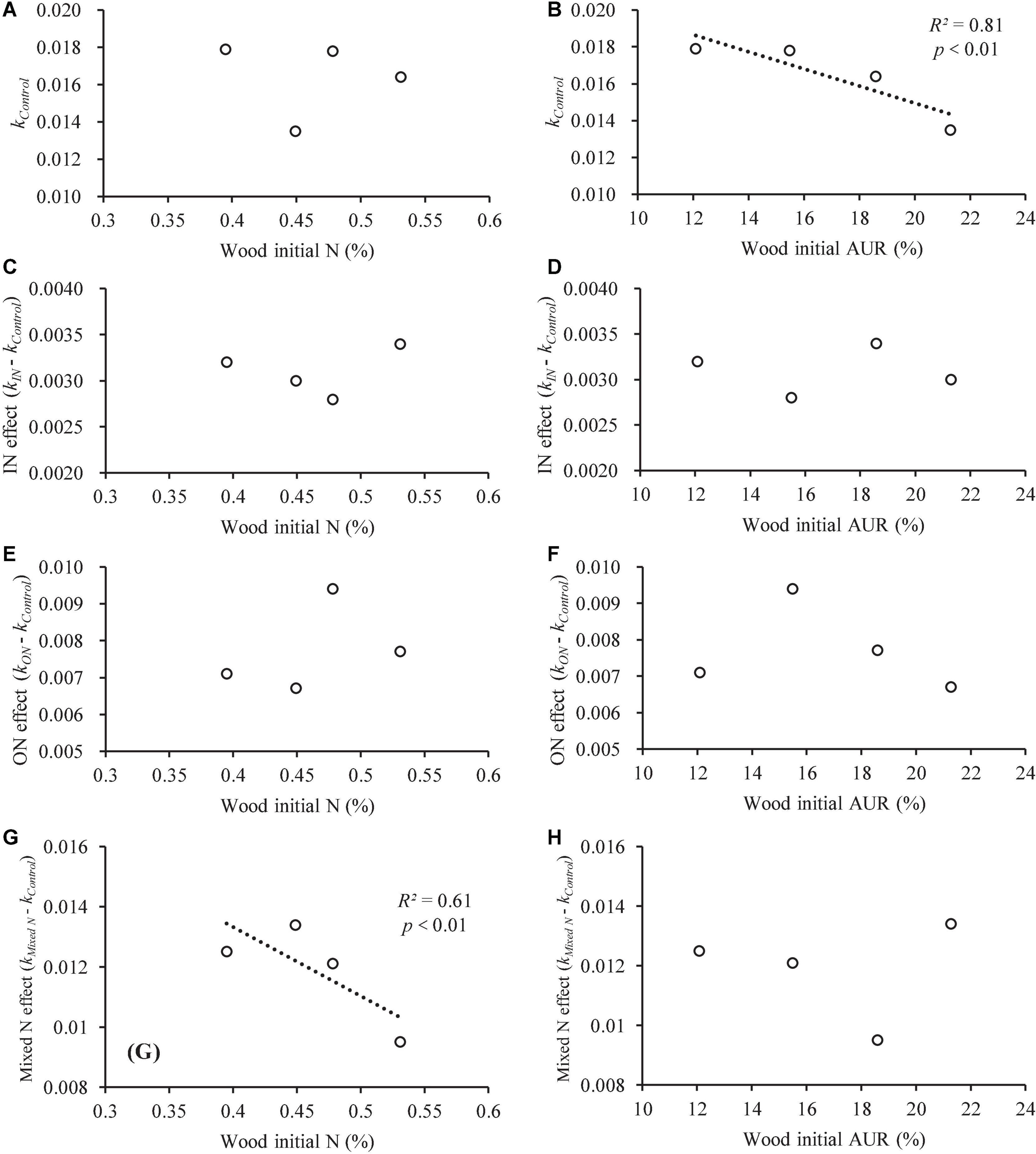
Figure 3. The linear regressions (p < 0.05) between the initial wood N and AUR concentrations and different forms of N fertilizer (average of six replicates for the four studied species). (A–H) Represent the linear regressions between kControl, N effect (kIN - kControl), ON effect (kON - kControl), Mixed N effect (kMixed N - kControl) and wood initial N (%), and wood initial AUR (%), respectively.
3.4. Changes in the wood microbial community
The microbial community of wood measured as PLFA distribution was significantly different between tree species and different forms of N deposition, with the broadleaf species (LI and PL) being higher than coniferous species (CR and PI) (Figure 4). Compared with the control, the concentrations of total PLFAs in the four species increased significantly in the N treatment (p < 0.05) and were highest in N3 for coniferous species and in N4 for broadleaf species (p < 0.05, Figure 4). With respect to bacteria and fungi, the N3 and N4 treatments led to the highest significant increase in PLFA concentrations for the coniferous and broadleaf species, respectively (Figure 4). Overall, the abundance of the wood microbial community varied with different forms of N deposition and tree species during the 24-month experimental period (Figure 4).
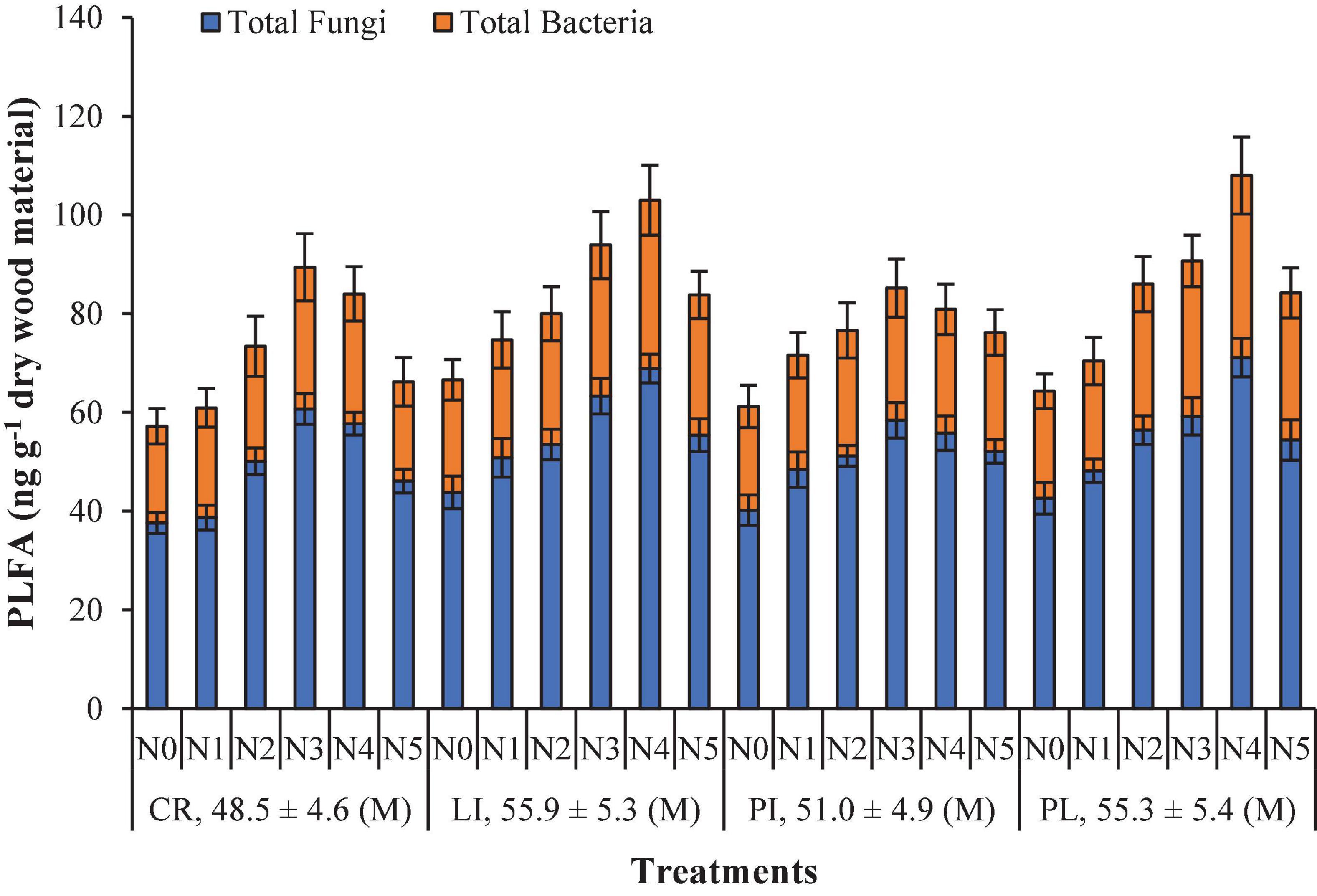
Figure 4. The phospholipid fatty acid (PLFA) (mean ± SE; ng g– 1 dry wood material) signatures of the four studied species under the different N fertilization treatments. Error bars indicate the standard error (n = 6). N5, IN:ON = 0:10; N4, IN:ON = 3:7; N3, IN:ON = 5:5; N2, IN:ON = 7:3; N1, IN:ON = 10:0 and N0, control. Cryptomeria japonica (CR), Platycarya strobilacea (PL), Pinus taiwanensis (PI) and Lithocarpus glaber (LI). M: mean value of different N fertilization treatments and the data including mean ± SE.
3.5. Changes in wood fauna
In all treatments, the dominant wood faunas were Acari (broadleaf species: proportion ranging between 28.4 and 76.4%; coniferous species: proportion ranging between 17.3 and 66.5%) and Collembola (broadleaf species: proportion ranging between 9.9 and 28.3%; coniferous species: proportion ranging between 7.4 and 16.9%) (Figure 5). Generally, the abundances of herbivores, carnivores, detritivorous mites and Collembola increased overall with different forms of N deposition (Figure 5). Lithocarpus glaber and P. strobilacea wood samples from the mixed N plots (N3 or N4) had a 1.75- and 1.80-fold higher soil faunal abundance than that in the single N-addition treatments (N1 and N5 treatments) (Figure 5). Cryptomeria japonica and P. taiwanensis samples from the mixed N plots (N3 or N4) had a 1.96- and 1.97-fold higher soil faunal abundance than that in the single N-addition treatments (N1 and N5 treatments) (Figure 5). In addition, the soil faunal abundance in the four wood samples was higher in the mixed N-addition treatments than in the single N-addition treatments (Figure 5).
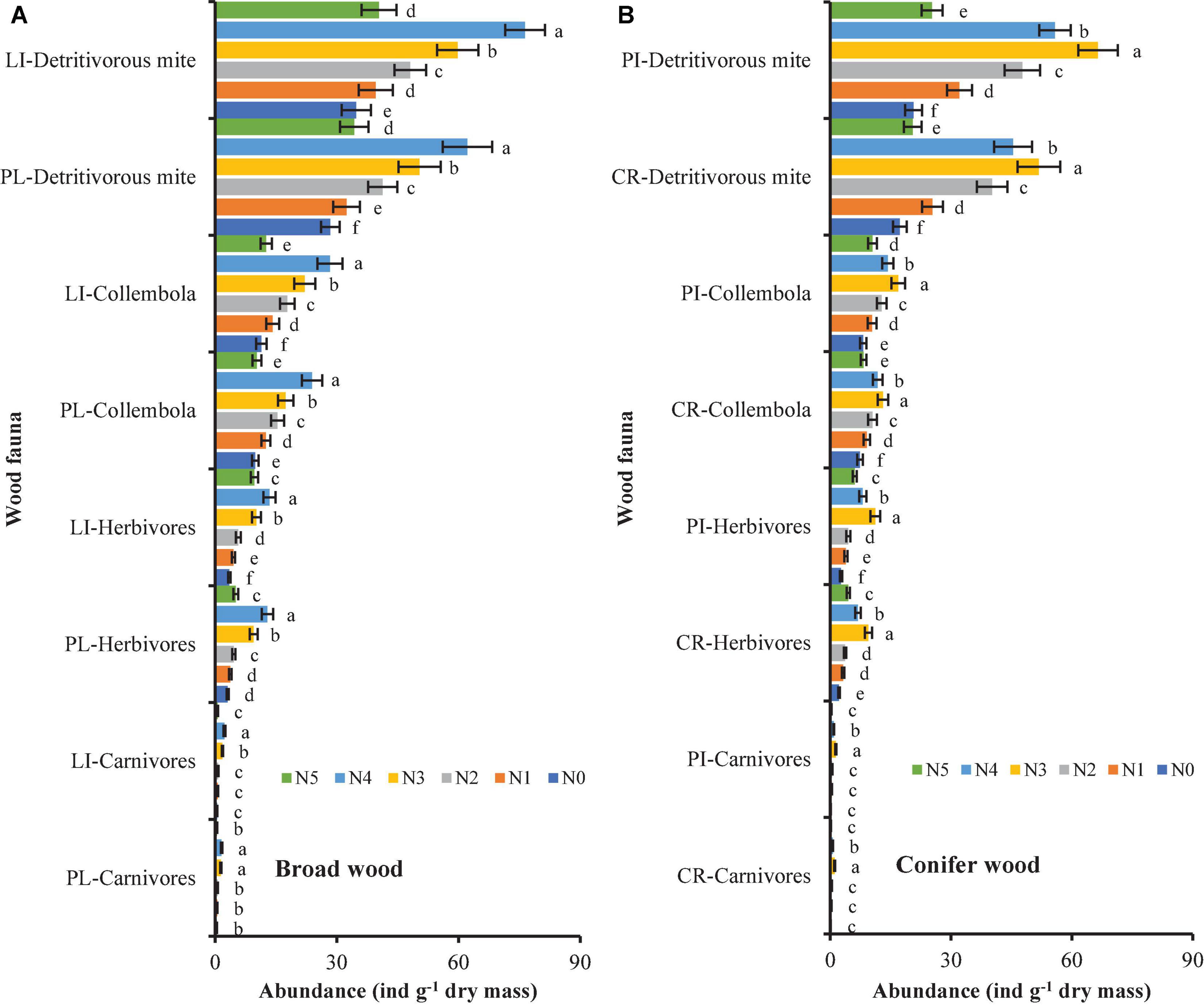
Figure 5. Change in the abundance of soil fauna functional groups present in (A) broadleaf (PL and LI) and (B) coniferous (CR and PI) wood samples under different N fertilization treatments over a 24-month experimental period. Error bars indicate the standard error (n = 6). Bars with different letters differ significantly among same wood fauna community and same tree species under different N fertilization treatments (N5, N4, N3, N2, N1 or N0) at p < 0.05. N5, IN:ON = 0:10; N4, IN:ON = 3:7; N3, IN:ON = 5:5; N2, IN:ON = 7:3; N1, IN:ON = 10:0 and N0, control. Cryptomeria japonica (CR), Platycarya strobilacea (PL), Pinus taiwanensis (PI) and Lithocarpus glaber (LI).
4. Discussion
During the 2-year experimental period, regardless of the form (organic N or inorganic N), exogenous N deposition accelerated the wood decomposition of the four species. In addition, the magnitude of the exogenous N effects varied with N form, with the mixed N (mixtures of organic N and inorganic N) resulting in the fastest wood decomposition rates. Therefore, the finding in this study is very interesting, as organic N is an important component in exogenous N deposition, which demonstrates that when evaluating and simulating atmospheric N deposition (Cornell, 2011), we should not ignore the important role of organic N deposition. The result supports hypothesis 1 that N deposition in both forms (organic or inorganic) accelerates wood decomposition by enhancing wood faunal and microbial abundance. To our knowledge, however, how different forms of N addition impact wood decomposition and the different effects of microorganisms and fauna on decomposing wood have not yet been studied (Hobbie, 2008; Wu et al., 2022).
Consistent with previous studies, we observed that the addition of N alone (ON or IN) positively promoted the decomposition of dead organic matter. For example, previous studies revealed that the initial decomposition rate in different forest ecosystems was stimulated by external N deposition (Hobbie, 2005, 2015; Hobbie et al., 2012). Wu et al. (2020, 2022) also found that short-term N fertilization had a stimulatory effect on wood decomposition. In this study, the large stimulatory effects of different forms of N deposition on wood decomposition can be explained by the variation in the faunal (e.g., insects and termites) and microbial communities and the soil physicochemical properties due to exogenous N fertilization (Seibold et al., 2021; Zanne et al., 2022). In turn, the activity of hydrolytic enzymes would also be promoted (Berg, 2014; Sun et al., 2016; Dong et al., 2019; Wu et al., 2022). For example, our previous study showed that the activities of cellobiohydrolase and β-1,4-glucosidase increased in decomposing wood under inorganic N fertilization treatments in a subtropical Chinese forest (Wu et al., 2020). However, when exogenous N deposition reaches N saturation, it may limit other available nutrients involved in microbial activity metabolism (Griffiths et al., 2012), thus leading to a negative influence on the decomposition of wood (Wang et al., 2022; Wu et al., 2022). Therefore, in this research, the stimulatory effects of N deposition on the abundance of the microbial and faunal communities may partly explain the significant stimulatory effect of different forms of N deposition on wood decomposition.
Our study is the first to test how mixed N-addition treatments affect wood decomposition, and the results indicated that the mixed N-addition treatments accelerated the rates of wood decomposition more than single N (IN or ON) treatments across the four species. The result supports hypothesis 2 that the combined effects of mixed ON and IN on wood decomposition would be significantly higher than the individual effects. These results are probably due to the sources of mixed N fertilizer satisfying the growth demands of higher diversity groups of biota decomposer communities compared with single N (IN or ON) fertilizer sources (Hobbie, 2005). In this study, the abundance of the microbial and faunal communities increased significantly in the mixed N plots compared with the single N plots (Figures 4, 5). This study further revealed that although the same amount of exogenous N was added, the effect of ON fertilizer on the decomposer communities was greater than that of IN fertilizer (Wang et al., 2011; Du et al., 2014; Hobbie, 2015). Lochhead and Chase (1943) and Hobbie (2005) found that different forms of N meet the demands of different types of decomposers; some special decomposers are unable to utilize a single IN source and need fertilizers with ON, such as amino acids. These results support hypothesis 3 that mixed-N deposition induced more soil fauna to decompose wood.
We observed that single N addition (ON or IN) had a greater effect on the wood decomposition of species with lower N concentrations (Figure 2 and Table 1). The decomposition rate was higher in L. glaber and P. strobilacea than that in C. japonica and P. taiwanensis with single N addition (ON or IN). This is probably because the decomposer communities in low-N wood species have a higher N demand than those in high-N wood species (Dong et al., 2020; Wu et al., 2022). In addition, in this study, the abundance of the decomposer community was higher in broadleaf species with lower N concentrations than in coniferous species with higher N concentrations (Figures 4, 5). In the control treatment, the significantly negative relationship between the decomposition rate and substrate AUR concentration supported the above finding (Figure 3B). Previous studies also demonstrated that the impact of N fertilization on wood decomposition was significantly correlated with the lignin concentration of the woody material in different regions and ecosystems (Waldrop et al., 2004a,b; Knorr et al., 2005; Hobbie, 2015). The results above support hypothesis 4 of this study that the effect of different forms of N deposition on wood decomposition is species specific.
Interestingly, in contrast to single IN or ON fertilizer, this study found that the influences of mixed N fertilizer were significantly negatively correlated with the wood N concentrations (Figure 3G). This result may be due to the mixed N treatments meeting the demands of the decomposer community compared with only ON, IN or substrate N alone (Hobbie, 2005). To further determine whether the results of this study are generally suitable and applicable to subtropical forests, future studies should test more tree species with their wood in different stages of decomposition.
5. Conclusion
This study showed that all forms of N deposition (ON and IN) increased the wood decomposition rates of all of the studied species by enhancing wood faunal and microbial abundance. The mixed N deposition had a greater effect on the wood decomposition rate compared with the individual effects of IN or ON fertilizer. Therefore, if we ignore the important role of organic N sources when simulating the N fertilization effect, we could underestimate the influence of N fertilization on biogeochemical cycling and primary productivity in terrestrial ecosystems. However, we must note that all of the results observed in our study in response to the different forms of N fertilizer were only during the initial decay period. A long-term study could be better suited to understand the influence of different forms of N deposition and the potential driving mechanism underlying wood decomposition and the forest carbon cycle.
Data availability statement
The raw data supporting the conclusions of this article will be made available by the authors, without undue reservation.
Author contributions
CW, CS, XY, BD, FS, YZ, and YL were responsible for study design, data collection and analysis, and writing the early drafts of this research. CW and YL substantially and equally contributed to further interpreting results and revising this manuscript. All authors contributed to the article and approved the submitted version.
Funding
This study was financially supported by the Jiangxi Provincial Natural Science Foundation (20224BAB203051 and 20212BAB213022), National Natural Science Foundation of China (31901292, 31960303, and 32201368), and Jiangxi Provincial Department of Education Foundation (GJJ211903).
Acknowledgments
We are grateful to the Lushan Mountain National Forest Ecological Station for providing the study sites. We thank the two reviewers and the editor of the journal for their suggestions on improving this manuscript.
Conflict of interest
The authors declare that the research was conducted in the absence of any commercial or financial relationships that could be construed as a potential conflict of interest.
Publisher’s note
All claims expressed in this article are solely those of the authors and do not necessarily represent those of their affiliated organizations, or those of the publisher, the editors and the reviewers. Any product that may be evaluated in this article, or claim that may be made by its manufacturer, is not guaranteed or endorsed by the publisher.
References
Berg, B. (2014). Decomposition patterns for foliar litter-A theory for influencing factors. Soil Biol. Biochem. 78, 222–232.
Carrillo, Y., Ball, B. A., Bradford, M. A., Jordan, C. F., and Molina, M. (2011). Soil fauna alter the effects of litter composition on nitrogen cycling in a mineral soil. Soil Biol. Biochem. 43, 1440–1449.
Chen, H., Harmon, M. E., and Griffths, R. P. (2001). Decomposition and nitrogen release from decomposing woody roots in coniferous forests of the Pacific Northwest: A chronosequence approach. Can. J. For. Res. 31, 246–260.
Chomel, M., Guittonny-Larcheveque, M., DesRochers, A., and Baldy, V. (2015). Home field advantage of litter decomposition in pure and mixed plantations under boreal climate. Ecosystems 18, 1014–1028.
Cornell, S. E. (2011). Atmospheric nitrogen deposition: Revisiting the question of the importance of the organic component. Environ. Pollut. 159, 2214–2222. doi: 10.1016/j.envpol.2010.11.014
Cornell, S. E., Jickells, T. D., Cape, J. N., Rowland, A. P., and Duce, R. A. (2003). Organic nitrogen deposition on land and coastal environments: A review of methods and data. Atmos. Environ. 37, 2173–2191.
Crossley, D. A., and Blair, J. M. (1991). A high-efficiency, ‘low-technology’ Tullgren-type extractor for soil microarthropods. Agric. Ecosyst. Environ. 34, 187–192. doi: 10.1016/0167-8809(91)90104-6
Dong, L. L., Berg, B., Sun, T., Wang, Z. W., and Han, X. G. (2020). Response of fine root decomposition to different forms of N deposition in a temperate grassland. Soil Biol. Biochem. 2020:107845. doi: 10.1016/j.soilbio.2020.107845
Dong, L., Berg, B., Gu, W., Wang, Z., and Sun, T. (2022). Effects of different forms of nitrogen addition on microbial extracellular enzyme activity in temperate grassland soil. Ecol. Process. 11, 1–8. doi: 10.1186/s13717-022-00380-2
Dong, L., Sun, T., Berg, B., Zhang, L., Zhang, Q., and Wang, Z. (2019). Effects of different forms of N deposition on leaf litter decomposition and extracellular enzyme activities in a temperate grassland. Soil Biol. Biochem. 134, 78–80. doi: 10.1016/j.soilbio.2019.03.016
Du, Y. H., Guo, P., Liu, J. Q., Wang, C. Y., Yang, N., and Jiao, Z. X. (2014). Different types of nitrogen deposition show variable effects on the soil carbon cycle process of temperate forests. Glob. Change Biol. 20, 3222–3228. doi: 10.1111/gcb.12555
Faber, J. H. (1991). Functional classification of soil fauna-a new approach. Oikos 62, 110–117. doi: 10.2307/3545458
Frostegård, Å, and Bååth, E. (1996). The use of phospholipid fatty acid analysis to estimate bacterial and fungal biomass in soil. Biol. Fertil. Soils 22, 59–65. doi: 10.1007/BF00384433
Frostegård, A., Tunlid, A., and Bååth, E. (2011). Use and misuse of PLFA measurements in soils. Soil Biol. Biochem. 43, 1621–1625. doi: 10.1016/j.soilbio.2010.11.021
Griffiths, B. S., Spilles, A., and Bonkowski, M. (2012). C:N:P stoichiometry and nutrient limitation of the soil microbial biomass in a grazed grassland site under experimental P limitation or excess. Ecol. Process. 1:6. doi: 10.1186/2192-1709-1-6
Harmon, M. E., Fasth, B. G., Yatskov, M., Kastendick, D., Rock, J., and Woodall, C. W. (2020). Release of coarse woody detritus-related carbon: A synthesis across forest biomes. Carbon Balance Manag. 15, 1–21. doi: 10.1186/s13021-019-0136-6
Harmon, M. E., Franklin, J. F., Swanson, F. J., Sollins, P., Gregory, S. V., Lattin, J. D., et al. (1986). Ecology of coarse woody debris in temperate ecosystems. Adv. Ecol. Res. 15, 133–302.
Hobbie, S. E. (2005). Contrasting effects of substrate and fertilizer nitrogen on the early stages of litter decomposition. Ecosystems 8, 644–656.
Hobbie, S. E. (2008). Nitrogen effects on decomposition: A five-year experiment in eight temperate sites. Ecology 89, 2633–2644. doi: 10.1890/07-1119.1
Hobbie, S. E. (2015). Plant species effects on nutrient cycling: Revisiting litter feedbacks. Trends Ecol. Evol. 30, 357–363. doi: 10.1016/j.tree.2015.03.015
Hobbie, S. E., Eddy, W. C., Buyarski, C. R., Adair, E. C., Ogdahl, M. L., and Weisenhorn, P. (2012). Response of decomposing litter and its microbial community to multiple forms of nitrogen enrichment. Ecol. Monogr. 82, 389–405. doi: 10.1890/11-1600.1
IPCC (2007). Climate change 2007: The physical science basis. Cambridge: Cambridge University Press.
Ji, Y. L., Li, Q., Tian, K., Yang, J. B., Hu, H. J., Yuan, L. H., et al. (2020). Effect of sodium amendments on the home-field advantage of litter decomposition in a subtropical forest of China. Forest Ecol. Manag. 468:118148. doi: 10.1016/j.foreco.2020.118148
Knorr, M., Frey, S. D., and Curtis, P. S. (2005). Nitrogen additions and litter decomposition: A meta-analysis. Ecology 86, 3252–3257. doi: 10.1890/05-0150
Lamarque, J. F., Kiehl, J. T., Brasseur, G. P., Butler, T., Cameron-Smith, P., Collins, W. D., et al. (2005). Assessing future nitrogen deposition and carbon cycle feedback using a multi-model approach: Analysis of nitrogen deposition. J. Geophys. Res. 110:D19303. doi: 10.1029/2005JD005825
Langley, J. A., Dijkstra, P., Drake, B. G., and Hungate, B. A. (2003). Ectomycorrhizal colonization, biomass, and production in a regenerating scrub oak forest in response to elevated CO2. Ecosystems 6, 424–430. doi: 10.1007/PL00021509
Liu, G. S. (1996). Soil physical and chemical analysis and description of cross-section. Beijing: China Standard Press.
Liu, X. Z., and Wang, L. (2010). Scientific survey and study of biodiversity on the Lushan Nature Reserve in Jiangxi Province. Beijing: Science Press.
Lochhead, A. G., and Chase, F. E. (1943). Nutritional requirements of the predominant bacterial flora. Soil Sci. 55, 185–195. doi: 10.1097/00010694-194302000-00007
Lü, C. Q., and Tian, H. Q. (2007). Spatial and temporal patterns of nitrogen deposition in China: Synthesis of observational data. J. Geophys. Res. 112, 10–15. doi: 10.1029/2006JD007990
Lu, R. K. (1999). Analysis methods of soil science and agricultural chemistry. Beijing: Agricultural Science and Technology Press.
Moore, J. C., McCann, K., and de Ruiter, P. C. (2005). Modeling trophic pathways, nutrient cycling, and dynamic stability in soils. Pedobiologia 49, 499–510. doi: 10.1016/j.pedobi.2005.05.008
Neff, J. C., Holland, E. A., Dentener, F. J., McDowell, W. H., and Russell, K. M. (2002). The origin, composition and rates of organic nitrogen deposition: A missing piece of the nitrogen cycle? Biogeochemistry 57, 99–136. doi: 10.1007/978-94-017-3405-9_3
Olson, J. S. (1963). Energy-storage and balance of producers and decomposers in ecological-systems. Ecology 44, 322–331. doi: 10.2307/1932179
Olsson, P. A., Jakobsen, I., Thingstrup, I., and Bååth, E. (1999). Estimation of the biomass of arbuscular mycorrhizal fungi in a linseed field. Soil Biol. Biochem. 31, 1879–1887. doi: 10.1016/S0038-0717(99)00119-4
Peierls, B. L., and Paerl, H. W. (1997). Bioavailability of atmospheric organic nitrogen deposition to coastal phytoplankton. Limnol. Oceanogr. 42, 1819–1823. doi: 10.3389/fmicb.2022.915255
R Core Team (2020). R: A language and environment for statistical computing. Vienna: R Foundation for Statistical Computing.
See, C. R., McCormack, M. L., Hobbie, S. E., Flores-Moreno, H., Silver, W. L., and Kennedy, P. G. (2019). Global patterns in fine root decomposition: Climate, chemistry, mycorrhizal association and woodiness. Ecol. Lett. 22, 946–953. doi: 10.1111/ele.13248
Seibold, S., Rammer, W., Hothorn, T., Seidl, R., Ulyshen, M. D., Lorz, J., et al. (2021). The contribution of insects to global forest deadwood decomposition. Nature 597, 77–81.
Sun, T., Dong, L., Wang, Z., Lü, X., and Mao, Z. (2016). Effects of long-term nitrogen deposition on fine root decomposition and its extracellular enzyme activities in temperate forests. Soil Biol. Biochem. 93, 50–59.
Van Groenigen, J. W., Van Kessel, C., Hungate, B. A., Oenema, O., Powlson, D. S., and Van Groenigen, K. J. (2017). Sequestering soil organic carbon: A nitrogen dilemma. Environ. Sci. Technol. 51, 11503–11504.
Verhoef, H. A., and Brussaard, L. (1990). Decomposition and nitrogen mineralization in natural and agroecosystems – The contribution of soil animals. Biogeochemistry 11, 175–211.
Waldrop, M. P., Zak, D. R., and Sinsabaugh, R. L. (2004a). Microbial community response to nitrogen deposition in Northern forest ecosystems. Soil Biol. Biochem. 36, 1443–1451.
Waldrop, M. P., Zak, D. R., Sinsabaugh, R. L., Gallo, M., and Lauber, C. (2004b). Nitrogen deposition modifies soil carbon storage through changes in microbial enzymatic activity. Ecol. Appl. 14, 1172–1177.
Wang, B., Verheyen, K., Baeten, L., and De Smedt, P. (2021). Herb litter mediates tree litter decomposition and soil fauna composition. Soil Biol. Biochem. 152:108063.
Wang, C., Han, G., Jia, Y., Feng, X., Guo, P., and Tian, X. (2011). Response of litter decomposition and related soil enzyme activities to different forms of nitrogen fertilization in a subtropical forest. Ecol. Res. 26, 505–513.
Wang, H., Wu, C., Liu, J., Chen, Q., Li, C., Shu, C., et al. (2022). Changes in soil microbial communities induced by warming and N deposition accelerate the CO2 emissions of coarse woody debris. J. For. Res. 28, 1–13.
Wu, C. S., Mo, Q. F., Wang, H. K., Zhang, Z. J., Huang, G. X., Ye, Q., et al. (2018a). Moso bamboo (Phyllostachys edulis (Carriere) J. Houzeau) invasion affects soil phosphorus dynamics in adjacent coniferous forests in subtropical China. Ann. For. Sci. 75:24.
Wu, C. S., Zhang, Z. J., Wang, H. K., Li, C., Mo, Q. F., and Liu, Y. Q. (2018b). Photodegradation accelerates coarse woody debris decomposition in subtropical Chinese forests. For. Ecol. Manag. 409, 225–232.
Wu, C. S., Shu, C. J., Zhang, Z. J., Li, Y. Y., Zhang, Y., and Liu, Y. Q. (2021c). Forest fragmentation slows the decomposition of coarse woody debris in a subtropical forest. For. Sci. 67, 682–693.
Wu, C. S., Ulyshen, M., Shu, C. J., Zhang, Z. J., Zhang, Y., Liu, Y. Q., et al. (2021a). Stronger effects of termites than microbes on wood decomposition in a subtropical forest. For. Ecol. Manag. 493:119263.
Wu, C. S., Shu, C. J., Zhang, Z. J., Zhang, Y., and Liu, Y. Q. (2021b). Phosphorus deposition accelerates wood decomposition and temperature sensitivity in a subtropical forest. Ecol. Indic. 128:107819.
Wu, C. S., Shu, C. J., Zhang, Z. J., Zhang, Y., and Liu, Y. Q. (2022). Effect of N deposition on the home-field advantage of wood decomposition in a subtropical forest. Ecol. Indic. 140:109043.
Wu, C. S., Zhang, Z. J., Wang, H. K., Huang, G. X., Shu, C. J., Kong, F. Q., et al. (2019b). Home-field advantage of CWD decomposition in subtropical forests varied by field sites. For. Ecol. Manag. 444, 127–137.
Wu, C. S., Wang, H. K., Mo, Q. F., Zhang, Z. J., Huang, G. X., Kong, F. Q., et al. (2019a). Effects of elevated UV-B radiation and N deposition on the decomposition of coarse woody debris. Sci. Total Environ. 663, 170–176. doi: 10.1016/j.scitotenv.2019.01.271
Wu, C. S., Wei, X. H., Mo, Q. F., Li, Q. L., Li, X. D., Shu, C. J., et al. (2015). Effects of stand origin and near-natural restoration on the stock and structural composition of fallen trees in mid-subtropical forest. Forests 6, 4439–4450.
Wu, C. S., Zhang, Z. J., Shu, C. J., Mo, Q. F., Wang, H. K., Kong, F. Q., et al. (2020). The response of coarse woody debris decomposition and microbial community to nutrient additions in a subtropical forest. For. Ecol. Manag. 460:117799.
Yu, G. R., Jia, Y. L., He, N. P., Zhu, J. X., Chen, Z., Wang, Q. F., et al. (2019). Stabilization of atmospheric nitrogen deposition in China over the past decade. Nat. Geosci. 12, 424–429.
Zak, D. R., Ringelberg, D. B., Pregitzer, K. S., Randlett, D. L., White, D. C., and Curtis, P. S. (1996). Soil microbial communities beneath Populus grandidentata grown under elevated atmospheric CO2. Ecol. Appl. 6, 257–262.
Zanne, A. E., Flores-Moreno, H., Powell, J. R., Cornwell, W. K., Dalling, J. W., Austin, A. T., et al. (2022). Termite sensitivity to temperature affects global wood decay rates. Science 377, 1440–1444. doi: 10.1126/science.abo3856
Zelles, L. (1999). Fatty acid patterns of phospholipids and lipopolysaccharides in the characterisation of microbial communities in soil: A review. Biol. Fertility Soils 29, 111–129.
Zhang, Y., Song, L., Liu, X. J., Li, W. Q., Lü, S. H., Zheng, L. X., et al. (2012). Atmospheric organic nitrogen deposition in China. Atmos. Environ. 46, 195–204.
Zhao, Y. H., Zhang, L., Chen, Y. F., Liu, X. J., Xu, W., Pan, Y. P., et al. (2017). Atmospheric nitrogen deposition to China: A model analysis on nitrogen budget and critical load exceedance. Atmos. Environ. 153, 32–40.
Appendix
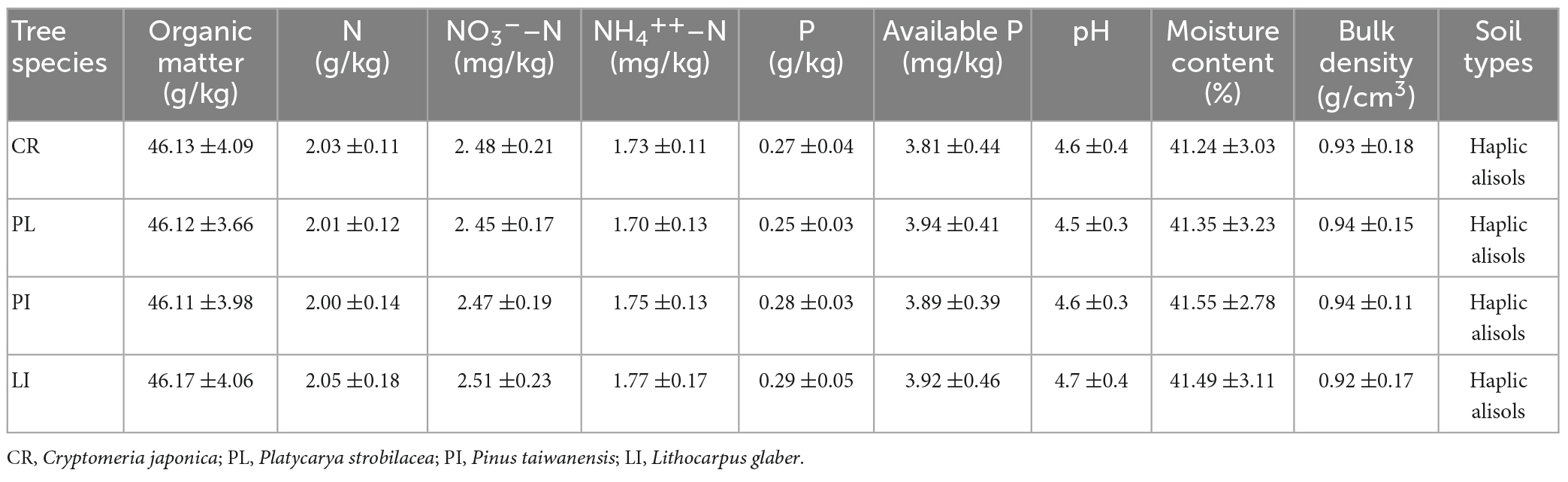
Table A1. Means of the initial soil physical and chemical characteristics (0–5 cm depth) in the broadleaved mixed (BLF) subtropical forest in Lu Mountain; values are means ± SEs for n = 6.
Keywords: wood decomposition, organic nitrogen, inorganic nitrogen, nitrogen deposition, subtropical forests
Citation: Wu C, Shu C, Yuan X, Deng B, Shen F, Zhang Y and Liu Y (2023) Response of wood decomposition to different forms of N deposition in subtropical forests. Front. For. Glob. Change 6:1129681. doi: 10.3389/ffgc.2023.1129681
Received: 22 December 2022; Accepted: 13 February 2023;
Published: 28 February 2023.
Edited by:
Fenghui Yuan, University of Minnesota Twin Cities, United StatesReviewed by:
Licong Dai, Hainan University, ChinaQifeng Mo, South China Agricultural University, China
Copyright © 2023 Wu, Shu, Yuan, Deng, Shen, Zhang and Liu. This is an open-access article distributed under the terms of the Creative Commons Attribution License (CC BY). The use, distribution or reproduction in other forums is permitted, provided the original author(s) and the copyright owner(s) are credited and that the original publication in this journal is cited, in accordance with accepted academic practice. No use, distribution or reproduction is permitted which does not comply with these terms.
*Correspondence: Chunsheng Wu, wucs0109@163.com; Yuanqiu Liu,
liuyq404@163.com