- 1School of Biological Sciences, University of Canterbury, Christchurch, New Zealand
- 2Department of Plant Science and Biotechnology, University of Jos, Jos, Nigeria
- 3Nigerian Montane Forest Project, Yelwa, Taraba, Nigeria
- 4Forest Global Earth Observatory, Smithsonian Tropical Research Institute, Washington, DC, United States
Tree seedling dynamics underpin subsequent forest structure and diversity as different species/guilds respond variously to abiotic and biotic stresses. Thus, understanding differential seedling responses to stresses helps us to predict forest trajectories. Because forests vary in both environment and species composition, generalisations across tropical forests are difficult. Afromontane forests are important carbon stores, harbour high diversity and provide critical ecosystem services, yet they are vulnerable to climate change. Here, we investigate the importance of key abiotic and biotic factors on survival of seedling guilds along spatial and temporal scales in a montane forest in south-eastern Nigeria. We use data from 318 seedling plots censused every 3 months from 2017 to 2020 to identify seven key abiotic and four biotic factors influencing seedling survival. We used the Kaplan–Meier method to estimate the persistence time of 1,145 seedlings at community and guild levels. At the community level, newly recruited seedlings had a median survival time of 15 months and about 37% of the seedlings sampled where still alive after 24 months. Understory tree species survived significantly longer than the other growth form guilds and seedling survival did not differ across shade tolerance guilds. Conspecific adult density and steeper, more north facing slopes had a negative effect on tree seedlings survival. In addition, tree seedlings that recruited in October (end of wet season/beginning of dry) had lower survival probabilities compared to those that recruited in the other months. Except for initial height, seedling survival in lianas was not impacted by any of the abiotic and biotic variables tested. Our results suggest that under the current environment forest structure and diversity is changing, most noticeably lianas are increasing in abundance relative to trees.
1. Introduction
The seedling stage constitutes a significant bottleneck for forest regeneration (Harper, 1977; Comita et al., 2009; Martini et al., 2019; Ssali et al., 2019), so that understanding factors influencing seedling survival is essential for predicting future forest community structure and dynamics (Johnson et al., 2017; Lin et al., 2017). Moreover, because seedling recruitment varies greatly across both time and space (Janzen, 1970; Connell, 1971; Fricke et al., 2014; Zhu et al., 2018) incorporating both spatial and temporal variation into investigations of forest trajectories is essential (Gomes et al., 2020).
Abiotic and biotic factors influencing seedling dynamics are variable and complex (Martini et al., 2019). For example, biotic factors can be intrinsic, such as a seed’s resources or a seedling’s vigour, or extrinsic, such as predation and intraspecific or interspecific competition (Comita et al., 2014; Lu et al., 2015; Downey et al., 2018). Additionally, intrinsic factors may influence a seedling’s response to extrinsic factors (Comita and Hubbell, 2009). For example, understory species generally produce fewer seeds than canopy species, resulting in fewer recruits than canopy trees (Terborgh et al., 2014). Fewer recruits reduce conspecific negative density dependence (CNDD) and associated repercussions (Lu et al., 2015). The local biotic neighbourhood has a significant impact on seedling survival dynamics through density and/or distance dependent factors (Harms et al., 2000; Hille Ris Lambers et al., 2002; Comita et al., 2014; Ramage et al., 2017; Forrister et al., 2019). Strong intraspecific competition or host-specific pests and pathogens can limit the survival of a seedling within a neighbourhood having a high density of same species neighbours (Janzen, 1970; Connell, 1971). Alternatively, a seedling in such a conspecific neighbourhood may benefit from intraspecific facilitation, favouring its survival (Baldeck et al., 2013; Lebrija-Trejos et al., 2014).
Abiotic factors are also important for seedling recruitment (Martini et al., 2019). While seedling survival is typically limited by only a few resources, including light, water, and soil nutrients, these resources can be limiting. Limited resources initiate trade-offs in performance among species in a community bringing about niche differentiation and coexistence (Johnson et al., 2017). Light is one of the most important abiotic factors limiting seedlings in tropical forests (Comita et al., 2009). Seedlings show increased growth and survival in higher light conditions (Lu et al., 2018). Oshima et al. (2015) in their study of dipterocarps in South-East Asia reported that canopy openness was a major factor affecting seedling survival and D’andrea et al. (2020) suggest that in Barro Colorado Island, Panama forest community structure may be driven by competition for light.
Topographical features can influence seedling dynamics; slope, elevation, and aspect alter abiotic factors such as soil moisture and nutrients which affect the availability of resources in a site and a seedling’s ability to acquire them (Comita et al., 2009; Comita and Engelbrecht, 2014). Microhabitats vary in space and time, and this influences recruitment success. For example, Ma et al. (2014) demonstrated a negative effect of increasing slope on seedling survival in a broad-leafed evergreen forest in China. In contrast, Lin et al. (2017) showed no effect of slope on seedling recruitment in a tropical Karst forest. Aspect has been shown to significantly impact the species assemblage in a tropical forest in Sri Lanka (Punchi-Manage et al., 2013), but did not affect adult tree mortality in a subtropical forest in Central China (Wu et al., 2017). Microtopography, such as pit and mound (Barker Plotkin et al., 2017) is another feature that can influence seedling survival in tropical forests. Born et al. (2015) demonstrate this for Dipterocarp seedlings in Malaysia, faster growing seedling species were more susceptible to flooding than slower growing species. Survival of particular Inga species in Neotropical lowland forests is strongly associated with microtopography (Endara and Jaramillo, 2011).
In addition to abiotic and biotic factors, seedling survival may also be influenced by timing of recruitment, especially in seasonal forests (Lin et al., 2017). Seedlings that establish in the wet season may have higher survival advantage to those that establish in the dry season because they are likely to grow faster, thus acquiring an initial size advantage (Bai et al., 2012).
Species traits are yet another component to understanding seedling dynamics in tropical forests (Zhu et al., 2018). For example, light requirement is often linked to a seedling’s probability of survival (Wright et al., 2010; Kobe and Vriesendorp, 2011). Fast-growing, light-demanding species have higher mortality rates compared to slow growing, shade-tolerant species because the latter have functional traits such as higher wood density, longer lived leaves and better defence against pests that increase longevity (Kobe, 1999).
The many long-term studies that have assessed abiotic and biotic factors on seedling survival in tropical forests show that while some generalisations can be made (Comita et al., 2009; Paine and Harms, 2009; Chen et al., 2010; Lin et al., 2012; Johnson et al., 2017; Martini et al., 2019), different forest types behave differently (Spicer et al., 2020). Moreover, such studies are heavily biassed toward lowland tropical and subtropical forests. The role of abiotic and biotic factors in shaping plant recruitment and establishment in montane forests is poorly understood and they have rarely been examined together. A review by Chapman et al. (2016) points out how very little is known about regeneration of montane ecosystems and how it is important for research to include this valuable ecosystem which is highly susceptible to the negative impacts of climate change. With this in mind, we examined the abiotic and biotic factors contributing to seedling survival in a tropical montane forest in Nigeria. West African montane forests experience a unique set of abiotic and biotic stresses; they are typically restricted to steep slopes protected from fire and gazing (Chapman and Chapman, 2001), rainfall is limited to 6 months in a year and the dry season is made extremely severe by the dry Saharan desert Harmattan wind which lasts for 2–3 months (Jenik and Hall, 1966).
In this study we asked the following questions: (i) How important are abiotic and biotic factors for the survival of newly recruited seedlings in the first 3-month interval after recruitment and is this affected by the time of recruitment? (ii) Are abiotic stresses more influential in determining seedling fate than the biotic neighbourhood? (iii) How does seedling survival vary across growth form and shade tolerance guilds? We hypothesised that abiotic factors such as slope, elevation, and aspect which influence light, wind, and moisture levels will impact seedling survival more than biotic factors in this Afromontane environment. Drought in particular may be a strong seedling filter. Dry conditions can also alter the spatial patterns and activities of herbivores and fungal pathogens (Inman-Narahari et al., 2016), thus reducing any Janzen–Connell effects. We expected seedling survival to be higher for understory and shade tolerant species because they usually have fewer, more resilient seedlings that are less likely to succumb to negative density dependence (NDD) effects than canopy species.
2. Materials and methods
2.1. Study area
This study was carried out in the 20.28-ha Ngel Nyaki Forest Dynamics Plot (07°04′05″N; 11°03′24″E) in the Ngel Nyaki Forest Reserve on the Mambilla Plateau of south-eastern Nigeria. Ngel Nyaki forest reserve is 4,600 ha of mainly savanna but including two patches of species-rich sub-montane dry forest, which together cover 76 ha in area (Chapman and Chapman, 2001; Beck and Chapman, 2008). Details on the study area, study site, and anthropogenic threats to the forests are recorded in Abiem et al. (2020). Of particular note is that the forest itself is small with extensive edges bordering onto overgrazed Sporobolus spp. Grassland that is burned annually as a management tool for cattle grazing. The reserve is usually protected from fire by creating fire breaks at the boundary, however in some years, fire accidentally gets into the reserve and burns portions of the grass land and the forest edges. Also, cattle sometimes enter the forest edges and trample. Hunting has greatly reduced population sizes of large bodied frugivores such as chimpanzees and hornbills. There are other indigenous fauna like duikers and antelopes whose populations have reduced as well.
2.2. Data collection
2.2.1. Seedling censuses
To study seedling regeneration in the forest, we established 318 m × 1 m seedling plots in the Ngel Nyaki Forest Dynamics Plot in October and November 2017. Seedling plots were associated with seed traps (see Figure 1) placed systematically along trails in the plot at the beginning of 2017 (sensu Wright et al., 2005). Each seedling plot was assigned to a 5 × 5 m subquadrat of the plot. The first seedling census was conducted in October 2017. All seedlings ≤50 cm tall and including trees, shrubs, and lianas encountered within the seedling plots were tagged, identified, measured for height, and their leaves counted. Nine repeat censuses were conducted at 3-month intervals after the first census (January–February 2018, April–May 2018, July–August 2018, October–November 2018, January–February 2019, April–May 2019, July–August 2019, October–November 2019, and January–February 2020). For every repeat census, we recorded the status of a previously encountered seedling as alive or dead and re-measured the height and number of leaves for those seedlings that were alive. We also tagged, identified and measured any newly recruited seedlings. Focal seedling data used in this study were from seedlings recruited in the first eight repeat censuses for which we had at least a 3-month interval survival status data on.
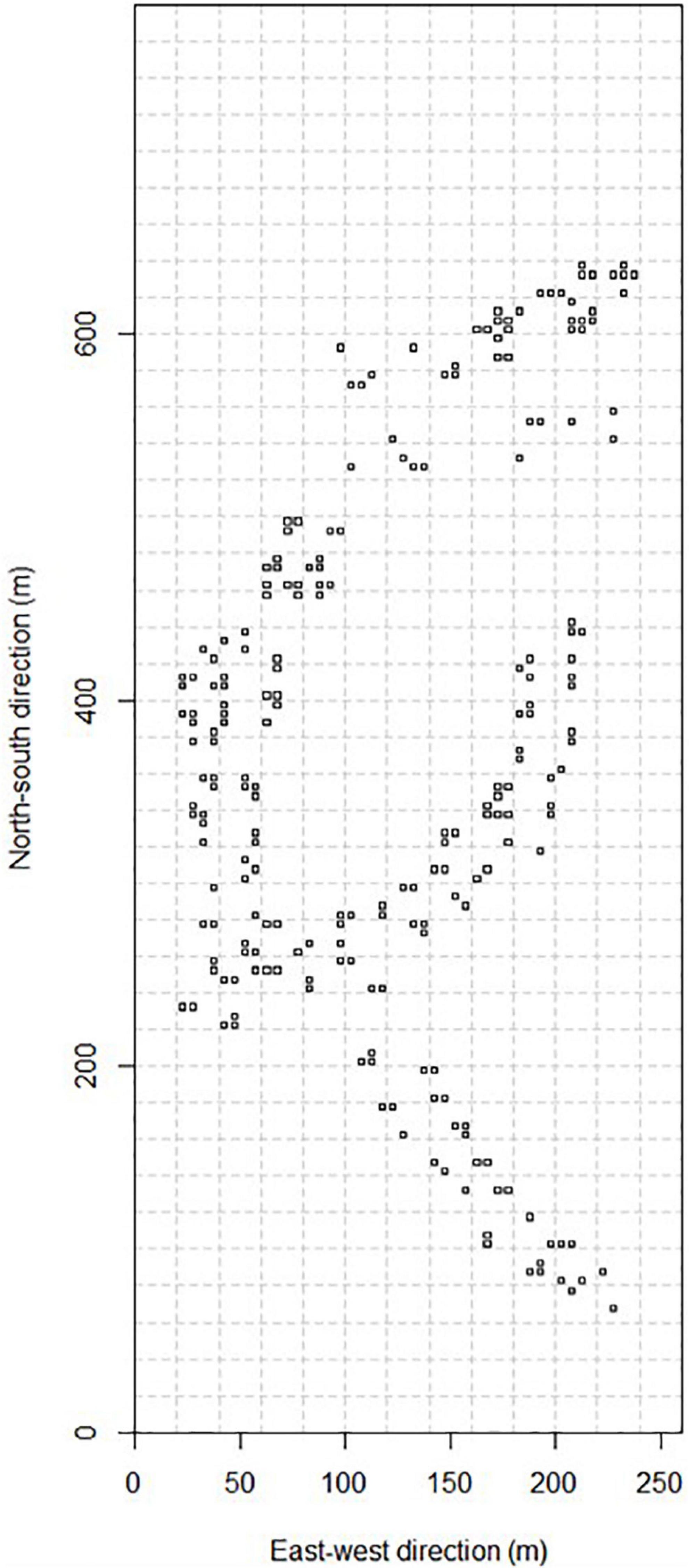
Figure 1. Approximate positions of seedling plots in the 20.28 ha Ngel Nyaki Forest Dynamics Plot. Only seedling plots from which we sampled newly recruited seedlings are indicated.
2.3. Measurement of biotic factors
We quantified the local biotic neighbourhood in which every newly recruited seedling was growing. We calculated the total seedling density (S.tot) and the densities of conspecific and heterospecific seedlings (S.con and S.het) in each plot. Seedling neighbour densities for each seedling were estimated for the census period the seedling was first encountered. To account for the effect of saplings and larger trees, we calculated the density of all stems ≥1 cm dbh by summing the inverse-distance weighted basal areas of all sapling and adults within a 20 m radius of the centre of the subquadrat where the seedling plot is located. We calculated total adult densities (A.tot) and separate densities for conspecific and heterospecific adults (A.con and A.het).
2.4. Measurement of abiotic factors
Topography: We calculated elevation, aspect, slope and landform. In every seedling plot, we used a Global Positioning Service (GPS) device (Garmin GPSMAP 64s GPS) to measure elevation and a compass to measure aspect. Percent slope was calculated for each seedling plot by dividing the difference in elevation between two marked points in the plot (rise) by the horizontal distance between them (run) and then multiplying the quotient by 100 [i.e., (rise/run) × 100]. Landform for each plot was assessed visually and labelled as pit (concave-shaped), mound (convex shaped), or flat. We also estimated litter depth for each seedling plot by measuring the depth of leaf litter and partially decomposed organic matter that accumulates on top of the mineral soil from five points in the plot using a metre rule and estimating the mean value for the plot.
Light intensity: We took light meter readings from three points within the seedling plot and concurrently from three points at a nearby large open gap. Measurements were taken at a height of 1.3 m. We used a PAR Quantum sensor (LI-190R, LI-COR Biosciences, Lincoln, NE, USA) with a light meter (LI-250A, LI-COR Biosciences, Lincoln, NE, USA). We calculated available light by dividing measurements taken in the forest by those taken in the open gap nearby within a 10-min interval (Moustakas and Evans, 2015).
2.5. Data analyses
We used generalised linear mixed-effects models with binomial errors to model the probability of an individual seedling surviving its first 3-month census interval as a function of the abiotic and biotic neighbourhood factors measured (described above). Log-transformed initial seedling height and initial number of leaves were included in the models as fixed effects. Aspect is a circular variable and so we transformed it to estimate northness and eastness using cos(aspect) which ranges between 1 for due north and −1 for due south; and sin(aspect) which ranges between 1 for due east and −1 for due west (Schwarz et al., 2003; Lu et al., 2015; Martini et al., 2019). We also included the month in which a seedling census was carried out as recruitment timing. January and April censuses were in the dry season while the July and October censuses were in the wet season. For each seedling, we assigned values of seedling densities and abiotic variables recorded for the census when they initially recruited. We standardised the values of all continuous independent variables by subtracting the mean and dividing by 1 SD. Species identity and seedling plot identity were included as random effects in the models to account for species variability and spatial autocorrelation respectively. Species vary in their survival probabilities and seedlings from the same plot tend to have similar survival probabilities compared to seedlings from other plots.
We analysed seedling survival for trees and shrubs separately from lianas. This is because during the census of woody stems ≥1 cm dbh, lianas were not included and so, we do not have estimates on conspecific adult density for the lianas. We therefore analysed community-level survival for seedlings of tree and shrub species present in the adult census and also analysed community-level survival for seedlings of liana species. We also did not include the small understory shrubs Dracaena sp. and Brillantaisia owariensis as focal seedlings in our community-level analysis because their adults rarely reach ≥1 cm dbh but they were included (and the lianas as well) in estimating neighbourhood densities. Initial plant height was significantly correlated to leaf number so we used only initial height in our models.
Our data were insufficient to perform species-specific and life-history guild analyses. All the tree species recorded had ≤51 observations on seedling survival.
We used the non-parametric Kaplan–Meier method to estimate survival time. We compared survivorship curves across growth forms (emergent tree, canopy tree, understory tree/shrub, and liana) and shade tolerance (light-demanding, intermediate light-demanding, and shade tolerant) groups.
All statistical analyses were performed using R.3.5.0. statistical software package (R Core Team, 2018) with lme4 1.1.15 package (Bates et al., 2015) and survival package (Therneau, 2019).
3. Results
Between January 2018 and January 2020, we recorded and monitored 1,145 newly recruited seedlings belonging to 49 species (See Supplementary Table 1 for full species list and their abundances). Of these, 388 individuals, ∼34%, belonged to species recorded in the previous 2015 adult census (Abiem et al., 2020), 654, ∼57% were liana species and 103 ∼ 9% belonged to the shrubs Dracaena sp. and B. owariensis. The proportion of tree and shrub species recruitment versus lianas varied among the census times. Higher recruitment of tree and shrub seedlings occurred in the third census (July–August 2018) while a higher recruitment of liana seedlings occurred in the first census (January–February 2018). Recruitment of tree and shrub seedlings was lowest in the sixth census (April–May 2019) while the seventh census (July–August 2019) recorded the lowest recruitment of liana seedlings. Taken together, higher recruitment in trees and shrubs occurred in the wet season (July and October) than in the dry season (January and April), while for lianas the opposite was true. Most of the recorded seedlings (82%) were between the height range of 5 and 20 cm.
3.1. Effect of biotic and abiotic factors on seedling survival
In all the censuses, the tree seedling community had 22% mortality while the liana community had 16% mortality. Both abiotic and biotic factors affected seedling survival (Figures 2, 3). Initial plant height was strongly associated with seedling survival; for both tree and liana communities, taller seedlings had a higher probability of survival (Log odds ratio = 0.52, P < 0.001 and Log odds ratio = 0.49, P < 0.001; Figures 2, 3). The conspecific adult density showed a significant negative impact on seedling survival (Log odds ratio = −0.26, P = 0.047; Figure 2). In contrast, the biotic neighbourhood-heterospecific “tree” adult density, conspecific seedling density, and heterospecific seedling density, had no significant effect on seedling survival in the liana community. Among the abiotic predictors tested in the tree community, only slope and aspect were significant (Figure 2). Slope had a negative effect on seedling survival meaning steeper sites had lower survival. Sin(aspect) had a positive effect on seedling survival meaning survival was higher on east-facing slopes than west-facing slopes; while cos(aspect) had a negative effect on seedling survival meaning southward facing slopes had higher seedling survival than northward facing slopes (Figure 2). Tree and shrub seedlings that recruited in the month of October, toward the very end of the wet season, had significantly lower survival probability than those that recruited in the other months (Log odds ratio = −1.46, P = 0.01).
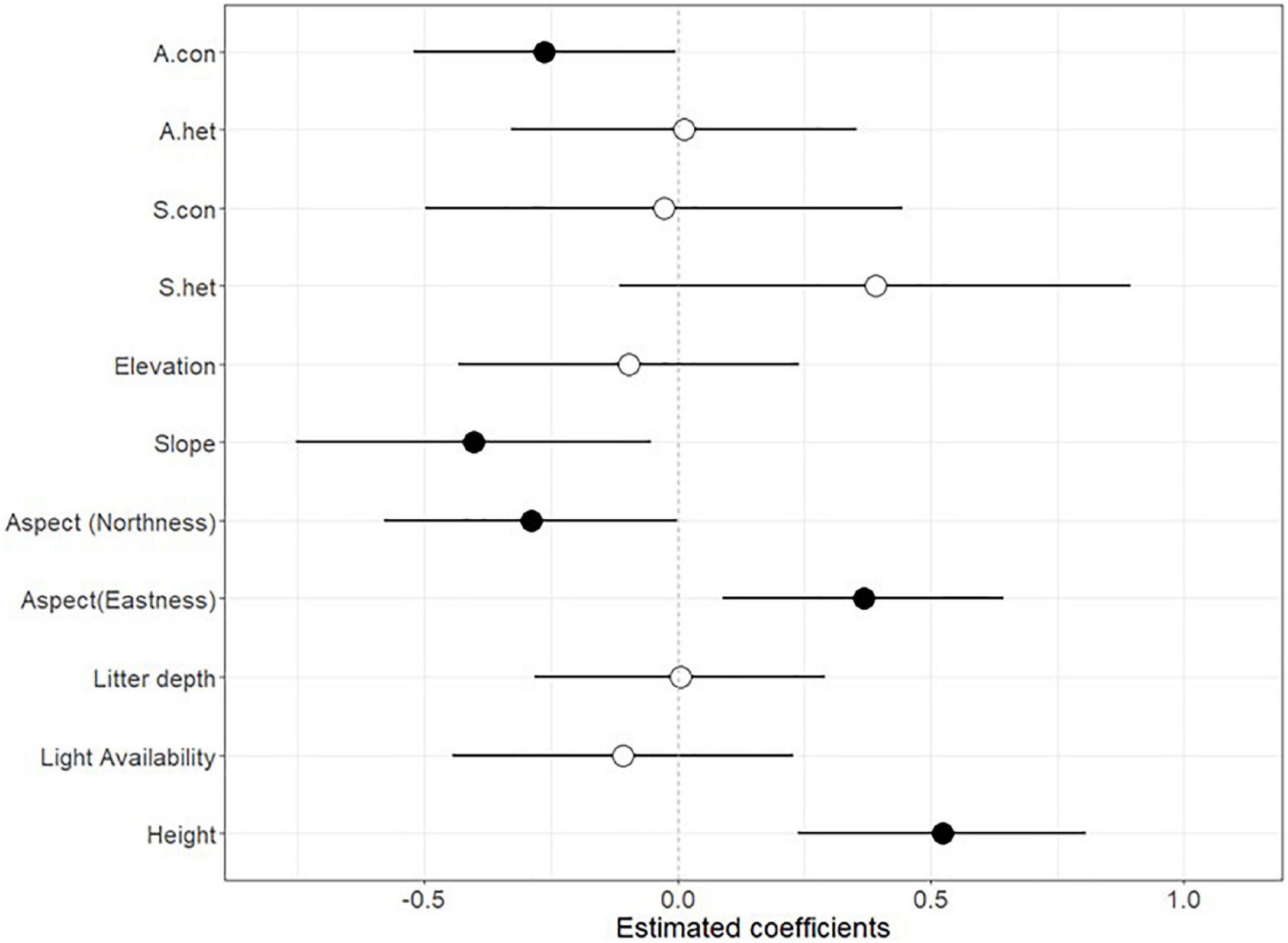
Figure 2. Parameter estimates (±2 SE) from generalised linear mixed effects model (GLMM) fitted to test the effects of abiotic and biotic factors on seedling survival of tree and shrub species in the Ngel Nyaki forest. Filled points indicate significant effects (P < 0.05).
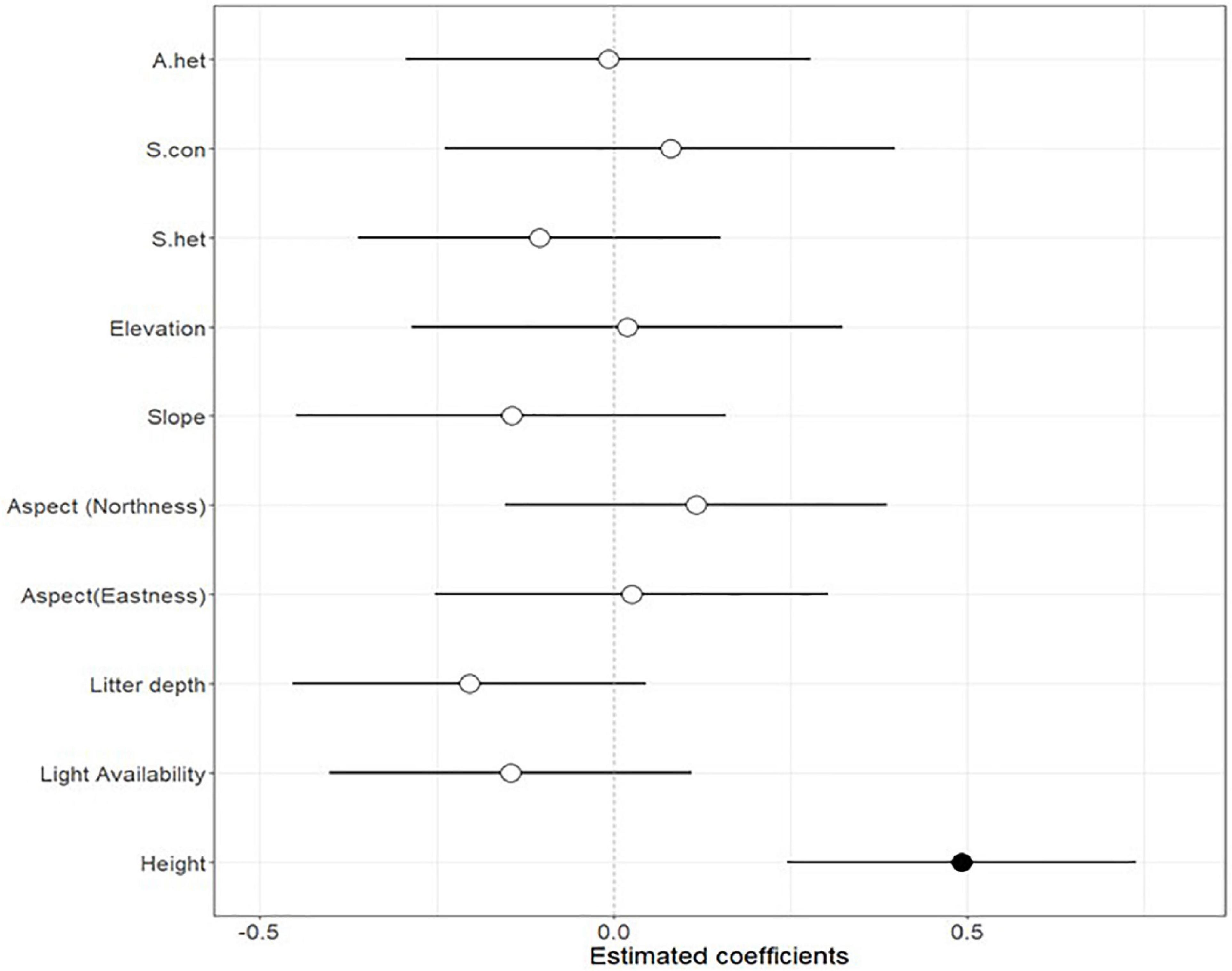
Figure 3. Parameter estimates (±2 SE) from generalised linear mixed effects model (GLMM) fitted to test the effects of abiotic and biotic factors on seedling survival of liana species in the Ngel Nyaki forest. Filled points indicate significant effects (P < 0.05).
For liana seedlings, the neighbourhood abiotic and biotic factors did not significantly affect seedling survival (Figure 3).
The summaries of the generalised linear mixed effects models used are recorded in Supplementary Tables 2, 3.
3.2. Survival analyses
In the survival analysis using the Kaplan–Maier method, the median survival time of seedlings was 15 months (Figure 4). Approximately 37% of the seedlings sampled where still alive after 24 months (Figure 4). The results showed that the probability on an understory species surviving was higher compared to other growth forms for the duration of the study (P < 0.0001; Figure 5). Seedling survival did not differ across shade tolerance guilds although shade tolerant species had higher survival probability over time (Figure 6).
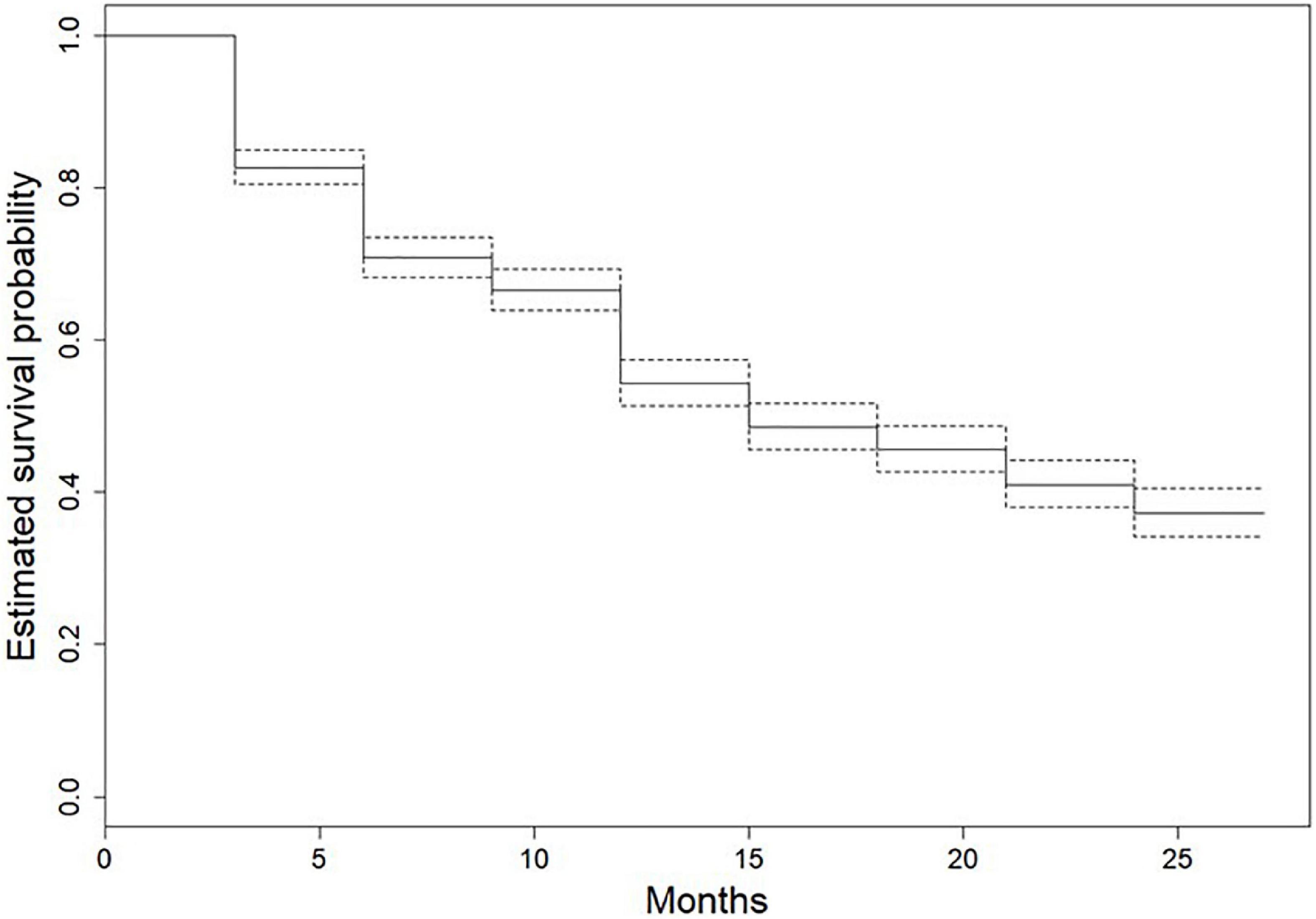
Figure 4. Estimated survival probabilities of all species seedlings encountered in seedling censuses in the Ngel Nyaki Forest Dynamics Plot from January 2018 to January 2020. Solid line represents estimated survival average probabilities, and the dotted lines show the 95% CI. The survival function was calculated using the Kaplan–Meier method.
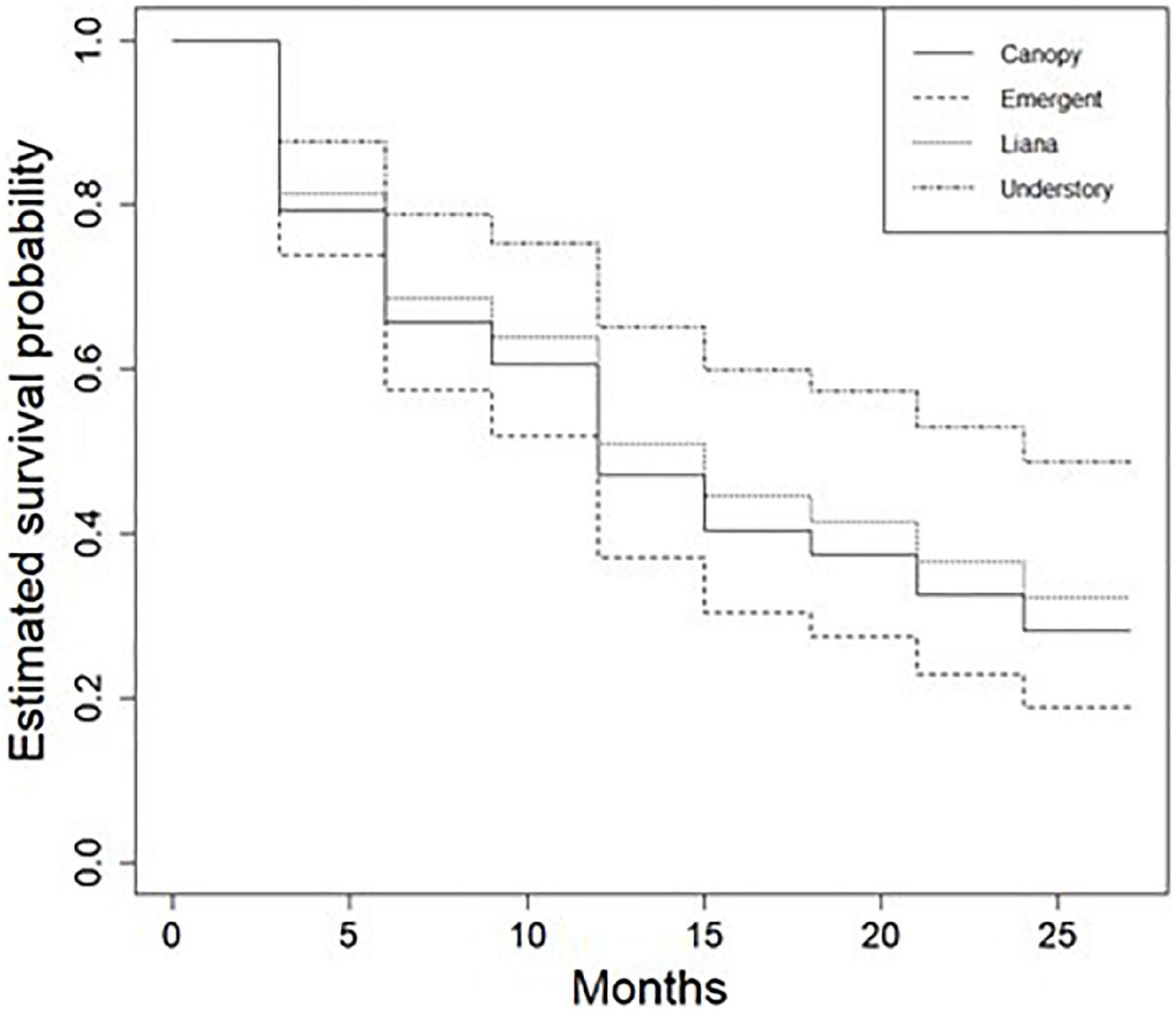
Figure 5. Estimated survival probabilities of seedlings across growth form guilds in the Ngel Nyaki Forest Dynamics Plot from January 2018 to January 2020. The lines represent estimated survival average probabilities for the different groups. The survival functions were calculated using the Kaplan–Meier method.
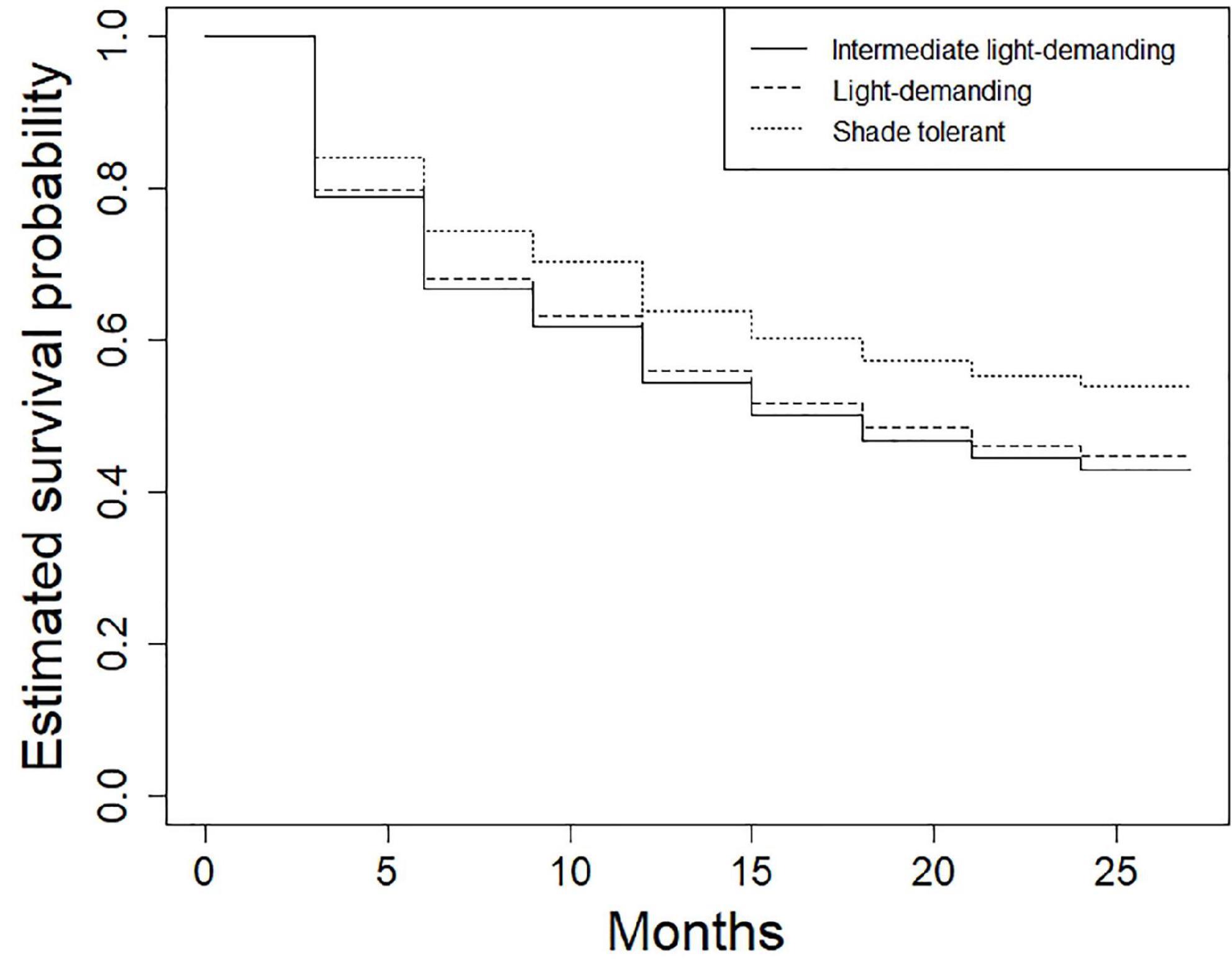
Figure 6. Estimated survival probabilities of seedlings across shade-tolerance guilds in the Ngel Nyaki Forest Dynamics Plot from January 2018 to January 2020. The lines represent estimated survival average probabilities for the different groups. The survival functions were calculated using the Kaplan–Meier method.
4. Discussion
The aim of our research was to explore key abiotic and biotic factors influencing the seedling dynamics of tree and liana seedlings in the Ngel Nyaki Forest Dynamics Plot to better understand Afromontane forest demographics and thus help us in predicting future forest structure and diversity. Most noticeable in terms of species trajectories was that almost two-thirds of all recruited seedlings in our study belonged to just two liana species – Landolphia sp. (Apocynaceae) and Paullinia pinnata (Sapindaceae). An abundance of liana relative to tree seedlings corroborates reports from other tropical forests that liana density is disproportionally increasing (Schnitzer et al., 2012; Sun et al., 2022), with potentially adverse consequences for trees (Schnitzer and Bongers, 2011; Venegas-González et al., 2020), but see Umaña et al. (2020). One explanation for an increase in liana seedlings could be a seasonal growth advantage (SGA), because lianas are able to grow faster than trees during seasonal drought (Sun et al., 2022 and references within). However, while this may be the case for adult lianas (Sun et al., 2022), to what extent this applies to seedlings is unclear and results are mixed. Ledo and Schnitzer (2014) and Umaña et al. (2019) found that liana seedlings did benefit from dry conditions and disturbance, Vogado et al. (2022) attributed increasing liana abundances to increased CO2 concentrations, while light availability has been found to be more important for liana seedling survival in some other studies (Addo-Fordjour and Rahmad, 2015; De Campos Franci et al., 2016). The only significant factor we found affecting liana seedling survival in this study was seedling height, which was not linked to any other measured abiotic or biotic factors. Our findings corroborate those of Dewalt et al. (2015) who similarly reported that seedling survival in lianas was unaffected by the abiotic and biotic neighbourhood in a Dominican rain forest. Moreover, our rainfall records at Ngel Nyaki do not suggest that seasonal droughts are increasing, although other anthropogenic factors may be altering conditions in the forest in ways that benefit liana seedling survival. For example, cattle grazing is increasing edge effects; Fulani herdsmen drive their cattle into the forest reserve and their trampling opens up canopy cover.
4.1. Abiotic and biotic factors determining seedling survival
As has been reported elsewhere we found initial seedling height was the most significant factor influencing tree seedling survival. Seedling height has been reported from other tropical permanent forest plots as being an important driver of seedling survival (Comita et al., 2009; Ma et al., 2014; Johnson et al., 2017; Martini et al., 2019; Jiang et al., 2022). Jiang et al. (2022) exploring the mechanisms for this relationship in a temperate forest found seedling functional traits, especially leaf area to be key; larger leaves promoted seedling growth, albeit at a slow rate.
We found conspecific adult neighbours to have a significant negative impact on seedling survival, consistent with the Janzen–Connell hypothesis. CNDD mortality has been observed widely in tropical and temperate forests (Comita et al., 2014; Chen et al., 2018; Umaña et al., 2018; Jia et al., 2020) and this result suggests that CNDD may play a role in tree community structuring in Ngel Nyaki forest, corroborating part of the findings of an earlier study which incorporated some of the same and some different tree species (Abiem et al., 2021). A detailed examination of the causes of CNDD in Afromontane forests is yet to be undertaken but Matthesius et al. (2011), again in Ngel Nyaki forest, observed increased herbivory of seedlings close to, relative to away from, conspecific adults. Negative effects of conspecific neighbours are often related to the activities of host-specific pathogens and herbivores (Bagchi et al., 2014).
Of all the abiotic factors sampled only two topographic features, slope and aspect had a significant impact on tree seedling survival. Topography is known to be an important filter of tree species in tropical forests, directly through its influence on climate, light, soil moisture, and soil nutrient content availability (Xia et al., 2016; Jin et al., 2018; Song et al., 2018) and indirectly through its influence on plant–plant interactions (Huang et al., 2022; O’Brien and Escudero, 2022). We found seedling survival to be lower on steeper slopes which is consistent with several other studies (Nagamatsu et al., 2002; Daws et al., 2005; Charles et al., 2018) but in disagreement with Du et al. (2017) who reported that about 80% of species in a Karst forest in Southwest China were associated with steep slopes. Huang et al. (2022) also reported higher overall survival rates on slopes than in valley bottoms in Taiwanese subtropical forest. We had expected high seedling survival on steep slopes relative to valleys in our study site because steep slopes are a characteristic feature of many Afromontane forests. However, lower seedling survival on slopes may reflect low water retention, increased water run-off and soil erosion, making it difficult for seedlings to establish. While Daws et al. (2005) argue that steep slopes may favour the recruitment of small, light seeds because large, heavier seeds are washed downhill, we do not think this applies to Ngel Nyaki forest because we have found no evidence of large-seeded seedlings congregating on valley floors. Moreover, in seasonally dry, semi-deciduous Ngel Nyaki forest (Chapman and Chapman, 2001) large seeds are easily trapped by litter, understory vegetation and/or tree roots and so may not necessarily be washed downhill.
Aspect strongly filtered seedlings at our study site, with seedling survival and species diversity being significantly higher on south and east, as opposed to north and west facing slopes. While aspect is known to be a significant determinant of vegetation structure in temperate regions (Singh, 2018), in the tropics it is likely to be most influential in strongly seasonal climates, such as the 6 months wet and 6 months dry season at our study site (Méndez-Toribio et al., 2017). At Ngel Nyaki, as across West Africa, the extreme wet and dry seasons are governed by two winds, the rain bearing south-west winds from the Atlantic and the dry, dust, and often ash-filled Harmattan wind blowing southeast from the Sahara (Jenik and Hall, 1966; Stoorvogel et al., 1997). The Harmattan is strongest between December and January and must disproportionately impact north facing slopes, causing severe evapotranspiration (Jenik and Hall, 1966), drying leaf litter on the forest floor and covering leaves in a grey, nutrient rich dust (Hazel Chapman, personal communication). While we have not yet tested for the eco-physiological effects of the Harmattan, based on Jenik and Hall (1966) who report the ecological effects of the wind on species composition on a nearby massif in Ghana, they are likely to be extreme. The south facing slopes in contrast may receive more rain during the wet season and have more year-round fog than the north facing slopes. The issue of fog contribution to moisture and its distribution across Ngel Nyaki forest remains to be measured and understood.
Census month was also a significant factor in our tree community model. Seedlings which recruited in October, just before the onset of the dry season and Harmattan winds, had significantly lower survival compared to the other months. However, seedlings recruiting toward the middle and end of the dry season January–April recorded had a higher probability of surviving than wet season seedling recruits (July). While it would seem intuitive that wet season recruits should have a survival advantage over dry season recruits because of water availability, it may be that the high humidity associated with the wet season favours pathogens and herbivores (Inman-Narahari et al., 2016; Lin et al., 2017). Again, further research is needed to fully understand what is driving this result.
4.2. Survival analyses
The results of the Kaplan–Meier analyses showed that most newly recruited seedlings persisted for more than a year in Ngel Nyaki forest. Seedlings median survival time was 15 months and about 40% of seedlings are predicted to persist for more than 2 years. Seedling persistence here was higher than that observed by Lin et al. (2017) in the tropical Karst forest in Taiwan where only 11% of seedlings persisted more than 2 years, but lower than that observed by Delissio et al. (2002) in the Lambir forest of Malaysia where 50% of the seedlings survived up to 10 years. Understory trees seedlings showed significantly higher persistence compared to the other growth form groups. Understory species usually have fewer seedlings than canopy species and so are less likely to be affected by CNDD, likely explaining King et al. (2006) observations of higher seedling survival in first-year seedlings of understory relative to canopy tree species.
Unfortunately, because of small numbers we were unable to carry out species-level analyses, which would have allowed us to determine how species vary in their response to abiotic and biotic factors, necessary information for predicting species responses to changing environmental conditions.
Another potential limitation of our study is that it has been conducted over just 27 months (just over 2 years), which raises the concern that our results measure only a snapshot in time. However, we argue that it adequately fulfils its intent which was to examine the role of abiotic and biotic factors on the short-term survival of seedlings in the Ngel Nyaki forest. There were eight repeat censuses, conducted over different seasons of the year for 2 years. An ongoing longer study will provide data to increase confidence in the trends we did see and to allow for species-specific predictions.
5. Conclusion
Our analysis of the 3-month survival of 1,145 newly recruited seedlings of 47 woody species in the Ngel Nyaki Permanent Forest Plot provides the first detailed assessment to our knowledge of the role of abiotic and biotic factors in shaping species composition and diversity in an Afromontane forest. Given the significant differences between tropical African montane and lowland forests (Chapman et al., 2016), we had predicted some differences in the effect of factors on species’ survival. For example, because of the steep terrain typical of Afromontane forests we hypothesised that seedlings would survive as well or better on steep slopes than in valley bottoms. Instead, our results were consistent with those reported from several other lowland tropical forest studies (Dewalt et al., 2015; Lin et al., 2017; Martini et al., 2019). CNDD, topography and timing of recruitment (recruitment season) most strongly influenced tree seedling survival probability, suggesting that CNDD and niche partitioning are important in shaping species coexistence in West African montane forests. Tree seedlings had a higher probability of surviving on valley bottoms than on steep slopes. In contrast, the abiotic and biotic neighbourhood had no effect on liana seedling survival, and liana seedling appear to be increasing in number relative to tree seedlings, again corroborating results and trends from elsewhere in the tropics (Umaña et al., 2019). Future studies will include control experiments with sufficient individual species sample sizes to determine the role of the abiotic and biotic neighbourhood on the survival of individual species. In addition, more specific abiotic factors such as soil water, soil nutrient composition, fog, Harmattan wind dust need to be measured which may make interpretations of relationships observed with proxy variables more robust.
Data availability statement
The raw data supporting the conclusions of this article will be made available by the authors, without undue reservation.
Author contributions
IA led data collection with support from HC and DK. IA and HC formulated the idea for the manuscript with inputs from DK. IA analysed the data and wrote the initial draft of the manuscript with major comments and edits by HC. All authors contributed to the article and approved the submitted version.
Funding
The Ngel Nyaki Forest Dynamics Plot project was primarily funded by an extremely generous donation from Rtd. Gen. T. Y. Danjuma to the Nigerian Montane Forest Project (NMFP), and by a grant from the Smithsonian Institute’s Forest Global Earth Observatory (ForestGEO). IA received a ForestGEO research grant (Project ID 341243) for this project. This study was a part of IA’s Ph.D. thesis that was funded by the University of Jos NEEDS assessment programme, Nigeria and a grant from the A. G. Leventis Foundation.
Acknowledgments
IA was grateful to Liza Comita, S. Joseph Wright, and Daniel Johnson for providing useful advice on data analyses. We wish to thank the management and staff of NMFP for field work and logistic support. We especially want to thank the forest plot team who have helped with all the censuses since 2016.
Conflict of interest
The authors declare that the research was conducted in the absence of any commercial or financial relationships that could be construed as a potential conflict of interest.
Publisher’s note
All claims expressed in this article are solely those of the authors and do not necessarily represent those of their affiliated organizations, or those of the publisher, the editors and the reviewers. Any product that may be evaluated in this article, or claim that may be made by its manufacturer, is not guaranteed or endorsed by the publisher.
Supplementary material
The Supplementary Material for this article can be found online at: https://www.frontiersin.org/articles/10.3389/ffgc.2023.1108257/full#supplementary-material
References
Abiem, I., Arellano, G., Kenfack, D., and Chapman, H. (2020). Afromontane forest diversity and the role of grassland-forest transition in tree species distribution. Diversity 12:30. doi: 10.3390/d12010030
Abiem, I., Dickie, I., Kenfack, D., and Chapman, H. (2021). Conspecific negative density dependence does not explain coexistence in a tropical Afromontane forest. J. Veg. Sci. 32, e12990. doi: 10.1111/jvs.12990
Addo-Fordjour, P., and Rahmad, Z. B. (2015). Environmental factors associated with liana community assemblages in a tropical forest reserve, Ghana. J. Trop. Ecol. 31, 69–79. doi: 10.1017/S0266467414000522
Bagchi, R., Gallery, R. E., Gripenberg, S., Gurr, S. J., Narayan, L., Addis, C. E., et al. (2014). Pathogens and insect herbivores drive rainforest plant diversity and composition. Nature 506, 85–88. doi: 10.1038/nature12911
Bai, X., Queenborough, S. A., Wang, X., Zhang, J., Li, B., Yuan, Z., et al. (2012). Effects of local biotic neighbors and habitat heterogeneity on tree and shrub seedling survival in an old-growth temperate forest. Oecologia 170, 755–765. doi: 10.1007/s00442-012-2348-2
Baldeck, C. A., Harms, K. E., Yavitt, J. B., John, R., Turner, B. L., Valencia, R., et al. (2013). Habitat filtering across tree life stages in tropical forest communities. Proc. R. Soc. Lond. B Biol. Sci. 280:20130548. doi: 10.1098/rspb.2013.0548
Barker Plotkin, A., Schoonmaker, P., Leon, B., and Foster, D. (2017). Microtopography and ecology of pit-mound structures in second-growth versus old-growth forests. Forest Ecol. Manag. 404, 14–23. doi: 10.1016/j.foreco.2017.08.012
Bates, D., Maechler, M., Bolker, B., and Walker, S. (2015). Fitting linear mixed-effects models using lme4. J. Stat. Softw. 67, 1–48. doi: 10.18637/jss.v067.i01
Beck, J., and Chapman, H. (2008). A population estimate of the endangered chimpanzee Pan troglodytes vellerosus in a Nigerian montane forest: implications for conservation. Oryx 42, 448–451. doi: 10.1017/S0030605308001397
Born, J., Bagchi, R., Burslem, D., Nilus, R., Tellenbach, C., Pluess, A. R., et al. (2015). Differential responses of dipterocarp seedlings to soil moisture and microtopography. Biotropica 47, 49–58. doi: 10.1111/btp.12180
Chapman, H., Cordeiro, N. J., Dutton, P., Wenny, D., Kitamura, S., Kaplin, B., et al. (2016). Seed-dispersal ecology of tropical montane forests. J. Trop. Ecol. 32, 437–454. doi: 10.1017/S0266467416000389
Chapman, J., and Chapman, H. (2001). The Forests of Taraba and Adamawa States, Nigeria. An Ecological Account and Plant Species Checklist. New Zealand: University of Canterbury Press.
Charles, L. S., Dwyer, J. M., Smith, T. J., Connors, S., Marschner, P., and Mayfield, M. M. (2018). Species wood density and the location of planted seedlings drive early-stage seedling survival during tropical forest restoration. J. Appl. Ecol. 55, 1009–1018. doi: 10.1111/1365-2664.13031
Chen, L., Comita, L. S., Wright, S. J., Swenson, N. G., Zimmerman, J. K., Mi, X., et al. (2018). Forest tree neighborhoods are structured more by negative conspecific density dependence than by interactions among closely related species. Ecography 41, 1114–1123. doi: 10.1111/ecog.03389
Chen, L., Mi, X., Comita, L. S., Zhang, L., Ren, H., and Ma, K. (2010). Community-level consequences of density dependence and habitat association in a subtropical broad-leaved forest. Ecol. Lett. 13, 695–704. doi: 10.1111/j.1461-0248.2010.01468.x
Comita, L. S., and Engelbrecht, B. M. (2014). “Drought as a driver of tropical tree species regeneration dynamics and distribution patterns,” in Forest and Global Change, eds D. A. Coomes, D. F. R. B. Bursiem, and W. D. Simonson (Cambridge: Cambridge University Press) doi: 10.1017/CBO9781107323506.013
Comita, L. S., and Hubbell, S. P. (2009). Local neighborhood and species’ shade tolerance influence survival in a diverse seedling bank. Ecology 90, 328–334. doi: 10.1890/08-0451.1
Comita, L. S., Queenborough, S. A., Murphy, S. J., Eck, J. L., Xu, K., Krishnadas, M., et al. (2014). Testing predictions of the janzen–connell hypothesis: a meta-analysis of experimental evidence for distance- and density-dependent seed and seedling survival. J. Ecol. 102, 845–856. doi: 10.1111/1365-2745.12232
Comita, L. S., Uriarte, M., Thompson, J., Jonckheere, I., Canham, C. D., and Zimmerman, J. K. (2009). Abiotic and biotic drivers of seedling survival in a hurricane-impacted tropical forest. J. Ecol. 97, 1346–1359. doi: 10.1111/j.1365-2745.2009.01551.x
Connell, J. H. (1971). “On the role of natural enemies in preventing competitive exclusion in some marine animals and in rain forest trees,” in Dynamics of Populations. Centre for Agricultural Publishing and Documentation, eds P. J. Den Boer and G. R. Gradwell (Wageningen: Centre for Agricultural Publishing and Documentation).
D’andrea, R., Guittar, J., O’dwyer, J. P., Figueroa, H., Wright, S., Condit, R., et al. (2020). Counting niches: abundance-by-trait patterns reveal niche partitioning in a Neotropical forest. Ecology 101:e03019. doi: 10.1002/ecy.3019
Daws, M. I., Pearson, T. R., Burslem, D. F. P., Mullins, C. E., and Dalling, J. W. (2005). Effects of topographic position, leaf litter and seed size on seedling demography in a semi-deciduous tropical forest in Panama. Plant Ecol. 179, 93–105. doi: 10.1007/s11258-004-5801-4
De Campos Franci, L., Nabe-Nielsen, J., Svenning, J.-C., and Martins, F. R. (2016). Short-term spatial variation in the demography of a common Neotropical liana is shaped by tree community structure and light availability. Plant Ecol. 217, 1273–1290. doi: 10.1007/s11258-016-0655-0
Delissio, L. J., Primack, R. B., Hall, P., and Lee, H. (2002). A decade of canopy-tree seedling survival and growth in two Bornean rain forests: persistence and recovery from suppression. J. Trop. Ecol. 18, 645–658. doi: 10.1017/S0266467402002420
Dewalt, S. J., Taylor, B. N., and Ickes, K. (2015). Density-dependent survival in seedlings differs among woody life-forms in tropical wet forests of a Caribbean island. Biotropica 47, 310–319. doi: 10.1111/btp.12216
Downey, H., Lewis, O. T., Bonsall, M. B., Fernandez, D. C., and Gripenberg, S. (2018). Insect herbivory on seedlings of rainforest trees: effects of density and distance of conspecific and heterospecific neighbors. Ecol. Evol. 8, 12702–12711. doi: 10.1002/ece3.4698
Du, H., Hu, F., Zeng, F., Wang, K., Peng, W., Zhang, H., et al. (2017). Spatial distribution of tree species in evergreen-deciduous broadleaf karst forests in southwest China. Sci. Rep. 7:15664. doi: 10.1038/s41598-017-15789-5
Endara, M. J., and Jaramillo, J. L. (2011). The influence of microtopography and soil properties on the distribution of the speciose genus of trees, Inga (Fabaceae: Mimosoidea), in Ecuadorian Amazonia. Biotropica 43, 157–164. doi: 10.1111/j.1744-7429.2010.00668.x
Forrister, D. L., Endara, M.-J., Younkin, G. C., Coley, P. D., and Kursar, T. A. (2019). Herbivores as drivers of negative density dependence in tropical forest saplings. Science 363, 1213–1216. doi: 10.1126/science.aau9460
Fricke, E. C., Tewksbury, J. J., and Rogers, H. S. (2014). Multiple natural enemies cause distance-dependent mortality at the seed-to-seedling transition. Ecol. Lett. 17, 593–598. doi: 10.1111/ele.12261
Gomes, E. P. C., De Nascimento, L., Domínguez, A., Balguerías, E., Méndez, J., Fernández-Lugo, S., et al. (2020). Seedling bank demography over 11 years in an island laurel forest, Tenerife, Canary Islands. For. Ecol. Manag. 462:118001. doi: 10.1016/j.foreco.2020.118001
Harms, K. E., Wright, S. J., Calderón, O., Hernández, A., and Herre, E. A. (2000). Pervasive density-dependent recruitment enhances seedling diversity in a tropical forest. Nature 404, 493–495. doi: 10.1038/35006630
Hille Ris Lambers, J., Clark, J. S., and Beckage, B. (2002). Density-dependent mortality and the latitudinal gradient in species diversity. Nature 417, 732–735. doi: 10.1038/nature00809
Huang, T., Huang, C., Lin, Y., and Sun, I. (2022). Seedling survival simultaneously determined by conspecific, heterospecific, and phylogenetically related neighbors and habitat heterogeneity in a subtropical forest in Taiwan. Ecol. Evol. 12:e8525. doi: 10.1002/ece3.8525
Inman-Narahari, F., Ostertag, R., Hubbell, S. P., Giardina, C. P., Cordell, S., and Sack, L. (2016). Density-dependent seedling mortality varies with light availability and species abundance in wet and dry Hawaiian forests. J. Ecol. 104, 773–780. doi: 10.1111/1365-2745.12553
Janzen, D. H. (1970). Herbivores and the number of tree species in tropical forests. Am. Naturalist 104, 501–528. doi: 10.1086/282687
Jenik, J., and Hall, J. B. (1966). The ecological effects of the harmattan wind in the djebobo massif (Togo Mountains. Ghana). J. Ecol. 54, 767–779. doi: 10.2307/2257816
Jia, S., Wang, X., Yuan, Z., Lin, F., Ye, J., Lin, G., et al. (2020). Tree species traits affect which natural enemies drive the Janzen-Connell effect in a temperate forest. Nat. Commun. 11:286. doi: 10.1038/s41467-019-14140-y
Jiang, F., Cadotte, M. W., and Jin, G. (2022). Size-and environment-driven seedling survival and growth are mediated by leaf functional traits. Proc. R. Soc. B 289:20221400. doi: 10.1098/rspb.2022.1400
Jin, Y., Russo, S. E., and Yu, M. (2018). Effects of light and topography on regeneration and coexistence of evergreen and deciduous tree species in a Chinese subtropical forest. J. Ecol. 106, 1634–1645. doi: 10.1111/1365-2745.12911
Johnson, D. J., Condit, R., Hubbell, S. P., and Comita, L. S. (2017). Abiotic niche partitioning and negative density dependence drive tree seedling survival in a tropical forest. Proc. R. Soc. B Biol. Sci. 284:201722. doi: 10.1098/rspb.2017.2210
King, D. A., Wright, S. J., and Connell, J. H. (2006). The contribution of interspecific variation in maximum tree height to tropical and temperate diversity. J. Trop. Ecol. 22, 11–24. doi: 10.1017/S0266467405002774
Kobe, R. K. (1999). Light gradient partitioning among tropical tree species through differential seedling mortality and growth. Ecology 80, 187–201. doi: 10.1890/0012-9658(1999)080[0187:LGPATT]2.0.CO;2
Kobe, R. K., and Vriesendorp, C. F. (2011). Conspecific density dependence in seedlings varies with species shade tolerance in a wet tropical forest. Ecol. Lett. 14, 503–51010. doi: 10.1111/j.1461-0248.2011.01612.x
Lebrija-Trejos, E., Wright, S. J., Hernández, A., and Reich, P. B. (2014). Does relatedness matter? Phylogenetic density-dependent survival of seedlings in a tropical forest. Ecology 95, 940–951. doi: 10.1890/13-0623.1
Ledo, A., and Schnitzer, S. A. (2014). Disturbance and clonal reproduction determine liana distribution and maintain liana diversity in a tropical forest. Ecology 95, 2169–2178. doi: 10.1890/13-1775.1
Lin, L., Comita, L. S., Zheng, Z., and Cao, M. (2012). Seasonal differentiation in density-dependent seedling survival in a tropical rain forest. J. Ecol. 100, 905–914. doi: 10.1111/j.1365-2745.2012.01964.x
Lin, Y. C., Comita, L. S., Johnson, D. J., Chen, M. R., and Wu, S. H. (2017). Biotic vs abiotic drivers of seedling persistence in a tropical karst forest. J. Veg. Sci. 28, 206–217. doi: 10.1111/jvs.12479
Lu, D., Wang, G. G., Yu, L., Zhang, T., and Zhu, J. (2018). Seedling survival within forest gaps: the effects of gap size, within-gap position and forest type on species of contrasting shade-tolerance in Northeast China. For. Int. J. For. Res. 91, 470–479. doi: 10.1093/forestry/cpy007
Lu, J., Johnson, D. J., Qiao, X., Lu, Z., Wang, Q., and Jiang, M. (2015). Density dependence and habitat preference shape seedling survival in a subtropical forest in central China. J. Plant Ecol. 8, 568–577. doi: 10.1093/jpe/rtv006
Ma, L., Chen, C., Shen, Y., Wu, L.-F., Huang, Z.-L., and Cao, H.-L. (2014). Determinants of tree survival at local scale in a sub-tropical forest. Ecol. Res. 29, 69–80. doi: 10.1007/s11284-013-1100-7
Martini, F., Zou, C., and Goodale, U. M. (2019). Intrinsic biotic factors and microsite conditions drive seedling survival in a species with masting reproduction. Ecol. Evol. 9, 14261–14272. doi: 10.1002/ece3.5861
Matthesius, A., Chapman, H., and Kelly, D. (2011). Testing for Janzen–Connell effects in a west African montane forest. Biotropica 43, 77–83. doi: 10.1111/j.1744-7429.2010.00664.x
Méndez-Toribio, M., Ibarra-Manríquez, G., Navarrete-Segueda, A., and Paz, H. (2017). Topographic position, but not slope aspect, drives the dominance of functional strategies of tropical dry forest trees. Environ. Res. Lett. 12:085002. doi: 10.1088/1748-9326/aa717b
Moustakas, A., and Evans, M. R. (2015). Effects of growth rate, size, and light availability on tree survival across life stages: a demographic analysis accounting for missing values and small sample sizes. BMC Ecol. 15:6. doi: 10.1186/s12898-015-0038-8
Nagamatsu, D., Seiwa, K., and Sakai, A. (2002). Seedling establishment of deciduous trees in various topographic positions. J. Veg. Sci. 13, 35–44. doi: 10.1111/j.1654-1103.2002.tb02021.x
O’Brien, M. J., and Escudero, A. (2022). Topography in tropical forests enhances growth and survival differences within and among species via water availability and biotic interactions. Funct. Ecol. 36, 686–698. doi: 10.1111/1365-2435.13977
Oshima, C., Tokumoto, Y., and Nakagawa, M. (2015). Biotic and abiotic drivers of dipterocarp seedling survival following mast fruiting in Malaysian Borneo. J. Trop. Ecol. 31, 129–137. doi: 10.1017/S026646741400073X
Paine, C. E. T., and Harms, K. E. (2009). Quantifying the effects of seed arrival and environmental conditions on tropical seedling community structure. Oecologia 160, 139–150. doi: 10.1007/s00442-008-1269-6
Punchi-Manage, R., Getzin, S., Wiegand, T., Kanagaraj, R., Savitri Gunatilleke, C. V., Nimal Gunatilleke, I. A. U., et al. (2013). Effects of topography on structuring local species assemblages in a Sri Lankan mixed dipterocarp forest. J. Ecol. 101, 149–160. doi: 10.1111/1365-2745.12017
R Core Team (2018). R: a Language and Environment for Statistical Computing. Vienna: R Foundation for Statistical Computing.
Ramage, B. S., Johnson, D. J., Gonzalez-Akre, E., Mcshea, W. J., Anderson-Teixeira, K. J., Bourg, N. A., et al. (2017). Sapling growth rates reveal conspecific negative density dependence in a temperate forest. Ecol. Evol. 7, 7661–7671. doi: 10.1002/ece3.3298
Schnitzer, S. A., and Bongers, F. (2011). Increasing liana abundance and biomass in tropical forests: emerging patterns and putative mechanisms. Ecol. Lett. 14, 397–406. doi: 10.1111/j.1461-0248.2011.01590.x
Schnitzer, S. A., Mangan, S. A., Dalling, J. W., Baldeck, C. A., Hubbell, S. P., Ledo, A., et al. (2012). Liana abundance, diversity, and distribution on Barro Colorado Island. Panama. PLoS One 7:e52114. doi: 10.1371/journal.pone.0052114
Schwarz, P. A., Fahey, T. J., and Mcculloch, C. E. (2003). Factors controlling spatial variation of tree species abundance in a forested landscape. Ecology 84, 1862–1878. doi: 10.1890/0012-9658(2003)084[1862:FCSVOT]2.0.CO;2
Singh, S. (2018). Understanding the role of slope aspect in shaping the vegetation attributes and soil properties in Montane ecosystems. Trop. Ecol. 59, 417–430.
Song, X., Hogan, J. A., Lin, L., Wen, H., Cao, M., and Yang, J. (2018). Canopy openness and topographic habitat drive tree seedling recruitment after snow damage in an old-growth subtropical forest. For. Ecol. Manag. 429, 493–502. doi: 10.1016/j.foreco.2018.07.038
Spicer, M. E., Mellor, H., and Carson, W. P. (2020). Seeing beyond the trees: a comparison of tropical and temperate plant growth forms and their vertical distribution. Ecology 101: e02974. doi: 10.1002/ecy.2974
Ssali, F., Moe, S., and Sheil, D. (2019). Damage to artificial seedlings across a disturbed Afromontane forest landscape. Biotropica 51, 652–663. doi: 10.1111/btp.12703
Stoorvogel, J. J., Van Breemen, N., and Jassen, B. H. (1997). The nutrient input by Harmattan dust to a forest ecosystem in Cote d’Ivoire, Africa. Biogeochemistry 37, 145–157. doi: 10.1023/A:1005734225727
Sun, Z., Prachanun, N., Sonsuthi, A., Chanthorn, W., Brockelman, W. Y., Nathalang, A., et al. (2022). Whole-plant seedling functional traits suggest lianas also support “Fast-Slow” plant economics spectrum. Forests 13:990. doi: 10.3390/f13070990
Terborgh, J., Zhu, K., Álvarez-Loayza, P., and Valverde, F. C. (2014). How many seeds does it take to make a sapling? Ecology 95, 991–999. doi: 10.1890/13-0764.1
Umaña, M. N., Forero-Montaña, J., Nytch, C. J., Thompson, J., Uriarte, M., Zimmerman, J., et al. (2019). Dry conditions and disturbance promote liana seedling survival and abundance. Ecology 100:e02556. doi: 10.1002/ecy.2556
Umaña, M. N., Manzané-Pinzón, E., and Comita, L. S. (2020). Long-term dynamics of liana seedlings suggest decelerating increases in liana relative abundance over time. J. Ecol. 108, 460–469. doi: 10.1111/1365-2745.13297
Umaña, M. N., Zipkin, E. F., Zhang, C., Cao, M., Lin, L., and Swenson, N. G. (2018). Individual-level trait variation and negative density dependence affect growth in tropical tree seedlings. J. Ecol. 106, 2446–2455. doi: 10.1111/1365-2745.13001
Venegas-González, A., Mello, F., Schnitzer, S., Cesar, R., and Filho, M. (2020). The negative effect of lianas on tree growth varies with tree species and season. Biotropica 52, 836–844. doi: 10.1111/btp.12796
Vogado, N. O., Engert, J. E., Linde, T. L., Campbell, M. J., Laurance, W. F., and Liddell, M. J. (2022). Climate change affects reproductive phenology in lianas of australia’s wet tropics. Front. For. Glob. Change 5:787950. doi: 10.3389/ffgc.2022.787950
Wright, S. J., Kitajima, K., Kraft, N. J., Reich, P. B., Wright, I. J., Bunker, D. E., et al. (2010). Functional traits and the growth–mortality trade-off in tropical trees. Ecology 91, 3664–3674. doi: 10.1890/09-2335.1
Wright, S. J., Muller-Landau, H. C., Calderón, O., and Hernandéz, A. (2005). Annual and spatial variation in seedfall and seedling recruitment in a neotropical forest. Ecology 86, 848–860. doi: 10.1890/03-0750
Wu, H., Franklin, S. B., Liu, J., and Lu, Z. (2017). Relative importance of density dependence and topography on tree mortality in a subtropical mountain forest. For. Ecol. Manag. 384, 169–179. doi: 10.1016/j.foreco.2016.10.049
Xia, S.-W., Chen, J., Schaefer, D., and Goodale, U. M. (2016). Effect of topography and litterfall input on fine-scale patch consistency of soil chemical properties in a tropical rainforest. Plant Soil 404, 385–398. doi: 10.1007/s11104-016-2854-9
Keywords: Afromontane forest, biotic neighbourhood, negative density dependence, Ngel Nyaki, Nigeria, seedling survival
Citation: Abiem I, Kenfack D and Chapman HM (2023) Assessing the impact of abiotic and biotic factors on seedling survival in an African montane forest. Front. For. Glob. Change 6:1108257. doi: 10.3389/ffgc.2023.1108257
Received: 25 November 2022; Accepted: 09 February 2023;
Published: 23 February 2023.
Edited by:
Alexandra C. Morel, University of Dundee, United KingdomReviewed by:
Zerihun Woldu, Addis Ababa University, EthiopiaPaul Dutton, Waikato Regional Council, New Zealand
Copyright © 2023 Abiem, Kenfack and Chapman. This is an open-access article distributed under the terms of the Creative Commons Attribution License (CC BY). The use, distribution or reproduction in other forums is permitted, provided the original author(s) and the copyright owner(s) are credited and that the original publication in this journal is cited, in accordance with accepted academic practice. No use, distribution or reproduction is permitted which does not comply with these terms.
*Correspondence: Iveren Abiem, abiemiveren@gmail.com