- 1Forest Ecology and Forest Management Group, Wageningen University, Wageningen, Netherlands
- 2Departamento de Ecología y Recursos Naturales, Facultad de Ciencias, Universidad Nacional Autónoma de México, Mexico City, Mexico
- 3Ejido Mena Nizanda, Asunción Ixtaltepec, Oaxaca, Mexico
- 4Posgrado en Ciencias Biológicas, Unidad de Posgrado, Universidad Nacional Autónoma de México, Mexico City, Mexico
Introduction: Tropical dry forests (TDF) are not only the most widespread tropical forest type but also the most threatened forest ecosystem worldwide. Yet, because their dynamics have been insufficiently studied, our knowledge about the factors responsible for the spatial and temporal variability in TDF dynamics remains very limited. In this study, we aimed (1) to assess the effects of two lithological substrates on TDF structure, diversity, and species composition, and (2) to determine whether and how these substrates affect temporal dynamics on TDF attributes.
Methods: We used information gathered through a 12-year long annual monitoring of 14 old-growth TDF permanent plots in southern Mexico, seven of which were established on limestone and seven on phyllite. Previous work shows that limestone-derived soils have higher humidity, conductivity, and phosphorous and calcium contents, but lower nitrogen content.
Results: TDF structure and diversity attributes were consistently higher on limestone TDF, while canopy height was higher on phyllite TDF. By contrast, temporal variation in TDF attributes, demographic rates (recruitment, growth, and mortality) and attribute residence times were indistinguishable between substrates. We also found a strong differentiation of species composition between substrates, yet substrates did not influence the temporal behavior of composition.
Discussion: Our results suggest that limestone is a more favorable environment for TDF development (soil-effects mechanism), but the flora of the forest on phyllite seems to be better adapted to cope with harsher environmental conditions (composition-effects mechanism), which counterbalances the environmental advantage of limestone, ultimately resulting in spatially homogeneous TDF dynamics at the landscape level. Future studies should examine the role of functional attributes on old-growth TDF dynamics, as this will allow a better understanding of the impacts of extreme climatic events on forest attributes and their dynamics, as well as to foresee potential ecosystem state shifts and tipping points.
1. Introduction
Tropical forests cover nearly 18.3 million km2 across five continents (Food and Agriculture Organization [FAO], 2020). Considering this immense area, it is natural that the regions where they occur encompass a huge variety of climates, topographies, and geologies, which results in an outstanding heterogeneity in tropical forest features, including their physiognomy, structure, biodiversity, and ecosystem functioning (Walter, 1973; ter Steege et al., 2006). Such a colossal variety of tropical forests is amazing and intriguing. Yet, at the same time it complicates their understanding, so that after several decades of study we have only attained the still limited knowledge that we currently have on their structure, diversity, and functioning.
One of the least understood tropical forests properties is their dynamics. Forest dynamics can be defined as the set of changes in stand structure, species composition and biotic interactions that forests experience over time (Frelich, 2016; McDowell et al., 2020). Forest dynamics arises from the complex interaction between the inherent demographic processes that plants undergo through their life cycles (recruitment, growth, reproduction and death) and the external factors that determine resources and conditions for plant development (e.g., climate and soil) (McDowell et al., 2020). External factors also include disturbances, which are sudden changes in environmental conditions resulting in the removal of biomass and individuals from the forest community. Good knowledge on tropical forest dynamics is essential for several purposes, ranging from the design of sound forest management practices to anticipating their responses to climatic changes (McDowell et al., 2020). At present, there is a sizeable number of studies on tropical moist and rain forest dynamics (Grubb, 1996; Sheil et al., 2000; Condit et al., 2004; Lewis et al., 2004; Laurance et al., 2009), but our understanding of tropical dry forest (TDF) dynamics lags considerably behind (Siyum, 2020; Muñoz et al., 2021). This is unfortunate, as TDFs account for more than half of the forest cover across the tropics and represent the tropical ecosystem most threatened by human activities (Janzen, 1988; Miles et al., 2006; Chaturvedi et al., 2017; Hasnat and Hossain, 2020).
Lithological substrates, hereafter referred to only as substrates, are a key factor explaining tropical forest heterogeneity within landscapes (Durán et al., 2006; Fayolle et al., 2012). Lithological substrates are the main prime material that soils are made of, and thus they largely determine soil physical attributes and chemical composition (Searcy et al., 2003). Substrates may potentially influence tropical forest attributes either (1) directly, through their impact on soil properties, mainly nutrient composition, texture, and water retention capacity (Peña-Claros et al., 2012; Hahm et al., 2014; Callahan et al., 2022), or (2) indirectly, through their influence on forest species composition (Searcy et al., 2003; Ribeiro et al., 2007). These two mechanisms will ultimately determine how substrates impact forest structure, diversity, and their temporal dynamics.
This study focuses on the TDF region of Nizanda, a climatically homogeneous area located in southern Mexico (Pérez-García et al., 2010). TDF in this region grows on soils derived from bedrocks of limestone (carbonate sedimentary rock) and siliciclastic phyllite (foliated metamorphic rock, hereafter phyllite) (Pérez-Gutiérrez et al., 2009). Lithology-driven differences in structure and physiognomy have been observed between the two communities. Forest growing on limestone tends to be better developed (i.e., bigger trees, more biomass) and richer in tree species compared to its counterpart on phyllite (Muñoz, 2015), apparently due to higher soil water content and nutrient availability in limestone-derived soils (Sandoval-Granillo, 2020). However, these differences in forest attributes have not been formally assessed, and it is unclear whether they are associated with different dynamic behaviors.
The objectives of this study were (1) to assess substrate effects on TDF structure, diversity, and species composition, and (2) to determine whether and how substrates affect temporal dynamics on these TDF attributes. Regarding (1), we hypothesized that more benign environmental conditions in limestone TDF (higher soil water content and nutrient availability) lead to higher vegetation development and diversity, while also contributing to a distinct species composition compared to TDF growing on phyllite, which offers harsher or more restrictive conditions for plant growth. Regarding (2), we hypothesized three alternative scenarios based on the two mechanisms of geological effects advanced earlier, i.e., direct effects on forest dynamics through soil conditions differentially affecting the same set of species (soil-effects mechanism) against indirect effects on forest dynamics through species composition, where different species respond differently to environmental factors (composition-effects mechanism). If phyllite represents a harsher environment for TDF growth than limestone, it is likely that trees growing on this substrate are better adapted to cope with water and nutrient limitations (Scholz et al., 2014; Díaz-Castellanos et al., 2022). In this case, the soil-effects mechanism would result in increased stability of limestone TDF by providing higher and more stable soil water content, regardless of interannual variation in precipitation. Conversely, based on the composition-effects mechanism, it is reasonable to expect higher forest stability on phyllite TDF because this community hosts an array of species furnished with more conservative functional traits, enabling them to better resist the impacts of climate-harsh (dry and hot) years at the cost of growing slower in climate-benign (wet and cool) years, in line with the fast-slow continuum hypothesis (Reich, 2014; Oliveira et al., 2021). Therefore, depending on the relative importance of each mechanism for TDF dynamics, these forests may be (1) more stable on limestone if the soil-effects mechanism acts with higher intensity, (2) more stable on phyllite if the composition-effects mechanism acts with higher intensity, or (3) equally stable across bedrocks if the two mechanisms act with similar intensity.
We examined the effects of two lithological substrates on TDF attributes and their dynamics by using annual information gathered on the vegetation of both substrates over 12 years. The results of this study will provide new insights into the role of lithology on tropical forest development potential and its responses to environmental and climate changes. Given the large extent of TDF across the tropics (Murphy and Lugo, 1986; Hasnat and Hossain, 2020) and the variety of lithologies characterizing the landscapes where it occurs (Mooney et al., 1995), the results of this study will advance the understanding of TDF dynamics at landscape and regional scales.
2. Materials and methods
2.1. Study site and vegetation monitoring
We conducted our study in the region of Nizanda, southern Mexico (16.66°N, 95.01°W; Figure 1). The region has a mean annual temperature of 27.7 °C and a mean annual precipitation of 902 mm year–1, with a marked rainy season spanning from mid-May to mid-October (precipitation > 50 mm month–1). Tropical dry forest (TDF) is the dominant vegetation type in the region and large undisturbed forest patches are still present in the landscape, alongside areas devoted to food production (mechanized and shifting agriculture, and cattle ranching) and extensive patches of secondary vegetation in different stages of succession. TDF in this region occurs on limestone and siliciclastic phyllite, two contrasting parental materials whose weathering results in different soil types. Limestone outcrops are associated with major faults in the terrain and can reach elevations of ca. 500 m above mean sea level (amsl). By contrast, the terrain on phyllite is hilly with much lower elevations (ca. 280 m amsl at most). These two substrates represent the main parental material of the most common soil types (Cambisols, Leptosols, and Regosols) on which the tropical dry forest develops (Miguel-Talonia, unpublished data). The physical characteristics of these soils such as color (an organic matter content indicator) and texture differ notably between the two substrates. Overall, limestone-derived soils are darker and have higher clay contents, and in some places of the limestone mountains the high clay content results in shrink-swell cracks during the dry season; consequently, water availability for plants is higher in limestone- than in phyllite-derived soils. Therefore, soil water content emerges as one of the major factors potentially driving differences for TDF development on these substrates (Covelo et al., 2017). In Nizanda, physiognomic differences between TDF developing on the two substrates are evident, and these seem to be linked to variations in structure and diversity between the two TDF subtypes.
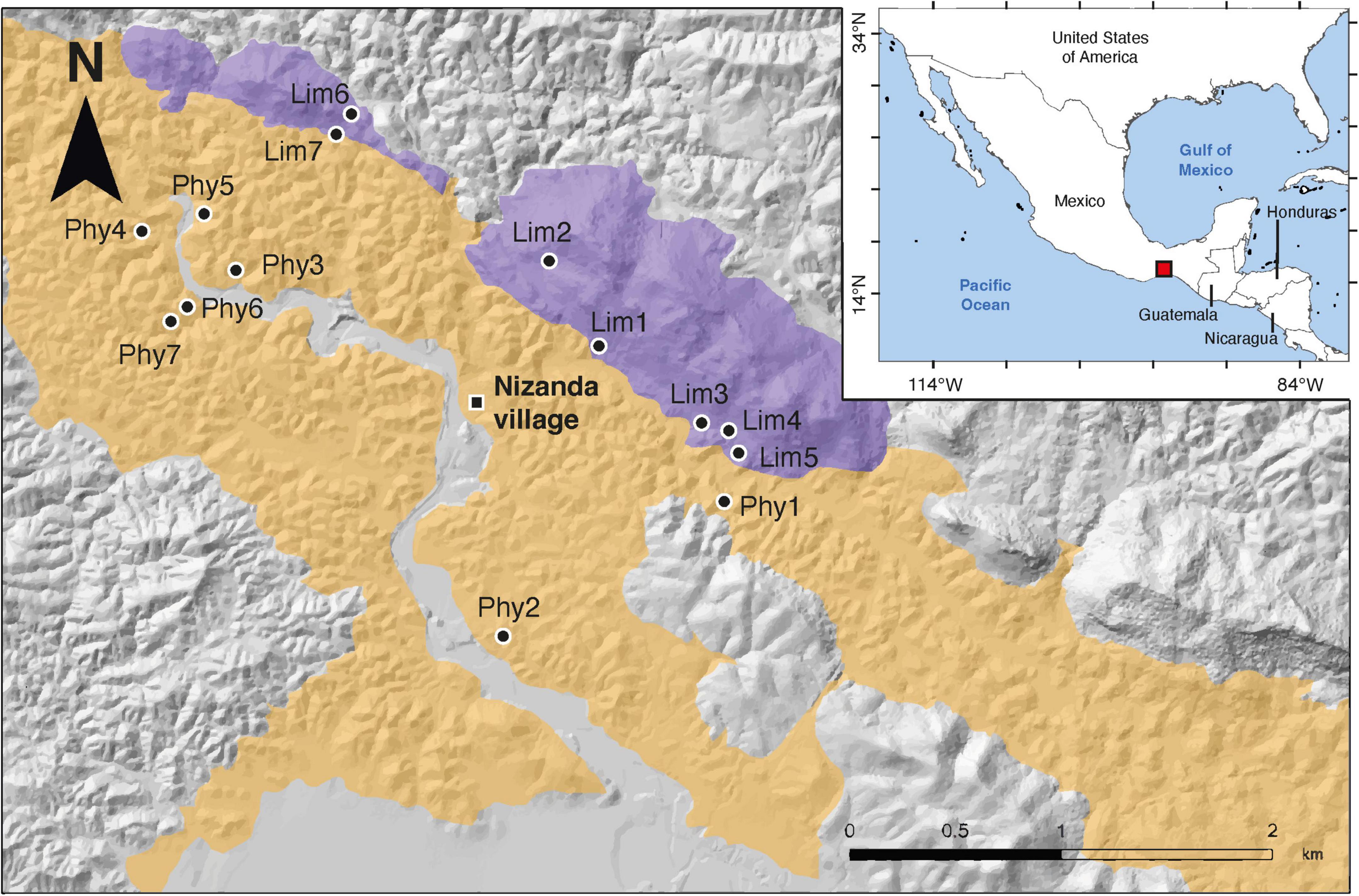
Figure 1. Map of the study area in the tropical dry forest (TDF) region of Nizanda, Mexico. Locations of the permanent old-growth TDF plots on soils derived from limestone bedrock (purple shading) and siliciclastic phyllite bedrock (yellow shading) are shown. Gray shading represents other parental materials occurring in the area (see Pérez-Gutiérrez et al., 2009). Inset map shows the location of the Nizanda region within Mexico.
In 2008 we established seven 500 m2 permanent forest plots in each forest subtype (14 permanent plots in total; Figure 1). At present, we continue monitoring these plots but for this study we used data for the 2008–2020 period. The plots are subdivided in five 100-m2 transects, each of which is further subdivided into four 25 m2 quadrats. We used a stratified, nested sampling design for measuring trees in the plots. Stems with a diameter at breast height (DBH) ≥ 5 cm were measured in the whole 500 m2 plot area, medium-sized stems with a DBH ≥ 2.5 cm were measured in a 375 m2 plot subarea, while small stems with a DBH ≥ 1 cm were only measured in a 125 m2 plot subarea. In each transect, 25 m2 quadrats were randomly assigned a minimum DBH criterion (5, 2.5 or 1 cm). All trees meeting the inclusion criterion in the quadrat were tagged, identified to species (96.1 % of all trees), and their DBH and heights were measured. At each annual census, survival, DBH, and height were recorded, as well as new recruits meeting the inclusion criteria.
2.2. Data analysis
Based on the annual vegetation censuses of permanent plots, we estimated four forest structure variables [basal area, aboveground biomass (AGB), tree density, and canopy height], and three community diversity variables [species richness (0D), common species (1D), and dominant species (2D)]. Aboveground biomass (AGB) was estimated by using a broadly used allometric equation (Chave et al., 2014) that integrates DBH, height, and species-specific wood density measurements. Canopy height was calculated as the average height of trees on the top canopy layer (details for canopy height estimation are provided in Supplementary Material 1). Community diversity values (0D, 1D, and 2D) correspond to Hill numbers of order q = 0 (total species richness), q = 1 (typical species), and q = 2 (dominant species), which represent the effective number of species in the community when the weight given to rare species is gradually reduced (Chao et al., 2014). These diversity metrics were estimated with the vegan package (Oksanen, 2007) in R by using species abundances. All community variables were estimated on an annual basis per plot.
To assess substrate effects on TDF structure and diversity, we estimated the mean value for the seven response attributes per substrate on an annual basis. Next, 95 % confidence intervals around these means were constructed through bootstrapping with 1,000 iterations per estimation. Mean values and their confidence intervals were plotted against time to illustrate the temporal trajectories of structure and diversity attributes on both substrates.
To compare the dynamics in TDF attributes between substrates, we calculated a mean value per TDF attribute per substrate (including all plot/year combinations over the study period). Then, we standardized individual plot-year estimates by dividing these values by their substrate mean. This allowed us to express plot variability relative to their substrate mean, a procedure that controlled for larger variation caused by larger absolute values in forest attributes. Next, we estimated substrate mean values again with 95 % bootstrapped confidence intervals on an annual basis. Standardized TDF attributes were plotted against time to visualize the temporal trajectories of the TDFs growing on the two substrates. For all forest attributes, a value of 1 represented the substrate average value for the entire study period.
Next, we assessed quantitative differences in TDF dynamics between substrates by comparing plot deviations from their substrate mean using linear mixed-effects models (LMM). For each standardized TDF attribute, we computed the absolute deviations between individual plot values and their entire-period substrate mean on an annual basis (i.e., |1–standardized plot value|). We then fitted an LMM of individual plot-year deviations as a function of substrate, adjusting a random intercept for each permanent plot. By including a plot-dependent intercept, we controlled for the pseudo-replication arising from repeatedly measuring the same plots over time. To assess whether variation in TDF dynamics is determined by substrates, we extracted the substrate coefficient from the previously fitted LMMs and then estimated their 95 % bootstrapped confidence intervals with 1,000 iterations. Coefficient estimates and their confidence intervals were plotted separately for each forest attribute to show whether TDF dynamics was more intense either on limestone or on phyllite.
We also compared differences in demographic rates (net rate of change, recruitment, growth, mortality, and residence time) between substrates for basal area, AGB, tree density, and species richness (0D). These four variables are, to some extent, additive forest attributes which can be decomposed into their gain and loss demographic components. To this end, we analyzed the individual tree trajectories over time (2008–2020) and determined, on an annual basis, what demographic process a given individual was undergoing: either recruitment, survival, or death. Based on these demographic classification, we determined the contribution of an individual toward a specific state variable (positive, negative, or null), and then added those change rates per demographic process, year, and plot (Muñoz et al., 2021). We performed this procedure for all four state variables analyzed. Later, we computed residence times per state variable as in Galbraith et al. (2013) by using Equation 1.
where τY is the residence time of a unit of the state variable Y, is the average value for Y during a yearly period, and is the productivity in Y during the yearly period resulting from the addition of recruitment and growth. We did not differentiate between biomass and density formulas as in Galbraith et al. (2013) to enable direct comparisons between the residence times of the four attributes analyzed. Annual demographic rates were averaged per plot and then per substrate to obtain substrate-level estimates. Bootstrapped confidence intervals (95 %, 1,000 iterations) were computed to compare demographic rates between substrates.
To assess whether sudden decreases and increases in TDF attributes were associated to El Niño and La Niña events (which are known to promote dry and rainy weather conditions, respectively), we ran non-parametric (Spearman) correlations between the Oceanic El Niño Index [ONI, referred to as NINO3.4 in van Oldenborgh et al. (2021)] and the net rates of change in all seven forest attributes. ONI values were retrieved from the National Oceanic and Atmospheric Administration historical El Niño/La Niña episodes (National Oceanic and Atmospheric Administration [NOAA], 2022). Net rates of change were retrieved from the analysis of demographic rates for basal area, AGB, tree density and species richness; for canopy height and diversity metrics 1D and 2D, rates of change were simply computed as (Yt+1–Yt)/Yt. Correlation coefficients and their respective P-values were reported per substrate. Although this analysis was not essential to test the core hypotheses of this work, we considered it would be appropriate to explain notorious tendencies on the dynamics of TDF attributes.
To visualize differences in species composition between TDF substrates, as well as their differences in dynamics, we used a non-parametric multidimensional scaling ordination (NMDS) of species composition and abundance data for all plots and years. Species were considered as variables and plot-year combinations were considered as observations. We used Bray-Curtis distance to estimate the dissimilarity matrices for the analysis, and projected the NMDS scores into a bidimensional scatterplot. Permanent plots from the same substrate were enclosed into an ellipse to show the ordination space occupied by each substrate based on its species composition. The NMDS ordination was performed using the vegan package in R (Oksanen, 2007).
To quantify differences in species composition dynamics between substrates, we computed three metrics of composition dynamics: annual mean distance, dispersion from centroid, and distance from initial to final point. Annual mean distance quantified the distance that any plot moved on average on the ordination space during an annual period. Dispersion from centroid quantified how broadly or tightly spread all datapoints belonging to each plot were on the ordination space. Distance from initial to final point, as its name implies, quantifies the distance between the 2008 and 2020 datapoints on the ordination space, and represents a proxy of circularity (i.e., floristic stability) in individual plot trajectories. To assess differences in these three metrics between substrates, we performed an LMM for annual mean distance and dispersion from centroid, and a standard linear regression for distance from initial to final point. In the three models, substrate was set as the fixed factor. For the LMMs, a plot-dependent random intercept was adjusted to account for the temporal autocorrelation between datapoints. Coefficients were extracted from the three models, and their 95 % bootstrapped confidence intervals were computed. The coefficients along with their confidence intervals were plotted to show differences in composition dynamics between substrates.
The datasets and the R code necessary to replicate all analyses, tables, and figures of this study were deposited in a Zenodo repository for reproduction and validation purposes (Muñoz et al., 2023).
3. Results
3.1. Comparison of TDF attributes between substrates
The TDF on limestone had consistently higher values for almost all forest attributes than the TDF on phyllite except for canopy height. The largest differences between substrates were observed for diversity variables, with limestone TDF displaying higher diversity, while differences in structure attributes were less pronounced (Figures 2A–G).
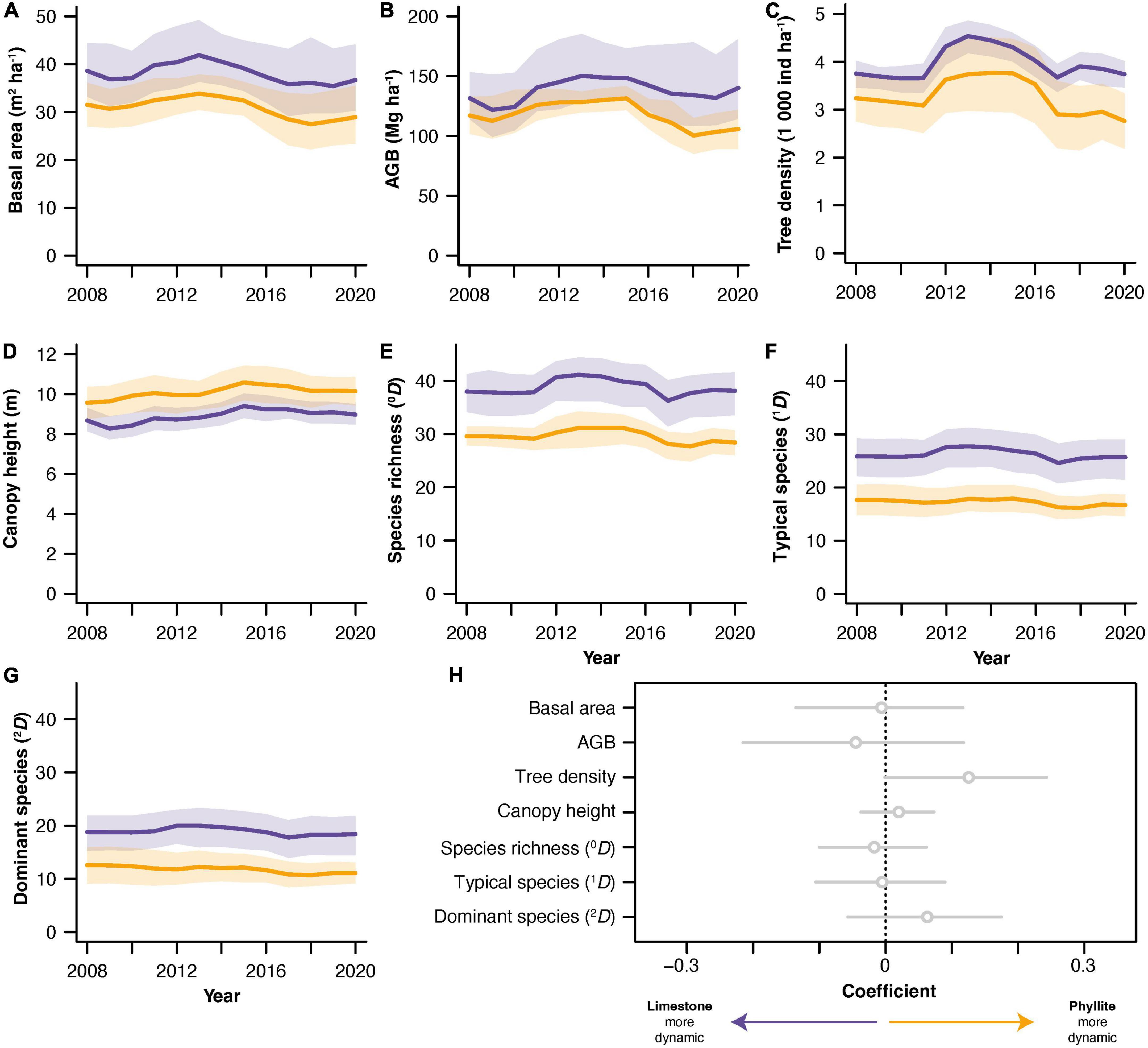
Figure 2. Dynamics of tropical dry forest attributes in limestone (purple) and siliciclastic phyllite (yellow) substrates. Panels show the dynamics of (A) basal area, (B) aboveground biomass (AGB), (C) tree density, (D) canopy height, (E) species richness (0D), (F) number of common species (1D), (G) number of dominant species (2D), and (H) the substrate-wise comparison of the dynamics of all seven TDF attributes analyzed. For panels (A–G), the thick lines represent substrate mean values (n = 7) and the shading around them represents 95 % confidence intervals. For panel (H), the substrate-wise comparison of dynamics was done by regressing the deviations of each plot from its substrate mean value as a function of forest substrate standardized dynamics shown in Supplementary Figure 1. Linear mixed-effects models were used to control for temporal autocorrelation due to repeated measurements, including plots as a random effect. Coefficients = 0 represent identical attribute dynamics between the two substrates, coefficients > 0 represent more intense dynamics in phyllite TDF, and coefficients < 0 represent more intense dynamics in limestone TDF. Mean coefficients and their 95 % confidence intervals are shown for each attribute.
3.2. Forest dynamics between substrates
The dynamic behavior of all TDF attributes was remarkably similar over time in terms of direction at both substrates, with limestone and phyllite TDF following nearly parallel trajectories (Figures 2A–G and Supplementary Figure 1). This weak differentiation between substrates was particularly evident for the standardized TDF attributes, which followed almost identical patterns over time on limestone and phyllite, both in terms of direction and magnitude of the variation on their dynamics (Supplementary Figure 1). Similarly, LMM modeling did not reveal a significant role of parental bedrock on the variability of TDF dynamics; the analysis of plot deviations in standardized TDF attributes showed that substrates did not yield large differences in dynamics for any of the attributes analyzed (Figure 2H).
3.3. Analysis of demographic rates
Temporal trends in net change, recruitment, growth, and mortality were remarkably similar between substrates over time (Figures 3A–D). Also, the analysis of mean demographic rates did not reveal any differences between demographic rates for any of the four TDF attributes analyzed from this demographic perspective (basal area, AGB, tree density, and species richness; Figures 3E–H). Similarly, residence times, which were estimated from recruitment, growth, and attribute values, did not show differences for any of the four TDF attributes analyzed (Figures 3I–L).
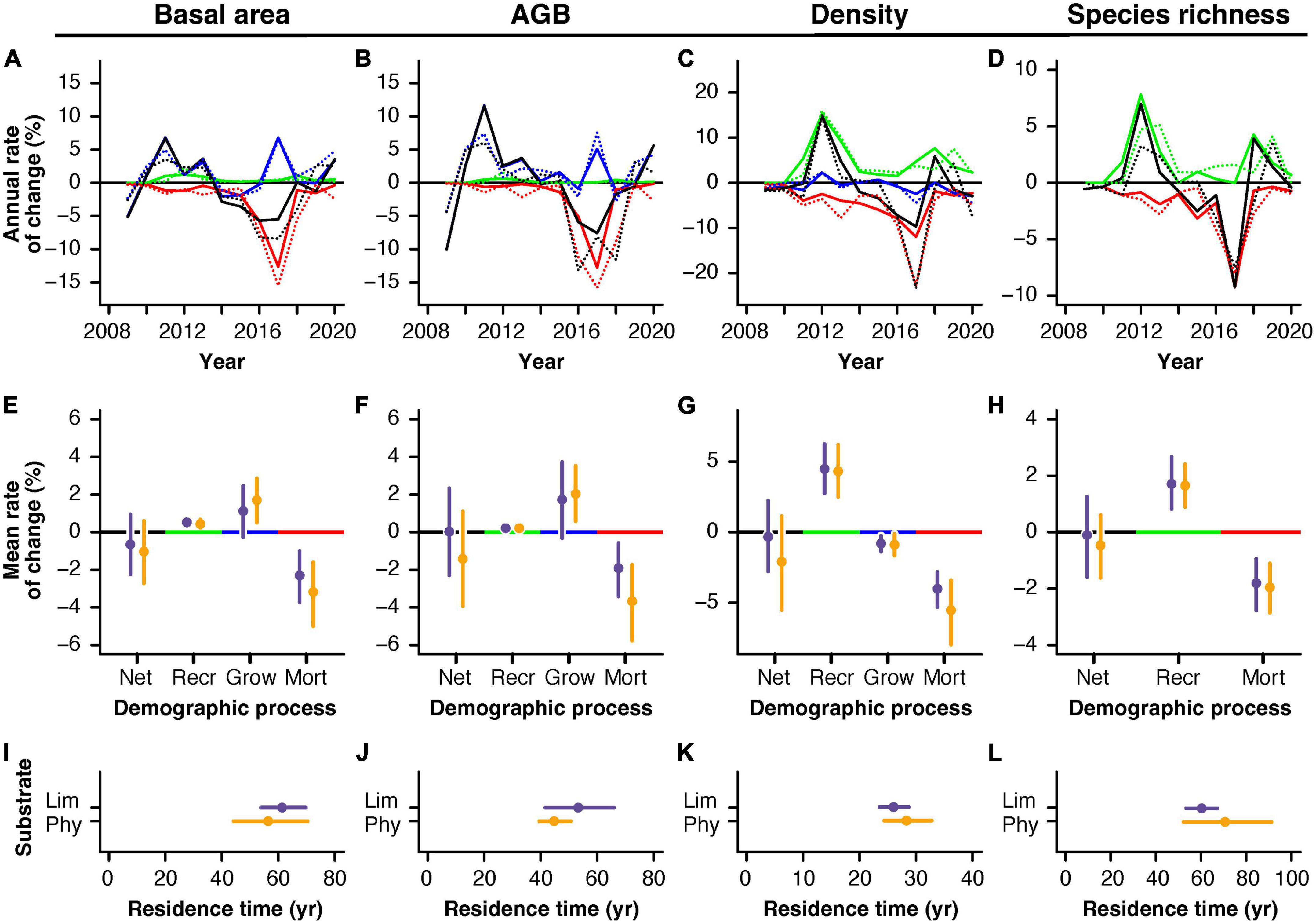
Figure 3. Demographic rates for basal area, aboveground biomass (AGB), tree density and species richness (0D) in a tropical dry forest established over limestone and phyllite substrates. (A–D) Temporal behavior of net change (Net; black), recruitment (Recr; green), growth (Grow; blue), and mortality (Mort; red) rates on an annual resolution for limestone (continuous) and phyllite (dotted lines) permanent plots. (E–H) Mean demographic rates per substrate (dots) with 84 % confidence intervals (whiskers); overlapping whiskers around the mean estimates for limestone (purple) and phyllite (yellow) indicate non-significant mean differences with a 95 % confidence level (Goldstein and Healy, 1995). (I–L) Mean residence time for the four forest attributes on limestone and phyllite TDF plots, including mean estimates (dots) and their 84 % confidence intervals (whiskers). Forest attributes represented in each panel are indicated above the panel.
3.4. Species composition dynamics
The NMDS analysis based on species composition recorded over the study period revealed a clear segregation of the plots from the two forest types on the ordination space (Figure 4A). The overlap of the ordination spaces corresponding to the forests on limestone and phyllite was relatively small, pointing to large differences in the presence and abundance of species between substrates. Interestingly, however, the dynamics of floristic composition did not show such a between-substrate differentiation: all three NMDS-based metrics (annual mean distance, dispersion from centroid, and distance between initial and final points) were indistinguishable between substrates (Figure 4B). Therefore, despite clear differences in species composition between the two substrates, there is no evidence of differences in composition dynamics over time.
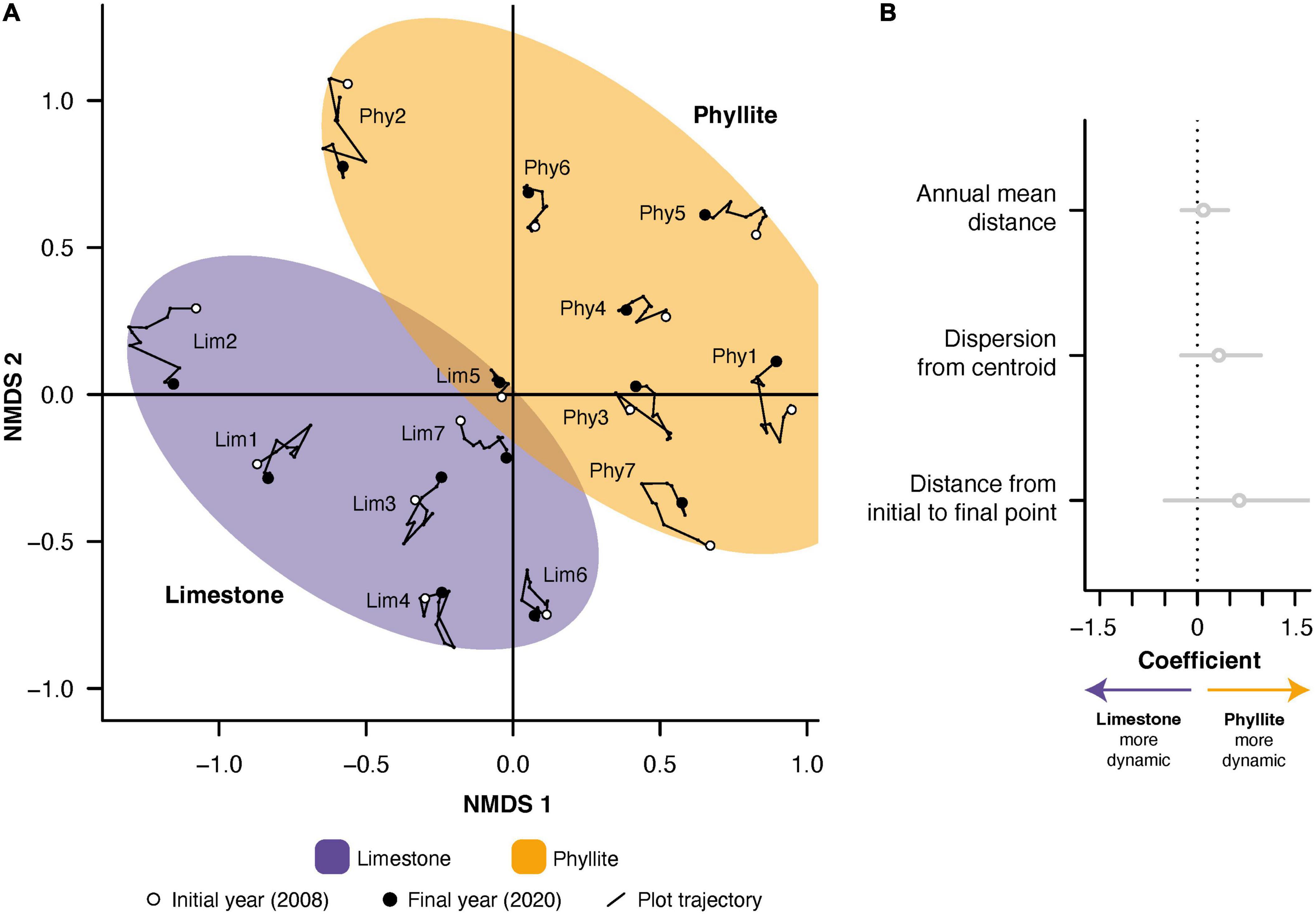
Figure 4. Temporal trajectories of tropical dry forest (TDF) species composition recorded in permanent plots established on limestone and siliciclastic phyllite. (A) NMDS ordination based on species abundance of all annual plot observations. For each plot, lines and dots depict its 12-year long compositional trajectory; open dots represent the initial year of the trajectory (2008), and closed dots represent the endpoint (2020). Purple and yellow ellipses depict the ordination space occupied by limestone and phyllite plots, respectively. (B) Quantitative comparison of three NMDS-based metrics: “Annual mean distance” was calculated as the arithmetic average of all distances between two annual measurements in the NMDS ordination space [i.e., mean length of line segments in panel (A)] for each substrate; “Dispersion from centroid” was calculated as the bidimensional dispersion from a plot’s centroid in the ordination space; “Distance from initial to final point” reflects the floristic difference in each plot between the initial (2008) and final (2020) points, as represented by the distance between them in the ordination space. Coefficients and their 95 % confidence intervals show the lack of significant substrate effects on the three NMDS-based metrics. See Supplementary Table 1 for a full list of the species recorded in the forest on each substrate.
3.5. General patterns in TDF dynamics
In the two forest subtypes, structure and diversity attributes increased around 2010–2011, remained high for several years, and then showed a sudden decrease around 2015–2016 (Figures 2A–G and Supplementary Figure 1). These generalized increments and reductions in vegetation development show some degree of association with both the rainy years produced by La Niña event of 2010–2012 and the drought years arising from El Niño event of 2015–2016 recorded in the region (Supplementary Figure 2).
4. Discussion
4.1. Vegetation development and diversity were higher on limestone
Our results confirmed previous observations that structure and diversity attributes were generally higher in limestone than in phyllite old-growth TDF. The only exception to this pattern was canopy height, which showed higher values in phyllite (Figure 2), probably suggesting different tree architectures and allometric relationships across communities, given that trees in the forest on limestone are taller. The fact that structure and diversity differences were consistent over the entire study period (2008–2020) suggests that this is a permanent pattern rather than a peculiarity of exceptionally dry or wet years. More importantly, these results provide strong support to our first hypothesis, which states that limestone represents a more favorable environment for plant growth and thus for TDF development due to higher water content and nutrient availability, because lime materials enhance the availability and plant uptake of elements such as molybdenum, phosphorus, calcium, and magnesium (Brady, 1984).
Tropical dry forests are typically water limited, as they grow in areas with high temperatures, relatively low annual precipitation and long dry seasons (≥ 3 months, but often up to 6 months) (Sánchez-Azofeifa et al., 2005; Allen et al., 2017). Therefore, they should benefit from a substrate characterized by higher soil water content to maximize water uptake periods. Limestone seems to provide such an environment. Preliminary analyses showed that soils derived from limestone have higher clay and lower sand contents that soils derived from phyllite, with gravimetric humidity being 37 % higher in limestone soils (Sandoval-Granillo, 2020). Therefore, water limitation should be weaker on limestone than on phyllite, consequently yielding a greater potential for vegetation development (Terra et al., 2018). Our observations for four out of the five forest structure variables analyzed here (basal area, AGB, tree density and canopy height) support this idea.
The higher diversity at all levels analyzed (Hill numbers of order q = 0, 1, and 2) in limestone TDF can also arise from weaker limitations related to soil water content and fertility in this substrate. Limestone-derived soils have higher conductivity, Ca, and P concentrations, while phyllite-derived soils have a higher concentration of total N, nitrates, and ammonia (Sandoval-Granillo, 2020). Given the high proportion of legume species in TDFs and their frequent symbioses with nitrogen-fixing Rhizobium bacteria (Gei et al., 2018), nitrogen limitations are probably unimportant for these forests. Therefore, the higher diversity documented here for limestone might be, at least partially, related to higher soil water content but also to higher P availability, an element known to limit vegetation development on TDFs (Campo and Vázquez-Yanes, 2004) and other forest ecosystems (Hahm et al., 2014). Empirical observations from sites on the two substrates also suggest that limestone environments are rockier and highly heterogeneous, with many small and irregular patches of developed soils, shallow soils, and bare rock (Miguel-Talonia, unpublished data). Thus, limestone environments may provide a wider variety of microhabitats than phyllite, thus allowing more species to establish on this TDF community (Ribeiro et al., 2007; Díaz-Castellanos et al., 2022).
4.2. Structure and diversity dynamics were similar between substrates
Unexpectedly, the dynamic behavior of TDF attributes was very similar between the two substrates, not only in terms of direction (increases and decreases in value) but also regarding its variability (magnitude of changes). In other words, changes in forest attributes over time were parallel in both forest types (Supplementary Figure 1), with all attributes showing non-significant differences in variation (Figure 2H). The finding of similar dynamics between limestone and phyllite TDF plots was also supported by the analysis of demographic rates. Rates of net change, recruitment, growth, mortality, and residence time were similar between substrates for the four forest attributes analyzed from a demographic perspective (basal area, AGB, density, and 0D). These results coincide with our third predicted scenario for TDF dynamics, which anticipated equally stable dynamics between substrates due to the soil-effects mechanism acting with an intensity similar to the composition-effects mechanism. This is the most intriguing finding of this study, as it suggests that, at least for our studied landscape, forest composition adapts to local environmental conditions (composition-effects mechanism) while ecosystem functioning remains spatially homogeneous even in patches with harsher environmental conditions (soil-effects mechanism).
The conclusion that soil- and composition-effects offset each other should be treated with care, as it relies on the assumption that species on phyllite TDF have rather conservative traits. However, the strong differences in structure and diversity, together with the soil differences observed by Sandoval-Granillo (2020), point to clearly different environmental conditions between substrates, as discussed earlier. As soils derived from phyllite seem drier and less fertile, one may reasonably assume that species in this substrate are better adapted to harsher environmental conditions (Díaz-Castellanos et al., 2022). For instance, communities in which conservative traits are prevalent in a Brazilian TDF showed higher productivity and biomass stocks than their counterparts with more acquisitive traits (Prado-Junior et al., 2016), which provides support to our hypothesis. Further research on the functional differences between the species occurring on limestone and phyllite TDF is needed to clarify if and how substrates influence forest functional profiles.
The result that in this region limestone TDF achieves an overall higher vegetation development without significant differences in dynamics is somewhat puzzling. Higher values in TDF attributes should arise with differing residence times, allowing limestone forests to keep a unit of a given attribute for a longer period in order to build higher attribute stocks. It should be noted that mortality was higher (more negative) for the variables analyzed on the demographic analysis; however, due to large within-substrate variation, results from the substrate-wise mortality comparison were not significant. Previous studies have shown that variation in attributes and rates increases with smaller samples (Galbraith et al., 2013). In this study, we used a surface area of 500 m2 for the 14 permanent TDF plots. While this sampling area might be enough to obtain a reliable estimate for some TDF attributes, demographic rates may require larger samples (larger areas, longer study periods, and/or more sampling units) to be fully representative of the vegetation type they embody. Still, probably the differences in dynamics and demographic rates are small, and thus the noise-to-signal ratio does not allow to detect potential differences in dynamics between substrates. Therefore, for this site, it is essential to continue the monitoring for a longer period of time, as this would be the most feasible way of increasing the reliability of the estimations arising from this set of permanent plots.
4.3. Species composition differs between substrates, but compositional dynamics is similar
In line with the results for TDF attributes, the NMDS demonstrated that species composition differed between substrates, with the respective floristic sets showing a relatively minor overlap on the ordination space (Figure 4A). Since the NMDS ordination was based on species abundances, year-to-year plot trajectories not only reflect differences in species presence/absence but also is species abundances between plots and substrates. Geology has been shown to affect species composition and abundance in other TDF sites (Durán et al., 2006), probably by differentially affecting soil fertility, soil water content and environmental physical heterogeneity. Soil fertility, which is largely dependent on parent material (Scholes, 1990; Augusto et al., 2017), has been shown to positively affect species dominance in TDFs (Peña-Claros et al., 2012) and to influence species and functional composition in tropical forests in general (Fayolle et al., 2012; Terra et al., 2018). Moreover, increasing soil water availability over time increased species richness and functional diversity in TDFs (Raymundo et al., 2019), and these results can be also extrapolated to a spatial dimension. Hence, lithological substrates, which determine soil spatial variability in TDFs (Pulla et al., 2016), can influence forest floristics through various mechanisms; thus, the finding of large differences in species composition and abundance is not surprising in these contrasting environments.
Contrary to the notorious compositional differences between the two forest subtypes, the NMDS ordination also revealed virtually identical dynamics regarding floristics in limestone and phyllite TDFs. The similarity in forest dynamics held regardless of the metric used to assess floristic dynamics (i.e., annual mean distance, dispersion from plot centroid, or the distance from initial and final points). In fact, the latter metric suggests a nearly circular pattern in the trajectories of most plots in the ordination space, irrespective of substrate. This observation implies a highly stable floristic composition, as is usually expected in old-growth forests under stable climatic regimes (Muñoz et al., 2021). The lack of differences in forest dynamics, even on these substrates associated with clearly dissimilar abiotic environments, is difficult to explain. Unfortunately, predicting species composition and its changes over time is extremely difficult, as the process of species assemblage in communities depends on too many biotic and abiotic factors and is of course species-specific (e.g., propagule and microhabitat availability, priority effects, nutrient limitations). Using a functional trait approach would likely be useful to understand floristic changes, as functional ecology links plant traits to plant responses to environmental stimuli. By doing so, changes in species composition and abundance could be framed in the context of the environments that these forests experience.
5. Conclusion
Lithological substrates had a strong effect on TDF structure, diversity, and species composition, with the TDF on limestone showing better vegetation development and higher diversity than its counterpart on phyllite. Nevertheless, the temporal dynamics and demographic rates of these forest attributes did not differ between substrates, suggesting that the harsher environmental conditions associated with the phyllite substrate are buffered by means of a species composition that is better adapted to cope with those conditions. The net result of this balance seems to be a relatively spatially homogenous dynamics across this geologically complex TDF landscape, implying that future analyses of the responses of these forests to novel climatic conditions need not pay attention to substrate heterogeneity.
However, a word of caution is important with regard to this conclusion, as it is based on results obtained over a 12-year period for the climatic conditions experienced by our study forests during this time. Extreme events (intense or prolonged drought and heat waves) could push one of these TDF subtypes beyond its eco-physiological threshold, thus decoupling their dynamics. We hypothesize that this is more likely to be the case for the TDF on limestone, as its species assemblage is adapted to more mesic conditions (Romero et al., 2019, 2022), which could potentially cause massive diebacks once their climatic tipping points are surpassed (Brodribb et al., 2020; Powers et al., 2020). Ecologists need to continue making great efforts to keep and expand the long-term monitoring of these ecosystems. Perhaps in the future we will be able to remove the infamous tag of the “most threatened and vulnerable forest type in the world” from these fascinating tropical ecosystems (Hasnat and Hossain, 2020).
Data availability statement
The original contributions presented in the study are publicly available. These data can be found here: https://doi.org/10.5281/zenodo.7680666.
Author contributions
RM and JAM conceptualized the study and led manuscript writing. JAM and FB led permanent plot establishment and secured project continuity and funding. RM and ME conducted data analyses. RDL-M coordinated field campaigns (2015 onward). CM-T provided advice related to the geological aspects of the study. All authors gathered field data, discussed results, commented on the manuscript, and approved its submission.
Funding
Field data collection and permanent site continuity were funded by the Mexican Council of Science and Technology (CONACYT, grant CB-2009-01-128136), the National Autonomous University of Mexico (PAPIIT-UNAM, grants IN216007, IN218416, and IN217620), and the Dutch Research Council (NWO, grant ALWOP.457). The writing of this manuscript was funded by the Dutch Research Council (NWO, grant ALWOP.457).
Acknowledgments
We thank dozens of students and field assistants that have enabled data collection for over 14 years. Special thanks to E. A. Pérez-García for helping in taxonomic identification of species and M. A. Romero-Romero for technical support. G. Escamilla-Zerón, I. E. Romero-Pérez, and G. L. Cervantes-Jiménez critically helped in field data collection. We also thank the Reyes-Manuel and the López-Mendoza families, together with all the villagers of Nizanda, for their continuous support and friendship over the last three decades.
Conflict of interest
The authors declare that the research was conducted in the absence of any commercial or financial relationships that could be construed as a potential conflict of interest.
The handling editor declared a shared affiliation with the authors at the time of review.
Publisher’s note
All claims expressed in this article are solely those of the authors and do not necessarily represent those of their affiliated organizations, or those of the publisher, the editors and the reviewers. Any product that may be evaluated in this article, or claim that may be made by its manufacturer, is not guaranteed or endorsed by the publisher.
Supplementary material
The Supplementary Material for this article can be found online at: https://www.frontiersin.org/articles/10.3389/ffgc.2023.1082207/full#supplementary-material
References
Allen, K., Dupuy, J. M., Gei, M. G., Hulshof, C., Medvigy, D., Pizano, C., et al. (2017). Will seasonally dry tropical forests be sensitive or resistant to future changes in rainfall regimes? Environ. Res. Lett. 12:023001. doi: 10.1088/1748-9326/aa5968
Augusto, L., Achat, D. L., Jonard, M., Vidal, D., and Ringeval, B. (2017). Soil parent material—A major driver of plant nutrient limitations in terrestrial ecosystems. Glob. Chang. Biol. 23, 3808–3824. doi: 10.1111/gcb.13691
Brodribb, T. J., Powers, J., Cochard, H., and Choat, B. (2020). Hanging by a thread? Forests and drought. Science 368, 261–266. doi: 10.1126/science.aat7631
Callahan, R. P., Riebe, C. S., Sklar, L. S., Pasquet, S., Ferrier, K. L., Hahm, W. J., et al. (2022). Forest vulnerability to drought controlled by bedrock composition. Nat. Geosci. 15, 714–719.
Campo, J., and Vázquez-Yanes, C. (2004). Effects of nutrient limitation on aboveground carbon dynamics during tropical dry forest regeneration in Yucatán, Mexico. Ecosystems 7, 311–319.
Chao, A., Chiu, C.-H., and Jost, L. (2014). Unifying species diversity, phylogenetic diversity, functional diversity, and related similarity and differentiation measures through hill numbers. Ann. Rev. Ecol. Evol. Syst. 45, 297–324.
Chaturvedi, R., Raghubanshi, A., Tomlinson, K., and Singh, J. (2017). Impacts of human disturbance in tropical dry forests increase with soil moisture stress. J. Veg. Sci. 28, 997–1007.
Chave, J., Rejou-Mechain, M., Burquez, A., Chidumayo, E., Colgan, M. S., Delitti, W. B., et al. (2014). Improved allometric models to estimate the aboveground biomass of tropical trees. Glob. Chang. Biol. 20, 3177–3190. doi: 10.1111/gcb.12629
Condit, R., Aguilar, S., Hernandez, A., Perez, R., Lao, S., Angehr, G., et al. (2004). Tropical forest dynamics across a rainfall gradient and the impact of an El Niño dry season. J. Trop. Ecol. 20, 51–72. doi: 10.1017/S0266467403001081
Covelo, F., Sales, F., Silva, M., and Garcia, C. (2017). Vegetation on limestone versus phyllite soils: A case study in the west Iberian Peninsula. Flora Med. 27, 159–173.
Díaz-Castellanos, A., Meave, J. A., Vega-Ramos, F., Pineda-García, F., Bonfil, C., and Paz, H. (2022). The above–belowground functional space of tropical dry forest communities responds to local hydric habitats. Biotropica 54, 1003–1014.
Durán, E., Meave, J. A., Lott, E. J., and Segura, G. (2006). Structure and tree diversity patterns at the landscape level in a Mexican tropical deciduous forest. Bol. Soc. Bot. México 79, 43–60.
Fayolle, A., Engelbrecht, B., Freycon, V., Mortier, F., Swaine, M., Réjou-Méchain, M., et al. (2012). Geological substrates shape tree species and trait distributions in African moist forests. PLoS One 7:e42381. doi: 10.1371/journal.pone.0042381
Food and Agriculture Organization [FAO] (2020). Global forest resources assessment 2020 (FRA 2020). Rome: Food and Agriculture Organization of the United Nations.
Galbraith, D., Malhi, Y., Affum-Baffoe, K., Castanho, A. D., Doughty, C. E., Fisher, R. A., et al. (2013). Residence times of woody biomass in tropical forests. Plant Ecol. Divers. 6, 139–157.
Gei, M., Rozendaal, D. M. A., Poorter, L., Bongers, F., Sprent, J. I., Garner, M. D., et al. (2018). Legume abundance along successional and rainfall gradients in Neotropical forests. Nat. Ecol. Evol. 2, 1104–1111. doi: 10.1038/s41559-018-0559-6
Goldstein, H., and Healy, M. J. (1995). The graphical presentation of a collection of means. J. R. Stat. Soc. Ser. A Stat. Soc. 158, 175–177.
Grubb, P. J. (1996). “Rainforest dynamics: The need for new paradigms,” in Tropical rainforest research – current issues: proceedings of the conference held in Bandar Seri Begawan, April 1993, eds D. S. Edwards, W. E. Booth, and S. C. Choy (Heidelberg: Springer Netherlands), 215–233.
Hahm, W. J., Riebe, C. S., Lukens, C. E., and Araki, S. (2014). Bedrock composition regulates mountain ecosystems and landscape evolution. Proc. Natl. Acad. Sci. U.S.A. 111, 3338–3343. doi: 10.1073/pnas.1315667111
Hasnat, G. T., and Hossain, M. K. (2020). “Global overview of tropical dry forests,” in Handbook of research on the conservation and restoration of tropical dry forests, eds R. Bhadouria, S. Tripathi, P. Srivastava, and P. Singh (Hershey, PA: IGI Global), 1–23.
Laurance, S. G. W., Laurance, W. F., Nascimento, H. E. M., Andrade, A., Fearnside, P. M., Rebello, E. R. G., et al. (2009). Long-term variation in Amazon forest dynamics. J. Veg. Sci. 20, 323–333. doi: 10.1111/j.1654-1103.2009.01044.x
Lewis, S. L., Phillips, O. L., Baker, T. R., Lloyd, J., Malhi, Y., Almeida, S., et al. (2004). Concerted changes in tropical forest structure and dynamics: Evidence from 50 South American long-term plots. Philos. Trans. R. Soc. Lond. B Biol. Sci. 359, 421–436. doi: 10.1098/rstb.2003.1431
McDowell, N. G., Allen, C. D., Anderson-Teixeira, K., Aukema, B. H., Bond-Lamberty, B., Chini, L., et al. (2020). Pervasive shifts in forest dynamics in a changing world. Science 368:eaaz9463. doi: 10.1126/science.aaz9463
Miles, L., Newton, A. C., DeFries, R. S., Ravilious, C., May, I., Blyth, S., et al. (2006). A global overview of the conservation status of tropical dry forests. J. Biogeogr. 33, 491–505.
Mooney, H., Bullock, S., and Medina, E. (1995). “Introduction: Seasonally dry tropical forests,” in Seasonally dry tropical forests, eds S. H. Bullock and H. A. Mooney (Cambridge: Cambridge University), 1–8.
Muñoz, R. (2015). Dinámica de la estructura y la diversidad de un bosque tropical caducifolio del Istmo de Tehuantepec, Oaxaca, México. B.Sc. thesis. Mexico City: Universidad Nacional Autónoma de México.
Muñoz, R., Bongers, F., Rozendaal, D. M., González, E. J., Dupuy, J. M., and Meave, J. A. (2021). Autogenic regulation and resilience in tropical dry forest. J. Ecol. 109, 3295–3307.
Muñoz, R., Enríquez, M., Bongers, F., López-Mendoza, R. D., Miguel-Talonia, C., and Meave, J. A. (2023). Data from “Lithological substrates influence tropical dry forest structure, diversity, and composition, but not its dynamics”. Zenodo. doi: 10.5281/zenodo.7680666
Murphy, P. G., and Lugo, A. E. (1986). Ecology of tropical dry forest. Ann. Rev. Ecol. Syst. 17, 67–88.
National Oceanic and Atmospheric Administration [NOAA], (2022). Historical El Nino/La Nina episodes (1950-present). Available online at: https://origin.cpc.ncep.noaa.gov/products/analysis_monitoring/ensostuff/ONI_v5.php (accessed January 30, 2023).
Oksanen, J. (2007). Vegan: Community ecology package. R package version 1.8-5. Available online at: http://www.cran.r-project.org (accessed November, 2020).
Oliveira, R. S., Eller, C. B., Barros, F. V., Hirota, M., Brum, M., and Bittencourt, P. (2021). Linking plant hydraulics and the fast–slow continuum to understand resilience to drought in tropical ecosystems. New Phytol. 230, 904–923. doi: 10.1111/nph.17266
Peña-Claros, M., Poorter, L., Alarcón, A., Blate, G., Choque, U., Fredericksen, T. S., et al. (2012). Soil effects on forest structure and diversity in a moist and a dry tropical forest. Biotropica 44, 276–283.
Pérez-García, E. A., Meave, J. A., Villaseñor, J. L., Gallardo-Cruz, J. A., and Lebrija-Trejos, E. E. (2010). Vegetation heterogeneity and life-strategy diversity in the flora of the heterogeneous landscape of Nizanda, Oaxaca, Mexico. Folia Geobot. 45, 143–161. doi: 10.1007/s12224-010-9064-7
Pérez-Gutiérrez, R., Solari, L. A., Gómez-Tuena, A., and Valencia, V. A. (2009). El terreno Cuicateco: ¿cuenca oceánica con influencia de subducción del Cretácico Superior en el sur de México? Nuevos datos estructurales, geoquímicos y geocronológicos. Rev. Mex. Cienc. Geol. 26, 222–242.
Powers, J. S., Vargas, G. G., Brodribb, T. J., Schwartz, N. B., Pérez-Aviles, D., Smith-Martin, C. M., et al. (2020). A catastrophic tropical drought kills hydraulically vulnerable tree species. Glob. Chang. Biol. 26, 3122–3133. doi: 10.1111/gcb.15037
Prado-Junior, J. A., Schiavini, I., Vale, V. S., Arantes, C. S., van der Sande, M. T., Lohbeck, M., et al. (2016). Conservative species drive biomass productivity in tropical dry forests. J. Ecol. 104, 817–827.
Pulla, S., Riotte, J., Suresh, H. S., Dattaraja, H. S., and Sukumar, R. (2016). Controls of soil spatial variability in a dry tropical forest. PLoS One 11:e0153212. doi: 10.1371/journal.pone.0153212
Raymundo, D., Prado-Junior, J., Alvim Carvalho, F., Santiago do Vale, V., Oliveira, P. E., and van der Sande, M. T. (2019). Shifting species and functional diversity due to abrupt changes in water availability in tropical dry forests. J. Ecol. 107, 253–264.
Reich, P. B. (2014). The world-wide ‘fast–slow’plant economics spectrum: A traits manifesto. J. Ecol. 102, 275–301.
Ribeiro, K. T., Medina, B. M. O., and Scarano, F. R. (2007). Species composition and biogeographic relations of the rock outcrop flora on the high plateau of Itatiaia, SE-Brazil. Braz. J. Bot. 30, 623–639.
Romero, E., Dávalos-Sotelo, R., Meave, J. A., and Terrazas, T. (2019). Wood density, deposits and mineral inclusions of successional tropical dry forest species. Eur. J. For. Res. 139, 369–381. doi: 10.1007/s10342-019-01236-9
Romero, E., Terrazas, T., González, E. J., and Meave, J. A. (2022). Wood anatomy of 13 species from a successional tropical dry forest: Description and ecological implications. IAWA J. 43, 1–31.
Sánchez-Azofeifa, G. A., Quesada, M., Rodríguez, J. P., Nassar, J. M., Stoner, K. E., Castillo, A., et al. (2005). Research priorities for neotropical dry forests. Biotropica 37, 477–485. doi: 10.1046/j.0950-091x.2001.00153.x-i1
Sandoval-Granillo, V. (2020). Perfiles funcionales foliares y filtrado. ambiental en un bosque tropical caducifolio: Comparación entre dos sustratos. B.Sc. thesis. Mexico City: Universidad Nacional Autónoma de México.
Scholes, R. J. (1990). The influence of soil fertility on the ecology of southern African dry savannas. J. Biogeogr. 17, 415–419. doi: 10.2307/2845371
Scholz, A., Stein, A., Choat, B., and Jansen, S. (2014). How drought and deciduousness shape xylem plasticity in three Costa Rican woody plant species. IAWA J. 35, 337–355.
Searcy, K. B., Wilson, B. F., and Fownes, J. H. (2003). Influence of bedrock and aspect on soils and plant distribution in the Holyoke Range, Massachusetts. J. Torrey Bot. Soc. 130, 158–169.
Sheil, D., Jennings, S., and Savill, P. (2000). Long-term permanent plot observations of vegetation dynamics in Budongo, a Ugandan rain forest. J. Trop. Ecol. 16, 865–882.
Siyum, Z. G. (2020). Tropical dry forest dynamics in the context of climate change: Syntheses of drivers, gaps, and management perspectives. Ecol. Process. 9:25. doi: 10.1186/s13717-020-00229-6
ter Steege, H., Pitman, N. C. A., Phillips, O. L., Chave, J., Sabatier, D., Duque, A., et al. (2006). Continental-scale patterns of canopy tree composition and function across Amazonia. Nature 443, 444–447. doi: 10.1038/nature05134
Terra, M. C. N. S., Santos, R. M., Prado Júnior, J. A., de Mello, J. M., Scolforo, J. R. S., Fontes, M. A. L., et al. (2018). Water availability drives gradients of tree diversity, structure and functional traits in the Atlantic–Cerrado–Caatinga transition, Brazil. J. Plant Ecol. 11, 803–814. doi: 10.1093/jpe/rty017
van Oldenborgh, G. J., Hendon, H., Stockdale, T., L’Heureux, M., De Perez, E. C., Singh, R., et al. (2021). Defining El Niño indices in a warming climate. Environ. Res. Lett. 16:044003.
Keywords: lithology, geology, tropical dry forest, structure, diversity, species composition, soil, vegetation dynamics
Citation: Muñoz R, Enríquez M, Bongers F, López-Mendoza RD, Miguel-Talonia C and Meave JA (2023) Lithological substrates influence tropical dry forest structure, diversity, and composition, but not its dynamics. Front. For. Glob. Change 6:1082207. doi: 10.3389/ffgc.2023.1082207
Received: 27 October 2022; Accepted: 13 February 2023;
Published: 15 March 2023.
Edited by:
David A. Prieto-Torres, Faculty of Higher Education Iztacala, National Autonomous University of Mexico, MexicoReviewed by:
Bradley Christoffersen, The University of Texas Rio Grande Valley, United StatesRavi Kant Chaturvedi, Xishuangbanna Tropical Botanical Garden (CAS), China
Copyright © 2023 Muñoz, Enríquez, Bongers, López-Mendoza, Miguel-Talonia and Meave. This is an open-access article distributed under the terms of the Creative Commons Attribution License (CC BY). The use, distribution or reproduction in other forums is permitted, provided the original author(s) and the copyright owner(s) are credited and that the original publication in this journal is cited, in accordance with accepted academic practice. No use, distribution or reproduction is permitted which does not comply with these terms.
*Correspondence: Rodrigo Muñoz, rodrigo.munozaviles@wur.nl; Jorge A. Meave, jorge.meave@ciencias.unam.mx