- 1Center for Systems Integration and Sustainability, Department of Fisheries and Wildlife, Michigan State University, East Lansing, MI, United States
- 2Center for Environmental Studies and Research, State University of Campinas, Campinas, Brazil
- 3Center for Global Change and Earth Observations, Michigan State University, East Lansing, MI, United States
- 4Department of Geography, University of North Carolina, Chapel Hill, NC, United States
- 5Department of Geography, King’s College London, London, United Kingdom
- 6Department of Natural Resources, Faculty of Geo-Information Science and Earth Observation (ITC), University of Twente, Enschede, Netherlands
- 7Department of Community Sustainability, Michigan State University, East Lansing, MI, United States
The world has entered the United Nations Decade on Ecosystem Restoration (2021–2030), yet many regions of the world still face environmental degradation. In this context a question arises: under what conditions may a given region shift from a trajectory of environmental degradation to environmental recovery? Answering this question constitutes an important endeavor for the scientific community, policymakers, and organizations leading the planning and implementation of restoration projects. This study examines some of the human-environment conditions underpinning the net gains in natural forest cover in a region that has experienced environmental degradation: the Atlantic Forest biome, Brazil. Using land-use/cover (LULC) data, we assessed the loss and successive gain in forest cover during the 1987–2001 and 2001–2015 periods. Municipality-level statistics on agriculture and economy, together with LULC and biophysical data, were used to develop models for assessing forest cover trajectories. Of the 1,972 municipalities experiencing net forest loss during the 1987–2001 period, 59% shifted their trajectory to a net gain during the 2001–2015 period. This shift, known as forest transition, followed a complex socio-economic pathway characterized by (i) the stagnation of traditional agricultural activities favoring the replacement of pasturelands by mechanized agriculture, and (ii) the emergence of non-agricultural rural activities together with multifunctional landscapes. Furthermore, a trend of decrease in precipitation seems to be associated with land abandonment, thus, favoring the return of natural vegetation. Our findings suggest that forest transition at the biome level is possible if environmental and land regulations take advantage of specific context-dependent situations that foster net forest gains.
1. Introduction
The loss of forests around the globe constitutes a major consequence of human agency during the last century (Curtis et al., 2018), which has important implications for conservation and sustainability worldwide (Ouyang et al., 2001; Burgess et al., 2002; Miranda et al., 2017; Liu, 2023). However, while the resilience and related regenerative capacity of forests after human disturbance are still debated (Safar et al., 2020; Poorter et al., 2021), many studies have demonstrated the capacity of natural forests to recover (Silva et al., 2016; Crouzeilles et al., 2017; Calaboni et al., 2018). The phenomenon of net forest cover gain after a previous period of net forest loss is known as “forest transition” (Mather, 1992; Meyfroidt and Lambin, 2011; Liu et al., 2018a). Generally, forest transition theory predicts two different pathways a region can go through after a deforestation period toward forest cover regrowth: the forest scarcity pathway and the economic pathway (Rudel et al., 2005). The former theorizes that deforestation provokes forest-based product shortages fostering the need for reforestation to renew forest supplies, while the latter theorizes that industrialization and economic development push rural out-migration leaving empty rural areas (land abandonment) that will follow natural forest regeneration. Forest transition is, therefore, particularly important given that the world is currently in the United Nations’ “Decade on Ecosystem Restoration (2021–2030),” a key global initiative for the sustainability of human-environment systems (IPCC, 2021). However, a large amount of financial investment is needed to implement ecosystem restoration (Crouzeilles et al., 2020; Garibaldi et al., 2020; Pinto and Voivodic, 2021). Hence, knowledge of natural regeneration capacity of forest ecosystems, together with underpinning human dimensions, are key for leveraging future plans and actions favoring ecosystem restoration (Yang et al., 2013; Chazdon and Uriarte, 2016).
Focusing on the Brazilian Atlantic Forest biome, here we consider the forest transition phenomenon as an indicator of tropical forest resilience—i.e., the system’s capacity to recover after disturbance (Nave et al., 2016; Chazdon et al., 2020; Poorter et al., 2021). Previous studies in this biome (Baptista, 2008; Farinaci and Batiatella, 2012; Silva et al., 2016; Calaboni et al., 2018) have demonstrated the occurrence of a forest transition at different scales (e.g., state, region, watershed), and as a response to land abandonment of former agricultural areas due to changes in socioeconomic conditions. Therefore, forest transition constitutes a reliable land change process that can be used to assess the regenerative capacity of forests in the biome.
This study aims to understand the conditions under which a human-environment system, characterized by a net loss of natural forest cover (NFC) shifts its trajectory to one that favors a net gain of NFC. To this effect, we analyzed a set of socioeconomic and environmental factors to identify the conditions under which either a net NFC loss or gain occurs over an observed time period. The Atlantic Forest is the most threatened biome in Brazil [e.g., over 80% of natural vegetation has been replaced by human land uses (Rezende et al., 2018)], despite its importance as a key hotspot of tropical biodiversity, and home to 70% of the Brazilian human population (Marques and Grelle, 2021). Using a set of thematic land-use and land-cover (LULC) maps based on Landsat satellite image time series, we assessed deforestation and NFC net gain at the municipality level between 1987 and 2015. These results, together with national statistics on agriculture and economy, and climate data, were used to evaluate forest change processes in the biome. Considering the complexity and the multiple pathways associated with forest transition, our spatiotemporal modeling approach deals with major factors associated with NFC change, suitable for developing knowledge for future management actions toward the recovery of one of the most endangered hotspots of biodiversity on Earth.
2. Materials and methods
This study was conducted through a series of spatial econometric models and statistical analyses applied to a multi-source dataset of variables consisting of a time series of thematic land-use and land-cover (LULC) maps, socioeconomic and agricultural statistics, and precipitation data. These datasets were aggregated to the municipality level (i.e., unit of analysis of our modeling approach). In Figure 1 we present the major methodological steps conducted.
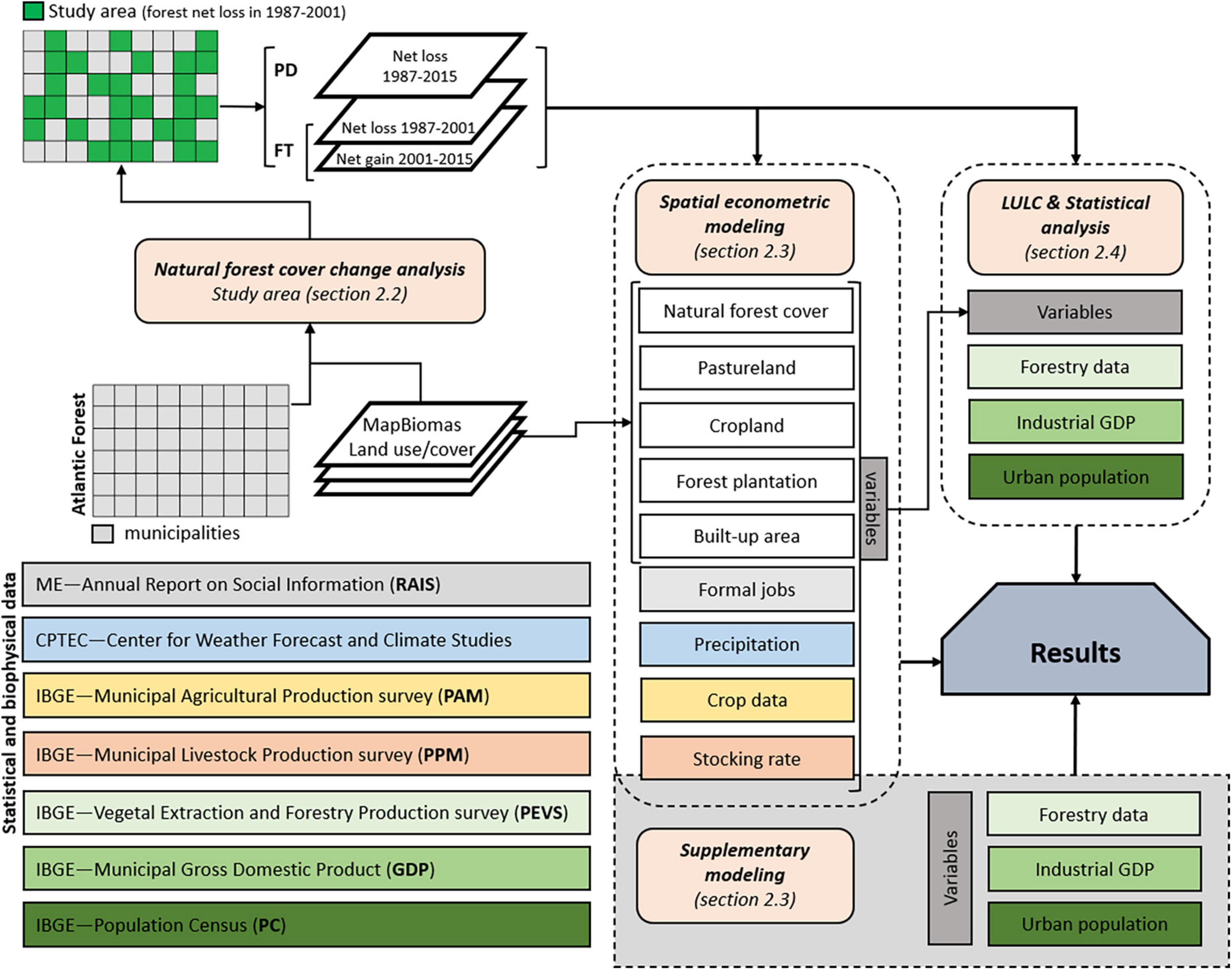
Figure 1. Methodological steps conducted in the study (municipality groups: PD—persistent deforestation, FT—forest transition).
2.1. Data sources
2.1.1. Land-use and land-cover data
Obtained from MapBiomas1 level 2 products at a 30 m pixel resolution, the LULC datasets from 1987 to 2015 derived from v.5.0 have an accuracy of 86.5% for the Atlantic Forest biome. This constitutes the highest accuracy among all MapBiomas collections for this biome (including the most recent v.7). Thus, we chose MapBiomas v.5.0 to be used in this study. The original level 2 classes of “pasture,” “forest plantation,” “urban infrastructure,” “water,” and “natural forest” were adopted. The level 2 classes of “agriculture” and “mosaic of agriculture and pasture” were merged into a single “cropland” class. In addition, we named the “urban infrastructure” class as “built-up area,” and “pasture” as “pastureland.” We also grouped other non-vegetation and anthropic classes into “other” (they represent only 1% of the study area). In Supplementary material we provide a brief description of the classes (SM—LULC data). The net change in natural forest cover (NFCNC) was calculated using the ‘natural forest’ class.
2.1.2. Statistical survey and biophysical data
From the agricultural statistics of the Brazilian Institute of Geography and Statistics (IBGE, 2012), we used a time series from 1987 to 2015 of the Municipal Agricultural Production survey—PAM (IBGE Table 5457) to derive data on agricultural production (i.e., crop diversity, and yield). The cattle statistics (e.g., number of animals) are available at the IBGE, Municipal Livestock Production survey—PPM (IBGE Table 3939). Forestry production data were provided by IBGE through the Vegetal Extraction and Forestry Production survey—PEVS (IBGE Table 291). The Socioeconomic statistics on formal jobs were obtained from the Annual Report on Social Information—RAIS of the Ministry of Economy—ME. Raster data at the 1 km/pixel resolution of the average annual precipitation were obtained from the Center for Weather Forecast and Climate Studies (2021). We also used the Municipal Gross Domestic Product—GDP (IBGE Tables 5938 and 21) of the industry sector, and urban population data from the Population Census—PC (IBGE Table 200). From the TOPODATA geomorphometric information system of Brazil, we downloaded a digital elevation model (DEM) at 30 m pixel resolution derived from Shuttle Radar Topographic Mission (SRTM) data (Valeriano, 2008). For more details on these variables see Supplementary material (SM—Dataset).
2.2. Study area (forest transition and persistent deforestation groups)
The last 15 years of the 20th century were characterized by major political changes in Brazil, starting, in 1984 with the end of the Brazilian dictatorship, followed by the public participation of broad segments of society in social and environmental affairs (Seino et al., 2013). This political context (termed re-democratization) led to the promulgation of the new National Constitution of 1988, which established the obligation of the state to secure a healthy environment for all Brazilian citizens, and included the protection of all Brazilian biomes (Article 225). In 1990, the social organization SOS Mata Atlântica in partnership with the National Institute for Space Research (INPE), published the first report on the conservation status of the Atlantic Forest biome. Results presented in this report not only alarmed but also motivated public authorities and the public at large to address the environmental degradation of this biome, which culminated in a series of national decrees over the 1990s to cope with deforestation and to stimulate the environmental recovery of the biome (Silva et al., 2017a). These decrees were later formalized into the Atlantic Forest Law of 2006 (Law n° 11428/2006). This period was fundamental to precede regeneration trends in the Atlantic Forest as these sociopolitical changes slowed-down deforestation at significant levels (Silva et al., 2017a; SOS Mata Atlântica, and INPE, 2022). During the first two decades of the 21st century, Brazil engaged in the international environmental governance agenda (Viola and Gonçalves, 2019), which included the implementation of sustainable environmental certification systems (Silva et al., 2019), switching from being a food importer to becoming a major global food supplier (Schierhorn et al., 2016), and making significant changes in the agricultural sector toward highly intensified production systems (Buainain et al., 2014). This period was also marked by the emergence of urban centers with high labor demands driving rural out-migration (Alves and Marra, 2009). All these factors fostered changes in land and social systems with impacts on the environment. The Atlas da Mata Atlântica, a deforestation monitoring system (SOS Mata Atlântica, and INPE, 2022) shows that 2001 had the largest drop in deforestation rates in the Atlantic Forest (since 1985), indicating a turning point for LULC trajectories in the biome. In this context, and considering historical deforestation trends throughout the Atlantic Forest biome (Silva et al., 2020b) and its regional forest cover dynamics (Baptista, 2008; Farinaci and Batiatella, 2012; Silva et al., 2016; Calaboni et al., 2018), we verified and analyzed forest transition trends at the municipality level (3,083 municipalities in total). Given the above-described sociopolitical context and based on this empirical evidence of changes in deforestation trends, we set the 2001 as the theoretical “inflection point” [i.e., year of the change from net forest loss to net gain (Mather, 1992; Wilson et al., 2017)] in the forest transition pathway.
To define the set of municipalities undergoing forest transition, we analyzed the NFC dynamics during the previous 15 years (1987–2001) in all municipalities of the Atlantic Forest biome (3,082). We then selected only the municipalities with observed deforestation (i.e., net loss) during the first period 1987–2001 as our units of analysis (Figure 2A)—1,972 (63%). We then split these municipalities into two groups according to the observed NFC trajectory through the second period 2001–2015: (1) forest transition (hereafter FT, n = 1,180) and (2) persistent deforestation (hereafter PD, n = 792). To define both processes (i.e., NFC net loss and net gain) at the municipality level, we evaluated NFC net change (NFCNC) by computing the difference (in hectares) in NFC during the 1987–2001 and 2001–2015 period [NFCNC = NFCfinal–NFCinitial]. Positive (negative) values indicate an NFC net gain (loss) in the municipality. To allow for a more robust characterization of the NFC trajectory and verify that net gains and losses followed a steady trend in each period, and to verify that the ‘inflection point’ truly defined a transition, we accounted aggregated NFC (ha) annually for both groups (FT and PD), between 1987 and 2015. Hence, our data highlights a clear pattern of forest transition with an ‘inflection point’ in 2001 for the FT group, and also a persistent deforestation trend in the PD group (Figure 2B). These results justify the selection of the spatial (i.e., municipality) and temporal (i.e., 1987–2001, 2001–2015 time periods) units of analysis utilized in the study. We recognize that different successional stages of the NFC may have different ecological values, with early stages having the lowest value (Poorter et al., 2021). However, in this study we focused on all successional stages of the NFC. This was important because according to the Atlantic Forest Law, NFC patches at any successional stage (from pioneer to old-growth forests) are protected by law and no deforestation actions are permitted. This legal condition has been demonstrated to be a pivotal factor in supporting the empirical evidence of the forest transition in this biome (Silva et al., 2017a).
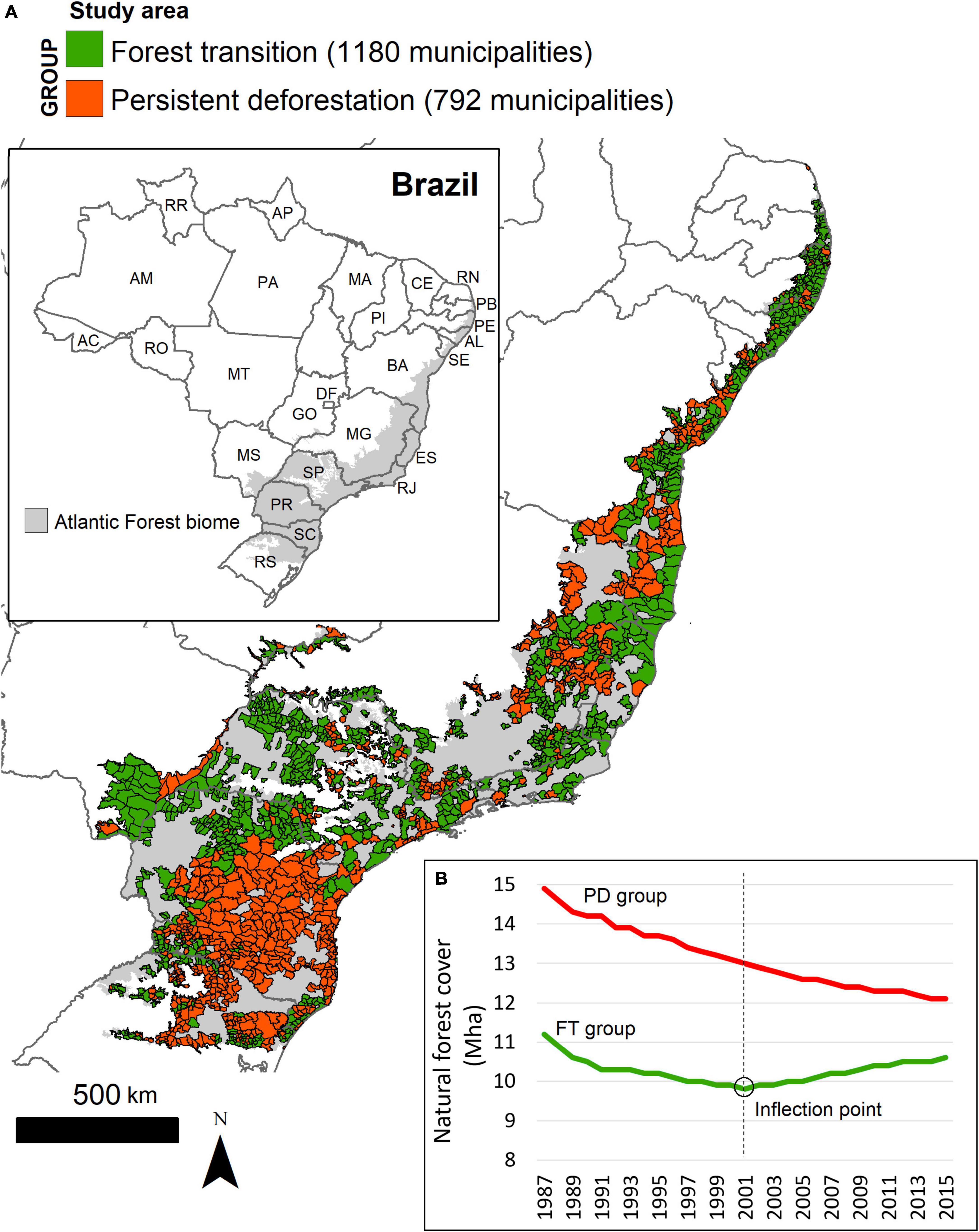
Figure 2. (A) Map with the location of the Atlantic Forest biome within the Brazilian territory, and the municipalities within study area divided in two groups (forest transition—FT, and persistent deforestation—PD). (B) Line charts show the temporal trajectory of natural forest cover for the FT and PD groups, annually, from 1987 to 2015, with the “inflection point” in the FT group in 2001.
2.3. Spatial econometric models
The spatial econometric models incorporate underlying driving forces (e.g., economic dynamics that underpin human actions) and proximate causes (e.g., immediate human actions such as agricultural expansion) to understand the phenomenon under investigation (Geist and Lambin, 2002). Thus, they constitute representations of complex human-environment systems (Liu et al., 2007). Variables representing land uses (pastureland, cropland, forest plantation, natural forest, and built-up area) were treated as proximate factors, while biophysical (i.e., precipitation) and socioeconomic (i.e., cattle stocking rate, crop yield, and formal jobs) variables were treated as underlying factors.
For the modeling of the spatiotemporal relationships among forest cover dynamics and independent variables, our study adopted the spatial error model (SARerr), which incorporates autoregressive processes in the error term to account for spatial trends (Gupta and Mackenzie, 2016). The SARerr model is described as:
Where β is the own-region vector of parameters (regression coefficients), y is the vector of observations of the dependent variable, ε is the disturbance vector of random errors from the regression model, λ is the autoregressive coefficient of the error terms, u is a vector of independent and identically distributed error terms, N represents the set of neighbors, X is the matrix of observations of independent variables, W is the standardized spatial weights matrix, and I is the identity matrix (Saputro et al., 2019).
Our modeling approach applied a first-order contiguity-based spatial weights matrix (“Queen” contiguity type) to account for spatial autocorrelation among municipalities (Chen et al., 2017). For all cases we applied diagnostic tests for spatial autocorrelation by the Robust Lagrange Multiplier, and Global Moran’s I test (Anselin, 1995). To avoid multicollinearity among independent variables, we set the variance inflation factor (VIF) value of 5 as the limit of acceptance (Garrett et al., 2018). Each model (three in total) used the same set of independent variables against the dependent variable (i.e., NFC net gain, NFC net loss), with corresponding variable values for each respective period of analysis. Hence, we obtained three models, one single model for the group of municipalities under NFC net loss from 1987 to 2015 (PD group—i.e., 29 years of change) and two models (one for the 1987–2001 period and another for the 2001–2015 period—i.e., 15 years of change each period) for the group of municipalities that changed from NFC net loss in 1987–2001 to net gain in 2001–2015 (FT group).
The selection of independent variables was based on previous studies on tropical forest cover change (Geist and Lambin, 2002; Silva et al., 2016; Crouzeilles et al., 2020; Espírito-Santo et al., 2020; Saraiva et al., 2020; García et al., 2021; Rosa et al., 2021). Among the independent variables, we have a set representing changes through the 15-years of each period (for the FT group), represented by
where EV is the independent variable value. For municipalities in the PD group, the period was represented by (Δt29), from 1987 to 2015. The variables represented by changes in the FT group (Δt15) [or (Δt29) in the PD group] are “pastureland,” “cropland,” “forest plantation,” “built-up area” (both measured in ha), “non-agricultural jobs” and “agricultural jobs” (both in number of people), crop yield (kg/ha), and “cattle stocking rate” (number of animals per hectare of pastureland). The initial NFC (ha) for each period was also used as an independent variable in all three models.
The variables “crop diversity” and “annual precipitation” were introduced in the models with the respective mean value over each period of analysis. From the information on planted area (PAM data) of temporary and permanent crops we calculated the crop diversity index [i.e., Shannon Diversity Index (Aguilar et al., 2015; Silva et al., 2020a)]. Crop yield (PAM data) was represented by the sum of the annual yields of major annual crops (SM—Crop data). In our models, “slope” and “elevation” were used as time-invariant variables (i.e., held constant across the study). Topography is known to be an important factor associated to natural vegetation cover dynamics in Atlantic Forest and then a key controlling factor (Silva et al., 2020b). From the DEM, we calculated the mean ‘slope’ and ‘elevation’ of every municipality in both groups. For instance, NFC regeneration has been observed in hilly terrain due to lower agricultural suitability (Piffer et al., 2021), while lower elevations are associated with greater natural vegetation production (Scaranello et al., 2012; Martins et al., 2015). Water availability is key for tillage agriculture and animal husbandry, thus, we used precipitation as an indicator of this availability (Espírito-Santo et al., 2020) and its impacts on NFC dynamics. Land use has direct effects on NFC, with major changes in LULC classes impacting the net changes in NFC (Silva et al., 2017b). The forest transition phenomenon has been noted in different parts of the world and has been associated with different socioeconomic factors (Lambin and Meyfroidt, 2010). Hence, agricultural (e.g., crop yield), demographic (e.g., population density), and economic indicators (e.g., jobs) may exhibit significant associations with the forest transition phenomenon. Given the unavailability of a suitable temporal time series data for industrial GDP, forestry (PEVS), and urban population (PC), we developed a supplementary spatial model for testing NFC net gains (forest transition model for the FT group in the period 2001–2015) with additional socioeconomic variables (SM—Supplementary model). Hence, the Supplementary model uses the same set of variables presented in the original FT model 2001–2015, but with the addition of the variables “industrial GDP,” “Eucalyptus cellulose pulp production,” “Pinus cellulose pulp production,” and “Population density.” The temporal approach incorporated into the econometric model is suitable for our study once NFC dynamics are linked with different social and economic processes at different temporal scales (Perz and Skole, 2003; Baptista, 2008; Lambin and Meyfroidt, 2010; Espírito-Santo et al., 2020). Hence, changes, such as NFC net gains (i.e., primarily driven by natural regeneration) over 15-year periods, may be addressed through this land change modeling framework (Baptista, 2008; Silva et al., 2017a; Crouzeilles et al., 2020; Piffer et al., 2021; Simiski et al., 2021).
2.4. Land-use and land-cover change and statistical analysis
Based on the same set of variables used in the spatial econometric models, we evaluated LULC and developed descriptive and inferential statistical analyses. This methodological step supports variable selection to the models and helps elucidating potential associations among independent variables, thus better interpretation of the results obtained from the models. While the spatial econometric models provide the significance of each independent variable on the NFC dynamics under a set of factors (Carlson et al., 2018), information obtained from statistical tests, and descriptive statistics increase the understanding of the dynamics of each specific variable, as well as the correlations among them. Hence, we performed t-tests to assess significant changes in precipitation levels throughout the study period. We also applied Spearman “rho” correlation tests (if Shapiro–Wilk tests rejected normal distribution at p < 0.05; Silva et al., 2020b) between variables, to test their associations at the municipality level (See SM for detailed results). For this methodological step we analyzed data from PEVS (i.e., timber and cellulose pulp production, and types of tree species), GDP and PC to develop descriptive statistics.
3. Results
3.1. Human-environment interactions and natural forest cover outcomes
Based on municipality level data, our models show that the same set of variables (i.e., initial forest cover, pastureland, cropland, and forest plantation) were positively correlated with forest net loss for both the FT group (deforestation 1987–2001) and the PD group (deforestation 1987–2015; Figure 3). These results indicate that deforestation trends in the municipalities investigated in the Atlantic Forest shared similar contexts associated with this process—i.e., an increase in the given independent variables is associated with an increase in NFC net loss (positive association). More interesting is that some variables not associated with NFC net loss in the models (i.e., crop diversity), or positively correlated with that process are now positively and significantly associated with NFC net gain in 2001–2015 (i.e., forest plantation, built-up area, and initial forest cover). This result, revealing variables that were associated with deforestation and now with forest cover increase, indicates a change effect of these variables on NFCNC trajectory (Figure 3). Our results indicate that higher precipitation levels were positively associated with NFC net loss in the FT group, and an expansion of livestock and cropland activities over natural forests in areas with higher agricultural suitability in the 1987–2001 period. However, precipitation was negatively and significantly associated with NFC net gain (Figure 3), revealing that lower precipitation levels during 2001–2015 were associated with the return of natural vegetation.
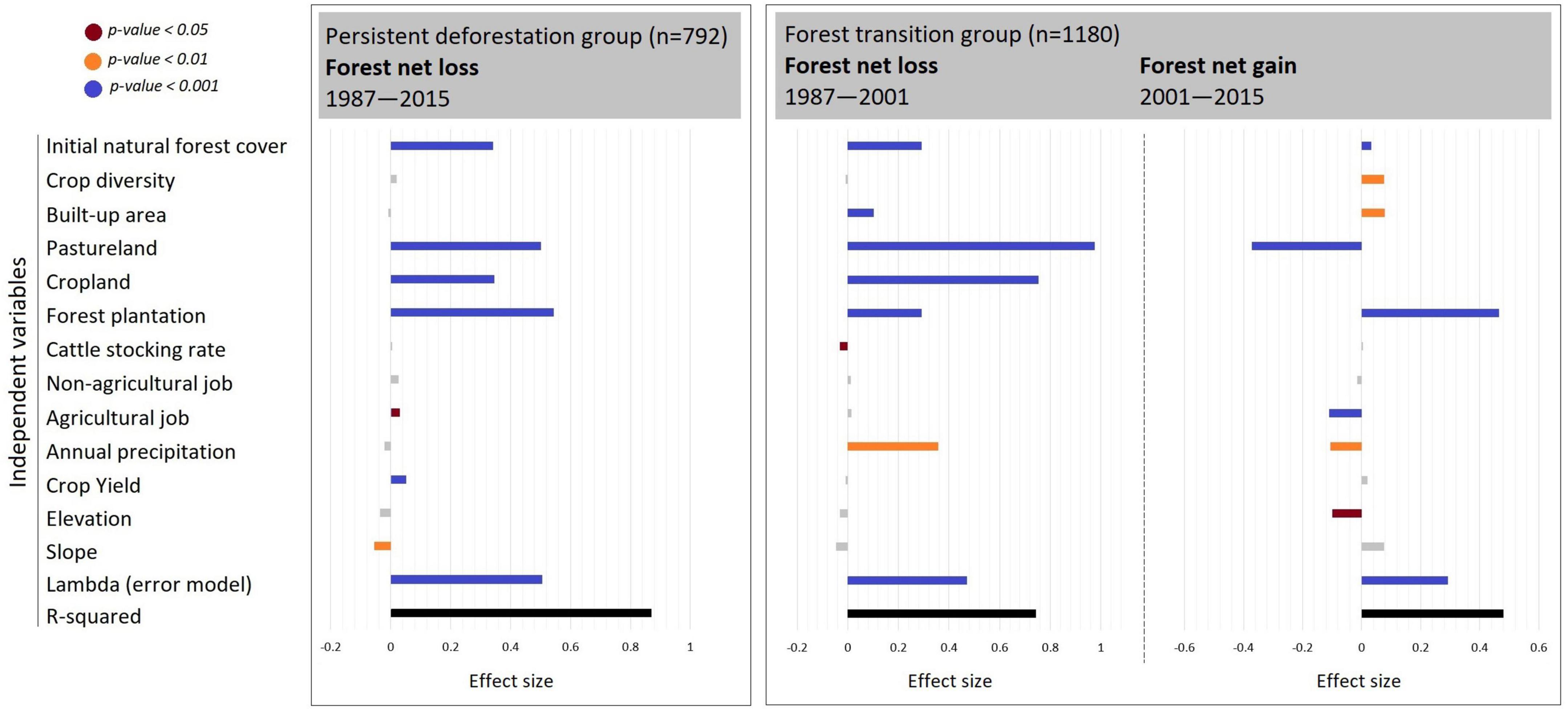
Figure 3. Spatial Error model results. To access the coefficient effects of each variable in the models, see SI—Model results.
These results suggest that among the municipalities experiencing a forest transition, those with a higher crop diversity and with lower levels of average annual precipitation were more likely to exhibit higher net gains in NFC. In the FT group models, the pastureland variable has a similar relationship as that found for precipitation. Hence, while in 1987–2001 the increase of grazing areas led to increases in NFC net loss (deforestation effects), in 2001–2015 an opposite trend was observed where decreases in pastureland led to increases in NFC net gain.
In the FT group we found that agricultural jobs negatively correlated with NFC net gain, indicating that a decrease in the number of rural employees at the municipality level was associated with net increases in NFC. It is interesting to note that the same independent variable was positively correlated with NFC net loss in the PD group (Figure 3), where agricultural activities expanded during the study period. This result reveals that in municipalities under persistent deforestation trends, increases in the number of rural employees was associated with higher NFC net losses. A similar association was found for the crop yield in the PD group. Interestingly, in our Supplementary model two additional variables were found to be positively and significantly correlated with NFC net gain (FT group): industrial GDP growth and cellulose pulp from eucalyptus (SM—Supplementary model).
To avoid multicollinearity, the variable cropland was not used in the NFC net gain model (Figure 3) given its high correlation with pastureland. For all other variables, the VIF values were lower than 5 in all three models. In addition, both the LM and Moran’s I tests were significant (p < 0.01), indicating the need for the use of SARerr (error model) models in our study.
3.2. Land-use and land-cover dynamics
During the 1987–2001 period, a total of 3.3 Mha (1.4 Mha in the FT, and 1.9 Mha in the PD group) of NFC were lost. However, in the following period (2001–2015), the FT group (i.e., 1,180 municipalities) had an NFC increase of ca. 8%, or ca. 800,000 ha, while in the PD group (i.e., 792 municipalities) ca. 905,000 ha experienced NFC loss (Figure 4A). The LULC changes revealed that over the entire period (1987–2015), forest plantations, as well as built-up areas and cropland, increased in both groups (Figure 4A). Pastureland in the FT group slightly increased along the first period while sharply decreased between 2001 and 2015. Cropland area in the same group increased between 2001 and 2015 compared to the previous period, and was significantly and negatively correlated with the pastureland area change (rho −0.85, p < 0.01). LULC changes are fully described for both groups through transition matrices (SM—Transition matrices). From the PAM data we found sugarcane as the major crop representing the cropland class in the FT group (SM—Crop data). In the PD group, the pastureland area change was lower and negatively correlated with the cropland area expansion (rho −0.52, p < 0.01) between 2001 and 2015. At the municipality level, pastureland area decreased in the PD group (pushing stocking rate increase, Figure 4B) when compared to the areal change in both periods (1987–2001 and 2001–2015), however it was 2.6 times lower than the observed decreasing rates in the FT group during the 2001–2015 period.
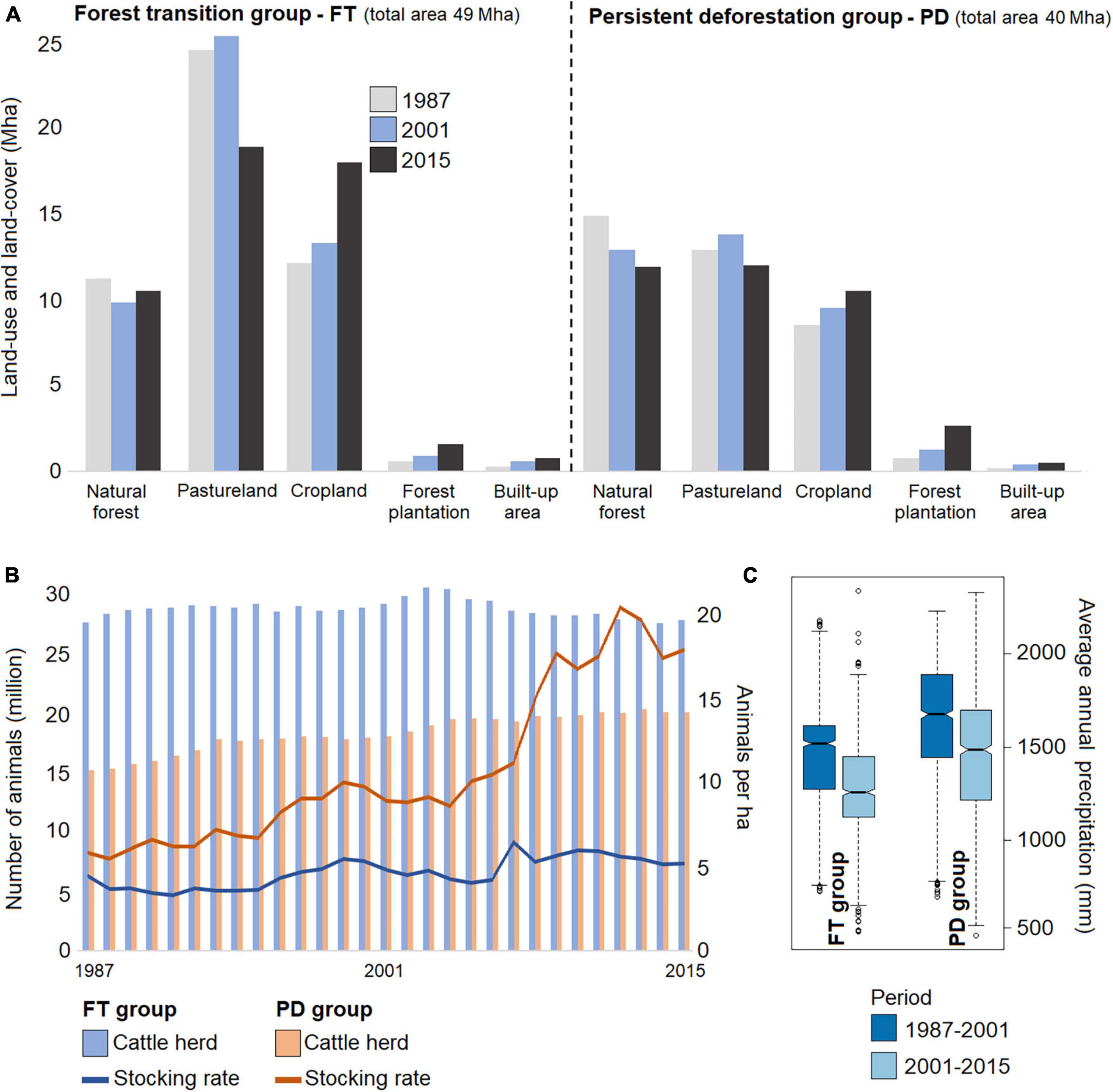
Figure 4. (A) Land-use and land-cover area in the years 1987, 2001, and 2015, in the Forest transition (FT) and Persistent deforestation (PD) groups. (B) Annual change in cattle herd between 1987 and 2015, and the respective stocking rate (number of animals per hectare of pastureland). (C) Average annual precipitation in the FT and PD groups, for the 1987–2001 and 2001–2015 periods, respectively.
The increasing stocking rates in the PD group were positively and significantly correlated with NFC net loss in 2001–2015 (rho 0.14, p < 0.01), and with cropland (rho 0.23, p < 0.01)—the last indicating the intensive livestock (i.e., higher number of cattle per land unit) tending to occur in areas over higher expansion of crop production, which replaced pasturelands. In the PD group we found soybean as the major production associated with the cropland class (SM—Crop data). Interestingly, we found higher precipitation levels positively and significantly correlated to the expansion of agropastoral activities (SM—Precipitation and agricultural trends). Additionally, precipitation was significantly higher (p < 0.01—t-test) in the PD group than in the FT group, however, in both groups, precipitation significantly decreased (p < 0.01—paired t-test) during the 2001–2015 period (Figure 4C).
From the 1987–2001 period to the 2001–2015, built-up areas experienced a reduction in their areal expansion in the FT group, from an average of around 216 ha (1987–2001) to an average of 173 ha (2001–2015) per municipality, but still higher (2 times) than in the PD group. The opposite trend was observed in forest plantations in the same FT group, which increased the expansion from an average of 230 ha per municipality during the 1987–2001 period, to 578 ha in 2001–2015. However, the expansion of forest plantations in the PD group was 2.1 times higher than in the FT group during the 2001–2015 period. This LULC class had a greater acceleration in the PD group, from an average of 661 ha per municipality between 1987 and 2001 to an average of 1,773 ha in 2001–2015. Through the PEVS data we found two major tree species associated with forest plantations in the study area (i.e., Pinus spp. and Eucalyptus spp.) and each one with different distribution and uses among municipalities [e.g., PD group exhibited more Pinus used for wood and charcoal; FT group exhibited more Eucalyptus used for cellulose pulp production (SM—Forestry production)]. For industrial GDP, the FT group accounted for 63% of the study area in the period 2001–2015, while accounting for 64% of the human population in 2010 (SM—People and GDP). In Figure 5 we present the spatial distribution of municipalities the respective natura forest cover net change of each period.
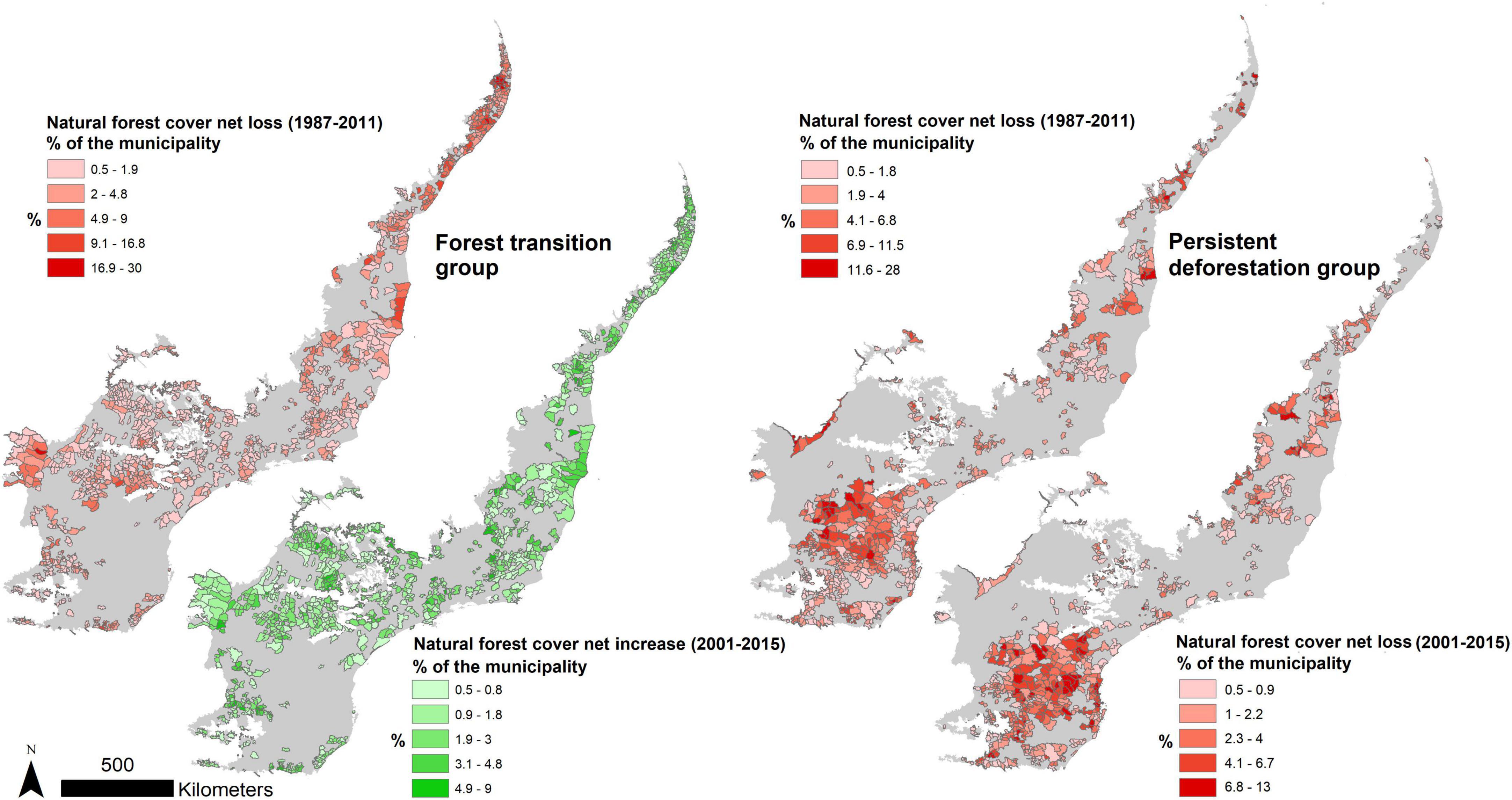
Figure 5. Natural forest cover (NFC) dynamics for a set of 1,972 municipalities in the Atlantic Forest biome that underwent NFC net loss between 1987 and 2001, a subset (n = 1,180) of municipalities which changed the NFC trajectory to net gain between 2001 and 2015 (Forest transition group—FT), and a subset (n = 792) of municipalities that kept the same trajectory of NFC net loss (Persistent deforestation group—PD).
4. Discussion
4.1. Human-environment dimensions of forest transition in the Atlantic Forest
Over the last three decades the Brazilian Atlantic Forest biome as a whole has exhibited a general steady-state in the conservation of natural areas (Silva et al., 2020b), which conceals multiple natural forest cover (NFC) trajectories at the municipality level, as revealed by this study. Our biome-scale analysis demonstrated that while some municipalities experienced a forest transition (i.e., net gain in NFC after a period of net loss) during the first 15 years of the 21st century, other municipalities persisted on a trend of forest net loss over the last 30 years (Figure 5), thus keeping the pressure on their remaining natural forests. These results show that the remaining patches of natural forest in the biome, which have high ecological value (Rosa et al., 2021), are still threatened.
Our results from spatial econometric models provide evidence that decreasing trends of agricultural activity are significantly correlated with NFC net gain in municipalities under forest transition—e.g., larger decreases in agricultural jobs are associated with NFC net gain (Figure 3). Agricultural labor data reinforce the evidence obtained from land-use and land-cover dynamics, where agricultural land uses (i.e., forest plantation, pastureland, and cropland combined) in the FT group lost 1.1 Mha in 2001–2015, and were mainly substituted by new NFC areas. This evidence supports the idea that decreases in rural labor favor the abandonment of less suitable agricultural areas (Macdonald, 2017; Abson, 2019). This land abandonment phenomenon is common in many parts of the globe (Chen et al., 2012; Li et al., 2013), thus is crucial for allowing natural vegetation recovery (Chazdon et al., 2020), and constitutes a key component of the regional forest transition observed in the Atlantic Forest (Silva et al., 2017b; Calaboni et al., 2018). Previous studies in the Atlantic Forest have also demonstrated that socioeconomic changes from the 1960s onward (i.e., industrialization) triggered the emergence of large urbanized areas (i.e., built-up class—significant and positively correlated with forest net gain, Figure 3) followed by a sharp rural out-migration process (Baeninger, 2005; Teixeira, 2005; Silva et al., 2017a). This phenomenon is attributed by landowners in the São Paulo Atlantic Forest as a reason for the lack of rural labor—i.e., a major factor for land abandonment and the return of NFC (Silva et al., 2018). Our Supplementary data (SM—Supplementary model) also shows that in the FT group during the 2001–2015 period, industrial GDP growth was positively associated with NFC net gain, which supports an economic development pathway of the forest transition theory.
Models for the FT group revealed precipitation as a key factor associated with NFCNC for both processes—i.e., net gain and loss. In the forest net loss model (Figure 3) we observed that higher precipitation is associated with deforestation at the municipality level, which is expected as it has been reported that high precipitation values are positively associated with agricultural expansion (Millington et al., 2021). Our data also demonstrated a significant correlation between higher precipitation levels and the expansion of cropland and cattle (SM—Precipitation and agricultural trends). However, precipitation levels significantly decreased in this group of municipalities during the 2001–2015 period (Figure 4C), which we found to be negatively correlated with NFC net gain (Figure 3). Municipalities experiencing lower average annual precipitation in the FT group may have reduced rain-fed cropping or cattle-ranching activities, leading to the abandonment of marginal lands and favoring the return of NFC. A similar trend already observed in tropical dry areas in Brazil (Espírito-Santo et al., 2020). Notice that precipitation levels were significantly lower in the FT group compared to PD group (Figure 4C). To reinforce this perspective, our Supplementary results (SM— Precipitation and agricultural trends) showed that increased yields, cropland area, and stocking rates were significantly associated with higher precipitation levels at the municipal level (i.e., better climate conditions seem to favor the expansion of agricultural activities). Previous studies in tropical regions have demonstrated that reductions in rainfall negatively affect agricultural revenues by decreasing agricultural productivity (Leite-Filho et al., 2021), thus compromising crop, pasture and cattle production, such as in the Atlantic Forest biome (Glickhouse, 2015; Silva et al., 2018; Castro, 2021). For instance, Silva et al. (2018) noted that producers in the Atlantic Forest biome are prone to abandon less suitable agricultural areas during periods of drastic rainfall shortages. This result is even more meaningful given the significant decrease in precipitation between the two periods of analysis (Figure 4C), leading municipalities with low precipitation levels in 1987–2001 to even lower levels in 2001–2015. Here we hypothesize that climate change (manifested by a significant decrease in precipitation) may also enhance natural forest regrowth through negative socio-ecological feedback mechanisms (e.g., agricultural abandonment), potentially leading to higher biomass production and carbon sequestration, thus reducing some of the negative effects of climate change.
As predicted by previous studies (Robinson et al., 2015; Silva et al., 2016; Viña et al., 2016; García et al., 2021), the presence of NFC areas was positively and significantly correlated with both trajectories, forest net loss and net gain. Our results (Figure 3) indicate that in municipalities experiencing a forest transition, the larger the areas of remaining forest, the higher the likelihood of increasing NFC. This relationship is explained by the fact that natural forest remnants support secondary succession and ecological restoration, due to their role as seed sources, enhancement of soil conditions in surrounding areas, and facilitating vital ecological interactions (Chazdon, 2003; Uriarte et al., 2011; Crouzeilles et al., 2017).
In municipalities experiencing a forest transition, a more diverse crop production was positively correlated with larger increases in NFC. Prior studies in Brazil have shown that land owned by small and medium farm producers, as well as municipalities with smaller areas per producer, tend to exhibit higher crop diversity (Silva et al., 2020a; Ricciardi et al., 2021). Additionally, beyond crop diversification, multi-functional rural landscapes are being developed toward the promotion of economic activities such as tourism, attracted by local food, traditions and scenic landscapes (Huylenbroeck et al., 2007; Barbieri and Valdivia, 2010). Thus, the transition of former agriculture-based rural areas to more multifunctional landscapes favor the emergence of non-agricultural rural economic activities (e.g., rural/eco-tourism and outdoor activities) allied to more diversified economic activities (Jongeneel et al., 2008; Jansuwan and Zander, 2022). In the Atlantic Forest biome (e.g., Paraíba Valley of São Paulo state) it has been noted that landowners of rural properties with tourist attractions are more prone to afforest and to conserve natural areas (Silva et al., 2018). This enhances the notion of NFC as a major asset for rural development.
The role of planted forests as a variable significantly correlated with both forest net loss and gain (Figure 3) helps us understand a key aspect of globalized supply-chains (Liu et al., 2013, 2018b; Kapsar et al., 2019). Based on the IBGE PEVS data (SM—Forestry production), we found that while in the FT group eucalyptus was the dominant tree species of the forest plantation class, in the PD group pine was the most dominant. As eucalyptus cellulose pulp (short-fiber pulp) produced in Brazil is mostly destined for global markets, its production is not only influenced by international demand (Ibá, 2019), but also by the commitment to sustainable production standards defined by the certification of the Forest Stewardship Council (FSC, since 2002). In 2019, 4.4 Mha (48%) of Brazilian forest plantations were certified by international eco-certification standards (Ibá, 2020). This has fostered the regrowth of natural vegetation in the Brazilian Atlantic Forest biome in recent decades (Silva et al., 2019). In contrast, pine plantations—the second largest tree species used in Brazilian forest plantations after eucalyptus and mostly occurring in the control group—are less committed to sustainable practices as they are largely destined for domestic consumption (Ibá, 2019). Furthermore, from the total charcoal production (derived mainly from eucalyptus) in the study area (i.e., accounting for both FT and PD groups of municipalities) in 2015, over 80% was produced in the PD group. Charcoal production [highly demanded (90% of Brazil’s production) by the national steel industry (Ministry of Energy [MME], 2018)] is a less regulated forestry sector, given the high costs associated with sustainability standards that are usually not paid-off by charcoal revenues, as it is a lower priced product. This dis-incentivizes the adoption of eco-certification approaches (Albuquerque, 2019). Our supplementary econometric results corroborate this association by showing cellulose pulp production from eucalyptus positively correlated with NFC net gain (SM—Supplementary model). These results on the impacts of forest plantations on NFC dynamics agree with the environmental impacts associated with the agricultural production for domestic and international markets, previously noted for beef (Zu Ermgassen et al., 2020).
4.2. Land-use and land-cover dynamics in the Atlantic Forest
The land-use and land-cover data also revealed that in the group of municipalities under persistent deforestation (PD group), land-use classes such as forest plantation increased at higher rates compared to the levels observed in the municipalities under forest transition (FT group). Although pastureland area decreased in both groups from 2001 to 2015 (Figure 4A), in the PD group the decrease was 3.6 times lower than in the FT group. Regarding this land-use class, we also found the cattle stocking rate significantly increased in PD municipalities and also with higher increases in cropland. This result suggests that when cropland is expanding, grazing areas decrease, leading rural producers to intensify their cattle production in more confined areas, although not necessarily inducing the return of NFC areas. In fact, this complex relationship seems to be inducing deforestation in neighboring municipalities, as it can be seen by the regional patterns observed in Figure 5 (PD group). A similar phenomenon was observed in agricultural areas in the Amazon and Cerrado biomes where increases in soybean production stimulated deforestation in neighboring municipalities (Silva et al., 2021). This is likely to be a major process occurring in the PD group, since increases in crop yield (Figure 3), where soybean is the major crop (SM—Crop data), were significantly correlated with NFC net loss.
Here we highlight a possible “rebound” effect of intensification (i.e., high yield increases) which instead of sparing land for conservation, drives more agricultural expansion (leading to more deforestation), as agricultural agents see intensification as a great economic opportunity (Garrett et al., 2018). This seems to foster an agricultural expansion leading to more deforestation (Hubert et al., 2010; García et al., 2020; Millington et al., 2021). Regarding changes in crop yield observed in the FT group, no significant correlation with NFC gain during 2001–2015 was observed (Figure 3). This result suggests that the forest transition process observed in the study area was not related with land sparing effects due to crop intensification.
In non-agricultural land use areas (i.e., forest plantation, pastureland, and cropland combined), where the PD group exhibited an increase of 300,000 ha during 2001–2015 while the FT group exhibited a decrease of 1.1 Mha (Figure 4A), built-up areas increased 2 times more in the FT group than in the PD group. This result suggests that built-up areas in the FT group represent larger centers of socioeconomic development [and with higher shares of population and industrial GDP compared to the PD group (SM—People and GDP), while GDP was positively associated with NFC net gain (SM—Supplementary model)], a key component of the forest transition development pathway (Mather, 1992; Meyfroidt and Lambin, 2011; Wilson et al., 2017). These major land changes highlight previous observations supporting regional Atlantic Forest transition pathways of economic and social development (Baptista, 2008; Silva et al., 2016).
4.3. Insights to enhance environmental governance toward forest transition
Garibaldi et al. (2020) found that “working landscapes”—farming, ranching and forestry activities—need the conservation of at least 20% of native habitats to promote healthy landscapes capable of supporting sustainably the human society. The FT group (1,180 municipalities) exhibited an average of ca. 20% of natural forest cover (NFC), although 743 municipalities exhibited lower values. Hence, our results support that the presence of local natural forest remnants with at least this minimum threshold may enhance biodiversity conservation (e.g., favoring natural vegetation regrowth) in landscapes dominated by agricultural activities. On this regard, we recall the “windows of opportunities” concept from the adaptive governance literature, which refers to the “existence of circumstances or events that trigger and promote governance changes to manage ecosystems and common-pool resources more sustainably” (Lorenzen et al., 2021). Thus, we argue that adaptive governance [the act of governing landscapes as complex human-environmental systems with the capacity to respond to ecosystem-wide changes (Schultz et al., 2015)] approaches do not necessarily have to emerge as a response to crises or to negative environmental outcomes, but may also emerge from positive changes, such as the forest transition. Since deforestation is still ongoing in many parts of the Atlantic Forest biome, processes that enhance a forest transition need to be considered within contemporary governance systems, including sectorial voluntary agreements (e.g., eco-certifications), technical assistance to promote informed decisions regarding the use of land and natural resources in rural settings, together with social engagement to promote a steady and long-term transition process.
We also call for the need for policies incentivizing the abandonment of areas less suitable for agricultural production, such as those with significant decreases in precipitation levels. From the adaptive governance perspective, policymakers and other stakeholders could take advantage of the potential negative socio-ecological feedback of precipitation to foster land-use abandonment of lower precipitation areas to allow for natural forest regeneration. The inclusion of payment for ecosystem services programs together with enhanced local food production systems under place-based public policy frameworks, would foster the transition of rural areas toward coupled rural-urban systems (Silva et al., 2017c). Such rural-urban linkages at local and regional scales may incentivize outdoor activities (e.g., cycling, trekking, camping), rural tourism, and respect for local cultures, which are associated with an increase in conservation values (Silva et al., 2017c). These relationships provide a feedback mechanism that promotes environmental conservation actions by landowners, hence favoring a forest transition.
5. Concluding remarks
Forest transition is an important outcome that not only contributes to ecosystem restoration, but also constitutes a valuable approach for the tasks proposed by the UN Decade on Ecosystem Restoration (2021–2030). Previous studies have noted that biophysical factors such as slope and proximity to water and natural vegetated areas contribute to forest regeneration (Borda-Niño et al., 2019). This study demonstrates that in addition to those factors, changes in the precipitation regime (i.e., reduced precipitation) also contribute to this land change phenomenon. Furthermore, in municipalities experiencing forest transition, significant changes in agricultural activities were observed in the form of decreases in agricultural land area and rural jobs, while urban areas are increasing at the same time, favoring natural forest net gain. These findings can be used to help design policies that enhance forest transition not only in the Atlantic Forest biome but also in other tropical areas, as agro-pastoral activities and environmental conservation actions can both thrive under better-planned landscapes. Such landscapes can be obtained through the implementation of policies that orient and organize human occupation and land uses, while also vigorously adopting sustainable production systems.
Data availability statement
The original contributions presented in this study are included in the article/Supplementary material, further inquiries can be directed to the corresponding author.
Author contributions
RB led the manuscript conceptualization, methodology, data-gathering, software, investigation, formal analysis, and the writing–original draft, writing-review and editing. EM, JL, AV, YD, SV, ML, and JM contributed to the investigation and conceptual framework of analysis, commented on the manuscript, and writing–review and editing. All authors contributed to the article and approved the submitted version.
Funding
JL, AV, EM, and RB were supported by the National Science Foundation of the United States, grants nos. 1924111 and 1531086.
Acknowledgments
We wish to thank the National Science Foundation of the United States (grants 1924111 and 1531086) and Michigan AgBioResearch for their financial support. We are very grateful to MapBiomas who generated the land-use and land-cover data used in this manuscript (Project MapBiomas—Collection v.5.0 of Brazilian Land Cover and Use Map Series, accessed on January 15, 2021 through the link: https://mapbiomas.org/colecoes-mapbiomas?cama_set_language=en).
Conflict of interest
The authors declare that the research was conducted in the absence of any commercial or financial relationships that could be construed as a potential conflict of interest.
Publisher’s note
All claims expressed in this article are solely those of the authors and do not necessarily represent those of their affiliated organizations, or those of the publisher, the editors and the reviewers. Any product that may be evaluated in this article, or claim that may be made by its manufacturer, is not guaranteed or endorsed by the publisher.
Supplementary material
The Supplementary Material for this article can be found online at: https://www.frontiersin.org/articles/10.3389/ffgc.2023.1071495/full#supplementary-material
Footnotes
References
Abson, D. (2019). “The economic drivers and consequences of agricultural specialization,” in Agroecosystem diversity, eds G. Lemaire, P. Carvalho, S. Kronberg, and S. Recous (Cambridge, MA: Academic Press), 301–315. doi: 10.1016/B978-0-12-811050-8.00019-4
Aguilar, J., Gramig, G., Hendrickson, J., Archer, D., Forcella, F., and Liebig, M. (2015). Crop species diversity changes in the United States: 1978–2012. PLoS One 10:e0136580. doi: 10.1371/journal.pone.0136580
Anselin, L. (1995). Local indicators of spatial association-LISA. Geogr. Anal. 27, 93–115. doi: 10.1111/j.1538-4632.1995.tb00338.x
Baeninger, R. (2005). São Paulo e sua migrações no final do século 20. São Paulo Perspect. 19, 84–96. doi: 10.1590/S0102-88392005000300008
Baptista, S. (2008). Metropolitanization and forest recovery in southern Brazil: A multiscale analysis of the Florianópolis city-region, Santa Catarina State, 1970 to 2005. Ecol. Soc. 13:5. doi: 10.5751/ES-02426-130205
Barbieri, C., and Valdivia, C. (2010). Recreation and agroforestry: Examining new dimensions of multifunctionality in family farms. J. Rural Stud. 26, 465–473. doi: 10.1016/j.jrurstud.2010.07.001
Borda-Niño, M., Meli, P., and Brancalion, P. (2019). Drivers of tropical forest cover increase. Land Degradat. Dev. 31, 1366–1379. doi: 10.1002/ldr.3534
Buainain, A., Alves, E., Silveira, J., and Navarro, Z. (2014). The rural world in Brazil in the 21st century. Brasília: Embrapa.
Burgess, N., Doggart, N., and Lovett, J. (2002). The uluguru mountains of eastern tanzania: The effect of forest loss on biodiversity. Oryx 36, 140–152. doi: 10.1017/S0030605302000212
Calaboni, A., Tambosi, L., Igari, A., Farinaci, J., Metzger, J., and Uriarte, M. (2018). The forest transition in São Paulo, Brazil: Historical patterns and potential drivers. Ecol. Soc. 23:7. doi: 10.5751/ES-10270-230407
Carlson, A., Zaehringer, J., Garrett, R., Silva, R., Furumo, P., Rey, A., et al. (2018). Toward rigorous telecoupling causal attribution: A systematic review and typology. Sustainability 10:4426. doi: 10.3390/su10124426
Castro, R. (2021). As pastagens acabaram’: Seca histórica destrói produção em cidade brasileira mais atingida. Época. Available online at: https://oglobo.globo.com/epoca/sociedade/um-so-planeta/as-pastagens-acabaram-seca-historica-destroi-producao-em-cidade-brasileira-mais-atingida-25017186 (accessed June 15, 2022).
Center for Weather Forecast and Climate Studies (2021). Dados Observacionais: Dados históricos. Available online at: http://bancodedados.cptec.inpe.br/ (accessed January 20, 2022).
Chazdon, R. (2003). Tropical forest recovery: Legacies of human impact and natural disturbances. Perspect. Plant Ecol. Evol. Syst. 6, 51–71. doi: 10.1078/1433-8319-00042
Chazdon, R., Lindenmayer, D., Guariguata, M., Crouzeilles, R., Benayas, J., and Chavero, E. (2020). Fostering natural forest regeneration on former agricultural land through economic and policy interventions. Environ. Res. Lett. 15:043002. doi: 10.1088/1748-9326/ab79e6
Chazdon, R., and Uriarte, M. (2016). Natural regeneration in the context of large-scale forest and landscape restoration in the tropics. Biotropica 48, 709–715. doi: 10.1111/btp.12409
Chen, H., Shao, L., Zhao, M., Zhang, X., and Zhang, D. (2017). Grassland conservation programs, vegetation rehabilitation and spatial dependency in Inner Mongolia, China. Land Use Policy 64, 429–439. doi: 10.1016/j.landusepol.2017.03.018
Chen, X., Frank, K., Dietz, T., and Liu, J. (2012). Weak ties, labor migration, and environmental impacts: Towards a sociology of sustainability. Organ. Environ. 25, 3–24. doi: 10.1177/1086026611436216
Crouzeilles, R., Beyer, H., Monteiro, L., Feltran-Barbieri, R., Pessôa, A., Barros, F., et al. (2020). Achieving cost-effective landscape-scale forest restoration through targeted natural regeneration. Conserv. Lett. 13:e12709. doi: 10.1111/conl.12709
Crouzeilles, R., Ferreira, M., Chazdon, R., Lindenmayer, D., Sansevero, J., Monteiro, L., et al. (2017). Ecological restoration success is higher for natural regeneration than for active restoration in tropical forests. Sci. Adv. 3:e1701345. doi: 10.1126/sciadv.1701345
Curtis, P., Slay, C., Harris, N., Tyukavina, A., and Hansen, M. (2018). Classifying drivers of global forest loss. Science 361, 1108–1111. doi: 10.1126/science.aau3445
Espírito-Santo, M., Rocha, A., Leite, M., Silva, J., Silva, L., and Sanchez-Azofeifa, G. (2020). Byophisical and socioeconomic factors associated to deforestation and forest recovery in Brazilian tropical dry forests. Front. For. Glob. Change 3:569184. doi: 10.3389/ffgc.2020.569184
Farinaci, J., and Batiatella, M. (2012). Variação na cobertura vegetal nativa em São Paulo: Um panorama do conhecimento atual. Rev. Árvore 36, 695–705. doi: 10.1590/S0100-67622012000400011
García, V., Caravaggio, N., Gaspart, F., and Meyfroidt, P. (2021). Long- and short-run forest dynamics: An empirical assessment of forest transition, environmental kuznets curve and ecologically unequal exchange theories. Forests 12:431. doi: 10.3390/f12040431
García, V., Gaspart, F., Kastner, T., and Meyfroidt, P. (2020). Agricultural intensification and land use change: Assessing country-level induced intensification, land sparing and rebound effect. Environ. Res. Lett. 15:085007. doi: 10.1088/1748-9326/ab8b14
Garibaldi, L., Oddi, F., Miguez, F., Bartomeus, I., Orr, M., Jobbágy, E., et al. (2020). Working landscapes need at least 20% native habitat. Conserv. Lett. 14:e12773. doi: 10.1111/conl.12773
Garrett, R., Koh, I., Lambin, E., Waroux, Y., Kastens, J., and Brown, J. (2018). Intensification in agriculture-forest frontiers: Land use responses to development and conservation policies in Brazil. Glob. Environ. Change 53, 233–243. doi: 10.1016/j.gloenvcha.2018.09.011
Geist, H., and Lambin, E. (2002). Proximate causes and underlying driving forces of tropical deforestation. Bioscience 52, 143–150. doi: 10.1641/0006-3568(2002)052[0143:PCAUDF]2.0.CO;2
Glickhouse, R. (2015). Brazil update: Historic drought takes toll on agriculture. New York, NY: Americas Quarterly.
Gupta, S., and Mackenzie, M. (2016). Spatial patterns of soil respiration links above and belowground process along a Boreal Aspen Fire Chronosequence. PLoS One 11:e165602. doi: 10.1371/journal.pone.0165602
Hubert, B., Rosegrant, M., Boekel, M., and Ortiz, R. (2010). The future of food: Scenarios for 2050. Crop Sci. 50, 33–50. doi: 10.2135/cropsci2009.09.0530
Huylenbroeck, G., Vandermeulen, V., Mettepenningen, E., and Verspecht, A. (2007). Multifunctionality of agriculture: A review of definitions, evidence and instruments. Living Rev. Landscape Res. 1:3. doi: 10.12942/lrlr-2007-3
IPCC (2021). “Summary for Policymakers,” in Climate Change 2021: The Physical Science Basis. Contribution of Working Group I to the Sixth Assessment Report of the Intergovernmental Panel on Climate Change, eds V. Masson-Delmotte, A. Zhai, S. L. Pirani, C. Connors, S. Péan, N. Berger, et al. (Cambridge: Cambridge University Press).
Jansuwan, P., and Zander, K. (2022). Multifunctional farming as successful pathway for the next generation of Thai farmers. PLoS One 17:e0267351. doi: 10.1371/journal.pone.0267351
Jongeneel, R., Polman, N., and Slangen, L. H. G. (2008). Why dutch farmers going multifunctional? Land Use Policy 25, 81–94. doi: 10.1016/j.landusepol.2007.03.001
Kapsar, K., Hovis, C., Silva, R., Buchholtz, E., Carlson, A., Dou, Y., et al. (2019). Telecoupling research: The first five years. Sustainability 11:1033. doi: 10.3390/su11041033
Lambin, E., and Meyfroidt, P. (2010). Land use transitions: Socio-ecological feedbacks versus socio-economic change. Land Use Policy 27, 108–118. doi: 10.1016/j.landusepol.2009.09.003
Leite-Filho, A., Soares-Filho, B., Davis, J., Abrahão, G., and Börner, J. (2021). Deforestation reduces rainfall and agricultural revenues in the Brazilian Amazon. Nat. Commun. 12:2591. doi: 10.1038/s41467-021-22840-7
Li, Y., Viña, A., Yang, W., Chen, X., Zhang, J., Ouyang, Z., et al. (2013). Effects of conservation policies on forest cover change in giant panda habitat regions. China. Land Use Policy 33, 42–53. doi: 10.1016/j.landusepol.2012.12.003
Liu, J. (2023). Leveraging the metacoupling framework for sustainability science and global sustainable development. Nat. Sci. Rev. 10:nwad090. doi: 10.1093/nsr/nwad090
Liu, J., Dietz, T., Carpenter, S., Alberti, M., Folke, C., Moran, E., et al. (2007). Complexity of coupled human and natural systems. Science 317:5844. doi: 10.1126/science.1144004
Liu, J., Hull, V., Batistella, M., DeFries, R., Dietz, T., Fu, F., et al. (2013). Framing sustainability in a telecoupled world. Ecol. Soc. 18:26. doi: 10.5751/ES-05873-180226
Liu, J., Viña, A., Yang, W., Li, S., Xu, W., and Zheng, H. (2018a). China’s environment on a metacoupled planet. Ann. Rev. Environ. Res. 43, 1–34. doi: 10.1146/annurev-environ-102017-030040
Liu, J., Dou, Y., Batistella, M., Challies, E., Connor, T., Friis, C., et al. (2018b). Spillover systems in a telecoupled anthropocene: Typology, methods, and governance for global sustainability. Curr. Opin. Environ. Sustain. 33, 58–69. doi: 10.1016/j.cosust.2018.04.009
Lorenzen, M., Orozco-Ramírez, Q., Ramírez-Santiago, R., and Garza, G. (2021). The forest transition as a window of opportunity to change the governance of common-pool resources: The case of Mexico’s Mixteca Alta. World Dev. 145:105516. doi: 10.1016/j.worlddev.2021.105516
Macdonald, J. (2017). Consolidation, concentration, and competition in the food system. Farm. Policy J. 14, 25–35.
Marques, M., and Grelle, C. (eds) (2021). The Atlantic Forest: History, biodiversity, threats and opportunities of the mega-diverse forest. Cham: Springer. doi: 10.1007/978-3-030-55322-7
Martins, S., Neto, E., Piccolo, M., Almeida, Q., Camargo, P., Carmo, J., et al. (2015). Soil texture and chemical characteristics along an elevation range in the coastal Atlantic Forest of Southeast Brazil. Geoderma Region. 5, 106–116. doi: 10.1016/j.geodrs.2015.04.005
Meyfroidt, P., and Lambin, E. (2011). Global forest transition: Prospects to an end to deforestation. Annu. Rev. Environ. Resour. 36, 343–371. doi: 10.1146/annurev-environ-090710-143732
Millington, J., Katerinchuk, V., Silva, R., Victoria, D., and Batistella, M. (2021). Modelling drivers of Brazilian agricultural change in a telecoupled world. Environ. Model. Softw. 139:105024. doi: 10.1016/j.envsoft.2021.105024
Ministry of Energy [MME] (2018). Brazilian energy balance. empresa de pesquisa energética. Available online at: https://www.epe.gov.br/en/publications/publications/brazilian-energy-balance/brazilian-energy-balance-2018 (accessed July 1, 2022).
Miranda, A., Altamirano, A., Cayuela, L., Lara, A., and González, M. (2017). Native forest loss in the Chilean biodiversity hotspot: Revealing the evidence. Region. Environ. Change 17, 285–297. doi: 10.1007/s10113-016-1010-7
Nave, A., Rodrigues, R., Brancalion, P., Farah, F., Silva, C., and Lamonato, F. (2016). Manual de Restauração Ecológica. Bioflora Tecnologia da Restauração, Piraciba. São Paulo: Fibria.
Ouyang, Z., Liu, J., Han, X., Tan, Y., and Zhang, H. (2001). An assessment of giant panda habitat in Wolong Nature Reserve. Sichuan, China. Acta Ecol. Sin. 21, 1869–1874.
Perz, S., and Skole, D. (2003). Secondary forest expansion in the Brazilian Amazon and the refinement of the Forest Transition Theory. Soc. Nat. Res. 16, 277–294. doi: 10.1080/08941920390178856
Piffer, P., Calaboni, A., Rosa, M., Schwartz, N., and Tambosi, L. (2021). Ephemeral forest regeneration limits carbon sequestration potential in the Brazilian Atlantic Forest. Glob. Change Biol. 28, 630–643. doi: 10.1111/gcb.15944
Pinto, L., and Voivodic, M. (2021). Reverse the tipping point of the Atlantic Forest for mitigation. Nat. Clim. Change 11, 364–365. doi: 10.1038/s41558-021-01035-4
Poorter, L., Craven, D., Jakovac, C., van der Sande, M., Amissah, L., and Bongers, F. (2021). Multidimensional tropical forest recovery. Science 374, 1370–1376. doi: 10.1126/science.abh3629
Rezende, C., Scarano, F., Assad, E., Joly, C., Metzger, J., Strassburg, B., et al. (2018). From hotspots to hope spot: An opportunity for the Brazilian Atlantic Forest. Perspect. Ecol. Conserv. 16, 208–214. doi: 10.1016/j.pecon.2018.10.002
Ricciardi, V., Mehrabi, Z., Wittman, H., James, D., and Ramankutty, N. (2021). Higher yields and more biodiversity on smaller farms. Nat. Sustain. 4, 651–657. doi: 10.1038/s41893-021-00699-2
Robinson, S., Berg, E., Meirelles, G., and Ostle, N. (2015). Factors influencing early secondary succession and ecosystem carbon stocks in Brazilian Atlantic Forest. Biodivers. Conserv. 24, 2273–2291. doi: 10.1007/s10531-015-0982-9
Rosa, M., Brancalion, P., Crouzeilles, R., Tambosi, L., Piffer, P., Lenti, F., et al. (2021). Hidden destruction of older forests threatens Brazil’s Atlantic Forest and challenges restoration programs. Sci. Adv. 7:eabc4547. doi: 10.1126/sciadv.abc4547
Rudel, T., Oliver, T., Achard, F., Angelsen, A., Xu, J., and Lambin, E. (2005). Forest transitions: Towards a global understanding of the land use change. Glob. Environ. Change 15, 23–31. doi: 10.1016/j.gloenvcha.2004.11.001
Safar, N., Magnago, L., and Schaefer, C. (2020). Resilience of lowland Atlantic forests in a highly fragmented landscape: Insights on the temporal scale of landscape restoration. For. Ecol. Manag. 470/471:118183. doi: 10.1016/j.foreco.2020.118183
Saputro, D., Muhsinin, R., Widyaningsih, P., and Sulistyaningsih. (2019). Spatial autoregressive with a spatial autoregressive error term model and its parameter estimation with two-stage generalized spatial least square procedure. J. Phys. 1217:012104. doi: 10.1088/1742-6596/1217/1/012104
Saraiva, M., Ferreira, M., Cunha, D., Daniel, L., Homma, A., and Pires, G. (2020). Forest regeneration in the Brazilian Amazon: Public policies and economic conditions. J. Clean. Prod. 269:122424. doi: 10.1016/j.jclepro.2020.122424
Scaranello, M., Alves, L., Vieira, S., Camargo, P., Joly, C., and Martinelli, L. (2012). Height-diameter relationships of tropical Atlantic moist forest trees in southeastern Brazil. Sci. Agric. 69, 26–37. doi: 10.1590/S0103-90162012000100005
Schierhorn, F., Meyfroidt, P., Kastner, T., Kuemmerle, T., Prishchepov, A., and Muller, D. (2016). The dynamics of beef trade between Brazil and Russia and their environmental implications. Glob. Food Sec. 11, 84–92. doi: 10.1016/j.gfs.2016.08.001
Schultz, L., Folke, C., Österblom, H., and Olsson, P. (2015). Adaptive governance, ecosystem management, and natural capital. PNAS 112, 7369–7374. doi: 10.1073/pnas.1406493112
Seino, E., Algarve, G., and Gobbo, J. (2013). Opening policy and Brazilian redemocratization: Between modern-conservative and a “new civil society”. Sem. Aspas 2, 31–42.
Silva, F., Batistella, M., Millington, J., Moran, E., Martinelli, L., Dou, Y., et al. (2020a). Three decades of changes in Brazilian municipalities and their food production systems. Land 9:422. doi: 10.3390/land9110422
Silva, F., Millington, J., Moran, E., Batistella, M., and Liu, J. (2020b). Three decades of land-use and land-cover change in mountain regions of the Brazilian Atlantic Forest. Landsc. Urban Plann. 204:103948. doi: 10.1016/j.landurbplan.2020.103948
Silva, R., Batistella, M., and Moran, E. (2016). Drivers of land change: Human environment interactions and the Atlantic Forest transition in the Paraíba Valley, Brazil. Land Use Policy 58, 133–144. doi: 10.1016/j.landusepol.2016.07.021
Silva, R., Batistella, M., and Moran, E. (2017a). Socioeconomic changes and environmental policies as dimensions of regional land transitions in the Atlantic Forest, Brazil. Environ. Sci. Policy 74, 14–22. doi: 10.1016/j.envsci.2017.04.019
Silva, R., Batistella, M., Moran, E., and Lu, D. (2017b). Land changes fostering Atlantic Forest transition in Brazil: Evidences from the Paraíba Valley. Prof. Geogr. 69, 80–93. doi: 10.1080/00330124.2016.1178151
Silva, R., Rodrigues, M., Vieira, S., Batistella, M., and Farinaci, J. (2017c). Perspectives for environmental conservation and ecosystem services on coupled rural–urban systems. Perspect. Ecol. Conserv. 15, 74–81. doi: 10.1016/j.pecon.2017.05.005
Silva, R., Batistella, M., and Moran, E. (2018). Regional socioeconomic changes affecting rural area livelihoods and Atlantic Forest transitions. Land 7:125. doi: 10.3390/land7040125
Silva, R., Batistella, M., Palmieri, R., Dou, Y., and Millington, J. (2019). Eco-certification protocols as mechanisms to foster sustainable environmental practices in telecoupled systems. For. Policy Econ. 105, 52–63. doi: 10.1016/j.forpol.2019.05.016
Silva, R., Viña, A., Moran, E., Dou, Y., Batistella, M., and Liu, J. (2021). Socioeconomic and environmental effects of soybean production in metacoupled systems. Sci. Rep. 11:18662. doi: 10.1038/s41598-021-98256-6
Simiski, A., Zambiazi, D., Santos, K., and Fantini, A. (2021). Dynamics of Natural Regeneration: Implications for Landscape Restoration in the Atlantic Forest, Brazil. Front. For. Glob. Change 4:576908. doi: 10.3389/ffgc.2021.576908
SOS Mata Atlântica, and INPE (2022). Atlas dos remanescentes florestais da Mata Atlântica: Período 2020-2021. São Paulo: INPE, 72.
Teixeira, J. (2005). Modernização da agricultura no brasil: Impactos econômicos, sociais e ambientais. Rev. Eletrôn. Assoc. Geógr. Brasil. 2, 21–42.
Uriarte, M., Anciaes, M., da Silva, M., Rubim, P., Johnson, E., and Bruna, E. (2011). Disentangling the drivers of reduced long- distance seed dispersal by birds in an experimentally fragmented landscape. Ecology 92, 924–937. doi: 10.1890/10-0709.1
Valeriano, M. M. (2008). TOPODATA: Guia para utilização de dados geomorfológicos locais. São José dos Campos: INPE.
Viña, A., McConnell, W. J., Yang, H., Xu, Z., and Liu, J. (2016). Effects of conservation policy on China’s forest recovery. Sci. Adv. 2:e1500965. doi: 10.1126/sciadv.1500965
Viola, E., and Gonçalves, V. (2019). Brazil ups and downs in global environmental governance in the 21st century. Rev. Brasil. Polít. Int. 62:e010. doi: 10.1590/0034-7329201900210
Wilson, S. J., Schelhas, J., Grau, R., Nanni, A. S., and Sloan, S. (2017). Forest ecosystem-service transitions: The ecological dimensions of the forest transition. Ecol. Soc. 22:38. doi: 10.5751/ES-09615-220438
Yang, W., Liu, W., Viña, A., Luo, J., He, G., Ouyang, Z., et al. (2013). Performance and Prospects of Payments for Ecosystem Services Scheme Programs: Evidence from China. J. Environ. Manag. 127, 86–95. doi: 10.1016/j.jenvman.2013.04.019
Keywords: forest resilience, regeneration, human dimensions, spatial modeling, complex systems toward a forest transition
Citation: Bicudo da Silva RF, Moran E, Viña A, Millington JDA, Dou Y, Vieira SA, Lopez MC and Liu J (2023) Toward a forest transition across the Brazilian Atlantic Forest biome. Front. For. Glob. Change 6:1071495. doi: 10.3389/ffgc.2023.1071495
Received: 16 October 2022; Accepted: 14 June 2023;
Published: 29 June 2023.
Edited by:
Jess K. Zimmerman, University of Puerto Rico, Puerto RicoReviewed by:
Celso H. L. Silva-Junior, University of California, Los Angeles, United StatesYan Gao, Universidad Nacional Autonoma de Mexico, Mexico
Copyright © 2023 Bicudo da Silva, Moran, Viña, Millington, Dou, Vieira, Lopez and Liu. This is an open-access article distributed under the terms of the Creative Commons Attribution License (CC BY). The use, distribution or reproduction in other forums is permitted, provided the original author(s) and the copyright owner(s) are credited and that the original publication in this journal is cited, in accordance with accepted academic practice. No use, distribution or reproduction is permitted which does not comply with these terms.
*Correspondence: Ramon Felipe Bicudo da Silva, cmJpY3Vkb0B1bmljYW1wLmJy