- 1Laboratorio de Ecología de Bosques and Laboratorio de Dendrocronología y Cambio Global, Facultad de Ciencias Forestales y Recursos Naturales, Instituto de Conservación, Biodiversidad y Territorio, Universidad Austral de Chile, Valdivia, Chile
- 2Center for Climate and Resilience Research (CR)2, Santiago, Chile
- 3Center for Fire and Socioecosystem Resilience (FireSES), Valdivia, Chile
- 4Departamento de Ciencias Naturales y Tecnología, Universidad de Aysén, Coyhaique, Chile
- 5Centro de Investigación Gaia Antártica and Cape Horn International Center (CHIC), Universidad de Magallanes, Punta Arenas, Chile
Forest disturbances influence Fitzroya cupressoides forest structure and carbon stocks at multiple spatial and temporal scales. Natural disturbances such as landslides and volcanism affect and give rise to the mostly pristine Fitzroya stands present in the Andean cordillera. On the other hand, mostly human-caused fires and logging have been the main processes shaping the structure of Fitzroya stands in the Coastal range and of Fitzroya small remnants in the Central depression. The main goal of this study was to assess the carbon stocks and accumulation rates of Fitzroya forest stands according to their development stage under different disturbance regimes and environmental conditions given by the three physiographic units where the species grows (Coastal range, Central depression, and Andean range). The site selection included an age sequence of stands, known as a chronosequence approach. We identified Fitzroya post-disturbance stands in three different stages of development: young forest stage (mean stand age of the main cohort ≤ 200 years old), mature forest stage (200–800 years old), and old growth forest stage (800–1,500 years old). The following biomass components were considered: living standing trees, dead standing trees (snags), and logs from dead trees laying on the ground (coarse woody debris). Old-growth Fitzroya forests reached a mean total carbon stock (standing live trees, snags, and coarse woody debris) of 507, 279, and 331 Mg C ha−1 in the Andean and Coastal ranges, and Central depression, respectively. Fitzroya cupressoides contributes, in average, more than 80% to the total carbon stock in the Andean and Coastal ranges, and 63% in the Central depression. The remainder corresponds mainly to Nothofagus spp. The high carbon stocks in old-growth stands in the Andean range are explained by Fitzroya longevity, larger size, wood decay resistance, and the low recurrence of volcanic events. Carbon accumulation rates differ between the forests in the three physiographic units (Central depression>Andean range>Coastal range), mainly due to the different growth rates and environmental conditions present in each unit. In the context of climate change, conserving old-growth stands with large biomass and carbon stocks and restoring Fitzroya forests should be recognized as a key contribution toward national and global goals to mitigate global warming.
Introduction
Forests and their soils are recognized as important components of the global carbon cycle and have received increasing attention during recent decades due to climate change (Luyssaert et al., 2008; Campioli et al., 2016; Duque et al., 2021; Malhi et al., 2021). Forest ecosystems, in their above- and belowground components, contain the majority of the terrestrial carbon stocks of the earth, and changes in disturbance regimes can have an important impact on them, as well as on the related atmospheric and biogeochemical cycles, biodiversity, and ecosystem services (Thom and Seidl, 2016; Seidl et al., 2017; Anderegg et al., 2020; McDowell et al., 2020). Although carbon sequestration has become an important objective as a policy mitigation measure to counteract the anthropogenic CO2 emissions and their effects on global warming (IPCC, 2022), there remains an incomplete understanding of old-growth forests as carbon sinks and the modifying impact of land-use change and large-scale disturbances (e.g., fire and volcanism) on carbon stocks (Keith et al., 2009; Hoover et al., 2012).
Periods of carbon accrual following forest disturbances, typically range from decades to millennia, and the rate of recovery of total biomass can differ across stands according to climate, soil type, growth rate of the dominant tree species, and the frequency and intensity of natural or human disturbance regimes (Keith et al., 2009). Total living (aboveground) and dead biomass of old-growth primary forests from different regions around the world were reported to range considerably between 84 and 642 Mg C ha−1 (Keith et al., 2009). Carbon stocks in mature temperate forests can range from 100 to 800 Mg C ha−1 (Janisch and Harmon, 2002; Urrutia-Jalabert et al., 2015a and references therein) and can sometimes exceed 2,000 Mg C ha−1, such as in Sequoia sempervirens forests (Busing and Fujimori, 2005) and Eucalyptus regnans forests (including living plus dead aboveground biomass, Keith et al., 2009). In Chile, few studies have estimated forest carbon stocks. Total living and dead biomass carbon ranged between 310 and 470 Mg C ha−1 in Nothofagus-dominated old-growth Andean forests (Schlegel and Donoso, 2008). Total carbon stocks in an evergreen forest in the Chiloé Island reached between 286 and 384 Mg C ha−1 (Battles et al., 2002; Pérez-Quezada et al., 2015), and carbon stocks in broadleaved evergreen old-growth forests in Valdivia were estimated in 607 Mg C ha−1, including living, dead biomass, and carbon from the 30-cm topsoil (Gayoso, 2001). Old-growth millennial Fitzroya forests were reported to reach over 500 Mg C ha−1 of aboveground living biomass (Urrutia-Jalabert et al., 2015a), locating these forests' carbon stocks among the highest worldwide (Keith et al., 2009; Urrutia-Jalabert et al., 2015a).
Understanding the carbon dynamics of forests, particularly how carbon stocks vary in relation to land-use activities and forest disturbances, is recognized as a critical component for climate change mitigation (McMahon et al., 2010; Anderegg et al., 2020). In northwest Patagonia, forest landscapes are shaped by land use and disturbances influencing their composition, structure, and functional processes. Disturbances (such as fire, landslides, volcanism, or harvesting) play a significant role in the amount and distribution of carbon stocks and fluxes since they can release large amounts of carbon to the atmosphere (Keith et al., 2014; Thom and Seidl, 2016; Anderegg et al., 2020; Marcos-Martínez et al., 2022). However, key questions on the long-term influence of disturbance regimes mediated by physiographic location and environmental conditions (i.e., topography, climatic conditions, and soil fertility) on biomass and its allocation in living and dead biomass still remain largely unanswered (Pregitzer and Euskirchen, 2004; Hoover et al., 2012).
Despite progress in the quantification of carbon stocks in old-growth forests, few studies have been carried out in the long-lived Fitzroya cupressoides forests, which are the oldest closed-canopy forests in the world (Urrutia-Jalabert et al., 2015a). Disturbances in these forests are dominated by landslides and volcanism in the Andes and by human-induced or natural fires and logging both in the Coastal range and the Central depression (Lara, 1991; Fraver et al., 1999; Lara et al., 1999, 2003; Silla et al., 2002; Sibold et al., 2021). Following these types of disturbances, Fitzroya forests can accrue C from decades to millennia depending on the growth rate (net primary productivity) of the trees, site, and the frequency and intensity of natural or human-caused disturbances. Several authors have studied the change in the structure and composition of Fitzroya forest stands considering age, size (diameter), basal area, and vertical stratification since the last stand-devastating disturbance (Lara, 1991; Donoso et al., 1993; Veblen et al., 1995; Lara et al., 1999, 2003). However, there are no estimates of carbon stocks in Fitzroya stands according to their stage of development associated with the different disturbance regimes that characterize the physiographic units where they grow (Coastal range, Central depression, and Andean range). Moreover, there is no information on carbon allocated to live and dead pools in each of the studied conditions. Understanding the impact of forest disturbances on forest carbon sequestration from temperate ecosystems, which can contain trees older than 4,000 years (Lara et al., 2019), has the potential to provide unique information to address scientific issues that are globally relevant in the context of climate change (Luyssaert et al., 2008; Keith et al., 2009; Marcos-Martínez et al., 2022).
Within this context, the main goals of this study were (a) to assess the aboveground (living and dead) carbon stocks of Fitzroya forests according to their stand development stages in the three physiographic units where the species grows, and (b) to assess the rate of carbon stock accumulation at different stand ages, considering the contrasting environmental conditions and disturbance regimes that characterize the different physiographic units.
Our underlying hypothesis is that the physiographic distribution of Fitzroya and its associated disturbance regimes (type and frequency of disturbance) are fundamental factors in determining (or regulating) the amount and rate of C storage. In the Andes, volcanism would generate a slower accumulation of C, but disturbances are less frequent, and due to its great longevity and resistance to rotting, the species could accumulate a higher C content aboveground and as coarse woody debris (CWD). In the Coastal range and Central depression, trees have a higher growth rate and rapid accumulation of C (the central depression being more favorable than the coastal range), but the more frequent fires and logging would determine that stands rarely exceed 1,000 years of age, which would imply a lower C stock than in the Andean range.
Materials and methods
Study areas
For this study, Fitzroya cupressoides forests were selected to study in the Coastal and Andean ranges, and the Central depression of southern Chile (Figure 1). A brief overview of each of these study areas is presented in the following text.
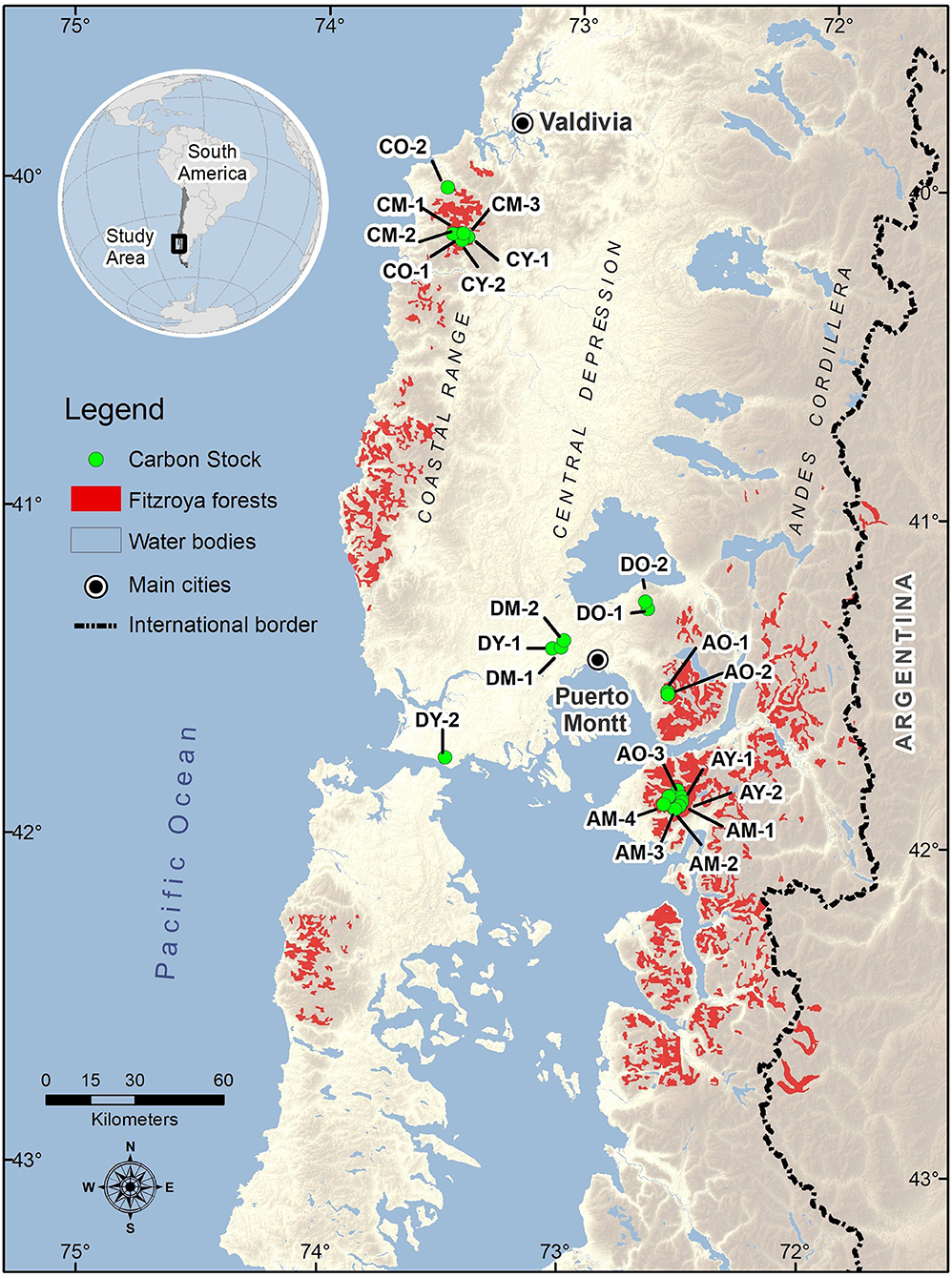
Figure 1. Study sites of Fitzroya cupressoides stands in the Coastal range, Central depression, and Andean range in south-central Chile. Stand codes and the main attributes for each stand are given in Supplementary Tables S1, S2. Map credit: Aldo Farías.
Coastal range
Fitzroya cupressoides forests grow in the Coastal range between 39° 50' and 42° 35'S from ca. 550–1,050 m a.s.l. This area is characterized by gentle slopes and flat plateaus of a metamorphic bedrock of Paleozoic to Precambrian age (Villagrán et al., 1993). The highest elevation corresponds to 1,048 m and soils, where Fitzroya grows, are generally sandy, nutrient-poor, very thin, acidic, poorly drained in winter, and with low water retention capacity (Lara et al., 1999; Urrutia-Jalabert et al., 2015a). This area experiences a temperate-type climate with a Mediterranean influence. The mean annual precipitation since 2010 is 3,770 mm, but annual values can reach up to 4,500 mm, with ca. 9% of this rainfall falling during summer (December-February; https://explorador.cr2.cl; Urrutia-Jalabert et al., 2015a, 2018a). Due to the harsh environmental conditions, Fitzroya mostly grows in pure stands or barely accompanied by Nothofagus nitida, Drimys winteri, Saxegothaea conspicua, and Weinmannia trichosperma (Donoso, 1993; Lara et al., 1999). In poorly drained areas, it grows with Pilgerodendron uviferum (Donoso, 1993). Fitzroya forests in this area have been historically affected by fires, which have occurred at least since the 1,300's (Lara et al., 1999; Sibold et al., 2021; Figure 2a). In addition to fires, logging has also been present, so the landscape is commonly characterized by second-growth Fitzroya forests and very few old-growth forests (Lara et al., 1999; Urrutia-Jalabert et al., 2015a). Regeneration in the area follows a catastrophic mode when disturbed by fires and logging and only occurs if parent trees remain and cattle grazing is absent (Veblen and Ashton, 1982).
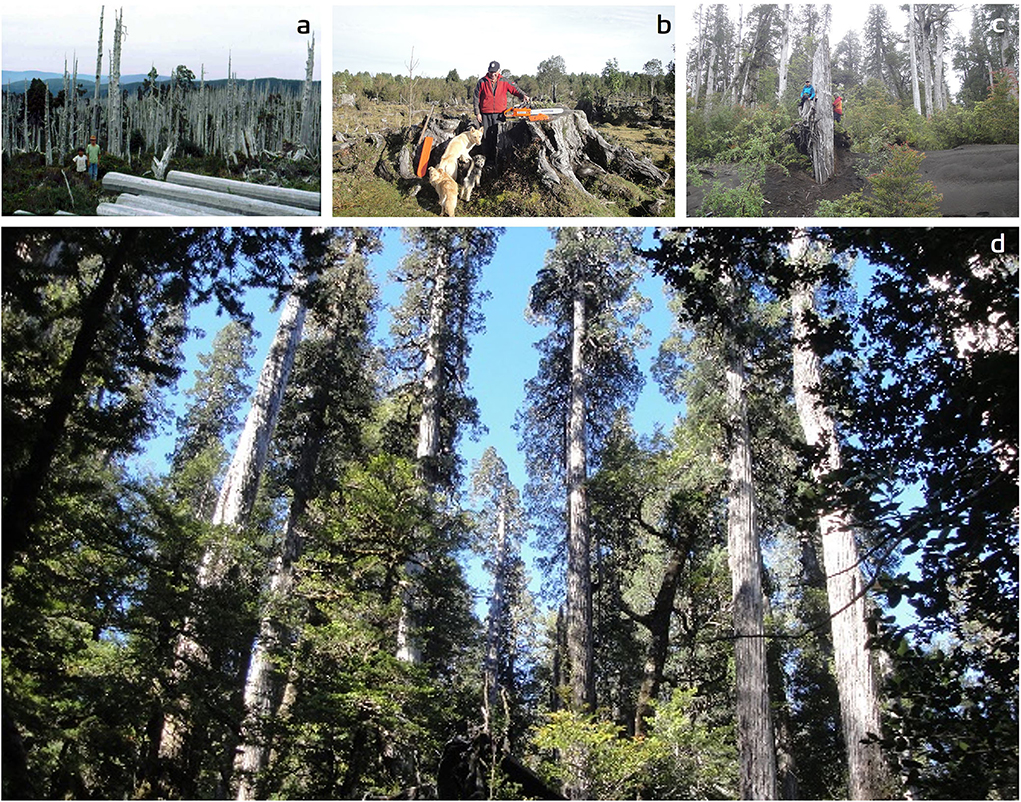
Figure 2. Main natural and anthropogenic disturbances that shape Fitzroya cupressoides forests in the study sites. (a) Burned and logged F. cupressoides forests in the Cordillera Pelada (Coastal range). (b) Old-growth F. cupressoides forests logged at the end of the 19th century in the Central depression. Large stumps still remain due to the highly resistant, low-decaying wood, although they are currently disappearing due to their use for firewood and the production of handicrafts by local people. (c) Large snag of F. cupressoides partially buried by tephra in the Andes range (known as well trees). Trees of this diameter are commonly found completely buried as evidence of the destruction of the previous Fitzroya forest by the eruption of the volcano Apagado. (d) Old-growth forest cohort established after volcan Apagado eruption (500 BC). Photo credits: (a) A. Lara; (b–d) M.E. González.
Central depression
Fitzroya forests in the central depression (at ~ 41° S) are only present as small and scarce fragments that were left after the widespread human-induced fires and logging to colonize the area in the 1850's (Fraver et al., 1999; Silla et al., 2002; Figure 2b). Here, Fitzroya grows in a flat and low-altitude area (40 to 400 m a.s.l) that was part of the central valley that later sank due to geological upheavals (Seno de Reloncaví). Climate is temperate with oceanic influence, and mean annual precipitation reaches 1,783 mm, with 15% of it falling during summer (Lara et al., 2008; Urrutia-Jalabert et al., 2018a). Soils in the area are locally known as “ñadis,” and they are silty to clay loams, shallow, and acidic, with a high organic matter content and have developed from volcanic ashes laid on top of a fluvioglacial stratum that is relatively impermeable (Tosso, 1985; Lara et al., 2008). Each of the Fitzroya remnant patches are 0.8–3 ha, and they are isolated from each other (Fraver et al., 1999). Most forest patches are relatively young (<100 years old), although they can reach up to 200–300 years old in a couple of sites (Silla et al., 2002). Regeneration in this area also follows a catastrophic mode associated mainly with fires and logging (Silla et al., 2002).
Andes cordillera
Fitzroya grows in the Andean range between 41° and 43° 'S in mountainous sites from ca. 500 to 1,200 m a.s.l (Veblen et al., 1995). Climate is characterized by very high annual precipitation, ranging from 4,000 to more than 6,000 mm, with a reduced Mediterranean influence and a shorter growing season than the Coastal range (Donoso et al., 1990; Urrutia-Jalabert et al., 2015a). The Andes was extensively glaciated during the Pleistocene, and soils are mostly formed from recent andesitic volcanic deposits (Veblen et al., 1976). Thus, soils are mostly volcanic and acidic, with a high organic matter content and a high C/N ratio (Peralta et al., 1982). Trees in this area generally reach a larger size than those in the Coastal range and Central depression mainly because forests are less frequently affected by disturbances, and due to their remote location, human impacts have been less ubiquitous. Thus, it is more common to find old-growth Fitzroya stands, with large trees, which have mostly developed after devastating disturbances, mainly volcanic eruptions and landslides (Lara, 1991; Veblen et al., 2005; Figures 2c,d). Fitzroya mostly grows with evergreen broadleaved species, such as Nothofagus nitida, Nothofagus betuloides, Laureliopsis philippiana, and Amomyrtus luma, as well as with evergreen conifers like Podocarpus nubigenus and Saxegothaea conspicua (Donoso et al., 1990, 2006). It has been reported that some forests in this area can be an example of the “additive basal area” phenomenon, due to the high basal area of Fitzroya plus its long-lived characteristic and emergent status, exerting low light competition on the remaining evergreen species (Urrutia-Jalabert et al., 2015a). Regeneration of the species in this area mostly occurs after large natural disturbances but can also occur in large gaps on top of fallen logs (Donoso et al., 1993).
Selecting and sampling Fitzroya forest stands
Stand selection
Carbon cycling and storage mostly depend on time since disturbance (i.e., forest age) (Chapin and Mooney, 2002). To understand forest dynamics and development patterns (change in structure and composition over time), one of the most widely used approaches is the substitution of space for time (Pickett, 1989), also known as chronosequence (Walker et al., 2010). Thus, we used a chronosequence approach for site selection to understand age-dependent trends of carbon stocks (Walker et al., 2010). This approach involves identifying and arranging the forest patches in different stages of development after stand-replacing disturbances. This approach, assumed as a successional sequence, has limitations because of the assumptions of similar environments, regeneration processes (i.e., soils, microclimate, and availability of propagules), and especially the stand disturbance history (e.g., type and severity of disturbance) that gave rise to the stand. Recognizing these limitations, in the stand selection we used our experience and knowledge about disturbance ecology and forest dynamics of these forest ecosystems throughout their geographic range (Lara, 1991; Donoso et al., 1993; Fraver et al., 1999; Lara et al., 1999; Silla et al., 2002; Urrutia, 2002; Urrutia-Jalabert et al., 2015a). However, there were two cases where the selection presented difficulties. In the Coastal range, given the extensive logging and past fires, there were difficulties finding old-growth stands, and one of the selected stand (stand CO-2) is located in a more protected, low-elevation waterlogged flat site, comparatively different from the environmental conditions of the other stands sampled in this range. There was a similar problem finding old-growth Fitzroya cupressoides stands in the Central depression since all of them were logged or cleared for pasturelands in the late 1800's (Fraver et al., 1999). Therefore, the forest structure and carbon stock had to be reconstructed from the cut stumps using volume functions developed for Fitzroya growing in the Coastal range.
For stand selection, we scouted areas in the Coastal range, Central depression, and Andean range. From our previous experience in the study of Fitzroya forests, we observed a series of attributes of these stands in order to estimate their degree of development after massive disturbance. These attributes were mean and maximum diameter at breast height (dbh), mean and maximum height, tree density, vertical structure (i.e., mono-tier and multi-tier), and presence of snags and coarse woody debris. According to these attributes, three age categories were considered for stand classification: young, mature, and old-growth. In each selected stand, we considered the following components of biomass: living standing trees, dead standing trees (snags), and logs from dead trees laying on the forest floor (coarse woody debris). For the young and mature development stages, we established between two and four 0.1- and 0.2-ha plots, respectively, in each physiographic unit (total = 15; Supplementary Table S1). For the old-growth stage, we established two or three 0.3-ha permanent plots in each physiographic site (total = 7). Considering the absence of old-growth Fitzroya forests in the central depression, we reconstructed the original forest structure (density, basal area, volume, and biomass) from the stumps left after fire and logging, which, in many sites, still remain (Silla et al., 2002; Lara et al., 2003). The reconstruction considered only Fitzroya trees since the remains of the other tree species were not present, probably due to rotting.
Stand age
Trees in each plot were cored near the base and up to the tree center, if possible, to determine the age of cohorts (Kitzberger et al., 2000). When the trees had rotten sections, we took up to three cores for a better estimation of their age. For large trees, we used 28-inch (71 cm)-long increment borers, but these were, in some cases, not enough to reach the tree centers (or when tree centers were rotten). In such cases, only a minimum age was determined. The age of the old-growth logged stands in the Central depression could not be determined due to the high degree of decay of the center of the large stumps (200–280 cm in diameter). Based on the determined stand ages, we reconfirmed our classification of Fitzroya post-disturbance stands in the three different stages of development (Franklin et al., 2018): young forest stage (YFS; mean stand age of the main cohort ≤ 200 yrs old), mature forest stage (MFS; > 200 but ≤ 800 yrs old), and old-growth forest stage (OGF; > 800 yrs old) (Supplementary Tables S1, S2).
Inventory of carbon stocks in Fitzroya forests
In each plot representing distinct stages of forest development, all living trees ≥ 5 cm of dbh in the YFS (≥10 cm dbh for MFS and OGF) were censused to estimate tree density, basal area, and biomass of living trees. For live trees and snags, the stem volume of Fitzroya was estimated using volume functions for individual trees developed by Urrutia-Jalabert et al. (2015a) for the Coastal and Andean ranges. The function developed for the Coastal range was used for the Central depression since the diameter–height relationships are similar between the stands in both physiographic areas. The conversion of volume to biomass was made using wood basic density and a biomass expansion factor developed by Urrutia-Jalabert et al. (2015a). For other species, available biomass equations including stem and branches were used (Gayoso et al., 2002). Dry biomass of living trees were converted to C using the standard coefficient 0.4964 for Chilean temperate species (Gayoso and Guerra, 2005; Urrutia-Jalabert et al., 2015a) and the same was done for snags and CWD. Coarse woody debris (CWD) was inventoried in each plot based on the methodology developed by Stewart and Burrows (1994). We measured all logs on the forest floor with a stem diameter ≥ 20 cm and a length ≥ 1 m. For each log or branch on the forest floor within the limits of the plot, we recorded total length (m) and diameters (cm) at large and small ends. Snags and stumps, with a diameter at the base ≥ 20 cm and heights ≥ 1 m and ≥ 0.2, respectively, were measured. The structural integrity of each log and snag was determined using the stage of decay classification adapted from Stewart and Burrows (1994) (Supplementary Table S3). Dry biomass was obtained by multiplying the moist volume of each piece by the wood-specific basic density (g/cm3). Wood density for the various classes of decay in fallen logs and snags was determined in the Wood Products Laboratory at Universidad Austral de Chile (Supplementary Table S4).
Data analyses
To assess the effect of development stage and physiographic area (and their interaction) on total biomass stocks, we performed a two-way ANOVA. Homogeneity of variance and normality of residuals were assessed using Levene's test and the Shapiro–Wilk test, respectively. Tukey's honestly significant difference test was used for the post hoc effects of significant factors. These analyses were performed in R (R Development Core Team, 2014).
To estimate the rate of carbon accumulation across the different physiographic units and in each one separately, linear regressions were performed between C stocks and stand age. The maximum age of any tree in the plot belonging to the cohort was used.
Results
Carbon stocks in different pools by forest development stages in each physiographic region
Living biomass
As expected, living carbon stocks increased through more advanced stages of Fitzroya forest development in the Coastal, Central depression, and Andean physiographic regions. Living C in young, mature, and old-growth forest stages ranged, in average, between 15 and 59 Mg C ha−1, 146 and 192 Mg C ha−1, and 243 and 424 Mg C ha−1, respectively (Figure 3, Table 1, Supplementary Table S2). Carbon stocks in living biomass represented, in average, 98, 90, and 90.2% of the total C content for young, mature, and old-growth stage developments across the physiographic regions. Living carbon stocks in OGFs in the Andean range were 43% higher than C stored in OGFs in the Coastal range, and 22% higher than the OGFs in the Central depression.
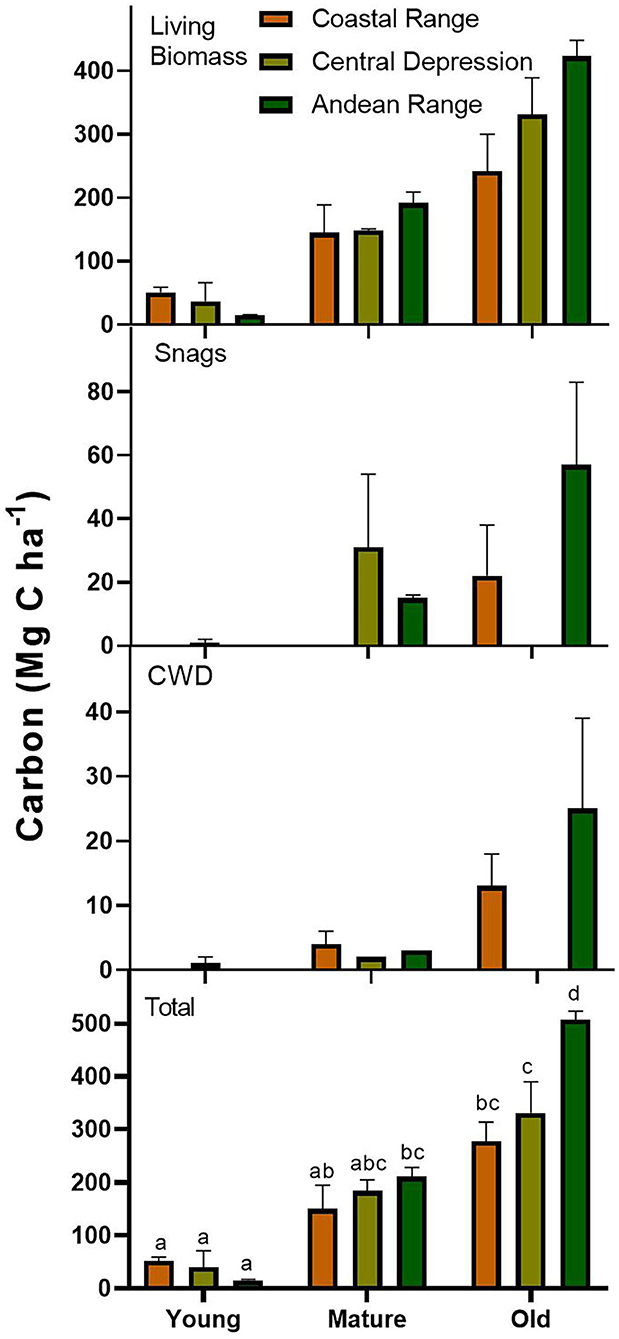
Figure 3. Carbon stocks (Mg C ha−1) in the different pools of living biomass, snags, and coarse woody debris (CWD). Total carbon aboveground is also shown for young, mature, and old-growth forest development stages across the Coastal range, Central depression, and Andean range. Bars indicate standard errors and letters in the bottom panel indicate significant differences in total C stocks among physiographic units and stages of development.
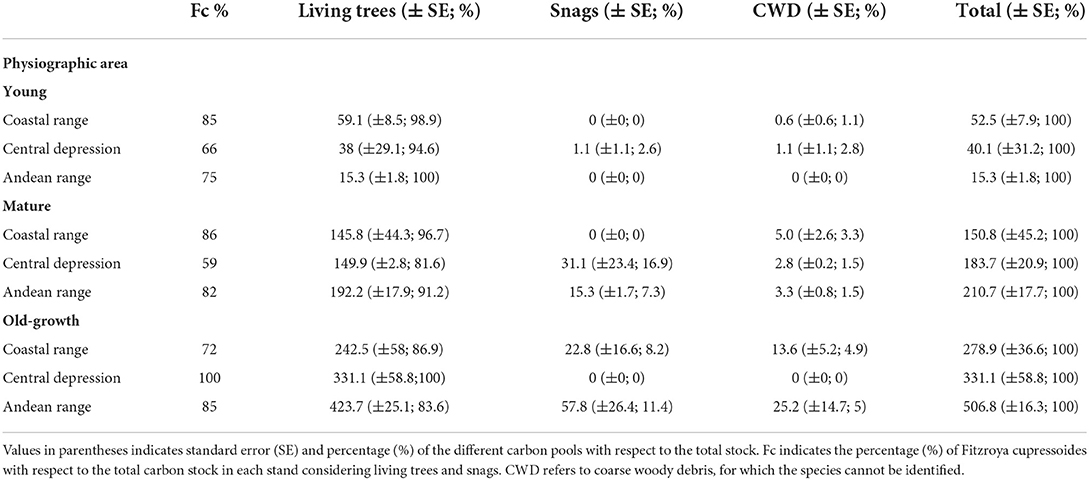
Table 1. Mean carbon stocks for the different physiographic units, stand development stages, and carbon pools (Mg C ha−1).
Snags
The pool size of snags in YFSs was relatively small or absent in all physiographic regions. In the Central depression, just one site in the YFSs had snags with a C stock of 2.1 Mg C ha−1 (Supplementary Table S2). The average C content for MFSs was 15.3 and 31.1 Mg C ha−1 in the Andean range and Central depression, respectively, and the snag C pool was inexistent in the Coastal range (Figure 3, Table 1). In the case of OGF, the average C content was 22.8 and 58.8 Mg C ha−1 for the Coastal and Andean ranges, respectively (Figure 3, Table 1). Because carbon stocks in the OGFs of the Central depression were reconstructed from stumps in forest stands that were logged 100–150 years ago, there were no snags, CWD, or stumps from species other than Fitzroya. Carbon stocks of snags represented 1.0, 8.5, and 10.3% of the total C content in young, mature, and old-growth forests, as averages that include the different physiographic regions (estimated from figures in Table 1). OGFs in the Central depression were not considered.
CWD
The pool size of CWD across all forest stages was relatively small, except for the old-growth Fitzroya stage in the Coastal and Andean ranges, for which the C content of CWD reached, in average, 13.6 and 25.2 Mg C ha−1, respectively (Figure 3, Table 1). Carbon stocks of CWD represented 1.6, 2.0, and 4.9% of the total C content in young, mature, and old-growth forests, as averages that include the different physiographic regions (estimated from figures in Table 1). OGFs in the Central depression were not considered.
Total aboveground carbon stocks
Total C increased toward more advanced stages of forest development in all physiographic regions, following a similar pattern described for living biomass. Total C in OGFs reached, in average, 279, 331, and 507 Mg C ha−1 at the Coastal range, Central depression, and Andean range, respectively. Fitzroya contributed, in average, between 82 and 83% of the total carbon stock in the Coastal and Andean ranges, respectively, and 63% in the Central depression; the latter did not include the OGF in the calculation (Table 1). The remainder corresponded mainly to evergreen Nothofagus species. Mean total carbon stocks in OGFs of the Andes range were 45% higher than C stored in OGFs of the Coastal range, and 35% higher than the OGFs of the central depression (Table 1).
According to the two-way ANOVA, stage of development (p < 0.001), physiographic unit (p < 0.01), and the interaction between them were significant (p = 0.01). Residuals were normally distributed (W = 0.97, p = 0.68), and the variance of the model was homogeneous (p = 0.66). The total C stock of the Andean OGF was significantly different (higher) to all the other situations, and the total C stock of the Central depression OGF was similar to that in the Coastal range and to the mature stands from the Andes and Central depression (Figure 3).
Carbon stock accumulation for each of the physiographic areas through time
A strong relationship between stand age and total carbon was found for all stands pooled together (R2 = 0.81 N = 20, Figure 4A). A strong relationship between stand age and total carbon was also found for each of the physiographic units (R2 ranged between 0.70 (N = 6) for the Coastal range and 0.95 (N = 5) for the Central depression (Figure 4B).
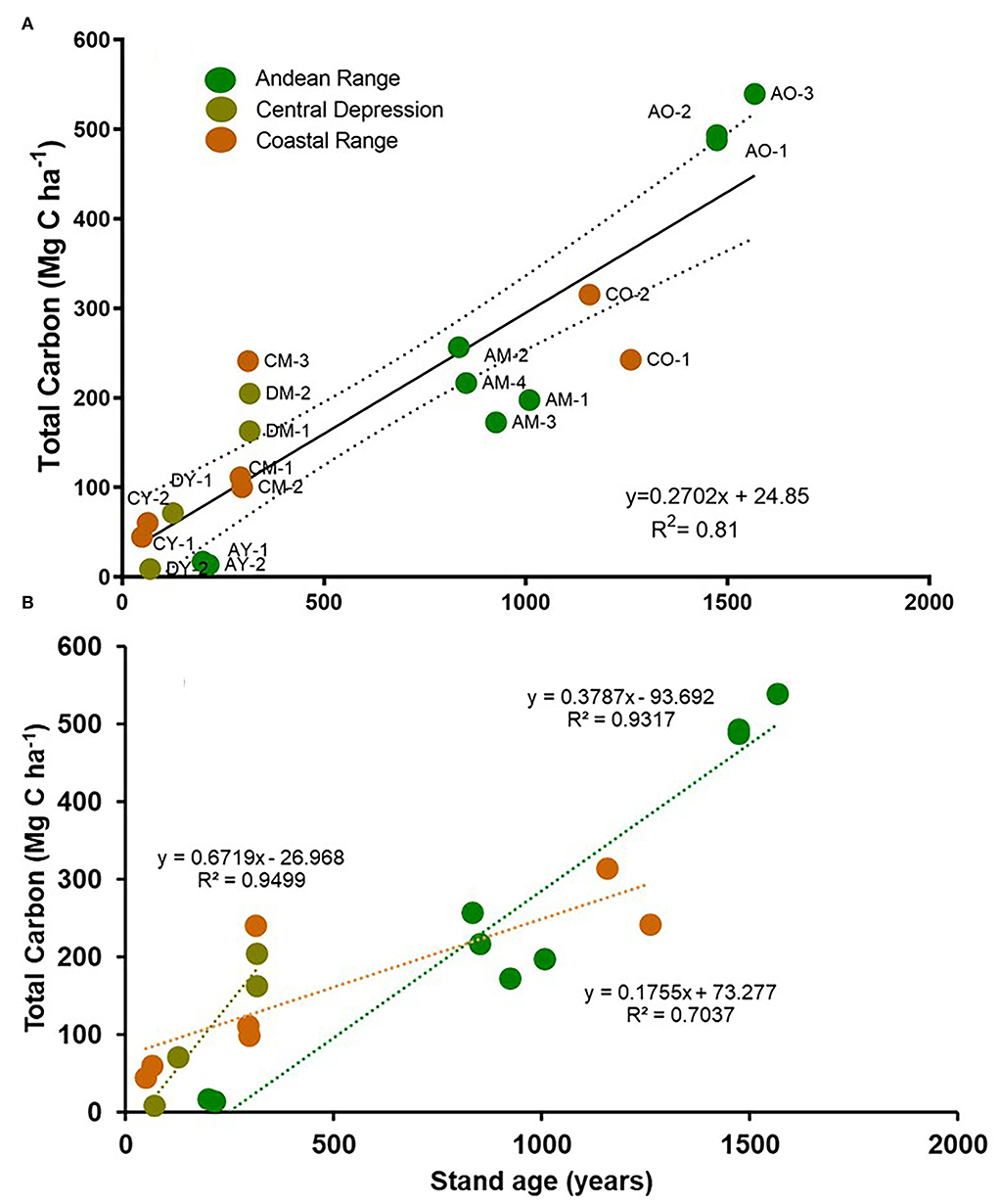
Figure 4. Total carbon stocks related to stand age for (A) all Fitzroya stands studied in the three physiographic units. Each dot represents a plot. Stand age was determined from the maximum age of any tree in the plot belonging to the cohort. Dotted lines represent the confidence interval (P < 0.05); (B) Fitzroya stands from each physiographic unit, separately. Regression dotted lines are indicated as follows: Coastal range (orange), Central depression (dark green), and Andean range (light green). Old-growth stands in the central depression are not included neither in (A) or (B) since their maximum age could not be determined due to the hollow rotten center of the large stumps (200–280 cm in diameter).
Discussion
Carbon accumulation by forests essentially depends on the growth rate of dominant trees and environmental conditions. Disturbance regimes and land-use practices also play a significant role in regulating the potential carbon storage. In this study, we documented that C stocks and C accumulation rates of Fitzroya cupressoides forests highly depend on the environmental conditions given by the physiographic units where these forests grow, their associated disturbance regimes, and the successional stage indicated by stand age.
Carbon stock variation along forest development stages in the three physiographic regions
Andean old-growth Fitzroya forests reached a higher C stock than OGFs growing in the Coastal range and Central depression. The higher biomass C content in Andean OGFs would be mainly the result of the low-recurrence disturbance regime and the particular mechanisms associated with it (i.e., heat and burial; Swanson and Major, 2005), which play a distinguishing role in determining the distribution of carbon in the different pools. At high elevations in the Andean range (800–1,200 m), large-scale disturbances such as the deposition of volcanic tephra and landslides are important stand-initiating disturbances. Given the catastrophic regeneration mode (sensu Veblen, 1992), this pioneer species can be the first to colonize sites devasted by volcanic ash deposition. Fitzroya regeneration and stand development have advantages in these harsh sites compared to other species, such as Nothofagus betuloides or Nothofagus nitida, forming stands that are highly dominated by Fitzroya (Lara, 1991; Donoso et al., 2006). Depending on soil fertility, age and size structures of Fitzroya are typically bell-shaped, indicating, in most cases, a single post-disturbance cohort (Lara, 1991).
On the other hand, human-caused or natural fires are the main stand-initiating disturbance in the Coastal range and Central depression. Several studies have demonstrated the ability of Fitzroya to colonize relatively rapidly these burned sites as long as they have not been burned again or affected by cattle browsing (Veblen and Ashton, 1982). Fires have been a prevalent type of disturbance during the past 1,000 years, and anthropogenic fires, associated largely with Fitzroya logging, have mainly occurred since the 1700's (Molina, 1995; Lara et al., 1999; Sibold et al., 2021). Therefore, the large C stock in the living biomass of forests from the Andean range can be explained by the greater development period (centuries and millennia) before being affected by a stand-replacing disturbance again.
In all sites, C stored in snags and CWD was very low or inexistent in the early development stage (YFS). In the Andean range, the YFSs selected were located 0.5–1 km from the center of the cinder cone of the Apagado volcano, being heavily impacted by the eruption that was radiocarbon dated at 2.6 calibrated (cal) ka BP (Watt et al., 2011). This eruption wiped out the forest cover and buried all biological legacies and soil, following similar patterns to other severe volcanic eruptions (e.g., Franklin et al., 1985; Dale et al., 2005; Swanson et al., 2005). In the case of the Coastal range and Central depression, the null or low C stored in snags and CWD in the young development stage (YFS) could also be influenced by the forest logging that has occurred since the 1700's (Molina, 1995; Fraver et al., 1999). This logging takes place even today through the extraction of Fitzroya wood from snags and well-preserved CWD. The increase in the snag and CWD pool size over time is the result of the stand's own input. Legacies of dead standing or fallen trees are the result of natural mortality of different tree species. Highly durable Fitzroya snags may persist for several centuries (Urrutia-Jalabert et al., 2015a) or up to 4,000–5,000 years when buried by volcanic tephra layers up to 4.5 meters in depth. These are so well preserved that have been used in a dendrochronological study for temperature reconstruction (Lara et al., 2020). Old-growth Andean stands can actually reach a very long wood residence time for living trees, the highest documented worldwide (>1,300 years; Urrutia-Jalabert et al., 2015a). Values of dead biomass found in the Andean old-growth stands are within the values reported for broadleaved evergreen old-growth stands from the Chiloé Island (90 and 190 Mg C ha−1, Carmona et al., 2002).
Carbon stock buildup for each of the physiographic areas through time
In this study, we document that carbon stocks in living trees increased, in average, by 4.6 times from the young to the old-growth stage in the Coastal range, by 8.7 times in the Central depression, and by 28.5 times in the Andean range (calculated from data provided in Table 1). The first two figures are similar to those reported by Badalamenti et al. (2019) for Mediterranean ecosystems in Europe (carbon increased from 20 to 40 Mg C ha−1 in a high maquis to 209 Mg C ha−1 in an old-growth forest, along a 100-year secondary succession). The outstanding ratio for the Andean range may be explained by the change from very open low-stocked stands established in a harsh environment to highly stocked old-growth stands (with large trees) over a period of more than 1,500 years (Figure 3).
Total carbon stocks and carbon accumulation rates may be explained by different combinations of site conditions, disturbance regimes, and stand development stages as indicated by tree age. In all the studied stands, which are single-cohort, maximum age represents the time since carbon accumulation started. In the case of old-growth stands, this does not correspond to the maximum age because Fitzroya may reach massive sizes (dbh ranging from 200 and 250 cm), so age determination using 60- to 70-cm-long increment borers does not get to the pith or near it.
Considering the relationship between stand age and total carbon stock for all stands pooled together (R2 = 0,81 N = 20, Figure 4A), the main factor that could explain this pattern is the disturbance origin of the studied stands that have developed as a single cohort highly dominated by Fitzroya, with a relatively even-aged structure.
When relating total carbon with stand age in each physiographic unit separately, we found a strong relationship too, with differences in the slope and strength of it. Maximum stand age in the Coastal range reached 1,260 years, and the maximum carbon stock was 242–315 Mg C ha−1 compared to 1,567 years and 487–539 Mg C ha−1 for the Andean range (Figure 4B, Supplementary Table S2). The overall differences between the Andean and the Coastal ranges may be explained by the repeated fires and their shorter mean return period compared to single catastrophic disturbances that originated the stands studied in the Andean range (Lara, 1991; Lara et al., 1999; Urrutia-Jalabert et al., 2015a).
Regarding the different mean rates of carbon accumulation estimated from the slope of the regressions between carbon stocks and stand age, the fastest mean rate occurred in the Central depression (0.67 Mg C ha−1 year−1), followed by the Andean and the Coastal ranges (0.38 and 0.18 Mg C ha−1 year−1, respectively; Figure 4). Nevertheless, the Central depression estimate has the limitation that it is only based on the young and mature stands. The high carbon accumulation rate in this site may be associated with the fast mean radial growth rate, 0.4–0.8 mm/year, compared to 0.2–0.5 for the Coastal range and <0.1–0.3 mm/year for the Andean range (data not shown). This higher growth rate may be explained by the long growing season (mild temperatures) and high water availability throughout the year due to the highly organic soils, which remain moist most of the year in these flat central depression sites (Lara et al., 2008; Urrutia-Jalabert et al., 2018a).
The carbon accumulation rate in the Andean range may be explained by a very low carbon stock in the young stands associated with the slow establishment and growth rate of Fitzroya trees in very incipient soils developed from tephra on relatively steep slopes (Lara, 1991). As soil develops, the growth rate increases, and under an even precipitation distribution along the year, carbon stock increases, as indicated by the high values in mature stands (mainly contained in living trees, Figure 3, Table 1). In old-growth stands, soil continues to develop (Urrutia-Jalabert et al., 2015a), and given the old age of the stands (minimum 1,473–1,557 years), snags and CWD mainly from other species become important carbon pools, while the living trees continue to accumulate carbon (Figure 3, Table 1). It is important to mention that given the underestimation of the maximum age of Fitzroya trees in old-growth stands, the slope of the curve in the Andean range may be overestimated.
The Coastal range has the lowest slope in the regression between carbon stocks and stand age, indicating the slowest rate of carbon accumulation among the three physiographic units (Figure 4). This is somewhat surprising, given the higher radial growth rates of trees in the Coastal range than in the Andes (Urrutia-Jalabert et al., 2015b). The lower maximum height of trees (20 m in the coastal range compared to 38–40 m in the Andes, data not shown) determines a lower volume (and therefore lower biomass and carbon stocks for a given dbh of an individual tree). The volume functions that were used in this study (Urrutia-Jalabert et al., 2015a) indicate that the volume for a 40-cm-dbh tree in the Andes is 7.1% higher than that in the coastal range, and this difference increases to 17.9 % and 25% for a 60- and an 80-cm-dbh tree, respectively (not shown). This determines that despite higher radial growth in the Coastal range, there is a lower increase in biomass and carbon accumulation along stand age, compared to the Andean range. Total height at a given age is a typical indicator of site quality. Soils are more restrictive in the Coastal range, especially because they are highly lixiviated metamorphic soils, characterized for being shallow and waterlogged during winter, with a low water retention capacity and very low fertility and toxic aluminum levels, compared with the volcanic soils in the Andes (Urrutia-Jalabert et al., 2015a).
Final remarks on Fitzroya forests conservation
Due to the disturbance history that characterizes the Coastal range, it is more difficult for large Fitzroya trees to dominate the forested landscape, being more common to find mixed species stands in which there are a few number of large and old Fitzroya trees (e.g., CO-1 stand). In fact, the structure of stand CO-2 is very difficult to find in the Coastal range since most of large Fitzroya trees were cut or burned in the past. It has been reported that large-diameter trees store disproportionally large amounts of carbon (Mildrexler et al., 2020), so the repeated history of cuttings and fires has probably prevented forests to reach higher amounts of carbon stocks in the Coastal range. The largest carbon stocks of Fitzroya forests found in the Andean range can be also associated with the better conservation status of these forests due to their inaccessibility. Particularly, C stocks would have been even higher if trees buried by tephra (e.g., well trees) had been measured. Larger carbon stocks in Fitzroya forests could be achieved in the Coastal range and Central depression if illegal cuttings and human-caused fires were definitely stopped. Another recent threat for Fitzroya forests is urban sprawl, real estate expansion in conglomerates of 0.5-ha parcels (the minimum subdivision in rural areas permitted by law in Chile), and the development of associated infrastructure (e.g., roads and power lines) in the Central depression where human population and their activities are concentrated. This threat is affecting areas of young isolated Fitzroya stands, reducing the potential of recovery of these ecosystems and restoration actions.
In many parts of the world, forest disturbances have been intensified, and climate change is expected to amplify this trend, compromising profoundly forest carbon stocks and sinks in the future (Seidl et al., 2017). For instance, in recent years, forest landscapes in south-central Chile have experienced the largest wildfires of the past 50 years associated with dry and warm climate conditions (Bowman et al., 2018; González et al., 2018, 2020; Urrutia-Jalabert et al., 2018b; Garreaud et al., 2020). In this region, 99% of the fires are caused by humans (Pozo et al., 2022), and the risk of fires is increasing with climate change (Veblen et al., 2011; Urrutia-Jalabert et al., 2018b; IPCC, 2022), so stronger prevention and control programs for wildfires are needed.
Climate-induced forest dieback and reduced growth are also important threats to the conservation of Fitzroya cupressoides forests. Although this long-lived species seems to be somewhat resistant to water scarcity, warmer and drier conditions negatively affect its growth, and trees from the coastal range are actually decreasing their growth rates (Urrutia-Jalabert et al., 2015b, 2020; Lara et al., 2020). Moreover, resistance of Fitzroya to drought seems to be mediated by age and location, being young and adult trees less tolerant to drought in the Central depression than adult trees, but not young trees in the Coastal range (Urrutia-Jalabert et al., 2018a). The extent to which the species growing in different sites will be affected by climate change is still largely unknown and requires to be further investigated.
Long-lived Fitzroya cupressoides forests are unique and globally significant in terms of their ecological, environmental, and cultural values. These ecosystems are key not only for providing critical ecosystem services and biodiversity but also as sentinels of climate change and carbon sinks. Although Fitzroya is a threatened species protected by law, the sustained fragmentation and deforestation of these forests during recent decades, especially in the Central depression, demand an increasing effort to protect and restore these ecosystems.
Data availability statement
The raw data supporting the conclusions of this article will be made available by the authors upon request.
Author contributions
MG, AL, RU-J, and JA: conception and design of the study. MG, AL, JA, RU-J, AB-S, and CR-G: data collection. All authors: data analysis and writing of the manuscript. All authors contributed to the article and approved the submitted version.
Funding
All authors acknowledge support from ANID/FONDAP/15110009. MG, JA, and AL acknowledge support from FONDECYT (Grant 1130410) and the Center for Fire and Resilience of Socio Ecosystems (FireSES, Universidad Austral de Chile). AL and RU-J appreciate FONDECYT grant 1171496. RU-J appreciates FONDECYT Initiation Grant 11200710. AB-S acknowledges the support from the Graduate School of the Faculty of Forestry and Natural Resources of Universidad Austral de Chile, and the National Agency for Research and Development (ANID/National Ph.D. Grant/No 21140737). JA acknowledges support from ANID/BASAL FB210018.
Acknowledgments
We thank Corporación Nacional Forestal (CONAF) for granting permission to extract tree ring cores from the endangered Fitzroya cupressoides in the Alerce Costero National Park, Monumento Natural Lahuen Ñadi, and Alerce Andino National Park, as well as in private properties, and also for their long-term collaboration with our research and conservation efforts of Fitzroya forests. We thank numerous research assistants at Universidad Austral de Chile in the Dendrochronology and Global Change Laboratory for their help during fieldwork and sample analysis: E. Cuq, T. Cayul, A. Bahamondes, O. Concha, D. Aliste, and C. Rodríguez. We thank Aldo Farías for the preparation of Figure 1. Our gratitude to the private owners who gently allowed working in their Fitzroya forests: G. Vargas, A. Nuñez, E. Santibañez, and J. T. Elton, the Nature Conservancy.
Conflict of interest
The authors declare that the research was conducted in the absence of any commercial or financial relationships that could be construed as a potential conflict of interest.
Publisher's note
All claims expressed in this article are solely those of the authors and do not necessarily represent those of their affiliated organizations, or those of the publisher, the editors and the reviewers. Any product that may be evaluated in this article, or claim that may be made by its manufacturer, is not guaranteed or endorsed by the publisher.
Supplementary material
The Supplementary Material for this article can be found online at: https://www.frontiersin.org/articles/10.3389/ffgc.2022.960429/full#supplementary-material
References
Anderegg, W. R. L., Trugman, A. T., Badgley, G., Anderson, C. M., Bartuska, A., Ciais, P., et al. (2020). Climate-driven risks to the climate mitigation potential of forests. Science 368, eaaz7005 doi: 10.1126/science.aaz7005
Badalamenti, E., Battipaglia, G., Gristina, L., Novara, A., Rühl, J., Sala, G., et al. (2019). Carbon stock increases up to old growth forest along a secondary succession in Mediterranean island ecosystems. PLoS ONE. 14, e0220194. doi: 10.1371/journal.pone.0220194
Battles, J. J., Armesto, J. J., Vann, D. R., Zarin, D. J., Aravena, J C., Perez, C., et al. (2002). Vegetation composition, structure, and biomass of two unpolluted watersheds in the Cordillera de Piuchué, Chiloé Island, Chile. Plant Ecol. 158, 5–19. doi: 10.1023/A:1014741821292
Bowman, D., Moreira-Muñoz, A., Kolden, C., Chávez, R., Muñoz, A., Salinas, F., et al. (2018). Human–environmental drivers and impacts of the globally extreme 2017 Chilean fires. Ambio. 48, 350–362. doi: 10.1007/s13280-018-1084-1
Busing, R. T., and Fujimori, T. (2005). Biomass, production and woody detritus in an old coast redwood (Sequoia sempervirens) forest. Plant Ecol. 177, 177–188. doi: 10.1007/s11258-005-2322-8
Campioli, M. Y., Malhi, S., Vicca, S., Luyssaert, D., Papale, J., Peñuelas, M., et al. (2016). Evaluating the convergence between eddy-covariance and biometric methods for assessing carbon budgets of forests, Nat. Commun. 7 13717. doi: 10.1038/ncomms13717
Carmona, M. R., Armesto, J., Aravena, J. C., and Pérez, C. A. (2002). Coarse woody debris biomass in successional and primary temperate forests in Chiloe' Island, Chile. For. Ecol. Manage. 164, 265–275. doi: 10.1016/S0378-1127(01)00602-8
Chapin, F. S. III., Matson, P. A., and Mooney, H. A. (2002). Principles of Terrestrial Ecosystem Ecology. New York: Springer-Verlag.
Dale, V. H., Swanson, F. J., and Crisafulli, C. M. (2005). Ecological Responses of the 1980 Eruption of Mount St. Helens. New York: Springer Science. 342.
Donoso, C. (1993). Bosques Templados de Chile y Argentina. Variación, Estructura y Dinámica. Santiago, Chile: Editorial Universitaria, 484.
Donoso, C., Grez, R., and Sandoval, V. (1990). Caracterización del tipo forestal alerce. Bosque. 11, 21–34. doi: 10.4206/bosque.1990.v11n1-03
Donoso, C., Lara, A., Escobar, B., Premoli, A., and Souto, C. (2006). “Fitzroya cupressoides (Molina) I. M Johnst.” in Las Especies Arbóreas de los Bosques Templados de Chile y Argentina, eds Donoso, C,. Maria Cuneo Ediciones, (Valdivia), 68–81.
Donoso, C., Sandoval, V., Grez, R., and Rodríguez, J. (1993). Dynamics of Fitzroya cupressoides forests in southern Chile. J. Veg. Sci. 4, 303–312. doi: 10.2307/3235588
Duque, A., Peña, M. A., Cuesta, F., González-Caro, S., Kennedy, P., Phillips, O. L., et al. (2021). Mature Andean forests as globally important carbon sinks and future carbon refuges. Nat. Commun. 12, 2138. doi: 10.1038/s41467-021-23955-7
Franklin, J. F., Johnson, K. N., and Johnson, D. L. (2018). Ecological Forest Management (Long Grove, IL: Waveland Press, Inc.), 646.
Franklin, J. F., MacMahon, J. A., Swanson, F. J., et al. (1985). Ecosystem responses to the eruption of Mount St. Helens. Natl. Geogr. Res. 1, 198–216.
Fraver, S., González, M. E., Silla, F., and Lara, A. (1999). Composition and structure of remnant Fitzroya cupressoides forests of southern Chile's Central Depression. J. Torr. Bot. Soc. 126, 49–57. doi: 10.2307/2997254
Garreaud, R. D., Boisier, J. P., Rondanelli, R., Montecinos, A., Sepúlveda, H. H., and Veloso-Aguila, D. (2020). The central Chile mega drought (2010–2018): a climate dynamics perspective. Int. J. Climatol. 2019, 1–19. doi: 10.1002/joc.6219
Gayoso, A. J., and Guerra, C. J. (2005). Contenido de carbono en la biomasa aérea de bosques nativos en Chile. Bosque. 26, 33–38. doi: 10.4067/S0717-92002005000200005
Gayoso, J. (2001). Medición de la capacidad de captura de carbono en bosques nativos y plantaciones de Chile. Rev. For. Iberoam. 1, 1–13. Available online at: http://www.forest.ula.ve/rforibam/archivos/DOC1.pdf
Gayoso, J., Guerra, J., and Alarcón, D. (2002). Contenido de carbono y funciones de biomasa en especies nativas y exóticas. Documento N°1. Proyecto FONDEF Medición de la capacidad de captura de carbono en bosques de Chile y promoción en el mercado mundial (Valdivia: Universidad Austral de Chile e Instituto Forestal), 53.
González, M. E., Gómez-González, S., Lara, A., Garreaud, R., and Díaz-Hormazábal, I. (2018). The 2010-2015 Megadrought and its influence on the fire regime in central and south-central Chile. Ecosphere. 9, e02300. doi: 10.1002/ecs2.2300
González, M. E., Sapiains, R., Gomez-González, S., Garreaud, R., Miranda, A., Galleguillos, M., et al. (2020). Incendios forestales en Chile: causas, impactos y resiliencia. Centro de Ciencia del Clima y la Resiliencia (CR)2, Universidad de Chile, Universidad de Concepcion y Universidad Austral de Chile (Santiago), 80.
Hoover, C. M., Leak, W. B., and Keel, B. G. (2012). Benchmark carbon stocks from old-growth forests in northern New England, USA. For. Ecol. Manage. 266, 108–114. doi: 10.1016/j.foreco.2011.11.010
IPCC (2022). “Climate change: impacts, adaptation, and vulnerability,” in Contribution of Working Group II to the Sixth Assessment Report of the Intergovernmental Panel on Climate Change, eds Pörtner, H. O., Roberts, D. C., Tignor, M., Poloczanska, E. S., Mintenbeck, K., Alegríam, A., et al. Cambridge: Cambridge University Press. In Press.
Janisch, J. E., and Harmon, M. E. (2002). Successional changes in live and dead wood carbon stores: implications for net ecosystem productivity. Tree Physiol. 22, 77–89. doi: 10.1093/treephys/22.2-3.77
Keith, H., Lindenmayer, D. B., Mackey, B., Blair, D., Carter, L., McBurney, L., et al. (2014). Managing temperate forests for carbon storage: impacts of logging vs. forest protection on carbon stocks. Ecosphere 5, 1–34. doi: 10.1890/ES14-00051.1
Keith, H., Mackey, B. G., and Lindenmayer, D. B. (2009). Re-evaluation of forest biomass carbon stocks and lessons from the world's most carbon-dense forests. Proc. Nat. Acad. Sci. U. S. A. 106, 11635. doi: 10.1073/pnas.0901970106
Kitzberger, T., Veblen, T., T. and Villalba, R. (2000). “Métodos dendrocronológicos y sus aplicaciones en estudios de dinámica de bosques templados de Sudamérica,” in Dendrocronología en América Latina. Mendoza, ed Roig, F,. (Argentina: EDIUNC), 17–78.
Lara, A. (1991). The dynamics and disturbance regimes of Fitzroya cupressoides forests in the South-Central Andes of Chile. (Ph.D. Thesis), University of Colorado, Boulder.
Lara, A., Echeverría, C., Thiers, O., Huss, E., Escobar, B., Tripp, K., et al. (2008). “Restauración ecológica de coníferas longevas: el caso del alerce (Fitzroya cupressoides) en el sur de Chile,” Restauración de bosques en América Latina, eds González-Espinosa, M., Rey-Benayas, J. M., and Ramírez-Marcial, N., (México: Fundación Internacional para la Restauración de Ecosistemas (FIRE) y Editorial Mundi-Prensa México), 39–56.
Lara, A., Fraver, S., Aravena, J. C., and Wolodarsky-Franke, A. (1999). Fire and the dynamics of Fitzroya cupressoides (alerce) forests of Chile's Cordillera Pelada. Ecoscience. 6, 100–109. doi: 10.1080/11956860.1999.11952199
Lara, A., Urrutia-Jalabert, R., Reyes, R., González, M., Miranda, A., Altamirano, A., et al. (2019). “Bosques nativos,” in Informe País Estado del Medio Ambiente en Chile 2018, Centro de Análisis de Políticas Públicas. (Santiago, CL: Universidad de Chile), 171–219.
Lara, A., Villalba, R., Urrutia-Jalabert, R., González-Reyes, A., Aravena, J. C., Luckman, B. H., et al. (2020). +A 5680-year tree-ring temperature record for southern South America. Quat. Sci. Rev. 228, 2020. doi: 10.1016/j.quascirev.2019.106087
Lara, A., Wolodarsky-Franke, A., Aravena, J. C., Cortés, M., Fraver, S., and Silla, F. (2003). “Fire Regimes and Forest Dynamics in the Lake Region of South-Central Chile,” in Fire and Climatic Change in Temperate Ecosystems of the Western Americas, eds Veblen, T. T., Baker, W. L., Montenegro, G., and Swetman, T. W,. (Springer-Verlag New York, Inc.), 322–342.
Luyssaert, S., Schulze, E. D., Borner, A., Knohl, A., Hessenmoller, D., Law, B. E., et al. (2008). Old-growth forests as global carbon sinks. Nature. 455, 211–213. doi: 10.1038/nature07276
Malhi, Y., Girardin, C., Metcalfe, D. B, Doughty, C. E., Aragão, L. E., et al. (2021). The Global Ecosystems Monitoring network: Monitoring ecosystem productivity and carbon cycling across the tropics. Biol. Conserv. 253, 108889. doi: 10.1016/j.biocon.2020.108889
Marcos-Martínez, R., Sánchez, J. J., Srivastava, L., Soonsawad, N., and Bachelet, D. (2022). Valuing the Impact of Forest Disturbances on the Climate Regulation Service of Western U.S. Forests. Sustainability, 14, 903. doi: 10.3390/su14020903
McDowell, N., Allen, C.D., Anderson-Teixeira, K., Aukema, B.H., Bond-Lamberty, B., Chini, L., et al. (2020). Pervasive shifts in forest dynamics in a changing world. Science 368, eaaz9463 doi: 10.1126/science.aaz9463
McMahon, S. M., Parker, G. G., and Miller, D. R. (2010). Evidence for a recent increase in forest growth. Proc. Natl. Acad. Sci. U. S. A. 107, 3611–3615. doi: 10.1073/pnas.0912376107
Mildrexler, D. J., Berner, L. T., Law, B. E., Birdsey, R. A., and Moomaw, W. R. (2020). Large Trees Dominate Carbon Storage in Forests East of the Cascade Crest in the United States Pacific Northwest. Front. For. Glob. Change 3, 594274. doi: 10.3389/ffgc.2020.594274
Molina, R. O. (1995). Alerces y Alerceros en el Territorio Huilliche de San Juan de la Costa. II Congreso Chileno de Antropología. Colegio de Antropólogos de Chile A. G. (Valdivia).
Peralta, M., Ibarra, M., and Oyanedel, E. (1982). Suelos del tipo forestal Alerce. Ciencias Forestales, 2, 39–60.
Pérez-Quezada, J. F., Olguín, S., Fuentes, J. P., and Galleguillos, M. (2015). Tree carbon stock in evergreen forests of Chiloé, Chile. Bosque, 36, 27–39. doi: 10.4067/S0717-92002015000100004
Pickett, S. T. (1989). “Space-for-time substitution as an alternative to long-term studies,” in Long-term Studies in Ecology. (New York, NY: Springer), 110–135. doi: 10.1007/978-1-4615-7358-6_5
Pozo, R. A., Galleguillos, M., González, M. E., Vásquez, F., and Arriagada, R. (2022). Assessing the socio-economic and land-cover drivers of wildfire activity and its spatiotemporal distribution in south-central Chile. Sci. Total Environ. 810, 152002. doi: 10.1016/j.scitotenv.2021.152002
Pregitzer, K. S., and Euskirchen, E. S. (2004). Carbon cycling and storage in world forests: biome patterns related to forest age. Glob. Change Biol. 10, 2052–2077. doi: 10.1111/j.1365-2486.2004.00866.x
R Development Core Team (2014). R: a language and environment for statistical computing. R Foundation for Statistical Computing, Vienna, Austria. Available online at: https://www.r-project.org/ (accessed July 20, 2022).
Schlegel, B. C., and Donoso, P. J. (2008). Effects of forest type and stand structure on coarse woody debris in old-growth rainforests in the Valdivian Andes, south-central Chile. For. Ecol. Manage. 255, 1906–1914. doi: 10.1016/j.foreco.2007.12.013
Seidl, R., Thom, D., Kautz, M., et al. (2017). Forest disturbances under climate change. Nat. Clim. Chang. 1326, 395–402. doi: 10.1038/nclimate3303
Sibold, J., González, M. E., and Lara, A. (2021). “Fire history of Alerce (Fitzroya cupressoides) forests of the Cordillera Pelada, Chile,” in Symposium: The Change of the Fire Regime in the Forest Landscape of Central-southern Chile. XXVI International Congress of Geography (Valparaíso, Chile).
Silla, F., Fraver, S., Lara, A., Allnut, T., and Newton, A. (2002). Regeneration and stand dynamics of Fitzroya cupressoides (Cupressaceae) forests of southern Chile's Central Depression. For. Ecol. Manage. 165, 213–224. doi: 10.1016/S0378-1127(01)00619-3
Stewart, G. H., and Burrows, L. E. (1994). Coarse woody debris in old-growth temperate beech (Nothofagus) forests of New Zealand. Canadian. J. For. Res. 24, 1989–1996. doi: 10.1139/x94-255
Swanson, F. J., Crisafulli, C. M, and Yamaguchi, D. M. (2005). “Geological and ecological setting of Mount ST. Helen before May 18, 1980,” in Ecological responses to the 1980 eruption of Mount ST. Helens, eds. Dale, V. H., Swanson, F. J., and Crisafulli, C. M,. (New York, NY: Springer), 13–26. doi: 10.1007/0-387-28150-9_2
Swanson, F. J., and Major, J. J. (2005). “Physical events, environments, and geological-ecological interactions at Mount St. Helens: March 1980-2004,” in Ecological Responses to the 1980 Eruption of Mount St. Helens, eds Dale, V. H., Swanson, F. J., and Crisafulli, C. M,. (New York: Springer), 27–44. doi: 10.1007/0-387-28150-9_3
Thom, D., and Seidl, R. (2016). Natural disturbance impacts on ecosystem services and biodiversity in temperate and boreal forests. Biol. Rev. 91, 760–781. doi: 10.1111/brv.12193
Tosso, J. (1985). Suelos volcánicos de Chile. Instituto de Investigaciones Agropecuarias (Santiago: INIA), 716.
Urrutia, R. (2002). Desarrollo de una cronología de anchos de anillos para alerce (Fitzroya cupressoides) y reconstrucción de la historia de incendios en el área de Abtao, Parque Nacional Chiloé, X región. Tesis Ingeniería Forestal. Pontificia Universidad Católica de Chile (Santiago), 77.
Urrutia-Jalabert, R., González, M. E., González-Reyes, A., Lara, A., and Garreaud, R. (2018b). Climate variability and forest fires in central and south-central Chile. Ecosphere. 9, e02171. 10.1002/ecs2.2171. doi: 10.1002/ecs2.2171
Urrutia-Jalabert, R., Lara, A., Barichivich, J., Vergara, N., Rodriguez, C. G., and Piper, F. I. (2020). Low growth sensitivity and fast replenishment of non-structural carbohydrates in a long-lived endangered conifer after drought. Front. Plant Sci. 11, 905. doi: 10.3389/fpls.2020.00905
Urrutia-Jalabert, R., Malhi, Y., Barichivich, J., Lara, A., Delgado-Huertas, A., Rodríguez, C. G., and Cuq, E. (2015b). Increased water use efficiency but contrasting tree growth patterns in Fitzroya cupressoides forests of southern Chile during recent decades. J. Geophys. Res. Biogeosci. 120, 2505e2524. doi: 10.1002/2015JG003098
Urrutia-Jalabert, R., Malhi, Y., and Lara, A. (2015a). The oldest, slowest rainforests in the world? Massive biomass and slow carbon dynamics of Fitzroya cupressoides temperate forests in southern Chile. PLoS ONE, 10, e0137569. doi: 10.1371/journal.pone.0137569
Urrutia-Jalabert, R., Peña, M. P., Coopman, R., Carvajal, D., Jiménez-Castillo, M., Cosimo, D., et al. (2018a). Elucidating the hydraulic vulnerability of the longest-lived Southern Hemisphere conifer to aridification. For. Ecol. Manage. 430, 472484. doi: 10.1016/j.foreco.2018.08.027
Veblen, T. T. (1992). “Regeneration dynamics,” in Plant Succession: Theory and Prediction, eds D. C. Glenn-Lewin, R. K. Peet, and T. T. Veblen (London: Chapman and Hall), 152–187.
Veblen, T. T., Armesto, J. J., Burns, B. R., Kitzberger, T., Lara, A., León, B., et al. (2005). “The Coniferous forests of South America,” in Ecosystems of the World: Coniferous Forests, eds Andersson, F., and Gessel, S,. (Amsterdam, The Netherlands: Elsevier B.V.), 293–318.
Veblen, T. T., and Ashton, D. H. (1982). The regeneration status of Fitzroya cupressoides in the Cordillera Pelada, Chile. Biol. Conserv. 23, 141–161. doi: 10.1016/0006-3207(82)90036-2
Veblen, T. T., Burns, B. R., Kitzberger, T., Lara, A., and Villalba, R. (1995). “The ecology of the conifers of southern South America,” in Ecology of the Southern Conifers, eds Enright, N. J., and Hill, R. S,. (Carlton, Victoria: Melbourne University Press), 120–155.
Veblen, T. T., Delmastro, R. J., and Schlatter, J. E. (1976). The conservation of Fitzroya cupressoides and its environment in southern Chile. Environ. Conserv. 3, 291–301. doi: 10.1017/S0376892900019081
Veblen, T. T., Holz, A., Paritsis, J., Raffaele, E., Kitzberger, T., and Blackhall, M. (2011). Adapting toglobal environmental change in Patagonia: what role for disturbance ecology? Aust. Ecol. 36, 891–903. doi: 10.1111/j.1442-9993.2010.02236.x
Villagrán, C., Varela, J., Fuenzalida, H., Veit, H., Armesto, J., and Aravena, J. C. (1993). “Geomorphological and vegetational background for the analysis of the quaternary of the Chilean Lake District,” in The Quaternary of the Lake District of Southern Chile, ed Villagrán, C,. (Santiago), 1–50.
Walker, L. R., Wardle, D. A., Bardgett, R. D., and Clarkson, B. D. (2010). The use of chronosequences in studies of ecological succession and soil development. J. Ecol. 98, 725–736. doi: 10.1111/j.1365-2745.2010.01664.x
Keywords: carbon stocks, old-growth forests, endangered species, Fitzroya cupressoides, southern South America, disturbances
Citation: González ME, Lara A, Urrutia-Jalabert R, Bustos-Salazar A, Ruiz-Gómez C and Aravena JC (2022) Carbon stocks across different environments, disturbance regimes, and stand age in Fitzroya cupressoides forests, the longest-lived species of the southern hemisphere. Front. For. Glob. Change 5:960429. doi: 10.3389/ffgc.2022.960429
Received: 02 June 2022; Accepted: 29 July 2022;
Published: 13 September 2022.
Edited by:
Maxence Martin, Université du Québec en Abitibi Témiscamingue, CanadaReviewed by:
Panna Chandra Nath, Assam University, IndiaK. C. Cushman, NASA Jet Propulsion Laboratory (JPL), United States
Copyright © 2022 González, Lara, Urrutia-Jalabert, Bustos-Salazar, Ruiz-Gómez and Aravena. This is an open-access article distributed under the terms of the Creative Commons Attribution License (CC BY). The use, distribution or reproduction in other forums is permitted, provided the original author(s) and the copyright owner(s) are credited and that the original publication in this journal is cited, in accordance with accepted academic practice. No use, distribution or reproduction is permitted which does not comply with these terms.
*Correspondence: Mauro E. González, bWF1cm9nb256YWxleiYjeDAwMDQwO3VhY2guY2w=