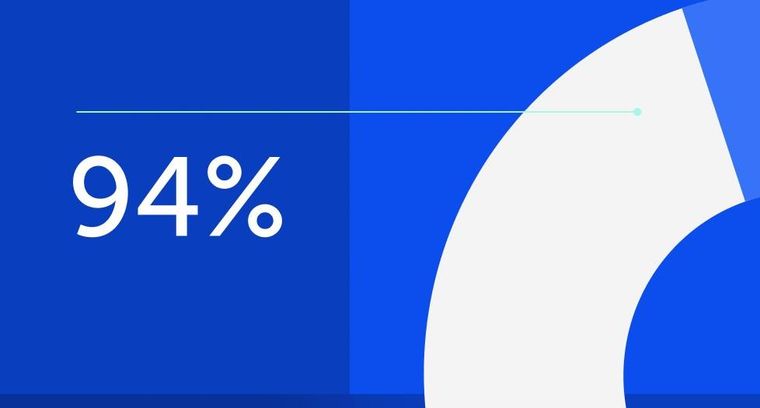
94% of researchers rate our articles as excellent or good
Learn more about the work of our research integrity team to safeguard the quality of each article we publish.
Find out more
ORIGINAL RESEARCH article
Front. For. Glob. Change, 19 October 2022
Sec. Forest Management
Volume 5 - 2022 | https://doi.org/10.3389/ffgc.2022.957189
This article is part of the Research TopicForest Carbon Credits as a Nature-Based Solution to Climate Change?View all 10 articles
California’s cap-and-trade compliance offset market incentivizes forest managers to maintain elevated carbon stocks. It provides these incentives without enforcing standardized fire mitigation practices despite many projects being located in fire prone regions. Here, we evaluated the difference between management actions in California forests that participated in the carbon offset market versus those that engaged with state programs to reduce wildfire risk via fuel reduction treatments. Using remotely sensed data from the California Forest Observatory and the Moderate Resolution Imaging Spectroradiometer, we compared the vertical forest structure and vegetation canopy trends on forest offsets with forests that are receiving fuel treatment. We found California forests managed for carbon under the Improved Forest Management (IFM) program by the California Air Resources Board had higher levels of biomass than forests managed for fire risk reduction as indicated by 2016 lidar-estimated fuel loads. In addition, IFM-participating forests did not reduce their fuel loads between 2016 and 2020, whereas lands receiving grants for fuel management did, indicating that on average, the IFM projects were not engaging in fuel reduction efforts. However, despite the differences in fuel management between IFM projects and active fuel treatments, we found that both types of management saw a declining trend in vegetation greenness between 2015 and 2021. While declining greenness is expected of active fuel treatments associated with vegetation removal, such a trend in the case of IFM indicates additional wildfire risk. Managing forests for long-term carbon storage and sequestration requires consideration of fire risk mitigation. Given the little evidence of fuel reduction in the first decade of IFM projects implementation we question whether the century-long duration of carbon stocks in these offsets is realistic. We recommend that policymakers reevaluate the incentives directed at carbon stock preservation or expansion to better encompass the growing wildfire risk in California.
Fire releases carbon stored in forest biomass into the atmosphere, making wildfires a threat to storing carbon in forests (Hurteau and Brooks, 2011; North and Hurteau, 2011). In California, wildfires are having a negative impact on aboveground live carbon stocks in forests: between 2001 and 2010 the estimated aboveground live carbon stocks in California declined by a rate of 0.8% per year. These declines were primarily driven by wildfire disturbances occurring on just 6% of the total forest area (Gonzalez et al., 2015). The emissions associated with burning forests drive most of the estimated greenhouse gas emissions from wildfires in California and these emissions may increase as climate change is expected to increase areas burned in California, in part from warming-driven fuel drying (Westerling et al., 2011; Williams et al., 2019; California Air Resources Board, 2020).
The growing impact of wildfire on carbon emissions is occurring within an already existing forest management crisis. Over a century of fire removal and suppression has altered species composition and function in the Western United States (Collins et al., 2017). Forests in California are, on average, supporting higher tree density, with an estimated average increase of 2.75 times more trees per acre (Safford and Stevens, 2017). Most forest stands in California support tree densities which place them in full competition, reducing tree growth efficiency and resilience to stressors like drought and fire, and putting them at high mortality risk (North et al., 2022). With expected increases in climatic stressors from climate change, these current forest densities are not sustainable. Using historic forest condition data to predict forest survival under future climate scenario RCP 8.5, research on the Sierra Nevada and Southern Cascade has found that forests may be unable to support aboveground biomass above 25% of its current average levels (Bernal et al., 2022). If this climate scenario were realized, it would mean many forests in these regions currently have aboveground biomass levels that pose an existential risk.
From a carbon perspective, increases in tree density do not necessarily translate to increased forest carbon because such increase has been primarily from small-diameter trees. The combination of removing frequent fires and the legacy of logging the largest trees have caused a decline in large trees in California forests by 50% between the 1930s to the 2000s, being replaced with smaller trees, and species composition shifting away from pine dominance (McIntyre et al., 2015). This shift in dominance to smaller, fire-sensitive trees has reduced total live carbon stocks (North et al., 2009). The largest 1% of trees make up about 30% of live aboveground biomass in the United States, and generally, large trees dominate carbon stocks in temperate US forests (Lutz et al., 2018; Mildrexler et al., 2020). Additionally, because carbon accumulation increases continuously with tree size, large-diameter trees being replaced with small-diameter trees also negatively impacts the rate of carbon sequestration (Stephenson et al., 2014). These changes in forest structure and density are exacerbated by acute drought stressors, which create forest conditions where subsequent human-made or natural disturbances have the potential to be more severe than they otherwise would have been (Stephens et al., 2018, 2022). Another consequence of increasing disturbance severity is that these more severe disturbances can alter plant successions away from forests to grass or shrublands (Falk et al., 2022). Forest conversion to other types of vegetation cover can bring with it reduced aboveground carbon storage capacities and fire regimes that burn more severely (Coop et al., 2020). This interplay between climate change, fire exclusion, and antecedent disturbances changing forest composition creates the conditions that can lead to mega-fires (Stephens et al., 2014).
Despite these challenges, for forests in the Western United States, management aimed at vegetation removal from either forest restoration or fuels reduction can promote forests better able to withstand changing fire, drought, and insect stressors (Stephens et al., 2021). Fuel treatments remove vegetation and other material that could combust in a fire, in aims of reducing fire behavior and fire severity for a future fire. The benefit to forest carbon that a fuel treatment provides is the difference between the reduced fire behavior and severity with what would have occurred without fuel treatments. While fuel treatments explicitly address the imminent threat of extreme wildfires, forest restoration efforts often use similar tools like prescribed fire and restoration thinning treatments to reduce forest density, also reducing available fuel and preventing the worst effects of extreme wildfire (Stephens et al., 2020). Along with effectively reducing fire severity (reduced tree mortality), fuel treatments can also reduce wildfire emissions (North and Hurteau, 2011). This potential emissions reduction means that widespread prescribed fire application could reduce fire related CO2 emissions in the Western US by 18-25% relative to a business-as-usual scenario (Wiedinmyer and Hurteau, 2010). The fuel treatments used in the Western United States thus are effective in reducing wildfire, associated emissions, and other forest stressors, while often aligning with other ecological restoration objectives.
To increase the pace of implementing fuel treatments in California, the California Department of Forestry and Fire Protection (Cal Fire) provides landowners funding, planning, and implementation support to reduce costs of implementing fuel treatments. There are five Cal Fire programs that support fuel reductions: The California Fire Plan, Vegetation Management Program, Forest Health Grants, Fire Prevention Grants, and California Vegetation Treatment Program. Landowners supported by these grants for fuels reduction can achieve their goals using a diversity of methods, including prescribed fire, tree thinning, pruning, chipping, and roadway clearance (California Department of Forestry and Fire Protection [CAL FIRE], 2022a). From a carbon accounting perspective, deciding to implement a fuel treatment means an immediate reduction in total amount of carbon, as fuel treatments remove or burn vegetation. Even if management causes some temporary reductions in carbon, over a century, large-scale restoration is projected to increase carbon stability (Liang et al., 2018). In fire prone ecosystems, stable carbon is the amount of carbon that is predicted to survive a wildfire. Managing stable carbon stocks in forests requires balancing total carbon pools against disturbance risks that emit carbon. Based on research from California forests, prescribed fire, mechanical treatments, or a combination thereof have been shown to increase stable live carbon (North et al., 2009; Foster et al., 2020). Programs like California Fire Plan explicitly reference fuel reductions as part of maintaining California’s carbon sinks and have overarching goals of improving forest health through fire risk reduction (State Board of Forestry and Fire Protection and California Department of Forestry and Fire Protection [CAL FIRE], 2018).
Stable forest carbon management, which includes fuel management, is needed to maintain carbon durably in California forests. Yet, a large portion of funding in response to climate change has been to pay landowners for increasing aboveground forest carbon to offset greenhouse gas emissions elsewhere. One example of such a program is California’s cap-and-trade compliance market. This program is administered by California’s Air Resources Board (CARB) which facilitates a carbon market where forest landowners can enroll in an Improved Forest Management (IFM) offset program and be paid for managing existing forests for carbon stock maintenance and expansion (California Air Resources Board, 2011, 2015). IFM projects in this market are intended to store carbon for 100-years above a regionally determined carbon baseline. Before the project is awarded carbon credits, the IFM project owner submits initial documents to registries disclosing how they intend on increasing aboveground live carbon for the next century. This can include strategies like increasing rotation length, decreasing harvest intensity, or switching to uneven age management. IFM projects, once validated and issued credits, cannot significantly reduce on-site carbon stocks without a penalty.
CARB and Cal Fire currently administer programs that pay private landowners for forest management, with both agencies interested in stabilizing and storing carbon in forests. Forest landowners in CARB’s Improved Forest Management (IFM) offset program are incentivized to increase aboveground carbon, while the grantees of the Cal Fire program are supported to temporarily remove aboveground carbon. Forest carbon offset programs that require sustaining already elevated forest biomass create the possibility for incentives that conflict with stable carbon management. CARB’s IFM program is relatively popular, generating 78.8% of carbon offset credits that have been retired during the compliance periods in California’s compliance market (California Air Resources Board Offset Credit Issuance Table, 2022). Focusing on increasing aboveground carbon in fire prone forests could lead to management decisions counter to stable carbon storage (Hurteau et al., 2008; North and Hurteau, 2011; Bernal et al., 2022). CARB and Cal Fire have created programs with conceptual differences in how to manage forest carbon: increasing forest carbon storage versus increasing carbon stability by reducing wildfire risk. It is unknown if these IFM projects effectively target forests where biomass increases or decreases will lead to long term stable carbon on the landscape.
Improved Forest Management projects are minimally incentivized to do fuel treatments under the current standard design. The buffer pool is a reserve of credits that every IFM project contributes a set amount of their issued credits to as an insurance policy. Typically, a project contributes 9-19% of issued credits, with an average around 15%, to this pool (California Air Resources Board, 2011). Projects that complete a ‘qualified’ fuel treatment can receive up to a 2% reduction on their buffer pool contribution, meaning the project owner receives a greater percentage of the credits generated. This 2% incentive for fuel treatments is the only way a project can reduce its contribution to the buffer pool for natural disturbance risks and requires a third-party auditor to confirm the fuel treatment. Yet, based on the observed contributions projects are giving to the buffer pool, most of the IFM projects in California are not taking advantage of this buffer pool contribution reduction. It is not clear why IFM projects are not using this buffer pool contribution reduction, but low adoption suggests that the costs of doing a qualified fuel treatment exceeds the benefit to the project owner of doing a fuel treatment.
The problem for assessing the effectiveness of IFM project management strategies is that it is difficult to determine exactly which types of management takes place within IFM projects. While the projects submit documentation to public registries, in practice, these documents vary in the level of detail and supplemental information provided describing management plans. Information like whether a project owner plans on implementing fuel management in the forms of mechanical thinning or prescribed fire between harvests is not required in the documentation, even if a IFM project owner intends on employing one of these techniques. The other limitation on relying on registry-submitted documentation is that it details a project owner’s intent, but they are not bound to execute the detailed management, they only need to maintain carbon levels above a regionally determined baseline and any crediting increases above that baseline. It would be reasonable to expect some of these projects are using fuel management given the legacy of fire suppression increasing tree density and the growing understanding that climate change will exacerbate threats to storing carbon in trees, but use of fuel treatment in IFM projects has never been quantified.
Here we use novel remotely sensed data and a host of statistical techniques to answer two main questions. First, how common are fuel treatments on IFM lands and how do these treatments compare to treatments carried out under Cal Fire fuel reduction programs? Second, we ask if forest health, as proxied by vegetation greenness, has changed on IFM forests relative to forests that have received Cal Fire grants. By answering these questions, we hope to better understand if California’s large carbon offset credit program is effectively addressing the tradeoffs between carbon, fuel management, and vegetation health outcomes. These tradeoffs point to a conceptual difference between CARB’s approach of increasing forest carbon storage and Cal Fire’s promotion of fuel treatments to increase carbon stability via reducing wildfire risk. If these different visions of forest carbon management are not appropriate for the landscape, these two state agencies could be working in opposition of strategic landscape carbon management.
Improved Forest Management projects under California’s compliance offsets market can be developed on any private forest in the United States. We limit our analysis to the IFM projects in California, where projects are in the North Coast and Southern Cascade regions (LANDFIRE, 2001). This research spans forests in California that are managed under the IFM offsets or Cal Fire fuel treatments from the same region (Figure 1). The North Coast and Southern Cascade have a Mediterranean climate, with warm, dry summers and mild, wet winters (latitude 37° to 40°). The North Coast has complex terrain from the Klamath mountains and is home to the most diverse conifer forest in North America. The Southern Cascade region has a relatively milder terrain, with elevation ranging from 60 m to 4,317 m, and is forested by conifer species. Historically, both regions conifer forests would have had fire regimes characterized by surface fires burning at low- to moderate-intensity (Skinner and Taylor, 2006; Skinner et al., 2006). We excluded fuel treatments from outside of these two regions because there may be regional variability in fuel loads and vegetation trends that would make them too different to compare to the IFM projects.
Figure 1. Study Area Map showing the North Coast and southern Cascade region in northern California. This map includes fires between 2016-2020 and locations of IFM projects and fuel treatments.
Cal Fire’s Management Activity Project Planning and Event Reporter (CalMAPPER) database includes location and project information for forest and fuel management projects across Cal Fire programs (California Department of Forestry and Fire Protection [CAL FIRE], 2022b). We used GIS treatment polygons produced by Cal Fire which record areas of grant funded fuel treatments to establish how fuel treatments alter vegetation in forests within the same regions as the IFM projects.
CARB-approved IFM projects submit GIS shapefiles or maps of project boundaries to the three registries approved for CARB offset use, American Carbon Registry, Climate Action Reserve, and Verra. The dataset we use to determine where IFM projects occur in California are based on these submitted project boundaries to the registries (Stapp, 2022). This data represents 31 of the 40 CARB-approved IFM projects in California that became boundaries for sampling in our study as ‘IFM projects.’ The remaining nine projects listed on the public registries lacked spatial data. We have no reason to believe projects with missing geospatial data are absent for a systemic reason and our sample of 31 projects were representative of geography and size of projects within California so we proceed with the analysis using the available data, considering this a representative dataset of the CARB-approved IFM projects in California.
We limited the CalMAPPER treatment polygons to projects that had ‘fuel reduction’ recorded as the “Treatment Objective” and were in the North Coast or the Southern Cascade region (California Department of Forestry and Fire Protection [CAL FIRE], 2022b). This limited our dataset to projects that were in the California Fire Plan, Vegetation Management Program, and Forest Health grants. This dataset included 448 Cal Fire-approved fuel reduction projects. Cal Fire grants span California and the data is provided both as a larger project boundary and the polygons where management activity occurs. This analysis uses the more specific polygons where management occurs for sampling, called the Treatment Polygons. We used the fuel treatments start and end dates to determine the status of fuel reduction activities and gain a better sense of how fuel treatments change vertical structures of fuel over time. Projects that had end dates prior to 2016 are recoded as “Completed Fuel Treatments,” projects that were completed by 2020 were recorded as “Active Fuel Treatments,” and projects that did not begin until after 2020 were recorded as “Planned Fuel Treatments.” This created 1,392 completed, 2,911 active, and 519 planned project polygons that we used to determine sample boundaries for creating our observation dataset.
The California Forest Observatory produced annual maps of fuels for forests in California at 10-meter spatial resolution from the summers of 2016 and 2020. The fuel structure data are modeled products based on satellite radar, satellite spectral imagery, and airborne lidar flights (California Forest Observatory, 2020). Airborne lidar is a useful tool for estimating fuel loads because it can penetrate canopy covers to estimate vertical structures of vegetation based on the timing and density of points that return to the sensor (Mutlu et al., 2008; Su et al., 2016; Jarron et al., 2020). The California Forest Observatory lidar-derived fuel maps represent the best available fuels dataset that has both high spatial resolution and is produced for multiple years. This spatial and temporal coverage was necessary for research studying how fuels change over time at a landscape scale. Fuel data includes ladder fuel density (%), surface fuel (unitless), canopy cover (%), canopy height (meters), canopy layer count (#), and canopy base height (meters), relevant to fuel and carbon discussion. Ladder fuel density (r2 = 0.78) considers the lidar point returns from 1 to 4 meters above ground returns which represent the proportion of surface fuels in the understory. Surface fuels are based on outputs from Scott & Burgan Fire Behavior Fuel Models where higher values show higher levels of surface fuels. Canopy cover (r2 = 0.91) reports the horizontal cover fraction from tree canopies. Canopy height (r2 = 0.86) is the distance between ground and top of canopy returns. Canopy layer count (r2 = 0.765) is the number of distinct vertical canopy layers, which could be a proxy for leaf area index or canopy complexity. And canopy base height (r2 = 0.70) is the distance between the ground and the lowest branches in the canopy, predictive of whether a surface fire will transition to a canopy fire (California Forest Observatory, 2020). For all but surface fuel estimates, the California Forest Observatory produces accuracy estimates (Supplementary Table 1). Using the California Forest Observatory produced API in Jupyter notebook, the six types of fuel data from 2016 and 2020 were queried for the study area.
The study period, 2016 to 2020, was constrained by the availability of the lidar-based datasets. While it is a brief period relative to the century-long forest management projects, we believed this was an appropriate study period because we were interested in how IFM project were managing or prioritizing fire risk and stable carbon storage during their initial project years. The first years of an IFM project are relevant to fuel treatments because it tends to be when projects receive the largest number of credits, which could fund non-commercial forest management like fuel treatments.
Using the “geemap” package for Google Earth Engine access in Python, this fuel data was sampled within IFM project and fuel treatment areas at a 300-m sample grid. We chose this grid size as a good trade-off between increased sample observations, Google Earth Engine image processing limits, and limiting spatial autocorrelation. On one hand, a denser sampling grid provides more information in terms of the number of observations, but the trade-off is increased spatial autocorrelation. The grid size of 300 m does a sufficient job of balancing this trade-off. Even so, we checked the residuals from our linear regression analysis and found there was still autocorrelation that could not be resolved (Chen, 2013). The implication of residual spatial autocorrelation is that the actual standard errors may be larger than estimated, meaning that we may be less confident in our results than standard significant test would suggest. Therefore, additional caution may be needed in evaluating our results. Sampled observations of fuel and fuel change were exported as a CSV file for further analysis in R Studio.
We compare changes in IFM projects with different stages of implemented fuel treatments (completed, active, and planned) to test if IFM projects alter fuels in ways that resembles fuel treatments. For example, if the IFM projects are managing fuels during the study period, then they might appear to change fuels in similar ways to active fuel treatments. We expected active fuel treatments would have a reduction in the fuel, while the completed and planned fuel treatments might have a positive change in fuel strata estimates, as vegetation recovers after previous fuel treatments or grows prior to future fuel treatments. Depending on how the IFM projects changed during this remeasurement relative to the three stages of fuel treatments, we can determine if fuels have been managed. The input of the fuel analysis is the CAFO lidar-based dataset which produces estimates of different vertical levels of carbon.
Because we were interested in observing which strata are most impacted by fuel treatments or IFM project management, we analyzed the six fuel lidar datasets—surface, ladder, canopy cover, canopy height, canopy layer count, canopy base height —as separate regressions to understand how these management approaches altered forest and fuel structure. For example, using a popular ‘thin from below’ silvicultural approach will maintain high levels of canopy cover and canopy height and remove the non-dominant trees that could carry surface flames to canopy. The result of such a treatment would be a reduction in surface and ladder fuel, an increase in canopy base height, a decrease canopy layer count, and little or no-change in canopy cover or canopy height. We report all tests for the following section for the six separate fuel strata because the direction of changes over time depends on the strata of fuel evaluated.
To understand if fuels differ between IFM projects and fuel treatments, we first compared fuel estimates for IFM projects and Cal Fire fuel treatments at the beginning of our study period. We compared offsets to the three categories of fuel treatments, and we visualized our results by creating a boxplot of the starting 2016 fuel estimates by fuel type and region (Figure 2).
Figure 2. Boxplots of the 2016 fuel estimates for the IFM projects and fuel treatments (planned, active, and completed) across the Southern Cascade and North Coast regions.
Forests managed as offsets may differ from forests eligible for grant-funded fuel treatments, creating a form of selection bias. Therefore, we use propensity score matching to prepare a dataset that limits the differences between our controls, or fuel treatments, relative to our treatments, or IFM offsets (Austin, 2011). We use factors associated with vegetation growth because we thought factors that might contribute to whether a forest is an offset or receives a grant-funded fuel treatment might be associated with how productively vegetation grows on that site. Along with sampling how fuel changed at each sample, we sampled climatic variables like total winter precipitation and max summer temperature as annual composites from 2016 to 2020, physical variables like elevation, slope, and aspect used in parametric matching (Table 1). The climatic variable selected were based on variables considered important for predicting forest survival (Bernal et al., 2022). We implemented this parametric matching to identify fuel treatments that were otherwise like IFM offsets using all the variables noted in Table 1, the fuel estimate from 2016, and a variable signifying which region the IFM project or fuel treatment is located.
Table 1. List of biophysical variables used for propensity score matching, indicated with asterisk, and full list of variables included in linear regression.
This matched dataset was used in a linear regression to predict the change in fuel load controlling for the variables used for matching, the starting fuel estimate from 2016, the region, a treatment term, indicating whether the sample was taken from an offset, or a fuel treatment completed by 2016, and an interaction term between region and treatment. We included region as a factor in linear regression because our samples spanned two distinct forest types that represent different ecologies with different fire regimes and captured this difference as the region variable. The interaction term allowed us to independently predict the treatment effects for the two regions studied. We used the output of the linear regression in an estimated mean marginal effects model to predict the change in fuel load based on treatment and region using the interaction term. To understand the effect of fuel load changes on IFM projects relative to fuel treatments, we used the linear regression outputs to predict changes in fuel for a four-year period for the IFM projects and fuel treatments. This allowed us to report the effect in the same units as the original fuel estimate, easing interpretation. Predicted changes in fuel estimates that are greater than zero indicate that fuel is predicted to increase for that group, whereas a change in fuel that is less than zero indicate that there is a predicted loss of fuel, consistent with vegetation removal. The pre-regression matching, linear regression, and prediction was repeated for the six types of fuels estimated from the California Forest Observatory dataset.
Beyond changes in total fuel amount changing vegetation greenness also has implications for forest carbon and fire risk. A forest may show signs of vegetation stress or declines in productivity through decrease in canopy vegetation greenness (Sims et al., 2006). Forests with reductions in greenness may also indicate areas of increased fuel dryness, making it more available to burn if there is a fire (Roberts et al., 2006). We used satellite-based spectral estimates of vegetation greenness using the Enhanced Vegetation Index (EVI). EVI was designed for use in high density vegetation areas and has been demonstrated to be efficient indicators of forest productivity and die back (Sims et al., 2006; Ogaya et al., 2015).
This estimate of vegetation greenness, was calculated in Google Earth Engine using the Moderate Resolution Imaging Spectroradiometer (MODIS) imagery, based on the Non-Parametric Trend Analysis tutorial (Clinton, 2022) The MODIS EVI data product was the basis of a constructed time series of vegetation greenness between 2015-2021 based on clear-sky, cloud-corrected imagery collected between August and September. A Kendall-Man non-parametric trend analysis was used to reduce the EVI time series imagery to identify areas of increasing or decreasing vegetation greenness. These analyzed timeseries data produced a single number of the observed trend in greenness for Objective 2. A positive EVI Kendall Mann value indicates that the vegetation greenness is increasing over time. The MODIS EVI data was sampled for the offset and fuel treatment project areas using the same sample grid at 300-m density that was used for the fuel analysis in Objective 1. This study period was selected to complement the first research question, 2016 to 2020, and could be expanded to larger periods.
To further analyze how the trends of vegetation greenness differ between the two types of forest management, we used a bootstrap to estimate the true differences between the sample median values. The strength of this approach is that it can take non-normally distributed observations, with differing variances, and develop a test that compares whether the two samples might be similar (Johnston and Faulkner, 2021). We used a two-sample bootstrap to test whether the median changes in vegetation greenness differed by offset and fuel treatment groups. This two-sample bootstrap test samples the different groups with 5,000 replications to estimate the true median differences. For observations like trends in vegetation greenness, where the observations may not be normally distributed, this is a non-parametric statistical test that can determine if there is a statistically significant difference between median values of the offset and fuel treatment groups and can estimate the magnitude of difference between groups. When the median differences for two groups contains zero, the two groups can be considered similar. To determine statistical significance, confidence intervals were constructed around the bootstrapped estimates at a 0.05 alpha level. Along with determining whether there is a statistically significant difference, this test also established the direction of differences between the two samples. A negative bootstrap differences indicate that the median IFM projects have vegetation greenness is lower than the median fuel treatments. A positive bootstrapped median difference indicates that the fuel treatments have improved vegetation greenness more than the IFM project.
Previous research has found that public datasets produced for tracking timber and non-timber management from the US Forest Service and Cal Fire over-represent areas of treatments (Knight et al., 2022). Since this work relied on Cal Fire’s CalMapper data to create the sampling boundaries for the fuel treatment areas, we assessed if there was a logical change in carbon levels based on our classification of ‘completed,’ ‘active,’ and ‘planned’ fuel treatments. We expected that planned projects that had not started by 2020, would have higher ladder and surface fuel estimates than active projects; the completed fuel treatments would have the lowest surface and ladder fuel estimates; and that the active fuel treatments would show the greatest reduction relative to the other two types of fuel treatments. Our validation check suggested that our sampling scheme using CalMAPPER was accurately capturing changes in fuel loads consistent with active, complete, and planned fuel treatments. Active fuel treatments tend to have more negative changes in fuels than the completed and planned fuel treatments (Supplementary Table 2).
Improved Forest Management projects have higher starting levels of fuels than the forest where fuel treatments were completed or were active during the observation period. Based on the 2016 CAFO fuel estimates, IFM projects have the higher average ladder, canopy cover, canopy height, and canopy layer count than the fuel treatments that were active after 2016 or completed by 2016 in both the Southern Cascade and North Coast region (Figures 2A–E).
For a fuel metric like canopy cover or canopy height, it makes sense that forests identified for carbon management would have high forest coverage, as tree height is correlated with tree size and dense forests would have higher canopy cover. The low canopy cover for fuel treatments in the Southern Cascade region, as low as 25% for Active fuel treatments, could be attributed to the higher number of fuel treatments that are linear, and possibly adjacent to roads that have reduced canopy cover. There are differences in the 2016 estimates of the vertical structure and canopy conditions estimates for forests where IFM projects are located relative to fuel treatments that to assess more accurately required limiting these differences.
The pre-regression matching improved the covariate balance between the control and treatment groups (Supplementary Figure 1). There remained some differences for the temperature and precipitation variables that our method for matching could not resolve. Because the matching did improve the mean differences in the relevant 2016 fuel estimate (e.g., cc_2016 for canopy cover in 2016), which our boxplots indicated were different between the IFM projects and fuel treatment groups, we felt that this matched dataset effectively reduced some bias between treatment and control observations. The pre-regression matching reduced the dataset from 24,051 observations to 11,378 observations with equal number of treatment and control observations. There were too few observations from the planned fuel treatments in the Southern Cascade region to make inference, therefore this group is not reported in the linear regressions.
IFM projects have greater predicted changes in vertical structure and canopy composition than the fuel treatments (Figure 3). This indicates that even controlling for differences in biophysical factors that impact forest growth and the starting fuel loads, the observed changes in forest structure on IFM projects is increasing faster than the forests that are receiving or will receive fuel treatments. IFM projects have changes greater than zero for ladder and surface fuels that indicate increasing fuel amounts. However, the IFM projects are also the only group that had a predicted positive increase for forest height and canopy cover, which both would be consistent with increasing carbon stored in live tree carbon. Because there are no overlapping standard errors between the IFM projects and the active fuel treatments for any of the metrics, we conclude IFM projects are not reducing fuels like forests that are receiving fuel treatments. Because there was spatial autocorrelation in the linear regression residuals, the standard errors may be larger than estimated, meaning that the finding of significant difference may be overstated and therefore some caution should be applied to interpreting these results.
Figure 3. Estimated change between 2016 to 2020 for (A) ladder fuel, (B) surface fuel, (C) canopy cover, (D) canopy height, (E) canopy layer count, (F) canopy base height between offsets and fuel treatments completed prior to 2016. These graphs should the predicted change from the matched regression using the 2016 variable (A–F), respectively, for each graph.
In the North Coast region, where there were enough observations also evaluate planned fuel treatments, predictions of IFM projects changes were the closest to planned fuel treatments for changes in canopy base height and changes in canopy layer count. Of the predicted values from the fuel treatments, IFM projects did have predicted changes closer to the observations on planned fuel treatments. In the Southern Cascade region, IFM projects have predicted values closer to completed fuel projects.
The average trend for the Kendall-Mann test of vegetation trends in greenness are reported for the IFM projects and fuel treatments for the two regions (Table 2). IFM projects in the North Coast had a negative trend in vegetation, expressed in a spectral EVI trend change of −29.10 (unitless). The negative value indicates a loss in vegetation greenness or productivity. The two IFM projects in the Southern Cascade region were the only group in our sample that had a positive trend in vegetation greenness, of 9.94 (unitless).
Table 2. Average Kendall-Mann Trend in vegetation and the number of samples within each of the categories.
In the North Coast region, the completed fuel treatment in the North Coast had the most negative mean vegetation greenness trend. This could be from delayed treatment effects from prescribed fire and fuel management after management. Completed fuel treatments were omitted from the Southern Cascade due to too few observations. In the Southern Cascade region, it is the planned fuel treatments that had the most negative value relative to the active and the IFM projects. For both regions, active fuel treatments cause a decline in vegetation greenness, indicated in the average negative vegetation trend (Table 2). This could be due to the vegetation removal or rearrangement associated with fuel treatments.
To further contextualize these observed trends in IFM projects relative to other forests, the bootstrap tests compare the vegetation trends on IFM projects with forests receiving fuel treatments. Active fuel treatments have similar median trends in vegetation to the IFM projects in both regions of California. The bootstrapped median difference for the IFM and active fuel treatments had a 95% confidence interval that contain zero, meaning the median differences in greenness trends between IFM projects and active fuel treatments are zero (Figures 4C–D).
Figure 4. Bootstrapped median differences in the trend of EVI between samples from offsets and fuel treatments between the North Coast (A,C,E) and Southern Cascade (B,D) regions.
The largest difference in vegetation trends is in the Southern Cascade region between planned fuel treatments to IFM projects at 73 (unitless). This is consistent with the mean values, because the two IFM projects in this region had the highest overall positive greenness trend and the planned fuel treatments had the most negative fuel treatment (Table 2). Both the sample mean trend for IFM and planned fuel treatments in the North Coast are negative. Our estimated median difference being positive 20 (unitless) indicates IFM projects have a less negative trend in vegetation greenness relative to the planned fuel treatments.
Completed fuel treatments in the North Coast had median vegetation trend that was −55 relative to the IFM projects mean vegetation trend suggesting that there is at least a temporary decline in vegetation greenness following fuel reductions. This could be the byproduct of our unique observation window or could be capturing some delayed ecological stress following management. Our results can only suggest a difference in the distributions and the relative direction of difference.
Previous research has used tools like modeling and field experiments to demonstrate that continuing to increase carbon in aboveground biomass will come with tradeoffs for long-term carbon management (Liang et al., 2018; Foster et al., 2020; Bernal et al., 2022). Using our remote sensing techniques, we found additional evidence that tradeoffs between mitigating short-term fire risk and maintaining elevated carbon stocks for carbon crediting are already present in California IFM carbon projects. We found that IFM offset projects had higher lidar-estimated fuel loads in 2016 than lands receiving fuel treatments under California’s Forest Health program, indicating that these forests have a high starting level of fuels. Between 2016 and 2020, these IFM projects did not reduce fuel loads in ways consistent with active or completed fuel treatments for ladder, surface, canopy cover, canopy height, canopy layer count, or canopy base height. This suggests that these forests are being managed distinctly from forests that are receiving fuel treatments, even controlling for biophysical differences between these forests. From our vegetation greenness trend analysis, we found the management between IFM projects and active fuel treatments are having similar outcomes on vegetation (Figure 4). IFM projects are on average not reducing fuel loads or altering canopy cover and canopy height, yet they are having reductions in vegetation greenness as if they were. Another reason why vegetation can lose greenness is a decline in fuel moisture, which also results in increased flammability (McEvoy et al., 2020). If these loses in vegetation greenness are from declining vegetation moisture, then the management occurring on IFM projects could be increasing fire risk through both not reducing fuel loads and increasing vegetation flammability.
Based on the first decade of the compliance offset market, our results suggest forests managed as offsets promote less beneficial outcomes for fire hazard reduction and vegetation greenness. An abundance of research supports the idea that management should be scaled up in these types of forests—both for forest resilience and for long term carbon storage, relevant for climate change mitigation (Liang et al., 2018; North et al., 2022). And yet, when this climate-focused policy has been applied to forests, we do not observe it incentivizing the average project to implement more fuels management. Only six of the 40 projects in California had qualified fuel treatments based on IFM project documentation submitted to carbon credit registries.
CARB’s forest offset protocol could create perverse incentives for landowners to maximize carbon today rather than prioritizing managing for stable carbon. The protocol generates credits at the start of the project equal to the demonstrated carbon stock above a theoretical baseline. Subsequent payments, represented as different vintages, are based on the additional growth. IFM projects may perform fuel treatment, but the protocol creates financial incentives not to do so. Any removal of onsite carbon beyond incremental growth from the previous inventory constitutes a reversal requiring the landowner to replace the reversed credits. Even when removal of surface fuel or small diameter trees does not disrupt the carbon accounting enough to be counted as a reversal (above the growth amount), it could reduce the credits a project receives and thus project owners may be encouraged to delay fuel treatments until the project is outside of credit generating years. Delaying or forgoing fuel treatments is not aligned with optimizing carbon stability in California (Liang et al., 2018). Forest management for climate change should be aligned with other policies promoting more stable carbon management.
Creating incentives for maximizing aboveground carbon stored in forest biomass allows forest managers to shift economics toward delaying harvests. Based on registry submitted data, eight projects in California demonstrate increasing aboveground carbon through increasing rotation length. While delaying timber harvests is not mutually exclusive with no management occurring in these forests, some of these projects have no other management planned at the time of project documentation submission. Within the existing California IFM offset program, we have identified five potential ways policymakers could increase fuel management within IFM projects in fire prone regions. Each of these outlined approaches is accompanied by research questions to address whether our proposed idea would be able to deliver the desired outcomes of increasing the fire risk reduction on IFM projects in fire prone ecosystems.
The first approach is creating further incentives in buffer pool reductions for fuel break maintenance. As previously discussed, fuel treatments are currently incentivized via a reduction in the percentage of credits a project contributes to the buffer pool. But we observed that IFM projects have high starting levels of fuels in their vertical fuel structure and most of the projects do not show signs of fuel reduction. It could be possible that an incentive greater than 2% on the project’s buffer pool contribution could drive more fire risk mitigation. Because the most carbon a project receives tends to be in the first year of accounting, a qualified fuel treatment approved at the start of the project would reduce the number of credits a project contributes to account for a century of natural disturbance insurance. If the 2% reduction in buffer pool contribution is not enough to offset this loss of credits generated, then this may be an area of policy improvement. Further research could study whether there are differences by landowners in their approach to management and how these approaches interact with disturbance risk. If only some types of landowners complete qualified fuel treatments, it could be useful for identifying other barriers to management by other landowner groups. Finally, climate driven disturbances are expected to undermine the effectiveness of forests as natural climate solutions (Anderegg et al., 2020). In order to reconcile the need for both deeper buffer pools with more compelling incentives, for managing this climate driven disturbance risk, a blended policy approach that caters to early alignment with fire risk management goals while backloading buffer pool requirements could be investigated.
Only in regions where there is moderate to high fire risk would it make sense for projects to proactively be treating fuels. Yet all regions of the contribute equal proportions of credits into the buffer pool and receive equal incentives to reduce disturbance risk. The buffer pool creates adverse selection like any insurance pool. Each landowner benefits from selling carbon credits, but the risk of reversal is spread out among all landowners.
This brings us to our second recommendation: qualified fuel treatments could be an eligibility requirement for IFM projects in moderate or high fire risk regions. There is not currently a requirement for projects to indicate on their project submission documents whether they believe fuel management will be part of the carbon management. At a minimum, IFM projects in fire prone regions could be required to include a statement about why they are not managing fuel explicitly as part of their carbon management strategy.
If changes directly to the carbon crediting standards are not feasible, policy could also target fuel treatments more directly. Our third and fourth recommendations include expansion of fuel treatments on or surrounding IFM projects. Instead of using the buffer pool to incentivize fuel reductions after they occur, our third proposed approach could use grants to fund landowners more directly in the IFM program to implement fuel reductions. The proceeds generated from the cap-and-trade auctions are deposited to the Greenhouse Gas Reduction Fund which supports California Climate Investments (CCI). Cal Fire’s Fire Prevention Grants and the Forest Health Program are supported by the CCI (California Air Resources Board, 2022). One possible approach would be proactively supporting landowners managing carbon pools instead of an insurance reduction after the cost of fuel treatment has been incurred.
The fourth recommendation could encourage the existing Cal Fire fuel treatments to surround IFM projects. If maintaining elevated carbon stocks on IFM projects is still the direction market administrators and forest managers wish to pursue, strategically placed landscape fuel treatments surrounding IFM projects could decrease fire severity and promote forest recovery, helping carbon sinks (Tubbesing et al., 2019). We already observe IFM projects and Cal Fire fuel treatments in proximity, sometimes even sharing project boundary borders. The efficacy of strategically placed adjacent fuel treatments in protecting carbon within IFM projects could be further investigated for the regions IFM projects occur. If strategically placed fuel treatments can increase the stable amount of aboveground carbon stored on the IFM projects, these co-occurring IFM projects and fuel treatments could be scaled up. While the idea of strategically placed fuel treatments is not novel, determining whether adjacent fuel treatments can effectively change fire behavior within IFM projects will need to be tested.
Finally, we would be remiss to not mention that more dramatic changes to carbon accounting could also shift the management occurring on IFM projects toward more proactive fire risk mitigation. For example, carbon credit-generating projects in fire prone regions might be evaluated not on total aboveground live carbon, but on the amount of carbon predicted to survive a fire disturbance. Such “avoided wildfire emissions” methodologies are already being developed by carbon offset registries like Climate Action Reserve (Climate Action Reserve, 2022). Another accounting change could reevaluate how the carbon sequestered in wood products is incorporated in carbon crediting. Better incorporating woody products could better align forest and carbon management outcomes (Cabiyo et al., 2021). An example of this could be using woody residue from fuel treatments for biofuel production, offsetting use of fossil fuels in transportation and expanding a market for non-timber wood products (Sanchez et al., 2021). Further research could explore the implications of different changes in carbon accounting and whether such changes would be compatible with current carbon offset protocols.
Our research focuses on California’s forest carbon management and the tensions between two state agencies creating programs for storing carbon today and creating stable carbon storage. While the particulars of this case are context specific, there are lessons applicable to broader geographies that incorporate natural climate solutions into climate policies, as the ecosystems that comprise these solutions are changing. Wildfires have struck managed forest in many areas around the world, and improved management to limit these fires could work in concert to increase carbon on the landscape. In California’s case, CARB’s IFM forest offset protocol officially addresses wildfire and other natural disturbance risks via the buffer pool and attempts to promote risk reduction via a reduction on a project buffer pool contribution. Yet our research finds that most of the projects are not managing fuels, while previous research has found that CARB’s buffer pool is undercapitalized for the expected carbon reversals (Badgley et al., 2022). This highlights a need to test assumptions of whether official policy intentions manifest on landscapes. The state of California is at the vanguard of the climate movement. Understanding shortcomings in their policies underscore the difficulty of designing programs that achieve their long-term objectives. Finding issues in an ambitious climate program should not be shocking, but failure of programs to adapt to research and new climate realities will result in programs that predictably fall short of their goals.
Climate change, fire exclusion, and antecedent disturbance contributes to the increasing presence of mega-fires as part of wildland fire regimes (Stephens et al., 2014). To disrupt this mega-fire triangle, fuel treatments or forest restoration can disrupt the forest conditions created from fire exclusion and antecedent disturbance in frequent-fire adapted forests. While policymakers can support funding and reducing frictions to implementing these vegetation management projects, reducing greenhouse gas emissions could also help break this mega-fire triangle. It is striking that those forests incorporated in cap-and-trade carbon markets enable others to emit greenhouse gases that will worsen the effect of climate change, while simultaneously expecting the forests that are being used to offset those emissions to somehow be immune to increasing threat from climate change. The current IFM offset protocol works counter to long term carbon stability and the goals of other California programs focused on disrupting this mega-fire triangle. To better align forest management with climate policy, the current CARB offset program must change the way it manages wildfire risk.
The raw data supporting the conclusions of this article will be made available by the authors, without undue reservation.
CH: data collection, analysis, and interpretation of results. All authors contributed to the study conception and design, involved in draft manuscript preparation, reviewed the results, and approved the final version of the manuscript.
We also thank Lotusland Investment Holdings, Inc., Bohn Valley, Inc., for a financial gift which supported the publication fees. Lotusland Investment Holdings, Inc., and Bohn Valley, Inc., were not involved in the study design, collection, analysis, interpretation of data, the writing of this article, or the decision to submit it for publication.
We thank Jared Stapp for sharing the spatial boundaries of IFM projects in California.
The authors declare that the research was conducted in the absence of any commercial or financial relationships that could be construed as a potential conflict of interest.
All claims expressed in this article are solely those of the authors and do not necessarily represent those of their affiliated organizations, or those of the publisher, the editors and the reviewers. Any product that may be evaluated in this article, or claim that may be made by its manufacturer, is not guaranteed or endorsed by the publisher.
The Supplementary Material for this article can be found online at: https://www.frontiersin.org/articles/10.3389/ffgc.2022.957189/full#supplementary-material
Abatzoglou, J. T. (2013). Development of gridded surface meteorological data for ecological applications and modelling. Int. J. Climatol. 33, 121–131. doi: 10.1002/joc.3413
Anderegg, W. R. L., Trugman, A. T., Badgley, G., Anderson, C. M., Bartuska, A., Ciais, P., et al. (2020). Climate-driven risks to the climate mitigation potential of forests. Science 368:eaaz7005. doi: 10.1126/science.aaz7005
Austin, P. C. (2011). An introduction to propensity score methods for reducing the effects of confounding in observational studies. Multivariate Behav. Res. 46, 399–424. doi: 10.1080/00273171.2011.568786
Badgley, G., Chay, F., Chegwidden, O. S., Hamman, J. J., Freeman, J., and Cullenward, D. (2022). California’s forest carbon offsets buffer pool is severely undercapitalized. Front. For. Glob. Change 5:930426. doi: 10.3389/ffgc.2022.930426
Bernal, A. A., Stephens, S. L., Collins, B. M., and Battles, J. J. (2022). Biomass stocks in California’s fire-prone forests: Mismatch in ecology and policy. Environ. Res. Lett. 17:044047. doi: 10.1088/1748-9326/ac576a
Cabiyo, B., Fried, J. S., Collins, B. M., Stewart, W., Wong, J., and Sanchez, D. L. (2021). Innovative wood use can enable carbon-beneficial forest management in California. Proc. Natl. Acad. Sci. U.S.A. 118:e2019073118. doi: 10.1073/pnas.2019073118
California Department of Forestry and Fire Protection [CAL FIRE] (2022a). Fuel reduction. Available online at: https://www.fire.ca.gov/fuels-reduction/ (accessed May 1, 2022).
California Department of Forestry and Fire Protection [CAL FIRE] (2022b). CalMAPPER forest and fuel management projects. Available online at: https://www.arcgis.com/home/item.html?id=4a73accda8314ccd9442d365bf32f51d (accessed April 12, 2022).
California Air Resources Board, (2011). Compliance Offset Protocol U.S. Forest Projects. Available online at: http://www.arb.ca.gov/regact/2014/capandtrade14/ctusforestprojectsprotocol.pdf (accessed April 12, 2022).
California Air Resources Board (2015). Compliance Offset Protocol U.S. Forest Projects. Available online at: https://www.arb.ca.gov/cc/capandtrade/protocols/usforest/forestprotocol2015.pdf (accessed May 7, 2019).
California Air Resources Board (2020). Greenhouse Gas Emissions of Contemporary Wildfire, Prescribed Fire, and Forest Management Activities. Available online at: https://ww3.arb.ca.gov/cc/inventory/pubs/ca_ghg_wildfire_forestmanagement.pdf (accessed April 12, 2022).
California Air Resources Board (2022). California climate investments 2021 Annual Report: Cap-and-Trade Auction Proceeds. Available online at: https://ww2.arb.ca.gov/sites/default/files/auction-proceeds/cci_annual_report_2022.pdf
California Air Resources Board Offset Credit Issuance Table, (2022). California Air Resources Board. Available online at: https://ww2.arb.ca.gov/our-work/programs/compliance-offset-program (accessed April 12, 2022).
California Forest Observatory (2020). Data description: Vegetation structure and fuels. Salo Sciences, Inc. Available online at: https://salo.ai/assets/pdf/Forest-Observatory-Data-Description.pdf (accessed April 12, 2022).
Chen, Y. (2013). New approaches for calculating moran’s index of spatial autocorrelation. PLoS One 8:e0068336. doi: 10.1371/journal.pone.0068336
Climate Action Reserve (2022). Avoided Wildfire Emissions Forecast Methodology in Development. Available Online at: https://climateforward.org/program/methodologies/avoided-wildfire-emissions/ (accessed April 12, 2022).
Clinton, N. (2022). Non-parametric trend analysis. Available online at: https://developers.google.com/earth-engine/tutorials/community/nonparametric-trends (accessed April 12, 2022)
Collins, B. M., Fry, D. L., Lydersen, J. M., Everett, R., and Stephens, S. L. (2017). Impacts of different land management histories on forest change. Ecol. Appl. 27, 2475–2486. doi: 10.1002/eap.1622
Coop, J. D., Parks, S. A., Stevens-Rumann, C. S., Crausbay, S. D., Higuera, P. E., Hurteau, M. D., et al. (2020). Wildfire-driven forest conversion in Western North American Landscapes. BioScience 70, 659–673. doi: 10.1093/biosci/biaa061
Falk, D. A., van Mantgem, P. J., Keeley, J. E., Gregg, R. M., Guiterman, C. H., Tepley, A. J., et al. (2022). Mechanisms of forest resilience. For. Ecol. Manage. 512:120129. doi: 10.1016/j.foreco.2022.120129
Farr, T. G., Rosen, P. A., Caro, E., Crippen, R., Duren, R., Hensley, S., et al. (2007). The shuttle radar topography mission. Rev. Geophys. 45:RG2004. doi: 10.1029/2005RG000183
Foster, D. E., Battles, J. J., Collins, B. M., York, R. A., and Stephens, S. L. (2020). Potential wildfire and carbon stability in frequent-fire forests in the Sierra Nevada: Trade-offs from a long-term study. Ecosphere 11:e03198. doi: 10.1002/ecs2.3198
Gonzalez, P., Battles, J. J., Collins, B. M., Robards, T., and Saah, D. S. (2015). Aboveground live carbon stock changes of California wildland ecosystems, 2001-2010. For. Ecol. Manage. 348, 68–77. doi: 10.1016/j.foreco.2015.03.040
Hurteau, M. D., and Brooks, M. L. (2011). Short- and long-term effects of fire on carbon in US dry temperate forest systems. BioScience 61, 139–146. doi: 10.1525/bio.2011.61.2.9
Hurteau, M. D., Koch, G. W., and Hungate, B. A. (2008). Carbon protection and fire risk reduction: Toward a full accounting of forest carbon offsets. Front. Ecol. Environ. 6, 493–498. doi: 10.1890/070187
Jarron, L. R., Coops, N. C., MacKenzie, W. H., Tompalski, P., and Dykstra, P. (2020). Detection of sub-canopy forest structure using airborne LiDAR. Remote Sens. Environ. 244:111770. doi: 10.1016/j.rse.2020.111770
Johnston, M. G., and Faulkner, C. (2021). A bootstrap approach is a superior statistical method for the comparison of non-normal data with differing variances. New Phytol. 230, 23–26. doi: 10.1111/nph.17159
Knight, C. A., Tompkins, R. E., Wang, J. A., York, R., Goulden, M. L., and Battles, J. J. (2022). Accurate tracking of forest activity key to multi-jurisdictional management goals: A case study in California. J. Environ. Manage. 302:114083. doi: 10.1016/j.jenvman.2021.114083
LANDFIRE (2001). Existing Vegetation Type Layer. Available online at: http://www.landfire/viewer (accessed April 12, 2022).
Liang, S., Hurteau, M. D., and Westerling, A. L. (2018). Large-scale restoration increases carbon stability under projected climate and wildfire regimes. Front. Ecol. Environ. 16, 207–212. doi: 10.1002/fee.1791
Lutz, J. A., Furniss, T. J., Johnson, D. J., Davies, S. J., Allen, D., Alonso, A., et al. (2018). Global importance of large-diameter trees. Glob. Ecol. Biogeogr. 27, 849–864. doi: 10.1111/geb.12747
McEvoy, D. J., Pierce, D. W., Kalansky, J. F., Cayan, D. R., and Abatzoglou, J. T. (2020). Projected changes in reference evapotranspiration in California and Nevada: Implications for drought and Wildland Fire Danger. Earths Future 8, 1–17. doi: 10.1029/2020EF001736
McIntyre, P. J., Thorne, J. H., Dolanc, C. R., Flint, A. L., Flint, L. E., Kelly, M., et al. (2015). Twentieth-century shifts in forest structure in California: Denser forests, smaller trees, and increased dominance of oaks. Proc. Natl. Acad. Sci. U.S.A. 112, 1458–1463. doi: 10.1073/pnas.1410186112
Mildrexler, D. J., Berner, L. T., Law, B. E., Birdsey, R. A., and Moomaw, W. R. (2020). Large trees dominate carbon storage in forests east of the cascade crest in the United States Pacific Northwest. Front. For. Glob. Change 3, 1–15. doi: 10.3389/ffgc.2020.594274
Mutlu, M., Popescu, S., Stripling, C., and Spencer, T. (2008). Mapping surface fuel models using lidar and multispectral data fusion for fire behavior. Remote Sens. Environ. 112, 274–285. doi: 10.1016/j.rse.2007.05.005
North, M., Hurteau, M., and Innes, J. (2009). Fire suppression and fuels treatment effects on mixed-conifer carbon stocks and emissions. Ecol. Appl. 19, 1385–1396. doi: 10.1890/08-1173.1
North, M. P., and Hurteau, M. D. (2011). High-severity wildfire effects on carbon stocks and emissions in fuels treated and untreated forest. For. Ecol. Manage. 261, 1115–1120. doi: 10.1016/j.foreco.2010.12.039
North, M. P., Tompkins, R. E., Bernal, A. A., Collins, B. M., Stephens, S. L., and York, R. A. (2022). Operational resilience in western US frequent-fire forests. For. Ecol. Manage. 507:120004. doi: 10.1016/j.foreco.2021.120004
Ogaya, R., Barbeta, A., Başnou, C., and Peñuelas, J. (2015). Satellite data as indicators of tree biomass growth and forest dieback in a Mediterranean holm oak forest. Ann. For. Sci. 72, 135–144. doi: 10.1007/s13595-014-0408-y
Roberts, D. A., Dennison, P. E., Peterson, S., Sweeney, S., and Rechel, J. (2006). Evaluation of Airborne Visible/Infrared Imaging Spectrometer (AVIRIS) and Moderate Resolution Imaging Spectrometer (MODIS) measures of live fuel moisture and fuel condition in a shrubland ecosystem in southern California. J. Geophys. Res. Biogeosci. 111:B12S35. doi: 10.1029/2005JG000113
Safford, H. D., and Stevens, J. T. (2017). Natural range of variation for yellow pine and mixed-conifer forests in the Sierra Nevada, southern Cascades, and Modoc and Inyo National Forests, California, USA. General Technical Report - Pacific Southwest research station, USDA Forest Service. PSW-GTR-256, 229. Washington, DC: USDA.
Sanchez, D. L., Fingerman, K., Herbert, C., and Uden, S. (2021). Policy Options for Deep Decarbonization and Wood Utilization in California’s Low Carbon Fuel Standard. Front. Clim. 3:665778. doi: 10.3389/fclim.2021.665778
Sims, D. A., Rahman, A. F., Cordova, V. D., El-Masri, B. Z., Baldocchi, D. D., Flanagan, L. B., et al. (2006). On the use of MODIS EVI to assess gross primary productivity of North American ecosystems. J. Geophys. Res. Biogeosci. 111:G04015. doi: 10.1029/2006JG000162
Skinner, C. N., and Taylor, A. H. (2006). “South cascades bioregion,” in Fire in California’s bioregions, eds N. G. Sugihara, J. W. van Wagtendonk, J. Fites-Kaufman, K. E. Shaffer, and A. E. Thode (Berkeley, CA: University of California Press), 195–224.
Skinner, C. N., Taylor, A. H., and Agee, J. K. (2006). “Klamath mountains bioregion,” in Fire in California’s ecosystems, eds N. G. Sugihara, J. W. van Wagtendonk, J. Fites-Kaufman, K. E Shaffer, and A. E. Thode (Berkeley, CA: University of California Press), 170–194. doi: 10.1525/california/9780520246058.003.0009
Stapp, J. R. (2022). Early indications of effectiveness in California’s forest offset program. Unpublished doctoral dissertation. Berkeley, CA: University of California.
State Board of Forestry and Fire Protection and California Department of Forestry and Fire Protection [CAL FIRE] (2018). 2018 Strategic Fire Plan for California. Available online at: https://osfm.fire.ca.gov/media/5590/2018-strategic-fire-plan-approved-08_22_18.pdf (accessed April 12, 2022).
Stephens, S. L., Battaglia, M. A., Churchill, D. J., Collins, B. M., Coppoletta, M., Hoffman, C. M., et al. (2021). Forest restoration and fuels reduction: Convergent or divergent? BioScience 71, 85–101. doi: 10.1093/biosci/biaa134
Stephens, S. L., Bernal, A. A., Collins, B. M., Finney, M. A., Lautenberger, C., and Saah, D. (2022). Forest Ecology and Management Mass fire behavior created by extensive tree mortality and high tree density not predicted by operational fire behavior models in the southern Sierra Nevada. For. Ecol. Manage. 518:120258. doi: 10.1016/j.foreco.2022.120258
Stephens, S. L., Burrows, N., Buyantuyev, A., Gray, R. W., Keane, R. E., Kubian, R., et al. (2014). Temperate and boreal forest mega-fires: Characteristics and challenges. Front. Ecol. Environ. 12, 115–122. doi: 10.1890/120332
Stephens, S. L., Collins, B. M., Fettig, C. J., Finney, M. A., Hoffman, C. M., Knapp, E. E., et al. (2018). Drought, tree mortality, and wildfire in forests adapted to frequent fire. BioScience 68, 77–88. doi: 10.1093/biosci/bix146
Stephens, S. L., Westerling, A. L. R., Hurteau, M. D., Peery, M. Z., Schultz, C. A., and Thompson, S. (2020). Fire and climate change: Conserving seasonally dry forests is still possible. Front. Ecol. Environ. 18, 354–360. doi: 10.1002/fee.2218
Stephenson, N. L., Das, A. J., Condit, R., Russo, S. E., Baker, P. J., Beckman, N. G., et al. (2014). Rate of tree carbon accumulation increases continuously with tree size. Nature 507, 90–93. doi: 10.1038/nature12914
Su, Y., Guo, Q., Collins, B. M., Fry, D. L., Hu, T., and Kelly, M. (2016). Forest fuel treatment detection using multi-temporal airborne Lidar data and high-resolution aerial imagery: A case study in the Sierra Nevada Mountains, California. Int. J. Remote Sens. 37, 3322–3345. doi: 10.1080/01431161.2016.1196842
Tubbesing, C. L., Fry, D. L., Roller, G. B., Collins, B. M., Fedorova, V. A., Stephens, S. L., et al. (2019). Strategically placed landscape fuel treatments decrease fire severity and promote recovery in the northern Sierra Nevada. For. Ecol. Manage. 436, 45–55. doi: 10.1016/j.foreco.2019.01.010
Westerling, A. L., Bryant, B. P., Preisler, H. K., Holmes, T. P., Hidalgo, H. G., Das, T., et al. (2011). Climate change and growth scenarios for California wildfire. Clim. Change 109, 445–463. doi: 10.1007/s10584-011-0329-9
Wiedinmyer, C., and Hurteau, M. D. (2010). Prescribed fire as a means of reducing forest carbon emissions in the Western United States. Environ. Sci. Technol. 44, 1926–1932. doi: 10.1021/es902455e
Keywords: wildfire, forest carbon, fuel management, Improved Forest Management, offsets, remote sensing
Citation: Herbert C, Haya BK, Stephens SL and Butsic V (2022) Managing nature-based solutions in fire-prone ecosystems: Competing management objectives in California forests evaluated at a landscape scale. Front. For. Glob. Change 5:957189. doi: 10.3389/ffgc.2022.957189
Received: 30 May 2022; Accepted: 20 September 2022;
Published: 19 October 2022.
Edited by:
Breno Pietracci, Environmental Defense Fund, United StatesReviewed by:
Marcelo Arturi, National University of La Plata, ArgentinaCopyright © 2022 Herbert, Haya, Stephens and Butsic. This is an open-access article distributed under the terms of the Creative Commons Attribution License (CC BY). The use, distribution or reproduction in other forums is permitted, provided the original author(s) and the copyright owner(s) are credited and that the original publication in this journal is cited, in accordance with accepted academic practice. No use, distribution or reproduction is permitted which does not comply with these terms.
*Correspondence: Claudia Herbert, Y2xhdWRpYWhlcmJlcnRAYmVya2VsZXkuZWR1
Disclaimer: All claims expressed in this article are solely those of the authors and do not necessarily represent those of their affiliated organizations, or those of the publisher, the editors and the reviewers. Any product that may be evaluated in this article or claim that may be made by its manufacturer is not guaranteed or endorsed by the publisher.
Research integrity at Frontiers
Learn more about the work of our research integrity team to safeguard the quality of each article we publish.