- 1Department of Forest Botany and Tree Physiology, University of Göttingen, Göttingen, Germany
- 2Biological Sciences Department, University of Zambia, Lusaka, Zambia
- 3Soil Ecology, University of Freiburg, Freiburg, Germany
- 4Centre of Biodiversity and Sustainable Land Use, University of Göttingen, Göttingen, Germany
Fine roots are crucial for water and nutrient acquisition in plants; yet it is unknown how nutrient inputs and soil fertility in forests influence fine root biomass seasonal trajectories. Here, we hypothesized that standing fine root biomass increases with addition of a limited resource and shows different seasonal patterns depending on nutrient availability and phenology. We further hypothesized that the influence of climate is greater in the organic layer, causing larger responses of root biomass to climate in the organic layer and stronger responses to nutrient changes in mineral soil. We conducted our study in three European beech (Fagus sylvatica) forests representing a soil fertility gradient with high, medium, and low phosphorus (P) contents. A fully factorial fertilization regime with N and P was applied at each forest site. To test our hypotheses, we conducted soil coring in spring and fall for 2.5 years. Soil cores were fractionated into organic layer (Oe + Oa layer) and mineral soil (A horizon) and used to determine fine root biomass, soil pH and moisture, total concentrations of soil and root nutrients (basic cations, micronutrients, S, P, N, C), soluble concentrations of soil and root P. Fine root biomass in the mineral soil at the forest site with the lowest soil P content increased in response to P addition. Pheno-seasonal changes caused increases in soil P and N in spring and opposing cycling of biomass and fine root labile P contents at the P-medium and P-high sites. Contrary to our expectation, we observed stronger climatic effects on fine root biomass in the mineral soil, whereas soil moisture was more important in explaining fine root biomass variation in the organic layer. Our results show that seasonal patterns of fine root biomass are dependent on the stratification of environmental factors with soil depth and imply negative consequences for fine root biomass in the organic soil layer due to acute soil water content fluctuations and climate change effects in the mineral horizon due to long-term precipitation changes.
Introduction
In many temperate forest ecosystems, nitrogen (N) and phosphorus (P) limit tree growth (Vitousek et al., 2010; Du et al., 2020). Increased anthropogenic N deposition (Galloway et al., 2008) can stimulate net primary productivity in N-limited forests (Du and De Vries, 2018). Enhanced productivity requires enhanced supply with mineral nutrients and thus, can shift tree nutrition from N to P limitation (Peñuelas et al., 2013; Jonard et al., 2015). Therefore, deterioration of P nutrition might be a consequence of enhanced N availabilities (Talkner et al., 2015). Increased availabilities of both P and N often had synergistic, positive effects on tree species, while effects of single nutrient applications on tree growth were less clear (Elser et al., 2007).
Tree fine roots play a central role in nutrient and water uptake (Vogt et al., 1996; McCormack et al., 2015). Fine roots exhibit distinct seasonal trends of production and mortality (Brassard et al., 2009). Further, root production is highly plastic, responding to soil nutrient and water availabilities (Nadelhoffer, 2000; Brassard et al., 2009; Delpierre et al., 2016; Leuschner, 2020). Field observations in northern forests and greenhouse studies with tree seedlings showed that an increase in N decreased the root-to-shoot ratio (Högberg, 2007; Meyer-Grünefeldt et al., 2015; Dziedek et al., 2017), specifically due to decreases in the fine root biomass (Li et al., 2016). Since fine roots are key to soil foraging, the decrease in fine root biomass along with increased aboveground biomass is thought to increase drought sensitivity of trees (Högberg et al., 1993). However, some tree species may compensate this risk by enhanced fine root production under drought stress (Leuschner, 2020). Thus, climate variables and nutrients are both important drivers of root biomass (Vogt et al., 1996; Gao et al., 2021) but the relative importance of these factors in soils with strongly different nutrient pools is not yet fully understood.
In Central Europe, European beech (Fagus sylvatica L.) is a dominant forest tree species stocking on a vast range of soil types (Leuschner, 2020). In beech forests, fine root biomass and turnover are highly variable, depending on the environmental conditions and soil depth (Kirfel et al., 2019; Meller et al., 2020). Climatic factors such as repeated drought events (Zwetsloot and Bauerle, 2021) or soil warming (Kengdo et al., 2022) stimulated fine root biomass production but did not result in significant increases in standing fine root biomass. However, Lwila et al. (2021) found higher fine root biomass at drier and nutrient poor sites than in moist and nutrient rich beech stands. Lang et al. (2017) reported increasing fine root biomass of beech in the top soil layers with decreasing soil P stocks in the mineral horizon. Clausing and Polle (2020) showed that organic and mineral soil layers played crucial but divergent roles for P uptake under high and low P availabilities. In P poor soil, high fine root biomass and efficient mycorrhizal associations in the organic layer governed P uptake, whereas in P rich soil, P foraging was mainly taking place in the mineral top soil (Clausing and Polle, 2020). Although inverse relationships of soil P contents and root biomass have often been observed (Schneider et al., 2001; Lang et al., 2017), the importance of soil P, soil N or other mineral elements relative to climate and season for the dynamics of fine root biomass in temperate beech forests remains unknown. Since roots also constitute a significant carbon sink (Godbold and Brunner, 2007; Robinson, 2007, Whitehead, 2011; Reich et al., 2014), it is highly relevant to understand which variables are the major drivers for root biomass in beech forests.
In this study, we investigated temporal variation of P, N, and fine root biomass in the organic layer and mineral top soil of three beech forests differing in soil P stocks and climate. To assess the impact of enhanced P and N availabilities, we used P, N, or N + P fertilized as well as unfertilized plots in each forest (Clausing et al., 2021). Our previous study showed that the fertilizer treatments had only small effects on soil and root-associated fungal community composition (Clausing et al., 2021). Here, we focused on seasonal changes of standing root biomass and P contents in roots and soil after fertilization. We expected that P fertilization could increase the standing root biomass, when soil P availability was a limiting factor. In soils with high P availability, we expected no effect of P fertilization on root biomass but under high soil P availabilities, N might be a limiting resource. Therefore, we also fertilized with N and N + P to disentangle interactive effects of P and N on fine root biomass and root P and N contents. We hypothesized: (i) fertilization increases standing fine root biomass, if the added nutrient was severely growth-limiting and decreases root biomass under luxurious resource availabilities, because less investment in root biomass is required to support nutrient supply; (ii) the effects of P or N fertilization on standing root biomass are independent of seasonal changes of root biomass. (iii) Climate has a dominant effect on standing fine root biomass in the organic soil layer because of stronger impact of fluctuations in precipitation and temperature on fine roots close to forest floor than in the mineral soil. To test our hypotheses, we determined the trajectories of fine root biomass, soil and root nutrients by soil coring in spring and fall for 2.5 years and dissected the contributions of climate, fertilization, soil and root chemistry for fine root biomass by variance partitioning.
Materials and methods
Site description
This study was carried out in European beech (Fagus sylvatica L.) dominated forests that exhibit variation in soil P stocks and parent material (Lang et al., 2017). The P-low site (Unterlüss) is located in North German Plain (52°50′21.7″N, 10°16′2.3″E) on an elevation of 115 m above sea level (a.s.l) with mean annual temperature and precipitation of 8.0°C and 779 mm, respectively (Table 1). The beech forests at the P-low site are about 132 years old. The soil is sandy with sandy till parent material. The P-medium site (Mitterfels) in Bavarian Forest (48°58′34.1″N, 12°52′46.7″E) is located at an elevation of 1023 m above sea level (a.s.l). The average beech stand age is 131 years. The soil type is loamy sand with paragneiss parent material (Table 1). The P-medium site has mean annual temperature and precipitation of 4.9°C and 1200 mm, respectively. The P-high site (Bad Brückenau) is situated in “Bayerische Rhön” biosphere reservation (50°21′7.2″N, 9°55′44.5″E) on basalt parent material with silt clay soils. The elevation, mean annual temperature and precipitation at the P-high site are 809 m (a.s.l), 5.8°C and 1031 mm, respectively. The beech trees on the P-rich site have an age of 137 years (Table 1). Further climate, stand and soil characteristics of the study sites have been compiled in Table 1.
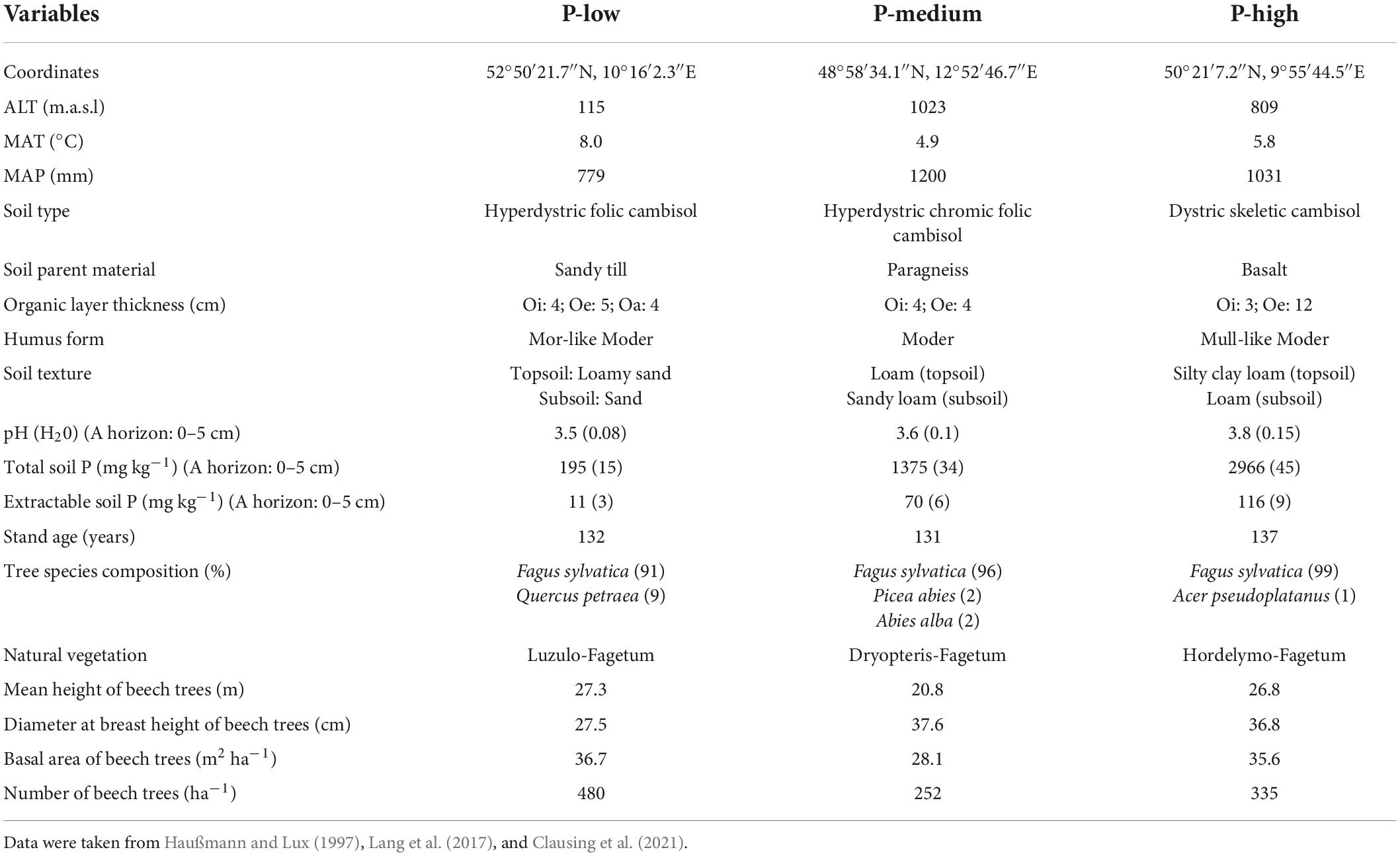
Table 1. Description of the climate (MAT, mean annual temperature; MAP, sum of annual precipitation), altitude (ALT), soil and stand characteristics of the study sites (Unterlüss: P-low; Mitterfels: P-medium; Bad Brückenau: P-high).
In each forest site, we set up an N, P, and P + N fertilization experiment (details: Clausing et al., 2021). Briefly, 12 study plots, each with an area of 400 m2 were established in each study site in summer 2016. The plots were treated with either nitrogen (N), phosphorous (P), combined nitrogen and phosphorus (P + N), or were kept as control (Con). Each treatment was replicated three times per study site, yielding a total of 36 plots. P was applied once in the late summer 2016 in form of KH2PO4 (50 kg P ha–1) to the P and P + N treatments. N was applied as NH4NO3 (30 kg N ha–1) to the N and P + N treatments at five time points (August 2016, May 2017, September–October 2017, April–May 2018, and September–October 2018). KCl was applied to the Con and N treatments to account for the K in the KH2PO4 fertilizer applied to the P and P + N treatments. The fertilizer was dissolved in tap water and sprayed on the soil surface of the plots.
Sampling
In each plot, soil samples were collected in fall 2016 (Start = 0 month), spring 2017 (9 months), fall 2017 (14 months), spring 2018 (20 months), and fall 2018 (25 months). The dates for the fertilization and soil sampling are provided in Supplementary Table 1. Since we were interested in the long-term effects of the fertilizer, we did not sample directly after fertilizer application to avoid the immediate impacts of P or N addition. Twelve soil cores (21 cm depth × 5.5 cm diameter) were randomly taken and separated into organic layer (OL) and mineral top soil (ML) as described by Clausing et al. (2021). Then, the 12 samples of the OL were pooled resulting in one sample per plot. The ML samples were also pooled. The samples were sieved (4 mm mesh size) and divided into bulk soil and roots and further sieved to separate fine roots (< 2 mm) and coarse roots (> 2 mm). The roots were washed with distilled water before weighing. Bulk soil, fine roots and coarse roots were transported in cooling boxes (approximately 4°C) to the laboratory for analysis.
Root biomass and soil water content
The weight of the fresh soil and root samples was determined. The samples were oven-dried (40°C) and weighed. The water content in the soil (WC_s) was calculated as:
The fine root biomass (FR) in the mineral soil was determined as:
The fine root biomass in the organic layer was determined correspondingly.
Soil properties and root chemistry
Dry soil samples were mixed with deionized water (1:2.5 ratio of soil to water) and shaking (1 h at 200 rpm). After sedimentation of particles, pH was measured with a pH meter (WTW, Weilheim, Germany).
To determine C and N contents aliquots of dry soil and root samples were milled using a ball mill (MN400, Retsch GmbH, Haan, Germany). Aliquots of about 2–12 mg (soil) and 1.5 mg (fine roots) were weighed into 4 mm × 6 mm tin capsules (IVA Analysentechnik, Meerbusch, Germany) on a microbalance (Model: Cubis MSA 2.7S-000-DM, Sartorius, Göttingen, Germany). Variation in C contents between the soil layers and among soil types led to overflow of the measuring unit in the spectrometer when the same sample weights were used for analyses. Therefore, we used different weights for the soil samples. We used the CN analyzer (vario MICRO cube CN analyzer, Elementar Analysensysteme, GmbH, Langenselbold Germany) or a mass spectrometer (Delta Plus, Finnigan MAT, Bremen, Germany) at the Kompetenzzentrum für Stabile Isotope (KOSI, Göttingen, Germany) to measure the C and N concentrations. Acetanilide (10.36 % N, 71.09% C) was used as the standard.
To measure the contents of total P (Pt) and other elements (K, Ca, Na, Mg, Mn, Fe, Al, and S), 50 mg of each milled sample (roots, soil) was extracted in 65 % HNO3 (25 ml) for 12 h at 160°C (Heinrichs et al., 1986). Labile P (Ps) was determined by extracting 100 mg of sample in 150 ml of Bray-1 solution (0.03 N NH4F, 0.025 N HCl) (Bray and Kurtz, 1945). The resulting extracts were subjected to filtration using phosphate free filter paper (MN 280 1/4, Macherey-Nagel, Düren, Germany) after which elemental analysis by inductively coupled plasma–optical emission spectroscopy (ICP-OES) (iCAP 7000 Series ICP–OES, Thermo Fisher Scientific, Dreieich, Germany) was done at the following wavelengths (nm) (Na: 589.592; K: 766.490; Ca: 317.933; Mg: 285.213; Mn: 260.569; Fe: 238.204; Al: 308.215; S: 182.034; P: 185.942). The calibration was performed using concentrations of element standards (0.1–20 mg l–1) (Einzelstandards, Bernd Kraft, Duisburg, Germany). An overview on the nutrient data for soil and roots has been compiled in Supplementary Table 2. Data for soil and root nutrients in 2018 were downloaded from the dryad repository (Clausing et al., 2021).
Climate and weather
Data for climate and weather were obtained from Wetterzentrale1 because their weather stations are next to the plots. Mean temperature (T) for each sampling date was calculated as mean temperature of 3 months (month of sampling and 2 months before sampling). Similarly, precipitation (Prec) was calculated as the sum of the precipitation for the month of sampling and 2 months before sampling. We calculated the deviation from the long-term climatic conditions (dfc) as the difference between monthly mean temperature of the sampling month and the long-term mean of the temperature in the corresponding months for the period 1981–2010. The deviation from the long-term precipitation was calculated as the difference between the monthly sum of precipitation in the sampling month and the long-term mean of the sum of precipitation in the sampling month. The data shown in Supplementary Table 3.
Statistical analysis
The statistical analyses were performed with R software (R Core Development Team, 2020). The data were checked for normal distribution and homogeneity of variances using linear model residuals (function “lm” from R package “lme4”) and the Shapiro–Wilk test (Shapiro and Wilk, 1965). The data were log or square root transformed if the normality assumption was violated. Non-parametric test (Kruskal–Wallis test) was used if the normality assumption was not met after data transformation. When the normality assumption was met, we used linear mixed effect models (function: “lmer,” R package: “lme4”), analysis of variance (ANOVA) and a post hoc Tukey HSD test for comparison of means (package: “multcomp”) to test for the effect of site, fertilizer treatment and season on fine root biomass, on P and N in the soil and fine roots. We used plot (n = 3 per site) as random factor in the mixed models. Differences of the means at p ≤ 0.05 were considered to indicate significant effects.
To determine the contribution of explanatory variables to fine root mass, we constructed a linear model incorporating all variables {site (P-rich, P-medium, P-poor), treatment (Con, N, P, P + N), season (spring, fall), climate [Temperature (T, T dfc), precipitation (Prec, Prec dfc)], soil chemistry (C/N ratio, C, N, Pt, Ps, Na, K, Ca, Mg, Mn, Fe, S, WC_s and pH), and root chemistry (C/N ratio, C, N, Pt, Ps, Na, K, Ca, Mg, Mn, Fe, S)}. To obtain a model with good fit (lowest AIC), the constructed model was subjected to backward stepwise regression by using the “stepAIC” function from R “MASS” package (Venables and Ripley, 2002). The variables retained by stepwise regression were divided into five categories: site, climate, season, soil properties (mineral elements and WC_s), and root chemistry (mineral elements). Factors in each of the four categories for the organic (site, soil properties, root chemistry), and mineral (Season, soil properties, root chemistry, and climate) soil layers were retained (Table 2). We partitioned the variance of fine root biomass explained by each category using “calc.relimp” function from R “Relaimpo” package (Grömping, 2006) based on the lmg method (averaging sequential sum of squares over all orderings) developed by Lindeman et al. (1980).
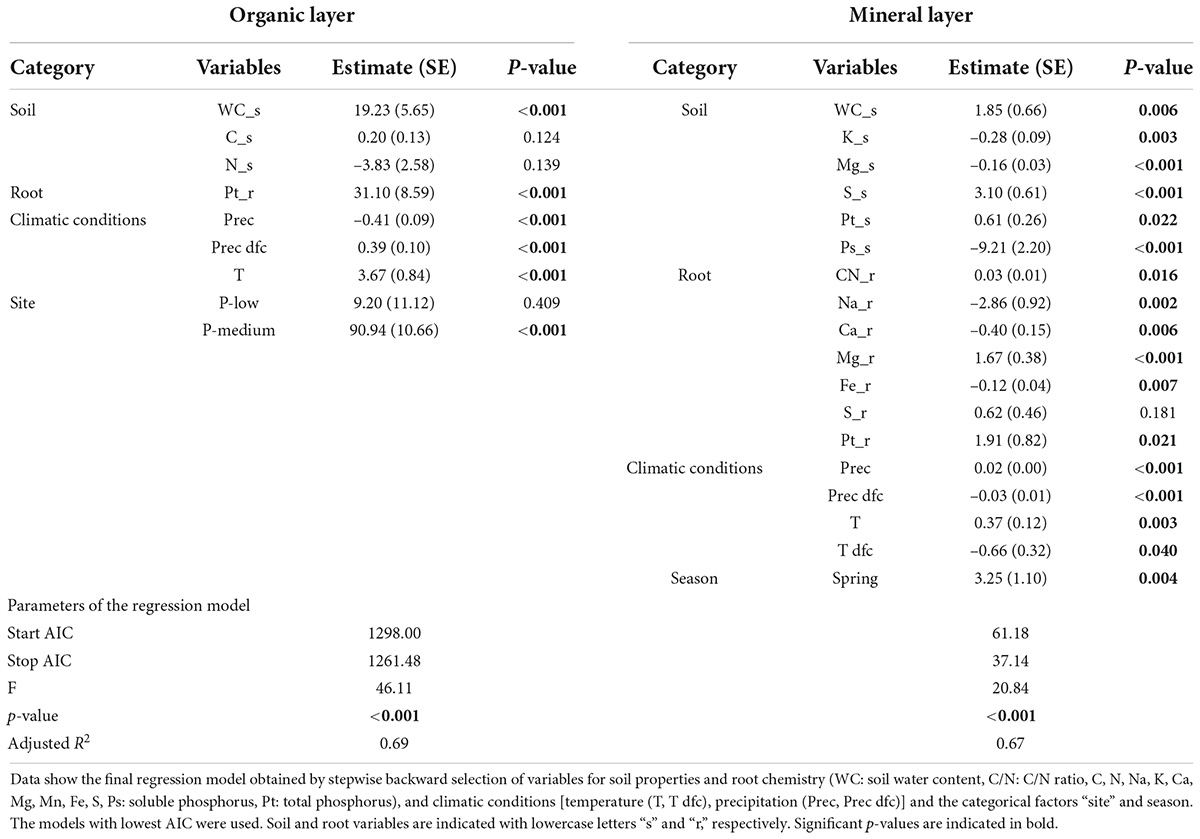
Table 2. Linear regression models for variation in fine root biomass in the organic and mineral soil.
Results
Fertilizer treatment and season affect phosphorus in soil
P application caused significant increases in total (p = 0.039) and soluble P (p = 0.008) in the mineral soil of the P-low forest in spring but not in fall (Figures 1A,B). We did not find any significant effects of P or N treatment or the combination of both on the P contents in the organic layer. No significant fertilization effects were found for the total or soluble P contents in the P-medium or P-rich forest soils (Figures 1A,B).
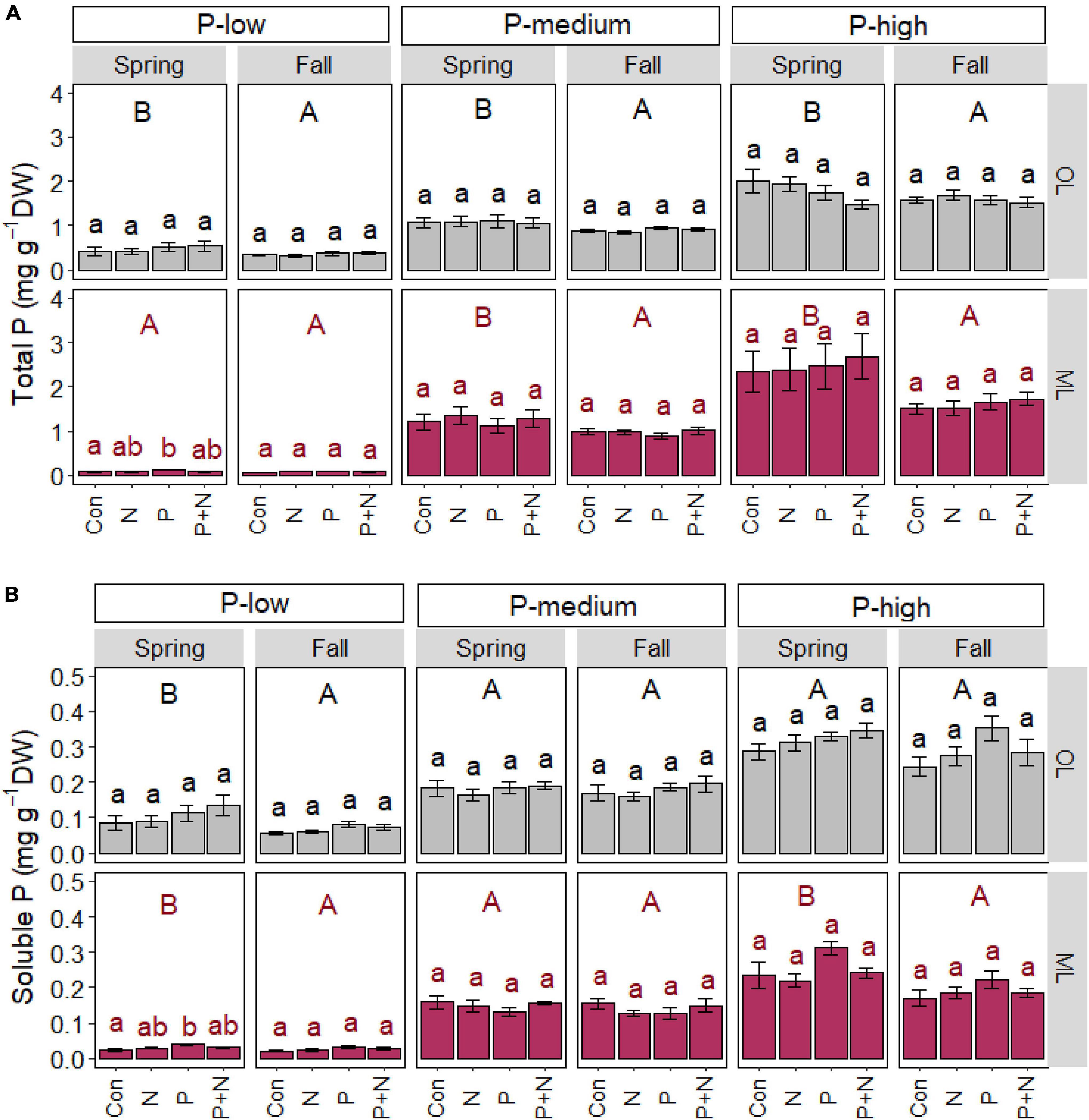
Figure 1. Total (A) and soluble (B) phosphorus content in the organic (OL) and mineral (ML) soil in beech (Fagus sylvatica) forests characterized by low, medium, and high P contents. The study was conducted at the forest sites Unterlüss (P-low), Mitterfels (P-medium), and Bad Brückenau (P-high) using 3 plots per treatment (Con = control = no fertilizer treatment, N = 5 × 30 kg ha–1 nitrogen fertilizer, P = 50 kg ha–1 phosphorus fertilizer, P+N = combined N and P fertilizer treatment). Samples were collected in fall 2016, 2017, 2018 and in spring 2017 and 2018. Data show means per season and treatment (spring: n = 6, fall: n = 9, ± SE). Significant differences at p ≤ 0.05 are indicated for the fertilizer treatment (lowercase letters) and season (uppercase letters) for each forest site.
Irrespective of the fertilizer treatments, total P contents were higher in the organic layer in spring than in fall, indicating seasonal cycling at each of the three studied forest sites (Figure 1A). In the mineral soil, seasonal increases in total P in spring were also significant in the P-medium (p = 0.002) and P-high forest (p = 0.001) but not in the P-low forest (p = 0.103, Figure 1A). In the P-low forest, soluble P contents were higher in spring than in fall in both soil layers studied (p < 0.010, Figure 1B). A significant increase in soluble P in spring was also observed in the mineral soil of the P-high forest (p < 0.001, Figure 1B) but not in the organic layer.
The comparison of the P contents in the three forests confirmed significant differences among the sites in the order P-low < P-medium < P-rich forest (p < 0.001, Figure 1) both soil layers, in agreement with previous investigations in these forests (Zavišić et al., 2016; Lang et al., 2017; Table 1).
Fertilizer treatment and season affect phosphorus in roots
P application as a single factor or in combination with N resulted in forest- and soil layer-specific effects on the P content of fine roots (Figure 2). In the P-low forest, fine roots from the mineral layer contained increased total (p = 0.014) and soluble P contents (p = 0.003) in spring after combined fertilization with P and N (Figure 2A). In the P-low forest, the soluble P contents roots from the mineral soil was also enhanced after P (p < 0.001) application (Figure 2B). In fall, no fertilization effects were found in roots from the mineral soil and organic layer in the P-low forest and the total and soluble P contents were lower than in spring (Figures 2A,B).
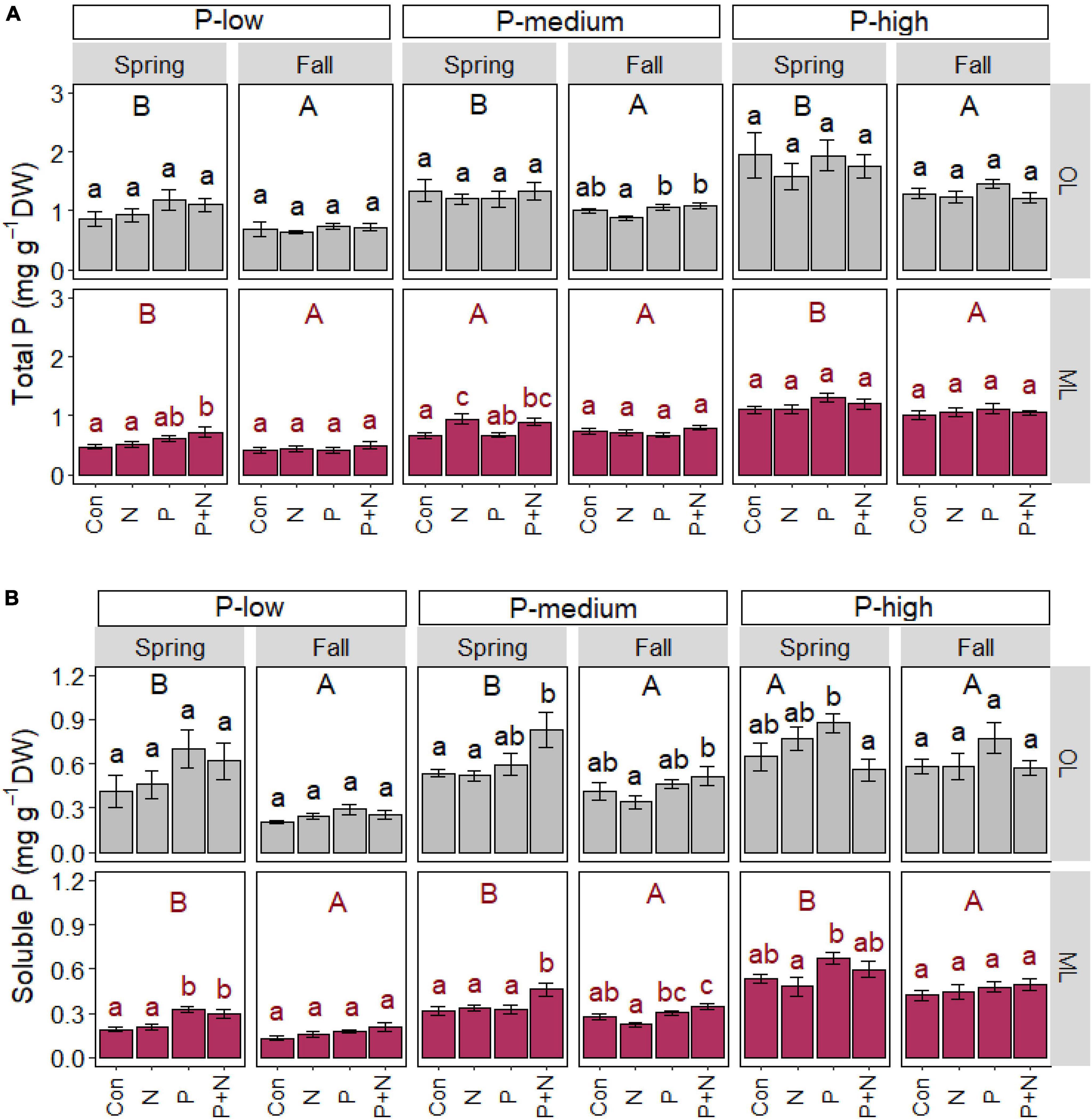
Figure 2. Total (A) and soluble (B) phosphorus contents of beech (Fagus sylvatica) fine roots in the organic (OL) and mineral (ML) soil layer. The study was conducted at the forest sites Unterlüss (P-low), Mitterfels (P-medium), and Bad Brückenau (P-high) using 3 plots per treatment (Con = control = no fertilizer treatment, N = 5 × 30 kg ha–1 nitrogen fertilizer, P = 50 kg ha–1 phosphorus fertilizer, P+N = combined N and P fertilizer treatment). Samples were collected in fall 2016, 2017, 2018 and in spring 2017 and 2018. Data indicate means for each season and treatment (spring: n = 6, fall: n = 9, ± SE). Significant differences at p ≤ 0.05 are indicated for the fertilizer treatment (lowercase letters) and season (uppercase letters) in each forest site.
The total P contents in roots from the P-high forest did not respond to any fertilization treatment (Figure 2A) but the soluble P levels in roots from the organic layer and from the mineral soil were enhanced in spring in the P-high forest after P fertilization (Figure 2B).
In the P-medium forest, root total P contents increased in spring under N or combined N and P fertilization but only in roots from mineral soil (Figure 2A). Combined N and P treatment also caused increased soluble P contents in these roots in spring (p = 0.020, Figure 2B). Roots from the P-medium forest further showed P or combined N and P treatment effects in fall, resulting in enhanced total P contents in roots from the organic layer and higher soluble P contents in roots from both soil layers (p = 0.012, Figure 2B).
In addition to fertilization effects, the total P contents in roots were higher in spring than in fall in the organic layer and in the mineral soil, with the exception of roots in the mineral soil at the P-medium site (Figure 2A). The soluble P contents in roots from both the organic layer and the mineral soil also were higher in spring than in fall, with the exception of roots in the organic layer at the P-high forest (Figure 2B).
We also found differences for the root P contents among the forest sites: total P contents in roots increased in the order P-low < P-medium < P-high in both seasons and soil layers (p < 0.001). Soluble P in roots from the mineral layer showed a pattern similar to that of total P (p < 0.001), whereas roots from the organic layer showed this pattern only in spring (p = 0.006).
Season but not fertilizer treatment affect nitrogen in soil and roots
In addition to P, we investigated the impact of fertilizer application and season on soil and root N contents (Figure 3). We did not observe any changes in soil or root N in response to N, P or the combination of N and P application, neither in the organic layer nor in the mineral soil (Figures 3A,B).
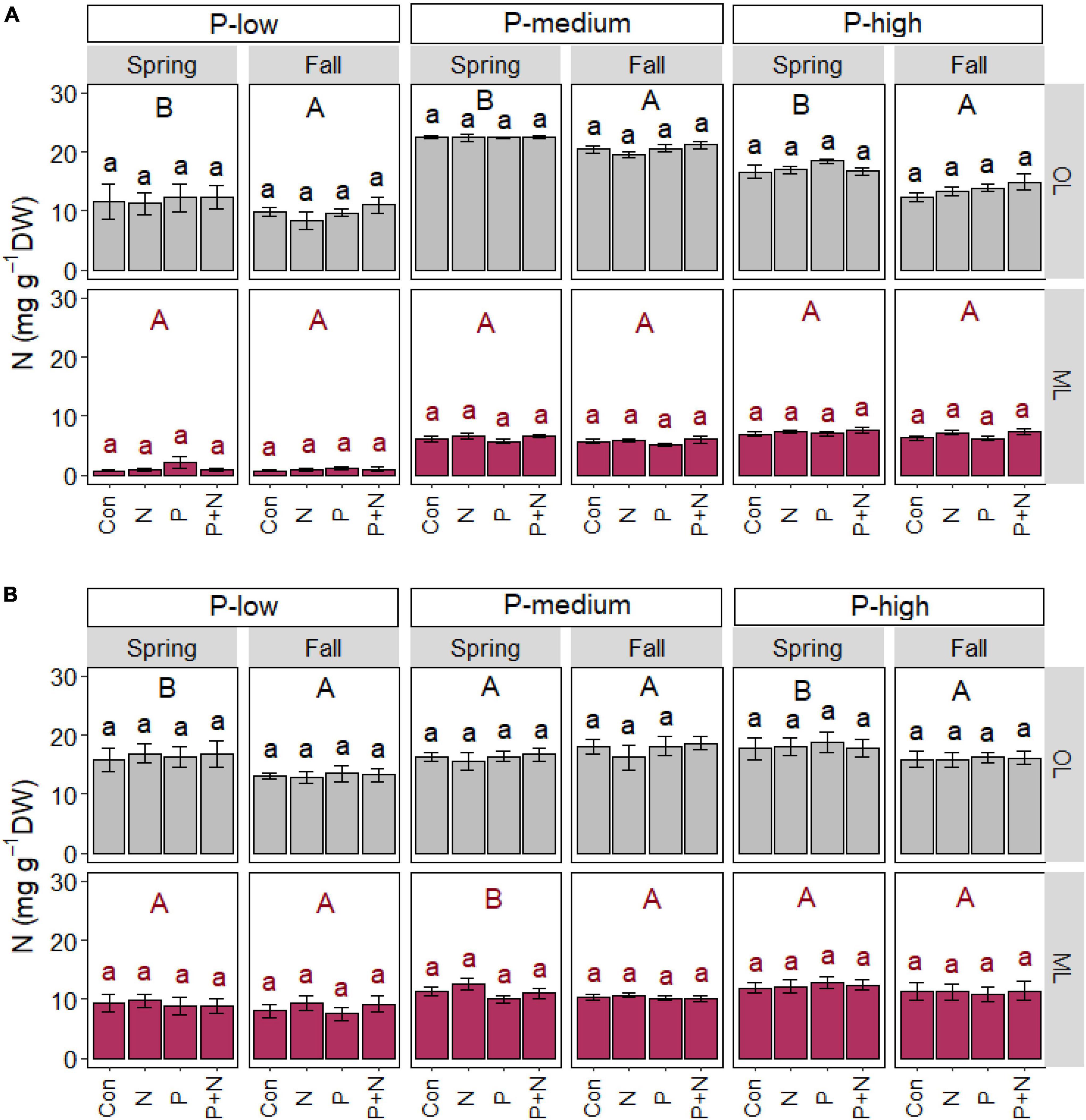
Figure 3. Soil (A) and fine root (B) nitrogen contents of beech (Fagus sylvatica) in the organic (OL) and mineral (ML) soil layer of forests characterized by low, medium, and high soil P contents. The study was conducted at the forest sites Unterlüss (P-low), Mitterfels (P-medium), and Bad Brückenau (P-high) using 3 plots per treatment (Con = control = no fertilizer treatment, N = 5 × 30 kg ha–1 nitrogen fertilizer, P = 50 kg ha–1 phosphorus fertilizer, P+N = combined N and P fertilizer treatment). Samples were collected in fall 2016, 2017, 2018 and in spring 2017 and 2018. Data indicate means for each season and treatment (spring: n = 6, fall: n = 9, ± SE). Significant differences at p ≤ 0.05 are indicated for season (uppercase letters) in each forest site.
The organic layer contained higher N contents in spring than in fall in each forest site (Figure 3A). In the organic layer in the P-low and P-rich forest, root N was also higher in spring than fall, while in the P-medium forest, root N was higher in the mineral soil in spring than in fall (p = 0.043, Figure 3B).
Fine root biomass is mainly controlled by multiple site conditions and marginally by P fertilization
A significant fertilizer effect on fine root biomass was not found in the organic layer (Figure 4A) and only observed at the P-low site in the mineral layer in fall (Figure 4), where root biomass was approximately 1.3-fold greater after P application than in the control plots (p = 0.018) (Figure 4B).
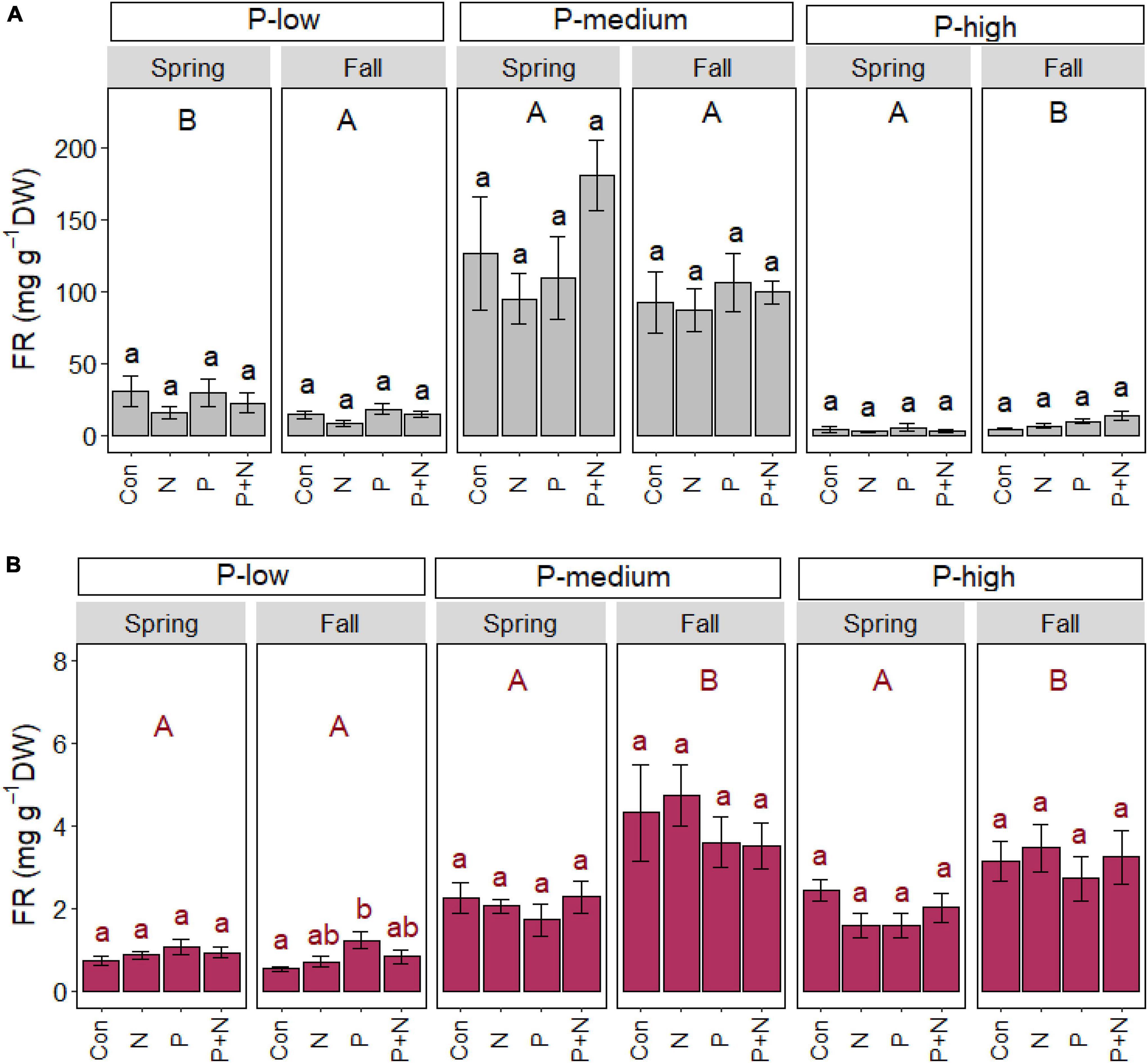
Figure 4. Beech (Fagus sylvatica) fine root mass in the organic (A) and mineral (B) soil layer of forests characterized by low, medium, and high soil P contents. The study was conducted at the forest sites Unterlüss (P-low), Mitterfels (P-medium), and Bad Brückenau (P-high) using 3 plots per treatment (Con = control = no fertilizer treatment, N = 5 × 30 kg ha–1 nitrogen fertilizer, P = 50 kg ha–1 phosphorus fertilizer, P+N = combined N and P fertilizer treatment). Samples were collected in fall 2016, 2017, 2018 and in spring 2017 and 2018. Data indicate means for each season and treatment (spring: n = 6, fall: n = 9, ± SE). Significant differences at p ≤ 0.05 are indicated for the fertilizer treatment (lowercase letters) and season (uppercase letters) in each forest site.
Root biomass showed significant seasonal changes, with contrasting patterns in different forests (Figure 4). In the organic layer, root mass was higher in spring than fall in the P-low forest (p = 0.006) and lower in spring than in fall in the P-high forest (p < 0.001). In the mineral soil, root mass was higher in fall than spring in the P-medium (p < 0.001) and P-high (p < 0.001) forests (Figure 4).
Root biomass was further affected by the forest site, irrespective of season: in the organic layer, root biomass contents (mg g–1 soil) increased in the order P-high < P-low < P-medium (spring: p < 0.001; fall: p < 0.001). In the mineral layer, root mass was lower in the P-low than in P-medium (spring: p < 0.001; fall: p < 0.001) and P-high forests (spring: p < 0.001; fall: p < 0.001).
To evaluate the impact of climatic conditions, season, site, fertilizer, soil properties, and root chemistry on fine root biomass, we used a total of 33 variables (specified under materials and methods) and determined those retained by stepwise linear regression (Table 2). The model for root biomass in the organic layer (p < 0.001, adjusted R2 = 0.69) contained parameters for the following categories: soil properties (water, carbon and nitrogen contents), root chemistry (total P), climatic conditions (temperature and precipitation) and site (Table 2). The model for root biomass in mineral soil (p < 0.001, adjusted R2 = 0.67) contained parameters for the following categories: soil properties (water, potassium, magnesium, sulfur, and soluble and total P contents), root chemistry (C/N ratio, sodium, calcium, magnesium, iron and total P contents), climatic conditions (temperature and precipitation), and season (Table 2). To determine the contribution of each category, we partitioned the variance that explained fine root biomass (Figure 5). In the organic layer, the variation of fine root biomass was mainly explained by soil properties (37%) and site (27%), whereas root chemistry and climate only explained little variation of fine root mass (1 and 5%, respectively). In the mineral soil, fine root biomass variation was attributed to soil properties (30%), root chemistry (20%), and climatic conditions (16%). In both soil layers, we observed a high contribution of soil chemistry to fine root biomass variation. A major difference between the factors explaining root biomass variation were higher contributions of root chemistry and climate in mineral soil than in the organic layer.
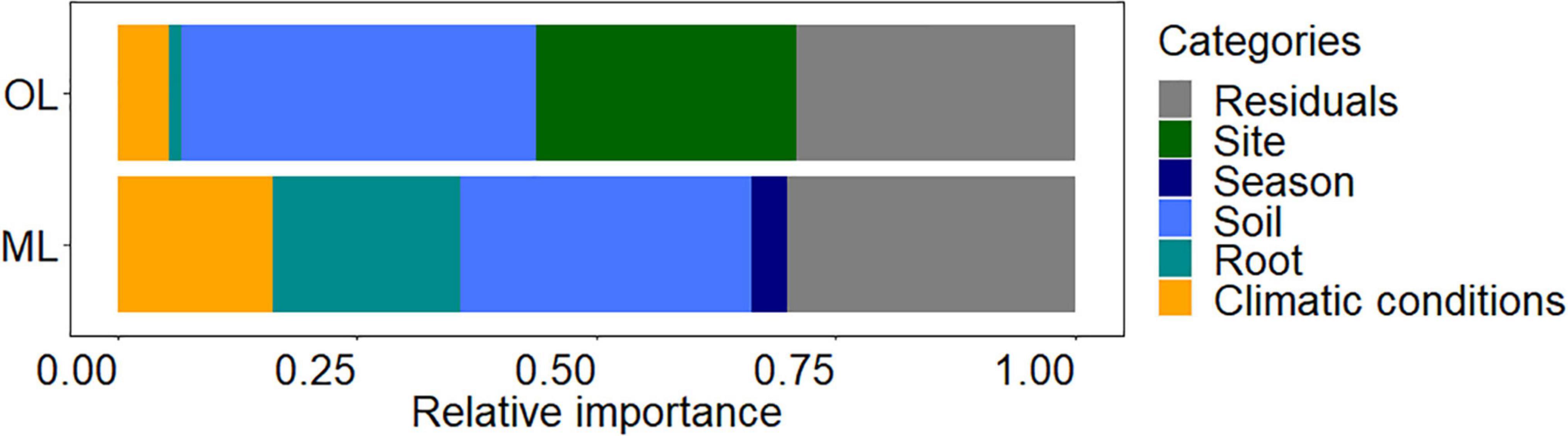
Figure 5. Proportion of fine root biomass variance explained by site, treatment, season, soil, root, and climatic condition categories in the organic layer (OL) and mineral (ML) soil. The variables within each category were retained by stepwise regressing model (provided in Table 2) and explain 71% (OL) and 70% (ML) of the variation of fine root biomass. Residuals refers to unexplained proportion of fine root biomass variance.
Discussion
Soil and root P contents show seasonal dynamics and site-specific fertilization effects
In this study, we investigated key factors that determine root biomass in temperate forests such as temperature, nutrient and water availability (Pregitzer et al., 1993; Eissenstat et al., 2000; Hertel et al., 2013). A novel aspect was that we also included root chemistry because we reasoned that seasonal allocation shifts in nutrients may also influence root biomass. Several studies have shown that beech trees mobilize internal P resources in spring from stem and roots to support leaf flush (Netzer et al., 2017; Zavišić and Polle, 2018), while root growth is favored later in the growth season (this study, Brassard et al., 2009; Zavišić and Polle, 2018). Internal P retranslocation in spring involves transformation of organic P into labile P forms, their utilization for growth and replenishment of plant storage pools in late summer and fall (Netzer et al., 2017; Spohn et al., 2018; Zavišić and Polle, 2018). Mesocosm experiments with 33P tracers showed that P uptake by beech trees is low in spring, despite high P demand and internal resource allocation to leaf production, while P uptake is high in late summer and fall (Spohn et al., 2018; Zavišić and Polle, 2018). These results appear counterintuitive to the present results, showing increased P contents in soil and fine roots in spring and decreased P contents in these compartments in fall. However, these patterns most likely reflect physiological acclimation of P transport systems, which are highly sensitive to P availabilities, resulting in decreased P uptake capacities under high and increased P uptake capacities under low P supply (Kavka and Polle, 2016, Kavka et al., 2021), thus, complying with seasonal fluctuation of P contents in our study.
The observed seasonal trajectory occurred on top of the differences in soil and root P contents due to the P fertility gradient of our study sites (Lang et al., 2017) and was likely caused by P and N inputs due to leaf shedding. In the forests, which we studied here, the P respective N inputs by annual litter fall amount approximately (P/N) 0.16/2.9 g m–2 at the P-low, 0.21/3.6 g m–2 at the P-medium and 0.23/3.2 g m–2 at the P-high site (Lang et al., 2017). Nutrient release and leaching from leaf litter was found over the summer and caused cycling between higher spring and lower fall P and N contents in the organic layer (Fetzer et al., 2021, 2022). Our results confirm this seasonal pattern for N and P in the organic soil layer. Overall, our results support that tree phenology promotes nutrient cycling via litter feedback (Augusto et al., 2002; Hobbie, 2015).
An interesting observation was that seasonal variations in total and soluble P but not in total N (with the exception of the P-medium site) were also found in mineral soil and roots from mineral soil across the P-fertility gradient. The divergent behavior of P and N can be ascribed to different mineralization rates of N and P from degradation of organic compounds in leaf litter (Brödlin et al., 2019a), differences in mobility and sorption in forest soil (Kaiser et al., 2003; Fetzer et al., 2022), seasonal P loss due to drying and re-wetting cycles (Brödlin et al., 2019b), and other abiotic or biotic factors (Augusto et al., 2002). Here, N fertilization rarely affected root nutrient contents but a decrease in root P after N fertilization at the P-medium site (with the highest N contents) suggests that excess N inputs may have triggered imbalance of the N:P ratio (Peñuelas and Sardans, 2022). Unexpectedly, P or P + N fertilization caused increases in labile P not only in roots at the P-low but also at the P-medium and P-high sites (mineral soil), revealing that added P was captured by the trees, irrespective of the soil P contents. The observed P increments occurred in most cases only in spring and not in fall, probably because of higher soil moisture and mineralization rates in spring than in fall (De Feudis et al., 2016; Fetzer et al., 2022).
Impact of nutrient resources and climate on root mass varies between organic and mineral soil
Central questions of our study were whether fertilization and climatic factors affected standing biomass of fine roots. In agreement with our first hypothesis, we observed an increase in fine root biomass following P addition in the mineral layer at the P-low site, i.e., only in the soil with the least P availability (Lang et al., 2017). Since we found neither positive synergistic effects of P + N (Elser et al., 2007) nor negative effects of N (van Dijk et al., 1990; Clemensson-Lindell and Persson, 1995; Nadelhoffer, 2000) on root biomass, we may speculate that P was a growth-limiting factor and therefore, increases in P availability stimulated root biomass production. It is also possible that P fertilization affected root turnover because an increase in root biomass can also be the consequence of a longer root lifetime. The increment in fine root biomass was moderate and only found in fall and therefore cannot be not explained by fertilization treatment as the only factor. Whether minute positive root biomass responses due to P fertilization could counteract P deterioration in European forests (Jonard et al., 2015; Talkner et al., 2015) remains a matter of debate. However, in agreement with other studies (Finér et al., 2007), site fertility had profound influence beech standing root biomass.
Tree nutrition depends not only on root biomass but also on plant-microbial interactions, which show stratification between the organic and mineral soil horizons (Baldrian, 2017; Brabcová et al., 2018; Khokon et al., 2021). In the forests studied here, fertilization did not strongly influence the fungal community composition but P application enhanced the abundance of mycorrhizal species from the family of Boletaceae, which have increased capacities for P retention (Clausing et al., 2021). Thereby, mycorrhizal fungi may have mediated higher P uptake in the fertilized plots without notable effects on fine root biomass.
Enhanced root biomass occurred in most cases in fall. These results agree with other studies (López et al., 2001; Montagnoli et al., 2012) but exceptions have also been reported, showing higher fine root biomass in spring (our study: organic layer at the P-low site; Grier et al., 1981; Burton et al., 2000). These findings highlight root plasticity, most likely reflecting that plants invest in organs that are essential for acquisition of the least available resources (Bridgham et al., 1995; Niklas, 2004). Despite cycling of fine root biomass, season was only a minor factor explaining root biomass variation, probably because we included other potentially strong drivers of root biomass in our models such as mineral nutrients and soil moisture.
Our regression model also elucidated important differences between the impact of P on the variation of root mass in the organic and mineral soil. In the organic layer, soil P was not among the nutrients that could explain fine root biomass variations, despite the importance of the organic layer for P mobilization and tree nutrition (Heuck and Spohn, 2016; Hauenstein et al., 2018; Zederer and Talkner, 2018). In contrast to the organic layer, the labile P fraction was the most important explanatory variable for root biomass in mineral soil. Although the difference in fine root biomass variation explained by “soil chemistry” between the soil horizons was minimal (about 6%), the relevant explanatory factors differed between the soil compartments. Therefore, an important insight of our study was that fine roots along vertical profiles obviously responded autonomously to differences in the surrounding environment. This idea had further support because “root chemistry,” mainly attributable to root P and root basic cation contents, explained a remarkable fraction of root biomass variation in mineral soil (19%) and but very little in the organic soil (1%).
A further category that explained variations in the standing fine root biomass were climatic conditions but in contrast to our initial hypothesis, we found stronger impact of climate in the mineral soil (16%) than in the forest floor (5%). A likely explanation is that the soil water content (category “soil”) was a stronger factor in the organic than in the mineral layer. This finding suggests higher sensitivity of fine roots close to the surface of the forest floor to changes in the actual water availability, whereas fluctuations in water content in deeper soil layers are initially buffered by the water holding capacity of the soil structure, thus, leading to a stronger impact as the consequence of long-term changes in precipitation. Other studies also reported the importance of climatic factors for standing fine root biomass and root turnover (Meier and Leuschner, 2010; Hertel et al., 2013; Gao et al., 2021). Since the organic layer is especially important for tree P nutrition in the P-low site (Lang et al., 2017; Hauenstein et al., 2018; Clausing and Polle, 2020), lower water contents and higher temperatures may put root biomass at this site at a higher risk of failure than in the other forests of our study.
Conclusion
In agreement with our first hypothesis, we show that P-fertilization led to increased fine root biomass only in the mineral soil with the least P availability, while soil or root P contents increased in all soil types. Root biomass showed seasonal shifts, which were associated with changes in soil and root nutrient levels but were unaffected by fertilizer treatments, in contrast to second hypothesis. The seasonal differences in standing root biomass were more important in the mineral than in the organic soil. We propose that higher importance of soil and root nutrients for root biomass in the mineral as compared to the organic layer may be attributable to differences in microbial nutrient cycling. These processes are dominant in the organic layer and may have overruled the impact of soil or root chemistry. In contrast to our third hypothesis, root biomass variation was driven to a much higher extent by climate in the mineral than in the organic layer. Therefore, our results imply that climate change with long-term warmer and drier conditions is likely to endanger root systems below the organic layer. Therefore, negative climate effects may not only be expected for forests on nutrient-poor parent material that rely on the organic layer for nutrient supply but also for forests on more fertile sites, where roots forage in the mineral horizon.
Data availability statement
The datasets presented in this study can be found in online repositories. The names of the repository/repositories and accession number(s) can be found below: https://doi.org/10.5061/dryad.rn8pk0pdd.
Author contributions
AP, SC, LEL, JK, and FL contributed to the design of the study. JK and FL maintained the plots and the fertilization experiment. LEL and SC conducted field sampling. SC conducted measurements. AP supervised the measurements and revised the draft. LEL analyzed the data and wrote the first draft of the manuscript. All authors contributed to the article and approved the submitted version.
Funding
This research was funded by Deutsche Forschungsgemeinschaft (DFG) to the projects Po362/22–2 (AP) and LA1398/13–2 (FL) under the umbrella of the Priority Research program (Ecosystem Nutrition, SPP1685). LEL was an associate Ph.D. student of the research training group (RTG2300 project SP4) funded by the University of Zambia under the Special Research Fellowship Program and by a scholarship provided by the Bernhard-Ulrich Stiftung (Faculty for Forest Sciences and Forest Ecology, University of Göttingen). Publication was supported by the Georg-August Universität Göttingen open access publication fund.
Acknowledgments
We express our gratitude to M. Franke-Klein (Forest Botany and Tree Physiology, Göttingen) and G. Lehmannn (Laboratory for Radio-Isotopes, LARI, Göttingen) for technical assistance. We thank Nadine Kubsch and Karolin Müller for assistance with field harvests.
Conflict of interest
The authors declare that the research was conducted in the absence of any commercial or financial relationships that could be construed as a potential conflict of interest.
Publisher’s note
All claims expressed in this article are solely those of the authors and do not necessarily represent those of their affiliated organizations, or those of the publisher, the editors and the reviewers. Any product that may be evaluated in this article, or claim that may be made by its manufacturer, is not guaranteed or endorsed by the publisher.
Supplementary material
The Supplementary Material for this article can be found online at: https://www.frontiersin.org/articles/10.3389/ffgc.2022.955327/full#supplementary-material
Footnotes
References
Augusto, L., Ranger, J., Binkley, D., and Rothe, A. (2002). Impact of several common tree species of European temperate forests on soil fertility. Ann. For. Sci. 59, 233–253. doi: 10.1051/forest:2002020
Baldrian, P. (2017). Forest microbiome: diversity, complexity and dynamics. FEMS Microbiol. Rev. 41, 109–130. doi: 10.1093/femsre/fuw040
Brabcová, V., Štursová, M., and Baldrian, P. (2018). Nutrient content affects the turnover of fungal biomass in forest topsoil and the composition of associated microbial communities. Soil Biol. Biochem. 118, 187–198. doi: 10.1016/j.soilbio.2017.12.012
Brassard, B. W., Chen, H. Y. H., and Bergeron, Y. (2009). Influence of environmental variability on root dynamics in Northern forests. Crit. Rev. Plant Sci. 28, 179–197. doi: 10.1080/07352680902776572
Bray, R. H., and Kurtz, L. T. (1945). Determination of total, organic, and available forms of phosphorus in soils. Soil Sci. 59, 39–46.
Bridgham, S. D., Pastor, J., McClaugherty, C. A., and Richardson, C. J. (1995). Nutrient-use efficiency: A litterfall index, a model, and a test along a nutrient-availability gradient in North Carolina peatlands. Am. Nat. 145, 1–21.
Brödlin, D., Kaiser, K., and Hagedorn, F. (2019a). Divergent patterns of carbon, nitrogen, and phosphorus mobilization in forest soils. Front. For. Glob. Change 2:66. doi: 10.3389/ffgc.2019.00066
Brödlin, D., Kaiser, K., Kessler, A., and Hagedorn, F. (2019b). Drying and rewetting foster phosphorus depletion of forest soils. Soil Biol. Biochem. 128, 22–34. doi: 10.1016/j.soilbio.2018.10.001
Burton, A. J., Pregitzer, K. S., and Hendrick, R. L. (2000). Relationships between fine root dynamics and nitrogen availability in Michigan northern hardwood forests. Oecologia 125, 389–399. doi: 10.1007/s004420000455
Clausing, S., Likulunga, E. L., Janz, D., Feng, H., Schneider, D., Daniel, R., et al. (2021). Impact of nitrogen and phosphorus addition on resident soil and root mycobiomes in beech forests. Biol. Fertil. Soils 57, 1031–1052. doi: 10.1007/s00374-021-01593-x
Clausing, S., and Polle, A. (2020). Mycorrhizal phosphorus efficiencies and microbial competition drive root P uptake. Front. For. Glob. Change 3:54. doi: 10.3389/ffgc.2020.00054
Clemensson-Lindell, A., and Persson, H. (1995). Fine root vitality in a Norway spruce stand subjected to various nutrient supplies. Plant Soil 168-169, 167–172. doi: 10.1007/BF00029325
De Feudis, M., Cardelli, V., Massaccesi, L., Bol, R., Willbold, S., Cocco, S., et al. (2016). Effect of beech (Fagus sylvatica L.) rhizosphere on phosphorous availability in soils at different altitudes (Central Italy). Geoderma 276, 53–63. doi: 10.1016/j.geoderma.2016.04.028
Delpierre, N., Vitasse, Y., Chuine, I., Guillemot, J., Bazot, S., Rutishauser, T., et al. (2016). Temperate and boreal forest tree phenology: from organ-scale processes to terrestrial ecosystem models. Ann. For. Sci. 73, 5–25. doi: 10.1007/s13595-015-0477-6
Du, E., and De Vries, W. (2018). Nitrogen-induced new net primary production and carbon sequestration in global forests. Environ. Pollut. 242, 1476–1487. doi: 10.1016/j.envpol.2018.08.041
Du, E., Terrer, C., Pellegrini, A. F., Ahlström, A., van Lissa, C. J., Zhao, X., et al. (2020). Global patterns of terrestrial nitrogen and phosphorus limitation. Nat. Geosci. 13, 221–226. doi: 10.1038/s41561-019-0530-4
Dziedek, C., Fichtner, A., Calvo, L., Marcos, E., Jansen, K., Kunz, M., et al. (2017). Phenotypic plasticity explains response patterns of European beech (Fagus sylvatica L.) saplings to nitrogen fertilization and drought events. Forests 8:91. doi: 10.3390/f8030091
Eissenstat, D. M., Wells, C. E., Yanai, R. D., and Whitbeck, J. L. (2000). Building roots in a changing environment: implications for root longevity. New Phytol. 147, 33–42. doi: 10.1046/j.1469-8137.2000.00686.x
Elser, J. J., Bracken, M. E. S., Cleland, E. E., Gruner, D. S., Harpole, W. S., and Hillebrand, H. (2007). Global analysis of nitrogen and phosphorus limitation of primary producers in freshwater, marine and terrestrial ecosystems. Ecol. Lett. 10, 1135–1142. doi: 10.1111/j.1461-0248.2007.01113.x
Fetzer, J., Frossard, E., Kaiser, K., and Hagedorn, F. (2022). Leaching of inorganic and organic phosphorus and nitrogen in contrasting beech forest soils – seasonal patterns and effects of fertilization. Biogeosciences 19, 1527–1546. doi: 10.5194/bg-19-1527-2022
Fetzer, J., Loeppmann, S., Frossard, E., Manzoor, A., Brödlin, D., Kaiser, K., et al. (2021). Leaching of Phosphomonoesterase activities in beech forest soils: consequences for phosphorus forms and mobility. Front. For. Glob. Change 4:684069. doi: 10.3389/ffgc.2021.684069
Finér, L., Helmisaari, H. S., Lõhmus, K., Majdi, H., Brunner, I., Børja, I., et al. (2007). Variation in fine root biomass of three European tree species: beech (Fagus sylvatica L.), Norway spruce (Picea abies L. Karst.), and Scots pine (Pinus sylvestris L.). Plant Biosyst. 141, 394–405. doi: 10.1080/11263500701625897
Galloway, J. N., Townsend, A. R., Erisman, J. W., Bekunda, M., Cai, Z., Freney, J. R., et al. (2008). Transformation of the nitrogen cycle: recent trends, questions, and potential solutions. Science 320, 889–892. doi: 10.2307/20054730
Gao, G., Liu, Z., Wang, Y., Wang, S., Ju, C., and Gu, J. (2021). Tamm review: fine root biomass in the organic (O) horizon in forest ecosystems: global patterns and controlling factors. For. Ecol. Manag. 491:119208. doi: 10.1016/j.foreco.2021.119208
Godbold, D. L., and Brunner, I. (2007). The platform for European root science, COST action E38: an introduction and overview. Plant Biosyst. Int. J. Deal. Aspects Plant Biol. 141, 390–393. doi: 10.1080/11263500701625715
Grier, C. C., Vogt, K. A., Keyes, M. R., and Edmonds, R. L. (1981). Biomass distribution and above- and below-ground production in young and mature Abies amabilis zone ecosystems of the Washington Cascades. Can. J. For. Res. 11, 155–167.
Grömping, U. (2006). Relative importance for linear regression in R: the package relaimpo. J. Stat. Softw. 17, 1–27. doi: 10.18637/jss.v017.i01
Hauenstein, S., Neidhardt, H., Lang, F., Krüger, J., Hofmann, D., Pütz, T., et al. (2018). Organic layers favor phosphorus storage and uptake by young beech trees (Fagus sylvatica L.) at nutrient poor ecosystems. Plant Soil 432, 289–301. doi: 10.1007/s11104-018-3804-5
Haußmann, T., and Lux, W. (1997). Dauerbeobachtungsfl€achen zur Umweltkontrolle im Wald: Level II. Bonn: Erste Ergebnisse.
Heinrichs, H., Brumsack, H. J., Loftfield, N., and König, N. (1986). Verbessertes Druckaufschlußsystem für biologische und anorganische Materialien. Z. Für Pflanzenernährung Bodenkunde 149, 350–353. doi: 10.1002/jpln.19861490313
Hertel, D., Strecker, T., Müller-Haubold, H., and Leuschner, C. (2013). Fine root biomass and dynamics in beech forests across a precipitation gradient – is optimal resource partitioning theory applicable to water-limited mature trees? J. Ecol. 101, 1183–1200. doi: 10.1111/1365-2745.12124
Heuck, C., and Spohn, M. (2016). Carbon, nitrogen and phosphorus net mineralization in organic horizons of temperate forests: stoichiometry and relations to organic matter quality. Biogeochemistry 131, 229–242. doi: 10.1007/s10533-016-0276-7
Hobbie, S. E. (2015). Plant species effects on nutrient cycling: revisiting litter feedbacks. Trends Ecol. Evol. 30, 357–363. doi: 10.1016/j.tree.2015.03.015
Högberg, P., Johannisson, C., and Hällgren, J. E. (1993). Studies of 13C in the foliage reveal interactions between nutrients and water in forest fertilization experiments. Plant Soil 152, 207–214. doi: 10.1007/bf00029090
Jonard, M., Fürst, A., Verstraeten, A., Thimonier, A., Timmermann, V., Potočić, N., et al. (2015). Tree mineral nutrition is deteriorating in Europe. Glob. Change Biol. 21, 418–430. doi: 10.1111/gcb.12657
Kaiser, K., Guggenberger, G., and Haumaier, L. (2003). Organic phosphorus in soil water under a European beech (Fagus sylvatica L.) stand in northeastern Bavaria, Germany: seasonal variability and changes with soil depth. Biogeochemistry 66, 287–310.
Kavka, M., Majcherczyk, A., Kües, U., and Polle, A. (2021). Phylogeny, tissue-specific expression, and activities of root-secreted purple acid phosphatases for P uptake from ATP in P starved poplar. Plant Sci. 307:110906. doi: 10.1016/j.plantsci.2021.110906
Kavka, M., and Polle, A. (2016). Phosphate uptake kinetics and tissue-specific transporter expression profiles in poplar (Populus x canescens) at different phosphorus availabilities. BMC Plant Biol. 16:206. doi: 10.1186/s12870-016-0892-3
Kengdo, S. K., Persoh, D., Schindlbacher, A., Heinzle, J., Tian, Y., Wanek, W., et al. (2022). Long-term soil warming alters fine root dynamics and morphology, and their ectomycorrhizal fungal community in a temperate forest soil. Glob. Change Biol. 28, 3441–3458. doi: 10.1111/gcb.16155
Khokon, A. M., Schneider, D., Daniel, R., and Polle, A. (2021). Soil layers matter: vertical stratification of root-associated fungal assemblages in temperate forests Reveals differences in habitat colonization. Microorganisms 9:2131. doi: 10.3390/microorganisms9102131
Kirfel, K., Heinze, S., Hertel, D., and Leuschner, C. (2019). Effects of bedrock type and soil chemistry on the fine roots of European beech – A study on the belowground plasticity of trees. For. Ecol. Manag. 444, 256–268. doi: 10.1016/j.foreco.2019.04.022
Lang, F., Krüger, J., Amelung, W., Willbold, S., Frossard, E., Bünemann, E. K., et al. (2017). Soil phosphorus supply controls P nutrition strategies of beech forest ecosystems in Central Europe. Biogeochemistry 136, 5–29. doi: 10.1007/s10533017-0375-0
Leuschner, C. (2020). Drought response of European beech (Fagus sylvatica L.) – a review. Perspect. Plant Ecol. Evol. Syst. 47:125576. doi: 10.1016/j.ppees.2020.125576
Li, Y., Niu, S., and Yu, G. (2016). Aggravated phosphorus limitation on biomass production under increasing nitrogen loading: a meta-analysis. Glob. Change Biol. 22, 934–943. doi: 10.1111/gcb.13125
Lindeman, R. H., Merenda, P. F., and Gold, R. Z. (1980). Introduction to Bivariate and Multivariate Analysis. Glenview, IL: Scott, Foresman and Company.
López, B., Sabaté, S., and Gracia, C. (2001). Fine-root longevity of Quercus ilex. New Phytol. 151, 437–441. doi: 10.1046/j.0028-646x.2001.00189.x
Lwila, A. S., Mund, M., Ammer, C., and Glatthorn, J. (2021). Site conditions more than species identity drive fine root biomass, morphology and spatial distribution in temperate pure and mixed forests. For. Ecol. Manag. 499:119581. doi: 10.1016/j.foreco.2021.119581
McCormack, M. L., Dickie, I. A., Eissenstat, D. M., Fahey, T. J., Fernandez, C. W., Guo, D., et al. (2015). Redefining fine roots improves understanding of below-ground contributions to terrestrial biosphere processes. New Phytol. 207, 505–518. doi: 10.1111/nph.13363
Meier, I. C., and Leuschner, C. (2010). Variation of soil and biomass carbon pools in beech forests across a precipitation gradient. Glob. Change Biol. 16, 1035–1045. doi: 10.1111/j.1365-2486.2009.02074.x
Meller, S., Frossard, E., Spohn, M., and Luster, J. (2020). Plant nutritional status explains the modifying effect of provenance on the response of beech sapling root traits to differences in soil nutrient supply. Front. For. Glob. Change 3:535117. doi: 10.3389/ffgc.2020.535117
Meyer-Grünefeldt, M., Friedrich, U., Klotz, M., von Oheimb, G., and Härdtle, W. (2015). Nitrogen deposition and drought events have non-additive effects on plant growth - Evidence from greenhouse experiments. Plant Biosyst. 149, 424–432. doi: 10.1080/11263504.2013.853699
Montagnoli, A., Terzaghi, M., Di Iorio, A., Scippa, G. S., and Chiatante, D. (2012). Fine-root seasonal pattern, production and turnover rate of European beech (Fagus sylvatica L.) stands in Italy Prealps: possible implications of coppice conversion to high forest. Plant Biosyst. 146, 1012–1022. doi: 10.1080/11263504.2012.741626
Nadelhoffer, K. J. (2000). The potential effects of nitrogen deposition on fine-root production in forest ecosystems. New Phytol. 147, 131–139. doi: 10.1111/nph.17460
Netzer, F., Schmid, C., Herschbach, C., and Rennenberg, H. (2017). Phosphorus nutrition of European beech (Fagus sylvatica L.) during annual growth depends on tree age and P-availability in the soil. Environ. Exp. Bot. 137, 194–207. doi: 10.1016/j.envexpbot.2017.02.009
Niklas, K. J. (2004). Plant allometry: is there a grand unifying theory? Biol. Rev. 79, 871–889. doi: 10.1017/s1464793104006499
Peñuelas, J., Poulter, B., Sardans, J., Ciais, P., van der Velde, M., Bopp, L., et al. (2013). Human-induced nitrogen–phosphorus imbalances alter natural and managed ecosystems across the globe. Nat. Commun. 4:2934. doi: 10.1038/ncomms3934
Peñuelas, J., and Sardans, J. (2022). The global nitrogen-phosphorus imbalance. Science 375, 266–267. doi: 10.1126/science.abl4827
Pregitzer, K. S., Hendrick, R. L., and Fogel, R. (1993). The demography of fine roots in response to patches of water and nitrogen. New Phytol. 125, 575–580. doi: 10.1111/j.1469-8137.1993.tb03905.x
R Core Development Team (2020). R: A Language and Environment for Statistical Computing. Vienna: R foundation for statistical computing.
Reich, P. B., Luo, Y. J., Bradforde, J. B., Poorter, H., Perry, C. H., and Oleksyn, J. (2014). Temperature drives global patterns in forest biomass distribution in leaves, stems, and roots. Proc. Natl. Acad. Sci. U.S.A. 111, 13721–13726. doi: 10.1073/pnas.1216053111
Robinson, D. (2007). Implications of a large global root biomass for carbon sink estimates and for soil carbon dynamics. Proc. R. Soc. B 274, 2753–2759. doi: 10.1098/rspb.2007.1012
Schneider, K., Turrion, M.-B., Grieson, P. F., and Gallardo, J. F. (2001). Phosphatase activity, microbial phosphorus, and fine root growth in forest soils in the Sierra de Gata, western central Spain. Biol. Fertil. Soils 34, 151–155. doi: 10.1007/s003740100387
Shapiro, S. S., and Wilk, M. B. (1965). An analysis of variance test for normality (complete samples). Biometrika 52, 591–611. doi: 10.1093/biomet/52.3-4.591
Spohn, M., Zavišić, A., Nassal, P., Bergkemper, F., Schulz, S., Marhan, S., et al. (2018). Temporal variations of phosphorus uptake by soil microbial biomass and young beech trees in two forest soils with contrasting phosphorus stocks. Soil Biol. Biochem. 117, 191–202. doi: 10.1016/j.soilbio.2017.10.019
Talkner, U., Meiwes, K., Potočič, N., Seletković, I., Cools, N., De Vos, B., et al. (2015). Phosphorus nutrition of beech (Fagus sylvatica L.) is decreasing in Europe. Ann. For. Sci. 72, 919–928. doi: 10.1007/s13595-015-0459-8
van Dijk, H. G., De Louw, M. H. J., Roelofs, J. G. M., and Verburgh, J. J. (1990). Impact of artificial, ammonium-enriched rainwater on soils and young coniferous tress in a greenhouse. Part II - Effects on the trees. Environ. Pollut. 63, 41–59. doi: 10.1016/0269-7491(90)90102-i
Venables, W. N., and Ripley, B. D. (2002). Modern Applied Statistics with S, 4th Edn. New York, NY: Springer.
Vitousek, P. M., Porder, S., Houlton, B. Z., and Chadwick, O. A. (2010). Terrestrial phosphorus limitation: mechanisms, implications, and nitrogen–phosphorus interactions. Ecol. Appl. 20, 5–15. doi: 10.1890/08-0127.1
Vogt, K. A., Vogt, D. J., Palmiotto, P. A., Boon, P., O’Hara, J., and Asbjornsen, H. (1996). Review of root dynamics in forest ecosystems grouped by climate, climatic forest type and species. Plant Soil 187, 159–219. doi: 10.1007/BF00017088
Whitehead, D. (2011). Forests as carbon sinks—benefits and consequences. Tree Physiol. 31, 893–902. doi: 10.1093/treephys/tpr063
Zavišić, A., Nassal, P., Yang, N., Heuck, C., Spohn, M., Marhan, S., et al. (2016). Phosphorus availabilities in beech (Fagus sylvatica L.) forests impose habitat filtering on ectomycorrhizal communities and impact tree nutrition. Soil Biol. Biochem. 98, 127–137. doi: 10.1016/j.soilbio.2016.04.006
Zavišić, A., and Polle, A. (2018). Dynamics of phosphorus nutrition, allocation and growth of young beech (Fagus sylvatica L.) trees in P-rich and P-poor forest soil. Tree Physiol. 38, 37–51. doi: 10.1093/treephys/tpx146
Zederer, D. P., and Talkner, U. (2018). Organic P in temperate forest mineral soils as affected by humus form and mineralogical characteristics and its relationship to the foliar P content of European beech. Geoderma 325, 162–171. doi: 10.1016/j.geoderma.2018.03.033
Keywords: soil fertility, phosphorus, nitrogen, Fagus sylvatica, fine roots, seasonal variation, mineral soil, organic soil
Citation: Likulunga LE, Clausing S, Krüger J, Lang F and Polle A (2022) Fine root biomass of European beech trees in different soil layers show different responses to season, climate, and soil nutrients. Front. For. Glob. Change 5:955327. doi: 10.3389/ffgc.2022.955327
Received: 28 May 2022; Accepted: 30 August 2022;
Published: 20 September 2022.
Edited by:
Arshad Ali, Hebei University, ChinaReviewed by:
Hu Bin, Southwest University, ChinaChang-Bae Lee, Kookmin University, South Korea
Thomas J. Dean, Louisiana State University, United States
Copyright © 2022 Likulunga, Clausing, Krüger, Lang and Polle. This is an open-access article distributed under the terms of the Creative Commons Attribution License (CC BY). The use, distribution or reproduction in other forums is permitted, provided the original author(s) and the copyright owner(s) are credited and that the original publication in this journal is cited, in accordance with accepted academic practice. No use, distribution or reproduction is permitted which does not comply with these terms.
*Correspondence: Likulunga Emmanuel Likulunga, llikulu@gwdg.de