- College of Forestry, Hebei Agricultural University, Baoding, China
Intra-annual monitoring of stem radial growth dynamics of trees and detecting how tree growth responds to changing climatic conditions are crucial for predicting the future growth dynamics under increasing drought conditions. Here, we monitored the intra-annual stem radial growth dynamics of seven Larix principis-rupprechtii using point dendrometers to investigate the influence of climate variables on the intra-annual growth of L. principis-rupprechtii in the growing season. The average stem radial growth of L. principis-rupprechtii started on 29 April and stopped on 17 August based on the sigmoid Gompertz functions. The intra-annual stem radial growth of L. principis-rupprechtii showed a parabolic trend, with its growth decreased when the temperature and sunlight duration hours exceeded certain thresholds. The vapor pressure deficits (VPD) strongly influenced tree intra-annual growth over other climatic factors in the growing season. Stem radial growth of L. principis-rupprechtii decreased significantly with increasing VPD when VPD ranged from 0.5 to 0.8 kPa. Intra-annual stem radial growth of L. principis-rupprechtii was severely inhibited when VPD was higher than 0.8 kPa. In contrast, tree stem radial growth reached the maximum when VPD was lower than 0.5 kPa. Our study highlighted the important influences of major limiting climatic factors on the stem radial growth of trees in semi-arid regions.
Introduction
Climate changes significantly influenced intra-annual tree growth and eventually affected forest productivity (McDowell et al., 2008; Deslauriers et al., 2017). Changing climatic conditions influenced the stem radial growth of trees to different degrees (Betsch et al., 2011; Cabon et al., 2020; Zhang Q. et al., 2022). Growth declines due to rapid warming have been observed in Dahurian larch populations in the western Daxingan Mountains of northeastern China (Zhang X. L. et al., 2016). High vapor pressure deficits (VPD) due to changes in temperature and moisture conditions has become a significant limiting factor for the intra-annual growth of trees (Battipaglia et al., 2014; Trotsiuk et al., 2021). Therefore, understanding the response of the stem radial growth variation to climate factors is crucial to predict tree stem growth under future climate change.
Dendrometer provides a continuous automatic recording of intra-annual stem radial growth processes without destroying samples (Zweifel et al., 2001; Deslauriers et al., 2003). It is widely used in studies exploring the relationship between intra-annual stem changes in trees and climate change (Zweifel and Hasler, 2000; Oberhuber et al., 2014). Stem radius fluctuations recorded by dendrometer include growth-induced irreversible stem expansion and tree water deficit-induced reversible stem shrinkage (Deslauriers et al., 2007). Tree stem growth can be separated from daily variation in water effects by using a zero-growth model (Zweifel et al., 2016). Temperature was the most important factor limiting tree stem radial growth at high altitudes and latitudes (Turcotte et al., 2009; Li et al., 2013). Stem radial increment was positively correlated with temperature before the growing season, while it was mainly limited by precipitation and relative humidity after early July for Taiwan pine (Liu X. S. et al., 2018). Therefore, exploring the intra-annual radial growth of trees is essential to understand the relationship between tree stem growth and climate.
Many large-scale forestry projects have been recently carried out in northern China (Zhang Y. et al., 2016). Larix principis-rupprechtii is one of the main species used in these projects. L. principis-rupprechtii is widely distributed in the mountainous areas of north-central China and is the main species in the alpine coniferous forest belt of North China. However, planted forests were sensitive to climate change (Payn et al., 2015). L. principis-rupprechtii had significantly lower resistance and resilience than other tree species during extreme drought (Xiao et al., 2021). Rapid warming has led to a growth decline in the southern edge of larch populations (Liu Y. Y. et al., 2018). Traditional dendrochronology usually focuses on analyzing the relationship between inter-annual tree growth and climatic factors (Evans et al., 2017; Jiang et al., 2018). How the intra-annual stem radial growth of L. principis-rupprechtii is affected by climate factors is still lacking.
Here, we investigated the intra-annual daily stem growth of L. principis-rupprechtii and its responses to climatic factors based on high-resolution dendrometer data. The aims of our study were to: (a) analyze the relationship between the intra-annual growth of L. principis-rupprechtii and climate factors to clarify the major limiting climate factors and (b) explore the response of intra-annual stem radial growth of L. principis-rupprechtii to changes in major climatic factors.
Materials and methods
Study area
The study area is located in the mountainous regions of north-central China (41.52°N, 117.40°E, Figure 1A). It is a semi-arid and semi-humid climate zone. Long-term records (1990–2021) from the meteorological station in WeiChang county (41.58°N, 117.46°E) were obtained from China Meteorological Administration.1 The annual mean temperature is 5.5°C. The coldest month is January, with an average temperature of -12.4°C and an extreme minimum of 23.7°C. The hottest month is July, with an average temperature of 21.3°C (Figure 2). Annual precipitation ranges from 300 to 560 mm. Precipitation is mainly concentrated in summer, with June to August accounting for more than 60% of the annual precipitation. The main types of soil in the mountainous areas of the study region are gray forest soil, black calcium soil, and light black calcium soil (Wang et al., 2015).
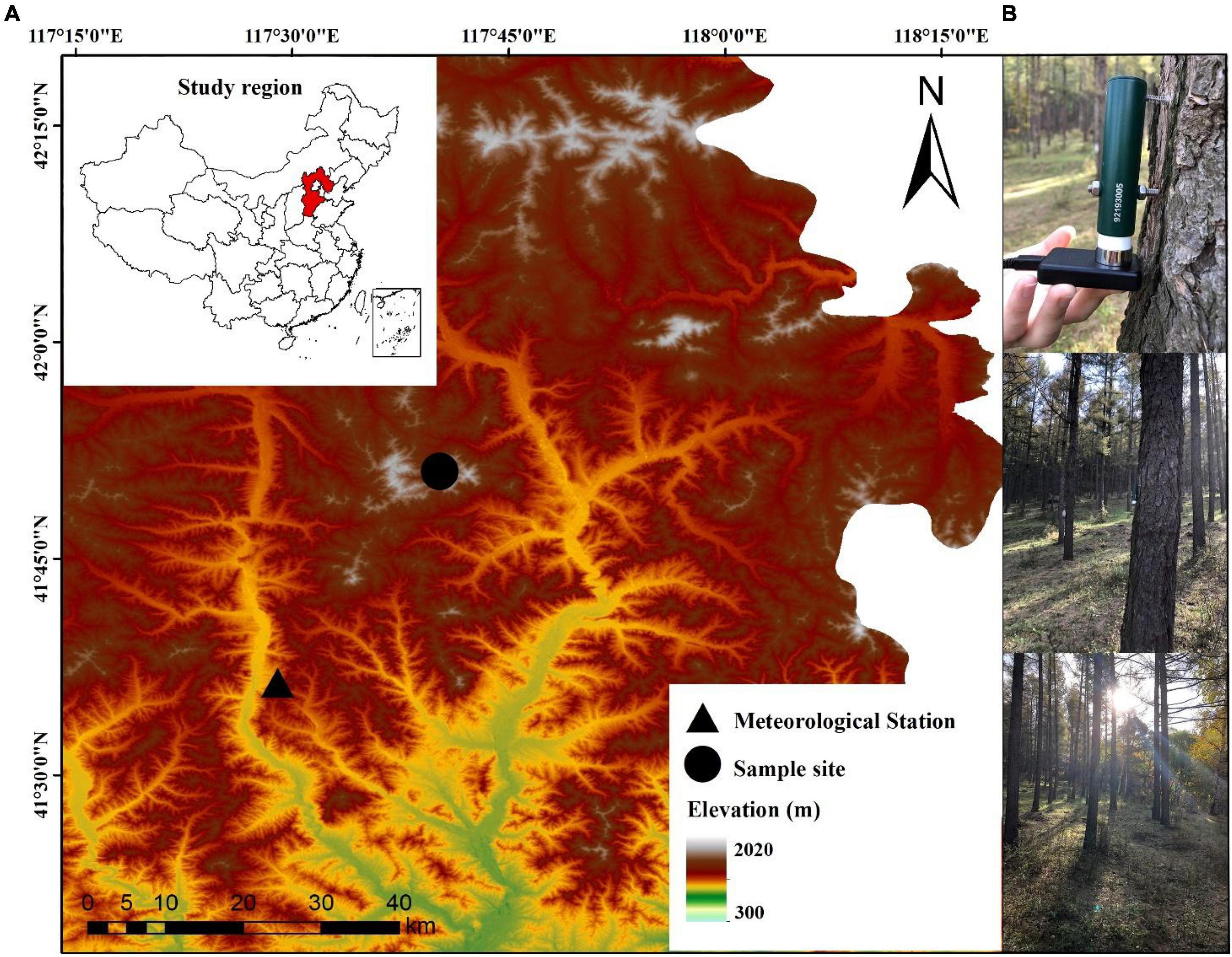
Figure 1. (A) Location of the monitoring site (black circle) and the nearest meteorological station (black triangle). (B) Pictures show the forest structure and composition of our monitoring site, from top to bottom.
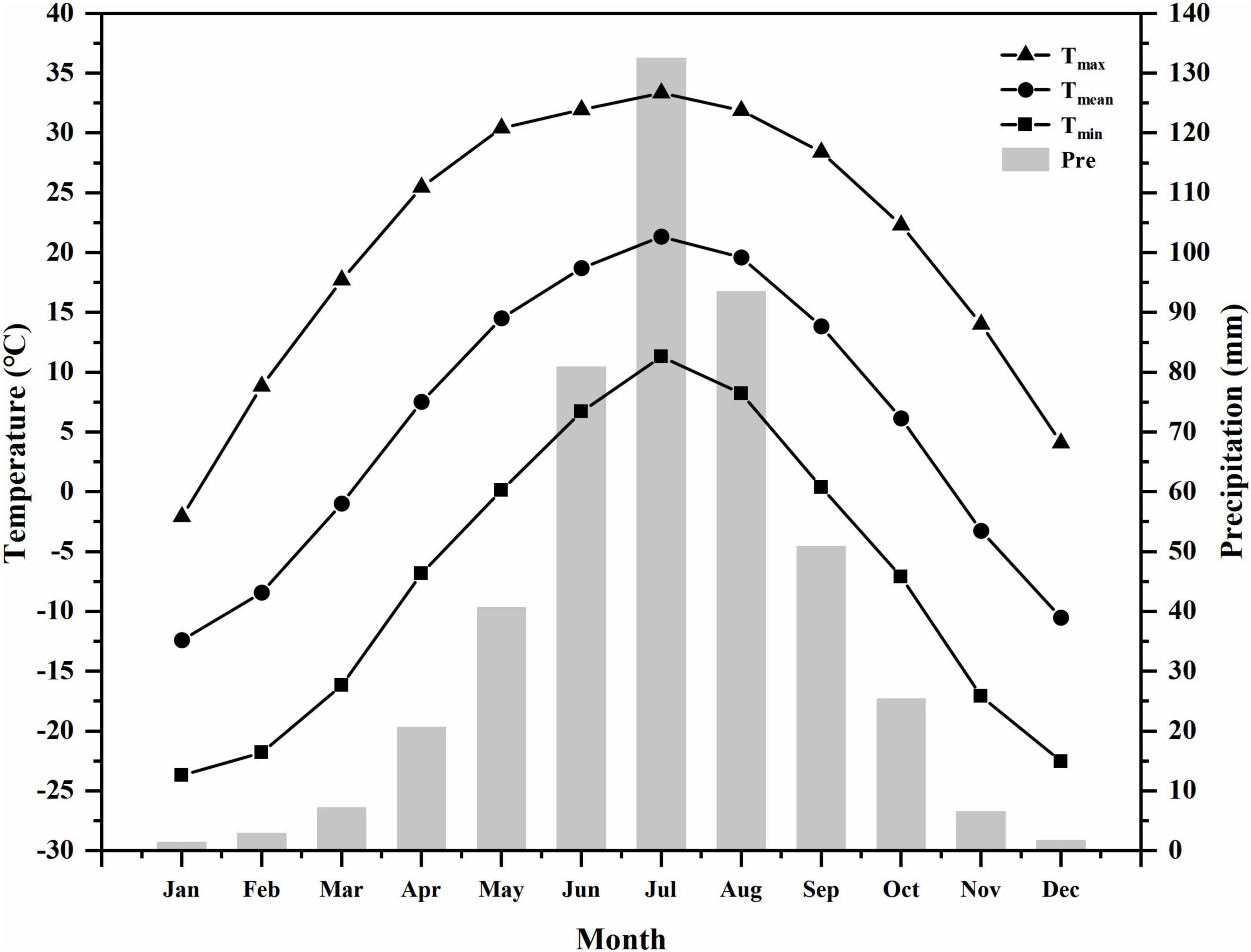
Figure 2. Monthly maximum (Tmax), mean (Tmean), and minimum (Tmin) temperatures and monthly precipitation (Pre) for WeiChang county from 1990 to 2021.
L. principis-rupprechtii is the dominant tree species in the study area (Figure 1B). The main shrub species are Padus racemosa, Populus davidiana Dode, Sorbus pohuashanensis, Lespedeza daurica, Rosa davurica, etc. The understory herbs are Carex lanceolata, Juncus effusus, Potentilla longifolia, Sanguisorba officinalis, Thalictrum peticulata, etc.
Data collection
Dendrometer measurements
Dendrometer can monitor stem radial growth changes in trees and characterize growth-climate relationships with high temporal resolution without substantial damage to the formative layer and woody tissue (Deslauriers et al., 2003). The sensing probe can sense the stem radial variation of the tree and convert it into a current signal, which is transmitted to the dendrometer data logger. Dendrometers could record intra-annual stem radial growth (Deslauriers et al., 2003; Vieira et al., 2013; Oberhuber et al., 2014). In July 2020, we selected seven well-grown L. principis-rupprechtii trees with relatively straight trunks in the study area (1308 m). The stem radial variations include reversible and irreversible processes (Zweifel et al., 2006). To retain a more realistic stem radial growth signal, we used a knife to carefully remove the outermost outer bark and dead bark tissue without damaging the cambium to ensure close contact of the dendrometer with the stem to reduce the impact of bark expansion and contraction on the dendrometer data and then installed the dendrometer (Model: D1, measurement range 8890 μm, linearity < 5%, resolution: 0.27 μm thermal expansion coefficients of < 0.1 μm/K, TMOST, Praha, Czechia). All point densitometers were installed at 1.3 m breast height on each sampled tree. The monitoring period spanned from July 2020 to September 2021. Daily trunk shrinkage and swelling dynamics were recorded at 15-min intervals, with 96 data recorded daily. Considering that transpiration during the daytime causes stems shrinkage (Zweifel et al., 2001), we chose to take readings during the morning hours (7:30 to 8:30 a.m.) to reduce the bias caused by transpiration during the daytime hours.
Climatic data
We obtained daily climate data from the China Meteorological Administration (see text footnote 1) for the WeiChang station (41.58°N, 117.46°E, Figure 1), which is 34.7 km far from the study area. Daily precipitation (Pre), air temperature (mean, Tmean; minimum, Tmin; and maximum, Tmax), soil temperature (T), relative humidity (RH), and sunshine duration (SD) were collected. The vapor pressure deficit (VPD) was calculated based on air temperature (T) and relative humidity (RH) using the following equation (Campbell and Norman, 1998):
where a = 0.6108, b = 17.27, c = 237.3.
Historical and future monthly temperature and relative humidity data from CMIP 5 Models were downloaded from the following website http://climexp.knmi.nl/selectfield_cmip5.cgi?id=someone@somewhere. The gridded data in the study area were selected from multi-model ensemble simulations to calculate the regional mean temperature and relative humidity. Historical and projected VPD were calculated based on temperature and relative humidity for the RCP26 scenario for the period 1990–2100.
Extraction of stem radial variation and determination of growing season
We used high-precision dendrometers to monitor the daily stem radial growth variation of L. principis-rupprechtii, recording 96 data per day. The maximum value was defined as the maximum measurement value of the daily cycle in a day (Bouriaud et al., 2005). The daily maximum values from tree stem radius data were extracted by the “dendRoAnalyst” package of the R software (R Core Team, 2010; Aryal et al., 2020) to obtain the maximum daily stem radial variation series for each tree. The data from seven monitored trees were averaged to obtain the average growth condition of the species. The difference between the maximum values for two consecutive days was calculated as the daily radial change (SRC) of tree trunks. The sigmoid Gompertz growth model was used to fit the cumulative daily stem radial growth variation curve to simulate the intra-annual stem radial growth process because of its flexibility and asymmetry (Deslauriers et al., 2003; Duchesne et al., 2012). The function was as follows:
where y is the daily stem radial growth maximum raw measurements; A is the upper asymptote; β is the x-axis placement parameter; k is the rate of change parameter; and t is time (expressed as a day of the year, DOY).
Based on the results of the model, the onset and end days of tree stem radial growth were defined when 5 and 95% of the total cumulative radial variation were reached. The length of the growing season was the difference between the onset and end days (van der Maaten et al., 2018).
A zero-growth model was used to distinguish between tree water deficit-induced reversible stem shrinkage (TWD) and growth-induced irreversible stem expansion (GRO) (Zweifel et al., 2016). The model assumes that cells grow stagnant when the stem radius is less than the previous maximum (saturated state). Under this assumption, the irreversible growth processes and reversible water fluctuations are separated from continuous stem radius fluctuations (Figure 3). Stem growth was defined as the process in which the stem radius exceeds the last great value. The daily stem radial increase (SRI) was the difference between the GRO of two consecutive days.
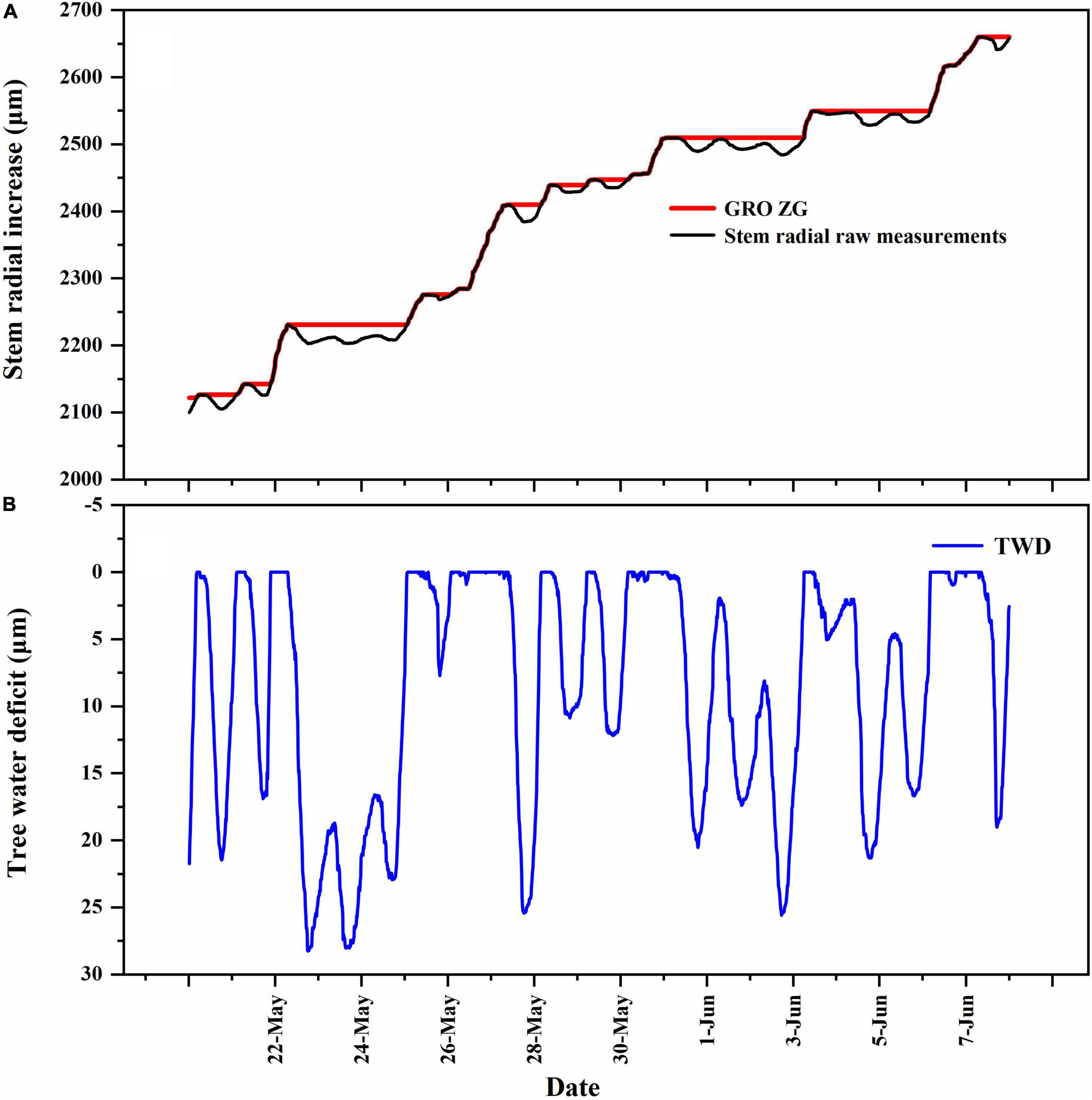
Figure 3. Tree stem radial raw measurements (black line, A) and the growth-induced irreversible stem expansion (GRO) according to the zero growth concept (red line, B). The difference between irreversible growth and stem radial raw measurements is tree water deficit (TWD) (blue line, B). Data are for the period from May 21 to June 8 in 2021.
The growth process of the zero model was calculated as follows:
where t is the current moment; < t is from the beginning to the current moment; GRO is growth-induced irreversible stem expansion; SR is the stem radius fluctuations.
Statistical analysis
Spearman correlation analysis was used to analyze the relationship between SRI and meteorological factors (e.g., VPD, Air temperature, Precipitation, Relative humidity). A machine learning algorithm, Random Forests, was used to represent variable importance (VIM) and to rank the important variables (Anderegg et al., 2018). The importance of climatic factors on the irreversible growth of L. principis-rupprechtii was assessed using Random Forests. Linear mixed models (LMM) were used to analyze the relative contribution of different climate variables to SRI. After excluding the less correlated climatic factors by the model selection, we selected the climatic factors which were significantly correlated to growth as fixed effects. Individual trees and years were set as random effects. The influence of different climate variables on daily stem radial growth was analyzed by assessing standardized regression coefficients to understand the relatively important climatic factors affecting stem radial growth. The model was built using the “nlme” package of the R software (Pinheiro et al., 2015).
To quantify the response of intra-annual stem radial growth of L. principis-rupprechtii to climate factors, we grouped Tmean per degree Celsius (°C) and the sunshine duration (SD) per 1 hour within their respective ranges in 2021. The curves were fitted with the parabolic functions. To obtain threshold values of VPD and its relationship with the GRO variation of L. principis-rupprechtii, we fitted the logistic curves to assess how L. principis-rupprechtii intra-annual growth variation varies with different levels of VPD. VPD was divided into eight different scales on a gradient of 0.1 kPa. Since fewer VPD values lay between 0–0.4 and 1.0–1.5 kPa, we combined these bins. The monitoring period was relatively short, and the data for 2020 was incomplete, so the end time was referenced to the end of stem radial growth in 2021.
Results
Growth season identification
The Gompertz curves explained 96 and 99% of the change in daily maximum stem radial data for L. principis-rupprechtii in 2021 (Supplementary Table 1). The stem radial growth curves of L. principis-rupprechtii were all “S” shaped (Figure 4A). The stem radial growth rate of the tree was single-peaked and showed a slow-fast-slow trend (Figure 4B). The onset days of the stem radial growth in L. principis-rupprechtii in 2021 ranged from 12 April to 15 May (DOY 102–135), and the cessation of stem growth ranged from 20 July to 4 August (DOY 219–249). The average stem radial growth started on 29 April and stopped on 17 August 2020 (Table 1). Consequently, the average growing season lasted 110 days. The average cumulative growth of the seven sample trees was 2.21 mm ± 1.12 mm, and the maximum daily growth rate was 30.7 μm ± 19.4 μm (Table 1).
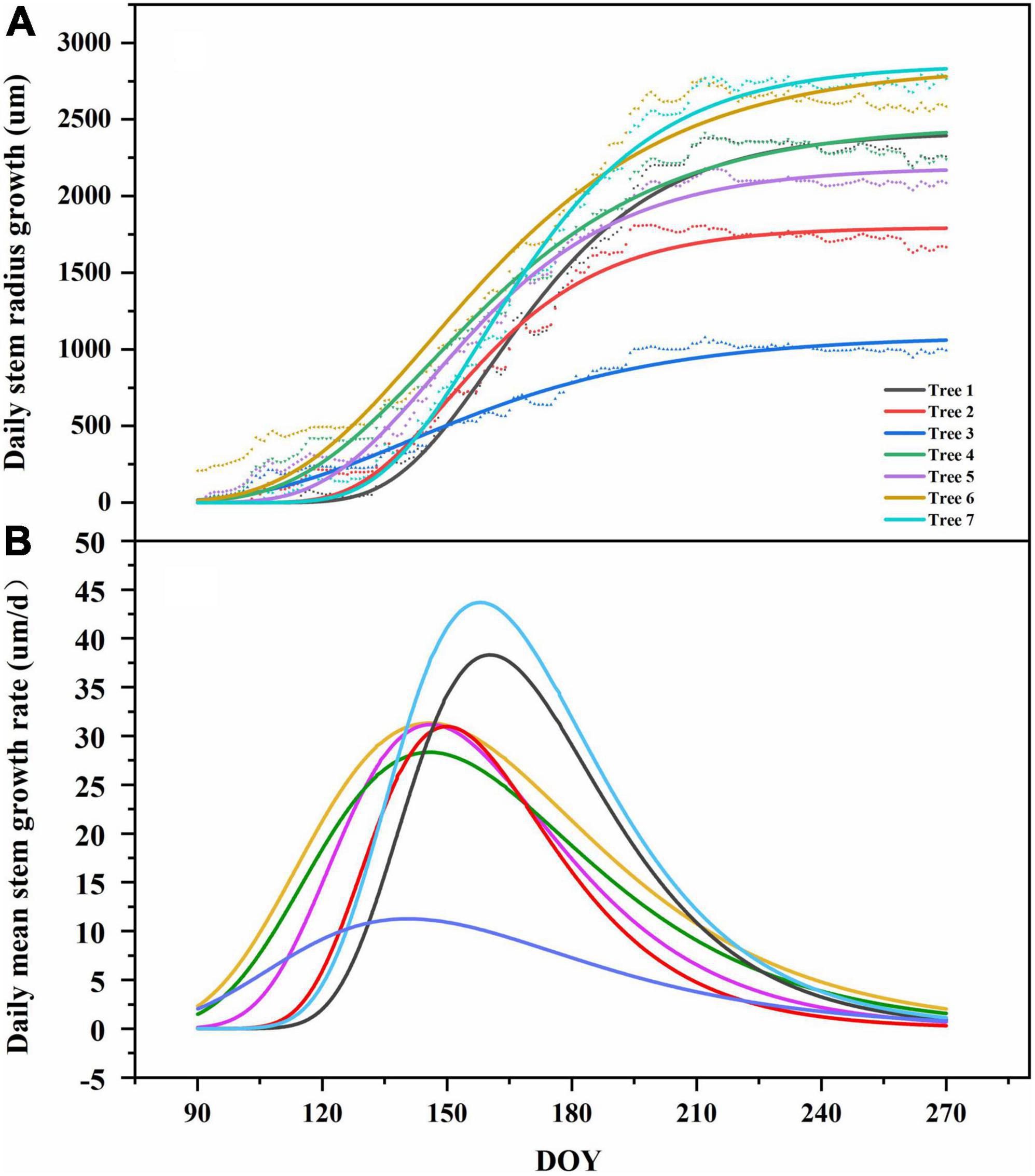
Figure 4. Observed and modeled stem radial increment (A) and daily growth rates of seven L. principis-rupprechtii trees in 2021 (B). The points and lines indicate the daily maximum radius and the original measurements of the sigmoid Gompertz function model curves.
Relationship between stem radial growth and climatic factors
Precipitation in July–August 2020 was significantly less than the same period in 2021. Mean VPD in July–August 2021 was 0.42 kPa, with a maximum of 0.82 kPa, while mean VPD in the same period was 0.54 kPa with a maximum of 1.27 kPa in 2020, the average VPD was 0.12 kPa higher compared to 2021.
Stem radial increase had significant differences between the 2 years (Figure 5). SRC ranged from -24 to 97 μm in July–August of 2021, compared to a maximum of 120 μm and a minimum of -97 μm in July–August of 2020. SRC and VPD showed a significant negative correlation during the growing season (p < 0.001, Supplementary Table 2).
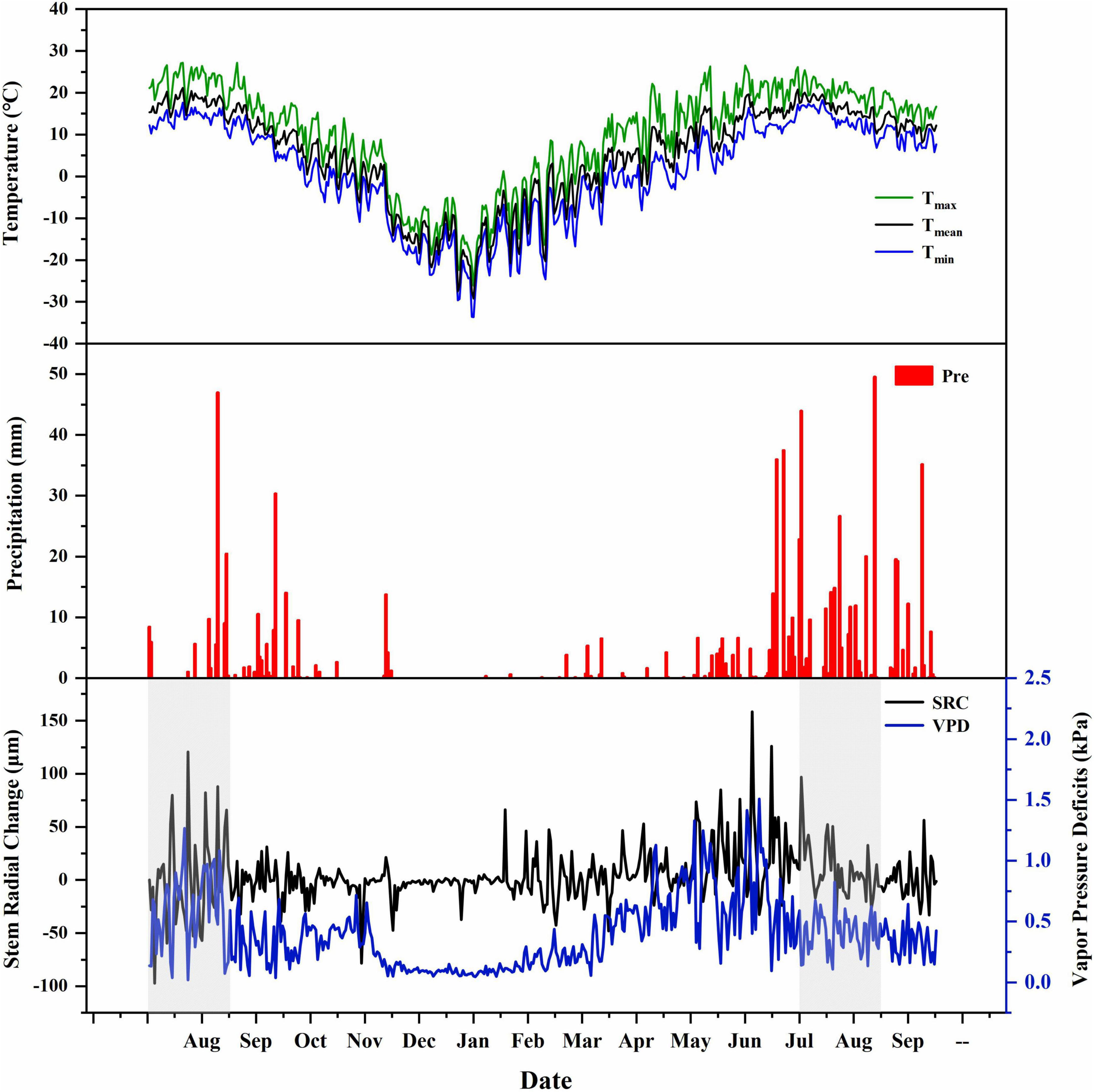
Figure 5. Daily climatic conditions at the meteorological stations in WeiChang station during the monitoring period from July 2020 to September 2021. From top to bottom: daily minimum (blue), average (black), and maximum (green) temperature; precipitation (red); changes in stem radial change (black line: SRC) of mean trees and vapor pressure deficits (blue line: VPD). The shaded part is the comparison of SRC and VPD in the same period in 2 years.
Stem radial increase of L. principis-rupprechtii was significantly and positively correlated with precipitation and relative humidity (RH). Mean soil temperature (ST), maximum air temperature (Tmax), vapor pressure deficit (VPD), and sunshine duration (SD) had negative influences on daily stem radial growth during the growing season (Figure 6).
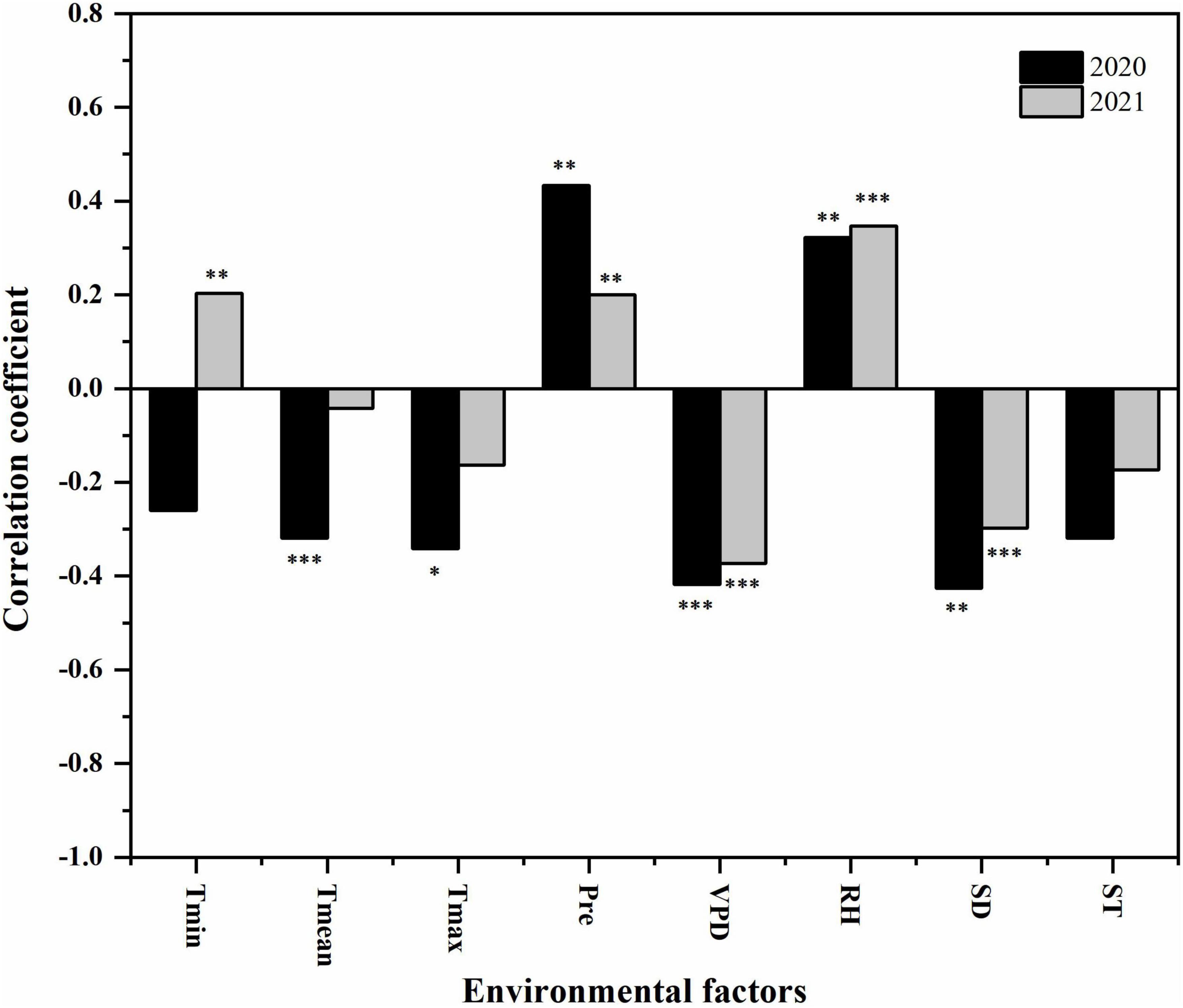
Figure 6. Spearman correlations of SRI and air temperature (mean, Tmean; minimum, Tmin; and maximum, Tmax), precipitation (Pre), relative humidity (RH), vapor pressure deficit (VPD), sunshine duration (SD), and soil temperature (ST) in 2020 and 2021. Significance levels (*p < 0.05; **p < 0.01; ***p < 0.001).
The results from Random Forests showed that VPD had the highest value of the increase in mean squared error (%IncMSE), indicating that VPD mainly influenced the SRI in both 2020 and 2021 (Supplementary Figure 1). LMM analyzed the contribution of each climatic factor to the SRI in L. principis-rupprechtii. The results of LMM confirmed that the absolute value of the standardized regression coefficient for VPD was the largest one (Table 2 and Supplementary Figure 2). The intra-annual stem radial growth of L. principis-rupprechtii was relatively more influenced by VPD.
The results of the parabolic fit between the intra-annual stem radial growth in 2021 and climate factors of L. principis-rupprechtii showed that the highest growth increase occurred at an optimum sunshine duration of about 7 h (Figure 7A). L. principis-rupprechtii grew most rapidly when the temperature at approximately 18°C (Figure 7B).
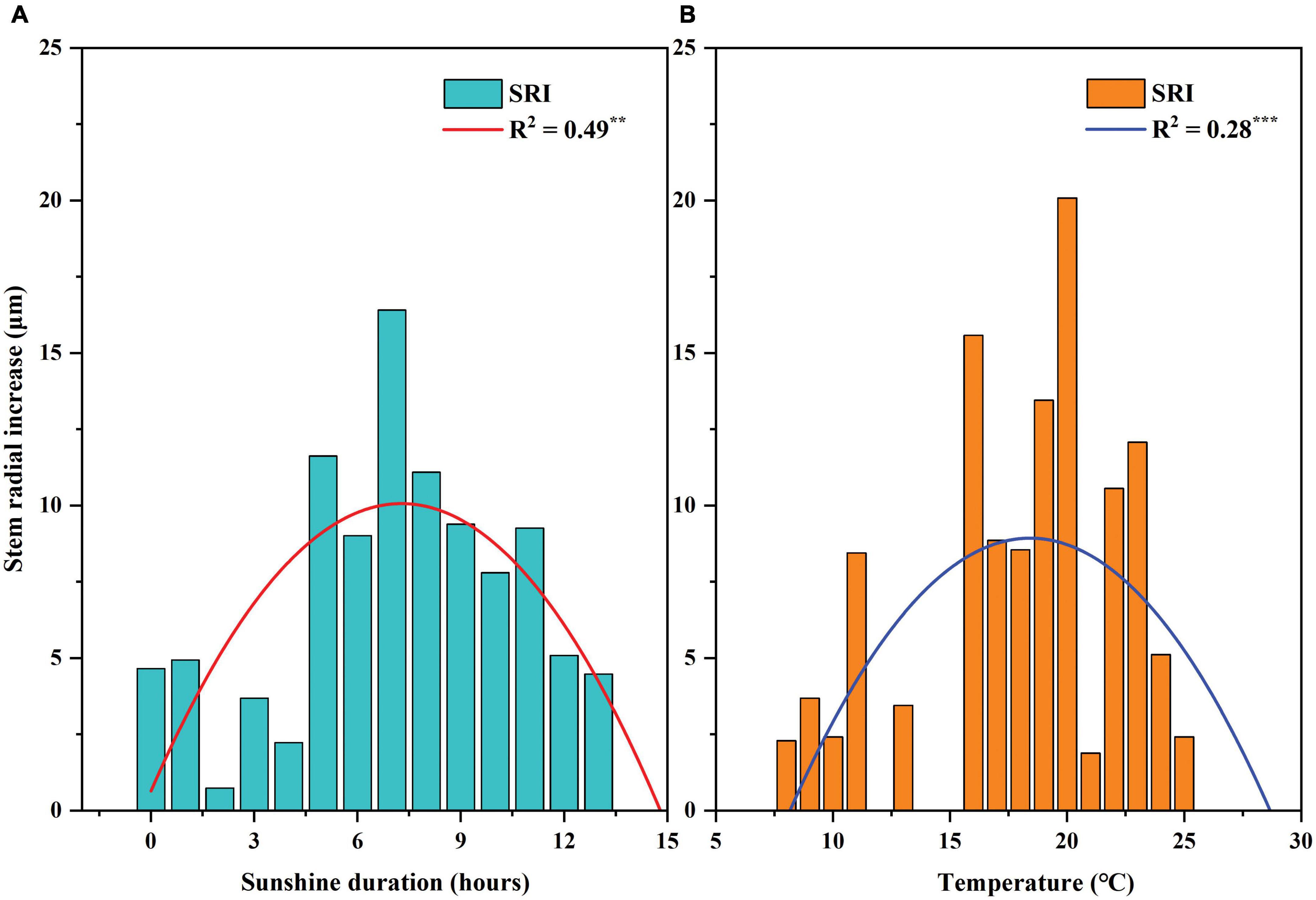
Figure 7. Changes in the intra-annual stem radial growth with sunshine duration (A) and temperature (B) of L. principis-rupprechtii. The bars represent the variation of L. principis-rupprechtii on different gradients of climate factors. The blue and red parabolas are fitted curves of the trends in 2021. Significance levels (***p < 0.001, **p < 0.01).
Threshold values of vapor pressure deficits on the stem radial growth
Logistic models explained 98% (2020) and 99% (2021) of the total variation between GRO and VPD in L. principis-rupprechtii (Supplementary Table 3). The intra-annual stem radial growth (2020–2021) comparison demonstrated that the intra-annual stem growth of L. principis-rupprechtii showed different sensitivity to changes in VPD ranges (Figure 8). When VPD < 0.5 kPa, GRO was less influenced by VPD, and tree stem growth reached a maximum value. Radial stem growth was significantly reduced when VPD ranged at 0.5 kPa < VPD < 0.6 kPa. The GRO of L. principis-rupprechtii was severely restricted when VPD was above the range of 0.8–0.9 kPa.
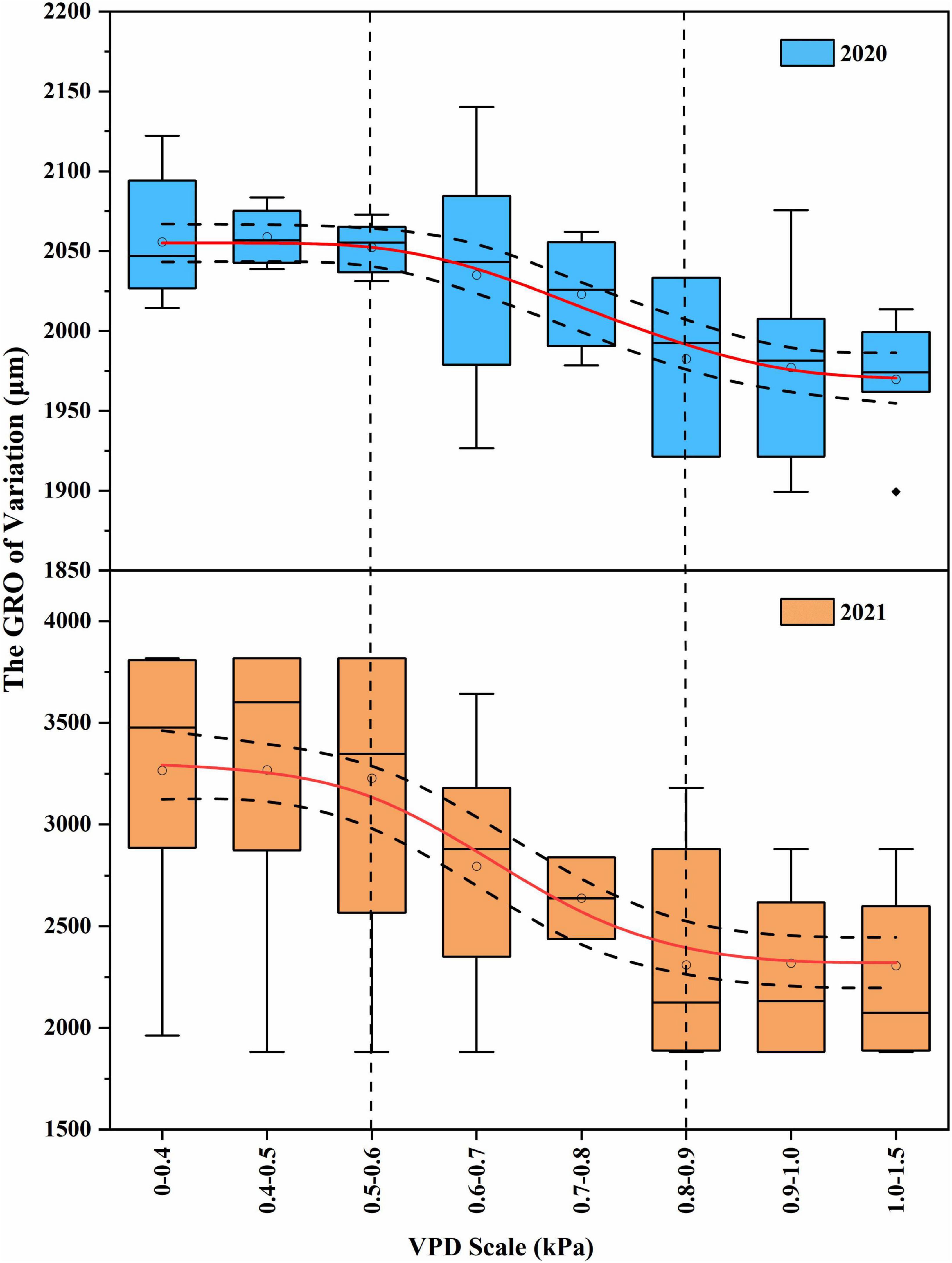
Figure 8. The boxplot of the GRO variation of trees for different VPD threshold ranges in 2020 and 2021. The stem radial growth decreases with increasing VPD. The dashed part is the confidence interval obtained with the Logistic models.
Future changes in vapor pressure deficits
From 1990 to 2020, the mean vapor pressure deficit (MVPD) in May–July showed an increasing trend, and VPD already exceeded 0.8 kPa in 2020 (Figure 9A). In the future, VPD may continue to rise in our study area. MVPD will increase significantly during 2021–2050 (Figure 9B). From 2051 to 2100, MVPD will fluctuate horizontally with no clear trend. It is worth noting that MVPD values will always be above the 0.8 kPa level from 2051 to 2100 (Figure 9C).
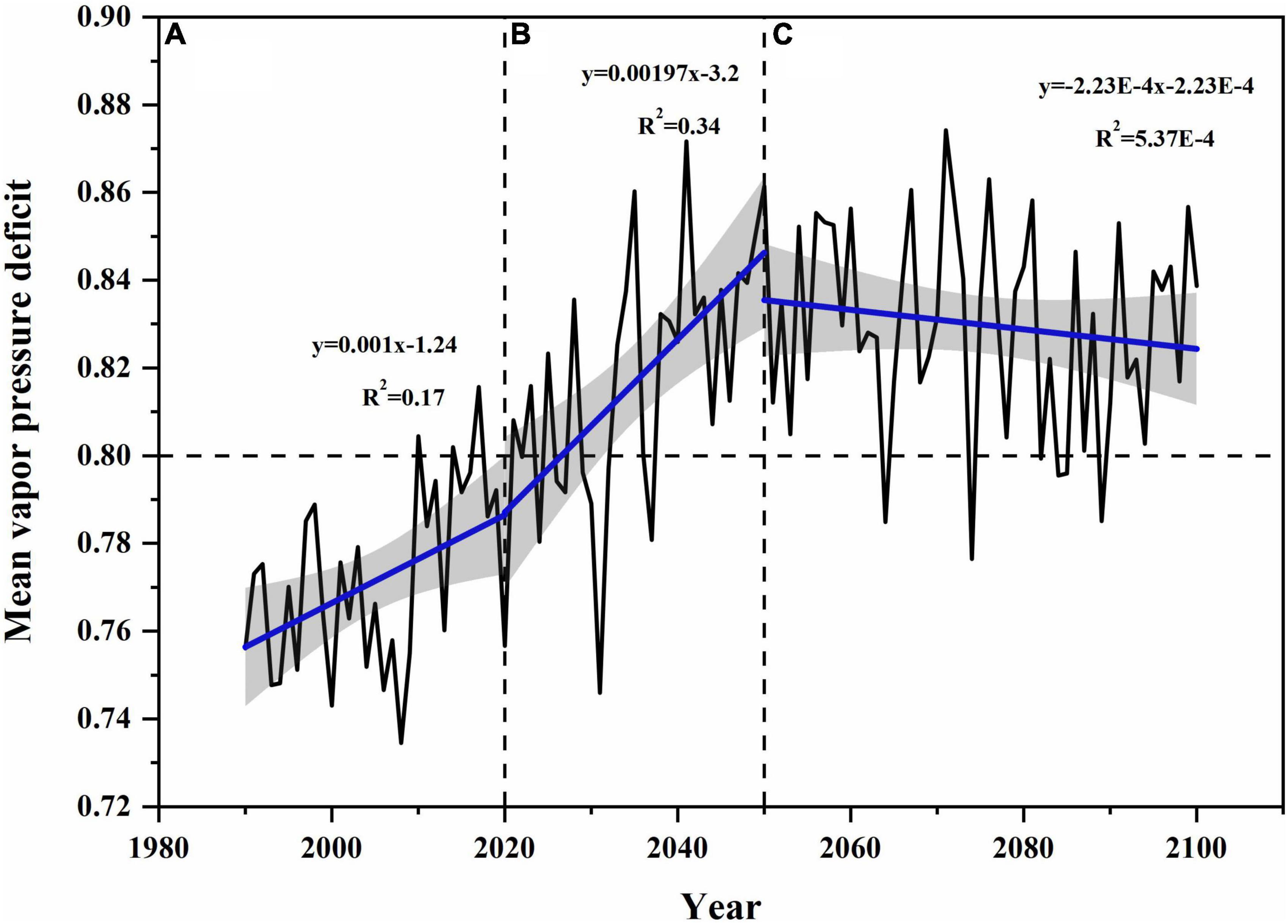
Figure 9. Trends of mean vapor pressure deficit (MVPD) in May–July during the period 1990–2020 (A) and future projections of VPD for 2020–2050 (B) and 2051–2100 (C). The gray shaded areas are the confidence interval obtained with the linear fit.
Discussion
Impact of environmental factors on intra-annual stem radial growth
Tree growth was influenced by multiple climatic factors (Zhang et al., 2019; Zhang X. et al., 2022). Temperature affected the transport and distribution of the products of photosynthesis (Rossi et al., 2008; Deslauriers et al., 2016). In general, the positive effect of temperature on stem radial growth may be associated with the optimum temperature (Buras et al., 2017; Oladi et al., 2017). The maximum stem radial growth of L. principis-rupprechtii roughly corresponded to an average temperature of 18°C during the growing season (Figure 7B). However, higher temperatures and longer sunshine durations (> 7 h, Figure 7A) led to a decrease in relative humidity and an increase in VPD, which increased water stress in L. principis-rupprechtii (Zhang Q. et al., 2022). By contrast, the positive effect of precipitation and relative humidity on tree stem radial growth may be explained by swelling trunk hydrological processes (Supplementary Figure 3). Rainfall events can alleviate water deficit in tree trunks by increasing soil moisture and promote trunk cell expansion and growth (Steppe et al., 2006; Urrutia-Jalabert et al., 2015). The effects of moist atmospheric conditions on stem radial growth were manifest in short time scales (Oberhuber et al., 2014). In addition, rainfall events can reduce the demand for internal plant water, and rainwater intercepted by tree branches can be used to meet the transpiration demand of canopy (Katz et al., 1989; Deslauriers et al., 2007).
The influences of VPD on intra-annual stem radial growth of L. principis-rupprechtii was relatively stronger than other climatic factors, which indicated that moisture played a significant role in stem radial growth (Figure 6 and Table 2). Water deficiency directly affected cell differentiation in the xylem-forming layer (Rossi et al., 2013). Stomata partially close with increasing VPD, which reduces cell expansion pressure in the forming layer region of the tree (Kocher et al., 2012) and ultimately results in a decrease in radial stem growth (Cornic, 2000; Lawlor and Cornic, 2002). Higher temperatures negatively affected stem radial increment rates, probably due to increased water VPD and increased evaporative dissipation in dry forests (Mendivelso et al., 2016).
L. principis-rupprechtii mainly depended on deep soil moisture during the growing season (Zhang et al., 2018). The effect of soil moisture on tree root uptake was the dominant cause of cell division in tree forming layer, but the restriction of water conditions within the plant on tree stem radial growth began manifest before soil moisture was restricted (de Carcer et al., 2018; Xue et al., 2022). In addition, the diurnal trend of trunk shrinkage and expansion in the growing season of L. principis-rupprechtii (Supplementary Figure 4) was caused by the strong coupling between water potential gradient and transpiration process (Zweifel and Hasler, 2000). Transpiration came from water stored in the stems of the trees rather than soil moisture (Cermak et al., 2007; Betsch et al., 2011). A correlation between diurnal stem radial variation curve of trees and tree transpiration has been found in semi-arid forests of northern China (Ji et al., 2020).
Threshold effects of vapor pressure deficits on the GRO variation of Larix principis-rupprechtii
Our results demonstrate that VPD has threshold effects on the GRO variation of L. principis-rupprechtii. Water storage capacity can provide a significant proportion of daily and seasonal water consumption (Cermak et al., 2007). As VPD rises, trees face higher evapotranspiration demands and more severe soil water deficits. Trees had to draw on internal water storage to maintain transpiration demand (Zweifel et al., 2001; De Schepper and Steppe, 2010). When VPD reaches a certain high threshold, there is not enough water inside trees to replenish the stem from daytime losses, which can turn into strong plant water stress. Tree stems may experience more intense shrinkage in this case, which eventually affects the stem radial growth by reducing cell expansion pressure (Dai, 2013). That is why stem radial growth was strongly reduced when VPD exceeded a specific threshold range (0.5 kPa < VPD < 0.6 kPa), and the stem radial growth of L. principis-rupprechtii was severely limited when VPD > 0.8 kPa (Figure 8). These results are consistent with the results that the effect of drought on radial growth had been the most dominant for larch species in semi-arid regions in recent years. For example, the radial growth of L. principis-rupprechtii reduced by 62% at PDSI < -4.5 (Zhang et al., 2021). High VPD was found to be detrimental to stem radial growth in all species during the main growing season in drought-prone mixed conifer forests in high mountains (Oberhuber et al., 2014).
The intra-annual radial growth of trees responded differently to different VPD thresholds. Trees can control stomata to regulate water loss in transpiration (Brodribb and Holbrook, 2003; Zweifel et al., 2006). Stomata of most tree species began to respond at VPD > 0.5 kPa (Oren et al., 1999; Grossiord et al., 2020; Zweifel et al., 2021), and stomata can only respond at VPD > 2 kPa for more drought-tolerant species (Gil-Pelegrin et al., 2017). Norway spruce stomata in European Slovakia closed above a certain VPD threshold (VPD > 1.5 kPa), which greatly limited the growth of trees (Kurjak et al., 2012). Similarly, biomass production of young European beech trees significantly reduced under a specific VPD range (0.05 kPa < VPD < 1.4 kPa), and almost all new shoots died in the driest treatment (VPD = 1.4 kPa) (Lendzion and Leuschner, 2008).
Trees under water stress and those growing in arid areas were more sensitive to VPD (Addington et al., 2004; Aasamaa and Sober, 2011). Above the maximum VPD threshold for tree stem radial growth, stomata did not suddenly close completely, but photosynthesis was inhibited and affected CO2 uptake (Hetherington and Woodward, 2003). Stem radial growth is influenced by water potential thresholds and growth occurs mainly at night (Supplementary Figure 4). There is evidence that low VPD (VPD < 0.4 kPa) conditions at night improved the water status of trees, thus providing favorable conditions for growth. In contrast, high VPD (VPD > 0.4 kPa) during the day reduced the water potential and swelling pressure of the forming layer, which led to inhibition of cell division and growth (Zweifel et al., 2021).
Future implication of elevated vapor pressure deficits on Larix principis-rupprechtii
GRO of L. principis-rupprechtii had similar responses to VPD in different years, which justifies the comparisons of the reactions of trees to VPD thresholds in future monitoring. Our results indicate that VPD will continue to increase in the future (Figure 9), which is consistent with global VPD trend (Liu and Sun, 2017; Yuan et al., 2019; McDowell et al., 2022). Water deficits will become more severe in the context of continuously increasing VPD, as larch trees consume water more rapidly than many other tree species (Zhang et al., 2018). More severe droughts may reach the threshold of lethal plant dehydration and highly restrict stem radial growth in the future (Brodribb et al., 2020). The growth of L. principis-rupprechtii reduced significantly in extreme drought years (Zhang et al., 2021). Although it is difficult to make a causal link between drought injury and tree mortality, the results are consistent with other studies that stem radial growth was severely inhibited by high VPD due to the effects of rising VPD (Rowland et al., 2015; Wolf et al., 2016). VPD above 0.8 kPa did not frequently occur in the study region and may not lead to large-scale tree mortality in the past 2 years. However, stem radial growth may face more severe water stress in the context of regional aridification. Therefore, our findings are expected to be useful to predict stem radial growth based on VPD changes in semi-arid regions of northern China.
Conclusion
The average stem radial growth of L. principis-rupprechtii started on 29 April and stopped on 17 August. The stem of the L. principis-rupprechtii showed optimal growth when temperature was about 18°C and sunshine duration was about 7 h. VPD had threshold effects on stem radial growth of L. principis-rupprechtii during the growing season. Maximum stem radial growth occurred at VPD below 0.5 kPa. Stem radial growth had a linear response to elevated VPD when VPD ranges from 0.5 to 0.8 kPa. Considering VPD values will always be above the 0.8 kPa level from 2051 to 2100, stem radial growth will be more limited by water deficits in the future.
Data availability statement
The raw data supporting the conclusions of this article will be made available by the authors, without undue reservation.
Author contributions
XZ conceive the idea. WL, FY, CW, and JL collected tree cores. WL performed most analysis and wrote the manuscript. XZ provided valuable suggestions and co-wrote the manuscript. All authors contributed to the article and approved the submitted version.
Funding
This work was funded by the Special Project for Local Science and Technology Development Guided by the Central Government of China (226Z6801G), Education Department of Hebei Province (BJ2020025), and Talent Introduction Program in Hebei Agricultural University (YJ201918).
Conflict of interest
The authors declare that the research was conducted in the absence of any commercial or financial relationships that could be construed as a potential conflict of interest.
Publisher’s note
All claims expressed in this article are solely those of the authors and do not necessarily represent those of their affiliated organizations, or those of the publisher, the editors and the reviewers. Any product that may be evaluated in this article, or claim that may be made by its manufacturer, is not guaranteed or endorsed by the publisher.
Supplementary material
The Supplementary Material for this article can be found online at: https://www.frontiersin.org/articles/10.3389/ffgc.2022.948022/full#supplementary-material
Footnotes
References
Aasamaa, K., and Sober, A. (2011). Stomatal sensitivities to changes in leaf water potential, air humidity, CO2 concentration and light intensity, and the effect of abscisic acid on the sensitivities in six temperate deciduous tree species. Environ. Exp. Bot. 71, 72–78. doi: 10.1016/j.envexpbot.2010.10.013
Addington, R. N., Mitchell, R. J., Oren, R., and Donovan, L. A. (2004). Stomatal sensitivity to vapor pressure deficit and its relationship to hydraulic conductance in Pinus palustris. Tree Physiol. 24, 561–569. doi: 10.1093/treephys/24.5.561
Anderegg, W. R. L., Konings, A. G., Trugman, A. T., Yu, K. L., Bowling, D. R., Gabbitas, R., et al. (2018). Hydraulic diversity of forests regulates ecosystem resilience during drought. Nature 561, 538–541. doi: 10.1038/s41586-018-0539-7
Aryal, S., Hausser, M., Griessinger, J., Fan, Z. X., and Brauning, A. (2020). “dendRoAnalyst”: A tool for processing and analysing dendrometer data. Dendrochronologia 64:125772. doi: 10.1016/j.dendro.2020.125772
Battipaglia, G., De Micco, V., Brand, W. A., Saurer, M., Aronne, G., Linke, P., et al. (2014). Drought impact on water use efficiency and intra-annual density fluctuations in Erica arborea on Elba (Italy). Plant Cell Environ. 37, 382–391. doi: 10.1111/pce.12160
Betsch, P., Bonal, D., Breda, N., Montpied, P., Peiffer, M., Tuzet, A., et al. (2011). Drought effects on water relations in beech: The contribution of exchangeable water reservoirs. Agric. For. Meteorol. 151, 531–543. doi: 10.1016/j.agrformet.2010.12.008
Bouriaud, O., Leban, J. M., Bert, D., and Deleuze, C. (2005). Intra-annual variations in climate influence growth and wood density of Norway spruce. Tree Physiol. 25, 651–660. doi: 10.1093/treephys/25.6.651
Brodribb, T. J., and Holbrook, N. M. (2003). Stomatal closure during leaf dehydration, correlation with other leaf physiological traits. Plant Physiol. 132, 2166–2173.
Brodribb, T. J., Powers, J., Cochard, H., and Choat, B. (2020). Hanging by a thread? Forests and drought. Science 368, 261–266. doi: 10.1126/science.aat7631
Buras, A., Sassklaassen, U., and Wilmking, M. (2017). “Growth divergence: A challenging opportunity for dendrochronology,” in Proceedings of the EGU general assembly conference, Vienna.
Cabon, A., Fernandez-de-Una, L., Gea-Izquierdo, G., Meinzer, F. C., Woodruff, D. R., Martinez-Vilalta, J., et al. (2020). Water potential control of turgor-driven tracheid enlargement in Scots pine at its xeric distribution edge. New Phytol. 225, 209–221. doi: 10.1111/nph.16146
Campbell, G. S., and Norman, J. M. (1998). An introduction to environmental biophysics, 2nd Edn. New York, NY: Springer-Verlag.
Cermak, J., Kucera, J., Bauerle, W. L., Phillips, N., and Hinckley, T. M. (2007). Tree water storage and its diurnal dynamics related to sap flow and changes in stem volume in old-growth Douglas-fir trees. Tree Physiol. 27, 181–198. doi: 10.1093/treephys/27.2.181
Cornic, G. (2000). Drought stress inhibits photosynthesis by decreasing stomatal aperture–not by affecting ATP synthesis. Trends Plant Sci. 5, 187–188. doi: 10.1016/s1360-1385(00)01625-3
Dai, A. G. (2013). Increasing drought under global warming in observations and models. Nat. Clim. Change 3, 52–58. doi: 10.1038/nclimate1633
de Carcer, P. S., Vitasse, Y., Penuelas, J., Jassey, V. E. J., Buttler, A., and Signarbieux, C. (2018). Vapor-pressure deficit and extreme climatic variables limit tree growth. Glob. Change Biol. 24, 1108–1122. doi: 10.1111/gcb.13973
De Schepper, V., and Steppe, K. (2010). Development and verification of a water and sugar transport model using measured stem diameter variations. J. Exp. Bot. 61, 2083–2099. doi: 10.1093/jxb/erq018
Deslauriers, A., Anfodillo, T., Rossi, S., and Carraro, V. (2007). Using simple causal modeling to understand how water and temperature affect daily stem radial variation in trees. Tree Physiol. 27, 1125–1136. doi: 10.1093/treephys/27.8.1125
Deslauriers, A., Fonti, P., Rossi, S., Rathgeber, C. B. K., and Gricar, J. (2017). “Ecophysiology and plasticity of wood and phloem formation,” in Dendroecology: Tree-ring analyses applied to ecological studies, eds M. M. Amoroso, L. D. Daniels, P. J. Baker, and J. J. Camarero (Cham: Springer International Publishing Ag), 13–33.
Deslauriers, A., Huang, J. G., Balducci, L., Beaulieu, M., and Rossi, S. (2016). The contribution of carbon and water in modulating wood formation in black spruce saplings. Plant Physiol. 170, 2072–2084. doi: 10.1104/pp.15.01525
Deslauriers, A., Morin, H., Urbinati, C., and Carrer, M. (2003). Daily weather response of balsam fir (Abies balsamea (L.) Mill.) stem radius increment from dendrometer analysis in the boreal forests of Quebec (Canada). Trees Struct. Funct. 17, 477–484. doi: 10.1007/s00468-003-0260-4
Duchesne, L., Houle, D., and D’Orangeville, L. (2012). Influence of climate on seasonal patterns of stem increment of balsam fir in a boreal forest of Quebec, Canada. Agric. For. Meteorol. 162, 108–114. doi: 10.1016/j.agrformet.2012.04.016
Evans, M. E. K., Falk, D. A., Arizpe, A., Swetnam, T. L., Babst, F., and Holsinger, K. E. (2017). Fusing tree-ring and forest inventory data to infer influences on tree growth. Ecosphere 8:e01889. doi: 10.1002/ecs2.1889
Gil-Pelegrin, E., Saz, M. A., Cuadrat, J. M., Peguero-Pina, J. J., and Sancho-Knapik, D. (2017). “Oaks under Mediterranean-type climates: Functional response to summer aridity,” in Oaks physiological ecology. Exploring the functional diversity of genus Quercus L, eds E. GilPelegrin, J. J. PegueroPina, and D. SanchoKnapik (Cham: Springer International Publishing Ag), 137–193.
Grossiord, C., Buckley, T. N., Cernusak, L. A., Novick, K. A., Poulter, B., Siegwolf, R. T. W., et al. (2020). Plant responses to rising vapor pressure deficit. New Phytol. 226, 1550–1566. doi: 10.1111/nph.16485
Hetherington, A. M., and Woodward, F. I. (2003). The role of stomata in sensing and driving environmental change. Nature 424, 901–908. doi: 10.1038/nature01843
Ji, Q. W., Zheng, C. Y., Zhang, L., and Zeng, F. X. (2020). Stem radial growth dynamics of Pinus sylvestris var. mongolica and their relationship with meteorological factor in Saihanba, Hebei, China. Chin. J. Plant Ecol. 44, 257–265.
Jiang, X. Y., Huang, J. G., Cheng, J., Dawson, A., Stadt, K. J., Comeau, P. G., et al. (2018). Interspecific variation in growth responses to tree size, competition and climate of western Canadian boreal mixed forests. Sci. Total Environ. 631–632, 1070–1078. doi: 10.1016/j.scitotenv.2018.03.099
Katz, C., Oren, R., Schulze, E. D., and Milburn, J. A. (1989). Uptake of water and solutes through twigs of Picea abies (L.) Karst. Trees Struct. Funct. 3, 33–37.
Kocher, P., Horna, V., and Leuschner, C. (2012). Environmental control of daily stem growth patterns in five temperate broad-leaved tree species. Tree Physiol. 32, 1021–1032. doi: 10.1093/treephys/tps049
Kurjak, D., Strelcova, K., Ditmarova, L., Priwitzer, T., Kmet, J., Homolak, M., et al. (2012). Physiological response of irrigated and non-irrigated Norway spruce trees as a consequence of drought in field conditions. Eur. J. For. Res. 131, 1737–1746. doi: 10.1007/s10342-012-0611-z
Lawlor, D. W., and Cornic, G. (2002). Photosynthetic carbon assimilation and associated metabolism in relation to water deficits in higher plants. Plant Cell Environ. 25, 275–294. doi: 10.1046/j.0016-8025.2001.00814.x
Lendzion, J., and Leuschner, C. (2008). Growth of European beech (Fagus sylvatica L.) saplings is limited by elevated atmospheric vapour pressure deficits. For. Ecol. Manage. 256, 648–655. doi: 10.1016/j.foreco.2008.05.008
Li, X. X., Liang, E. Y., Gricar, J., Prislan, P., Rossi, S., and Cufar, K. (2013). Age dependence of xylogenesis and its climatic sensitivity in Smith fir on the south-eastern Tibetan Plateau. Tree Physiol. 33, 48–56. doi: 10.1093/treephys/tps113
Liu, W. B., and Sun, F. B. (2017). Projecting and attributing future changes of evaporative demand over China in CMIP5 climate models. J. Hydrometeorol. 18, 977–991. doi: 10.1175/jhm-d-16-0204.1
Liu, X. S., Nie, Y. Q., and Wen, F. (2018). Seasonal dynamics of stem radial increment of Pinus taiwanensis Hayata and its response to environmental factors in the Lushan Mountains, Southeastern China. Forests 9:387. doi: 10.3390/f9070387
Liu, Y. Y., Wang, A. Y., An, Y. N., Lian, P. Y., Wu, D. D., Zhu, J. J., et al. (2018). Hydraulics play an important role in causing low growth rate and dieback of aging Pinus sylvestris var. mongolica trees in plantations of Northeast China. Plant Cell Environ. 41, 1500–1511. doi: 10.1111/pce.13160
McDowell, N., Pockman, W. T., Allen, C. D., Breshears, D. D., Cobb, N., Kolb, T., et al. (2008). Mechanisms of plant survival and mortality during drought: Why do some plants survive while others succumb to drought? New Phytol. 178, 719–739. doi: 10.1111/j.1469-8137.2008.02436.x
McDowell, N. G., Sapes, G., Pivovaroff, A., Adams, H. D., Allen, C. D., Anderegg, W. R. L., et al. (2022). Mechanisms of woody-plant mortality under rising drought, CO2 and vapour pressure deficit. Nat. Rev. Earth Environ. 3, 294–308. doi: 10.1038/s43017-022-00272-1
Mendivelso, H. A., Camarero, J. J., Gutiérrez, E., and Castaño-Naranjo, A. (2016). Climatic influences on leaf phenology, xylogenesis and radial stem changes at hourly to monthly scales in two tropical dry forests. Agric. For. Meteorol. 216, 20–36.
Oberhuber, W., Gruber, A., Kofler, W., and Swidrak, I. (2014). Radial stem growth in response to microclimate and soil moisture in a drought-prone mixed coniferous forest at an inner Alpine site. Eur. J. For. Res. 133, 467–479. doi: 10.1007/s10342-013-0777-z
Oladi, R., Elzami, E., Pourtahmasi, K., and Bräuning, A. (2017). Weather factors controlling growth of oriental beech are on the turn over the growing season. Eur. J. For. Res. 136, 345–356.
Oren, R., Sperry, J. S., Katul, G. G., Pataki, D. E., Ewers, B. E., Phillips, N., et al. (1999). Survey and synthesis of intra- and interspecific variation in stomatal sensitivity to vapour pressure deficit. Plant Cell Environ. 22, 1515–1526. doi: 10.1046/j.1365-3040.1999.00513.x
Payn, T., Carnus, J. M., Freer-Smith, P., Kimberley, M., Kollert, W., Liu, S. R., et al. (2015). Changes in planted forests and future global implications. For. Ecol. Manage. 352, 57–67. doi: 10.1016/j.foreco.2015.06.021
Pinheiro, J. C., Bates, D. M., Debroy, S., Sarkar, D., and R Core Team (2015). Linear and nonlinear mixed effects models. R Package Version 3, 1–83.
R Core Team (2010). R: A Language and Environment for Statistical Computing. Vienna: R Foundation for Statistical, 12–21.
Rossi, S., Anfodillo, T., Cufar, K., Cuny, H. E., Deslauriers, A., Fonti, P., et al. (2013). A meta-analysis of cambium phenology and growth: linear and non-linear patterns in conifers of the northern hemisphere. Ann. Bot. 112, 1911–1920. doi: 10.1093/aob/mct243
Rossi, S., Deslauriers, A., Griçar, J., Seo, J. W., Rathgeber, C. B., Anfodillo, T., et al. (2008). Critical temperatures for xylogenesis in conifers of cold climates. Glob. Ecol. Biogeogr. 17, 696–707.
Rowland, L., da Costa, A. C. L., Galbraith, D. R., Oliveira, R. S., Binks, O. J., Oliveira, A. A. R., et al. (2015). Death from drought in tropical forests is triggered by hydraulics not carbon starvation. Nature 528, 119–122. doi: 10.1038/nature15539
Steppe, K., De Pauw, D. J. W., Lemeur, R., and Vanrolleghem, P. A. (2006). A mathematical model linking tree sap flow dynamics to daily stem diameter fluctuations and radial stem growth. Tree Physiol. 26, 257–273. doi: 10.1093/treephys/26.3.257
Trotsiuk, V., Babst, F., Grossiord, C., Gessler, A., Forrester, D. I., Buchmann, N., et al. (2021). Tree growth in Switzerland is increasingly constrained by rising evaporative demand. J. Ecol. 109, 2981–2990. doi: 10.1111/1365-2745.13712
Turcotte, A., Morin, H., Krause, C., Deslauriers, A., and Thibeault-Martel, M. (2009). The timing of spring rehydration and its relation with the onset of wood formation in black spruce. Agric. For. Meteorol. 149, 1403–1409. doi: 10.1016/j.agrformet.2009.03.010
Urrutia-Jalabert, R., Rossi, S., Deslauriers, A., Malhi, Y., and Lara, A. (2015). Environmental correlates of stem radius change in the endangered Fitzroya cupressoides forests of southern Chile. Agric. For. Meteorol. 200, 209–221.
van der Maaten, E., Pape, J., van der Maaten-Theunissen, M., Scharnweber, T., Smiljanic, M., Cruz-Garcia, R., et al. (2018). Distinct growth phenology but similar daily stem dynamics in three co-occurring broadleaved tree species. Tree Physiol. 38, 1820–1828. doi: 10.1093/treephys/tpy042
Vieira, J., Rossi, S., Campelo, F., Freitas, H., and Nabais, C. (2013). Seasonal and daily cycles of stem radial variation of Pinus pinaster in a drought-prone environment. Agric. For. Meteorol. 180, 173–181. doi: 10.1016/j.agrformet.2013.06.009
Wang, D. Z., Zhang, D. Y., Jiang, F. L., Bai, Y. H., Zhang, Z. D., and Huang, X. R. (2015). A site index model for Larix principis-rupprechtii plantation in Sehanba, North China. Chin. J. Appl. Ecol. 26, 3413–3420. doi: 10.13287/j.1001-9332.20150915.003
Wolf, A., Anderegg, W. R. L., and Pacala, S. W. (2016). Optimal stomatal behavior with competition for water and risk of hydraulic impairment. Proc. Natl. Acad. Sci. U.S.A. 113, E7222–E7230. doi: 10.1073/pnas.1615144113
Xiao, J. Y., Zhang, W. Y., Mou, Y. M., and Lyu, L. X. (2021). Differences of drought tolerance of the main tree species in Dongling Mountain, Beijing, China as indicated by tree rings. Chin. J. Appl. Ecol. 32, 3487–3496. doi: 10.13287/j.1001-9332.202110.001
Xue, F., Jiang, Y., Dong, M. Y., Wang, M. C., Ding, X. Y., Yang, X. J., et al. (2022). Seasonal and daily variations in stem water relations between co-occurring Larix principis-rupprechtii and Picea meyeri at different elevations. For. Ecol. Manage. 504:119821. doi: 10.1016/j.foreco.2021.119821
Yuan, W. P., Zheng, Y., Piao, S. L., Ciais, P., Lombardozzi, D., Wang, Y. P., et al. (2019). Increased atmospheric vapor pressure deficit reduces global vegetation growth. Sci. Adv. 5:eaax1396. doi: 10.1126/sciadv.aax1396
Zhang, Q., Lyu, L., and Wang, Y. (2022). Patterns of daily stem growth in different tree species in a warm-temperate forest in northern China. Dendrochronologia 72:125934.
Zhang, X., Manzanedo, R. D., D’Orangeville, L., Radmacher, T. T., and Pederson, N. (2019). Snowmelt and early to mid-growing season water availability augment tree growth during rapid warming in southern Asian boreal forests. Glob. Change Biol. 25, 3462–3471. doi: 10.1111/gcb.14749
Zhang, X., Manzanedo, R. D., Lv, P., Xu, C., Hou, M., Huang, X., et al. (2022). Reduced diurnal temperature range mitigates drought impacts on larch tree growth in North China. Sci. Total Environ. 848:157808. doi: 10.1016/j.scitotenv.2022.157808
Zhang, X. L., Bai, X. P., Chang, Y. X., and Chen, Z. J. (2016). Increased sensitivity of Dahurian larch radial growth to summer temperature with the rapid warming in Northeast China. Trees Struct. Funct. 30, 1799–1806. doi: 10.1007/s00468-016-1413-6
Zhang, X. L., Li, X., Manzanedo, R. D., D’Orangeville, L., Lv, P. C., Wang, C. K., et al. (2021). High risk of growth cessation of planted larch under extreme drought. Environ. Res. Lett. 16:014040. doi: 10.1088/1748-9326/abd214
Zhang, Y., Peng, C. H., Li, W. Z., Tian, L. X., Zhu, Q. Q., Chen, H., et al. (2016). Multiple afforestation programs accelerate the greenness in the ‘Three North’ region of China from 1982 to 2013. Ecol. Indic. 61, 404–412. doi: 10.1016/j.ecolind.2015.09.041
Zhang, Y. P., Jiang, Y., Wang, B., Jiao, L., and Wang, M. C. (2018). Seasonal water use by Larix principis-rupprechtii in an alpine habitat. For. Ecol. Manage. 409, 47–55. doi: 10.1016/j.foreco.2017.11.009
Zweifel, R., Haeni, M., Buchmann, N., and Eugster, W. (2016). Are trees able to grow in periods of stem shrinkage? New Phytol. 211, 839–849. doi: 10.1111/nph.13995
Zweifel, R., and Hasler, R. (2000). Frost-induced reversible shrinkage of bark of mature subalpine conifers. Agric. For. Meteorol. 102, 213–222. doi: 10.1016/s0168-1923(00)00135-0
Zweifel, R., Item, H., and Hasler, R. (2001). Link between diurnal stem radius changes and tree water relations. Tree Physiol. 21, 869–877. doi: 10.1093/treephys/21.12-13.869
Zweifel, R., Sterck, F., Braun, S., Buchmann, N., Eugster, W., Gessler, A., et al. (2021). Why trees grow at night. New Phytol. 231, 2174–2185. doi: 10.1111/nph.17552
Keywords: stem radial growth, point dendrometer, climate response, vapor pressure deficits (VPD), threshold effects, Larix principis-rupprechtii
Citation: Li W, Yue F, Wang C, Liao J and Zhang X (2022) Climatic influences on intra-annual stem variation of Larix principis-rupprechtii in a semi-arid region. Front. For. Glob. Change 5:948022. doi: 10.3389/ffgc.2022.948022
Received: 19 May 2022; Accepted: 16 August 2022;
Published: 15 September 2022.
Edited by:
Zuoqiang Yuan, Northwestern Polytechnical University, ChinaReviewed by:
Liangjun Zhu, Central South University of Forestry and Technology, ChinaJunzhou Zhang, Lanzhou University, China
Copyright © 2022 Li, Yue, Wang, Liao and Zhang. This is an open-access article distributed under the terms of the Creative Commons Attribution License (CC BY). The use, distribution or reproduction in other forums is permitted, provided the original author(s) and the copyright owner(s) are credited and that the original publication in this journal is cited, in accordance with accepted academic practice. No use, distribution or reproduction is permitted which does not comply with these terms.
*Correspondence: Xianliang Zhang, emh4aWFubGlhbmc4NUBnbWFpbC5jb20=