- 1EARTH University, San José, Costa Rica
- 2Department of Ecology and Evolutionary Biology, University of Connecticut, Storrs, CT, United States
- 3Tropical Forests and People Research Centre, University of the Sunshine Coast, Sippy Downs, QLD, Australia
- 4Organization for Tropical Studies, La Selva Biological Station, Puerto Viejo de Sarapiquí, Costa Rica
Despite the critical role of seed dispersal for tree colonization during forest succession in wet tropical forests, successional trajectories of seed dispersal modes are poorly documented at the community level. Overall successional trends in wet tropical forests indicate that, over time, animal-dispersed and large-seeded species increase in relative abundance in woody vegetation, whereas wind-dispersed and small-seeded species decline. Increased abundance of animal-dispersed trees during succession may be attributed to higher rates of seed deposition by animals and higher survival rates of animal-dispersed species with larger seeds (diaspores) compared to wind-dispersed species. We compiled categorical information on seed size (maximum seed length) and dispersal mode (anemochory, autochory, or zoochory) for 240 canopy tree species in six naturally regenerating forests (11–45 years since the abandonment of pasture) and two old-growth forests in lowland wet forests in northeastern Costa Rica. We evaluated dispersal mode and seed size among tree species classified as second growth and old growth specialists, and generalists based on relative abundance data in these plots. Further, we compared long-term trajectories in the dispersal and seed-size profile for 240 species of canopy tree species for seedlings, saplings, and trees ≥5 cm dbh using annual vegetation survey data from 1997 to 2013. Seed size was significantly associated with dispersal mode, which was driven by anemochorous species with intermediate seeds 6 mm to 15 mm and autochorous seeds with large seeds >15 mm. Dispersal modes of canopy tree species exhibited clear directional trajectories, with decreasing relative abundance of anemochory and increasing relative abundance of zoochory. Zoochorous seedlings showed higher survival rates than anemochorous seedlings. Species with seeds ≤ 6 mm decreased in relative abundance through succession, while species with seeds >15 mm increased within seedling and sapling communities. Seedlings with seeds >15 mm showed higher survival rates than smaller seeds in second-growth forests. The study further demonstrates the importance of seed traits, such as dispersal mode and seed size, for community assembly during forest regrowth, and the importance of frugivorous animals in this process. The abundance of animal-dispersed tree species in different size classes can be a useful indicator of the recovery of biodiversity and species interactions during forest succession in wet tropical regions.
Introduction
Seed dispersal is a critical process for tree species colonization and community assembly during tropical forest succession. Yet, this process remains poorly documented for long-term vegetation dynamics in naturally regenerating tropical forests, which now cover more area than old-growth forests worldwide (Food and Agriculture Organization of the United Nations and UNESCO, 2020). Forests undergoing succession following cessation of agricultural land use provide critical habitat for forest species in human-managed landscapes (Dent and Wright, 2009; Gardner et al., 2009; Poorter et al., 2021). Since all colonizing plants initially arrive at the regrowing area via seed dispersal from another forest patch, dispersal is an important mechanism that potentially drives successional trajectories of tree species composition (Huanca Nuñez et al., 2021). The absolute or relative abundance of species, individuals, or traits measured over time reveals the successional trajectory or pathway of forest tree species composition (Brown and Lugo, 1990; Finegan, 1996; Chazdon, 2014). The scarcity of long-term studies on forest succession measured over consecutive census years leaves unanswered questions about seed dispersal trajectories and their impact on forest composition, as these trajectories may vary across different landscape contexts, disturbance regimes, or land-use histories (Muniz-Castro et al., 2012; Reid et al., 2015).
Chronosequence studies provide most of our knowledge regarding seed dispersal in forests of different ages undergoing secondary succession (Chazdon et al., 2003, 2007; Lebrija-Trejos et al., 2010; Williamson et al., 2012; Mora et al., 2014). During the initial stages of succession, small-seeded, early successional species previously dispersed to the site can germinate from the seed bank (Butler and Chazdon, 1998; Dupuy and Chazdon, 1998) or seeds can be newly deposited by wind or by frugivorous birds and bats that defecate small seeds while perching, roosting, or flying (Thomas et al., 1988; Levey et al., 2002; Muscarella and Fleming, 2007). In some cases, pioneer trees can resprout from rootstocks (Rocha et al., 2016). As forest succession proceeds from initial to more advanced stages, animal-dispersed trees produce fruits, and frugivores deposit imported seeds (large or small) from previous meals while they perch and feed (Martínez-Ramos and Soto-Castro, 1993; Sezen et al., 2005; Holbrook, 2011; Jansen et al., 2012; Huanca Nuñez et al., 2021). As 70–−90% of tropical tree species rely on zoochory (Howe and Smallwood, 1982), it is not surprising that chronosequence studies show that a large percentage of tree species found in later stages of natural forest regeneration are dispersed by animals (Chazdon et al., 2003; Liebsch et al., 2008; Piotto et al., 2009; Jara-Guerrero et al., 2011; Dupuy et al., 2012; Palma et al., 2021). Although we know animals are key to dispersal processes during forest regeneration, much remains to explore about how the initial composition of early second-growth forests may affect the variation in successional trajectories.
Trajectories of increasing relative abundance of zoochorous seedlings could result from an increase in local seed abundance or the differential establishment and survival of zoochorous species, or both factors. As tree composition and forest structure change over time, some species could be favored in recruitment regardless of the mechanism and quantity of seeds dispersed. Seed size is a species-specific trait linked to rates of germination, seedling establishment, growth, and survival (Kitajima, 2002; Moles et al., 2004; Baraloto and Forget, 2007). During the initial stages of forest succession where conditions are relatively open, small-seeded, fast-growing seedlings may be favored, as they can quickly escape mortality from physical factors, pathogens, and herbivores (Moles and Westoby, 2004). In closed-canopy forests with low understory light levels, however, larger seeds with stored energy sources tend to show better survival (Chazdon et al., 2003; Chazdon, 2008; Liebsch et al., 2008; Piotto et al., 2009). Seed size can influence whether an animal ingests a seed, or if it cache, bury, or secondarily disperse the seed (Nathan and Muller-Landau, 2000; Kuprewicz and García-Robledo, 2019). Although these trends are confirmed by many studies, we lack information from actual successional trajectories that reveal how the accumulation of zoochorous species occurs and how seed dispersal patterns relate to trajectories in seed size and seedling survival.
Here, we describe successional trajectories of two plant functional traits, dispersal mode, and seed size, using a multi-year dataset for seedlings, saplings, and trees of canopy species in plots of wet tropical forests of northeastern Costa Rica spanning 11–45 years since the establishment on former cattle pastures. We ask how trajectories of relative abundance of individuals change with increasing forest age and examine whether these trajectories show similar patterns across plots, an assumption of chronosequence studies. Our study explores the factors driving the accumulation of zoochorous individuals within sites based on these questions: (1) Are dispersal mode and seed size associated with successional specialists or generalists? (2) Is dispersal mode associated with differences in seed size? (3) Does seedling survival vary according to dispersal mode or seed size? Our study extends the concept of successional feedback in seed rain (Huanca Nuñez et al., 2021) to seedling recruitment of animal-dispersed trees and emphasizes the importance of differential recruitment of large-seeded animal-dispersed seedlings during tropical forest succession.
Methods
Study area
We conducted our study in Sarapiquí, northeastern Costa Rica, at La Selva Biological Station, and nearby forest areas. Sarapiquí is in the wet tropical forest bioregion of Caribbean lowlands (Holdridge et al., 1975), characterized by ~4,300 mm of annual precipitation, without a strong dry season (Sanford et al., 1994; McClearn et al., 2016). Sarapiquí has been greatly deforested since the 1960s. Forest reserves of various sizes exist, including the 1,615 ha La Selva Research Station connected to La Selva Protected Zone and Braulio Carrillo National Park, which creates an elevational gradient with a largely intact fauna (Ahumada et al., 2013). In 2011, the region had ~50% forest cover, with 25–30% of the total area composed of mature forests, 15% in native species reforestation and second-growth forests on former pastures, and 5% reforestation plantations with exotic species (Fagan et al., 2013).
In 1997, four 1-ha permanent forest monitoring plots were installed at former pasture sites in second-growth forests that ranged in age from 12 to 25 years at the time of the first plot census (Chazdon et al., 2007). In 2005, two additional 1-ha plots were established in the second-growth forest that were 11 years old, and two plots in nearby old-growth forests that have not been logged in recent history (at least 200 years) (de Almeida et al., 2020; Table 1). All trees greater or equal to 5 cm diameter at breast height (dbh) were identified to species, marked, and mapped annually in the plots. In six plots, seedlings and saplings of canopy tree species were monitored annually from 2005 to 2011. Seedlings (>20 cm and <1 cm dbh) were monitored in 0.2 ha of each plot in 5 transects (2 m by 200 m). Saplings (≥1 cm dbh and <5 cm dbh) were censused in 5 transects (5 m by 200 m) for a total of 0.5 ha per plot. Species identifications were corroborated by floristic experts from different institutions (OTS, CATIE, INBIO, etc.) during the long-term study, and voucher species for trees were verified with specimens from La Selva Research Station herbarium and a subset of the voucher specimens were deposited at the National Museum Herbarium, San José, Costa Rica. For this study, we compiled a “project species pool” of 240 canopy tree species recorded in the plots.
Dispersal and seed size data
For each species, we investigated its dispersal mode and seed size. In addition to the 240 canopy species, we also collected dispersal mode and seed size data for 132 understory tree species. We defined a seed in this study as the embryonic material plus the endosperm and seed coat (husk) that protects the embryo during germination. We did not include the size of auxiliary dispersal structures that decay soon after dispersal, for example, wings that arise from fruit (e.g., Hymenolobium) or flower (e.g., Cordia) derived tissues. We defined seed size as the longest length of the seed that approximates the overall size of the embryo and nutritive tissue of the seed. We grouped species into seed-size categories based on the maximum value for dry specimens, defined in the following categories, where y = maximum observed seed length: (a) y ≤ 1 mm; (b) 1 < y ≤ 6 mm; (c) 6 ≤ y ≤ 15 mm; (d) 15 < y ≤ 30 mm; (e) 30 < y ≤ 50 mm; and (f) y > 50 mm. For all of the analyses presented here, the categories were consolidated into three: y ≤ 6 mm; 6 < y ≤ 15 mm; and y > 15 mm. We recognize that seed size can vary among individuals of the same species and thus we used the longest maximum reported value or measured the maximum seed length of available specimens; we assumed that the consolidated seed size categories would more accurately group the species by size given individual variation. The small category size limit was estimated to be a size that could still pass through the gut of many animal dispersers, while the lower limit of the largest size category was estimated to be seeds that would require animal handling or manipulation or other types of dispersal to move (mostly too big to pass through disperser intestinal track).
We gathered seed size information from a variety of sources. Literature sources were searched for size information (Supplementary Table 1). We measured dried seeds available in the herbaria at La Selva Biological Station and the Instituto Nacional de Biodiversidad (INBio) in Santo Domingo, Costa Rica; and from dried samples taken from seed traps placed beneath bat tents in Sarapiquí (Wendt, 2014). We also examined field data books from several efforts to document species at the La Selva Biological Station. When it was not possible to collect information from the aforementioned sources, we examined photographic references with a scale bar published in the Flora Digital of La Selva (http://www.ots.ac.cr/floradigital).
We defined dispersal mode as the primary method by which seeds move from the parent tree, divided into the following categories: anemochory, autochory, zoochory, and other/unknown (van der Pijl, 1969). Zoochory includes epichory (passive transport), endochory (seed ingestion), or synchory (cached, buried, or moved seeds). Autochory includes mechanical dispersal, gravity, dehiscence, or water (post-gravity). Anemochorous seeds have a diaspore formed by adaptive structures and the embryo. To determine seed dispersal categories, we examined literature sources (Supplementary Table 1).
Data analysis
Species incidence for dispersal mode and seed size
We used the seed size and dispersal mode database for the 240 canopy species to determine the percentage of species in each category for the project species pool. We performed a G-test (log-likelihood ratio) to determine if there was a significant relationship between seed size and dispersal mode. To decrease the number of cells with fewer than five individuals, we excluded the unknown species for which either seed size or dispersal mode could not be classified. These species represented only 2.92% and 3.75% of species for dispersal mode and seed size, respectively, and few individuals.
Dispersal mode and seed size for tree specialist groups
We classified tree species into each of the specialist groups determined by Chazdon et al. (2011) by their dispersal mode and seed size. The old-growth specialist, second-growth specialist, and generalist dataset represented only 71 of the 240 canopy species classified in our plots, as most tree species were too rare to classify. Furthermore, for two species, we had only dispersal information and no seed size data, thus 69 species were used for the seed-size analysis. We used a G-test (likelihood ratio) to determine if the tree specialist group (three categories) was associated independently with seed size (three categories) or dispersal mode (three categories).
Temporal trajectories of dispersal mode and seed size
We explored the trajectories of the relative abundance of dispersal mode and seed size over time for our project species pool. We compared the life stages (trees y ≥ 10 cm dbh, trees 5 cm ≤ y <10 cm dbh, saplings, and seedlings) in each category, for each plot in each census year. The available temporal dataset spanned 6–16 years depending on the plot and life stage (Table 1).
Site vs. chronosequence trajectories
We assessed whether the trajectories of relative abundance of zoochory or large seeds (y > 15 mm) within plots were similar across the chronosequence of sites. To address this question, we focused only on second-growth sites and used linear mixed-effects models using the lme4 package in R (Bates et al., 2014). We followed the methodology of Zuur et al. (2009) for model evaluation. Forest age was our independent variable and fixed effect, while the plot was a random variable. To determine the best random structure to use in models, we compared the AIC values among models with no random factor, a random intercept, a random slope, or with both random slope and intercept (correlated or not correlated). The values were computed with the AICcmodavg package in R (Mazerolle, 2014). When AIC values were within 2 units, we used ANOVA (“anova”) to confirm the selection of the simplest model with the lower AIC. To determine R-squared values for marginal (fixed) effects and conditional (total) effects, we used code from http://jonlefcheck.net/2013/03/13/r2-for-linear-mixed-effects-models/ based on Nakagawa and Schielzeth (2013) and Johnson (2014).
Seedling survival disaggregated by dispersal mode and seed size
We examined the rate of survival of anemochorous, autochorous, and zoochorous seedlings over six census years in four second-growth plots with a Cox proportional hazards model (Cox, 1972). The model was developed with the R package “survival,” using the coxph and survfit function (Therneau, 2014). We conducted the same analysis for seedlings in the three seed-size categories.
Results
Species incidence for dispersal mode and seed size
The greatest percentage of species from all sites are zoochorous, representing 83.5% (200 spp) of the species pool. The largest percentage of species, 37.5%, have seeds in the intermediate size category, between 6 < y ≤ 15 mm (Tables 2A,B).
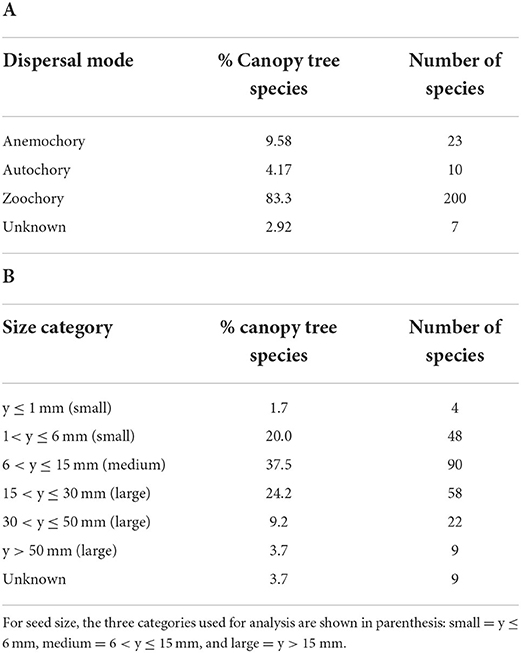
Table 2. For 240 canopy tree species considered in the study, the proportion and number of species in each category are shown for two seed traits: (A) dispersal mode; and (B) seed size.
Seed size class was significantly associated with dispersal mode (X2 = 11.1683, df = 4, p = 0.02474; Figure 1). The significant trend was driven by two patterns. First, 61% of anemochorous species have seeds in the intermediate size class (6–15 mm). Second, 70% of autochorous species have large seeds (y > 15 mm). Only 17% of amenochorus species have seeds >15 mm compared to 40% of zoochorous species. Zoochory is prevalent across all seed-size categories.
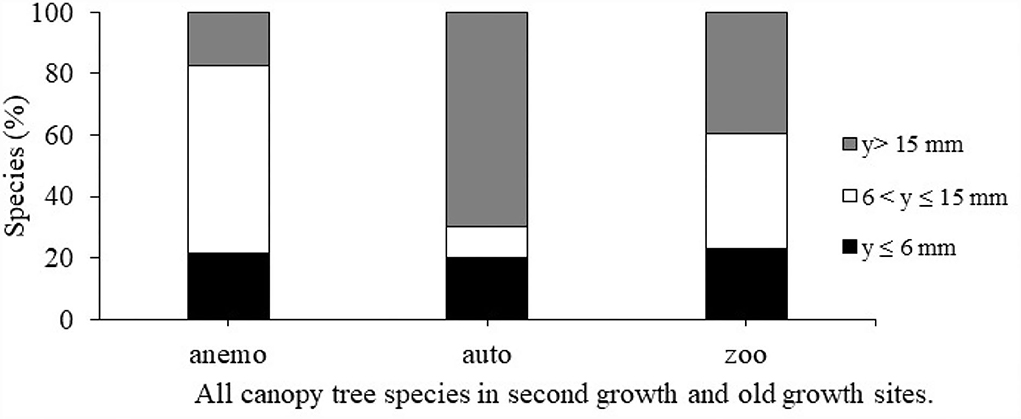
Figure 1. The percent of species in each dispersal mode in three size categories: y ≤ 6 mm; 6 < y ≤ 15 mm; y > 15 mm. Each bar represents 100% of species in that category.
Dispersal mode and seed size for tree specialist groups
Dispersal mode and canopy tree specialist group were significantly associated (X2 = 9.68, df = 4, p = 0.046; Table 3). Generalists were the only group for which autochory was present as a dispersal mode, and second-growth specialists had the greatest number of anemochorous species. The relationship between seed size and specialist group was marginally significant (X2 = 9.4216, df = 4, p = 0.05138). Old-growth specialists have larger seeds than second-growth specialists, but generalists also include many large-seeded species. Two very large-seeded species (y > 50 mm), Carapa nicaraguensis and Maranthes panamensis, are classified as generalists. Second-growth specialists and generalists have a similar number of small-seeded species, but second-growth specialists have a greater proportion of small-seeded species than generalists.
Temporal trajectories of dispersal mode and seed size
Dispersal modes of canopy tree species exhibited directional shifts over time (Figures 2–4). For all life stages, anemochory decreased and zoochory increased as age increased for the chronosequence. Anemochory declined to <10% of stems across all life stages over the study period, while zoochory increased to over 75% of stems. The response of autochory varied for the life stages (seedlings, saplings, and trees). Seedlings have a lower relative abundance for anemochory and autochory compared to trees (Figures 3, 4), while the relative abundance of zoochorous seedlings is greater than the relative abundance of zoochorous adults. For saplings, the change in slope was greater than for other life stages for autochorous (reduced) (Figure 4) and zoochorous (increased) individuals (Figure 2). Trees 5 ≤ y <10 cm dbh display contrasting patterns for autochory, as relative abundance decreases for younger plots and increases or is stable for older plots (Figure 4).
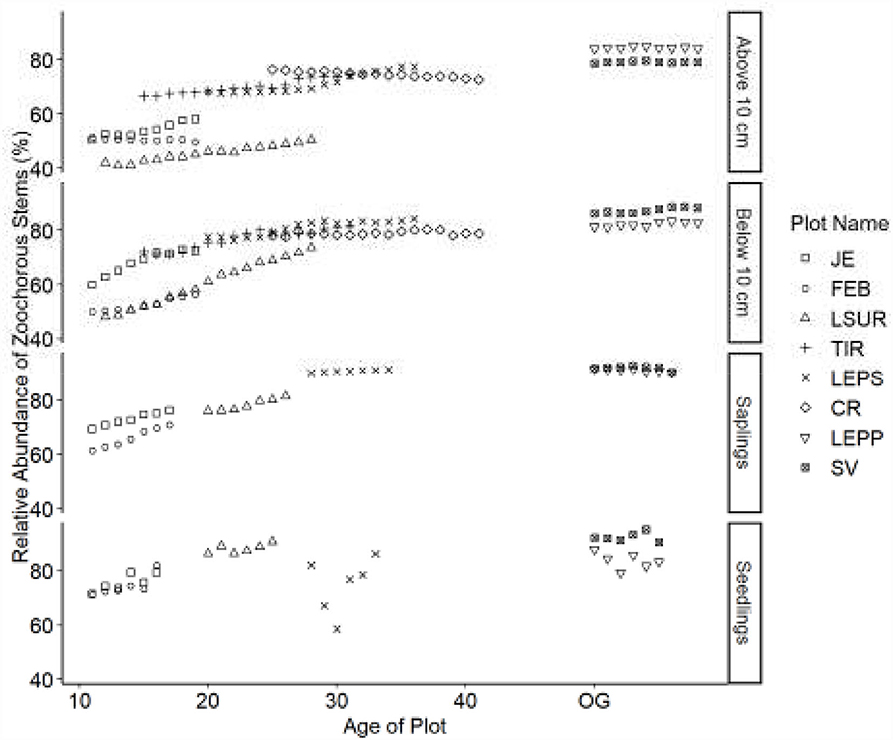
Figure 2. Relative abundance of zoochorous stems. Table 1 indicates the plot acronyms, which appear from youngest to oldest, top to bottom in the legend.
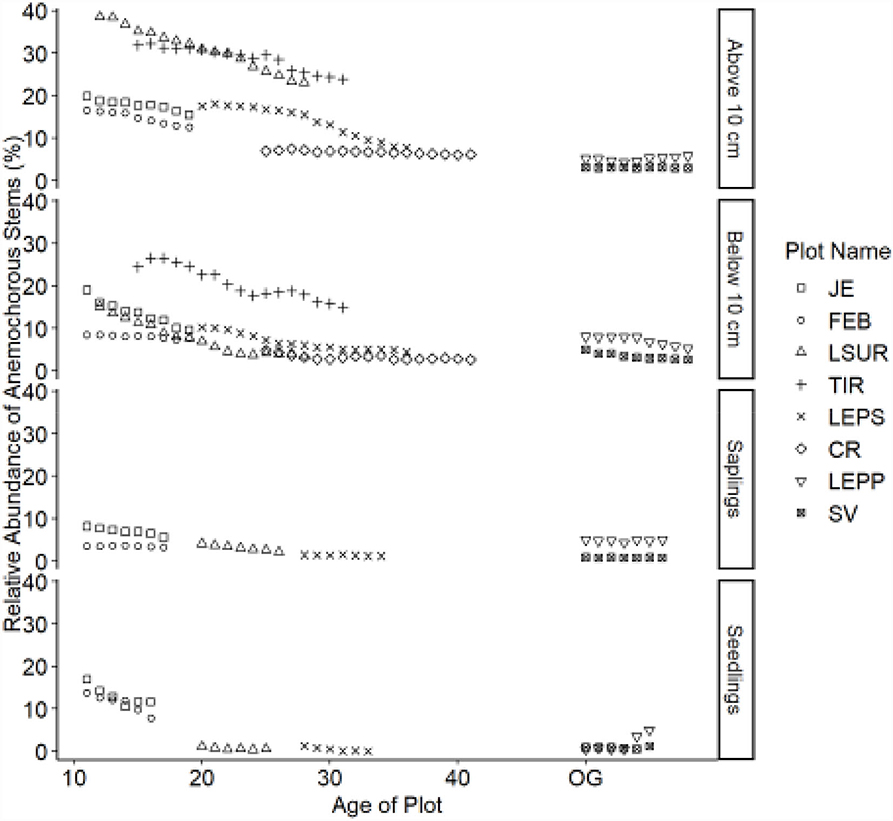
Figure 3. Relative abundance of anemochorous stems. Table 1 indicates the plot acronyms, which appear from youngest to oldest, top to bottom in the legend.
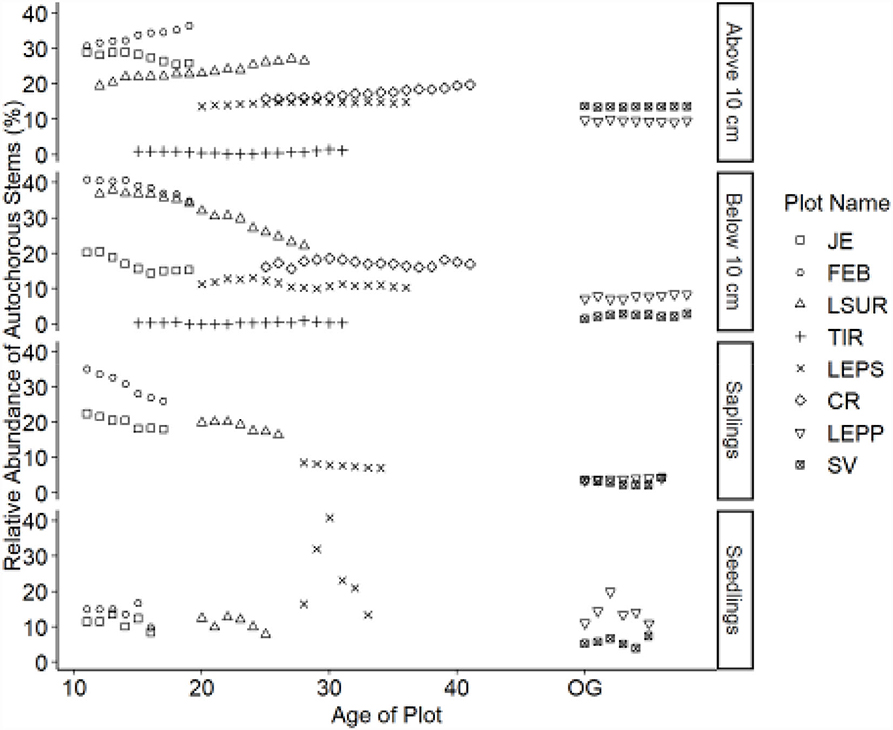
Figure 4. Relative abundance of autochorous stems. Table 1 indicates the plot acronyms, which appear from youngest to oldest, top to bottom in the legend.
Trajectories in seed size also demonstrated directional changes for canopy tree species. Overall, the relative abundance of species with small seeds decreased over time, whereas species with large seeds increased. Species with intermediate-sized seeds showed fewer directional changes. Seed size trajectories did not exhibit the same directional changes for all life stages. Seedlings showed a decline in the relative abundance of small-seeded species (Figure 5) and an increase in large-seeded species (Figure 6). Saplings showed similar trajectories compared to seedlings but also showed a reduced relative abundance of species with intermediate seed size (Figure 7). We noted no consistent directional changes for the seed-size trajectories for small trees, 5 ≤ y <10 cm dbh. The slopes of the trajectories for trees ≥10 cm dbh were less than the slopes for seedlings for seed size, similar to the trajectories for dispersal mode between the two life stages.
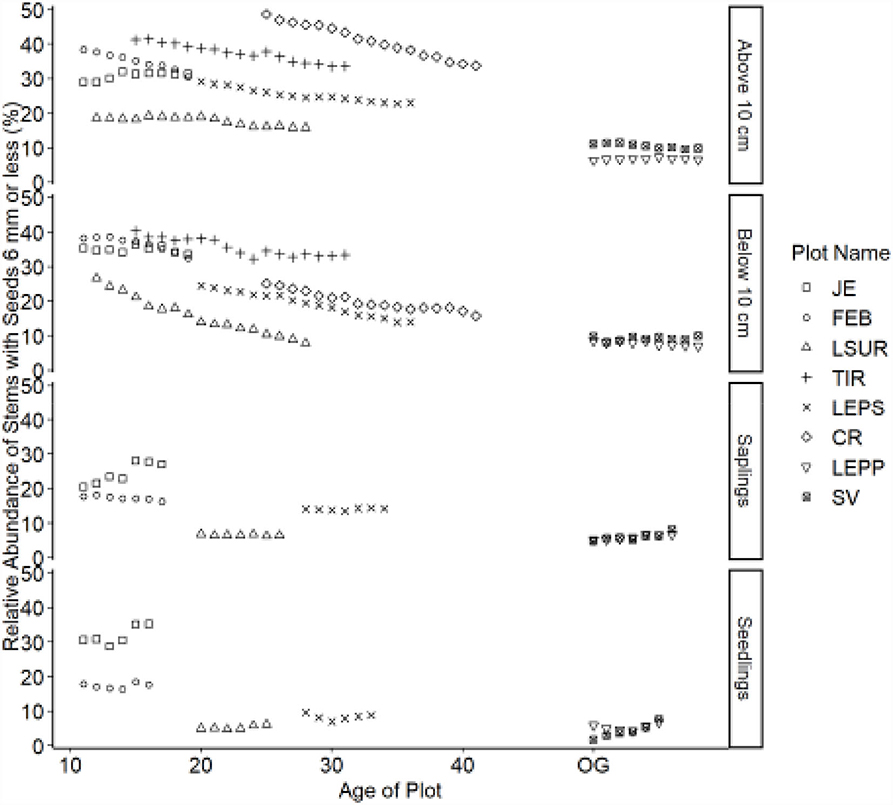
Figure 5. Relative abundance of stems with seeds y ≤ 6 mm (small) category. Table 1 indicates the plot acronyms, which appear from youngest to oldest, top to bottom in the legend.
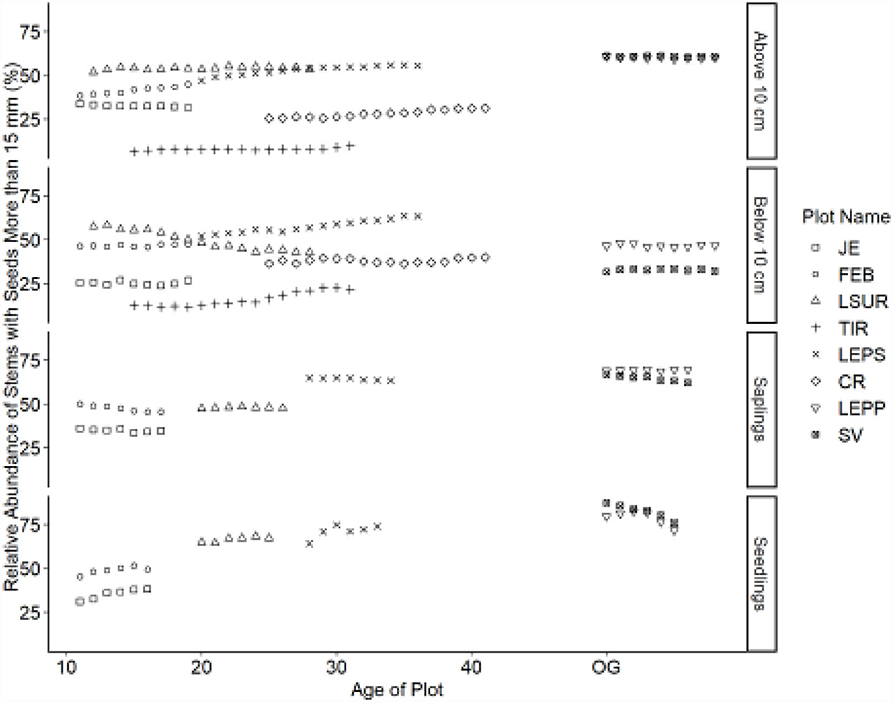
Figure 6. Relative abundance of stems with seeds y > 15 mm (large) category. Table 1 indicates the plot acronyms, which appear from youngest to oldest, top to bottom in the legend.
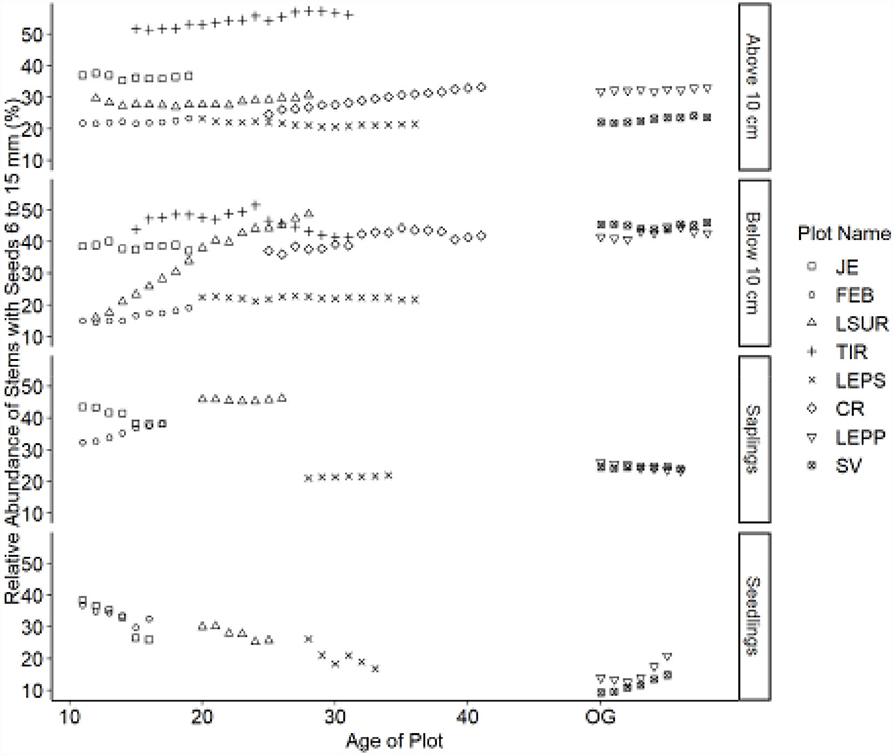
Figure 7. Relative abundance of stems with seeds 6 < y ≤ 15 mm (medium) category. Table 1 indicates the plot acronyms, which appear from youngest to oldest, top to bottom in the legend.
Site vs. chronosequence trajectories
Although zoochory increases over time, plots varied in their trajectories in most life stages. The results of linear mixed-effects models suggest important life stage differences for the fixed effect of age during succession vs. random plot effects for zoochorous individuals and large-seeded individuals (y > 15 mm) at second-growth sites (Table 4). For zoochory, seedlings and trees 5 ≤ y <10 cm dbh had similar fixed effects of age ( = 0.321 and 0.355, respectively) across study plots. Saplings had the strongest fixed effects of age ( = 0.522), whereas trees above 10 cm dbh had the lowest fixed effects.
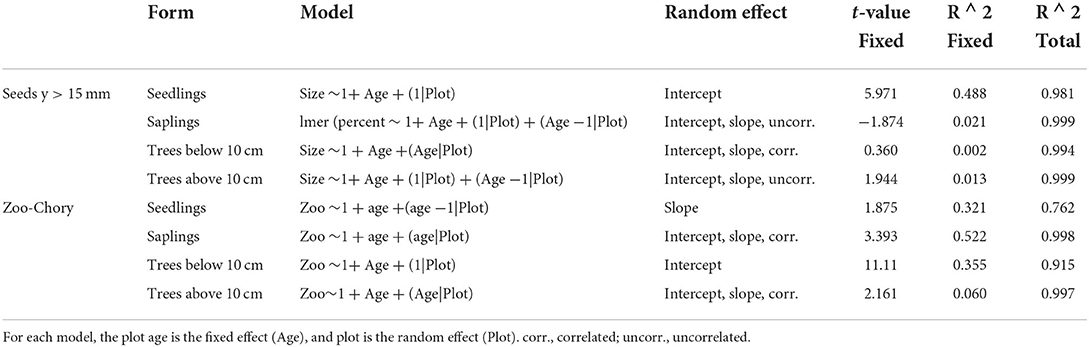
Table 4. Linear mixed models for large seeds (Size) and zoochory (Zoo) for seedlings, saplings, trees 5 ≤ y <10 cm dbh, and trees y ≥ 10 cm dbh in second-growth plots.
The relative abundance of seedlings from species with large seeds showed strong and consistent temporal effects (age), with minimal plot (random) effects ( = 0.488); large-seeded seedlings increase in relative abundance at approximately the same rate as age increases. Although large seeds increase in relative abundance during succession for saplings and trees, these trajectories show strong plot-specific (random) effects.
Seedling survival disaggregated by dispersal mode and seed size
Across all second growth plots, zoochorous seedlings show higher rates of survival compared to anemochorous seedlings (Figure 8, p <0.001). Similarly, seedlings of large-seeded species showed higher survival rates than small-seeded species (Figure 9, p <0.001).
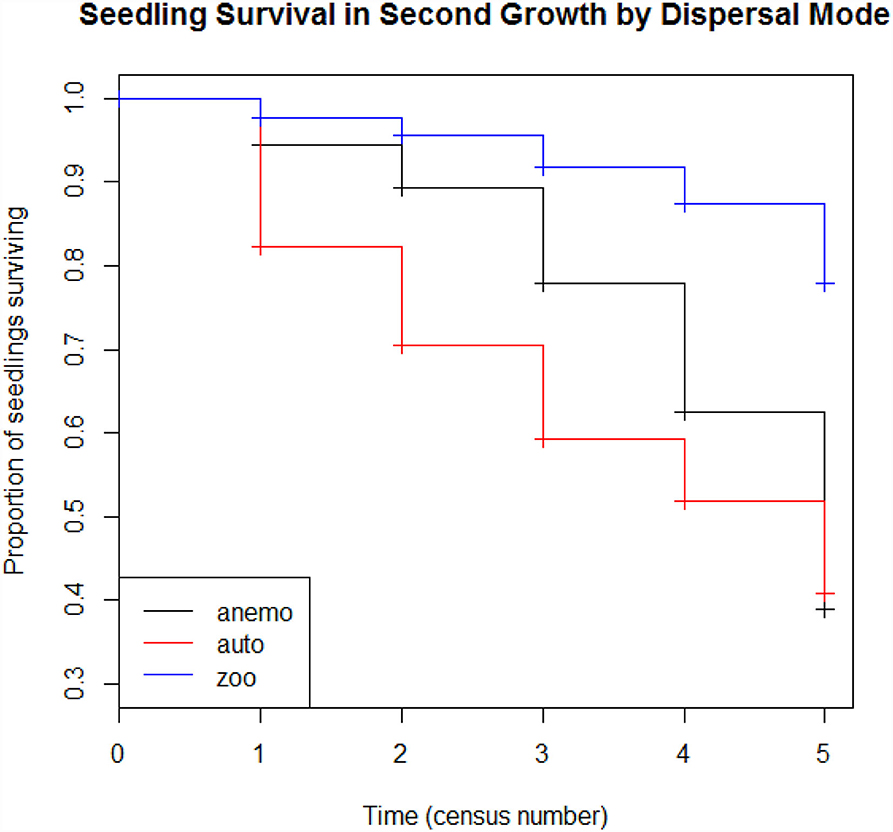
Figure 8. Seedling survival model for anemochorous, autochorous, and zoochorous seedlings in four second-growth plots during six census periods.
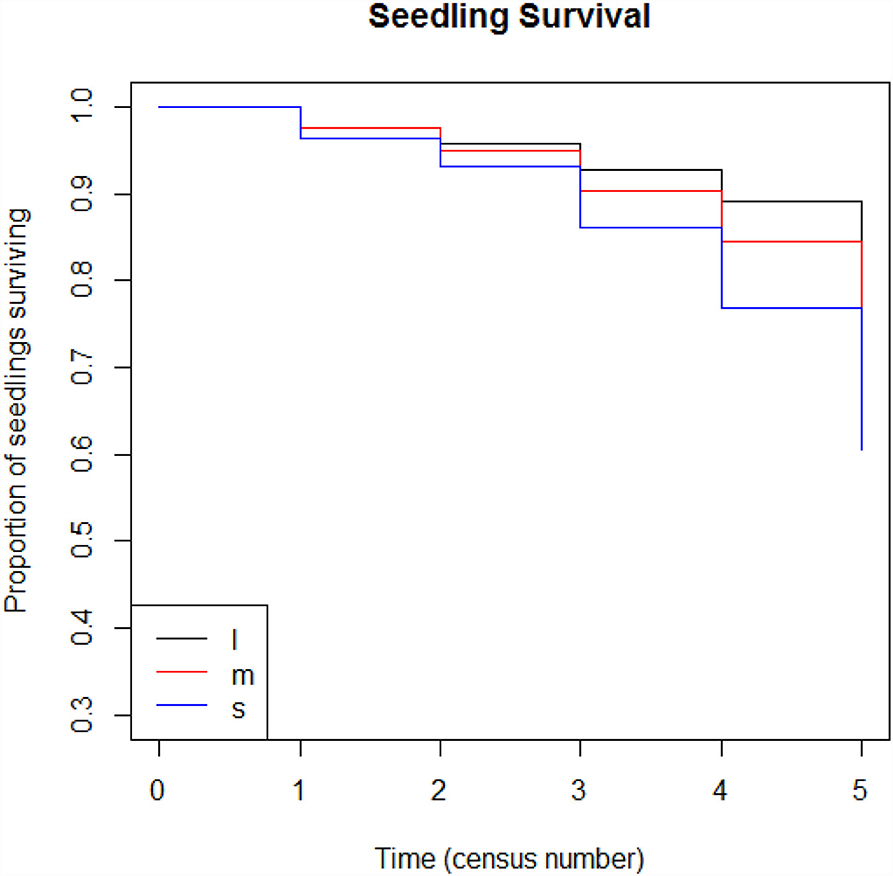
Figure 9. Seedling survival model for seedlings in four second-growth plots during six census periods by seed size: l = y > 15 mm, m = 6 < y ≤ 15, s = y ≤ 6 mm.
Discussion
Our study provides strong evidence of directional changes in successional trajectories of dispersal mode and seed size for canopy tree species over multiple census years. The relative abundance of zoochorous tree species increased over time, whereas anemochorous species declined in relative abundance, supporting a long-standing hypothesis of the critical importance of seed dispersal by animals during forest regeneration. Zoochorous seedlings increase in relative abundance faster than saplings and trees, driven by higher rates of survival and recruitment compared to anemochorous seedlings. These directional changes reflect underlying species-specific attributes and changing species relative abundances across successional gradients (Chazdon et al., 2011; Letcher et al., 2015; Boukili and Chazdon, 2017). They also reflect successional changes in the seed rain in these sites (Huanca Nuñez et al., 2021). Second-growth specialist tree species tend to have wind-dispersed seeds and smaller seeds in comparison to old-growth specialists.
In Sarapiquí, where large areas of conserved forest remain in the landscape, the relative abundance of zoochorous individuals during succession increases rapidly. The relative abundance of zoochory increased in second-growth plots exceeded 75% during 17 years of regrowth for seedlings, 20 years for saplings and trees 5 ≤ y <10 cm dbh, and at around 35 years for trees y ≥ 10 cm dbh. Liebsch et al. (2008) estimated through modeling that it would take 65 years of regrowth to reach this level of relative abundance of zoochory at plots in Brazil. About 83% of our project species pool of canopy trees relies on animals for dispersal, which is similar to findings in the Atlantic forests of Brazil (Piotto et al., 2009) and drier forests of Mexico (Dupuy et al., 2012). Therefore, we cannot attribute the rapid increase of zoochory at our plots to a greater proportion of zoochorous species in the region. Rather, we consider the landscape context of the Sarapiquí region to be beneficial to colonization and dispersal of species to the regenerating sites, due to the large extent of remnant forests in the Sarapiquí region and the largely intact fauna, which favor the rapid recovery of zoochorous species (Norden et al., 2009). In concert with the increased relative abundance of zoochory, large seeds (y> 15 mm) increased in relative abundance during succession, particularly in the seedling community. The majority of these individuals were dispersed by animals to the plot, where environmental conditions favored their establishment and recruitment. This pattern differs from documented recruitment patterns in other landscapes, where forest loss and treeless areas can cause recruitment failure (Arasa-Gisbert et al., 2021).
In our project species pool, size and dispersal mode were significantly associated. Most of the anemochorous canopy tree species have seeds between 6 and 15 mm, contrary to our hypothesis that anemochorous species would have small seeds. In fact, for each dispersal mode, 20% of canopy tree species were small-seeded (Figure 1). We propose that for anemochorous canopy trees to be successful, a larger seed would pose an advantage to the establishment in low-light conditions under a canopy. In Panama, the anemochorous canopy tree species Platypodium elegans also has a large seed (~20 mm) that germinates quickly, perhaps as an advantage against a fungal pathogen that attacks under shaded conditions (Augspurger, 1983).
Zoochorous canopy tree species vary widely in seed size, including early-successional pioneer species with small seeds and late-successional species with large seeds. In fact, in our canopy tree species pool, all tree seeds ≤ 1 mm were associated with zoochorous species (Supplementary Table 1). The increase in the relative abundance of zoochorous trees that establish during the first 10 to 15 years of succession creates a feedback mechanism that promotes seed dispersal and increases species richness by attracting frugivores that visit and deposit other species of seeds while feeding or perching. The animal-dispersed seeds that establish as seedlings have a higher likelihood of survival, which eventually enriches the contribution of animal-dispersed species in the sapling and tree size classes. This pattern is enhanced when considering seed size, as large-seeded seedlings also have higher survival, thus large-seed zoochorous species at the seedling life stage recruit into larger life stages. A similar pattern was observed over 20 years in the seed rain sampled in these plots, where the proportion of large-seeded species, animal-dispersed species, and shade-tolerant species increased dramatically (Huanca Nuñez et al., 2021). In addition, the taxonomic composition of seed rain became more similar to the composition of reproductive trees within the same forest plot (Huanca Nuñez et al., 2021).
Increased relative abundance of zoochorous species can occur through several mechanisms, including a decrease in abundance of individuals with other dispersal types, increased recruitment of zoochorous individuals of species already present at the plot, or the recruitment of zoochorous individuals of species newly colonizing the plot (Huanca Nuñez et al., 2021). Another mechanism is the persistent survival of already recruited individuals during advanced regeneration, as census years are not independent when considering all living individuals. Some of the increase in the relative abundance of zoochory can be attributed to increased forest development as basal area increases and canopy closure affects light availability in the understory. As age and basal area increase, the survival advantage of zoochorous seedlings leads to an “accumulation” of abundance. Relative abundance would therefore increase if the increase of the absolute abundance of zoochorous species is coupled with a decrease in the absolute abundance of individuals of other dispersal modes. Seedling survival and recruitment via seed rain therefore strongly influence patterns of abundance in the seedling community.
The relative abundance of individuals in life stages (plant size classes) presents different trends, reflecting previous or current dispersal community, and successional changes in light and other environmental factors. For instance, seedlings often showed annual spikes in the recruitment of wind or autochorous-dispersed species, whereas saplings were much more stable and consistently zoochorous. Non-reproductive functional traits, such as wood density and specific leaf area, can shape community assembly processes of later life stages (Lasky et al., 2014). More research will be needed to determine the exact traits associated with environmental filtering at the seedling stage that promotes differential survival of animal-dispersed seedlings. Landscape conditions such as the distance from forest patches and total forest cover can also affect plant recruitment that structures the dispersal and seed-size profiles of regenerating forest (Lebrija-Trejos et al., 2010; Arroyo-Rodriguez et al., 2017). The TIR plot, for example, showed a much lower relative abundance of large-seeded trees than the other sites, which could reflect greater isolation from mature forest patches. It is also important to note that site-level characteristics such as remnant tree species and distance to established farms may also affect the composition of colonizing tree species, including cultivated/domesticated or invasive species. Our species pool contained trees such as Euterpe oleracea, Citris sinensis, Mangifera indica, and Syzygium jambos, whose presence at specific sites was related to previous land use history or specific recruitment events from surrounding farms. Fruiting domesticated species can affect disperser behavior and subsequent seedling recruitment. We did not record domesticated species in high relative abundances at our plots, however, more research needs to be conducted on the impact of exotic species, such as Euterpe oleracea, in the Sarapiquí region.
Given current threats to animal populations in many tropical forests (Dirzo et al., 2014), many species of seed dispersers are locally reduced, extirpated, or even extinct. Our data suggest that dispersal drives regrowth when early-recruited animal-dispersed trees become reproductive, with much of the enrichment in species with seeds 15 mm or longer. Animals, either through endochory or ingestion followed by regurgitation, move these species. Cramer et al. (2007) found in the Brazilian Amazon that large seeds were not being dispersed in forest fragments. Large-seeded tree species are also under-represented in forest restoration plantings and nurseries in the Brazil's Atlantic Forest region (Brancalion et al., 2018). Small forest fragments in the Atlantic rainforest of Brazil had impoverished seedling communities (Santo–Silva et al., 2013) resulting from isolation from source populations of animals and plants, scarce or absent fruit or shelter resources in forests to attract animals, and defaunation of key animal dispersers.
Conclusion
The abundance and diversity of animals in the surrounding landscape play a crucial role in tropical forest succession. Flora and fauna conservation efforts in the Sarapiquí region contribute to the regeneration potential of abandoned agricultural sites close to intact forests; however, forests that regenerate without key dispersers will undoubtedly have different compositions and structures. Tropical forests of the future will be significantly altered in landscapes that are missing frugivorous animals that move seeds and promote recovery of species richness and forest ecosystem services.
Data availability statement
The data for trees presented in this article are available at https://datadryad.org/stash/dataset/doi:10.5061%2Fdryad.ncjsxksvr. The datasets for seedlings and saplings presented in this article are not readily available because there are additional data that were not used in the present study and these will be made available in Dryad in the future. Requests for data for seedlings and saplings should be directed to RC, cmNoYXpkb25AZ21haWwuY29t.
Author contributions
AW and RC conceptualized the manuscript and wrote the manuscript. AW and OV collected the data. OV provided critical technical guidance. AW analyzed the data. All authors contributed to the article and approved the submitted version.
Funding
AW was supported by the Organization for Tropical Studies, Bat Conservation International, Fulbright Program, Garden Club of America, University of Connecticut Center for Environmental Sciences and Engineering, and the University of Connecticut Bamford Fund. RC was supported by the Andrew W. Mellon Foundation, NSF DEB-0424767, NSF DEB-0639393, NSF DEB-1147429, NASA Terrestrial Ecology Program, and the University of Connecticut Research Foundation.
Acknowledgments
We thank D. Solano, D. Santamaria, and N. Zamora formerly from the Instituto Nacional de la Biodiversidad (INBIO), Santo Domingo, Costa Rica, for their contribution and access to the INBIO herbarium. Field work was assisted by J. Paniagua, M. Molina, B. Paniagua, and E. Salicetti. Alex Defrancesco contributed to data collection from literature sources. Comments from M. Willig and K. Holsinger significantly improved the manuscript.
Conflict of interest
The authors declare that the research was conducted in the absence of any commercial or financial relationships that could be construed as a potential conflict of interest.
Publisher's note
All claims expressed in this article are solely those of the authors and do not necessarily represent those of their affiliated organizations, or those of the publisher, the editors and the reviewers. Any product that may be evaluated in this article, or claim that may be made by its manufacturer, is not guaranteed or endorsed by the publisher.
Supplementary material
The Supplementary Material for this article can be found online at: https://www.frontiersin.org/articles/10.3389/ffgc.2022.946541/full#supplementary-material
References
Ahumada, J. A., Hurtado, J., and Lizcano, D. (2013). Monitoring the status and trends of tropical forest terrestrial vertebrate communities from camera trap data: A tool for conservation. PLoS ONE 8, e73707. doi: 10.1371/journal.pone.0073707
Arasa-Gisbert, R., Arroyo-Rodríguez, V., Galán-Acedo, C., Meave, J. A., and Martínez-Ramos, M. (2021). Tree recruitment failure in old-growth forest patches across human-modified rainforests. J. Ecol. 109, 2354–2366. doi: 10.1111/1365-2745.13643
Arroyo-Rodriguez, V., Melo, F., Martinez-Ramos, M., Bongers, F., Chazdon, R., Meave, J., et al. (2017). Multiple successional pathways in human-modified tropical landscapes: new insights from forest succession, forest fragmentation and landscape ecology research. Biol. Rev. 92, 326–340. doi: 10.1111/brv.12231
Augspurger, C. A. (1983). Seed dispersal of the tropical tree, Platypodium elegans, and the escape of its seedlings from fungal pathogens. J. Ecol. 71, 759–771. doi: 10.2307/2259591
Baraloto, C., and Forget, P. M. (2007). Seed size, seedling morphology, and response to deep shade and damage in Neotropical rain forest trees. Am. J. Bot. 94, 901–911. doi: 10.3732/ajb.94.6.901
Bates, D., Maechler, M., Bolker, B., and Walker, S. (2014). lme4: Linear Mixed-Effects Models Using Eigen and S4. R Package Version 1.1-7. Available online at: http://CRAN.R-project.org/package=lme4
Boukili, V. K., and Chazdon, R. L. (2017). Environmental filtering, local site factors and landscape context drive changes in functional trait composition during tropical forest succession. Perspect. Plant Ecol. Evol. Syst. 24, 37–47. doi: 10.1016/j.ppees.2016.11.003
Brancalion, P. H. S., Bello, C., Chazdon, R. L., Galetti, M., Jordano, P., Lima, R. A. F., et al. (2018). Maximizing biodiversity conservation and carbon stocking in restored tropical forests. Conserv. Lett. 11, e12454. doi: 10.1111/conl.12454
Brown, S., and Lugo, A. E. (1990). Tropical secondary forests. J. Trop. Ecol. 6, 1–32. doi: 10.1017/S0266467400003989
Butler, B. J., and Chazdon, R. L. (1998). Species richness, spatial variation, and abundance of the soil seed bank of a secondary tropical rain forest. Biotropica 30, 214–222. doi: 10.1111/j.1744-7429.1998.tb00056.x
Chazdon, R. L. (2008). “Chance and determinism in tropical forest succession,” in Tropical Forest Community Ecology, eds W. P. Carson, and S. A. Snitzer (Oxford: Blackwell), 384–408.
Chazdon, R. L. (2014). Second Growth: The Promise of Tropical Forest Regeneration in an Age of Deforestation. Chicago: University of Chicago Press.
Chazdon, R. L., Careaga, S., Webb, C., and Vargas, O. (2003). Community and phylogenetic structure of reproductive traits of woody species in wet tropical forests. Ecol. Monogr. 73, 331–348. doi: 10.1890/02-4037
Chazdon, R. L., Chao, A., Colwell, R. K., Lin, S.–Y., Norden, N., Letcher, S. G., et al. (2011). A novel statistical method for classifying habitat generalists and specialists. Ecology 92, 1332–1343. doi: 10.1890/10-1345.1
Chazdon, R. L., Letcher, S. G., van Breugel, M., Martinez–Ramos, M., Bongers, F., and Finegan, B. (2007). Rates of change in tree communities of secondary neotropical forests following major disturbances. Philos. Trans. R. Soc. B Biol. Sci. 362, 273–289. doi: 10.1098/rstb.2006.1990
Cox, D. R. (1972). Regression models and life tables (with discussion). J. R. Stat. Soc. Ser. B 34, 187–220. doi: 10.1111/j.2517-6161.1972.tb00899.x
Cramer, J. M., Mesquita, R. C., and Williamson, B. G. (2007). Forest fragmentation differentially affects seed dispersal of large and small–seeded tropical trees. Biol. Conserv. 137, 415–423. doi: 10.1016/j.biocon.2007.02.019
de Almeida, D. R. A., Almeyda Zambrano, A. M., Broadbent, E. N., Wendt, A. L., Foster, P., Wilkinson, B. E., et al. (2020). Detecting successional changes in tropical forest structure using GatorEye drone-borne lidar. Biotropica 52, 1155–1167. doi: 10.1111/btp.12814
Dent, D. H., and Wright, S. J. (2009). The future of tropical species in secondary forests: a quantitative review. Biol. Conserv. 142, 2833–2843. doi: 10.1016/j.biocon.2009.05.035
Dirzo, R., Young, H. S., Galetti, M., Ceballos, G., Isaac, N. J., and Collen, B. (2014). Defaunation in the anthropocene. Science 345, 401–406. doi: 10.1126/science.1251817
Dupuy, J. M., and Chazdon, R. L. (1998). Long-term effects of forest regrowth and selective logging on the seed bank of tropical forests in NE Costa Rica. Biotropica 30, 223–237. doi: 10.1111/j.1744-7429.1998.tb00057.x
Dupuy, J. M., Hernández-Stefanoni, J. L., Hernández-Juárez, R. A., Tetetla-Rangel, E., López-Martínez, J. O., Leyequién-Abarca, E., et al. (2012). Patterns and correlates of tropical dry forest structure and composition in a highly replicated chronosequence in Yucatan, Mexico. Biotropica 44, 151–162. doi: 10.1111/j.1744-7429.2011.00783.x
Fagan, M. E., DeFries, R. S., Sesnie, S. E., Arroyo, J. P., Walker, W., Soto, C., et al. (2013). Land cover dynamics following a deforestation ban in northern Costa Rica. Environ. Res. Lett. 8, 034017. doi: 10.1088/1748-9326/8/3/034017
Finegan, B. (1996). Pattern and process in Neotropical secondary forests: the first 100 years of succession. Trends Ecol. Evol. 11, 119–124. doi: 10.1016/0169-5347(96)81090-1
Food and Agriculture Organization of the United Nations and UNESCO (2020). Global Forest Resources Assessment 2020. Rome: FAO.
Gardner, T., Barlow, J., Chazdon, R. L., Ewers, R., Harvey, C. A., and Sodhi, N. S. (2009). Prospects for tropical forest biodiversity in a human–modified world. Ecol. Lett. 12, 561–582. doi: 10.1111/j.1461-0248.2009.01294.x
Holbrook, K. M. (2011). Home range and movement patterns of toucans: implications for seed dispersal. Biotropica 43, 357–364. doi: 10.1111/j.1744-7429.2010.00710.x
Holdridge, L. R., Grenke, W. G., Hatheway, W. H., Liang, T., and Tosi, J. A. (1975). Forest Environments in Tropical Life Zones. New York, NY: Pergamon Press.
Howe, H. F., and Smallwood, J. (1982). Ecology of seed dispersal. Ann. Rev. Ecol. Syst. 13, 201–228. doi: 10.1146/annurev.es.13.110182.001221
Huanca Nuñez, N., Chazdon, R. L., and Russo, S. E. (2021). Seed rain–successional feedbacks in wet tropical forests. Ecology 102, e03362. doi: 10.1002/ecy.3362
Jansen, P. A., Hirsch, B. T., Emsens, W.-J., Zamora-Gutierrez, V., Wikelski, M., and Kays, R. (2012). Thieving rodents as substitute dispersers of megafaunal seeds. Proc. Natl. Acad. Sci. U.S.A. 109, 12610–12615. doi: 10.1073/pnas.1205184109
Jara-Guerrero, A., De la Cruz, M., and Méndez, M. (2011). Seed dispersal spectrum of woody species in south Ecuadorian dry forests: environmental correlates and the effect of considering species abundance. Biotropica 43, 722–730. doi: 10.1111/j.1744-7429.2011.00754.x
Johnson, P. C. D. (2014). Extension of Nakagawa & Schielzeth's R2GLMM to random slopes models. Methods Ecol. Evol. 5, 944–946. doi: 10.1111/2041-210X.12225
Kitajima, K. (2002). Do shade-tolerant tropical tree seedlings depend longer on seed reserves? Functional growth analysis of three Bignoniaceae species. Funct. Ecol. 16, 433–444. doi: 10.1046/j.1365-2435.2002.00641.x
Kuprewicz, E. K., and García-Robledo, C. (2019). Deciphering seed dispersal decisions: Size, not tannin content, drives seed fate and survival in a tropical forest. Ecosphere 10, e02551. doi: 10.1002/ecs2.2551
Lasky, J. R., Uriarte, M., Boukili, V. K., and Chazdon, R. L. (2014). Trait-mediated assembly processes predict successional changes in community diversity of tropical forests. Proc. Natl. Acad. Sci. U.S.A. 111, 5616–5621. doi: 10.1073/pnas.1319342111
Lebrija-Trejos, E., Pérez-García, E. A., Meave, J. A., Bongers, F., and Poorter, L. (2010). Functional traits and environmental filtering drive community assembly in a species-rich tropical system. Ecology 91, 386–398. doi: 10.1890/08-1449.1
Letcher, S. G., Lasky, J. R., Norden, N., Chazdon, R. L., Andrade, A., Andrade-Torres, J. L., et al. (2015). Environmental gradients and the evolution of successional habitat specialization: a test case with 14 Neotropical forest sites. J. Ecol. 103, 1276–1290. doi: 10.1111/1365-2745.12435
Levey, D. J., Silva, W. R., and Galetti, M. (Eds.). (2002). Seed Dispersal and Frugivory: Ecology, Evolution, and Conservation. CABI.
Liebsch, D., Marques, M., and Goldenberg, R. (2008). How long does the Atlantic Rain Forest take to recover after a disturbance? Changes in species composition and ecological features during secondary succession. Biol. Conserv. 141, 1717–1725. doi: 10.1016/j.biocon.2008.04.013
Martínez-Ramos, M., and Soto-Castro, A. (1993). “Seed rain and advanced regeneration in a tropical rain forest,” in Frugivory and Seed Sispersal: Ecological and Evolutionary Aspects, eds T. H. Fleming and A. Estrada (Dordrecht: Springer), 299–318. doi: 10.1007/978-94-011-1749-4_21
Mazerolle, M. J. (2014). AICcmodavg: Model Selection and Multimodel Inference Based on (Q)AIC(c). Available online at: http://CRAN.R-project.org/package=AICcmodav
McClearn, D., Arroyo-Mora, J. P., Castro, E., Coleman, R. C., Espeleta, J. F., García-Robledo, et al. (2016). The Caribbean Lowland Evergreen Moist and Wet Forests. University of Chicago Press.
Moles, A. T., Warton, D. I., Stevens, R. D., and Westoby, M. (2004). Does a latitudinal gradient in seedling survival favour larger seeds in the tropics? Ecol. Lett. 7, 911–914. doi: 10.1111/j.1461-0248.2004.00647.x
Moles, A. T., and Westoby, M. M. (2004). Seedling survival and seed size: a synthesis of the literature. J. Ecol. 92, 372–383. doi: 10.1111/j.0022-0477.2004.00884.x
Mora, F., Martínez-Ramos, M., Ibarra-Manríquez, G., Pérez-Jimenez, A., Trilleras, J., and Balvanera, P. (2014). Testing chronosequences through dynamic approaches: time and site effects on tropical dry forest succession. Biotropica 47, 38–48. doi: 10.1111/btp.12187
Muniz-Castro, M. A., Williams-Linera, G., and Martínez-Ramos, M. (2012). Dispersal mode, shade tolerance, and phytogeographical affinity of tree species during secondary succession in tropical montane cloud forest. Plant Ecol. 213, 339–353. doi: 10.1007/s11258-011-9980-5
Muscarella, R., and Fleming, T. H. (2007). The role of frugivorous bats in tropical forest succession. Biol. Rev. 82, 573–590. doi: 10.1111/j.1469-185X.2007.00026.x
Nakagawa, S., and Schielzeth, H. (2013). A general and simple method for obtaining R2 from generalized linear mixed-effects models. Methods Ecol. Evol. 4, 133–142. doi: 10.1111/j.2041-210x.2012.00261.x
Nathan, R., and Muller-Landau, H. C. (2000). Spatial patterns of seed dispersal, their determinants and consequences for recruitment. Trends Ecol. Evol. 15, 278–285. doi: 10.1016/S0169-5347(00)01874-7
Norden, N., Chazdon, R. L., Chao, A., Jiang, Y. H., and Vílchez-Alvarado, B. (2009). Resilience of tropical rain forests: tree community reassembly in secondary forests. Ecol. Lett. 12, 385–394. doi: 10.1111/j.1461-0248.2009.01292.x
Palma, A. C., Goosem, M., Fensham, R. J., Goosem, S., Preece, N. D., Stevenson, P. R., et al. (2021). Dispersal and recruitment limitations in secondary forests. J. Veg. Sci. 32, e12975. doi: 10.1111/jvs.12975
Piotto, D., Montagnini, F., Thomas, W., Ashton, M., and Oliver, C. (2009). Forest recovery after swidden cultivation across a 40–year chronosequence in the Atlantic forest of southern Bahia, Brazil. Plant Ecology 205, 261–272. doi: 10.1007/s11258-009-9615-2
Poorter, L., Rozendaal, D. M., Bongers, F., de Jarcilene, S. A., Álvarez, F. S., and Andrade JL, Westoby, M. (2021). Functional recovery of secondary tropical forests. Proc. Natl. Acad. Sci. U.S.A. 118, e2003405118. doi: 10.1073/pnas.2003405118
Reid, J. L., Holl, K. D., and Zahawi, R. A. (2015). Seed dispersal limitations shift over time in tropical forest restoration. Ecological Applications 25, 1072–1082. doi: 10.1890/14-1399.1
Rocha, G. P. E., Vieira, D. L. M., and Simon, M. F. (2016). Fast natural regeneration in abandoned pastures in southern Amazonia. For. Ecol. Manage. 370, 93–101. doi: 10.1016/j.foreco.2016.03.057
Sanford, R. I. Jr., Paaby, P., Luvall, J. C., and Phillips, E. (1994). “Climate, geomorphology and aquatic systems,” in La Selva: Ecology and Natural History of a Neotropical Rain Forest, eds L. A. Mc Dade, K. S. Bawa, H. A. Hespenheide, and G. S. Hartshorn (Chicago, IL: University of Chicago Press), 19–33.
Santo–Silva, E. E., Almeida, W. R., Melo, F. P., Zickel, C. S., and Tabarelli, M. (2013). The nature of seedling assemblages in a fragmented tropical landscape: implications for forest regeneration. Biotropica 45, 386–394. doi: 10.1111/btp.12013
Sezen, U. U., Chazdon, R. L., and Holsinger, K. E. (2005). Genetic consequences of tropical second–growth forest regeneration. Science 307, 891. doi: 10.1126/science.1105034
Therneau, T. (2014). A Package for Survival Analysis in S. R package version 2.37-7, Available online at: http://CRAN.R-project.org/package=survival
Thomas, D. W., Cloutier, D., Provencher, M., and Houle, C. (1988). The shape of bird- and bat- generated seed shadows around a tropical fruiting tree. Biotropica 20, 347–348. doi: 10.2307/2388330
van der Pijl, L. (1969). Principles of Dispersal in Higher Plants. Berlin: Springer–Verlag. doi: 10.1007/978-3-642-87925-8
Wendt, A. L. (2014). Seed Dispersal, Seed Size and Seed Predation in Tropical Regrowth Forests (PhD Dissertation), University of Connecticut, Storrs, CT (United States).
Williamson, G. B., Bentos, T. V., Longworth, J. B., and Mesquita, R. C. G. (2012). Convergence and divergence in alternative successional pathways in Central Amazonia. Plant Ecol. Diver. 7, 1–8. doi: 10.1080/17550874.2012.735714
Keywords: chronosequence plots, dispersal profile, La Selva Biological Station, mixed-effect models, neotropics, second-growth forest, tree specialist groups, tropical wet forest
Citation: Wendt AL, Chazdon RL and Vargas Ramirez O (2022) Successional trajectories of seed dispersal mode and seed size of canopy tree species in wet tropical forests. Front. For. Glob. Change 5:946541. doi: 10.3389/ffgc.2022.946541
Received: 17 May 2022; Accepted: 26 July 2022;
Published: 14 September 2022.
Edited by:
Bonnie Grace Waring, Imperial College London, United KingdomReviewed by:
Gopal Shankar Singh, Banaras Hindu University, IndiaGerardo Avalos, University of Costa Rica, Costa Rica
Copyright © 2022 Wendt, Chazdon and Vargas Ramirez. This is an open-access article distributed under the terms of the Creative Commons Attribution License (CC BY). The use, distribution or reproduction in other forums is permitted, provided the original author(s) and the copyright owner(s) are credited and that the original publication in this journal is cited, in accordance with accepted academic practice. No use, distribution or reproduction is permitted which does not comply with these terms.
*Correspondence: Amanda L. Wendt, YXdlbmR0QGVhcnRoLmFjLmNy