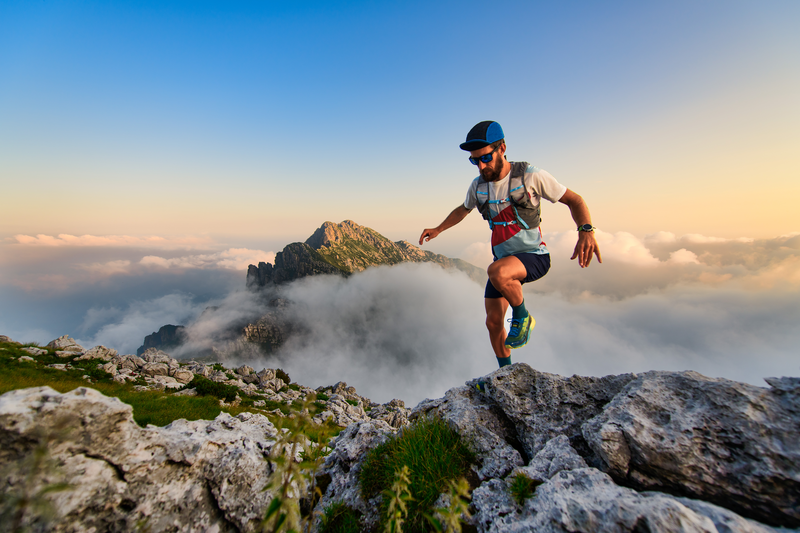
94% of researchers rate our articles as excellent or good
Learn more about the work of our research integrity team to safeguard the quality of each article we publish.
Find out more
ORIGINAL RESEARCH article
Front. For. Glob. Change , 16 August 2022
Sec. Forest Disturbance
Volume 5 - 2022 | https://doi.org/10.3389/ffgc.2022.940302
This article is part of the Research Topic Applications of Tree Rings in Altered Forests View all 6 articles
To sustainably manage forests, it is important to understand the historical fire regimes including the severity, frequency, seasonal timing of fires as well as the relationship between climate and fire in order to develop management plans that mimic and/or complements the natural disturbance pattern. The objectives of this study were to reconstruct the natural fire regime within a Pinus lumholtzii site in order to understand the fire regimes within this forest type as well as adjacent high timber value mixed-pine forests where historical fire regimes are not available due to past timber harvesting activities. Using 36 fire-scarred trees, we reconstructed the historical fire regimes in San Dimas, Durango, México for the last 238 years. We found a pattern of frequent fires but an absence of fire since 1962, with most fires (98%) being recorded in the spring season. The lack of fire for over six decades within these historically frequent fire forests could result in high fuel accumulation. Such conditions in combination with increasing temperatures and aridity in the region could increase the risk of high severity wildfires that could jeopardize the sustainability of these forests and the human communities that these forests support.
Timber harvesting within forested ecosystems has a long human history. Over time however, intensively managed forests tend to lose many important properties including productivity, diversity, and ultimately health and resilience. In turn, such forests are more prone to large-scale disturbances and potential-type conversion threatening their sustainability (Coop et al., 2020). To create long-term sustainable forests, it is important to understand the dynamics of those forests including natural disturbances such as fire (Agee, 1993; Allen et al., 2002) and the climate-fire relationship (Brown et al., 2008; Yocom et al., 2010; Falk et al., 2011; Cerano-Paredes et al., 2019).
In general, fire regimes within forested systems often dictate species composition, with pines generally dominating in areas with high fire frequencies (Fulé and Covington, 1997; Swetnam and Betancourt, 1998; Brown and Wu, 2005; Iniguez et al., 2016). To sustainably manage forests, it is important to understand the historical fire regimes including the severity, frequency, and seasonality as well as the relationship between climate and fire, in order to develop management plans that mimic and/or complements natural disturbance patterns (Agee, 1993; Allen et al., 2002; Fagre and Peterson, 2002). This strategy, in turn, allows for optimal forest health which makes forest more resilient to other potentially devastating disturbances such as climate change (Agee and Skinner, 2005). It is also important to understand how these fire regimes vary over time and in particular, how these regimes have changed recently due to social and political pressures (Covington et al., 1997; Heyerdahl and Alvarado, 2003; Yocom Kent et al., 2017).
Timber production is one of the primary economic factors in Mexico's Sierra Madre Occidental (SMO), highlighting the need to develop sustainable forest management plans based on an understanding of natural fire regimes. Timber harvesting within the Sierra Madre Occidental varies widely, but in general based on selectively thinning a percentage of the standing timber, targeting older or damaged individuals (Torres-Rojo et al., 2016; Quiñonez-Barraza et al., 2018). However, most often residual slash remains on-site increasing the amount of fuel within these forests. Timber harvesting has significantly reduced the amount of undisturbed natural areas (González Elizondo M., et al., 2017; González-Elizondo M. S., et al., 2017). As a result, fire regimes within these high-value timber-producing forests are unknown. Pinus lumholtzii, however, has low commercial value and is a quasi-endemic species of the SMO, meaning that it is found outside SMO but is very rare. Pure Pinus lumholtzii forests consisting of small stands surrounded, but are often adjacent to mixed-pine forest stands, that include P. durangensis and P. cooperi, which are important high-value commercial species (Pompa-García et al., 2013; Graciano-Ávila et al., 2020). Due to the low commercial value of Pinus lumholtzii, it is still possible to find sites with long and well-preserved fire scar records (González-Elizondo M. S., et al., 2017; Cerano-Paredes et al., 2022). Some previous dendrochronological studies report that this species can live for over 300 years (Chávez-Gándara et al., 2017; Villanueva-Díaz et al., 2018). Given the close proximity of these forest types, the lack of barriers to fire spread between forest types and similarity in species traits, it is resealable to assume that these forests shared similar historical fire regimes particularly fire frequencies and seasonality. Therefore, the information generated in P. lumholtzii forests is the only source of information available for understanding the role of fire within these forested landscapes as a whole. Understanding the role of fire and if/how fire regimes have changed is important information that allows forest and fire managers to develop plans that sustain these ecologically and commercially valuable forests into the future.
Unlike similar dry forests in the western United States, where fire regimes changed within a short period after the completion of the railroad, fire regime changes in northern Mexico vary in both time and space (Heyerdahl and Alvarado, 2003; Yocom Kent et al., 2017). For example, continuous or unaltered fire frequencies have been reconstructed within some sites in the SMO (Cerano-Paredes et al., 2010; Fulé et al., 2011; Molina-Pérez et al., 2017). However, in many other sites, abrupt changes in fire frequencies have been reconstructed with the timing often differing between sites (Fulé and Covington, 1997, 1999; Heyerdahl and Alvarado, 2003; Fulé et al., 2005; Cerano-Paredes et al., 2019). Given the importance of fire and the consequences of altered fire intervals, it is critical to determine not only if fire regimes changed but when those changes occurred, in order to evaluate forest structure changes and develop sustainable management plans moving forward. The objectives of this study were to:
(1) determine the natural fire regimes (severity, seasonality, and frequency of fires) within a pure P. lumholtzii site in the Sierra Madre Occidental of northern Mexico,
(2) determine if (and when) these natural fire regimes changed in the last century,
(3) determine the role of climate patterns in dictating fire occurrence.
Given the consequences of fire frequency changes within similar dry forests in the western U.S., including potential-type conversion and non-sustainable forests, it is clear that understanding fire regimes and their changes is a critical need in developing sustainable forest plans particularly in the face of climate change.
The study area was located in Río de Miravalles, San Dimas, Durango, Mexico, within the Sierra Madre Occidental, at an altitude of 2,500 m above sea level. The geographic coordinates of the site are 24° 18' 59.4” N and 105° 34' 50.5” W (Figure 1). The climate is temperate-humid with summer rains, and an average annual temperature of 18°C, with the temperature of the coldest month ranging from −3 to 18 °C. In contrast, temperatures in the warmest month range from 22 to 33°C. The mean annual precipitation varies from 500 to 1,500 mm, with precipitation in the driest month being less than 40 mm (García, 2004). Two types of soil are found in the study area, luvisol and regosol. Luvisol soils occur on flat or slightly sloping terrain (IUSS, 2015). Regosol soils, which are fragile and susceptible to erosion, are common in arid areas and mountainous terrain, which have a low moisture retention capacity (IUSS, 2015). The Pinus and Quercus genera represent more than 70% of the vegetation in the study area although pines tend to dominate the overstory while oaks dominate the understory. Among the most common species are Pinus lumholtzii, Pinus cooperí C.E. Blanco, Pinus durangensis Martínez and Fern., Pinus teocote Schlecht and Cham. and Quercus rugosa Nee. In particular P. lumholtzii, occurs as small stands on rocky and shallow soils (Rzedowski, 1978).
Figure 1. Study site location in the northern Mexico (A) and within the state of Durango (B). Location on 40 fire scarred trees collected within the 50-ha study site at Río de Miravalles (C).
At the study site, we collected fire-scar samples within an area of approximate 50 ha (Figures 1, 2), using a selective sampling strategy. That is, within the study site, we selected trees based on specific characteristics including: evidence of fire scars, number of scars, and longest-lived trees (Figures 2C,D) (Arno and Sneck, 1977; Cerano-Paredes et al., 2020). A total of 40 fire-scarred samples were collected, including eight partial cross-sections from live trees (Figures 2A,C) and 32 dead tree cross-sections from stumps, logs, or standing snags (Figure 2B). The sampling focused primarily on dead trees to minimize potential damage to live trees (Baisan and Swetnam, 1990).
Figure 2. Field photos of a live Pinus lumholtzii tree growing in rocky soils (A). Fire-scarred samples were collected from a combination of down logs (B) and standing live tree (C). Example of fire-scar sample that has been collected, prepared, and cross-dated using dendrochronological techniques, with this particular individual having 11 fire-scar dates (D).
In the laboratory, severely damaged samples (rotten and sectioned) were glued together and stabilized by mounting them on plywood. Once stabilized, each sample was cut to a uniform thickness between 3 and 5 cm, and polishing using sequentially finer sandpaper ranging from coarse (30 grit) to fine grain (1,200 grit). This process allowed for clear visibility of tree-ring growth structures, including fire-scar formations. The dating of each sample was carried out using standard dendrochronological techniques (Stokes and Smiley, 1996). The quality of the dating was then statistically verified using COFECHA (Holmes, 1983).
Once dated, position of each fire-scar within the growth ring was determined based on the methodology proposed by Baisan and Swetnam (1990), using the following categories: EE (beginning of earlywood), ME (middle of earlywood), LE (end of earlywood), L (latewood), and D (dormancy or ring limit). The different categories were grouped into two periods 1) spring (D + EE) and 2) summer (ME + LE + L) (Grissino-Mayer, 2001).
The fire-scar database was analyzed in the FHX2 software version 3.2 (Grissino-Mayer, 2001). The fire-scar record began with the earliest fire scar recorded in 1738 and ending on the field sampling year (2016). The period of analysis included only years with adequate sample depth defined as years when more than 10% of fire-scarred trees were recording at the site (1798–2016) (Grissino-Mayer et al., 1994). For each period, descriptive fire history metrics were generated including: mean fire interval (MFI), maximum and minimum fires intervals, and Weibull median probability interval (WMPI) (Grissino-Mayer, 2001). For each metric, three fire-scar filters were considered: 1) all fire-scarred years, 2) years where 10% or more of samples recorded a fire-scar, and 3) years where 25% or more of all samples recorded a fire-scar (Fulé et al., 2005; Yocom et al., 2010; Cerano-Paredes et al., 2020). The 25% filter represents years when fires were generally more widespread (Swetnam and Baisan, 2003). We also calculated the individual-tree fire interval using all cross-dated trees within our study site as a way to further understand fire intervals without having to use filters. To examine fire frequency changes over time, we calculated the number of fire years within a 20-year moving windows (Swetnam and Betancourt, 1998; Iniguez et al., 2008).
The relationship between climate variability and fire years was determined using Superposed Epoch Analysis (SEA) using the FHX2 software (Grissino-Mayer, 2001). Two climate proxies we used for this analysis including: local seasonal winter-spring precipitation reconstruction based on P. lumholtzii, which accounted for 52% (r = 0.73; p < 0.01) of the variability (Chávez-Gándara et al., 2017) and winter index NIÑO 3 SST estimate of El Niño Southern Oscillation (ENSO) variability (Cook, 2000). The two variables were calculated during the fire year as well as 5 years before and 2 years after the fire year. To evaluate the statistical significance of the SEA analysis, confidence intervals (95%) were calculated using the bootstrapped distribution of the climatic data with 1,000 replicates.
A total of 40 samples were collected in the field and 36 (90%) were successfully cross-dated, including all living trees (eight) and 28 of the 32 dead trees. The 36 samples yielded a total of 264 cross-dated fire scars. The oldest and most recent tree-rings were from 1653 and 2016, respectively. Similarly, the oldest and most recent fire-scars were recorded in 1738 and 1986, respectively. Our period of analysis, when greater than 10% of samples were recorded, ranged from 1798 to 2016 (Figures 3A,B).
Figure 3. Fire history timeline in a Pinus lumholtzii forest site in Rio Miravalles, San Dimas, Durango. (A) Percentage of recording fire scarred trees (blue line) and percent of trees that recorded a fire by year. (B) Individual fire-scarred tree timeline represented by horizontal lines in blue and vertical black dashes representing fire-scars recorded by that tree. Year when 10% or more trees recorded a fire are displayed along the bottom of the graph. The orange highlighted area indicates more extensive fire years. The green-highlighted area shows a period without extensive fires and a change in fire frequency after 1962.
Our results suggest the most fires within these forests were of relatively low severity. This is based on the fact that we were able to reconstruct the fire regime using individual trees that had multiple fire scars (Figure 3B). In general, based on the fire intervals, fires appear to be frequent in the P. lumholtzii forest, generally occurring at intervals between 3 and 20 years. Based on all fire scarred years, we found a MFI and WMPI of 3 years (Table 1). Similarly, based on the ≥10% filter, MFI and WMPI were 4.6 and 4.0 years, respectively. More extensive fires that scarred ≥25% of the samples occurred at intervals of less than 10 years (MFI and WMPI of 8.0 and 7.2 years, respectively). The average fire interval based on individual trees was 16.0 years.
Table 1. Historical fire interval statistics for a Pinus lumholtzii forest, in the Sierra Madre Occidental, Durango, Mexico.
Since 1962, our study site has experienced a drastic change in fire frequency (Figure 3B, green area) with no extensive fires (≥25% of the samples) being recorded in the last 54 years. Before 1980, the fire frequencies at the site varied between 1 and 8 fires per 20-year period, with high fire frequencies around 1900, and low fire frequencies around 1875 and 1935–1945 based on the 25% fire-scarred filter. Since 1980, the frequency of large fires (25% filter) has decreased and has remained at zero fires per 20 year, a pattern that was not observed in any previous period in the reconstructed fire history record (Figure 4C).
Figure 4. Spring-winter precipitation and NIÑO 3 SST condition in relation to fire frequencies within a Pinus lumholtzii sire in the Sierra Madre Occidental of northern Mexico. Tree-ring recorded fire years are identified by red stars and occur mainly during year with low precipitation (A) (Chávez-Gándara et al., 2017) and negative NIÑO 3 SST (B) (Cook, 2000), based on tree ring reconstructed of climate variables. Fire frequency is measured using a 20-year moving average based on fire years recorded by 25% or more of the fire-scarred trees (C). Yellow-highlighted area corresponds to a period of synchrony between drought conditions and high fire frequency and while a more recent period with no extensive fires in recent decades is highlighted in pink.
Seasonality was determined for 53% of the fire scars (Table 2), but the remaining 47% could not be determined due to small annual tree-rings (periods of suppression) (González-Elizondo M. S., et al., 2017) or advanced decay which made it impossible to reliably identify the position of the scar within the tree-ring (Cerano-Paredes et al., 2020). Most (98%) fires were recorded at the beginning of early wood (EE) and 2% recorded in the middle of the early wood (ME). No fire-scars were recorded at the end of earlywood (LE), latewood (L), and dormancy period (D). Therefore, 98% of the fires were categorized as spring events and 2% as summer events (Table 2). It was impossible to determine the seasonality of 47% of the fire scars due to wood decay or periods of growth suppression (Table 2).
The fire-climate analysis showed that over the last 2 centuries, there were 46 years with extensive fires (≥10% fire scarred samples) reconstructed in these P. lumholtzii site, which coincide with dry climatic condition indicated by below-average spring-winter precipitation (Figure 5A) and negative NIÑO 3 SST indices (Figure 5B), however this relationship was not significant. Similarly, no significant relationship was found with moisture conditions 1 or 2 years prior to these fires.
Figure 5. Superposed Epoch analysis shows the relationship between fire occurrence and reconstructed local winter-spring precipitation variability (A) and NIÑO 3 SST indices (B), for all fires recorded by ≥ 10% of fire-scarred samples. The year of the fire is indicated by year 0, with bars corresponding to the average climate anomaly for fire years along with climate conditions 5 years prior to the fire (negative values) and 2 years after the fire (positive values). The upper and lower dotted lines represent the 95% confidence interval.
Although we did not find a statistically significant (p > 0.05) relationship between fire occurrence and annual drought, more than 50% of the extensive fires reconstructed (≥10% filter) for the period between 1798 and 1962 were recorded during spring-winter drought years as indicated by below average precipitation (27 of 46 fires; 59%) (Figure 4A) and negative NIÑO 3 SST values (25 of 46 fires, 54%) (Figure 4B). Conversely, fire frequency analysis using a 20-year moving window shows no fires in the last decades despite the occurrence of drought conditions (precipitation below the average) during that time (Figure 4C).
Using a combination of dead and live trees, we were able to reconstruct the fire history the last 278 years at this P. lumholtzii site. Most of the work was conducted using samples from dead wood to minimize damaging living trees, as suggested by Baisan and Swetnam (1990). Likewise, it is corroborated that these forests are long-lived and allow us to reconstruct fire regimes over several centuries.
Our results suggest that since the beginning of the 18th century, these forests have experienced frequent low severity fires. The abundance of fire-scarred trees with multiple fire scars within the site suggests that fires burned within the sites, but did not kill the majority of mature trees which not only continued growing but recorded multiple fire events. When considering all fire scar years, the MFI was between 3 and 4 years, similar to that reported in other forests of the SMO (Fulé and Covington, 1997; Heyerdahl and Alvarado, 2003; Molina-Pérez et al., 2017; Cerano-Paredes et al., 2019). The frequency of extensive fire years (≥25% filter) was 8 years, similar to other studies in mixed pine forest types within the SMO which have reported MFI in this range (Fulé and Covington, 1997, 1999; Heyerdahl and Alvarado, 2003; Molina-Pérez et al., 2017; Cerano-Paredes et al., 2019). Few sites in the SMO report frequencies greater than 10 years (Fulé and Covington, 1997, 1999), however based on individual-tree fire intervals we found an MFI of 16 years.
Our results show a clear change in fire frequency after 1960 (Figure 3B) similar to that reported in mixed-pine forests in northern Mexico (Fulé and Covington, 1999; Heyerdahl and Alvarado, 2003; Cerano-Paredes et al., 2019). The abrupt change in fire frequency after 1950 in northern Mexico is due to a combination of local land-use changes rather than regional climate variability given fires did not generally cease synchronously across the region (Heyerdahl and Alvarado, 2003). That is, in some areas, frequent fires continue up to the present (Cerano-Paredes et al., 2010; Fulé et al., 2011; Molina-Pérez et al., 2017). In many other sites however, an abrupt cessation of fires has been attributed to land-use changes, the construction of roads and fire-breaks, timber harvesting and the formation of ejidos (communally own lands) (Fulé and Covington, 1997, 1999; Heyerdahl and Alvarado, 2003; Fulé et al., 2005; Yocom et al., 2010; Cerano-Paredes et al., 2019). The distribution of ejido lands resulted in the migration of people to forested ejidos in the SMO starting in the middle of the 20th century. This human migration has altered the fire regimes by introducing or intensifying cattle grazing (Fulé and Covington, 1997; Kaib, 1998; Heyerdahl and Alvarado, 2003).
Most fires in these P. lumholtzii forests appeared to have occurred in spring (98%) (Table 2), which is consistent with the seasonality reported in previous studies in mixed-pine forests within the SMO region (Fulé and Covington, 1997, 1999; Molina-Pérez et al., 2017; Cerano-Paredes et al., 2019). This suggests that historically most fires occurred after annual growth initiation, but before the onset of the monsoon rains. Spring fires in this region is likely associated with the low humidity, high temperatures, and high lightning occurrence that occur during this season [Servicio Meteorológico Nacional (SMN), 2017]. Similarly, during this time of year, the NDVI values are low (March–April), suggesting vegetation stress (Tucker et al., 2005) and creating ideal conditions for fire spread (Figure 6).
Figure 6. Monthly average precipitation (blue circle solid line), temperature (red triangles dashed line), lightning activity (pink squares solid line) and normalized difference vegetation index (NDVI) (green stars dashed line). Variables are average values recorded from 1951 to 2010 at weather stations close to our P. lumholtzii forest site [Servicio Meteorológico Nacional (SMN), 2017]. Values of NDVI indicate the photosynthetic rate of the vegetation or the level of vegetation stress. The NDVI values are monthly averages for the period 1981–2006 for the vegetation (Tucker et al., 2005). The gray highlighted areas indicate the spring period when most fires historically occurred.
The strong link between fire and climate (Swetnam and Betancourt, 1998) highlights the relevance of knowing the climate-fire relationship within different forest ecosystems. Interannual climatic variability (precipitation) and NIÑO 3 SST (ENSO) were not the significant factor in the occurrence of fires at this P. lumholtzii site. This is likely due to the fact that our study including a single site and climate patterns are more likely to drive regional fire pattern and only have a limited influence on fire timing within individual sites. However, extensive fires (≥10% of samples) were recorded during years with below-average regional precipitation and climatic indices (NIÑO 3) (Figures 5A,B). Likewise, during the 1798–1962 period, extensive fires within out study site occurred during below-average precipitation years and negative NIÑO 3 indices, which represents drought conditions for this region in the state of Durango (Figures 4A,B, respectively). In the future, the Inter-governmental Panel on Climate Change predicts decreasing precipitation and increasing temperature for this region of northern Mexico (Intergovernmental Panel on Climate Change IPCC, 2013), such conditions will likely contribute to an increasing risk of forest fires.
Annual climate variability in Mexico is partly due to ENSO, which significantly affects precipitation (Stahle and Cleaveland, 1993; Magaña et al., 2003; Cerano-Paredes et al., 2011; Stahle et al., 2012) and has two phases, El Niño and La Niña. El Niño favors wet winters that decrease fire activity, while La Niña favors dry winters resulting in a long fire season and increasing the probability of widespread fires (Heyerdahl and Alvarado, 2003; Cerano-Paredes et al., 2019). Although a significant ENSO-fire relationship was not found at our study area, it is important to consider future La Niña phase events that lead to vegetation stress and can trigger greater fire risk in the forests of this region of northern Mexico.
In general, frequent fires within dry forests maintained low tree densities, low fuel accumulations, and open forest conditions, which in turn resulted in low severity fires (Fulé and Covington, 1997). Conversely, numerous studies have documented that eliminating frequent fires can potentially facilitate greater tree establishment, and accumulation of fuels including ladder fuels leading to more severe fires in the future (Covington et al., 1997; Fulé and Covington, 1997, 1999; Heyerdahl and Alvarado, 2003; Fulé et al., 2005; Yocom et al., 2010; Rodríguez, 2014; Cerano-Paredes et al., 2019). That is, the high-frequency low-severity fire regime we found at this site prior to 1960 could be changing to a low-frequency high-severity fire regime in the future (Covington et al., 1997). Similar changes in fire regimes have been documented in dry forests across the western U.S., where recent fires are getting more severe (Singleton et al., 2019). Moreover, many of these pine species are not serotinous, but instead reproduce by seed from surviving individuals. This limits their recovery particularly within large high severity patches (Owen et al., 2019). Additionally, many of these forests also contain a number of oak species that are better adapted to high severity fires given their ability to resprout (Barton and Poulos, 2018). Post-fire dominance of oaks could further facilitate the transition of large areas from forests to shrublands. A decrease in forested area could jeopardize timber production and negatively affect local economies within these rural communities.
Given the importance of forested ecosystems to local economies in the SMO, it is critical to develop sustainable management plans that include fire in accordance with the natural fire regime which these ecosystems have adapted to. Pyrosilviculture or the idea of using fire to manage forest structure within fire-adapted forests is a new concept that could be particularly useful in actively managed forests of the SMO (North et al., 2021; York et al., 2021). Fire in the form of prescribed fire or naturally ignited fires for resourced benefit (Hunter et al., 2011, 2014) could potentially be used in the SMO to reduce tree densities and accumulated fuels which would decrease the probability of stand replacing fires in the future (Rodríguez, 2014). However, given the lack of fire for more than 60 years, fuel conditions may not favor restoring fire within Spring season similar to our results. Instead, fire may need to be re-introduced within the shoulder of the peak fire season (Young et al., 2020), either in the fall or winter, when weather and fuel conditions would be more favorable to achieving objectives while keeping fires within defined containment lines. Failing to restore frequent low-severity wildfires within these dry forests will likely result in more intense fires during drought years in the future. Such fires could be difficult to suppress, resulting in the loss of forests and timber production as well as other ecosystem services including water, recreation, infrastructure, cultural resources, and human communities.
Stand of pure P. lumholtzii forests is found in small pockets usually surrounded by mixed-pine forests; therefore, it is likely that the fires recorded within these forests also burned within adjacent forests. Therefore, the information provided in this study will be useful both for fire management and conservation of long-lived forests of P. lumholtzii and for fire management in adjacent forest types with high commercial value, where it is impossible to find long-lived individuals that allow us to reconstruct the history of the fire regime due to timber harvesting.
The raw data supporting the conclusions of this article will be made available by the authors, without undue reservation.
JC-P contributed to conception and design of the study. JC-P, GE-A, and JN-L collected the data. JC-P, JG-C, and RC-M cross-dated the fire-scars samples. JC-P, RC-M, and JI organized the database and performed the statistical analysis. JC-P and JI wrote the first draft of the manuscript with contributions from VCS. All authors contributed to the interpretation of the results and to the manuscript revision, and read and approved the submitted version.
The present investigation was carried out thanks to the financing through the project: CONAFOR-2014, C01-234547. Support was provided by the U.S. Forest Service, Rocky Mountain Research Station.
We gratefully acknowledge the M.C. Marcia Polet Chávez-Gándara, M. C. Viridiana Pereda-Breceda, and M. C. Teresita Herrera-Palacios for their assistance in field collections. Thanks the M. C. Sergio Jimenez and M. C. Mariana Marcial for their support with the spatial data. We would also like to acknowledge the comments from two reviewers whom provided excellent feedback that greatly improved this paper.
The authors declare that the research was conducted in the absence of any commercial or financial relationships that could be construed as a potential conflict of interest.
All claims expressed in this article are solely those of the authors and do not necessarily represent those of their affiliated organizations, or those of the publisher, the editors and the reviewers. Any product that may be evaluated in this article, or claim that may be made by its manufacturer, is not guaranteed or endorsed by the publisher.
Agee, J. K., and Skinner, C. N. (2005). Basic principles of forest fuel reduction treatments. Forest Ecol. Manage. 211, 83–96. doi: 10.1016/j.foreco.2005.01.034
Allen, C. D., Savage, M., Falk, D. A., Suckling, K. F., Swetnam, T. W., Schulke, T., et al. (2002). Ecological restoration of southwestern ponderosa pine ecosystems: a broad perspective. Ecol. Applic. 12, 1418–1433. doi: 10.1890/1051-0761(2002)012[1418:EROSPP]2.0.CO;2
Arno, S. F., and Sneck, K. M. (1977). “A method for determining fire history in coniferous forests of the mountain west,” in: Forest, I. (Ed.), Intermountain Forest and Range Experiment Station, Forest Service. US Department of Agriculture. p. 28.
Baisan, C. H., and Swetnam, T. W. (1990). Fire history on a desert mountain range: Rincon Mountain Wilderness, Arizona, USA. Canadian J. Forest Res. 20, 1559–1569. doi: 10.1139/x90-208
Barton, A. M., and Poulos, H. M. (2018). Pine vs. oaks revisited: conversion of Madrean pine-oak forest to oak shrubland after high-severity wildfire in the Sky Islands of Arizona. Forest Ecol. Manage. 414, 28–40. doi: 10.1016/j.foreco.2018.02.011
Brown, P. M., Heyerdahl, E. K., Kitchen, S. G., and Weber, M. H. (2008). Climate effects on historical fires (1630–1900) in Utah. Int. J. Wildl. Fire 17, 28–39. doi: 10.1071/WF07023
Brown, P. M., and Wu, R. (2005). Climate and disturbance forcing of episodic tree recruitment in a southwestern ponderosa pine landscape. Ecology 86, 3030–3038. doi: 10.1890/05-0034
Cerano-Paredes, J., Iniguez, J. M., Villanueva-Diaz, J., Cervantes-Martinez, R., Cambron-Sandoval, V. H., Estrada-Arellano, J. R., et al. (2020). Using tree-rings to reconstruct fire history information from forested areas. JoVE 164, e61698. doi: 10.3791/61698
Cerano-Paredes, J., Szejner, P., Gutiérrez-García, G., Cervantes-Martínez, R., Cambrón-Sandoval, V. H., Villanueva-Díaz, J., et al. (2022). How to Extract Climate Variability from Tree-Rings. J. Vis. Exp. 181, e63414. doi: 10.3791/63414
Cerano-Paredes, J., Villanueva-Díaz, J., Valdez-Cepeda, R. D., Arreola-Ávila, J. G., and Constante-García, V. (2011). El Niño Oscilación del Sur y sus efectos en la precipitación en la parte alta de la cuenca del río Nazas. Rev. Chapingo serie ciencias forestales y del ambiente 17, 207–215. doi: 10.5154/r.rchscfa.2010.09.076
Cerano-Paredes, J., Villanueva-Díaz, J., Vázquez-Selem, L., Cervantes-Martínez, R., Magaña-Rueda, V. O., Constante-García, V., et al. (2019). Climatic influence on fire regime (1700 to 2008) in the Nazas watershed, Durango, Mexico. Fire Ecol. 15, 1–14. doi: 10.1186/s42408-018-0020-x
Cerano-Paredes, J., Villanueva-Díaz, J., and Fulé, P. Z. (2010). Reconstrucción de incendios y su relación con el clima para la Reserva Cerro El Mohinora, Chihuahua. Rev. Mexicana de Ciencias Forestales 1, 63–74. doi: 10.29298/rmcf.v1i1.654
Chávez-Gándara, M. P., Cerano-Paredes, J., Nájera-Luna, J. A., Pereda-Breceda, V., Esquivel-Arriaga, G., Cervantes-Martínez, R., et al. (2017). Reconstrucción de la precipitación invierno-primavera con base en anillos de crecimiento de árboles para la región de San Dimas, Durango, México. Bosque (Valdivia) 38, 387–399. doi: 10.4067/S0717-92002017000200016
Cook, E. R. (2000). Niño 3 Index Reconstruction. International Tree-Ring Data Bank. IGBP PAGES/World Data Center-A for Paleoclimatology Data Contribution Series Number 2000-2052. Boulder, Colorado, USA: NOAA/NGDC Paleoclimatology Program.
Coop, J. D., Parks, S. A., Stevens-Rumann, C. S., Crausbay, S. D., Higuera, P. E., Hurteau, M. D., et al. (2020). Wildfire-driven forest conversion in western North American landscapes. BioScience 70, 659–673. doi: 10.1093/biosci/biaa061
Covington, W. W., Fule, P. Z., Moore, M. M., Hart, S. C., Mast, J. M., Sackett, S. S., et al. (1997). Restoring ecosystem health in ponderosa pine forests of the Southwest. J Forest. 95.
Fagre, D. B., and Peterson, D. L. (2002). “Modelling and monitoring ecosystem responses to climate change in three North American mountain ranges,” in Mountain biodiversity: a global assessment (C. Körner and EM Spehn, eds.). Parthenon, London. 249–259. doi: 10.4324/9780429342585-20
Falk, D. A., Heyerdahl, E. K., Brown, P. M., Farris, C., Fulé, P. Z., McKenzie, D., et al. (2011). Multi-scale controls of historical forest-fire regimes: new insights from fire-scar networks. Front. Ecol. Environ. 9, 446–454. doi: 10.1890/100052
Fulé, P. Z., and Covington, W. W. (1997). Fire regimes and forest structure in the Sierra Madre Occidental, Durango, Mexico. Acta Bot. Mex. 41, 43–79. doi: 10.21829/abm41.1997.791
Fulé, P. Z., and Covington, W. W. (1999). Fire regime changes in La Michilía Biosphere Reserve, Durango, Mexico. Conserv. Biol. 13, 640–652. doi: 10.1046/j.1523-1739.1999.97512.x
Fulé, P. Z., Ramos, G. M., Cortes, M. C., and Miller, A. M. (2011). Fire regime in a Mexican forest under indigenous resource management. Ecol. Appl. 21, 764–775 doi: 10.1890/10-0523.1
Fulé, P. Z., Villanueva, D. J., and Ramos, G. M. (2005). Fire regime in a conservation reserve, Chihuahua, Mexico. Can. J. For. Res. 35, 320–330. doi: 10.1139/x04-173
García, E. (2004). Modificaciones al sistema de clasificación climática de Köppen. Universidad Nacional Autónoma de México.
González Elizondo, M., González Elizondo, M. D. S., Villanueva Díaz, J., and Cerano Paredes, J. (2017). Evaluación del potencial dendroclimático de Pinus lumholtzii BL Rob. and Fernald. Rev. mexicana de ciencias forestales 8, 28–54. doi: 10.29298/rmcf.v8i42.18
González-Elizondo, M. S., González-Elizondo, M., López-Enríquez, I. L., Tena-Flores, J. A., González-Gallegos, J. G., Ruacho-González, L., et al. (2017). Diagnóstico del conocimiento taxonómico y florístico de las plantas vasculares del norte de México. Botanical Sci. 95, 760–779. doi: 10.17129/botsci.1865
Graciano-Ávila, G., Alanís-Rodríguez, E., Aguirre-Calderón, O. A., González-Tagle, M. A., Treviño-Garza, E. J., Mora-Olivo, A., et al. (2020). Cambios estructurales de la vegetación arbórea en un bosque templado de Durango, México. Acta Botánica Mexicana. 127, 1–16. doi: 10.21829/abm127.2020.1522
Grissino-Mayer, H. D. (2001). FHX2—software for analyzing temporal and spatial patterns in fire regimes from tree rings. Tree. Res. 57, 115–124
Grissino-Mayer, H. D., Baisan, C. H., and Swetnam, T. W. (1994). Fire History and Age Structure Analyses in the Mixed Conifer and Spruce-Fir Forests of Mount Graham. Final Report. Mount Graham Red Squirrel Study Committee. Phoenix, AZ, USA: U.S. Fish and Wildlife Service. 73.
Heyerdahl, E. K., and Alvarado, E. (2003). “Influence of climate and land use on historical surface fires in pine-oak forests, Sierra Madre Occidental, Mexico,” in Fire and Climatic Change in Temperate Ecosystems of the Western Americas; New York, NY, USA: Springer. 196–217. doi: 10.1007/0-387-21710-X_7
Holmes, R. L. (1983). Computer-assisted quality control in tree ring dating and measurement. Tree-Ring Bull. 43, 69–75.
Hunter, M. E., Iniguez, J. M., and Farris, C. A. (2014). “Historical and current fire management practices in two wilderness areas in the southwestern United States: The Saguaro Wilderness Area and the Gila-Aldo Leopold Wilderness Complex,” in Gen. Tech. Rep. RMRS-GTR-325. Fort Collins, CO: US Department of Agriculture, Forest Service, Rocky Mountain Research Station. 38. doi: 10.2737/RMRS-GTR-325
Hunter, M. E., Iniguez, J. M., and Lentile, L. B. (2011). Short-and long-term effects on fuels, forest structure, and wildfire potential from prescribed fire and resource benefit fire in southwestern forests, USA. Fire Ecol. 7, 108–121. doi: 10.4996/fireecology.0703108
Iniguez, J. M., Swetnam, T. W., and Baisan, C. H. (2016). Fire history and moisture influences on historical forest age structure in the sky islands of southern Arizona, USA. J. Biogeogr. 43, 85–95. doi: 10.1111/jbi.12626
Iniguez, J. M., Swetnam, T. W., and Yool, S. R. (2008). Topography affected landscape fire history patterns in southern Arizona, USA. Forest Ecol. Manage. 256, 295–303. doi: 10.1016/j.foreco.2008.04.023
Intergovernmental Panel on Climate Change IPCC (2013). “Climate Change: the physical science basis,” in Contribution of Working Group I to the IPCC Fifth Assessment Report of the IPCC, eds T. F. Stocker, D. Qin, G. K. Plattner, M. Tignor, S. K. Allen, J. Boschung, A. Nauels, Y. Xia, V. Bex, P. M. Midgley (Cambridge, UK; New York, NY: Cambridge University Press).
IUSS (2015). IUSS Working Group WRB (International Union of Soil Sciences). Base referencial mundial del recurso suelo 2014. Actualización 2015. Sistema internacional de clasificación de suelos para la nomenclatura de suelos y la creación de leyendas de mapas de suelos. Informes sobre recursos mundiales de suelos 106. Roma: FAO.
Kaib, J. M. (1998). Fire history in riparian canyon pine-oak forests and the intervening desert grasslands of the southwest borderlands: a dendroecological, historical, and cultural inquiry. Arizona: The University of Arizona.
Magaña, V. O., Vázquez, J. L., Pérez, J. L., and Pérez, J. B. (2003). Impact of El Niño on precipitation in Mexico. Geofísica Internacional 42, 313–330. doi: 10.22201/igeof.00167169p.2003.42.2.269
Molina-Pérez, I. M., Cerano-Paredes, J., Rosales-Mata, S., Villanueva-Díaz, J., Cervantes-Martínez, R., Esquivel-Arriaga, G., et al. (2017). Historical fire frequency (1779-2013) in pine-oak forests in the community of Charcos, Mezquital, Durango. Rev. Chapingo serie ciencias forestales y del ambiente 23, 91–104. doi: 10.5154/r.rchscfa.2016.03.017
North, M. P., York, R. A., Collins, B. M., Hurteau, M. D., Jones, G. M., Knapp, E. E., et al. (2021). Pyrosilviculture needed for landscape resilience of dry western United States forests. J. Forestry 119, 520–544. doi: 10.1093/jofore/fvab026
Owen, S. M., Patterson, A. M., Gehring, C. A., Sieg, C. H., Baggett, L. S., and Fulé, P. Z. (2019). Large, high-severity burn patches limit fungal recovery 13 years after wildfire in a ponderosa pine forest. Soil Biol. Biochem. 139, 107616. doi: 10.1016/j.soilbio.2019.107616
Pompa-García, M., Cerano-Paredes, J., and Fulé, P. Z. (2013). Variation in radial growth of Pinus cooperi in response to climatic signals across an elevational gradient. Dendrochronologia 31, 198–204. doi: 10.1016/j.dendro.2013.05.003
Quiñonez-Barraza, G., Zhao, D., De Los Santos-Posadas, H. M., and Corral-Rivas, J. J. (2018). Considering neighborhood effects improves individual dbh growth models for natural mixed-species forests in Mexico. Ann Forest Sci. 75, 1–11. doi: 10.1007/s13595-018-0762-2
Rodríguez, T. D. A. (2014). “Incendios de vegetación: su ecología, manejo e historia,” in ColPos, C.P., UACH, ANCF, AMPF (Eds.), Parque Nacional Iztaccíuatl Popocatépetl. México: CONAFOR, CONANP, vol. 1. p. 814.
Servicio Meteorológico Nacional (SMN). (2017). Información Climatológica. Available online at: http://smn.cna.gob.mx/es/climatologia/informacion-climatologica (accessed February, 2022).
Singleton, M. P., Thode, A. E., Meador, A. J. S., and Iniguez, J. M. (2019). Increasing trends in high-severity fire in the southwestern USA from 1984 to 2015. Forest Ecol. Manage. 433, 709–719. doi: 10.1016/j.foreco.2018.11.039
Stahle, D. W., Burnette, D. J., Villanueva-Díaz, J., Heim, R. R., Fye, F. K., Cerano-Paredes, J., et al. (2012). Pacific and Atlantic influences on Mesoamerican climate over the past millennium. Climate Dyn. 39, 1431–1446. doi: 10.1007/s00382-011-1205-z
Stahle, D. W., and Cleaveland, M. K. (1993). Southern Oscillation extremes reconstructed from tree rings of the Sierra Madre Occidental and southern Great Plains. J. Climate 6, 129–140. doi: 10.1175/1520-0442(1993)006<0129:SOERFT>2.0.CO;2
Stokes, M. A., and Smiley, T. L. (1996). An Introduction to Tree-Ring Dating. Chicago: University of Chicago Press. 73.
Swetnam, T. W., and Baisan, C. H. (2003). “Tree-ring reconstructions of fire and climate in the Sierra Nevada and Southwestern United States,” in Veblen, T.T., Baker, W.L., Montenegro, G., Swetnam, T.W. (Eds.), Fire and Climate Changes in Temperate Ecosystems of the Western Americas. New York, NY: Springer. p. 158–195. doi: 10.1007/0-387-21710-X_6
Swetnam, T. W., and Betancourt, J. L. (1998). Mesoscale disturbance and ecological response to decadal climatic variability in the American Southwest. J. Clim. 11, 3128–3147. doi: 10.1175/1520-0442(1998)011<3128:MDAERT>2.0.CO;2
Torres-Rojo, J. M., Moreno-Sánchez, R., and Mendoza-Briseño, M. A. (2016). Sustainable forest management in Mexico. Curr. Forestry Rep. 2, 93–105. doi: 10.1007/s40725-016-0033-0
Tucker, C. J., Pinzon, J. E., Brown, M. E., Slayback, D. A., Pak, E. W., Mahoney, R., et al. (2005). An extended AVHRR 8-km NDVI dataset compatible with MODIS and SPOT vegetation NDVI data. Int. J. Remote Sens. 26, 4485–4498. doi: 10.1080/01431160500168686
Villanueva-Díaz, J., González-Elizondo, M., Cerano-Paredes, J., Estrada Ávalos, J., Martínez Sifuentes, A. R., and Rosales Mata, S. (2018). Red dendrocronológica de pino triste (Pinus lumholtzii BL Rob. and Fernald) en la Sierra Madre Occidental para reconstrucción de lluvia estacional. Madera y bosques 24, 1–14. doi: 10.21829/myb.2018.2421530
Yocom Kent, L. L., Fulé, P. Z., Brown, P. M., Cerano-Paredes, J., Cornejo-Oviedo, E. C., Cortés-Montaño, C., et al. (2017). Climate drives fire synchrony but local factors control fire regime change in northern Mexico. Ecosphere 8, e01709. doi: 10.1002/ecs2.1709
Yocom, L. L., Fulé, P. Z., Brown, P. M., Cerano, P. J., Villanueva, D. J., Falk, D. A., et al. (2010). El Niño Southern Oscillation effect on a fire regime in northeastern Mexico has changed over time. Ecology 91, 1660–1671. doi: 10.1890/09-0845.1
York, R. A., Noble, H., Quinn-Davidson, L. N., and Battles, J. J. (2021). Pyrosilviculture: Combining prescribed fire with gap-based silviculture in mixed-conifer forests of the Sierra Nevada. Canadian J. Forest Res. 51, 781–791. doi: 10.1139/cjfr-2020-0337
Keywords: pino triste, wild fires, forest management, Anthropocene, drought
Citation: Cerano-Paredes J, Iniguez JM, González-Castañeda JL, Cervantes-Martínez R, Cambrón-Sandoval VH, Esquivel-Arriaga G and Nájera-Luna JA (2022) Increasing the risk of severe wildfires in San Dimas, Durango, Mexico caused by fire suppression in the last 60 years. Front. For. Glob. Change 5:940302. doi: 10.3389/ffgc.2022.940302
Received: 10 May 2022; Accepted: 08 July 2022;
Published: 16 August 2022.
Edited by:
Giovanna Battipaglia, University of Campania Luigi Vanvitelli, ItalyReviewed by:
Alan Tepley, University of Montana, United StatesCopyright © 2022 Cerano-Paredes, Iniguez, González-Castañeda, Cervantes-Martínez, Cambrón-Sandoval, Esquivel-Arriaga and Nájera-Luna. This is an open-access article distributed under the terms of the Creative Commons Attribution License (CC BY). The use, distribution or reproduction in other forums is permitted, provided the original author(s) and the copyright owner(s) are credited and that the original publication in this journal is cited, in accordance with accepted academic practice. No use, distribution or reproduction is permitted which does not comply with these terms.
*Correspondence: Julián Cerano-Paredes, Y2VyYW5vLmp1bGlhbkBnbWFpbC5jb20=
Disclaimer: All claims expressed in this article are solely those of the authors and do not necessarily represent those of their affiliated organizations, or those of the publisher, the editors and the reviewers. Any product that may be evaluated in this article or claim that may be made by its manufacturer is not guaranteed or endorsed by the publisher.
Research integrity at Frontiers
Learn more about the work of our research integrity team to safeguard the quality of each article we publish.