- 1Microbial Ecology Group, Department of Biology, Lund University, Lund, Sweden
- 2Centre for Environmental and Climate Science, Lund University, Lund, Sweden
- 3CAS Key Laboratory of Mountain Surface Processes and Ecological Regulation, Institute of Mountain Hazards and Environment, Chinese Academy of Sciences, Chengdu, China
A major fraction of nitrogen (N) in boreal forest soils is found in organic forms associated with soil organic matter (SOM) and mineral particles. The capacity of ectomycorrhizal (ECM) fungal symbionts to access this N is debated, considering that these fungi have lost many of the genes for decomposing organic matter that were present in their saprotrophic ancestors. To gain a molecular-level understanding of the N-mining processes in ECM fungi, we developed an experimental approach where the processes of decomposition were studied in parallel with the changes in the structure and properties of the organic matter. We showed that ECM fungi have significant capacities to assimilate organic N associated with SOM and mineral surfaces. The decomposition mechanisms differ between species, reflecting the lignocellulose decomposition mechanisms found in their saprotrophic ancestors. During N-mining, the ECM fungi processed the SOM to a material with increased adsorptive properties to iron oxide mineral particles. Two pathways contributed to these changes: Extracellular modifications of the SOM and secretion of mineral surface reactive metabolites. Some of these metabolites have iron(III)-reducing activities and can participate in extracellular Fenton reactions and redox reactions at iron oxide mineral surfaces. We conclude that the traditional framework for understanding organic N acquisition by ECM fungi from recalcitrant SOM must be extended to a framework that includes how those decomposition activities affect the stabilization and reactivity of mineral-associated SOM. The activity through these complex networks of reactions is decisive for the overall effect of ECM fungal decomposition on nutrients and C-cycling in forest ecosystems.
Introduction
Primary production in boreal forest ecosystems is commonly limited by nitrogen (N) availability (Högberg et al., 2017). A large fraction of the N in these soils is present in organic forms, primarily as proteinaceous compounds, but also as heterocyclic N molecules (Nannipieri and Paul, 2009; Leinweber et al., 2013), that result from the decomposition of plant, microbe, and animal tissues. This organic N is complexed with other organic molecules present in soil organic matter (SOM), including cellulose, hemicellulose, chitin, lignin, lipids, polyphenols, and other biomolecules (Simpson et al., 2007). The bioavailable fraction of organic N, i.e., compounds that can be taken up by plants and microbes, is considered to consist of water-soluble molecules of relatively small size. This fraction includes amino acids and small peptides, and plants have been shown to directly assimilate amino acids (Näsholm et al., 2009). However, dissolved N compounds account for a small fraction of the organic N pool (Jones et al., 2004). Although there is evidence that plants can increase the available N pool by secretion of proteases and subsequent proteolysis (Godlewski and Adamczyck, 2007; Adamczyk et al., 2008; Paungfoo-Lonhienne et al., 2008), for trees in boreal forests, fungal root symbionts including ectomycorrhizal (ECM) fungi are thought to play a key role in this process (Smith and Read, 2008; Högberg et al., 2017). To access the organic N compounds present in soil, ECM fungi must have the capacity to decompose at least partly the SOM complexes where N is embedded. ECM fungi have evolved several times from saprotrophic ancestors (Kohler et al., 2015; Tedersoo and Smith, 2017). The ancestral decomposition strategies likely resembled the enzymatic system for the decomposition of lignocellulose in white-rot (WR) wood-decaying fungi, the two-step mechanism involving hydroxyl radicals (⋅OH) generated by Fenton chemistry and hydrolytic enzymes in brown-rot (BR) wood-decaying fungi (Hatakka and Hammel, 2010), or enzymatic decomposition systems in litter decomposers, presumably similar to those of WR fungi (Floudas et al., 2020; Ruiz-Dueñas et al., 2020). The convergent loss of genes encoding plant cell wall-degrading enzymes (PCWDEs) in ECM fungi has been used as an argument against the view that ECM fungi have a significant capacity to decompose SOM (Pellitier and Zak, 2018). However, some genes coding for PCWDEs have been conserved and these display very diverse types and numbers across ECM lineages (Kohler et al., 2015), which suggest that ECM fungi have retained and adapted some features of the decomposition mechanisms found in their saprotrophic ancestors. Moreover, ECM lineages have retained the genes involved in the decomposition of microbial cell walls (Miyauchi et al., 2020), which suggests that ECM fungi might selectively target compounds of microbial origin that can be rich in N, such as chitin and proteins.
Assuming that the main function of the decomposition activity of ECM fungi is to mobilize nutrients entrapped in organic matter, rather than obtaining metabolic carbon (C) (Lindahl and Tunlid, 2015), an outstanding question then is if these decomposition activities render the remaining organic matter more or less suitable for further decomposition by saprotrophic microbes (Frey, 2019). SOM can be stabilized against microbial decomposition via polymerization reactions that lead to the formation of recalcitrant humic substances (Schnitzer and Monreal, 2011). The humification model for SOM stabilization has been increasingly questioned, and it has been proposed that SOM becomes protected from microbial decomposition by interacting with mineral particles or by being incorporated into molecular aggregates (Lehmann and Kleber, 2015). It follows that the processing of SOM accompanying the N-mining activities in ECM fungi may affect soil C stabilization via several different mechanisms.
A common focus among ecologists examining organic nitrogen acquisition in ECM fungi has been the enzymatic decomposition of SOM including the activity and expression of PCWDEs and proteolytic enzymes. Less attention has been paid to how decomposition by ECM fungi affects the stabilization of soil C. To cover both aspects, we have developed an experimental approach where the decomposition activity and organic N uptake by ECM fungi were examined in parallel with analyzing structural modifications and formation of mineral-stabilized SOM (Figure 1). Coupled with experimental manipulations, space and time-resolved experiments and modeling, our approach has made it possible to make connections between biology and chemistry, and to identify extracellular components and physiological conditions that control organic matter decomposition and N assimilation in ECM fungi. Below, we summarize and discuss some of the key findings from these experiments.
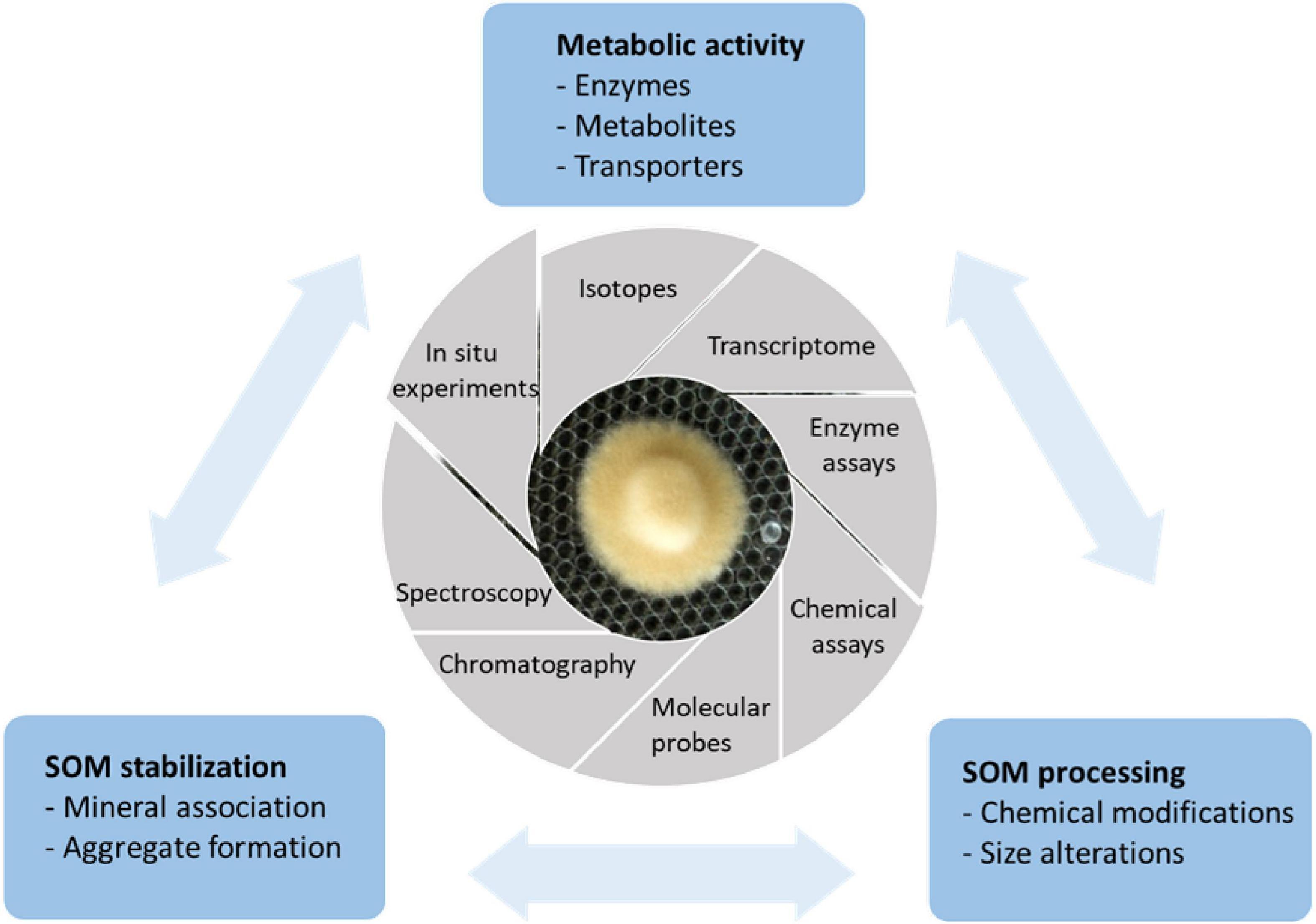
Figure 1. Laboratory-scale model systems and multimethod approaches used in our research group to unravel the mechanisms for organic N acquisition in ectomycorrhizal (ECM) fungi and their consequences for soil C stabilization. Single species of ECM fungi are grown on a layer of glass beads immersed in liquid medium containing organic matter or specific compounds representing the major compound classes present in organic matter. Soil organic matter has been extracted with water from the organic soil layer of a Norway spruce stand. This layer contains a large part of the soil but can also contain significant proportions of mineral constituents (Keiluweit et al., 2012). The extracted fraction is commonly referred to as dissolved organic matter (DOM). DOM is believed to be the most bioavailable fraction of SOM, and it plays a key role in the formation of organic matter-mineral associations and molecular aggregates that enhance the stabilization of soil C (Bolan et al., 2011; Lehmann and Kleber, 2015). In certain experiments, N-sources and DOM have been coated to pure Fe(III) minerals including ferrihydrite and goethite, which are abundant in forest soils and exhibit differences with respect to surface properties and redox potential. The metabolic activity of the fungi, including the uptake, secretion of oxidative and hydrolytic enzymes and metabolites have been analyzed using isotope, transcriptome, enzyme assays, and various chemical analyses. Molecular probes (e.g., coumarin) have been used to measure the production of ⋅OH radicals. In parallel, an array of different chromatographic and spectroscopic methods [e.g., NMR, infrared spectroscopy (IR), pyrolysis gas chromatography/mass spectrometry (Py-GC/MS), and X-ray absorption spectroscopy (XAS)] have been used to gain information on the structure, composition, and heterogeneity of SOM and its interactions with minerals. In order to perform more detailed studies on the interactions between components in the DOM and iron oxide minerals, culture filtrates have been collected for in situ experiments where the reactions at the mineral surface have been followed using infrared attenuated total reflectance (IR-ATR) spectroscopy.
Soil organic matter decomposition—diverse mechanisms but similar function
Spectroscopic analyses together with transcriptome profiling have provided firm experimental evidence that ECM fungi decompose SOM with mechanisms resembling those seen in their saprotrophic ancestors (Rineau et al., 2012; Shah et al., 2016). When five ECM fungi derived from BR, WR, or litter decomposing ancestors were grown on dissolved organic matter (DOM) from the organic soil layer of a Norway spruce stand as the only N source, they all oxidized compounds in the DOM, including polysaccharides and lignin residues (Shah et al., 2016). The observed oxidative modifications of the DOM during decomposition were similar across the examined ECM species, but the extent of the oxidation differed and was related to the growth rate and the rate of N uptake by the ECM fungi. The rapidly growing, long-distance-exploration types (Agerer, 2001) oxidized DOM and assimilated N to a greater extent than did the slow-growing, short- and medium-distance-exploration types.
Despite the similarity in the chemical transformation of the SOM extract, each species expressed a different set of transcripts encoding enzymes that are typically associated with oxidative degradation of plant cell wall compounds in wood-decaying fungi (Levasseur et al., 2013). The enzymes included: Laccases, for the oxidation of a wide range of organic compounds; glucose-methanol-choline oxidoreductases (GMCOs) and copper-radical oxidases (CROs), for reduction of oxygen to peroxide likely supporting Fenton chemistry and peroxidases (PODs) with extracellular hydrogen peroxide; various PODs and tyrosinases. The fungi also expressed a number of genes encoding extracellular peptidases, presumably involved in the hydrolysis of proteins released from the organic matter. The variation in the expression pattern of such peptidases was smaller than that of oxidoreductases associated with decomposition of lignocellulose (Shah et al., 2016).
Links between organic matter decomposition and the release of organic N, and how these processes are regulated by nutritional cues, have been identified in studies of the ECM fungi Paxillus involutus and Laccaria bicolor, representing species with BR and litter decomposing ancestors, respectively (Nicolás et al., 2019). In both fungi, assimilation of the N sources followed a temporal pattern. The readily available inorganic N source (i.e., ammonium) was utilized before assimilation of the organic amide-N sources commenced, and these organic N sources were liberated via SOM oxidation. Thus, SOM oxidation was initiated upon depletion of ammonium and ceased when the fungi experienced C limitation. Despite the different evolutionary histories and decomposition mechanisms of the two ECM species, there was functional convergence between them with a common tight link between SOM oxidation and liberation of organic N, and similar nutritional regulation of the processes. This suggests a dual control over the decomposition activities of ECM fungi, i.e., supply of photosynthetic products from the host plant and the characteristics of the N sources in soil.
Fenton reaction and organic N acquisition in Paxillus involutus
SOM decomposition by P. involutus is similar to the BR wood-decaying mechanism and involves a two-step process of ⋅OH oxidation and enzymatic hydrolysis (Nicolás et al., 2019). The ⋅OH radicals generated by the Fenton reaction also oxidize and thereby facilitate the liberation of N from proteins (Op De Beeck et al., 2018). Moreover, the oxidation of the protein by ⋅OH radicals is followed by the induction of extracellular proteolytic activity. Notably, oxidized proteins induced a greater extracellular proteolytic activity than did unmodified proteins, suggesting a functional link between oxidation and hydrolysis of protein substrates. The key factors regulating the Fenton reaction during protein acquisition by P. involutus were captured in a mathematical model that predicts that the production of ⋅OH radicals occurs in a burst and that the concentrations of ammonium and Fe(II) are the key factors controlling ⋅OH production (Op De Beeck et al., 2018). The timing and the onset of the Fenton burst are controlled by a positive feedback mechanism where the fungus senses and responds to degradation products of the oxidized protein substrate.
Transformation of organic matter through anabolic and catabolic processes
During decomposition and assimilation of N, P. involutus processes DOM components toward smaller size molecules and molecules with increased polar group content. This increases the propensity of the processed organic matter for adsorption to mineral particles (Wang et al., 2017). These results agree with the conceptual model of SOM formation and stabilization proposed by Lehmann and Kleber (2015). Less expected was the finding that SOM decomposition by P. involutus was accompanied by secretion of extensive amounts of low molecular weight compounds (> 10% new biomass C) that also contributed to enhanced mineral retention. NMR analyses of the secreted compounds revealed a range of functional groups, including alkyl, aromatic, phenolic, and carbonyl groups. The secreted compounds were mainly low molecular weight (below 6.5 kDa) metabolites, but also larger size fractions were detected as well as both mineral-surface reactive and non-reactive compounds. Overall, this suggests diverse biological functionalities among the secreted compounds.
Moreover, decomposition of DOM by P. involutus generates a modified DOM that displays an increased iron-reducing capacity (Rineau et al., 2012). Recent experiments showed that such processed DOM had a significant capacity to reductively dissolve ferrihydrite and goethite, two common iron-oxide minerals in boreal forest soils (Krumina et al., 2022). Detailed studies of the molecular reactions at the water–iron oxide interfaces using in situ IR spectroscopy showed that further oxidation of adsorbed DOM was triggered by adding H2O2, which initiated Fenton reactions both at the iron minerals surface (heterogenous Fenton reactions) and in solution (homogenous Fenton reactions). These processes caused an extensive radical oxidation of the organic matter-iron mineral associations generating a high concentration of surface-associated oxalate. Many soil microorganisms including ECM fungi have the capacity to secrete oxalic acids (Lapeyrie et al., 1987; Jones et al., 2003), and it is thought that oxalate plays a key role in the acquisition of nutrients from mineral particles (Landeweert et al., 2001; Keiluweit et al., 2015). Our results suggest an additional and overlooked extracellular source of oxalic acid in soils that needs to be considered.
Nitrogen acquisition from mineral-associated proteins
Approximately 20–50% of the SOM in boreal forest soils is associated with reactive minerals (Kramer and Chadwick, 2018). In particular, the protein fraction is known to bind strongly to such minerals (Kleber et al., 2007), and a common idea is that protein desorption must precede enzymatic degradation (Mikutta et al., 2007; Jilling et al., 2018). Accordingly, mineral-associated proteins are rarely considered as bioavailable N for soil microorganisms (Jilling et al., 2018). This assumption is challenged by recent results showing that the ECM fungi P. involutus, Hebeloma cylindrosporium, and Piloderma olivaceum assimilate N from iron mineral-associated proteins by hydrolyzing the protein directly at the mineral surface without initial desorption (Wang et al., 2020, 2021). This proteolytic surface activity was moderated by the secretion of low-molecular-weight metabolites that conditioned the mineral surface and thereby facilitated proteolysis. The chemical structures of these metabolites have not been characterized in detail, but IR spectroscopy indicated a chemical diversity that differed between ECM fungal species (Wang et al., 2020, 2021).
Discussion
The recent research summarized above emphasizes the need to develop a framework for organic N acquisition in ECM fungi that includes the effect of SOM decomposition activities on the stabilization of organic matter in addition to the commonly considered enzymatic mechanisms and mobilization of N from recalcitrant SOM (Figure 2). We have identified two processes in ECM fungi that affect the adsorptive properties of SOM: Ex vivo modifications where extracellular enzymes and oxygen radicals attack and transform DOM into a material with enhanced propensity to associate with mineral particles; in vivo turnover pathway where the fungi synthesize and secrete mineral-surface reactive metabolites (Liang et al., 2017). Apart from metabolites, dead ECM hyphae (i.e., necromass) may significantly contribute to the formation of stable organic matter in forest soils (Godbold et al., 2006; Clemmensen et al., 2013). Necromass is formed through the in vivo turnover pathway when mycelial growth is arrested due to stress conditions in the environment, typically C-limitation (Ekblad et al., 2013). Experiments suggest that the decomposing activities of ECM fungi can show diverse responses to prolonged C limitation (Nicolás et al., 2019), which emphasize that further studies are needed to establish links between microbial growth and SOM turnover, and how these processes are controlled by nutrient- and C conditions. It is also clear that different ECM fungi differ in the way they decompose SOM. Hence, the molecular mechanisms involved in SOM decomposition need to be characterized in more ECM fungi to get a better overview of the functional diversity of decomposition systems employed by ECM fungi across a wide phylogenetic range.
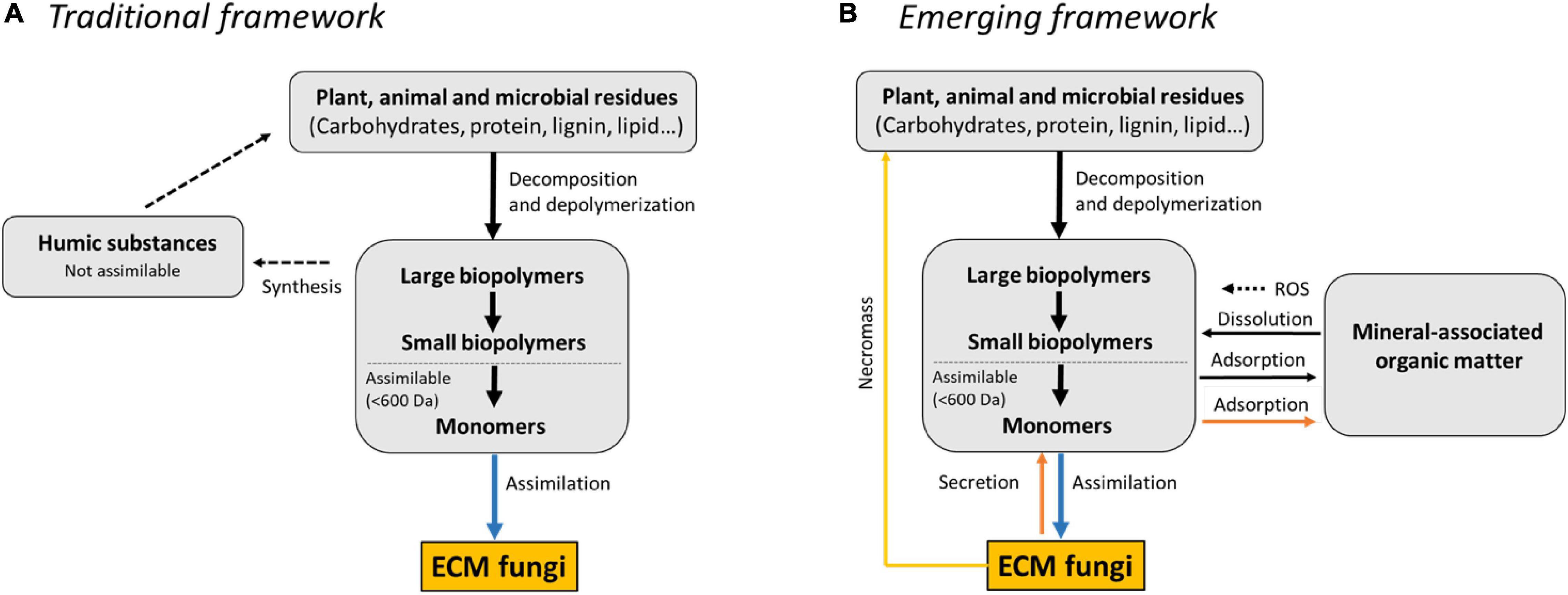
Figure 2. The traditional and emerging frameworks for organic nitrogen acquisition in ectomycorrhizal (ECM) fungi. (A) The traditional framework. In this framework, organic N molecules, including proteins, are considered to be embedded in soil organic matter (SOM) and are stabilized by selective preservation of recalcitrant plant biopolymers coupled with abiotic synthesis of complex, highly aromatic humic substances resistant to microbial decay (black, dotted arrow). SOM is decomposed by ECM fungi (black arrow) through the activity of extracellular enzymes to small, water-soluble molecules such as amino acids and peptides that can be assimilated by the fungi (blue arrow). (B) Emerging framework. In this framework, SOM is viewed as a range of organic fragments and microbial products that are continuously processed into smaller molecules by decomposing microorganisms. SOM is decomposed by ECM fungi (black arrow) through the activity of extracellular enzymes and oxygen radicals generated by Fenton chemistry to small, water-soluble molecules that can be assimilated by the fungi (blue arrow). Concomitantly, SOM is modified into a material with enhanced affinity to mineral particles. Moreover, SOM decomposition is accompanied with a substantial secretion of low-molecular-weight compounds (brown arrow). Some of them have Fe(III) reducing activity and can participate in the dissolution of iron oxide mineral particles and generation of reactive oxygen species (ROS) by Fenton-like mechanisms. Others bind strongly to mineral surfaces and can facilitate the hydrolysis of mineral-associated organic N sources (i.e., proteins) by conditioning the mineral surface in a way that protects direct sorption and subsequent deactivation of fungal proteases. ECM fungal biomass, in turn, also contributes substantially to the formation of SOM through necromass that is left behind after cell death.
Microbial processing of organic matter may also contribute to the formation of stabilized SOM by producing compounds with an increased propensity to become incorporated into molecular aggregates (Lehmann and Kleber, 2015). However, little is known about the molecular aggregates present in the organic matter extracted from boreal forest soils. A recent study from our group shows that ca. 40–70% of the total organic C in DOM extracted from boreal forest is present in a colloidal size fraction (Meklesh et al., 2022). Important challenges for future research are to examine whether nutrients present in colloidal fractions are accessible to ECM fungi and whether the decomposing activity of ECM fungi will alter the colloidal fractions of organic matter.
The significance of metabolites in decomposition processes has been questioned by soil scientists considering that soil microorganisms do not have the energy and nutrient resources needed for synthesizing metabolites in quantities required for their actions (Lehmann and Kleber, 2015). This may be true for saprotrophic fungi that encounter metabolic C limitations. However, the situation is different for ECM fungi, which can receive an extensive amount of energy from the host plants (Högberg and Högberg, 2002). The importance of metabolites has been highlighted in the so-called unbutton model where low molecular weight organic acids, such as citric and oxalic acids produced by ECM fungi, are suggested to play an important role in releasing nutrients from SOM aggregates. The acids disrupt the aggregates by forming complexes with network-forming metal ions (Clarholm et al., 2015). Our research suggests that ECM fungi secrete metabolites that can facilitate nutrient acquisition with other mechanisms involving Fenton chemistry and redox reactions at mineral surfaces. These metabolites work in concert with extracellular enzymes, and most likely ECM fungi have evolved mechanisms to keep the reactants and their products close to the hyphae. Extracellular polymeric substances (EPS) may be important functional components that assure that extracellular decomposition reactions occur at the right time and in the right place (Op De Beeck et al., 2020, 2021).
A recent discovery is the large capacity of ECM fungi with diverse evolutionary origins to reductively dissolve iron minerals (Wang et al., 2020, 2021). The mechanisms of these electron-transfer reactions are not known, but in agreement with mechanisms identified in iron-reducing bacteria (Glasser et al., 2017), we hypothesize that electrons are transferred from the cell to the mineral surface by small, secreted molecules, i.e., extracellular electron shuttles (EESs). This is supported by experiments showing that reductive dissolution of solid iron(III) complexes by P. involutus is accompanied with secretion of iron(III) reducing metabolites (Shah et al., 2021). The reductive dissolution of iron(III) minerals may, in the presence of O2 and/or H2O2, trigger the production of highly reactive oxygen species via Fenton-like reactions (Garrido-Ramirez et al., 2010; Krumina et al., 2022). Thus, the capacity to oxidize SOM by ⋅OH may be much more widespread among ECM fungi than has so far been anticipated. A recent study based on the analyses of reactive Mn(III) and Fe(II/III) species and oxidative activities suggests that the prevalence of Mn- vs. Fe-mediated oxidative mechanisms in forest soils varies with soil depth; Mn-mediated oxidation predominates in surface soil layers and Fe-mediated oxidation in deeper, mineral-containing soil layers (Jones et al., 2020). This differentiation correlates with the vertical distribution of fungal communities where saprotrophic litter decomposing fungi inhabit surface soil layers while ECM fungi predominate in deeper layers, following the colonization depth of the roots they grow on (Lindahl et al., 2007). We hypothesize that the success of ECM fungi in colonizing deeper soil layers is partly due to their ability to control iron redox chemistry, and exploiting this for oxidative decomposition and nutrient acquisition. This hypothesis is also supported by observations made on the landscape level, as Mn availability and the production of Mn-peroxidases are negatively correlated to carbon storage across different northern and temperate forests (Stendahl et al., 2017; Kranabetter et al., 2021). In temperate forests, the switch from Mn-peroxidases to Fenton-mediated decomposition with increased soil weathering resulted not only in deeper organic horizons but also a twofold increase in mineral soil C content (Kranabetter et al., 2021).
Reductive dissolution of mineral particles also facilitates the mobilization of other nutrient sources than N; in particular phosphorus (P). In soils, a major fraction of P is associated with Fe(III) minerals (Arai and Sparks, 2007). Several studies have shown that ECM fungi can access mineral-bound P (Wallander et al., 1997; Rosling, 2009), but the precise mechanism(s) are still being debated. A recent study has demonstrated that mineral-associated P can be released via reductive dissolution promoted by Fe(III) reducing bacterial metabolites (McRose and Newman, 2021). We propose that a similar mechanism may contribute to the mobilization of P by ECM fungi.
It should be noted that organic N mining and stabilization of soil C by ECM fungi in boreal forests soils include several processes and mechanisms that have not been examined in our experiments. For example, the production of fungal necromass might have a profound effect on soil C stabilization (Fernandez et al., 2016), and the release of low-molecular-weight compounds by mycorrhizal fungal exudation may stimulate the decomposition activities of saprotrophic microorganisms (Phillips et al., 2012). Recent research has shown that soil C sources might be stabilized not only by association with mineral particles but also by interactions with root-derived tannins (Adamczyk, 2021). Moreover, competition with free-living saprotrophic fungi may potentially suppress SOM decomposition (the so-called Gadgil effect) (Fernandez and Kennedy, 2016).
To conclude, laboratory-scale experiments with single ECM fungal species and multimethod analytical approaches have increased our current understanding of the complex mechanisms underlying soil organic N acquisition in ECM fungi, including their impacts on SOM stabilization. The activity of these pathways is likely to be strongly dependent on the soil geochemistry, the presence of other microorganisms, and the allocation of host photosynthate to the ECM mutualists (Frey, 2019; Zak et al., 2019). High priority must be given to identifying molecular markers that can be used for probing the activity in the decomposing pathways of ECM fungi in more complex laboratory systems and in the field. We believe that experiments in standardized laboratory systems coupled with transcriptomics and advanced spectroscopic methods will accelerate the discovery of molecular markers that can provide functional links between ECM fungal activities, SOM dynamics, and organic N uptake. Such markers will significantly increase our knowledge on the extent to which ECM fungi get access organic N embedded in SOM and adsorbed on mineral particles in situ. Combined with measurements of the fungal transfer of this N to the plant host, the research has important implications for understanding the role of ECM fungi to plant growth, and the cycling of C and N in boreal forest ecosystems. Key research questions to be addressed by future research include:
- Is SOM decomposition by ECM fungi the result of partial retention of ancestral mechanisms or development of novel mechanisms?
- How is SOM decomposition by ECM fungi regulated by the host C supply and the nutrient conditions in the soil environment?
- How do ECM physiology and growth strategy affect SOM decomposition and N transfer to the host plant?
- Are specific SOM molecules targeted by the decomposition activities of ECM fungi, and what are the chemical characteristics of ECM-decomposed SOM?
- Are nutrients present in the colloidal fraction of DOM available to ECM fungi and does DOM decomposition change the colloidal properties of DOM?
- How prevalent is the ECM capacity to reductively dissolve iron-oxide minerals and what are the consequences for Fenton-based oxidation of organic matter in boreal forest soils? (SOM chemistry).
- How can mechanisms and reaction pathways from laboratory-scale experiments accurately describing SOM decomposition by ECM fungi be incorporated into models that predict soil carbon budgets?
Author contributions
AT wrote the first draft of the manuscript. DF, MO, TW, and PP wrote sections of the manuscript. All authors contributed to manuscript revision, read, and approved the submitted version.
Funding
The work was supported by grants from the Knut and Alice Wallenberg Foundation (2013.00073) and the Swedish Research Council (621-2012-03890 and 2017-04261).
Conflict of interest
The authors declare that the research was conducted in the absence of any commercial or financial relationships that could be construed as a potential conflict of interest.
Publisher’s note
All claims expressed in this article are solely those of the authors and do not necessarily represent those of their affiliated organizations, or those of the publisher, the editors and the reviewers. Any product that may be evaluated in this article, or claim that may be made by its manufacturer, is not guaranteed or endorsed by the publisher.
References
Adamczyk, B. (2021). How do boreal forest soils store carbon? BioEssays 2021:10. doi: 10.1002/bies.202100010
Adamczyk, B., Godlewsky, M., Zimny, J., and Zimny, A. (2008). Wheat (Triticum aestivum) seedlings secrete proteases from the roots and, after protein addition, grow well on medium without inorganic nitrogen. Plant Biol. 10, 718–724. doi: 10.1111/j.1438-8677.2008.00079.x
Agerer, R. (2001). Exploration types of ectomycorrhizae. A proposal to classify ectomycorrhizal systems according to their patterns of differentiation and putative ecological importance. Mycorrhiza 11, 107–114. doi: 10.1007/s005720100108
Arai, Y., and Sparks, D. L. (2007). Phosphate reaction dynamics in soils and soil components: a multiscale approach. Adv. Agron. 94, 135–179. doi: 10.1016/S0065-2113(06)94003-6
Bolan, N. S., Adriano, D. C., Kunhikrishnan, A., James, T., McDowell, R., and Senesi, N. (2011). Dissolved organic matter: biogeochemistry, dynamics, and environmental significance in soils. Adv. Agron. 110, 3–75. doi: 10.1016/B978-0-12-385531-2.00001-3
Clarholm, M., Skyllberg, U., and Rosling, A. (2015). Organic acid induced release of nutrients from metal-stabilized soil organic matter–the unbutton model. Soil Biol. Biochem. 84, 168–176. doi: 10.1016/j.soilbio.2015.02.019
Clemmensen, K. E., Bahr, A., Ovaskainen, O., Dahlberg, A., Ekblad, A., Wallander, H., et al. (2013). Roots and associated fungi drive long-term carbon sequestration in boreal forest. Science 339, 1615–1618. doi: 10.1126/science.1231923
Ekblad, A., Wallander, H., Godbold, D. L., Cruz, C., Johnson, D., Baldrian, P., et al. (2013). The production and turnover of extramatrical mycelium of ectomycorrhizal fungi in forest soils: role in carbon cycling. Plant Soil 366, 1–27. doi: 10.1007/s11104-013-1630-3
Fernandez, C. W., and Kennedy, P. G. (2016). Revisiting the ‘Gadgil effect’: do interguild fungal interactions control carbon cycling in forest soils? New Phytol. 209, 1382–1394. doi: 10.1111/nph.13648
Fernandez, C. W., Langley, J. A., Chapman, S., McCormack, M. L., and Koide, R. T. (2016). The decomposition of ectomycorrhizal fungal necromass. Soil Biol. Biochem. 93, 38–49. doi: 10.1016/j.soilbio.2015.10.017
Floudas, D., Bentzer, J., Ahrén, D., Johansson, T., Persson, P., and Tunlid, A. (2020). Uncovering the hidden genomic diversity of litter-decomposition mechanisms in mushroom-forming fungi. ISME J. 14, 2046–2059. doi: 10.1038/s41396-020-0667-6
Frey, D. S. (2019). Mycorrhizal fungi as mediators of soil organic matter dynamics. Annu. Rev. Ecol. Evol. Syst. 50, 237–259. doi: 10.1111/j.1461-0248.2006.00948.x
Garrido-Ramirez, E. G., Theng, B. K. G., and Mora, M. L. (2010). Clays and oxide minerals as catalysts and nanocatalysts in Fenton-like reactions — A review. Appl. Clay Sci. 47, 182–192. doi: 10.1016/j.clay.2009.11.044
Glasser, N. R., Saunders, S., and Newmann, D. K. (2017). The colourful world of extracellular electron shuttles. Annu. Rev. Microbiol. 71, 731–751. doi: 10.1146/annurev-micro-090816-093913
Godbold, D. L., Hoosbeek, M. R., Lukac, M., Cotrufo, M. F., Janssens, I. A., Cuelemans, R., et al. (2006). Mycorrhizal hyphal turnover as a dominant process for carbon input into soil organic matter. Plant Soil 281, 15–24. doi: 10.1007/s11104-005-3701-6
Godlewski, M., and Adamczyck, B. (2007). The ability of plants to secrete proteases by roots. Plant Physiol. Biochem. 45, 657–664. doi: 10.1016/j.plaphy.2007.06.001
Hatakka, A., and Hammel, K. E. (2010). “Fungal biodegradation of lignocellulose,” in The Mycota Vol. X, Industrial applications, ed. M. Hofrichter (Berlin: Springer-Verlag), 319–340. doi: 10.1007/978-3-642-11458-8_15
Högberg, M. N., and Högberg, P. (2002). Extramatrical ectomycorrhizal mycelium contributes one-third of microbial biomass and produces, together with associated roots, half the dissolved organic carbon in a forest soil. New Phytol. 154, 791–795. doi: 10.1046/j.1469-8137.2002.00417.x
Högberg, P., Näsholm, T., Franklin, O., and Högberg, M. N. (2017). Tamm Review: On the nature of the nitrogen limitation to plant growth in Fennoscandian boreal forests. Forest Ecol. Manag. 403, 161–185. doi: 10.1016/j.foreco.2017.04.045
Jilling, A., Keiluweit, M., Contosta, A. R., Frey, S., Schimel, J., Schnecker, J., et al. (2018). Minerals in the rhizosphere: overlooked mediators of soil nitrogen availability to plants and microbes. Biogeochemistry 139, 103–122. doi: 10.1007/s10533-018-0459-5
Jones, D. L., Dennis, P. G., Owen, A. G., and van Hees, P. A. W. (2003). Organic acid behavior in soils - misconceptions and knowledge gaps. Plant Soil. 248, 31–41. doi: 10.1023/A:1022304332313
Jones, M. E., LaCroix, R. E., Zeigler, J., Ying, S. C., Nico, P. S., and Keiluweit, M. (2020). Enzymes, manganese, or iron? Drivers of oxidative organic matter decomposition in soils. Env. Sci. Technol. 54, 14114–14123. doi: 10.1021/acs.est.0c04212
Jones, D. L., Shannon, D., Murphy, D. V., and Farrar, J. (2004). Role of dissolved organic nitrogen (DON) in soil N cycling in grassland soils. Soil Biol. Biochem. 36, 749–756. doi: 10.1016/j.soilbio.2004.01.003
Keiluweit, M., Bougoure, J. J., Zeglin, L. H., Myrold, D. O., Weber, P. K., Pett-Ridge, J., et al. (2012). Nano-scale investigation of the association of microbial nitrogen residues with iron (hydro)oxides in a forest soil O-horizon. Geochim. Cosmochim. Acta 95, 213–226. doi: 10.1016/j.gca.2012.07.001
Keiluweit, M., Bougoure, J. J., Nico, P. S., Pett-Ridge, J., Weber, P. K., and Kleber, M. (2015). Mineral protection of soil carbon counteracted by root exudates. Nat. Clim. Change 5, 588–595. doi: 10.1038/nclimate2580
Kleber, M., Sollins, P., and Sutton, R. (2007). A conceptual model of organo-mineral interactions in soils: self-assembly of organic molecular fragments into zonal structures on mineral surfaces. Biogeochemistry 85, 9–24. doi: 10.1007/s10533-007-9103-5
Kohler, A., Kuo, A., Nagy, L. G., Morin, E., Barry, K. W., Buscot, F., et al. (2015). Convergent losses of decay mechanisms and rapid turnover of symbiosis genes in mycorrhizal mutualists. Nat. Genet. 47, 410–415. doi: 10.1038/ng.3223
Kranabetter, J. M., Philpott, T. J., and Dunn, D. E. (2021). Manganese limitations and the enhanced soil carbon sequestration of temperate rainforests. Biogeochemistry 156, 195–209. doi: 10.1007/s10533-021-00840-5
Kramer, M. G., and Chadwick, O. A. (2018). Climate-driven thresholds in reactive mineral retention of soil carbon at the global scale. Nat. Clim. Change 8, 1104–1108. doi: 10.1038/s41558-018-0341-4
Krumina, L., Op, De Beeck, M., Meklesh, V., Tunlid, A., and Persson, P. (2022). Ectomycorrhizal fungal transformation of dissolved organic matter: consequences for reductive iron oxide dissolution and Fenton-based oxidation of mineral-associated organic matter. Front. Earth Sci. 2022:763695. doi: 10.3389/feart.2022.763695
Landeweert, R., Hoffland, E., Finlay, R. D., Kuyper, T. W., and van Breemen, N. (2001). Linking plants to rocks: ectomycorrhizal fungi mobilize nutrients from minerals. Trends Ecol. Evol. 16, 248–254. doi: 10.1016/s0169-5347(01)02122-x
Lapeyrie, F., Chilvers, G. A., and Bhem, C. A. (1987). Oxalic acid synthesis by the mycorrhizal fungus Paxillus involutus (Batsch. ex Fr.) Fr. New Phytol. 106, 139–146. doi: 10.1111/j.1469-8137.1987.tb04797.x
Lehmann, J., and Kleber, M. (2015). The contentious nature of soil organic matter. Nature 528, 60–68. doi: 10.1038/nature16069
Leinweber, P., Kruse, J., Baum, C., Arcand, M., Knight, J. D., Farrell, R., et al. (2013). Advances in understanding organic nitrogen chemistry in soils using state-of-the-art analytical techniques. Adv. Agron. 119, 83–151. doi: 10.1016/B978-0-12-407247-3.00002-0
Levasseur, A., Drula, E., Lombard, V., Coutinho, P. M., and Henrissat, B. (2013). Expansion of the enzymatic repertoire of the CAZy database to integrate auxiliary redox enzymes. Biotechnol. Biofuels 6, 1–14. doi: 10.1186/1754-6834-6-41
Liang, C., Schimel, J. P., and Jastrow, J. D. (2017). The importance of anabolism in microbial control over soil carbon storage. Nat. Microbiol. 2:nmicrobiol2017105. doi: 10.1038/nmicrobiol.2017.105
Lindahl, B. D., Ihrmark, K., Boberg, J., Trumbore, S. E., Hogberg, P., Stenlid, J., et al. (2007). Spatial separation of litter decomposition and mycorrhizal nitrogen uptake in a boreal forest. New Phytol. 173, 611–620. doi: 10.1111/j.1469-8137.2006.01936.x
Lindahl, B., and Tunlid, A. (2015). Ectomycorrhizal fungi – potential organic matter decomposers, yet not saprophytes. Tansley insight review. New Phytol. 205, 1443–1447. doi: 10.1111/nph.13201
McRose, D. L., and Newman, D. K. (2021). Redox-active antibiotics enhance phosphorus bioavailability. Science 37, 1033–1037. doi: 10.1126/science.abd1515
Meklesh, M., Gentile, L., Andersson, E., Bhattacharya, A., de Farias, M. A., Cardoso, M. B., et al. (2022). Characterization of the colloidal properties of dissolved organic matter from forest soils. Front. Soil Sci. 2022:832706. doi: 10.3389/fsoil.2022.832706
Mikutta, R., Mikutta, C., Kalbitz, K., Scheel, T., Kaiser, K., and Jahn, R. (2007). Biodegradation of forest floor organic matter bound to minerals via different binding mechanisms. Geochim. Cosmochim. Ac. 71, 2569–2590. doi: 10.1016/j.gca.2007.03.002
Miyauchi, S., Kiss, E., Kuo, A., Drula, E., Kohler, A., Sánchez-García, M., et al. (2020). Large-scale genome sequencing of mycorrhizal fungi provides insights into the early evolution of symbiotic traits. Nat. Commun. 2020:18795. doi: 10.1038/s41467-020-18795-w
Nannipieri, P., and Paul, E. (2009). The chemical and functional characterization of soil N and its biotic components. Soil Biol. Biochem. 41, 2357–2369. doi: 10.1016/j.soilbio.2009.07.013
Näsholm, T., Kiellnd, K., and Ganeteg, U. (2009). Uptake of organic nitrogen by plants. New Phytol. 182, 31–48. doi: 10.1111/j.1469-8137.2008.02751.x
Nicolás, C., Martin-Bertelsen, T., Floudas, D., Bentzer, J., Smits, M., Johansson, T., et al. (2019). The soil organic matter decomposition mechanisms in ectomycorrhizal fungi are tuned for liberating soil organic nitrogen. ISME J. 13, 977–988. doi: 10.1038/s41396-018-0331-6
Op De Beeck, M., Troein, C., Peterson, C., Persson, P., and Tunlid, A. (2018). Fenton reaction facilitates organic nitrogen acquisition by an ectomycorrhizal fungus. New Phytol. 218, 335–343. doi: 10.1111/nph.14971
Op De Beeck, M., Troein, C., Siregar, S., Gentile, L., Abbondanza, G., Peterson, C., et al. (2020). Regulation of fungal decomposition at single-cell level. ISME J. 14, 896–905. doi: 10.1038/s41396-019-0583-9
Op De Beeck, M., Persson, P., and Tunlid, A. (2021). Fungal extracellular polymeric matrices – highly specialized microenvironments that allow fungi to control soil organic matter reactions. Soil Biol. Biochem. 159:108304. doi: 10.1016/j.soilbio.2021.108304
Paungfoo-Lonhienne, C., Lonhienne, T. G., Rentsch, D., Robinson, N., Christie, M., Webb, R. I., et al. (2008). Plants can use protein as a nitrogen source without assistance from other organisms. P. Natl. Acad. Sci. USA 105, 4524–4529. doi: 10.1073/pnas.0712078105
Pellitier, P. T., and Zak, D. R. (2018). Ectomycorrhizal fungi and the enzymatic liberation of nitrogen from soil organic matter: why evolutionary history matters. New Phytol. 217, 68–73. doi: 10.1111/nph.14598
Phillips, R. P., Meier, I. C., Bernhardt, E. S., Grandy, A. S., Wickings, K., and Finzi, A. C. (2012). Roots and fungi accelerate carbon and nitrogen cycling in forest exposed to elevated CO2. Ecol. Lett. 15, 1042–1049. doi: 10.1111/j.1461-0248.2012.01827.x
Rineau, F., Roth, D., Shah, F., Smits, M., Johansson, T., Canback, B., et al. (2012). The ectomycorrhizal fungus Paxillus involutus converts organic matter in plant litter using a trimmed brown-rot mechanism involving Fenton chemistry. Env. Microbiol. 14, 1477–1487. doi: 10.1111/j.1462-2920.2012.02736.x
Rosling, A. (2009). Trees, mycorrhiza and minerals—field relevance of in vitro experiments. Geomicrobiol. J. 26, 389–401. doi: 10.1080/01490450902929324
Ruiz-Dueñas, F. J., Barrasa, J. M., Sánchez-Garcia, M., Camarero, S., Miyauchi, S., Serrano, A., et al. (2020). Genomic analysis enlightens Agaricales lifestyle evolution and increasing peroxidase diversity. Mol. Biol. Evol. 38, 1428–1446. doi: 10.1093/molbev/msaa301
Schnitzer, M., and Monreal, C. M. (2011). Quo vadis soil organic matter research? A biological link to the chemistry of humification. Adv. Agron. 113, 139–213.
Shah, F., Nicolás, C., Bentzer, J., Ellström, M., Smits, M., Rineau, F., et al. (2016). Ectomycorrhizal fungi decompose soil organic matter using oxidative mechanisms adapted from saprotrophic ancestors. New Phytol. 209, 1705–1719. doi: 10.1111/nph.13722
Shah, F., Gressler, M., Nehzati, S., Op, De Beeck, M., Gentile, L., et al. (2021). Secretion of iron(III)-reducing metabolites during protein acquisition by the ectomycorrhizal fungus Paxillus involutus. Microorganisms 9:35. doi: 10.3390/microorganisms9010035
Simpson, A. J., Song, G., Smith, E., Lam, B., Novotny, E. H., and Hayes, M. H. (2007). Unraveling the structural components of soil humin by use of solution-state nuclear magnetic resonance spectroscopy. Environ. Sci. Technol. 41, 876–883. doi: 10.1021/es061576c
Stendahl, J., Berg, B., and Lindahl, B. D. (2017). Manganese availability is negatively associated with carbon storage in northern coniferous forest humus layers. Sci. Rep. 7, 1–6. doi: 10.1038/s41598-017-15801-y
Tedersoo, L., and Smith, M. E. (2017). “Ectomycorrhizal Fungal Lineages: Detection of Four New Groups and Notes on Consistent Recognition of Ectomycorrhizal Taxa in High-Throughput Sequencing Studies,” in Biogeography of Mycorrhizal Symbiosis, ed. L. Tedersoo (New York, NY: Springer), 125–142.
Wallander, H., Wickman, T., and Jacks, G. (1997). Apatite as a P source in mycorrhizal and non-mycorrhizal Pinus sylvestris seedlings. Plant Soil 196, 123–131.
Wang, T., Tian, Z., Tunlid, A., and Persson, P. (2020). Nitrogen acquisition from mineral-associated proteins by an ectomycorrhizal fungus. New Phytol. 228, 697–711.
Wang, T., Tian, Z., Bengtson, P., Tunlid, A., and Persson, P. (2017). Mineral surface-reactive metabolites secreted during fungal decomposition contribute to the formation of soil organic matter. Environ. Microbiol. 19, 5117–5129. doi: 10.1111/1462-2920.13990
Wang, T., Persson, P., and Tunlid, A. (2021). A widespread mechanism in ectomycorrhizal fungi to access nitrogen from mineral-associated proteins. Environ. Microbiol. 19, 5117–5129. doi: 10.1111/1462-2920.15539
Keywords: ectomycorrhizal fungi, decomposition, soil C stabilization, iron oxide minerals, nitrogen acquisition, metabolites
Citation: Tunlid A, Floudas D, Op De Beeck M, Wang T and Persson P (2022) Decomposition of soil organic matter by ectomycorrhizal fungi: Mechanisms and consequences for organic nitrogen uptake and soil carbon stabilization. Front. For. Glob. Change 5:934409. doi: 10.3389/ffgc.2022.934409
Received: 02 May 2022; Accepted: 01 July 2022;
Published: 22 July 2022.
Edited by:
Huajun Yin, Chengdu Institute of Biology (CAS), ChinaReviewed by:
Marty Kranabetter, Ministry of Forests and Range, CanadaBartosz Adamczyk, Natural Resources Institute Finland (Luke), Finland
Copyright © 2022 Tunlid, Floudas, Op De Beeck, Wang and Persson. This is an open-access article distributed under the terms of the Creative Commons Attribution License (CC BY). The use, distribution or reproduction in other forums is permitted, provided the original author(s) and the copyright owner(s) are credited and that the original publication in this journal is cited, in accordance with accepted academic practice. No use, distribution or reproduction is permitted which does not comply with these terms.
*Correspondence: Anders Tunlid, anders.tunlid@biol.lu.se