- 1Programa de Pós-Graduação em Ecologia, Departamento de Ecologia, Instituto de Biologia, Universidade Federal do Rio de Janeiro, Rio de Janeiro, Brazil
- 2Programa de Pós-Graduação em Meteorologia, Departamento de Meteorologia, Instituto de Geociências, Universidade Federal do Rio de Janeiro, Rio de Janeiro, Brazil
- 3Departamento de Ecologia, Instituto de Biologia, Universidade Federal do Rio de Janeiro, Rio de Janeiro, Brazil
Due to the increasing human impact on natural resources, we assessed the harvesting of non-timber forest products (NTFP) to verify demographic changes in populations of native palm trees. Euterpe edulis is native to the Atlantic Forest in Brazil, characterized by high deforestation and fragmentation. This palm is also targeted for palm heart and fruit harvesting. The threats posed by such factors motivated this study, as they might lead to a decrease in natural populations. The viability of sustainably harvesting the species in small fragmented areas is unknown. We performed simulations for palm heart and fruit harvesting in three small populations (entitled: SH, AJ, and ES) sampled in a 1-year interval (2010–2011) to verify whether these practices were sustainable. Different harvesting scenarios were simulated: (1) no harvesting; (2) harvesting of palm heart of reproductive individuals; (3) harvesting of palm heart of large individuals (diameter at ground level > 65 mm, including reproductive or not). and (4) fruit harvesting. Each scenario was simulated at different harvesting intensities (percentage of individuals or fruits harvested). Integral projection models were used to calculate two demographic parameters, namely, population growth rate (λ) and elasticity. In the no harvesting scenario, the populations had λ > 1 (SH = 1.0655, AJ = 1.0184, and ES = 1.0862). Palm heart harvesting proved to be sustainable in both scenarios, but at a higher intensity in scenario (2) (SH = 83%, AJ = 14%, and ES = 35%) than in scenario (3) (SH = 17%, AJ = 4%, and ES = 16%). Fruit harvesting was sustainable at any intensity for all three populations. As the survival of large individuals has a high impact on λ, palm heart harvesting was in most cases sustainable only at low intensities. In contrast, as fecundity and seedling survival have a low impact on λ, fruit harvesting still proved sustainable at high intensities. Although the populations are tolerant to harvesting to some degree, it must be conducted carefully. As populations are fragile due to the current condition of reduced population size, the removal of palms at any rate can affect population persistence and generate possible cascade effects on the forest.
Introduction
Several ecosystems around the world have been impacted by anthropogenic actions (Vitousek et al., 1997; Lindenmayer et al., 2017). One of the main causes is deforestation in order to clear areas for agriculture/pasture fields, urban expansion, or selective exploitation of species of commercial interest (Laurance et al., 2014; Potapov et al., 2017). Largely exploited nowadays, non-timber forest products (NTFP) are biological resources (e.g., fruits, leaves, and bark), with the exception of wood, extracted from nature for human use (De Beer and McDermott, 1989). Such resources are used as a food source, for medicine, craftwork, or construction materials, and contribute to income generation (Hall and Bawa, 1993; Iqbal, 1993). Unfortunately, the impact caused by the harvesting of NTFP on populations is commonly unknown, often resulting in overharvesting and unsustainable resource use (Sutherland, 2001; Fernandez et al., 2012).
Harvesting of individuals or parts of plants can generate demographic changes because they interfere with vital rates (i.e., survival, regression, growth, and reproduction) that may alter the population growth rate (λ) (Ticktin, 2004; Schmidt et al., 2011). As a consequence, harvested populations start to respond differently, e.g., reducing fruit production or the number of leaves, seedling recruitment, or changing population structure (Peres et al., 2003; Endress et al., 2004), which may generate cascade effects on the entire community (Moegenburg and Levey, 2003; Fernandez et al., 2012; Muler et al., 2014). Moreover, in the context of deforestation and fragmentation due to anthropogenic actions, populations of forest species may undergo changes in population structure or in demographic parameters (Portela et al., 2010; Melito et al., 2014; Souza and Prevedello, 2020). Previous studies have shown that the population growth rates of forest-dependent species are reduced in fragmented sites, which also reduces sustainable harvesting rates (Escalante et al., 2004; Zambrano and Salguero-Gómez, 2014). Thus, it is important to increase the existing knowledge on populations of species that occur in highly disturbed areas to develop sustainable harvesting strategies.
Several studies have used demographic analysis to assess the effects of harvesting on population growth rate and to propose sustainable management strategies for NTFP exploitation (Ticktin, 2004). Most of the studies focus on palm species, followed by trees and herbs (Schmidt et al., 2011). Different management methods have been evaluated, including harvesting time, intensity, frequency, life form, and plant part harvested (Ticktin et al., 2002; Endress et al., 2006; Vallejo et al., 2014). As the harvesting of herbs commonly leads to mortality, influences on demographic parameters are negative and stronger (see Ghimire et al., 2008; Torres-García et al., 2020), while leaf and fruit harvesting on palm and tree species, which do not cause the death of individuals tend to have weaker effects on population demography and be sustainable (see Zuidema and Boot, 2002; Zuidema et al., 2007; Sampaio et al., 2008). In general, harvesting has been shown to negatively affect vital rates and population growth rates (Schmidt et al., 2011). Isaza et al. (2017) found that destructive and nondestructive fruit harvesting methods (e.g., cutting adults vs. climbing, respectively) influence the decrease in population growth rate and density of mature individuals of Euterpe precatoria and Mauritia flexuosa, two Amazonian palm species. The destructive method leading to plant death was unsustainable for both species, even at low harvesting rates and any frequency, while the nondestructive method was sustainable at almost all levels of intensity and frequency. Also, Gaoue and Ticktin (2010) found that the environmental conditions (e.g., temperature and moisture) of harvesting areas must be considered when proposing sustainable harvesting strategies. Values of λ in populations of Khaya senegalensis changed according to site seasonality after bark and leaf harvesting, which led to changes in management strategies proposed for each population.
Global human population growth and income generation needs have created an exacerbated pressure on natural resources exploitation, mainly in tropical regions (Peres, 2010). The Atlantic Rainforest in Brazil is a highly biodiverse tropical forest threatened by human activities. Due to deforestation for crops and urban expansion, only ~28% of the native vegetation currently remains (Rezende et al., 2018), 80% of which is represented by small, fragmented areas (<50 ha) at high levels of isolation (>1,440 m from other fragments) (Ribeiro et al., 2009). The small cover of protected areas (30% of remaining forests) combined with the high richness of species of commercial interest attracts illegal hunters and harvesters who often overexploit available resources (Fernandez et al., 2012; Rezende et al., 2018). In addition, the heterogeneity of habitats in the biome leads to differentiations between species populations (e.g., different population structures and reproductive phenology, see Melito et al., 2014 and Portela et al., 2020), which makes it difficult to define management rules for a species at the biome level. The Atlantic Forest is therefore a study area where understanding the limits of natural resource exploitation is crucial, especially in fragmented areas, in order to propose viable strategies for species protection and use.
A well-known species used as NTFP in the Atlantic Rainforest is Euterpe edulis Mart. (Arecaceae) a solitary palm, known as palmito-juçara. It is much appreciated in Brazilian gastronomy, both for the fruits, which are usually harvested in nondestructive ways and especially for the palm heart, considered a delicacy, although harvesting kills the palm. For this reason, some E. edulis plantations have been established to supply commercial demand, but illegal harvesting in natural forest remnants is still a common practice as an income-generating alternative and food resource (Galetti and Fernandez, 1998; Souza et al., 2022). Freckleton et al. (2003) simulated different harvesting scenarios for E. edulis populations from a large and well-conserved forest fragment of semideciduous forests in the Atlantic Rainforest. The simulation results showed that if only mature individuals were harvested, the population tolerated a high level of exploitation before it became unsustainable (λ < 1). In a more realistic scenario simulation, which included immature individuals, the level of harvesting tolerated in a scenario of sustainability was reduced to a much lower level. Some places in Brazil have created management guidelines for palm heart harvesting in Atlantic Forest remnants (e.g., states of Paraná, Santa Catarina, and São Paulo; see Reis et al., 2000). However, information on sustainable management in other states in the biome is still unavailable, especially in highly fragmented regions, which hinders the development of strategies that benefit people as well as plant populations.
The aim of this study was to simulate the response of three E. edulis populations in small forest fragments to different simulation scenarios of harvesting and intensities. The following questions were asked: (1) What is the population growth rate of each population and what vital rates have the highest effect on λ? (2) How would different harvesting intensities of mature individuals (those with reproductive structures) influence the population growth rate? (3) How would different harvesting intensities of larger individuals (>65 mm of diameter at ground level) influence the population growth rate? (4) How would different fruit harvesting intensities influence the population growth rate? (5) What recommendations can we provide on sustainable palm management in fragmented areas? Based on Freckleton et al. (2003), we expected all three populations to tolerate low levels of palm heart harvesting. Only the harvesting scenario of mature individuals was expected to tolerate higher levels of exploitation than the scenario that also included larger individuals. Because fruit collection uses nondestructive methods, we expected populations to tolerate high harvesting levels.
Materials and methods
Study sites
The study was carried out in three small fragments of lowland Atlantic Forest located in Rio de Janeiro state, southeastern Brazil, locally known as Santa Helena (SH; 57 ha), Estreito (ES; 21 ha), and Afetiva-Jorge (AJ; 19 ha). The fragments are located on private properties in a sustainable use protected area (IUCN category V), known as Área de Proteção Ambiental da Bacia do Rio São João/Mico-Leão-Dourado (APA-SJ; Figure 1). The three fragments are mainly surrounded by pasture and agricultural fields (Project MapBiomas, 2018; Souza et al., 2020; collection 4.0). The climate in the region is classified as Walter and Lieth's equatorial type (Walter, 1971), with a 900-mm mean annual rainfall, varying with the locality (Portela et al., 2020). The forest fragments in the APA-SJ region support a high diversity of plant species, with a dominance of Fabaceae, Euphorbiaceae, Rubiaceae, Lauraceae, Moraceae, Myrtaceae, Annonaceae, and Sapotaceae taxa (Carvalho et al., 2008).
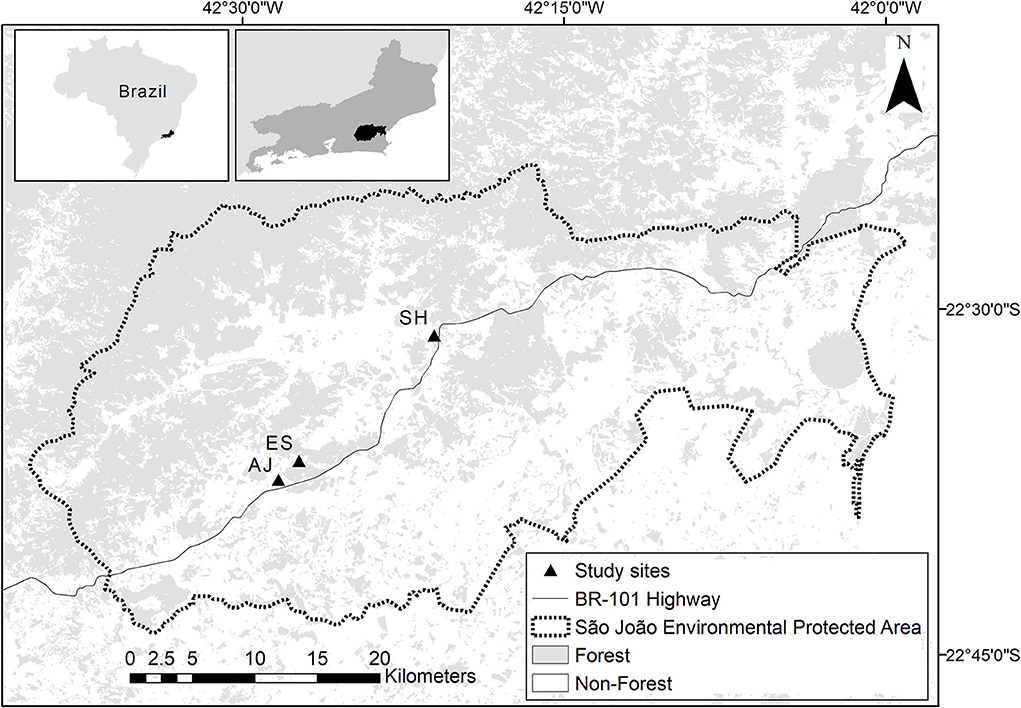
Figure 1. Limits of the São João Environmental Protected Area, located in the central portion of the state of Rio de Janeiro (southeastern region of Brazil), with forest and non-forest areas and the respective locations of the three study sites, i.e., SH, AJ, and ES (the western portion of the protected area). The limits of biological reserves are represented by dashed lines.
Focus species
The focus species of our study was E. edulis Mart. (Arecaceae), which has a large native range throughout the Atlantic Forest and in some regions of the Brazilian Cerrado, growing from sea level up to 1,000 m (Henderson et al., 1995). Euterpe edulis is a monoecious, single-stemmed, shade-tolerant palm. Slow-growing, mature individuals can reach 20 m in height and 15 cm in diameter at ground level (DGL). Each mature individual can produce 1,500 fruits per year (Matos and Watkinson, 1998), which are consumed by several birds and mammals that function as seed dispersers. For this reason, the species is considered a key resource for the Atlantic Forest fauna (Mantovani and Morellato, 2000; Galetti et al., 2013). Intensive harvesting of palm heart for commercial use has reduced the size of populations in nature, in some regions to the point of endangerment (Martinelli and Moraes, 2013).
Data collection
The three populations were sampled in the 2010–2011 period. In each fragment, nine 30 × 30 m plots were set up and distributed systematically in three blocks. Each block contained three sampling plots 50 m apart and was separated by a distance of 100 m from the next block. All plots were at least 50 m away from the fragment edge. A total of 0.81 ha was censused in each fragment. All E. edulis individuals were marked with numbered aluminum tags, including new individuals (recruitments) in 2011. We monitored the tagged palms, measured DGL using a digital caliper, and verified the presence or absence of reproductive structures (flowers or fruits).
Data analysis
To verify the population dynamics of E. edulis subject to and not subject to harvesting, we used a deterministic Integral Projection Model (IPM) analysis (Easterling et al., 2000). Using IPM, we calculated the finite λ, the dominant eigenvalue of the three populations for each sampling period, considering demographic parameters (survival, growth, regression, and fecundity). This method is similar to the matrix population model (Caswell, 2000), which uses a continuous variable instead of categorizing individuals into discrete stage classes (Easterling et al., 2000). This model describes the dynamics of a population, considering population size structure in different periods, according to the equation:
The above equation describes how a population with a given size structure at time one of an evaluation n(x,t) will have changed its size structure at time two, n(y, t + 1), based on survival, growth, regression, and contribution of recruits throughout the period. K is the kernel k(y, x) that represents all possible size transitions in the individual sizes at time t, represented as x, and at time t + 1, represented as y, with the range of possible sizes of all individuals, from L to U, and n is the distribution vector of the population. To estimate the kernel, it is necessary to calculate survival and growth rates p(x, y) (P), as well as fecundity f (x, y) (F) for the period considered (Easterling et al., 2000). Survival transition is estimated by evaluating the size of each individual at time t and after checking if the respective individual survived or not at time t + 1; if it did, growth can be evaluated by measuring plant size. Fecundity can be evaluated by checking the ratio between the number of new individuals (offspring) in time t + 1 and the number of mature individuals in time t (Easterling et al., 2000; Ellner and Rees, 2006). The model was selected based on the Akaike Information Criterion (AIC), relating growth and survival as a function of plant size (independent of size, dependent on size, size2, and size3). This model was used to select the function that provided the best fit to the data. For growth and survival, four different functions, namely, vital rate ~ 1, vital rate ~ size, vital rate ~ size + size2, and vital rate ~ size + size2 + size3, were tested. The model with the lowest AIC score, i.e., the simplest model, was selected using ΔAIC ≤ 2 (Supplementary Table S1). By performing an integral transformation of the projection kernel, we can determine λ for the given period. If λ was equal to 1, we considered the population to be stable during the period; if λ was lower than 1, the population was considered to be decreasing; and if λ was higher than 1, the population was considered to be increasing. We also performed an elasticity analysis, and a perturbation analysis to verify how changes in one vital rate affect λ (De Kroon et al., 1986). All data analyses were performed using the R software (R Development Core Team, 2019) using the packages such as IPMPack (Metcalf et al., 2013), gamm4 (Wood et al., 2017), popbio (Stubben and Milligan, 2007), and fields (Nychka et al., 2015).
Harvesting simulations
Different harvesting scenarios at different intensities were considered, all based on one single harvesting event in the period considered: (1) no harvesting (control); (2) palm heart harvesting of mature individuals (bearing reproductive structures); (3) palm heart harvesting of individuals with DGL > 65 mm (i.e., reproductive and pre-reproductive individuals); and (4) fruit harvesting. In scenarios (2) and (3), we simulated harvesting to occur after the reproductive season, which means that fecundity did not change during the analysis. In scenario (3), fecundity changed according to the intensity of harvesting simulated. It was calculated using the following equation:
In the above equation, h is the harvesting intensity used in the simulation, and f is fecundity in the absence of harvesting. We programmed the analysis to substitute f with the new value for all reproductive individuals in the census. Harvesting intensities varying from 0 (control) to 100% of the individuals harvested were simulated for scenarios (2) and (3), with 10% intervals. Harvesting intensities were simulated varying from 0 to 95% for fruit harvesting because the analysis requires at least two recruits and the possibility that fruits may be dispersed from nearby areas has to be considered, which prevented us from considering a 100% harvest.
Another calculation was made for the fruit harvesting scenario. We simulated fruit harvesting based on the seedling establishment observed in the period. According to Matos and Watkinson (1998), the germination rate for E. edulis is ~60% of the total fruits produced, and each mature individual produces ~1,500 fruits/year. Therefore, sustainable fruit harvesting was calculated using the following equation:
In the above equation, s is the harvesting intensity when λ = 1 (calculated previously, from 0 to 95%); g is the germination rate (here, 0.6 or 60%); and n is the number of fruits produced by a single mature individual per year (here, 1,500 fruits/year). The result indicates the total number of fruits that can be harvested per mature individual per year.
Population growth rates were calculated for each of the harvesting scenarios by bootstraps, with 2,000 repetitions. In each resample, individuals were removed at random, according to the harvesting scenario and intensity simulated. Confidence intervals were extracted using the mean of these λ values with 95% confidence. We considered the harvesting scenarios and intensities whose λ values were not significantly different from 1 or greater as sustainable because it is the maximum value tolerated by the population before harvesting leads to population decline.
Results
In 2010, the total density of individuals was higher in ES (523.45 ind/ha), followed by SH (181.48 ind/ha) and AJ (159.25 ind/ha). The density of mature individuals was higher in AJ (16.04 ind/ha), followed by SH (13.58 ind/ha) and ES (7.40 ind/ha). Despite the lower density of mature individuals in ES, the population had the highest density of recruits (new seedlings) in 2011 (59.25 ind/ha), followed by AJ (18.51 ind/ha) and SH (12.34 ind/ha).
The λ for no harvesting (control) was higher than 1 for all populations, indicating an increase in population size in the period of our study. The highest λ was found in ES [λ = 1.0862 (C.I. = 1.0809; 1.0911)], followed by SH [1.0655 (1.0595; 1.0715)] and AJ [1.0184 (1.0150; 1.0218)] (Figure 2). The elasticity analysis showed a tendency that larger palms have a stronger influence on λ values. In AJ, individuals > 90 mm DGL had the strongest influence, as well as all individuals > 65 mm DGL in SH and ES (Figure 2).
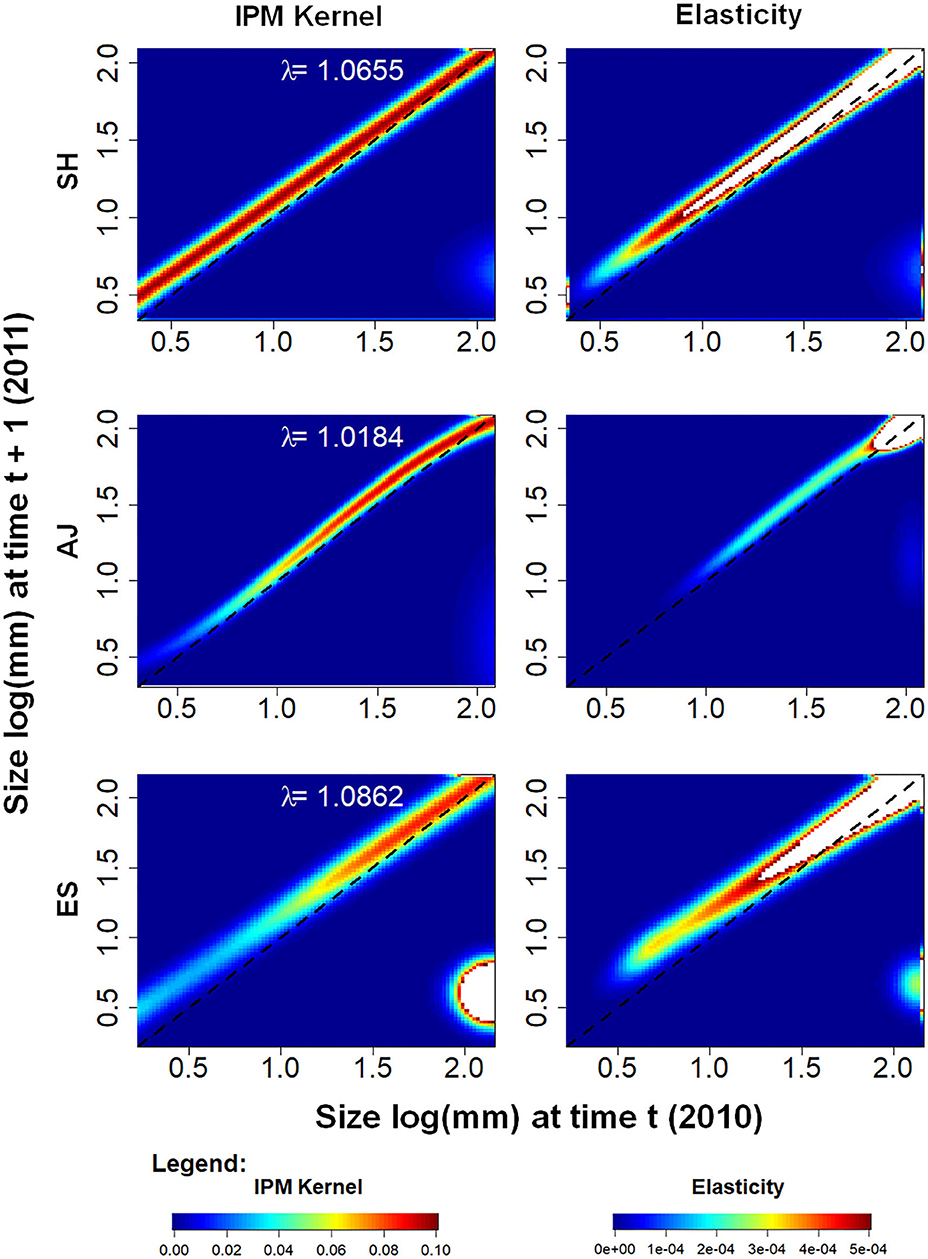
Figure 2. Euterpe edulis demographic analysis was performed with integral projection models (IPM) for a 1-year interval (2010–2011) in three different populations (SH, AJ, and ES) located in the Silva Jardim municipality, Rio de Janeiro state, Brazil. Left: images detailing the projection kernels, built from the P function (representing survival and growth, illustrated along the main diagonal of each image), and the F function (representing fecundity rate, illustrated on the lower right of each image). Right: images detailing elasticity kernels (illustrated along the main diagonal of each image). The dashed black line along the main diagonal is used as a reference for the probability of stasis. Individuals on the line did not change in size during the period of the study, while individuals above the line increased in size and individuals below the line decreased in size. Higher transition probabilities are represented by the warmest colors (including white). The x and y axes are on a logarithmic scale.
Palm heart harvesting of mature individuals (scenario 2) was considered sustainable at different harvesting intensities for each of the three populations assessed. At AJ, the sustainability of palm heart harvesting was assured only at low harvesting intensity—no more than 14% of the mature individuals could be harvested, while at ES, harvesting intensity could reach 35%. Finally, at SH, harvesting intensity could reach 83% (Figure 3A; Supplementary Table S2). In the scenario of palm heart harvesting of large individuals (scenario 3), harvesting was considered sustainable at AJ if at most 4% of the large individuals were harvested, while at ES, harvesting intensity could reach 16% and at SH, 17% (Figure 3B; Supplementary Table S2). Differently from palm heart harvesting, fruit harvesting (scenario 4) was sustainable in the three populations at all the harvesting intensities simulated (Figure 3C; Supplementary Table S2). For this scenario, even at the intensity of 95% harvesting, λ = 1.0245 (1.0209; 1.0281) at SH, λ = 1.0117 (1.0085; 1.0135) at ES, and λ = 1.0027 (1.0008; 1.0054) at AJ, a value close to the limit of sustainable harvesting.
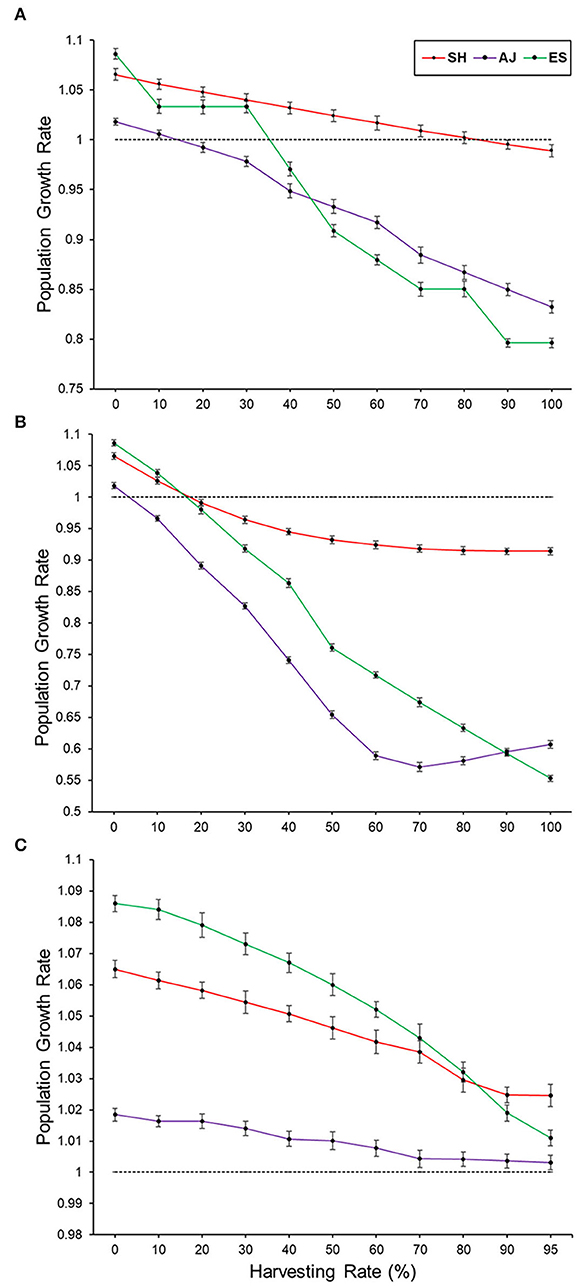
Figure 3. Relationship between different harvesting rates and population growth rates in different harvesting scenarios for three different populations. (A) Simulations for mature individuals harvesting only (scenario 2); (B) simulations for larger individuals (DGL > 65 mm; scenario 3); (C) simulations for fruit harvesting (scenario 4; see the “Harvesting simulations” subsection on how to calculate fruit harvesting limits). The dashed horizontal black line in axis y = 1 represents the threshold at which harvesting is sustainable (λ ≥ 1) or unsustainable (λ < 1).
Discussion
Our simulations on reduced E. edulis populations in very small forest fragments showed that palm heart harvesting requires more careful management than fruit harvesting. The three E. edulis populations tolerated higher intensities of palm heart harvesting of mature individuals than of large individuals (>65 mm of DGL), which included pre-reproductive individuals (immatures). This result is similar to what Freckleton et al. (2003) simulated for E. edulis populations in a large Atlantic Forest fragment in the state of São Paulo. Freckleton et al. (2003) found that E. edulis populations tolerated harvesting of all mature individuals without reaching the point of collapse, but if harvesting also included the pre-reproductive life stage, <10% of the individuals in these two stages could be harvested sustainably. Palm heart harvesting of large individuals, including mature and pre-reproductive plants, is probably more realistic in practice. However, high palm heart harvesting intensities of pre-reproductive individuals can compromise the number of mature individuals and, thus, seedling recruitment (Freckleton et al., 2003). Therefore, we propose that conservation strategies for E. edulis must include scenarios in which all large individuals, not only reproductive individuals, are considered for palm heart exploitation.
In ES and SH, we found that palm heart harvesting of reproductive individuals (35 and 83% harvesting, respectively) could be sustainable at high levels. However, when non-reproductive individuals are also harvested, the harvesting level must be reduced in order to remain ecologically sustainable (16 and 17%, respectively). This can be explained by the elasticity rate, which showed a high influence of large individuals (>65 mm of DGL), including immature and mature individuals, on λ. In AJ, palm heart harvesting was sustainable at a lower level of harvesting (14% when only mature individuals were cut and 4% when large individuals were cut). This can be explained by the high elasticity values of individuals > 90 mm of DGL, a size mostly represented by mature individuals.
In terms of fruit harvesting, we showed that the three populations could be exploited at high intensities without compromising the recruitment of new individuals in the short-term. Only ES had values close to one at high intensities (95%), still with λ > 1. Additionally, elasticity values for fecundity and seedling survival were the lowest in the study. Therefore, these values indicate that intense fruit harvesting is viable with insignificant changes on λ. This situation is similarly described in other studies on different palm species, with the harvesting of structures (e.g., fruits and leaves) usually being sustainable because it does not necessarily lead to plant death (Mendoza et al., 1987; Zuidema et al., 2007), although it may reduce the individuals growth (Sampaio et al., 2008). As suggested by Schmidt et al. (2011), studies on sustainable harvesting must observe and consider not only oscillations in population growth rate but also different parameters of influence such as elasticity, vital rate, and life table response experiment (LTRE). By analyzing how population growth rate and elasticity values are impacted by different harvesting scenarios, we have been able to understand how E. edulis populations in small forest fragments can be harvested sustainably.
The Atlantic Rainforest is formed by heterogeneous landscapes throughout the biome, represented by different vegetation types (Veloso et al., 1991), continuous forest cover, and forest fragments (Ribeiro et al., 2009). Thus, species populations from different areas can undergo changes, so possible adaptations in the sustainable harvesting strategies proposed may be necessary. Melito et al. (2014) showed that the density of E. edulis populations can vary in different types of forest, in remnants undergoing fragmentation, or still in areas with different levels of protection. A similar pattern was observed by Gaoue and Ticktin (2010) in Benin forests, where spatial differences between areas along with changes in temperature and moisture resulted in different strategies for the sustainable harvesting of Khaya senegalensis bark and leaves. Also, Portela et al. (2020) showed that phenological aspects (i.e., flowering and fruiting seasons), as well as seed size of E. edulis, change depending on forest altitudes and habitat type. In contrast to the study by Freckleton et al. (2003), conducted in a larger forest fragment with a much higher density of individuals, in our study, we evaluated harvesting in three small forest fragments at reduced population densities, which reflects the main condition of the Atlantic Forest. We have shown that even though palm heart and fruits can be harvested at a given level in these small areas, harvesting requires caution. We have carefully hypothesized the germination rate and seedling recruitment of E. edulis using data from populations in different ecological conditions (Matos and Watkinson, 1998), as the species has a broad seed germination niche (Braz et al., 2014). Also, the low number of larger and mature individuals in each area along with the respective high elasticity values show that palm heart harvesting must be conducted carefully in order to avoid impact on population survival.
In this study, we simulated fruit and palm heart harvesting using three different scenarios in 1 year of sampling, considering different harvesting intensities after the reproductive period. Some studies have shown that the evaluation of different harvesting techniques can help to improve them. These include harvesting time, season, frequency, a minimum size of individuals harvested, and artificial insertion of seedlings into the population (Ticktin, 2004; Endress et al., 2006; Torres-García et al., 2020). Freckleton et al. (2003) simulated E. edulis harvesting during the pre-reproductive and post-reproductive seasons and found that the populations tolerated higher levels of palm heart harvesting if it was conducted after the reproductive period, not previously. These results are justified by the production of new seedlings by adults, a situation that would not be viable if palms or fruits were harvested in the pre-reproductive period. In a similar perspective, Escalante et al. (2004) found that the palm Desmoncus orthacanthos tolerated 20–40% of adult harvesting depending on the locality. However, if an artificial insertion of juveniles was made into the population, the sustainable harvesting rate would increase by ~40%. As shown, several harvesting and management techniques are available but, depending on the species, some may produce more severe demographic effects than others. Thus, knowing the biology and ecology of the target species well is essential for the formulation of conservation strategies that focus mainly on the technique of greatest impact.
The three populations assessed showed demographic persistence in the period of our study and some tolerance to palm heart and fruit harvesting. However, extraction of NTFP can have consequences not only at the population level but also at the community and ecosystem levels (Ticktin, 2004). Euterpe edulis plays a keystone role for several vertebrates, especially birds and mammals, providing a food resource in fruit scarcity periods (Fadini et al., 2009). The possible decrease in fruit provision to such animals due to fruit harvesting or the death of large individuals due to palm heart extraction may trigger changes in community functioning and the overall forest structure, including cascading effects (Barlow et al., 2006; Fernandez et al., 2012; Muler et al., 2014). Some studies have shown that harvesting of palm heart and fruits from palm species, including E. edulis, is associated with a reduction in the occurrence, richness, and permanence time of seed-dispersing birds in impacted locations (Galetti and Aleixo, 1998; Moegenburg and Levey, 2003; Muler et al., 2014; Silva and Reis, 2019). As several bird species are also seed dispersers of other late-successional plant species, forest regeneration can be compromised (Rother et al., 2016). Moreover, as E. edulis is one of the most abundant species in the Atlantic Rainforest (Fantini and Guries, 2007), the harvesting of large individuals may also increase the incidence of light in the forest, facilitating seed germination of early successional species (Muler et al., 2014). Thus, despite the tolerance to harvesting found in our simulations, E. edulis populations should be managed carefully to avoid possible direct and indirect effects on the community and ecosystem.
Strategies for sustainable harvesting of NTFP should be planned, aiming not only to ensure the demographic persistence of populations and to avoid indirect effects but also to cater to human needs. Apart from the already mentioned conservation actions, the direct participation and dialogue with harvesters in the development of harvesting strategies have proven to be an important tool for increasing acceptance by people and improving existing rules (Ticktin et al., 2002). Currently, it is estimated that around 50,000 species of plants and animals are used for human consumption (IPBES, 2022). Several are exploited in an unsustainable way, which generates effects not only on populations but also on other species in the community (Fernandez et al., 2012). Although the populations of E. edulis tolerated some harvesting levels, due to the extremely low density of individuals, possible reduced occurrence of seed dispersers, and exacerbated fragmentation in the lowland Atlantic Rainforest, we recommend harvesting be conducted carefully to ensure population persistence. Furthermore, more studies are necessary on different forest types in the Atlantic Rainforest to increase the understanding of demographic issues and improve local management.
Data availability statement
The raw data supporting the conclusions of this article will be made available by the authors, without undue reservation.
Author contributions
EM and RP collected the data. EM, FG, and RP analyzed the data and wrote the manuscript. All authors contributed to the article and approved the submitted version.
Funding
Financial support was provided by FAPESP (Proc. No. 2005/60788-4) and CAPES fellowship to RP and EM (Proc. No. 88882.424935/2019-01), FAPERJ, CNPQ Universal.
Acknowledgments
We thank Eraldo dos Santos Almeida, Antonio Tavares de Oliveira, and Adilson Pintor for their indispensable help in the field. We are grateful to ICMBio for permission to work in their protected areas.
Conflict of interest
The authors declare that the research was conducted in the absence of any commercial or financial relationships that could be construed as a potential conflict of interest.
Publisher's note
All claims expressed in this article are solely those of the authors and do not necessarily represent those of their affiliated organizations, or those of the publisher, the editors and the reviewers. Any product that may be evaluated in this article, or claim that may be made by its manufacturer, is not guaranteed or endorsed by the publisher.
Supplementary Material
The Supplementary Material for this article can be found online at: https://www.frontiersin.org/articles/10.3389/ffgc.2022.932454/full#supplementary-material
References
Barlow, J., Peres, C. A., Henriques, L. M. P., Stouffer, P. C., and Wunderle, J. M. (2006). The responses of understorey birds to forest fragmentation, logging and wildfires: an Amazonian synthesis. Biol. Conserv. 128, 182–192. doi: 10.1016/j.biocon.2005.09.028
Braz, M. I. G., Portela, R. D. C. Q., Cosme, L. H. M., Marques, V. G. C., and de Mattos, E. A. (2014). Germination niche breadth differs in two co-occurring palms of the Atlantic Rainforest. Natureza e Conservação 12, 124–128. doi: 10.1016/j.ncon.2014.09.003
Carvalho, F. A., Nascimento, M. T., and Oliveira Filho, A. T. (2008). Composição, riqueza e heterogeneidade da flora arbórea da bacia do rio São João, RJ, Brasil. Acta Botanica Brasilica 22, 929–940. doi: 10.1590/S0102-33062008000400004
De Beer, J. H., and McDermott, M. (1989). The Economic Value of Non-timber Forest Products in Southeast Asia with Emphasis on Indonesia, Malaysia and Thailand. Amsterdam: Netherlands Committee for IUCN.
De Kroon, H., Plaisier, A., van Groenendael, J., and Caswell, H. (1986). Elasticity: the relative contribution of demographic parameters to population growth rate. Ecology 67, 1427–1431. doi: 10.2307/1938700
Easterling, M. R., Ellner, S. P., and Dixon, P. M. (2000). Size-specific sensitivity: applying a new structured population model. Ecology 81, 694–708. doi: 10.1890/0012-9658(2000)081[0694:SSSAAN]2.0.CO;2
Ellner, S. P., and Rees, M. (2006). Integral projection models for species with complex demography. Am. Nat. 167, 410–428. doi: 10.1086/499438
Endress, B. A., Gorchov, D. L., and Berry, E. J. (2006). Sustainability of a non-timber forest product: effects of alternative leaf harvest practices over 6 years on yield and demography of the palm Chamaedorea radicalis. Forest Ecol. Manag. 234, 181–191. doi: 10.1016/j.foreco.2006.07.020
Endress, B. A., Gorchov, D. L., and Noble, R. B. (2004). Non-timber forest product extraction: effects of harvest and browsing on an understory palm. Ecol. Appl. 14, 1139–1153. doi: 10.1890/02-5365
Escalante, S., Montaña, C., and Orellana, R. (2004). Demography and potential extractive use of the liana palm, Desmoncus orthacanthos Martius (Arecaceae), in southern Quintana Roo, Mexico. Forest Ecol. Manag. 187, 3–18. doi: 10.1016/S0378-1127(03)00228-7
Fadini, R. F., Fleury, M., Donatti, C. I., and Galetti, M. (2009). Effects of frugivore impoverishment and seed predators on the recruitment of a keystone palm. Acta Oecol. 35, 188–196. doi: 10.1016/j.actao.2008.10.001
Fantini, A. C., and Guries, R. P. (2007). Forest structure and productivity of palmiteiro (Euterpe edulis Martius) in the Brazilian Mata Atlântica. Forest Ecol. Manag. 242, 185–194. doi: 10.1016/j.foreco.2007.01.005
Fernandez, F. A. S., Antunes, P. C., Macedo, L., and Zucco, C. A. (2012). How sustainable is the use of natural resources in Brazil? Natureza e Conservação 10, 77–82. doi: 10.4322/natcon.2012.013
Freckleton, R. P., Matos, D. S., Bovi, M. L. A., and Watkinson, A. R. (2003). Predicting the impacts of harvesting using structured population models: the importance of density- dependence and timing of harvest for a tropical palm tree. J. Appl. Ecol. 40, 846–858. doi: 10.1046/j.1365-2664.2003.00842.x
Galetti, M., and Aleixo, A. (1998). Effects of palm heart harvesting on avian frugivores in the Atlantic rain forest of Brazil. J. Appl. Ecol. 35, 286–293. doi: 10.1046/j.1365-2664.1998.00294.x
Galetti, M., and Fernandez, J. C. (1998). Palm heart harvesting in the Brazilian Atlantic forest: changes in industry structure and the illegal trade. J. Appl. Ecol. 35, 294–301. doi: 10.1046/j.1365-2664.1998.00295.x
Galetti, M., Guevara, R., Côrtes, M.C., Fadini, R., Von Matter, S., Leite, A.B., et al. (2013). Functional extinction of birds drives rapid evolutionary changes in seed size. Science 340, 1086–1090. doi: 10.1126/science.1233774
Gaoue, O. G., and Ticktin, T. (2010). Effects of harvest of nontimber forest products and ecological differences between sites on the demography of African mahogany. Conserv. Biol. 24, 605–614. doi: 10.1111/j.1523-1739.2009.01345.x
Ghimire, S. K., Gimenez, O., Pradel, R., McKey, D., and Aumeeruddy-Thomas, Y. (2008). Demographic variation and population viability in a threatened Himalayan medicinal and aromatic herb Nardostachys grandiflora: matrix modelling of harvesting effects in two contrasting habitats. J. Appl. Ecol. 45, 41–51. doi: 10.1111/j.1365-2664.2007.01375.x
Hall, P., and Bawa, K. (1993). Methods to assess the impact of extraction of nontimber tropical forest products on plant populations. Econ. Bot. 47, 234–247. doi: 10.1007/BF02862289
Henderson, A., Galeano, G., and Bernal, R. (1995). Field guide to the palms of the Americas. NJ: Princeton University Press. p. 502.
IPBES. (2022). Summary for Policymakers of the Thematic Assessment of the Sustainable Use of Wild Species of the Intergovernmental Science-Policy Platform on Biodiversity and Ecosystem Services, eds J. M. Fromentin, M. R. Emery, J. Donaldson, M. C. Danner, A. Hallosserie, D. Kieling, G. Balachander, E. S. Barron, R. P. Chaudhary, M. Gasalla, M. Halmy, C. Hicks, M. S. Park, B. Parlee, J. Rice, T. Ticktin, and D. Tittensor (Bonn: IPBES secretariat). p. 33.
Iqbal, M. (1993). International Trade in Non-Wood Forest Products. An Overview. Rome: Food and Agriculture Organization.
Isaza, C., Bernal, R., Galeano, G., and Martorell, C. (2017). Demography of Euterpe precatoria and Mauritia flexuosa in the Amazon: application of integral projection models for their harvest. Biotropica 49, 653–664. doi: 10.1111/btp.12424
Laurance, W. F., Sayer, J., and Cassman, K. G. (2014). Agricultural expansion and its impacts on tropical nature. Trends Ecol. Evol. 29, 107–116. doi: 10.1016/j.tree.2013.12.001
Lindenmayer, D., Thorn, S., and Banks, S. (2017). Please do not disturb ecosystems further. Nat. Ecol. Evol. 1, 1–3. doi: 10.1038/s41559-016-0031
Mantovani, A., and Morellato, L. P. C. (2000). “Fenologia da floração, frutificação, mudan?a foliar e aspectos da biologia floral do palmiteiro," in Euterpe edulis Martius (palmiteiro): biologia, conservação e manejo. Herbario Barbosa Rodrigues, eds M. S. Reis and A. Reis (Italai), 23–38.
Martinelli, G., and Moraes, M. (2013). Livro Vermelho da Flora do Brasil (Red Book of Brazilian Flora). Rio de Janeiro: Jardim Botânico do Rio de Janeiro and Andrea Jakobson Estúdio, 1100.
Matos, D. M. S., and Watkinson, A. R. (1998). The fecundity, seed, and seedling ecology of the edible palm Euterpe edulis in southeastern Brazil. Biotropica 30, 595–603. doi: 10.1111/j.1744-7429.1998.tb00099.x
Melito, M. O., Faria, J. C., Amorim, A. M., and Cazetta, E. (2014). Demographic structure of a threatened palm (Euterpe edulis Mart.) in a fragmented landscape of Atlantic Forest in northeastern Brazil. Acta Botanica Brasilica 28, 249–258. doi: 10.1590/S0102-33062014000200011
Mendoza, A., Pinero, D., and Sarukhán, J. (1987). Effects of experimental defoliation on growth, reproduction and survival of Astrocaryum mexicanum. J. Ecol. 75, 545–554. doi: 10.2307/2260433
Metcalf, C. J. E., McMahon, S. M., Salguero-Gómez, R., and Jongejans, E. (2013). IPMpack: a R package for integral projection models. Methods Ecol. Evol. 4, 195–200. doi: 10.1111/2041-210x.12001
Moegenburg, S. M., and Levey, D. J. (2003). Do frugivores respond to fruit harvest? An experimental study of short-term responses. Ecology 84, 2600–2612. doi: 10.1890/02-0063
Muler, A., Rother, D., Brancalion, P., Naves, R. P., Rodrigues, R. R., and Pizo, M. A. (2014). Can overharvesting of a non-timber forest-product change the regeneration dynamics of a tropical rainforest? The case study of Euterpe edulis. For. Ecol. Manag. 324, 117–125. doi: 10.1016/j.foreco.2013.09.001
Nychka, D., Furrer, R., Paige, J., Sain, S., and Nychka, M. D. (2015). Package ‘fields'. Vienna: R Foundation for Statistical Computing.
Peres, C.A. (2010). “Overexploitation,” in Conservation Biology for All, eds N. J. Sodhi and P. R. Ehrlich (Oxford: Oxford University Press), 107–130.
Peres, C.A., Baider, C., Zuidema, P.A., Wadt, L.H.O., Kainer, K.A., Gomes-Silva, D.A.P., et al. (2003). Demographic threats to the sustainability of Brazil nut exploitation. Science 302, 2112–2114. doi: 10.1126/science.1091698
Portela, R., Silva, V., Mendes, E., Lima, T., Braz, M., Pintor, A., et al. (2020). Differential phenological shifts in Euterpe edulis Mart. During an extremely dry year along an altitudinal gradient. Oecol. Austr. 24, 389–405. doi: 10.4257/oeco.2020.2402.11
Portela, R. C. Q., Bruna, E. M., and Santos, F. A. M. (2010). Demography of palm species in Brazil's Atlantic forest: a comparison of harvested and unharvested species using matrix models. Biodiv. Conserv. 19, 2389–2403. doi: 10.1007/s10531-010-9846-5
Potapov, P., Hansen, M. C., Laestadius, L., Turubanova, S., Yaroshenko, A., Thies, C., et al. (2017). The last frontiers of wilderness: tracking loss of intact forest landscapes from 2000 to 2013. Sci. Adv. 3, e1600821. doi: 10.1126/sciadv.1600821
Project MapBiomas (2018). Coleção 4.1 da Série Anual de Mapas de Cobertura e Uso de Solo do Brasil. Rio de Janeiro: Project MapBiomas.
R Development Core Team (2019). R: A language and environment for statistical computing. Computer Software Manual, Vienna, Austria.
Reis, M. S., Fantini, A. C., Nodari, R. O., Reis, A., Guerra, M. P., and Mantovani, A. (2000). Management and conservation of natural populations in atlantic rain forest: the case study of palm heart (Euterpe edulis Martius). Biotropica 32, 894–902. doi: 10.1111/j.1744-7429.2000.tb00627.x
Rezende, C. L., Scarano, F. R., Assad, E. D., Joly, C. A., Metzger, J.P., Strassburg, B.N.B., et al. (2018). From hotspot to hopespot: an opportunity for the Brazilian Atlantic Forest. Perspect. Ecol. Conserv. 16, 208–214. doi: 10.1016/j.pecon.2018.10.002
Ribeiro, M. C., Metzger, J. P., Martensen, A. C., Ponzoni, F. J., and Hirota, M. M. (2009). The Brazilian Atlantic Forest: how much is left, and how is the remaining forest distributed? Implications for conservation. Biol. Conserv. 142, 1141–1153. doi: 10.1016/j.biocon.2009.02.021
Rother, D. C., Rodrigues, R. R., and Pizo, M. A. (2016). Bamboo thickets alter the demographic structure of Euterpe edulis population: a keystone, threatened palm species of the Atlantic forest. Acta Oecol. 70, 96–102. doi: 10.1016/j.actao.2015.11.008
Sampaio, M. B., Schmidt, I. B., and Figueiredo, I. B. (2008). Harvesting effects and population ecology of the buriti palm (Mauritia flexuosa L. f., Arecaceae) in the Jalapão region, Central Brazil. Econ. Bot. 62, 171. doi: 10.1007/s12231-008-9017-8
Schmidt, I. B., Mandle, L., Ticktin, T., and Gaoue, O. G. (2011). What do matrix population models reveal about the sustainability of non-timber forest product harvest? J. Appl. Ecol. 48, 815–826. doi: 10.1111/j.1365-2664.2011.01999.x
Silva, J. Z., and Reis, M. S. (2019). Consumption of Euterpe edulis fruit by wildlife: implications for conservation and management of the Southern Brazilian Atlantic Forest. Anais Acad. Brasil. Ciências 91, 537. doi: 10.1590/0001-3765201920180537
Souza, A. C., and Prevedello, J. A. (2020). The importance of protected areas for overexploited plants: evidence from a biodiversity hotspot. Biol. Conserv. 243, 108482. doi: 10.1016/j.biocon.2020.108482
Souza, A. C., Weber, M. M., and Prevedello, J. A. (2022). Protection status and density-dependent effects mediate the abundance-suitability relationship of a threatened species. Pers. Ecol. Conserv. 20, 168–176. doi: 10.1016/j.pecon.2022.03.002
Souza, C. M., Shimbo, J. Z., Rosa, M. R., Parente, L. L., Alencar, A. A., Rudorff, B. F. T., et al. (2020). Reconstructing three decades of land use and land cover changes in Brazilian biomes with landsat archive and earth engine. Remote Sens. 12, 2735. doi: 10.3390/rs12172735
Stubben, C., and Milligan, B. (2007). Estimating and analyzing demographic models using the popbio package in R. J. Statist. Softw. 22, 1–23. doi: 10.18637/jss.v022.i11
Sutherland, W. J. (2001). Sustainable exploitation: a review of principles and methods. Wildlife Biol. 7, 131–140. doi: 10.2981/wlb.2001.017
Ticktin, T. (2004). The ecological implications of harvesting non-timber forest products. J. Appl. Ecol. 41, 11–21. doi: 10.1111/j.1365-2664.2004.00859.x
Ticktin, T., Nantel, P., Ramirez, F., and Johns, T. (2002). Effects of variation on harvest limits for nontimber forest species in Mexico. Conserv. Biol. 16, 691–705. doi: 10.1046/j.1523-1739.2002.00372.x
Torres-García, I., León-Jacinto, A., Vega, E., Moreno-Calles, A. I., and Casas, A. (2020). Integral projection models and sustainable forest management of Agave inaequidens in Western Mexico. Front. Plant Sci. 11, 1224. doi: 10.3389/fpls.2020.01224
Vallejo, M. I., Galeano, G., Bernal, R., and Zuidema, P. A. (2014). The fate of populations of Euterpe oleracea harvested for palm heart in Colombia. Forest Ecol. Manag. 318, 274–284. doi: 10.1016/j.foreco.2014.01.028
Veloso, H. P., Rangel-Filho, A. L. R., and Lima, J. C. A. (1991). Classificação da vegetação brasileira, adaptada a um sistema universal. Rio de Janeiro: Ibge.
Vitousek, P. M., Mooney, H. A., Lubchenco, J., and Melillo, J. M. (1997). Human domination of Earth's ecosystems. Science 277, 494–499. doi: 10.1126/science.277.5325.494
Wood, S., Scheipl, F., and Wood, M. S. (2017). Package ‘gamm4'. Am. Statist. 45, 339. Available online at: https://cran.r-project.org/web/packages/gamm4/gamm4.pdf (Accessed August 05, 2022).
Zambrano, J., and Salguero-Gómez, R. (2014). Forest fragmentation alters the population dynamics of a late-successional tropical tree. Biotropica 46, 556–564. doi: 10.1111/btp.12144
Zuidema, P. A., and Boot, R. G. (2002). Demography of the Brazil nut tree (Bertholletia excelsa) in the Bolivian Amazon: impact of seed extraction on recruitment and population dynamics. J. Trop. Ecol. 18, 1–31. doi: 10.1017/S0266467402002018
Keywords: demography, Euterpe edulis, IPM, NTFP, sustainable harvest
Citation: Mendes E, Galdino F and Portela RdCQ (2022) Predicting the impacts of palm heart and fruit harvesting using Integral Projection Models. Front. For. Glob. Change 5:932454. doi: 10.3389/ffgc.2022.932454
Received: 29 April 2022; Accepted: 25 July 2022;
Published: 17 August 2022.
Edited by:
Eliane Ceccon, Universidad Nacional Autónoma de México, MexicoReviewed by:
Andressa Ribeiro, Federal University of Piauí, BrazilGerardo Avalos, University of Costa Rica, Costa Rica
Copyright © 2022 Mendes, Galdino and Portela. This is an open-access article distributed under the terms of the Creative Commons Attribution License (CC BY). The use, distribution or reproduction in other forums is permitted, provided the original author(s) and the copyright owner(s) are credited and that the original publication in this journal is cited, in accordance with accepted academic practice. No use, distribution or reproduction is permitted which does not comply with these terms.
*Correspondence: Eduardo Mendes, eduardotbm@yahoo.com