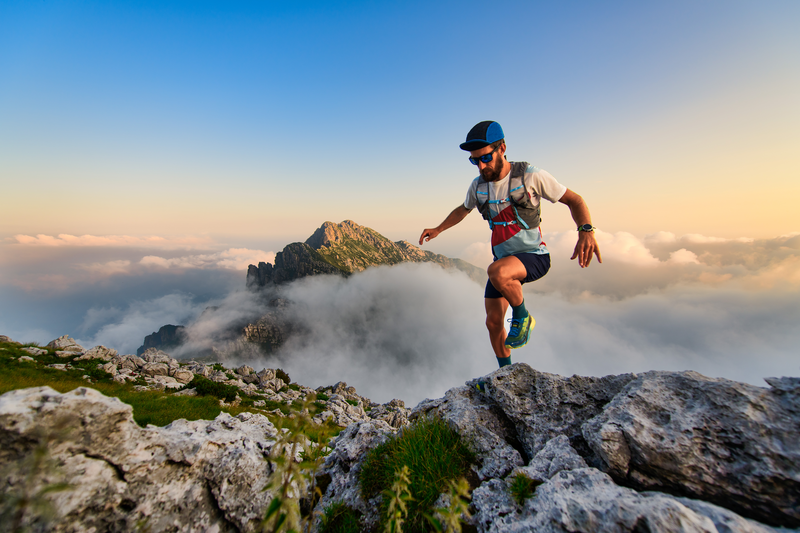
94% of researchers rate our articles as excellent or good
Learn more about the work of our research integrity team to safeguard the quality of each article we publish.
Find out more
ORIGINAL RESEARCH article
Front. For. Glob. Change , 08 July 2022
Sec. Tropical Forests
Volume 5 - 2022 | https://doi.org/10.3389/ffgc.2022.921028
This article is part of the Research Topic Monitoring and Predicting Bamboo Forest Dynamics Under a Changing Climate View all 4 articles
Moso bamboo (Phyllostachys edulis) is currently distributed across a wide geographical area in East Asia. As a common bamboo species occurring along a broad environmental gradient, there is a need to understand how environmental and biotic drivers affect belowground processes at large scales. In this study, we investigated the influence of climate, soil properties, stand characteristics, and organic matter input parameters as potential drivers of the initial decomposition process in Moso bamboo stands at a regional scale. Using the Tea Bag Index method, we estimated the initial decomposition rate (k) and stabilization factor (S; potential long-term carbon storage) from standard litter incubated at 13 sites across southern Japan and Taiwan. We found that both decomposition parameters were strongly affected by the climate. The climatic conditions during the incubation period better explained the variance in k. In contrast, the long-term climate was more important for S. Notably, temperature and precipitation interactively affected the initial decomposition rates. This interaction showed that in warmer sites, precipitation increased k, whereas in cooler sites, precipitation had no effect or even decreased k. Soil parameters had no influence on k and only had minor effects on S. A structural equation model showed that the stabilization factor was indirectly affected by stand density, which suggests that higher bamboo densities could increase litter stabilization by increasing above-and below-ground organic matter input. Our study highlights the central role of climate in controlling decomposition processes in Moso bamboo stands on a broad scale. Moreover, differences in stand structure can indirectly affect potential soil carbon storage through changes in organic matter input and soil conditions.
Moso bamboo [Phyllostachys edulis (Carrière) J. Houz, Poaceae] is one of the largest and most common bamboo species in East Asia. It was originally introduced from subtropical China to other regions, such as Japan, Korea, and Taiwan, for shoot and timber production (Canavan et al., 2017). Owing to their rapid growth and underground rhizome expansion, bamboo stands can rapidly displace the surrounding vegetation and progressively replace them with monospecific stands (Okutomi et al., 1996; Isagi and Torii, 1997). This species’ invasive characteristics and the abandonment of previously managed stands have contributed to the continuous expansion of unmanaged areas over the last 30 years (Shinohara et al., 2014; Suzuki, 2015). Bamboo-dominated areas are currently distributed over many geographical regions, and their expansion rates are expected to increase in the future (Shinohara et al., 2014; Takano et al., 2017). As Moso bamboo stands occur along a wide environmental gradient, there is a need to understand how environmental factors, namely climate and edaphic conditions, control key ecosystem processes, such as litter decomposition, at large scales. Understanding the relative importance of decomposition drivers at regional scales could facilitate predictions of how carbon and nutrient cycling in Moso bamboo stands will respond to future environmental changes.
Litter decomposition is an essential process regulating nutrient cycling and carbon storage in terrestrial ecosystems. The decomposition of organic matter is mainly controlled by climatic, biotic (litter quality and soil decomposers), and edaphic factors (Coûteaux et al., 1995; Aerts, 1997; Berg and McClaugherty, 2014). At larger scales, the interplay of environmental and biotic factors influences the decomposition rate and stabilized material, which later forms part of the soil organic matter (Prescott, 2010). Climate is regarded as one of the most important environmental controls on litter decomposition at regional scales (Meentemeyer, 1978; Berg et al., 1993; Epstein et al., 2002). The influence of climatic drivers on litter decomposition has often been evaluated independently; however, previous studies have shown that precipitation and temperature (or soil moisture and temperature) can interactively affect the decomposition process (Taylor et al., 2017; Althuizen et al., 2018; Petraglia et al., 2019). Determining the thresholds at which the effect of one climatic factor is conditioned by the other is key to understanding the environmental controls of litter decomposition and soil carbon sequestration at broad scales (Prescott, 2010).
In addition to directly affecting litter quality, plant communities can indirectly influence the decomposition process through changes in litter input, soil microclimate, and chemical conditions (Wardle et al., 2004; Fang et al., 2015; Joly et al., 2017). In plant communities dominated by a single species, stand density and canopy structure variations can affect litter accumulation on the forest floor and the input of belowground organic matter (Penne et al., 2010; Na et al., 2021). Differences in litter accumulation may alter the water-holding capacity of the surface layer and provide a different microclimate for decomposer communities (Facelli and Pickett, 1991; Fekete et al., 2016). Variations in below-and above-ground litter input can alter soil pH or nutrient status (Xu et al., 2013; Liu et al., 2019b) and may indirectly affect belowground processes. In addition, canopy coverage can influence soil moisture conditions through rainfall interception or by decreasing the rate of evaporation from the soil through decreased sunlight (Prescott, 2002). Previous studies have shown that canopy structure is an important indirect driver of decomposition in regional and large-scale analyses (Joly et al., 2017; Wallace et al., 2018; Zhang et al., 2019). However, the overall contribution of stand structure and organic matter input can be masked by considering only their direct effects on litter decomposition (Wallace et al., 2018). As several factors within the decomposition system often affect each other, evaluating their direct and indirect effects could help elucidate the underlying mechanisms that simultaneously control the decomposition process.
Variation in litter quality in plant communities is expected due to differences in climate and soil conditions at broad scales. Litter decomposes faster in its original environment, as decomposers are adapted to degrade native litter (Veen et al., 2015). This process is known as home-field advantage and can represent potential pitfalls when evaluating the effect of litter quality on the decomposition process. To avoid this potential bias, Keuskamp et al. (2013) developed the Tea Bag Index (TBI) protocol based on two types of tea substrate as standard litter material. The contrasting decomposability of the substrates allows the calculation of the initial decomposition rate (k) and stabilization factor (S) after a short incubation period. Similar to the decomposition of other standard materials (i.e., cellulose paper), the TBI approach cannot predict actual carbon dynamics of native litter. However, the decomposition of tea substrates represents well the decay patterns of plant materials (Didion et al., 2016; Blume-Werry et al., 2021) and has a similar response to variations in moisture and temperature as local litter (Didion et al., 2016; Mueller et al., 2018). Using substrates of known litter quality makes it possible to exclude litter bias and better evaluate how environmental factors affect the decomposition parameters k and S (Keuskamp et al., 2013; Didion et al., 2016). In several studies, the TBI protocol was a reliable method for estimating the initial decomposition rates and the later stabilization phase across different environmental conditions and ecosystem types (Althuizen et al., 2018; Mueller et al., 2018; Petraglia et al., 2019).
In this study, we aimed to determine the relative importance of environmental factors, namely, climate and soil, as drivers of litter decomposition in Moso bamboo stands. We selected 13 sites comprising managed and unmanaged stands distributed along a broad climatic and latitudinal gradient (spanning 23°N to 33°N) across southern Japan and Taiwan. Because the culm density of Moso bamboo varies depending on the management conditions (managed stands maintain densities of less than 4,000 culm ha–1, whereas abandoned stands exceed densities of 8,000 culm ha–1) (Inoue et al., 2018; Yin et al., 2019), we also considered the variation in stand properties and input of organic matter as potential drivers of decomposition. To avoid variation in litter quality and the possible influence of home-field advantage from local litter, we used the TBI method (Keuskamp et al., 2013) to estimate the decomposition parameters k and S as proxies for the initial decomposition rate and long-term carbon accumulation, respectively. We first investigated which controlling factors (climate, soil properties, stand properties, or organic matter input) exert predominant control over k and S. We then determined how particular indicators of stand properties, organic matter input, and soil parameters directly or indirectly affected the decomposition process. To understand the relative contribution of climate, two types of temporal scales were considered: temporal climate, which refers to the temperature and precipitation during the incubation period (90 days), and long-term climate. We hypothesized that climate would be an important driver of k and S. However, we expected that the temporal climate would mainly control k, while the long-term climate would be more important for S. We also expected that soil and organic matter input parameters would be relatively more important for S than for k. Finally, we expected that the stand structure variability of Moso bamboo stands would indirectly influence decomposition parameters through changes in organic matter input and edaphic properties.
We selected 13 sites with Moso bamboo stands distributed along a wide latitudinal gradient from 33°60′N to 23°40′N across Taiwan and southern Japan. Most of these sites were located on Kyushu Island (southern Japan), and one site was located in central Taiwan (Table 1). All locations corresponded to the warm temperate and humid climate biome (Breckle, 2002). Moso bamboo was the dominant species in the surveyed stands and few understory vegetation was present.
Table 1. Location, environmental features, and mean values of stand structure characteristics (stand density and DBH) and decomposition parameters (k and S) for the 13 sites.
Precipitation and temperature records were obtained from the Japan Meteorological Agency (2019) and Taiwan Central Weather Bureau (2019) from the meteorological station closest to each site. The mean annual temperature (MAT) and mean annual precipitation (MAP) for 10 years (2008–2018) ranged from 12.6 to 19.3°C, and 1,660–3,190 mm (Table 1). These data represented the “long-term climate.” The average daily temperature and total precipitation during the incubation period (June–September 2018) were obtained at the site level and represented the “temporal climate.” Precipitation and temperature values were not correlated across sites (rs = −0.14, P = 0.34), whereas long-term climate was positively correlated with temporal climate (temperature: rs = 0.49, P < 0.01; precipitation: rs = 0.85, P < 0.001).
We randomly established four 2 m × 2 m plots at each site, and surveyed soil properties at the plot level. Plots were carefully selected to minimize variations in other soil conditions (slope, aspect, and distance to stand edges). Three soil core samples were collected from a depth of 5 cm and oven-dried at 70°C to determine gravimetric water content. From the fine soil fraction (sieve < 2 mm diameter), total carbon and nitrogen concentrations were measured using a CN analyzer (Yanaco MT-700, Kyoto, Japan). Soil pH (H2O) (2:5 dry soil:distilled water ratio) was measured using a pH meter (Horiba D54-S, Kyoto, Japan), and soil bulk density was determined from the soil core samples at depths of 0–10 cm. The measured soil properties (pH, water content, bulk density, and C/N ratio) showed high variation among sites and low within-site variability, indicating high regional variation (Table 2 and Supplementary Figure 1).
Table 2. Description of factors and measured variables used in the structural equation models and the linear-mixed models.
We considered stand structure variability and the amount of organic matter input to be potential drivers of litter decomposition. In each plot, we recorded the diameter at breast height (DBH ≥ 5 cm) and counted the number of individual living culms to estimate the stand density. Canopy coverage was measured by obtaining hemispherical images 2 m above the ground using a fish-eye lens. The photographs were then analyzed using the software Gap Light Analyzer (Frazer et al., 1999).
In addition, leaf litter samples on the forest floor were collected using a 20 cm × 20 cm frame to determine the amount of litter accumulated in the organic layer. Litter samples were collected in mid-June 2018; therefore, they reflect the litterfall in Moso bamboo stands that occurred during April–May (Song et al., 2016). Fine roots were separated from the soil samples and weighed to estimate the fine root biomass. Fine root biomass and litter mass accumulated at the plot level were considered indicators of organic matter input in the stands.
Because some of the surveyed sites corresponded to managed stands (five sites, i.e., KMT, SBA, SSM, IZK, and TWN), high variability of stand density and organic matter input properties were captured among plots and sites (Table 2). Regardless of management status, there was no clear separation between managed and unmanaged sites in relation to stand structure and organic matter input variables (Supplementary Figure 2). Moreover, the high within-site variability indicated large local variation in the studied region (Supplementary Figure 2).
The TBI method was used to evaluate the influence of environmental factors and stand properties on decomposition. This method uses two types of tea (rooibos and green tea) as standard materials. Owing to their contrasting litter qualities, green tea decomposes faster than rooibos tea (Keuskamp et al., 2013). After approximately 90 days of litter decomposition, the mass loss of labile materials in green tea is used to calculate the stabilization factor (S), whereas the more recalcitrant materials still actively decomposing in rooibos tea allows for the estimation of the short-term decomposition rate (k) (Keuskamp et al., 2013).
At the beginning of the summer season (June 2018), two green (Lipton, Unilever, EAN: 87 22700 05552 5) and rooibos tea bags (Lipton, Unilever, EAN: 87 22700 18843 8) were placed on the soil in each plot, totaling 208 buried tea bags (4 tea bags × 4 plots × 13 sites). Following the method of Keuskamp et al. (2013), tea bags were collected after 3 months of incubation and oven-dried at 70°C for 48 h to calculate the mass loss of each substrate. Because the tea samples had soil particles adhered to the substrates after collection, the tea substrates were incinerated at 600°C for 6 h, and the ash mass was subtracted from the dry mass to eliminate soil contamination in the samples.
The stabilization factor (S), which represents the proportion of the stabilized fraction that becomes recalcitrant after the initial phase of decomposition, was calculated as follows:
where ag is the fraction of green tea remaining, and Hg is the hydrolysable fraction of green tea (0.842 g g–1). Assuming that S is the same for both tea substrates, the decomposable fraction of rooibos tea (ar) was calculated as follows:
where Hr is the hydrolysable fraction of rooibos tea (0.552 g g–1). The initial decomposition rate constant (k) was estimated using the following decomposition curve:
where X(t) represents the fraction of remaining rooibos tea at time t. The labile fraction (ar) decomposes at rate k, whereas the recalcitrant fraction (1 – ar) is assumed to have a negligible decomposition rate during the initial phase of decay (Keuskamp et al., 2013). The mean values of k and S were calculated per plot, except for one plot located at the Taiwan site, where teabags could not be recovered.
We used multiple linear regression analysis to test whether the climatic conditions affected the decomposition parameters. We selected the best temporal and long-term climate model to explain the variation of k and S. For each model, interactions between precipitation and temperature were also included. When these interactions were significant, we further analyzed the interactions using the Johnson-Neyman intervals (Bauer and Curran, 2005). We considered temperature as the conditional variable and then calculated the main temperature intervals at which there would be significant changes in the slope of precipitation to explain a decomposition parameter. The specified alpha level for significance was set at P < 0.05. We used an adjustment to account for inflating Type I errors to calculate intervals (Esarey and Sumner, 2018). Interaction analysis and correction of intervals were performed using the “sim_slopes” function of the interactions package (Long, 2021) in the R software v. 4.0.2 (R Core Team, 2021).
We used linear mixed-effects models to separately test the effects of stand structure (stand density and canopy cover), organic matter input (litter mass and root biomass), and soil parameters (pH, C/N, water content, and bulk density) on k and S. In these models, the explanatory variables were considered fixed factors and the sites were included as random factors. The lme4 package was used to fit the linear mixed-effect models (Bates et al., 2015). Collinearity among variables was evaluated using variance inflation factors (VIF). Model selection was performed based on the lowest AIC, and then the R2 (marginal and conditional) of the most parsimonious model was extracted using the “R.SquareGLMM” function of the MuMIn package (Bartoń, 2022).
We then used the partial least squares structural equation model (PLS-SEM) approach to explore the direct and indirect effects of stand structure, organic matter input, and soil variables on k and S. Climatic variables were not included in the model because they could affect the decomposition indices interactively, and SEM models cannot account for such interactions. For a specific a priori model, we hypothesized that stand structure properties would indirectly control decomposition. For instance, higher canopy coverage increases soil moisture by reducing soil surface evaporation, thereby affecting the decomposition parameters (Prescott, 2002; Joly et al., 2017). In addition, higher stand density increases litter mass and fine root biomass, indirectly affecting k and S (Na et al., 2021). We also expected that litter mass and root biomass would influence soil properties, such as pH, water content, bulk density, and C/N ratio (Facelli and Pickett, 1991; Xu et al., 2013; Liu et al., 2019b). Finally, we expected that soil properties would directly control both decomposition parameters (Prescott, 2010; Berg and McClaugherty, 2014). All data were standardized prior to performing the structural equation model, and non-parametric bootstrapping (5,000 resamples) was employed to obtain the PLS parameter estimates. We used a bootstrap confidence interval of 95% to determine the significance of the path coefficients, and only the significant pathways were retained in the final model (Hair et al., 2017). PLS-SEM analysis was performed using the SmartPLS software (v3.3.6, Ringle et al., 2015).
Across sites, the mean values of k and S ranged from 0.010 to 0.023, and from 0.08 to 0.20, respectively (Table 1). Climate significantly affected both the decomposition parameters (k and S). The most parsimonious model of the temporal climate explained 42% of the variance in k, whereas the long-term climate model explained only 16% (Table 3). Conversely, the long-term climate explained 21% of the variance in S, whereas the temporal climate explained 14% (Table 3). In both climatic models, precipitation and temperature interactively affected the decomposition rates. In the temporal climate model, the effect of precipitation on k was dependent on specific temperature thresholds. When the temperature was lower than 23.7°C, k decreased with increasing precipitation; when the temperature was between 23.7 and 26.8°C, precipitation did not affect k; and when the temperature was higher than 26.8°C, k increased with precipitation (Figure 1A). In the long-term climate model, the interaction showed that when MAT was higher than 15.6°C, k increased with MAP, whereas when MAT was lower than that value, MAP had no significant effect on k (Figure 1B). There was a nonlinear decrease in S with temperature in both the temporal and long-term climate models, while S increased nonlinearly with precipitation in the latter model (Table 3).
Figure 1. Johnson-Neyman plots showing the interactions between temperature and precipitation on the initial decomposition rate (k) in the (A) temporal climate and (B) long-term climate model. Dash lines show the temperature thresholds at which there are significant changes in the effect of precipitation on k.
The linear mixed-effects models showed that stand structure, organic matter input, and soil parameters had no significant influence on the initial decomposition rates across sites (Table 4). Fine root biomass had a marginal effect on S (P < 0.1) in the most parsimonious model of organic matter input and explained only 6% of the variance in S (Table 4).
Table 4. Results of multiple linear mixed models testing the effects of stand structure, organic matter input, and soil parameters on k and S.
PLS-SEM models accounted for 23 and 27% of the variation in k and S, respectively (Figure 2). The model revealed interconnected relationships among the decomposition drivers, but none of the stand structure, organic matter input, or soil variables directly or indirectly affected k (Figure 2A). However, the model showed indirect cascading effects from stand density to S, mediated by changes in organic matter input and soil bulk density (Figure 2B). Stand density had a significant direct influence on litter mass (0.28) and fine root biomass (0.25). Both fine root biomass and litter mass negatively affected the soil bulk density (−0.22 and −0.31, respectively), while the latter negatively influenced litter stabilization (−0.31). Overall, this indicated that stand density and organic matter input had indirect positive effects on S, mediated by changes in soil bulk density. Although canopy cover did not affect soil conditions (Figure 2), these results demonstrate that stand structure variability can modify the soil environment and indirectly influence litter stabilization.
Figure 2. Structural equation models showing the effects of stand structure (stand density and canopy cover), organic matter input (fine root biomass and litter mass), and soil variables (bulk density, pH, C/N ratio, and water content) on (A) the initial decomposition rate (k) and (B) the stabilization factor (S). R2 values represent the proportion of total variance explained by the model. Significant paths are shown in black arrows with solid and dashed arrows representing positive and negative effects, respectively. Thin gray lines show tested relationships that were not significant.
The climate variables were the most important drivers of the initial decomposition rate (k) and stabilization factor (S). We found that the temporal scales of the climate affected the decomposition indices differently. The temporal climate was relatively more important, explaining 42% of the variation in k. This could be attributed to the variation in temperature and moisture conditions during the incubation period, which can directly influence microbial activity and leaching of soluble compounds, the latter of which is an important driver of mass loss in the early phases of litter decomposition (Cotrufo et al., 2010; Berg and McClaugherty, 2014). Conversely, the stabilization factor was primarily controlled by the long-term climate (21%) compared with the temporal climate (14%). Similar to our results, Althuizen et al. (2018) found that the long-term climate regime was more important for litter stabilization than variations in the short-term climate. The selected long-term climate model showed that temperature had a stronger effect on S despite low variability across the climatic gradient. The temperature sensitivity of carbon stabilization is a key factor in regulating soil carbon stocks (von Lützow and Kögel-Knabner, 2009). In warm temperate areas, soil carbon losses occur as microbial activity and heterotrophic respiration increase with temperature (Jackson et al., 2017). Although we found a negative nonlinear relationship between temperature and S, this result contrasts with that of Xu et al. (2018), who reported that soil carbon stocks in Moso bamboo stands decreased linearly with mean annual temperature across a latitudinal gradient.
Compared to other vegetation types in the region (Suzuki et al., 2019), the decomposition parameters in Moso bamboo stands from this study were in the upper and lower range for k and S, respectively (Supplementary Figure 3). The differences in the decomposition of standard litter could be attributed to the variation in environmental and site-specific conditions. Moso bamboo invasion into neighboring forests can lead to changes in the decomposition environment through the input of more recalcitrant litter (Song et al., 2016; Liu et al., 2019a). The slightly higher S in Moso bamboo stands than that of evergreen broadleaved forests could be related to the recalcitrance of bamboo litter and its lower decomposition rates, which may result in higher soil organic matter accumulation in the long-term (Liu et al., 2021). In addition, belowground biomass, which is significantly higher in Moso bamboo in comparison with evergreen broadleaved forests (Wang et al., 2013), may potentially contribute to increased carbon input and organic matter accumulation (Qin et al., 2017). The average k in Moso bamboo were comparable with that of evergreen broadleaf forests and higher than those of deciduous broadleaf, coniferous, mixed (broadleaf-conifer) forests and alpine shrubs. Similar initial decomposition rates in bamboo stands and evergreen broadleaf forests suggests that k was mainly influenced by climatic factors.
The interaction between precipitation and temperature was an important determinant of the variation in k. In this regional study, the effect of precipitation on k was mainly controlled by temperature thresholds. Increasing precipitation accelerated the initial litter decomposition at warmer sites, whereas decomposition was not determined and was negatively influenced by precipitation at the middle-range temperature and cooler sites, respectively. In this study, cooler sites were located at high altitudes and corresponded to locations with high mean annual precipitation (>3,000 mm). The negative effect of precipitation at these sites could be explained by the high amount of rainfall and oxygen depletion in soils, which can affect microbial communities and limit decomposition rates under such conditions (Schuur, 2001; Althuizen et al., 2018). Previous studies using the TBI method found that the effects of soil temperature on decomposition rates were dependent on soil moisture (Petraglia et al., 2019; Sarneel et al., 2020), indicating that complex interactions can affect decomposition rates during the early stages. Taylor et al. (2017) showed that, similar to our sites, warmer locations in tropical forests were associated with enhanced decomposition only under conditions of higher precipitation.
In our regional study, neither stand characteristics nor site conditions affected the initial decomposition rate (k). Specifically, soil properties had no direct effect on k, despite being important modulators of the abundance and activity of soil microbial communities and microarthropods (Prescott, 2010; Berg and McClaugherty, 2014). The limited contribution of soil parameters to k may be related to the greater importance of the leaching process during the early phases of litter decomposition (Cotrufo et al., 2010). However, as we only focused on the controls of initial decomposition rates, the relative importance of soil parameters might change during the later stages of decomposition (García-Palacios et al., 2016). It is also important to note that climate data aggregated at the site level could lead to a larger contribution by climate on decomposition rates at large scales of analysis (Bradford et al., 2016). This could mask the contribution of other decomposition drivers at the local scale, such as variations in the microbial communities (Bradford et al., 2017). In addition, because water availability is an important driver of mass loss in tea bags (Djukic et al., 2018; Blume-Werry et al., 2021; Mori et al., 2021), the local-scale conditions of soil moisture measured continuously throughout the incubation period may better explain the variation in initial decomposition rates.
Contrary to our expectations, the linear mixed-effect model showed no influence of soil properties on S, despite being highly variable across the studied region. However, SEM analysis, which tests the causal relationships among variables, showed that soil bulk density was negatively related to the stabilization factor. Organic matter can be stabilized in the soil through the physical protection of soil microaggregates. This mechanism protects organic matter from decomposition and, consequently, favors its accumulation in the soil (Six et al., 2002). Generally, increasing soil bulk density reflects a high degree of compaction and low porosity in the soil (Soane, 1990). As such, high bulk densities could lead to decreased micro-aggregation in soils and thus negatively affect the stabilization of organic matter (Six et al., 2002). In a previous study, Zhang et al. (2015) showed that soil bulk density was negatively associated with the topsoil organic carbon and was a good predictor of its spatial variation in Moso bamboo stands. The association between soil bulk density and litter stabilization found in this study further suggests that variations in soil structure could be important determinants of organic matter stabilization and potential soil carbon sequestration.
Stand structure did not directly explain the variability of the decomposition parameters; however, the SEM model showed indirect cascading effects from stand density to S. In the present study, bamboo stands with higher densities had increased amounts of litter mass accumulated on the forest floor and higher fine root biomass. Increasing litter mass and fine root biomass indirectly and positively affected the stabilization factor (S) through their effects on soil bulk density and not through soil microclimatic conditions, as initially expected. Above- and below-ground organic matter inputs can substantially influence the soil structure and carbon accumulation (De Deyn et al., 2008). For instance, rhizodeposition from roots is a source of labile carbon in the soil that can increase the presence of soil aggregates and binding structures and enhance the soil structure (Jobbágy and Jackson, 2000; Traoré et al., 2000). By altering soil structure and pores in the soil, roots can modify soil aggregation and short-term stabilization (Morel et al., 1991; Six et al., 2004). Additionally, litter decomposition and root turnover allow the progressive accumulation of organic matter in the soil (Jastrow and Miller, 1998), which has the potential to improve soil structure and promote organic matter stabilization (Kay, 1998; Abiven et al., 2009). Yang et al. (2021) showed that increased input of organic matter in extensively managed Moso bamboo stands were positively associated with soil aggregate stability and soil organic carbon. Altogether, our results suggest that variations in stand density and organic matter input in bamboo stands can modulate changes in soil structure and represent important indirect biotic controls of litter stabilization.
Variations in stand density and organic matter input in bamboo stands can represent important plant controls affecting below-ground processes and may be particularly relevant in bamboo-dominated communities that form dense monospecific stands and underground root networks (Fukuzawa et al., 2007; Yin et al., 2019). Unmanaged stands have higher densities than managed stands (Chen et al., 2016; Yin et al., 2019). However, the managed stands considered in this study had great variation in stand densities and did not differ in stand structure and organic matter input variables compared to unmanaged stands (Supplementary Figure 2). Nevertheless, it is important to note that different management practices can affect soil conditions and may have further consequences for below-ground functions (Fu et al., 2014; Yuen et al., 2017; Yang et al., 2021). In addition, the time of management, or in the case of unmanaged stands, the time of abandonment, might also be factors to consider when evaluating below-ground processes and soil carbon storage (Yin et al., 2019; Yang et al., 2021). Although the effect of such factors may be inherent in the studied sites, our results still provide an understanding of the mechanisms by which stand structure controls organic matter stabilization and the potential soil carbon storage in bamboo stands. These results may provide further insights into how specific management practices (i.e., thinning) and the variability of stand density could influence the decomposition process in Moso bamboo stands.
Our study suggests that climatic factors are the main drivers of decomposition processes in Moso bamboo stands on a regional scale. Long-term climate greatly influenced litter stabilization, whereas k was mostly affected by temporal climatic conditions. Our analysis also revealed that k was strongly affected by the interaction between precipitation and temperature, suggesting that precipitation only accelerated the initial decomposition rates at warmer sites. Overall, stand structure and soil properties had limited direct effects on both the decomposition parameters. However, indirect cascading effects from stand density affected litter stabilization through variations in the amount of organic matter input and their consequent modifications to soil bulk density. These results demonstrate the importance of identifying direct and indirect pathways to disentangle the different mechanisms controlling litter decomposition and stabilization. Finally, the particular relevance of climate in this region suggests that changes in precipitation regimes or temperature would greatly affect the early phases of decomposition and potential soil carbon storage in Moso bamboo stands. Nevertheless, because this study only measured potential indicators of the decomposition process, further research considering litter quality and its interaction with other environmental factors is required to improve our understanding of the biogeochemical processes in Moso bamboo stands.
The original contributions presented in this study are included in the article/Supplementary Material, further inquiries can be directed to the corresponding author.
TE contributed to the conception and design of the study. MO conducted chemical and statistical analyses and wrote the manuscript. TE, SU, AI, SL, TK, SK, TH, and MO participated in the field survey and data collection. All authors contributed to manuscript revision, read, and approved the submitted version.
This work was supported by JSPS KAKENHI Grant Number JP19H02996.
The authors declare that the research was conducted in the absence of any commercial or financial relationships that could be construed as a potential conflict of interest.
All claims expressed in this article are solely those of the authors and do not necessarily represent those of their affiliated organizations, or those of the publisher, the editors and the reviewers. Any product that may be evaluated in this article, or claim that may be made by its manufacturer, is not guaranteed or endorsed by the publisher.
We thank the private landowners, Kazuo Dougomori (SSM and SSU) and Kenji Shiiba (SBA), for allowing us to use their land. We also thank Tsuyoshi Kajisa (Kagoshima University) and Yufu Tanizaki (Fukuoka Agricultural Research Center), and the staff of the University Forest of Kyushu and Kagoshima University for permitting us to use the research facilities at their institutions. We also extend our gratitude to Masaki Akesaka (Kyushu University), Asuha Ishihara (Kagoshima University), and Junya Matsushita (Kagoshima University) for their assistance in the field.
The Supplementary Material for this article can be found online at: https://www.frontiersin.org/articles/10.3389/ffgc.2022.921028/full#supplementary-material
Abiven, S., Menasseri, S., and Chenu, C. (2009). The effects of organic inputs over time on soil aggregate stability – a literature analysis. Soil Biol. Biochem. 41, 1–12. doi: 10.1016/j.soilbio.2008.09.015
Aerts, R. (1997). Climate, leaf litter chemistry and leaf litter decomposition in terrestrial ecosystems: a triangular relationship. Oikos 79, 439–449. doi: 10.2307/3546886
Althuizen, I. H. J., Lee, H., Sarneel, J. M., and Vandvik, V. (2018). Long-term climate regime modulates the impact of short-term climate variability on decomposition in alpine grassland soils. Ecosystems 21, 1580–1592. doi: 10.1007/s10021-018-0241-5
Bartoń, K. (2022). MuMIn: Multi-Model Inference. Available online at: https://CRAN.R-project.org/package=MuMIn (accessed February 26, 2022).
Bates, D., Mächler, M., Bolker, B., and Walker, S. (2015). Fitting linear mixed-effects models using lme4. J. Stat. Softw. 67, 1–48. doi: 10.18637/jss.v067.i01
Bauer, D. J., and Curran, P. J. (2005). Probing interactions in fixed and multilevel regression: inferential and graphical techniques. Multivar. Behav. Res. 40, 373–400. doi: 10.1207/s15327906mbr4003_5
Berg, B., Berg, M. P., Bottner, P., Box, E., Breymeyer, A., de Anta, R. C., et al. (1993). Litter mass loss rates in pine forests of Europe and Eastern United States: some relationships with climate and litter quality. Biogeochemistry 20, 127–159. doi: 10.1007/BF00000785
Berg, B., and McClaugherty, C. (2014). Plant Litter: Decomposition, Humus Formation, Carbon Sequestration, 3rd Edn. Berlin: Springer.
Blume-Werry, G., Di Maurizio, V., Beil, I., Lett, S., Schwieger, S., and Kreyling, J. (2021). Don’t drink it, bury it: comparing decomposition rates with the tea bag index is possible without prior leaching. Plant Soil 465, 613–621. doi: 10.1007/s11104-021-04968-z
Bradford, M. A., Berg, B., Maynard, D. S., Wieder, W. R., and Wood, S. A. (2016). Understanding the dominant controls on litter decomposition. J. Ecol. 104, 229–238. doi: 10.1111/1365-2745.12507
Bradford, M. A., Veen, G. F. C., Bonis, A., Bradford, E. M., Classen, A. T., Cornelissen, J. H. C., et al. (2017). A test of the hierarchical model of litter decomposition. Nat. Ecol. Evol 1, 1836–1845. doi: 10.1038/s41559-017-0367-4
Breckle, S.-W. (2002). Walter’s Vegetation of the Earth: the Ecological Systems of the Geo-biosphere, 4th Edn. Berlin: Springer.
Canavan, S., Richardson, D. M., Visser, V., Le Roux, J. J., Vorontsova, M. S., and Wilson, J. R. U. (2017). The global distribution of bamboos: assessing correlates of introduction and invasion. AoB Plants 9:lw078. doi: 10.1093/aobpla/plw078
Chen, T. H., Wang, D. H., and Wang, S. (2016). The trend of growth characteristics of Moso bamboo (Phyllostachys pubescens) forests under an unmanaged condition in central Taiwan. Taiwan J. For. Sci. 31, 75–87.
Cotrufo, M. F., Galdo, I. D., and Piermatteo, D. (2010). “Litter decomposition: concepts, methods and future perspectives,” in Soil Carbon Dynamics: An Integrated Methodology, eds A. Heinemeyer, M. Bahn, and W. L. Kutsch (Cambridge: Cambridge University Press), 76–90. doi: 10.1002/pmic.201300095
Coûteaux, M.-M., Bottner, P., and Berg, B. (1995). Litter decomposition, climate and liter quality. Trends Ecol. Evol. 10, 63–66. doi: 10.1016/S0169-5347(00)88978-8
De Deyn, G. B., Cornelissen, J. H. C., and Bardgett, R. D. (2008). Plant functional traits and soil carbon sequestration in contrasting biomes. Ecol. Lett. 11, 516–531. doi: 10.1111/j.1461-0248.2008.01164.x
Didion, M., Repo, A., Liski, J., Forsius, M., Bierbaumer, M., and Djukic, I. (2016). Towards harmonizing leaf litter decomposition studies using standard tea bags—a field study and model application. Forests 7:167. doi: 10.3390/f7080167
Djukic, I., Kepfer-Rojas, S., Schmidt, I. K., Larsen, K. S., Beier, C., Berg, B., et al. (2018). Early stage litter decomposition across biomes. Sci. Total Environ. 628–629, 1369–1394. doi: 10.1016/j.scitotenv.2018.01.012
Epstein, H. E., Burke, I. C., and Lauenroth, W. K. (2002). Regional patterns of decomposition and primary production rates in the U.S. Great Plains. Ecology 83, 320–327. doi: 10.1890/0012-96582002083
Esarey, J., and Sumner, J. L. (2018). Marginal effects in interaction models: determining and controlling the false positive rate. Comp. Polit. Stud. 51, 1144–1176. doi: 10.1177/0010414017730080
Facelli, J. M., and Pickett, S. T. A. (1991). Plant litter: its dynamics and effects on plant community structure. Bot. Rev. 57, 1–32. doi: 10.1007/BF02858763
Fang, X., Zhao, L., Zhou, G., Huang, W., and Liu, J. (2015). Increased litter input increases litter decomposition and soil respiration but has minor effects on soil organic carbon in subtropical forests. Plant Soil 392, 139–153. doi: 10.1007/s11104-015-2450-4
Fekete, I., Varga, C., Biró, B., Tóth, J. A., Várbíró, G., Lajtha, K., et al. (2016). The effects of litter production and litter depth on soil microclimate in a central European deciduous forest. Plant Soil 398, 291–300. doi: 10.1007/s11104-015-2664-5
Frazer, G. W., Canham, C. D., and Lertzman, K. P. (1999). Gap Light Analyzer (GLA), Version 2.0: Imaging Software to Extract Canopy Structure and Gap Light Transmission Indices from True-colour Fisheye Photographs. Burnaby: Simon Fraser University.
Fu, W., Jiang, P., Zhao, K., Zhou, G., Li, Y., Wu, J., et al. (2014). The carbon storage in Moso bamboo plantation and its spatial variation in Anji county of southeastern China. J. Soils Sediment. 14, 320–329. doi: 10.1007/s11368-013-0665-7
Fukuzawa, K., Shibata, H., Takagi, K., Satoh, F., Koike, T., and Sasa, K. (2007). Vertical distribution and seasonal pattern of fine-root dynamics in a cool–temperate forest in northern Japan: implication of the understory vegetation. Sasa dwarf bamboo. Ecol. Res. 22, 485–495. doi: 10.1007/s11284-006-0031-y
García-Palacios, P., Shaw, E. A., Wall, D. H., and Hättenschwiler, S. (2016). Temporal dynamics of biotic and abiotic drivers of litter decomposition. Ecol. Lett. 19, 554–563. doi: 10.1111/ele.12590
Hair, J. F., Hult, G. T. M., Ringle, C. M., and Sarstedt, M. (2017). A Primer on Partial Least Squares Structural Equation Modeling (PLS-SEM). Thousand Oaks, CA: Sage Publications.
Inoue, A., Sato, M., and Shima, H. (2018). Maximum size-density relationship in bamboo forests: case study of Phyllostachys pubescens forests in Japan. For. Ecol. Manag. 425, 138–144. doi: 10.1016/j.foreco.2018.05.044
Isagi, Y., and Torii, A. (1997). Range expansion and its mechanisms in a naturalized bamboo species, Phyllostachys pubescens, in Japan. J. Sustain. For. 6, 127–141. doi: 10.1300/J091v06n01_08
Jackson, R. B., Lajtha, K., Crow, S. E., Hugelius, G., Kramer, M. G., and Piñeiro, G. (2017). The ecology of soil carbon: pools, vulnerabilities, and biotic and abiotic controls. Annu. Rev. Ecol. Evol. Syst. 48, 419–445. doi: 10.1146/annurev-ecolsys-112414-054234
Japan Meteorological Agency (2019). Records of 2008-2018 Period. Available online at: http://www.data.jma.go.jp/obd/stats/etrn/ (accessed April 1, 2019).
Jastrow, J. D., and Miller, R. M. (1998). “Soil aggregate stabilization and carbon sequestration: feedbacks through organomineral associations,” in Soil Processes and the Carbon Cycle, eds R. Lal, J. M. Kimble, R. F. Follett, and B. A. Stewart (Boca Raton, FL: CRC Press), 207–224.
Jobbágy, E. G., and Jackson, R. B. (2000). The vertical distribution of soil organic carbon and its relation to climate and vegetation. Ecol. Appl. 10, 423–436.
Joly, F.-X., Milcu, A., Scherer-Lorenzen, M., Jean, L.-K., Bussotti, F., Dawud, S. M., et al. (2017). Tree species diversity affects decomposition through modified micro-environmental conditions across European forests. New Phytol. 214, 1281–1293. doi: 10.1111/nph.14452
Kay, B. D. (1998). “Soil structure and organic carbon: a review,” in Soil Processes and the Carbon Cycle, eds R. Lal, J. M. Kimble, R. F. Follett, and B. A. Stewart (Boca Raton, FL: CRC Press), 169–197.
Keuskamp, J. A., Dingemans, B. J. J., Lehtinen, T., Sarneel, J. M., and Hefting, M. M. (2013). Tea bag index: a novel approach to collect uniform decomposition data across ecosystems. Methods Ecol. Evol. 4, 1070–1075. doi: 10.1111/2041-210X.12097
Lin, M. Y., Hsieh, I. F., Lin, P. H., Laplace, S., Ohashi, M., Chen, T. H., et al. (2017). Moso bamboo (Phyllostachys pubescens) forests as a significant carbon sink? A case study based on 4-year measurements in central Taiwan. Ecol. Res. 32, 845–857. doi: 10.1007/s11284-017-1497-5
Liu, C., Zhou, Y., Qin, H., Liang, C., Shao, S., Fuhrmann, J. J., et al. (2021). Moso bamboo invasion has contrasting effects on soil bacterial and fungal abundances, co-occurrence networks and their associations with enzyme activities in three broadleaved forests across subtropical China. For. Ecol. Manag. 498:119549. doi: 10.1016/j.foreco.2021.119549
Liu, X., Lin, T.-C., Vadeboncoeur, M. A., Yang, Z., Chen, S., Xiong, D., et al. (2019b). Root litter inputs exert greater influence over soil C than does aboveground litter in a subtropical natural forest. Plant Soil 444, 489–499. doi: 10.1007/s11104-019-04294-5
Liu, X., Siemann, E., Cui, C., Liu, Y., Guo, X., and Zhang, L. (2019a). Moso bamboo (Phyllostachys edulis) invasion effects on litter, soil and microbial PLFA characteristics depend on sites and invaded forests. Plant Soil 438, 85–99. doi: 10.1007/s11104-019-04010-3
Long, J. A. (2021). Interactions: Comprehensive, User-friendly toolkit for Probing Interactions. Available online at: https://CRAN.R-project.org/package=interactions (accessed November 12, 2021).
Meentemeyer, V. (1978). Macroclimate and lignin control of litter decomposition rates. Ecology 59, 465–472. doi: 10.2307/1936576
Morel, J. L., Habib, L., Plantureux, S., and Guckert, A. (1991). Influence of maize root mucilage on soil aggregate stability. Plant Soil 136, 111–119. doi: 10.1007/BF02465226
Mori, T., Aoyagi, R., Taga, H., and Sakai, Y. (2021). Effects of water content and mesh size on tea bag decomposition. Ecologies 2, 175–186. doi: 10.3390/ecologies2010010
Mueller, P., Schile-Beers, L. M., Mozdzer, T. J., Chmura, G. L., Dinter, T., Kuzyakov, Y., et al. (2018). Global-change effects on early-stage decomposition processes in tidal wetlands – implications from a global survey using standardized litter. Biogeosciences 15, 3189–3202. doi: 10.5194/bg-15-3189-2018
Na, M., Sun, X., Zhang, Y., Sun, Z., and Rousk, J. (2021). Higher stand densities can promote soil carbon storage after conversion of temperate mixed natural forests to larch plantations. Eur. J. For. Res. 140, 373–386. doi: 10.1007/s10342-020-01346-9
Okutomi, K., Shinoda, S., and Fukuda, H. (1996). Causal analysis of the invasion of broad-leaved forest by bamboo in Japan. J. Veg. Sci. 7, 723–728. doi: 10.2307/3236383
Penne, C., Ahrends, B., Deurer, M., and Böttcher, J. (2010). The impact of the canopy structure on the spatial variability in forest floor carbon stocks. Geoderma 158, 282–297. doi: 10.1016/j.geoderma.2010.05.007
Petraglia, A., Cacciatori, C., Chelli, S., Fenu, G., Calderisi, G., Gargano, D., et al. (2019). Litter decomposition: effects of temperature driven by soil moisture and vegetation type. Plant Soil 435, 187–200. doi: 10.1007/s11104-018-3889-x
Prescott, C. E. (2002). The influence of the forest canopy on nutrient cycling. Tree Physiol. 22, 1193–1200. doi: 10.1093/treephys/22.15-16.1193
Prescott, C. E. (2010). Litter decomposition: what controls it and how can we alter it to sequester more carbon in forest soils? Biogeochemistry 101, 133–149. doi: 10.1007/s10533-010-9439-0
Qin, H., Niu, L., Wu, Q., Chen, J., Li, Y., Liang, C., et al. (2017). Bamboo forest expansion increases soil organic carbon through its effect on soil arbuscular mycorrhizal fungal community and abundance. Plant Soil 420, 407–421. doi: 10.1007/s11104-017-3415-6
R Core Team (2021). R: A Language and Environment for Statistical Computing. Vienna: R Foundation for Statistical Computing.
Ringle, C. M., Wende, S., and Becker, J. M. (2015). SmartPLS 3. Version 3.3.6. Oststeinbek: SmartPLS GmbH.
Sarneel, J. M., Sundqvist, M. K., Molau, U., Björkman, M. P., and Alatalo, J. M. (2020). Decomposition rate and stabilization across six tundra vegetation types exposed to >20 years of warming. Sci. Total Environ. 724:138304. doi: 10.1016/j.scitotenv.2020.138304
Schuur, E. A. G. (2001). The effect of water on decomposition dynamics in mesic to wet Hawaiian montane forests. Ecosystems 4, 259–273. doi: 10.1007/s10021-001-0008-1
Shinohara, Y., Kume, T., Ichihashi, R., Komatsu, H., and Otsuki, K. (2014). Moso bamboo forests in Japan: what are the effects of their area expansion on ecosystem services? J. Jpn. For. Soc. 96, 351–361. doi: 10.4005/jjfs.96.351
Six, J., Bossuyt, H., Degryze, S., and Denef, K. (2004). A history of research on the link between (micro)aggregates, soil biota, and soil organic matter dynamics. Soil Tillage Res. 79, 7–31. doi: 10.1016/j.still.2004.03.008
Six, J., Conant, R. T., Paul, E. A., and Paustian, K. (2002). Stabilization mechanisms of soil organic matter: implications for C-saturation of soils. Plant Soil 241, 155–176. doi: 10.1023/A:1016125726789
Soane, B. D. (1990). The role of organic matter in soil compactibility: a review of some practical aspects. Soil Tillage Res. 16, 179–201. doi: 10.1016/0167-1987(90)90029-D
Song, Q., Ouyang, M., Yang, Q., Lu, H., Yang, G., Chen, F., et al. (2016). Degradation of litter quality and decline of soil nitrogen mineralization after Moso bamboo (Phyllostachys pubescens) expansion to neighboring broadleaved forest in subtropical China. Plant Soil 404, 113–124. doi: 10.1007/s11104-016-2835-z
Suzuki, S. (2015). Chronological location analyses of giant bamboo (Phyllostachys pubescens) groves and their invasive expansion in a satoyama landscape area, western Japan. Plant Species Biol. 30, 63–71. doi: 10.1111/1442-1984.12067
Suzuki, S. N., Ataka, M., Djukic, I., Enoki, T., Fukuzawa, K., Hirota, M., et al. (2019). Harmonized data on early stage litter decomposition using tea material across Japan. Ecol. Res. 34, 575–576. doi: 10.1111/1440-1703.12032
Taiwan Central Weather Bureau (2019). Records of 2008-2018 Period. Available online at: https://www.cwb.gov.tw/V8/E/index.html (accessed January 13, 2019).
Takano, K. T., Hibino, K., Numata, A., Oguro, M., Aiba, M., Shiogama, H., et al. (2017). Detecting latitudinal and altitudinal expansion of invasive bamboo Phyllostachys edulis and Phyllostachys bambusoides (Poaceae) in Japan to project potential habitats under 1.5°C–4.0°C global warming. Ecol. Evol. 7, 9848–9859. doi: 10.1002/ece3.3471
Taylor, P. G., Cleveland, C. C., Wieder, W. R., Sullivan, B. W., Doughty, C. E., Dobrowski, S. Z., et al. (2017). Temperature and rainfall interact to control carbon cycling in tropical forests. Ecol. Lett. 20, 779–788. doi: 10.1111/ele.12765
Traoré, O., Groleau-Renaud, V., Plantureux, S., Tubeileh, A., and Boeuf-Tremblay, V. (2000). Effect of root mucilage and modelled root exudates on soil structure. Eur. J. Soil Sci. 51, 575–581. doi: 10.1111/j.1365-2389.2000.00348.x
Veen, G. F., Freschet, G. T., Ordonez, A., and Wardle, D. A. (2015). Litter quality and environmental controls of home-field advantage effects on litter decomposition. Oikos 124, 187–195. doi: 10.1111/oik.01374
von Lützow, M., and Kögel-Knabner, I. (2009). Temperature sensitivity of soil organic matter decomposition—what do we know? Biol. Fertil. Soils 46, 1–15. doi: 10.1007/s00374-009-0413-8
Wallace, K. J., Laughlin, D. C., Clarkson, B. D., and Schipper, L. A. (2018). Forest canopy restoration has indirect effects on litter decomposition and no effect on denitrification. Ecosphere 9:e02534. doi: 10.1002/ecs2.2534
Wang, B., Wei, W. J., Liu, C. J., You, W. Z., Niu, X., and Man, R. Z. (2013). Biomass and carbon stock in moso bamboo forest in subtropical China: characteristics and implications. J. Trop. For. Sci. 25, 137–148.
Wardle, D. A., Bardgett, R. D., Klironomos, J. N., Setälä, H., van der Putten, W. H., and Wall, D. H. (2004). Ecological linkages between aboveground and belowground biota. Science 304, 1629–1633. doi: 10.1126/science.1094875
Xu, M., Ji, H., and Zhuang, S. (2018). Carbon stock of Moso bamboo (Phyllostachys pubescens) forests along a latitude gradient in the subtropical region of China. PLoS One 13:e0193024. doi: 10.1371/journal.pone.0193024
Xu, S., Liu, L. L., and Sayer, E. J. (2013). Variability of above-ground litter inputs alters soil physicochemical and biological processes: a meta-analysis of litterfall-manipulation experiments. Biogeosciences 10, 7423–7433. doi: 10.5194/bg-10-7423-2013
Yang, C., Wang, A., Zhu, Z., Lin, S., Bi, Y., and Du, X. (2021). Impact of extensive management system on soil properties and carbon sequestration under an age chronosequence of Moso bamboo plantations in subtropical China. For. Ecol. Manag. 497:119535. doi: 10.1016/j.foreco.2021.119535
Yin, J., Ge, Z., Deng, X., Shi, Y., Xu, L., Zhou, G., et al. (2019). Abandonment lead to structural degradation and changes in carbon allocation patterns in Moso bamboo forests. For. Ecol. Manag. 449:117449. doi: 10.1016/j.foreco.2019.117449
Yuen, J. Q., Fung, T., and Ziegler, A. D. (2017). Carbon stocks in bamboo ecosystems worldwide: estimates and uncertainties. For. Ecol. Manag. 393, 113–138. doi: 10.1016/j.foreco.2017.01.017
Zhang, H., Zhuang, S., Qian, H., Wang, F., and Ji, H. (2015). Spatial variability of the topsoil organic carbon in the moso bamboo forests of southern china in association with soil properties. PLoS One 10:e0119175. doi: 10.1371/journal.pone.0119175
Keywords: Tea Bag Index, early-stage decomposition, Moso bamboo, stand structure, climate, stabilization factor, soil properties
Citation: Orrego M, Ugawa S, Inoue A, Laplace S, Kume T, Koga S, Hishi T and Enoki T (2022) Climate, Soil, and Plant Controls on Early-Stage Litter Decomposition in Moso Bamboo Stands at a Regional Scale. Front. For. Glob. Change 5:921028. doi: 10.3389/ffgc.2022.921028
Received: 15 April 2022; Accepted: 20 June 2022;
Published: 08 July 2022.
Edited by:
Nobuo Imai, Tokyo University of Agriculture, JapanReviewed by:
Xiaogai Ge, Chinese Academy of Forestry, ChinaCopyright © 2022 Orrego, Ugawa, Inoue, Laplace, Kume, Koga, Hishi and Enoki. This is an open-access article distributed under the terms of the Creative Commons Attribution License (CC BY). The use, distribution or reproduction in other forums is permitted, provided the original author(s) and the copyright owner(s) are credited and that the original publication in this journal is cited, in accordance with accepted academic practice. No use, distribution or reproduction is permitted which does not comply with these terms.
*Correspondence: Marly Orrego, bWFybHkub3JyZWdvLm1AZ21haWwuY29t
Disclaimer: All claims expressed in this article are solely those of the authors and do not necessarily represent those of their affiliated organizations, or those of the publisher, the editors and the reviewers. Any product that may be evaluated in this article or claim that may be made by its manufacturer is not guaranteed or endorsed by the publisher.
Research integrity at Frontiers
Learn more about the work of our research integrity team to safeguard the quality of each article we publish.