Corrigendum: Interactions Between Fire Refugia and Climate-Environment Conditions Determine Mesic Subalpine Forest Recovery After Large and Severe Wildfires
- Department of Geography, Portland State University, Portland, OR, United States
Infrequent stand-replacing wildfires are characteristic of mesic and/or cool conifer forests in western North America, where forest recovery within high-severity burn patch interiors can be slow, yet successful over long temporal periods (decades to centuries). Increasing fire frequency and high-severity burn patch size, under a warming climate, however, may challenge post-fire forest recovery, promoting landscape-level shifts in forest structure, composition, and distribution of non-forest patches. Crucial to a delay and/or impediment to this shift, fire refugia (i.e., remnant seed sources) may determine forest recovery trajectories and potential forest state-transitions. To examine how fire refugia attributes (i.e. extent, composition, and structure) interact with local climate and environmental conditions to determine post-fire forest recovery responses, we developed fine-grain maps of fire refugia via remote sensing and conducted field-based assessment of post-fire conifer tree establishment largely originating (i.e., dispersed) from fire refugium in the Central Cascade Range of the Pacific Northwest United States. We found that limitations on seed availability, represented by the distance2-weighted density (D2WD) of fine-grain refugia extent, largely explained post-fire tree establishment responses within our relatively mesic and cool subalpine study sites. Interactions between seed availability, climate, and environmental conditions indicated that the structural attributes of refugia (e.g., tree height) and site abiotic/biotic environmental controls (e.g., climate water deficit, canopy cover, and coarse woody debris cover) interplayed to constrain or enhance species-specific tree establishment responses. Importantly, these interactions illustrate that when seed availability is critically low for a given area, climate-environment conditions may strongly determine whether forests recover following fire(s). Toward modelling and predicting tree establishment responses and potential forest state-transitions after large stand-replacing fires(s), our study demonstrates the importance of accurately quantifying seed availability via the fine-grain extent, configuration, and attributes of remnant seed source legacies.
Introduction
Across montane forests in western North America, observed trends in mountain snowpack loss, earlier spring snowmelt, drier summers, and longer fire seasons are contributing to increasing wildfire frequency, size, and severity (Westerling, 2006; Abatzoglou and Williams, 2016; Gergel et al., 2017; Parks and Abatzoglou, 2020). As fire activity and warming conditions increase across landscapes, many forests may be at risk of fire- and climate-induced conversions to altered- or non-forest states (Coop et al., 2020), and especially in low elevation, dry forests (Stevens-Rumann et al., 2018; Davis et al., 2019; Kemp et al., 2019; Stewart et al., 2020). While large and severe infrequent fires are within the natural range of variation for mesic and/or cool high-elevation forest types (Agee, 1993; Baker, 2009), recently observed and projected increases in fire activity (Reilly et al., 2017; Halofsky et al., 2018, 2020; Higuera et al., 2021) may also limit post-fire tree establishment. Following fire events, many of these mesic and/or cool high-elevation forests evolved to recover during long fire-free periods (e.g., 50–200+ year fire return-interval; Agee, 1993; Turner and Romme, 1994). Where large and severe and/or frequent wildfires kill a significant number of live trees within a contingous area, with stand-replacing patch sizes exceeding tree species’ dispersal abilities, remnant live tree islands contained within fire perimeter boundaries—known as fire refugia—can be a critical bastion for forest persistence and recovery over time (Meddens et al., 2018; Blomdahl et al., 2019; Krawchuk et al., 2020). Strong relationships between post-fire conifer forest recovery, post-fire climate conditions, and fine-grain fire refugia extent at the landscape level has been demonstrated in dry mixed conifer forests of the western United States (Coop et al., 2019; Downing et al., 2019; Rodman et al., 2020). It is unknown, however, the way fine-grain spatial, structural, and compositional legacies of fire refugia interact with climate and environmental conditions to determine post-fire forest recovery responses and trajectories, and especially within mesic and/or cool high-elevation conifer forests.
Post-fire conifer forest recovery, across forest types and gradients of moisture availability, is strongly connected to processes that affect seed dispersal over space and time (Clark et al., 1999; Gill et al., 2022), and further, how climate and environmental conditions constrain or enhance the establishment of seeds that reach a given site (Kraft et al., 2015; Peeler and Smithwick, 2021). Thus, post-fire conifer forest recovery trajectories can be understood through the interactions between seed availability and biotic and abiotic controls on tree establishment over time. Post-fire seed availability is dictated by (1) the functional traits of seed sources, (2) structural attributes of seed sources (e.g., age, size, and height), (3) the distance to and density of seed sources over space, (4) the presence and patterns associated with local dispersal vectors (e.g., wind, water, snow, and animals), (5) the arrangement of dispersal barriers (e.g., topography, water, and vegetation), and (6) mean and interannual climate conditions that affect seed production (Clark et al., 1998, 1999; Gill et al., 2022). In the western United States, conifer species are primarily obligate seeders that reproduce from serotinous and/or non-serotinous wind-dispersed canopy seedbanks (McCaughey et al., 1986; Burns and Honkala, 1990). Large and severe fires pose a barrier to post-fire establishment by non-serotinous conifers when the distance to live trees exceeds species-specific dispersal abilities (Harvey et al., 2016; Stevens-Rumann and Morgan, 2019; Coop et al., 2020). Alternatively, short-interval fires may kill post-fire reestablishing serotinous and non-serotinous conifers before they can reach reproductive maturity (Buma et al., 2013). As a result, large, severe, and frequent fires may broadly overwhelm obligate seeding conifers reproductive traits and favor resprouting angiosperms instead (Kulakowski et al., 2013; Tepley et al., 2018; Keyser et al., 2020; Andrus et al., 2021). Unlike post-fire drought events that can temporarily inhibit conifer establishment for several years up to a decade (Andrus et al., 2018; Littlefield et al., 2020), dispersal limitations created by large, contigious patches of high-severity fire can largely inhibit tree establishment within patch interiors for decades, leading to long-term shifts in forest structure, composition, and extent (Johnstone et al., 2016; Gill et al., 2022).
Prior work quantifying the influence of complex seed source arrangement and structure in three dimensional space, as it relates to post-fire tree establishment, is limited in conifer forests in western North America, and especially among mesic and/or cool high-elevation forest types. Prior studies have primarily focused on forest patch edge concepts and metrics for quantifying dispersal limitations (e.g., Agee and Smith, 1984; Harvey et al., 2016; Kemp et al., 2016; Urza and Sibold, 2017; Busby et al., 2020), which only account for seed source linear distance as a contributing factor to seed dispersal and tree establishment patterns. Alternatively, other studies have focused on the seed source density (i.e., seed source cover within a specified area window surrounding a location; e.g., Haire and McGarigal, 2010; Rodman et al., 2020; Peeler and Smithwick, 2021), but at the expense of accounting for the distance of those individual seed sources to a location. More recent work has combined seed source distance and density into a single metric with compelling results (e.g., refugia distance2-weighted density; Coop et al., 2019; Downing et al., 2019), but that work was primarily limited to dry mixed-conifer forest types and did not consider the structural attributes of seed sources, nor how they interacted with climate and environmental conditions to shape establishment responses.
Finally, no known research has attempted to quantify the role of post-fire delayed tree mortality as it relates to changes in seed availability over time and thus potential differences in post-fire tree establishment responses. Tree mortality directly and indirectly related to fire effects can emerge over temporal periods that are much longer (e.g., ∼0–15 years; Brown et al., 2013; Hood and Varner, 2019) than the period commonly used for evaluating post-fire tree mortality at large scales (i.e., 1-year post-fire; Key, 2006; Eidenshink et al., 2007). As non-serotinous trees experience delayed mortality post-fire, their functional role as seed sources expires, diminishing seed availability among spatially proximate burned areas. Depending on the spatial arrangement, composition, and structure of fire refugia, and the favorability of post-fire climate conditions for tree establishment (e.g., Harvey et al., 2016; Andrus et al., 2018; Davis et al., 2019; Stewart et al., 2020), post-fire delayed tree mortality patterns have the potential to critically remove seed availability necessary for forest recovery within high-severity burn patch interiors. At present, it is unclear to what degree, and under what bioclimatic conditions, do post-fire delayed tree mortality patterns significantly influence tree establishment responses and conifer forest recovery trajectories.
Considering these research gaps, our objective in this study was to evaluate how the spatial and structural attributes of fire refugia, and their changes (temporal), interact with site climate and environmental conditions to influence post-fire forest recovery responses within lesser studied mesic, cool, high-elevation conifer forests. To support our study objectives, we mapped fine-grain fire refugia extent (tree cover) at the landscape-scale and surveyed forest structure and composition of fire refugium and conifer juvenile establishment largely originating (i.e., dispersed) from fire refugium after large, recent, stand-replacing wildfires in the Central Cascade Range of the Pacific Northwest region in the United States. Field data were collected to support assessment of the following research questions:
(1) How does post-fire conifer establishment differ among species and study sites at increasing distances from fire refugium?
(2) How do varying estimates of seed availability and forest structure, associated with fire refugia and their extent over time (i.e., considering delayed tree mortality), compare and interact as predictors of post-fire conifer establishment?
(3) How do the strongest estimates of forest structure and seed availability associated with fire refugia, as determined by question 2, compare and interact with climate and environmental conditions as predictors of post-fire conifer establishment?
Materials and Methods
Study Areas
We selected four wildfire perimeters in the Cascade Crest region of Oregon and Washington states to sample in this study; fire perimeters spanned a range of elevation and moisture gradients common to regional subalpine forests and experienced a range of time between when fires occurred and were sampled (7–23 years; Figure 1). These study fires were located on the southwestern face of Mt. Adams, WA (2012 Cascade Creek; ∼8,300 ha burned); the northeastern face of Mt. Hood, OR (2008 Gnarl Ridge; ∼1,400 ha burned); west of Big Lake, OR (2011 Shadow Lake; ∼2,800 ha); and northeast of Waldo Lake, OR (1996 Charlton; ∼3,800 ha burned). All study wildfires were lightning ignited, burned between August and September in their respective years, and have no other record of burning in recent history (>75 years since previous fire).
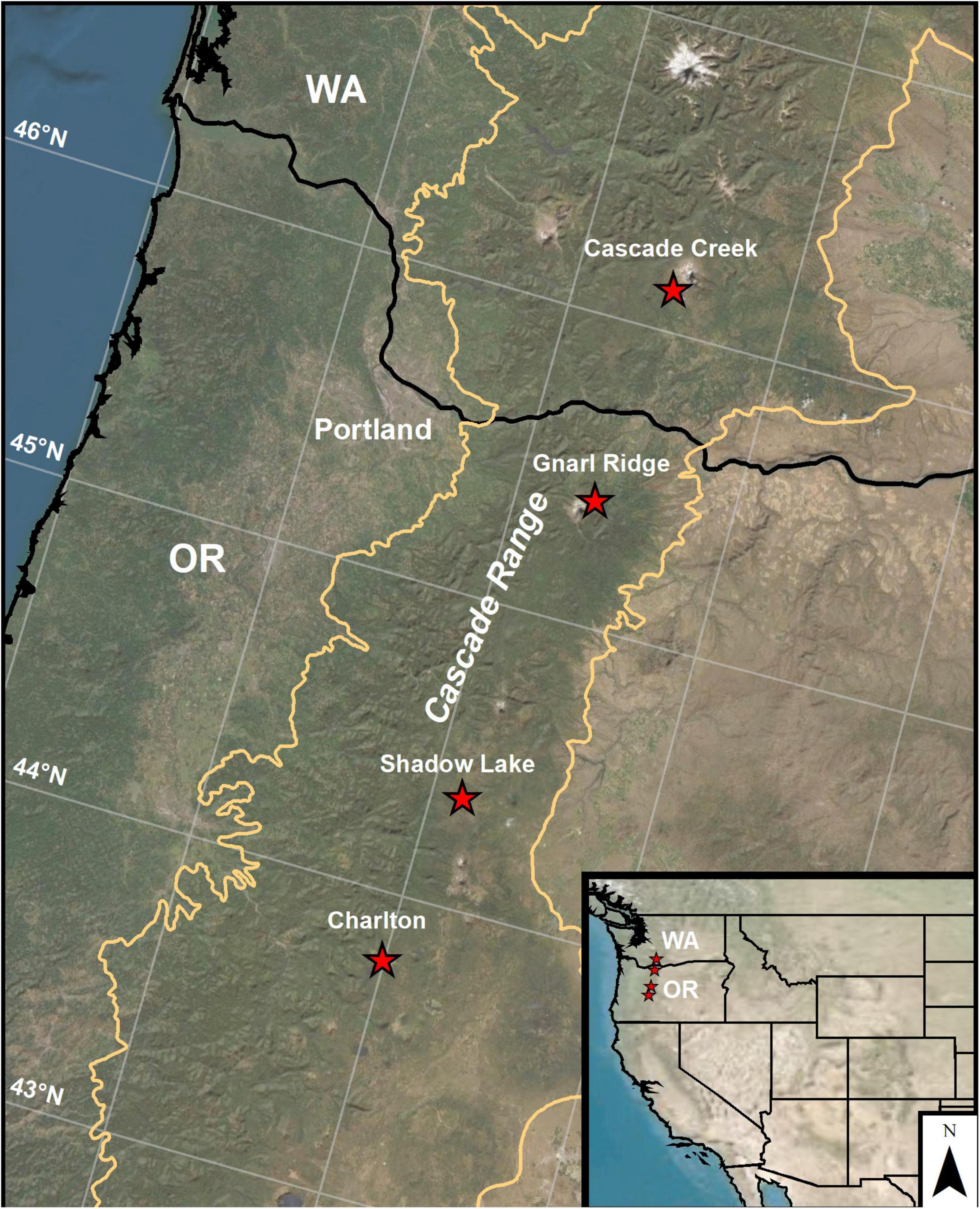
Figure 1. Geographic location of the four wildfires sampled in this study within mesic, high-elevation subalpine forests of the Cascade Crest region of Oregon and Washington states, United States.
To identify the four field sites for sampling, fire perimeters and burn severity patterns were analyzed from the Monitoring Trends in Burn Severity project (MTBS; Eidenshink et al., 2007) during the period of 1995–2012 in the Central Cascade Range of Oregon and Washington states. This range of fire years was considered to achieve the following criteria: (1) allow enough time since fire to initiate a post-fire conifer recruitment response (≥5 years) prior to field sampling; (2) high-resolution National Agricultural Imagery Program (NAIP) imagery would be available one and five years post-fire for each fire perimeter, except for one study area (see below); (3) fire perimeters were targeted which contained subalpine forest compositions (e.g. fir, spruce, mountain hemlock group) using the National Forest Type Dataset (USDA, 2008); (4) were accessible via road and trail access; and (5) had experienced contiguous high-severity burn patches of at least five hectares or greater in size (following Harvey et al., 2016). High-severity fire effects are commonly defined as causing 75–100% tree mortality one-year post-fire (Miller and Thode, 2007); 95–100% tree mortality (at the time of sampling; 2019) was commonly observed in our study areas. For question (2) we chose to analyze two post-fire temporal snapshots of live tree extent from NAIP, with the rationale that the 1- and ∼5-year post-fire images would respectively capture first- and second-order fire effects (i.e. initial and delayed post-fire tree mortality; Key, 2006; Hood and Varner, 2019), but not post-fire conifer sapling recruitment and/or substantial understory revegetation (Walker et al., 2019).
Elevation gradients across study fires ranged from 1350 to 1,800 m; at these elevations, substantial snowpack accumulates during winter months. Mean annual precipitation ranges 1,800–3,200 mm across our study fires and mean warmest month temperature ranges 13.6–14.9°C (1981–2010 means; ClimateNA; Wang et al., 2016). Sites in the 2011 Shadow Lake fire were, relatively, the warmest and driest, while sites in the 2008 Gnarl Ridge fire were the coolest and wettest. Soils in these landscapes are primarily well draining, ashy sandy loam andisols (USDA, 2018). Conifer forest composition in the subalpine stands we sampled were generally dominated by mountain hemlock (Tsuga mertensiana), true-firs (Abies lasiocarpa; Abies amabilis), and lodgepole pine (Pinus contorta var. latifolia) with a low abundance of Engelmann spruce (Picea engelmannii) and western white pine (Pinus monticola). Western larch (Larix occidentalis) was found exclusively in the 2008 Gnarl Ridge fire on Mt. Hood, where it supplemented lodgepole pine as the primary shade-intolerant tree species (Franklin and Dyrness, 1973).
Seed Availability
We delineated post-fire conifer refugia extent (i.e., cover) at a fine grain (i.e., the tree-level) to aid in field plot selection and for the purpose of quantifying conifer seed availability, at the landscape-level, across each study fire, using high-resolution (1 m) NAIP imagery. Since post-fire delayed tree mortality may alter the extent and pattern of fire refugia and thus affect seed dispersal and tree establishment patterns over time, we delineated fire refugia at two post-fire time periods to represent first-order (direct; initial) and second-order (indirect; extended) fire effects (Key, 2006). NAIP imagery was extracted 1- and 5-years post-fire for each fire perimeter, with the exception of the 1996 Charlton Fire; NAIP imagery was not available for the Charlton event until 2003, thus we only delineated its 7-year post-fire refugia extent after second-order fire effects. Fire perimeter polygons from MTBS were extended (buffered) 300 m outward in radial distance to ensure contiguous live forest edges were potentially captured in calculations of seed availability, as described below. Each buffered fire boundary was then used to clip NAIP imagery scenes to this specified extent. Next, fire refugia extent was classified in each clipped NAIP image using a semi-automated, object-based binary classification approach in ArcGIS (ESRI, 2020) following Walker et al. (2019). Contrary to Walker et al. (2019)’s work in dry conifer forests, we chose not to close holes in delineated refugia polygons in our mesic forests study areas, as these commonly represent canopy gaps within fire refugia patches that contribute to seed availability.
To assess image classification accuracy, we used equalized stratified random sampling to generate 100 validation points per class (fire refugia and non-forest) across each individual fire perimeter. We then used the source NAIP imagery, in each temporal snapshot (i.e., 1- and 5-years post-fire, and 7-year postfire for the Charlton Fire), to visually assign each validation point to either the fire refugia or non-forest class based on point overlap with visible canopy cover (Walker et al., 2019). A confusion matrix was generated among the 1- and ∼5-year post-fire snapshots of each fire perimeter to report imagery classification error (Congalton, 1991). Classification accuracy varied by fire perimeter but was generally very high; overall accuracy across fire perimeters ranged from 93 to 98% (Supplementary Table 1).
To spatially quantify post-fire seed availability at a fine-grain across each fire perimeter, we considered two metrics of seed availability originating from fire refugia, using the above classified refugia maps and a moving-window neighborhood approach. (1) Refugia density (e.g., Haire and McGarigal, 2010; Rodman et al., 2020) was calculated by summing all pixel values (refugia = 1; non-forest = 0) around each focal cell within a user-defined neighborhood radius, (2) refugia distance2-weighted-density (D2WD; Coop et al., 2019; Downing et al., 2019) was calculated by dividing pixel values (refugia = 1; non-forest = 0) by their squared distance to each focal cell (i.e., distance-weighting) and then summing all pixels within a neighborhood radius. Squaring of the distance term in the D2WD metric was used to account for exponential decay in seed dispersal at increasing distances from source trees (McCaughey et al., 1986; Clark et al., 1999; Coop et al., 2019). Considering varied seed dispersal traits reported among conifer species in our study areas and results by prior studies (e.g., Haire and McGarigal, 2010; Coop et al., 2019; Rodman et al., 2020), we calculated refugia density and refugia D2WD at three spatial scales using moving-window neighborhood radii of 150, 300, and 500 m. We also calculated refugia density and refugia D2WD at two post-fire temporal snapshots using the classified 1- and ∼5-year post-fire refugia maps referenced above. Because post-fire imagery was not available for the 1996 Charlton Fire until 2003, refugia density and refugia D2WD for that specific fire were only calculated on the 7-year post-fire refugia map.
Field Data Collection
To connect the structure and composition of fire refugia to post-fire conifer establishment responses, we established 30 m-diameter (0.07 ha) circular plots following Busby et al. (2020). One plot was located inside each targeted refugium (or green island) and four to five other plots located along predetermined post-fire regeneration transects extending from each refugium into expansive patches of severely burned forest. The refugium plots and the post-fire regeneration plots had different sampling protocols (see below). The specific location of our fire refugium plots and post-fire regeneration plots were predetermined using a distance-buffer ruleset and spatial analysis in ArcGIS to (1) maintain a minimum level of spatial independence between sampled fire refugium (>100 m) and post-fire regeneration transects (>200 m) and (2) reduce the influence of seed availability originating (i.e., dispersed) from live forest edges within our post-fire regeneration plots (>300 m; Supplementary Materials: Sample plot selection and distance-buffer ruleset; see Supplementary Figure 1 for diagram of the distance-buffer ruleset). Distances selected in this ruleset were based on a compromise between (1) maximizing sample size, (2) reducing spatial autocorellation between plots, and (3) ensuring that tree establishment responses observed in post-fire regeneration plots could be connected to the attributes of their associated refugium, based on known dispersal ranges of local conifer species (i.e., majority of seeds are dispersed within a 100–150 m range of live individuals; McCaughey et al., 1986; Stevens-Rumann and Morgan, 2019).
To quantify forest structure and composition at the refugium patch level, a single refugium plot was established inside each sampled fire refugium. Within each refugium plot, we recorded elevation, slope, aspect, and for all standing or uprooted trees (defined here as invididuals with diameter at breast height, DBH > 7.6cm; i.e., trees that contribute to meaningful seed dispersal; Stevens-Rumann and Morgan, 2016), species, status (live/dead), DBH, and vertical heights of live trees using a laser rangefinder (TruPulse 200/Laser Technology) were noted. Within each post-fire regeneration plot, physical site characteristics (elevation, slope, aspect), post-fire ground and overstory canopy cover, and post-fire conifer establishment were recorded. Post-fire ground cover [shrubs, total understory vegetation, coarse woody debris (CWD), and bare soil/rock] was visually assessed to the nearest 5% within five 1 m × 1m quadrats located at plot center and 7 m along the four cardinal direction transects; the five sample locations were then averaged to represent plot-level conditions (Busby et al., 2020). Overstory canopy density (cover) was measured at the same five sample quadrat locations using a densiometer at breast height; measurements were taken by one field crew member across plots to maintain observational consistency. Percent canopy cover was recorded facing each cardinal direction, averaged for the sample location, and then averaged among the five sample locations to similarly represent plot-level conditions (Lemmom, 1956).
Post-fire conifer juveniles (DBH < 7.6 cm) were counted in plots along each transect. We used variable size subplots to increase sampling efficiency depending upon plot-level juvenile densities, following Busby et al. (2020). For each juvenile, we recorded species, bud-scar count, and stem height; due to very high rates of mortality, we did not count juveniles in their 1st year of establishment (i.e. with cotyledon; Minore, 1986). Bud-scar count estimates were used to determine if juveniles had established pre or post-fire (Urza and Sibold, 2013). Finally, if present, the ten nearest live, sexually-mature conifer trees not contained within the targeted refugium were identified at the species level and their individual horizontal distance from (regeneration) plot center was recorded using a laser rangefinder (TruPulse 200/Laser Technology). Utilizing our sample plot selection procedure, we selected and sampled 41 fire refugia island plots and 213 post-fire regeneration plots distributed across the four study wildfire perimeters in summer of 2019.
Climate Water Deficit
To characterize the impact of water stress on post-fire conifer establishment across fire perimeters, we quantified 30-year mean (1981–2010) and post-fire (5-year period following each fire) mean and maximum climate water deficit (CWD; modelled potential evaporation minus actual evapotranspiration) at the plot level. We used the ClimateNA desktop application (Wang et al., 2016) and plot elevation derived from a 30 m Digital Elevation Model (DEM) to downscale gridded monthly climate data (PRISM; Daly et al., 2008; 800 m-initial resolution) to the (regeneration) plot level. A modified Thornthwaite-type water balance equation described by Lutz et al. (2010) was then used with (1) 30-year or annual-resolution mean monthly temperature and precipitation data, (2) soil water holding capacity data (POLARIS dataset; Chaney et al., 2016), and (3) a topographic heat load index (HLI; McCune and Keon, 2002) to calculate CWD. In this equation, the HLI is used as a terrain modifier to capture the effect of topographic microclimate on evaporative potential, thereby incorporating micro- and macro-scale water balance processes (Lutz et al., 2010).
Fire Refugia Attributes
To explore and compare what spatial, structural, and temporal attributes associated with fire refugia influenced post-fire conifer establishment, we developed a suite of fire refugia-related patch- and neighborhood-scale metrics derived from field data, remote sensing, or both sources combined, that could via seed availability potentially promote post-fire conifer establishment (Table 1). For the remote sensing derived seed availability variables, i.e. refugia density and distance2-weighted density (D2WD), we calculated multiple versions of these variables using (1) varying neighborhood radii and (2) 1- and ∼5-year post-fire refugia extent maps, respectively, to capture initial and delayed post-fire tree mortality patterns. Other variables were derived directly through field measurements (e.g., refugia distance, live mean tree height), by measuring refugium patch geometry using remote sensing (i.e., patch size, patch width), or by scaling plot-level refugium composition measured in the field to the refugium patch scale (e.g., total and live patch basal area), using remotely sensed refugium patch size, our fixed refugium plot size, and a multiplicative factor.
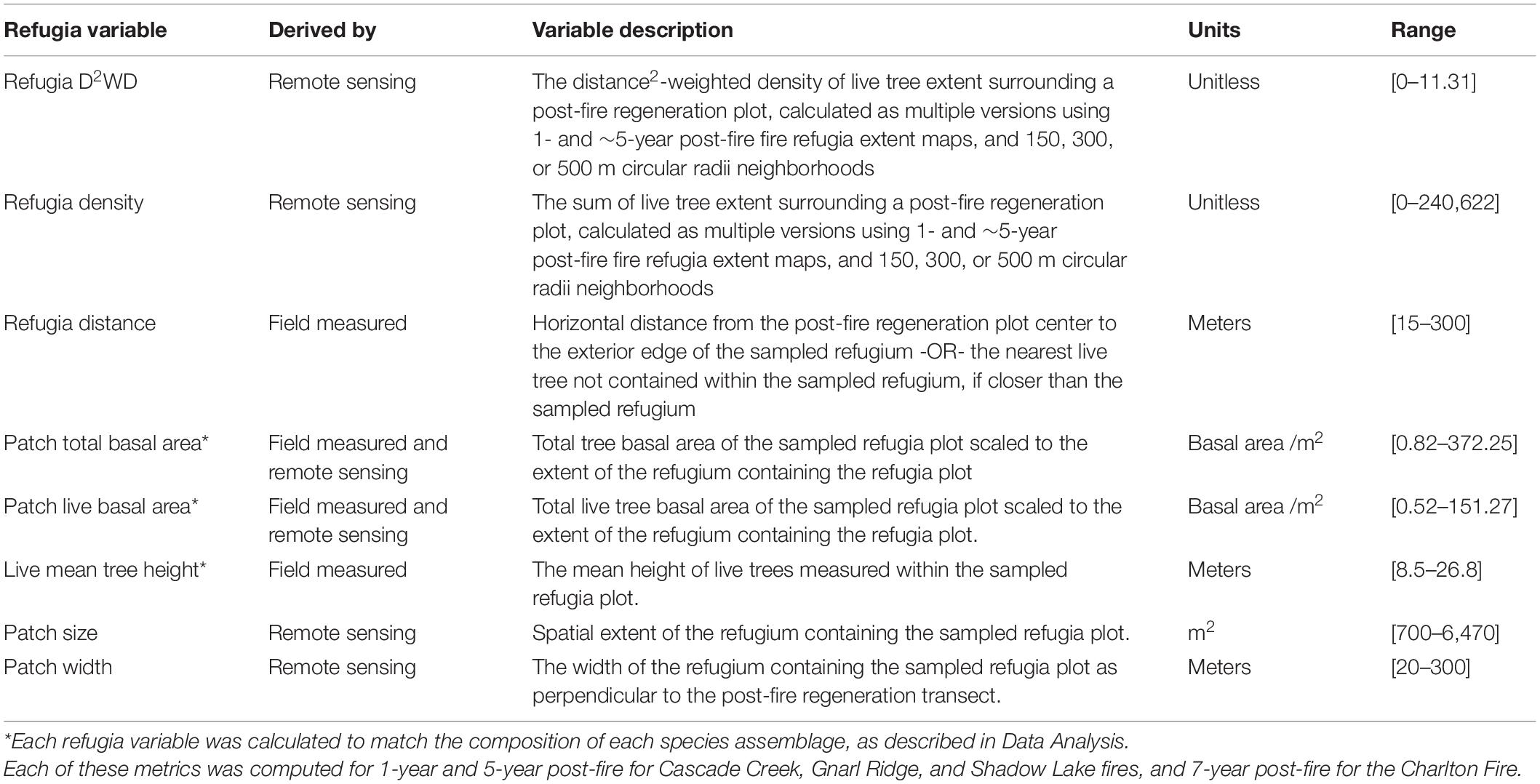
Table 1. Patch- and neighborhood-scale predictive variables associated with spatial, structural, and temporal attributes of fire refugia, as derived by field measurements, remote sensing, or both sources.
Data Analysis
To determine how post-fire conifer establishment and fire refugium attributes varied between study wildfires, we summarized observed median post-fire juvenile densities across post-fire regeneration sample plots, according to their distance from respective sampled refugium, across all fires combined and within individual wildfire perimeters. For all sampled refugium plots, we summarized species-level basal area/hectare (i.e., seed source composition and structure) across all fires combined and within individual wildfire perimeters.
To explore how estimates of forest structure, composition, and seed availability associated with fire refugia compare and interact as predictors of post-fire conifer establishment, and further, how they interact with and compare with site climate and environmental conditions as predictors of conifer establishment, we developed boosted regression tree (BRT) models, with a poisson error distribution, to explore relative variable importance, non-linear relationships, and interactions between sets of predictive variables and post-fire juvenile counts using the dismo package in R (R Core Team, 2020; Hijmans et al., 2021). Across modelling analyses described below, five species assemblage models were developed to represent the dominant species in our study areas and potential differences in adaptive traits between species, and thus post-fire establishment responses, including: (1) all species combined, (2) non-serotinous obligate seeding species (A. lasiocarpa; A. amabilis; L. occidentalis; P. engelmanni; P. monticola; T. mertensiana), (3) the potentially serotinous (e.g., Busby et al., 2020) lodgepole pine (P. contorta; PICO), (4) mountain hemlock (T. mertensiana; TSME), and (5) Abies (A. lasiocarpa; A. amabilis; Abies). Pacific-silver and subalpine fir juveniles were difficult to differentiate in the field by species, thus we combined them into a single Abies assemblage.
All BRT models were fit with a bag fraction setting of 0.5 to introduce stochasticity and learning rate of 0.01 to ensure at least 1000 trees were fit in each model (Elith et al., 2008). Tree complexity was set to one in the case of additive models (i.e., no interactions) and five in the case of interaction models. We fitted additive models when the modeling objective was to determine the best version of a single predictor and interaction models when determining relative importance of and interactions between sets of predictive variables. To account for the inherent spatial autocorrelation (SAC) in our sampling design between plots set along transects, and more broadly, plots set within the same wildfire perimeters, we computed and included a residual autocovariate (RAC) term in each final fitted BRT model following Crase et al. (2012). We used the autocov_dist function in the dismo package (Hijmans et al., 2021) to calculate RAC terms for each model, using a 3 km search neighborhood, equal weighting, and style “B” following Bardos et al. (2015). Model performance, or cross-validated (k = 10) total residual deviance explained (Leathwick et al., 2006), was reported between each BRT model with and without the RAC term fitted to illustrate model improvement due to accounting for SAC. Because BRTs are a stochastic modelling technique, we fitted 10 iterations of each model and reported results from the model with the highest cross-validated total residual deviance explained (i.e., best performing model iteration).
Our first modelling objective was to compare and determine the strongest performing version among the multiple calculated versions of our climate water deficit, refugia density, and refugia D2WD variables. Additive BRT models were fit on each species assemblage establishment response and all calculated versions of climate water deficit, refugia density, and refugia D2WD variables separately; relative variable importance was used to determine the version of each variable that exhibited the strongest relationship with each species assemblage post-fire establishment response. Our second modelling objective was to (a) compare the relative importance of fire refugia-related variables (Table 1), fitting only the strongest versions of refugia density and refugia D2WD as determined from the first analysis and (b) explore their interactions as predictors of conifer establishment. Interaction BRT models were fit on each species assemblage establishment response and all predictive variables from Table 1. Our third and final modeling objective was to compare the relative importance of and explore the interactions between the top three refugia-related variables determined from our second analysis, the top performing version of climate water deficit as determined from our first analysis, and a set of predictive variables describing biotic and abiotic site environmental conditions. These environmental variables included (1) percent remnant overstory canopy cover, as a proxy for shade, (2) percent understory vegetation cover, a proxy for competition or facilitation, (3) percent coarse downed woody debris cover, a proxy for facilitation, (4) topographic slope, (5) the heat load index (HLI), and (6) time since fire (sample year minus fire year; number of establishment opportunities).
Finally, to conceptually illustrate differences in spatial patterns of post-fire juvenile establishment across our study fire perimeters with distinctly different climate and topography, we fit an interaction BRT model on the juvenile establishment response of all species combined and a reduced set of explanatory variables with spatial data available across fire perimeters. Explanatory predictors included seed availability (refugia D2WD), climate (mean climate water deficit), and topography (slope and heat load index). Two spatial prediction maps of juvenile establishment density were generated respectively for the Shadow Lake and Gnarl Ridge fires for conceptual comparison using the raster package in R (Hijmans, 2021).
Results
Tree Establishment and Refugium Composition
Across fire perimeters, post-fire conifer establishment, as recorded in 2019, declined exponentially with increasing distance to fire refugium; the largest declines occurred between 15 and 90 m from refugium edges (Figure 2A). The rate (i.e. slope) of juvenile establishment decline over increasing distance was largest in the Shadow Lake Fire and lowest in the Gnarl Ridge Fire (Figures 2C,D), the warmest and driest, coolest and wettest sites, respectively. There was a wide range of variability among fires and among distances from refugium. At 300 m from refugium edges, total median conifer juvenile establishment was relatively low across all fires combined (∼250 juveniles/ha) yet potentially great enough to support forest recovery (e.g., Busby et al., 2020); responses varied substantially at the individual fire perimeter level, however (∼50 to ∼500 juveniles/ha). Closer to seed source, at 15 m from refugium edges specifically, total median juvenile establishment in the Charlton Fire was roughly two times higher than our other fires (Figure 2E); notably, the Charlton Fire was also the oldest fire by a factor of ∼2 (23 years since fire occurred). This relationship did not hold at distances greater than 15 m from refugium edges in the Charlton Fire, however.
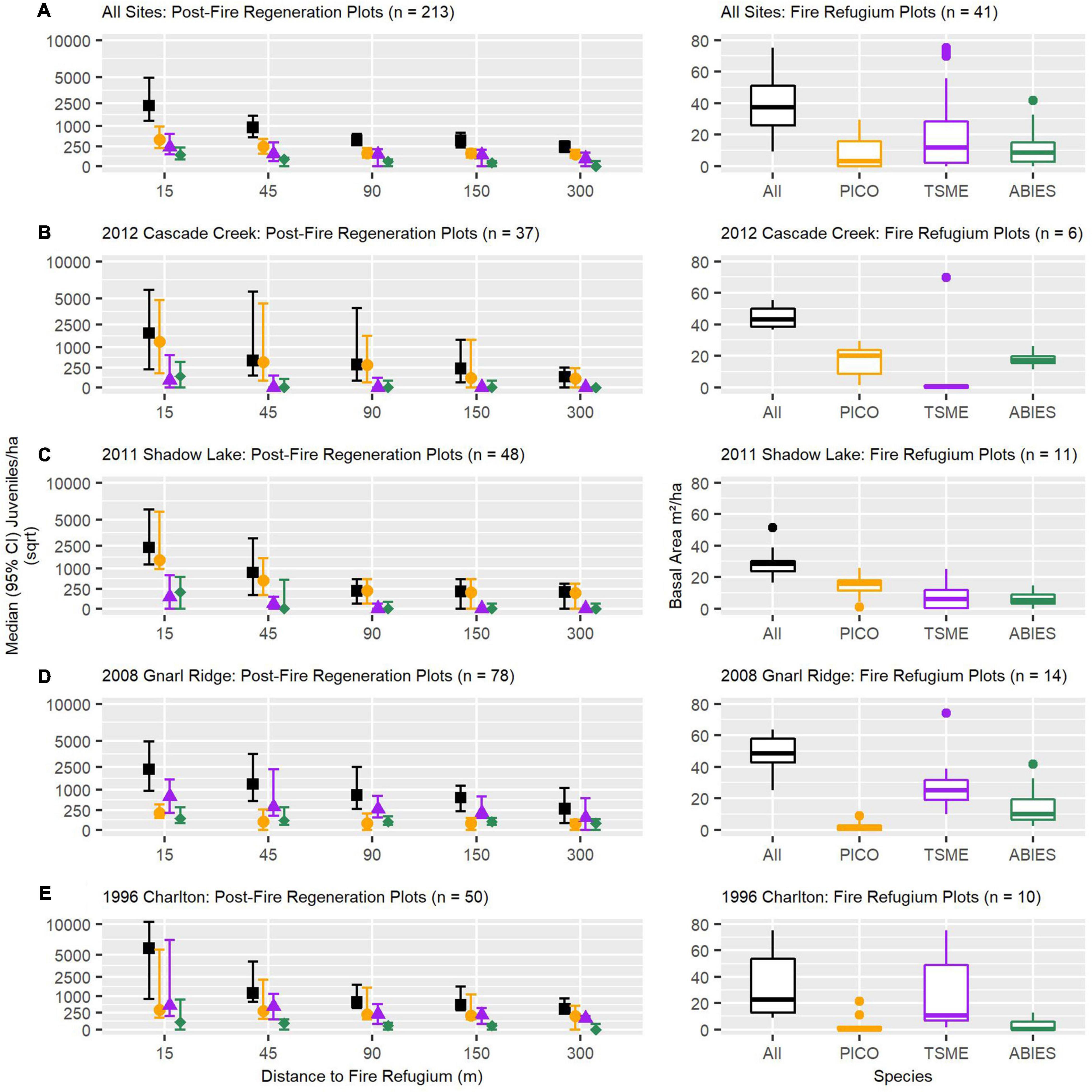
Figure 2. Observed post-fire median (95% confidence intervals) conifer establishment (density) as a function of increasing distance to refugium patch edge (left panels) and refugium patch basal area (right panels), represented across all and individual study fire perimeters (A–E), by dominant and all conifer species combined. TSME, mountain hemlock (Tsuga mertensiana); PICO, lodgepole pine (Pinus contorta); ABIES, pacific-silver fir and subalpine fir (Abies amabilis/lasiocarpa).
Post-fire conifer establishment by species generally reflected the composition of individual refugium. The Cascade Creek and Shadow Lake Fires were dominated by lodgepole pine (PICO) establishment, while a larger proportion of mountain hemlock (TSME) establishment followed the Gnarl Ridge and Charlton Fires (Figures 2B–E). Across fires, subalpine and Pacific-silver fir (Abies) establishment was very low even when these species were well represented within refugium as mature adults. Additionally, Abies species establishment reached zero or near zero at (and beyond) 45 m distance from any refugium edge.
Strongest Versions of Predictors
Among the three calculated versions of our climate water deficit (CWD) variable, our first modelling analysis determined that 30-year CWD was more important than post-fire mean or maximum CWD as a predictor of post-fire conifer establishment (density) across species assemblages (Supplementary Table 2). We also determined the strongest version (i.e., combination of 150, 300, or 500 m radii moving-window neighborhood and 1- or ∼5-year post-fire refugia extent snapshot) of our refugia density and refugia D2WD variables, which unlike CWD, varied by species assemblage response. For all species combined and the Abies assemblage, a 150m radius neighborhood and 1-year post-fire refugia extent snapshot were the strongest predictors of tree establishment; for non-serotinous species combined and mountain hemlock (TSME) a 500 m radius neighborhood and 1-year post-fire refugia extent snapshot were strongest, and for lodgepole pine (PICO) a 150 m radius neighborhood and ∼5-year post-fire refugia extent snapshot were strongest (Table 2).
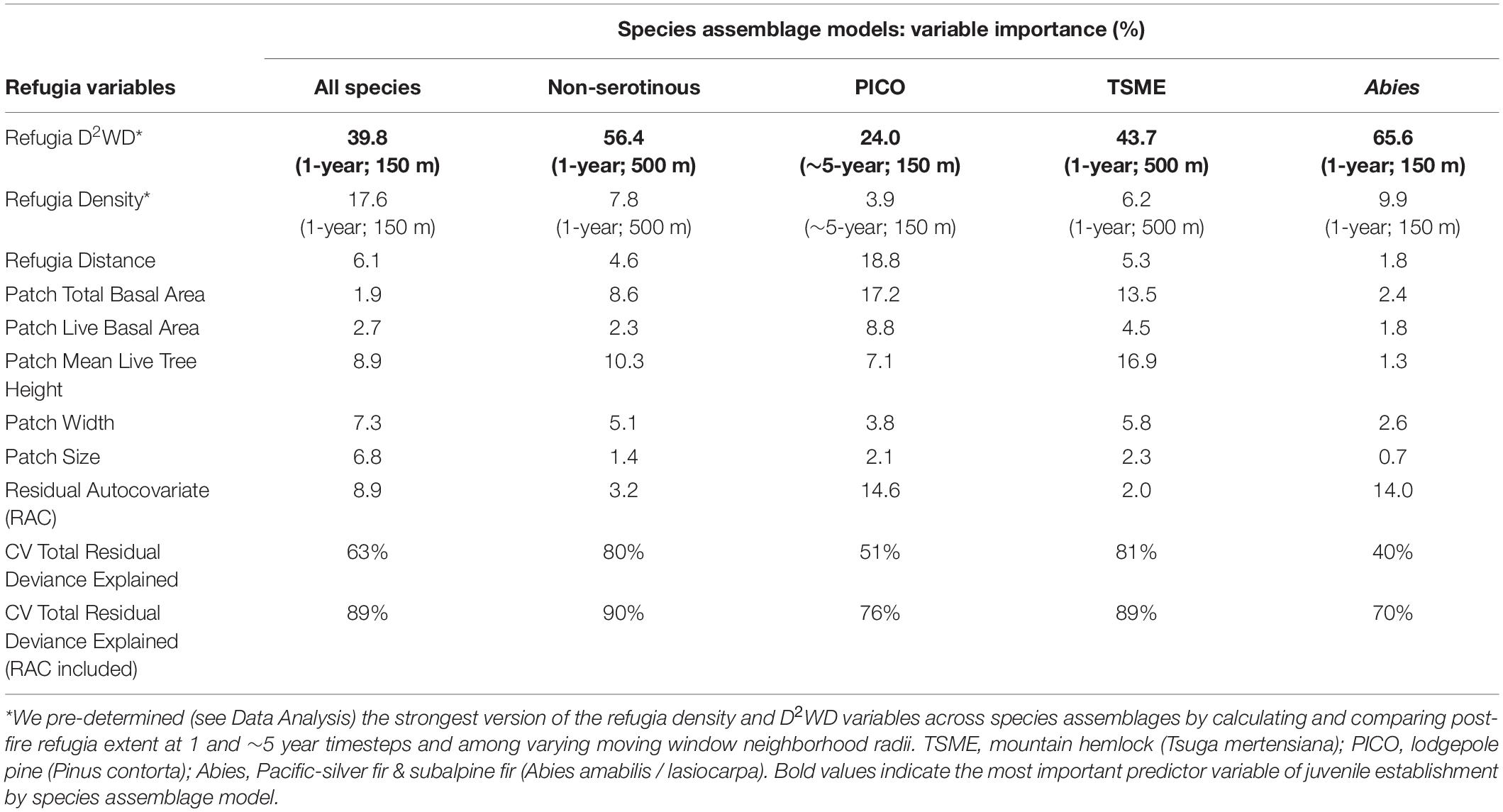
Table 2. Variable Importance and model performance from the BRT models evaluating relationships between observed conifer juvenile establishment and patch- or neighborhood-based estimates of fire refugia, by species assemblage.
Predictors of Tree Establishment and Interactions
Our second modelling analysis determined the top predictors of conifer juvenile establishment among our refugia-related seed availability metrics. For all species assemblages, refugia D2WD was the best predictor of post-fire conifer juvenile establishment, strongly outperforming other refugia-related variables derived from field measurements, remotely sensing, or combined sources (Table 2 and Supplementary Figure 2). From our field-based metrics, total refugium patch basal area was generally a better predictor of establishment than live basal area (at the time of sampling in 2019, 7–23 years post-fire), and was particularly important for lodgepole pine, in addition to refugia distance (Table 2). Mean live tree height within refugium was important for mountain hemlock, especially above ca. 16 m, to a lesser degree for non-serotinous species combined and lodgepole pine, and was unimportant for Abies species.
We observed the strongest pair-wise interactions among refugia variables and across species assemblages for (1) refugia distance and refugium live mean tree height, and (2) refugia distance and refugia D2WD (Figure 3). Taller live trees substantially increased juvenile establishment within ca. 90 m from refugium edges, but this relationship declined exponentially beyond ca. 90 m and was non-linear for lodgepole pine, where tree heights between ca. 14 and 19 m maximized establishment over heights that were lower or higher (Figure 3C). For all species combined, establishment was highest within ca. 90 m from refugia (Figure 3F), but at high values of refugia D2WD (e.g., ca. threshold of ∼2), establishment was still high regardless of refugia distance for all species assemblages, except lodgepole pine, where refugia distance exhibited a stronger influence than refugia D2WD (Figure 3H). At lower values of refugia D2WD, juvenile establishment declined much more strongly as refugia distance increased, compared to when refugia D2WD was held at higher values (Figure 3). Our third modelling analysis, comparing the top predictors of conifer juvenile establishment among the three best refugia-related metrics, best climate water deficit metric, and site environmental variables, determined that for all species combined and across species assemblages, refugia D2WD outweighed the importance of climate water deficit, topographic microclimate (i.e., slope and HLI), and post-fire biotic legacies (i.e., canopy cover, coarse woody debris cover, and understory vegetation cover) for conifer juvenile establishment (Table 3). The number of years from when fire occurred to time of field measurements was largely unimportant across models. Topographic microclimate, climate water deficit, and canopy cover was most important for explaining variability in lodgepole pine establishment. Coarse woody debris cover was important for non-serotinous species combined and mountain hemlock, while climate water deficit was important for the Abies species assemblage.
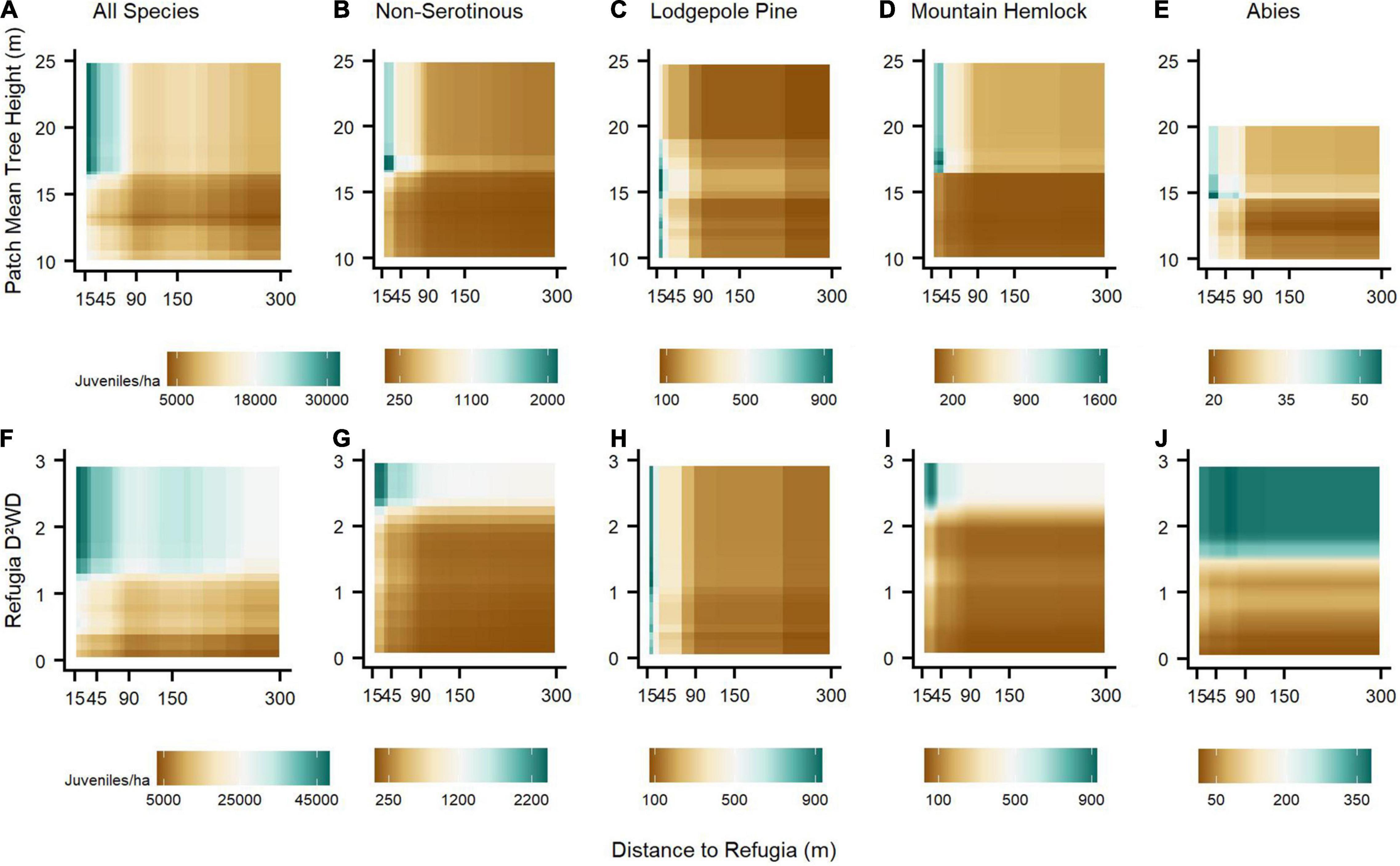
Figure 3. The strongest pair-wise interactions across species assemblage BRT models between three spatial or structural estimates of fire refugia: distance to refugia and (1) refugia patch mean live tree height (A–E), (2) refugia D2WD (F–J). Along the brown-to-blue-green color spectrum, brown shading indicates relatively reduced and blue-green shading relatively increased post-fire establishment due to pair-wise variable interactions.
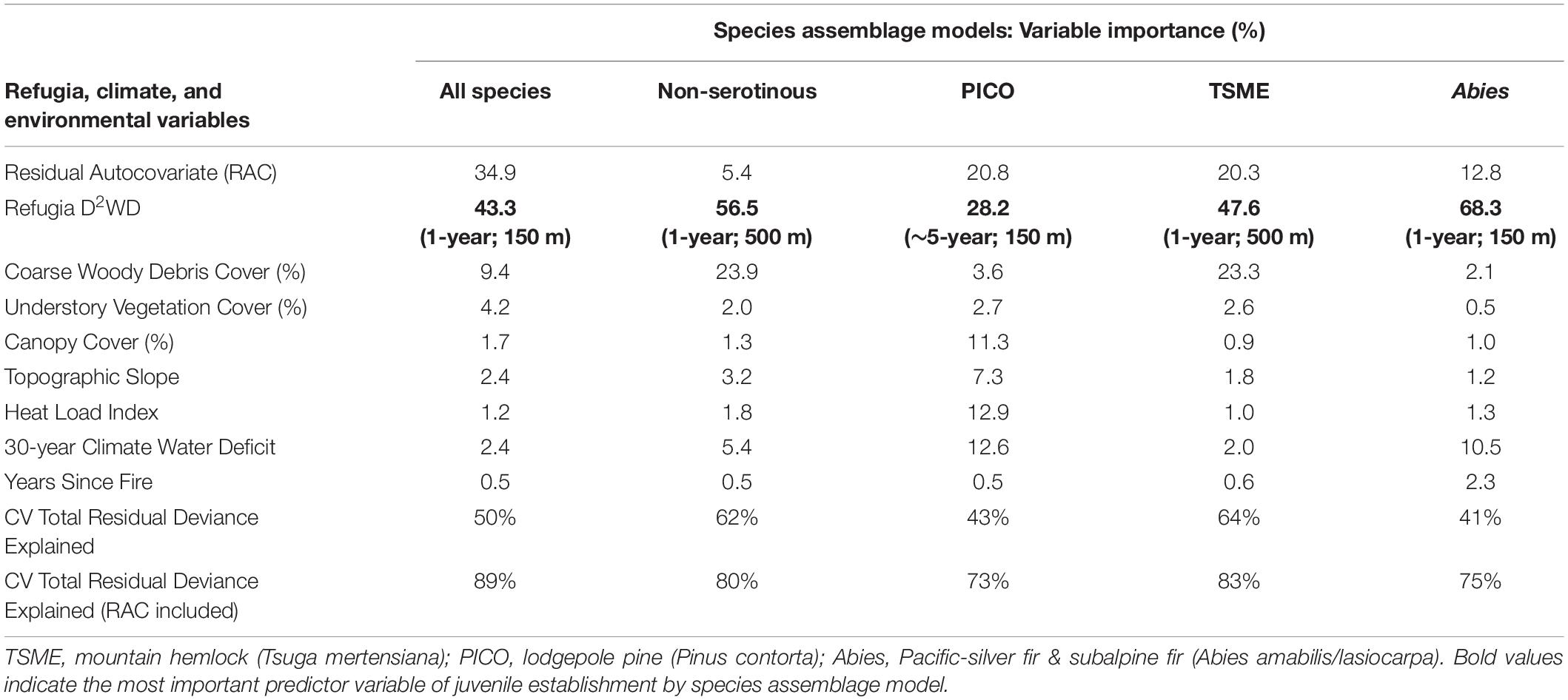
Table 3. Variable Importance and model performance from the BRT models evaluating relationships between observed conifer juvenile establishment and the best performing refugia-related, climate, and environmental predictors, by species assemblage.
Partial dependance plots illustrating relationships between predictive variables and species assemblage responses from our third analysis indicated some threshold-like relationships and some major differences in the response between lodgepole pine and other assemblages (Figure 4). Across assemblages, refugia D2WD generally had a much stronger effect on juvenile establishment than other variables (i.e., slope of establishment curves), and especially for the all species combined, non-serotinous species, and mountain hemlock assemblages. As refugia D2WD increased, juvenile establishment also increased exponentially up to a D2WD value threshold of ∼1 for lodgepole pine and ∼2–4 for other assemblages, after which increasing D2WD had no effect (Figure 4A). Further, and for lodgepole pine only, establishment under low values of refugia D2WD was still notably above zero (∼100 juveniles/ha). Lodgepole pine’s juvenile establishment increased under drier topographic settings (HLI), higher values of climate water deficit, and lower coarse woody debris cover in direct contrast to other assemblages (Figures 4B,E,F).
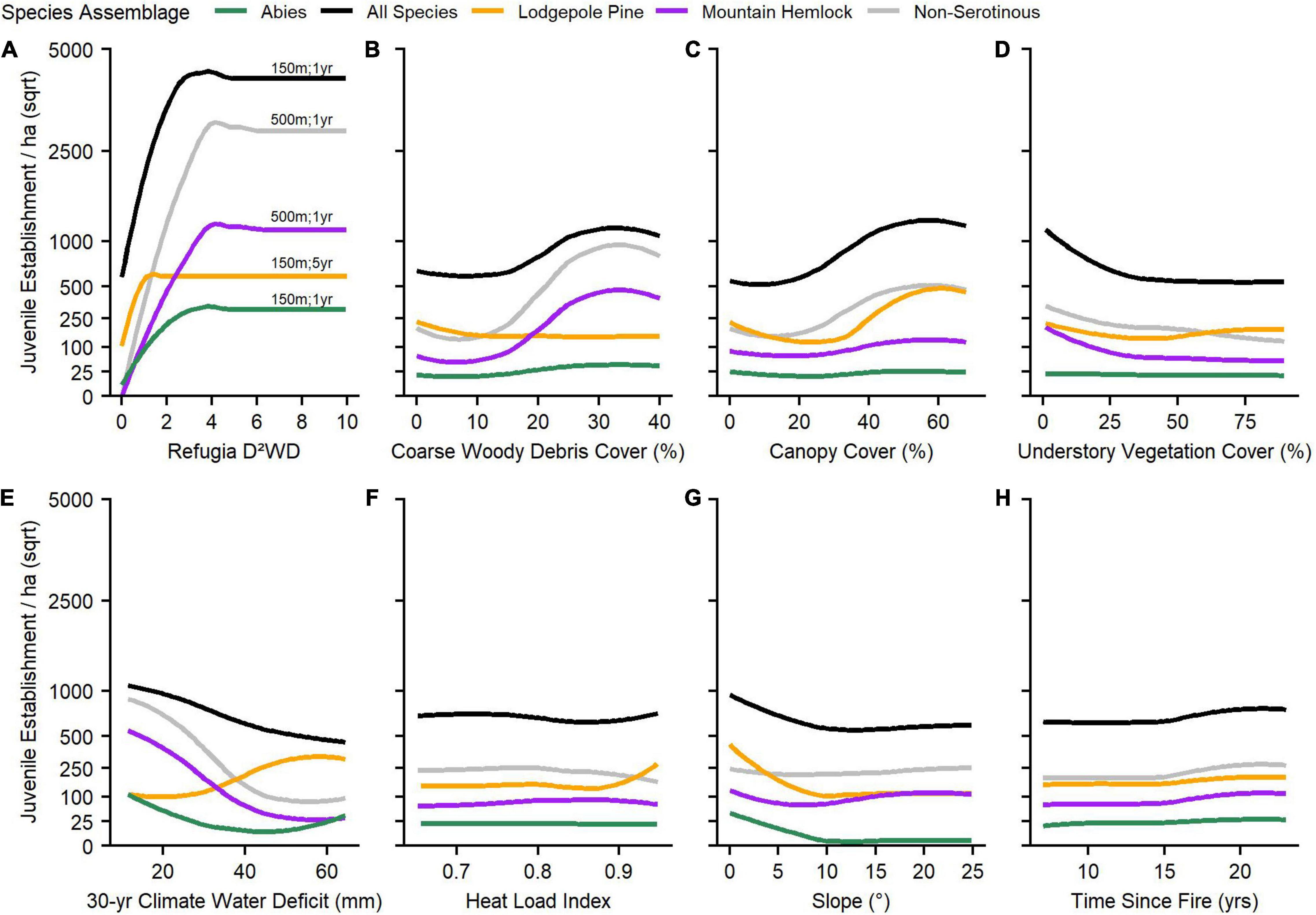
Figure 4. Partial dependence plots illustrating the smoothed flexible relationship between post-fire conifer juvenile establishment responses and refugia, climate, and environmental variables, by species assemblage (A–H). Each plot represents the fitted values of juvenile establishment response (y) due to the predictor variable (x), while keeping all other predictor variables fitted in models at their mean values. For the refugia D2WD variable, the best performing neighborhood radius and post-fire temporal snapshot associated with each species assemblage model is listed above each response curve (i.e., #m; #year). Refugia D2WD was the most important predictor of post-fire juvenile establishment responses across species assemblages (Table 3) and thus exhibited the greatest relative influence on establishment responses (y). Y-axes are shown in a square-root scale.
In our BRT models comparing refugia, climate, and environmental site variables combined, we observed the strongest pair-wise interactions across species assemblages between refugia D2WD and (1) climate water deficit, (2) coarse woody debris cover, and (3) canopy cover (Figure 5). At low levels of refugia D2WD (< ca. 1), increasing climate water deficit and decreasing coarse woody debris cover reduced establishment, whereas at high levels of D2WD the influence of these two variables on juvenile establishment was less important. Opposite to the other species assemblage responses, lodgepole pine establishment increased as climate water deficit increased and coarse woody debris cover decreased (Figures 5C,H). Alternatively, increasing canopy cover increased establishment for all species assemblages, as long as D2WD was above its threshold (between 1 and 2), except for lodgepole pine (Figures 5K–O). BRT model performance across species assemblages in the second and third analyses was high (70–90% CV total residual deviance explained) and generally greatly improved by calculating and including a residual autocovariate (RAC) term to account for spatial autocorrellation among sample plots (Tables 2, 3).
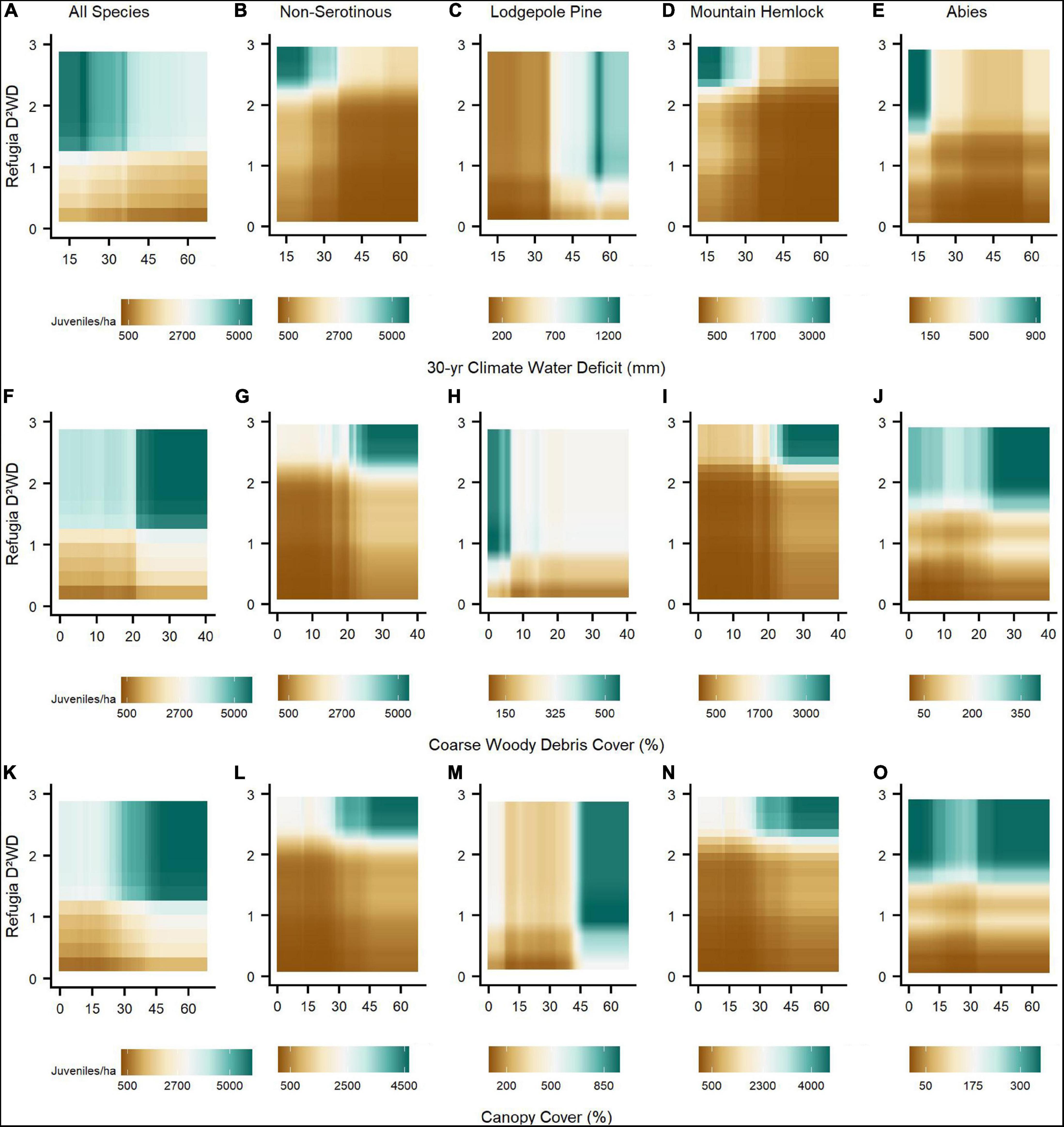
Figure 5. The strongest pair-wise interactions between refugia, climate, and environmental variables across species assemblage BRT models. Post-fire seed availability (refugia D2WD) interacted strongly with (1) 30-yr climate water deficit (mm; A–E), (2) coarse woody debris cover (%; F–J), and (3) canopy cover (%; K–O). Along the brown-to-blue-green color spectrum, brown shading indicates relatively reduced and blue-green shading relatively increased post-fire establishment due to pair-wise variable interactions. Refugia D2WD values ranged up to 10, but interaction responses did not change above a refugia D2WD value threshold of 3 across species assemblages.
Discussion
Toward quantifiying, predicting, and generalizing post-fire seed dispersal and tree establishment patterns from refugia after large stand-replacing fire(s), our study demonstrates the importance of capturing and quantifying the fine-grain extent and attributes of seed source legacies. Our findings suggest that within mesic subalpine conifer forests of the Pacific Northwest (PNW) Cascade Range, where the vast majority of species cannot resprout, post-fire conifer tree establishment after recent large, stand-replacing fires was primarily limited by landscape-level seed availability connected to the fine-grain spatial patterns of fire refugia, rather than to biophysical drivers (e.g. topography, climate, or understory vegetation). We found that for serotinous and non-serotinous wind-dispersed obligate seeding conifer species, post-fire juvenile density (i.e., a proxy for forest recovery from wildfire events) was largely explained by the distance2-weighted density (D2WD) of remnant live tree extent one-year post-fire, as derived from high-resolution aerial imagery. Species composition, stand structure, and the suite of functional traits expressed by conifer species within field-surveyed fire refugium further nuanced post-fire juvenile establishment responses. Although micro- and macro-scale biotic and abiotic environmental controls were overall less important predictors of juvenile establishment in our mesic study areas, they may be substantially important toward facilitating post-fire forest recovery within locations where seed availability is critically low and enviro-climatic enhancement or constrainment determine post-fire tree juvenile presence or absence.
Seed Availability and Tree Establishment
Seed dispersal patterns that contribute to post-fire conifer forest recovery in western North America, including the western United States, are strongly influenced by the arrangement of seed sources surrounding a site (Stevens-Rumann and Morgan, 2019), including the density of seed-bearing individuals over space and their individual distances to a site (Clark et al., 1999). Prior studies have generally focused on quantifying the influence of seed source distance and density separately, as metrics that relate to post-fire conifer forest recovery (e.g., Haire and McGarigal, 2010; Harvey et al., 2016; Kemp et al., 2016; Busby et al., 2020; Rodman et al., 2020), while others have attempted to combine seed source distance and density into a single metric (e.g., Coop et al., 2019; Downing et al., 2019). Among the refugia-related variables we analyzed describing spatial and structural attributes of patch- or neighborhood-level fire refugia, the refugia distance2-weighted density (D2WD) neighborhood metric strongly outweighed the importance of other refugia-related variables, including metrics that accounted for refugia distance or density separately. In dry to moist mixed-conifer forests of the Blue Mountains of Northeastern Oregon, Downing et al. (2019) also reported that refugia DWD outperformed simple distance to seed source metrics and was strongly correlated with post-fire forest recovery among non-serotinous conifer species. Among wind-dispersed species, empirical seed dispersal kernels (i.e., percent of seeds dispersed along a gradient of distance from source) typically take the form of a negative exponential probability density function, in which seed rain declines exponentially over increasing distances from sources (McCaughey et al., 1986; Clark et al., 1999). Thus, it is a commonly observed phenomena that post-fire juvenile densities decline rapidly at increasing distances from live, seed-bearing individual(s) for non-serotinous, obligate seeding tree species (Harvey et al., 2016; Kemp et al., 2016; Stevens-Rumann and Morgan, 2019; Busby et al., 2020). In dry mixed-conifer forests of the Southwestern United States, including data aggregated from Downing et al. (2019) in similar dry forest types, Coop et al. (2019) discovered that refugia DWD with a squared distance term (D2WD) was a stronger predictor of post-fire forest recovery than without, including this study, presumably due to accounting for exponential decay in seed dispersal over increasing distances from the source tree(s). These results illustrate the vital importance of accurately capturing and accounting for different spatial factors that affect seed dispersal processes when modelling and predicting post-fire establishment responses.
The strength of neighborhood metrics when modeling dispersal processes is often tied to species-specific traits, thus, experimentation with multiple neighborhoods among species with varying traits is standard protocol (Turner and Gardner, 2015). For dry-mixed conifer species (e.g., ponderosa pine and Douglas-fir), effective neighborhood radii calculated on dispersal-related neighborhood metrics assessed by previous studies have ranged 60–240 m, but have generally found that 100–150 m effectively captures dispersal patterns for these conifer species (e.g., Haire and McGarigal, 2010; Coop et al., 2019; Downing et al., 2019; Rodman et al., 2020). We found some variation in the effective dispersal neighborhoods across our species assemblage models, likely tied to the dispersal capabilities of subalpine species in our study areas, which were further supported by observed differences in post-fire establishment at increasing distances to sampled fire refugium patches among our species (Figure 2). All species combined, lodgepole pine, and Abies species’ post-fire establishment was best captured by a 150 m radius neighborhood, while a 500 m radius was best for mountain hemlock and all non-serotinous species combined (i.e., excluding lodgepole pine). This pattern was consistent between both D2WD and refugia density metrics. These observed and modelled differences indicate that some relatively fire sensitive species (e.g., mountain hemlock) in our subalpine study areas exhibit strong seed dispersal abilities that support juvenile establishment further into the interior of severely burned forest patches than others (e.g., subalpine and Pacific-silver fir; Busby et al., 2020). Thus, species composition of fine-scale fire refugia may initiate broadly different post-fire forest recovery responses.
Fire Refugia, Climate, and Environment
Post-fire tree establishment and persistence responses can be understood using the concept of environmental filtering, where dispersal limitations, then environmental conditions, then competition control species compositions over time (Crisafulli et al., 2015; Kraft et al., 2015). While dispersal limitations appeared to drive the majority of variance in post-fire conifer establish responses in our cool and mesic subalpine study areas, the non-linear relationship observed between establishment responses and refugia D2WD (e.g., Figure 4A) indicated that many sites relatively close to fire refugium (15–45 m) reached a point of seed saturation. This is to say, that increasing seed availability (i.e., greater density of nearer fire refugia) did not increase establishment responses beyond a species-specific threshold of D2WD, which likely varied based on differences in functional traits between species, and thus responses in those sites were primarily driven by climate and environmental controls (i.e., an environmental filter), and potentially competition by understory vegetation cover (i.e., a community interaction filter; Figure 4D). Although lodgepole pine experienced a weaker relationship with refugia D2WD than other species assemblages, this relationship was still significant and indicative of a mostly non-serotinous establishment response. In contrast, lodgepole pine establishment has been weakly or negatively correlated with refugia DWD and D2WD within dry to moist mixed-conifer forests elsewhere, (e.g., Coop et al., 2019; Downing et al., 2019). Although broad empirical evidence is limited, the post-fire establishment response of lodgepole pine in our high-elevation study areas suggests that the production of serotinous cones among lodgepole pine decreases with elevation in the Cascade Range (Busby et al., 2020), similar to findings in the N. Rockies, where decreasing elevation is still related to high severity fire regimes but with shorter fire return intervals and thus greater selective pressure towards serotinous cones among lodgepole pine (Lotan, 1976; Tinker, 1994; Schoennagel et al., 2003). The saturation point of refugia D2WD for lodgepole pine was also lower than other species assemblages (i.e., fewer seed sources are needed to facilitate a specific establishment response compared to other species), which may indicate in our subalpine study areas that the species is a prolific producer of non-serotinous (open cone) wind-dispersed seed crops (OECD, 2010).
Species-level dispersal traits are further augmented at the individual and stand scales by forest structure, where older, larger, and taller trees typically produce greater quantities of seeds (Clark et al., 1999; Kroiss and HilleRisLambers, 2015; Andrus et al., 2020) until senescence (Qiu et al., 2021), and disperse them further than younger trees (Gill et al., 2020). Strong pair-wise interactions in our statistical models indicated that mean tree height among fire refugium boosted juvenile establishment along a gradient of increasing distance from refugium edges when mean height was approx. greater than 15 m (Figure 3). As forests with large, tall trees are progressively lost due to fire and drought (Abatzoglou and Williams, 2016; Stovall et al., 2019) under warming climate conditions in the western United States, refugia increasingly composed of young and short trees may have greater difficulty dispersing seeds into the interior of large patches of high-severity fire than older refugia trees (Hart et al., 2019; Gill et al., 2020) with implications for forest recovery.
For landscape-scale processes such as post-fire forest recovery, a keen understanding of how dispersal processes interact with climate and environmental conditions is key to understanding and predicting forest resilience to increasingly large, severe, and frequent wildfires (Davis et al., 2019; Coop et al., 2020; Gill et al., 2022). Evidence from this study suggest that climate and biotic environmental legacies (e.g., climate water deficit, canopy cover, coarse woody debris cover; Figure 5) interact with dispersal processes to enhance or constrain juvenile establishment responses along gradients of seed availability, which are further influenced by the fine-grain structure, composition, and spatial configuration of proximate seed source legacies (Figure 6). Relationships between seed dispersal and tree establishment may be highly landscape-dependant, where environmental and climate conditions can constrain (arid; Figure 6B) or enhance (mesic; Figure 6C) establishment responses given the same level of seed availability. Although rare dispersal events (i.e., the thin right-skewed tail-end of a dispersal kernel; e.g., bottom y-axis of Figure 6A) make up a small overall proportion of total seeds dispersed, they may be disproportionally important for biogeographic processes (Clark et al., 1998; e.g. landscape-level population persistence, Morelli et al., 2020), where the difference between zero and marginal establishment leads to either long-term ecosystem state transitions or slow but eventual recovery. Thus, in the case of wind-dispersed conifer species, the degree to which climate and environmental conditions augment dispersal kernels (e.g., Figure 6A) may determine if and how forests recover after spatially expansive and contigious stand-replacing, high-severity wildfires.
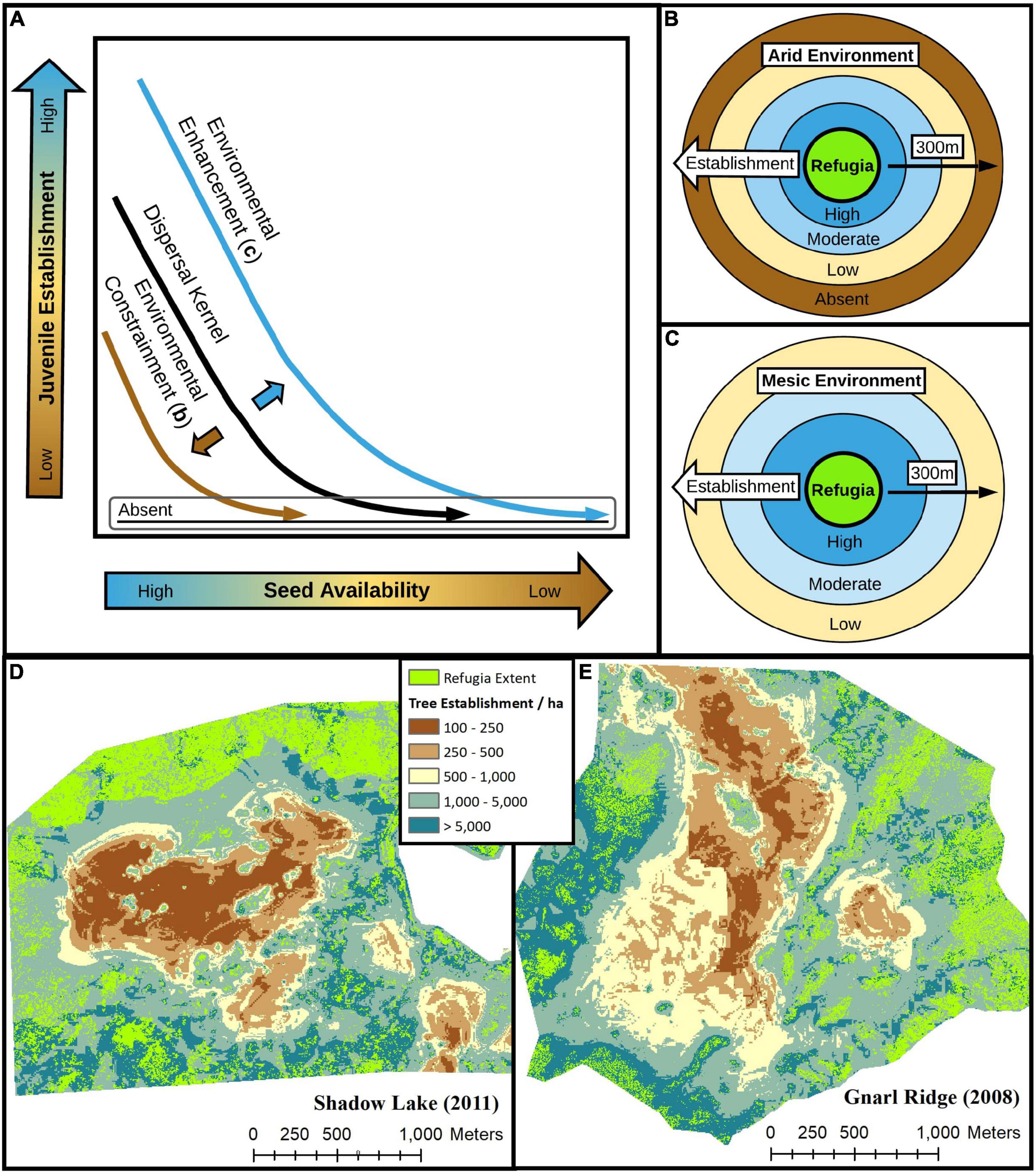
Figure 6. For wind-dispersed tree species along a gradient of seed availability, post-fire juvenile establishment responses are determined by interactions between biotic and abiotic environmental controls and the spatial and structural (e.g., age, size, height) configuration of seed sources, including fire refugia, at the landscape scale. Across gradients of water deficit to vegetation explained by macro-scale climatic and micro-scale topographic and biotic features, arid environments (B) constrain while mesic environments (C) enhance dispersal kernels (A) and thus juvenile establishment responses at increasing distances from fire refugia for most species, when refugia extent and structure remain constant. (D,E) Variability in modeled juvenile density patterns over space of all species combined (D,E) illustrate the important influence of and interactions between seed availability (refugia D2WD), mean climate conditions (climate water deficit), and topography (slope and heat load index) on post-fire tree establishment responses in panel (D) the warmer, drier and topographically flatter 2011 Shadow Lake Fire and (E) the cooler, wetter, and topographically steeper 2008 Gnarl Ridge Fire.
Post-fire Delayed Tree Mortality
Seed dispersal patterns influencing post-fire forest recovery are linked to the persistence of fire refugia over time, where seed dispersal, and thus tree establishment, may decline over time as sexually mature individuals die and are not replaced. If climate-environment conditions do not favor abundant seed production and juvenile establishment early post-fire (e.g., Stevens-Rumann et al., 2018; Davis et al., 2019; Kemp et al., 2019), delayed tree mortality and/or compound disturbances may eliminate fire refugia before they can initiate an establishment response that is sufficient for long-term forest recovery (Coop et al., 2020). We modelled the influence of fire refugia extent 1- and ∼5-years post-fire on conifer establishment responses and primarily observed that fire refugia extent 1-year post-fire was a superior predictor of tree establishment (Table 2). Further, the number of years between when a fire occurred and when we sampled sites (i.e., number of opportunities for establishment; time since fire; 7–23 years) was largely unimportant as a predictor of post-fire juvenile densities; climate water deficit calculated over a 30-year period was also a stronger predictor of establishment than immediate (1–5 years) post-fire conditions. These results may indicate that climate-environment conditions in our relatively mesic and cool subalpine study areas (i.e., low water deficit to vegetation) generally support early post-fire pulses of juvenile establishment, assuming seed availability is not severely limited (Harvey et al., 2016). Other factors, such as substrate quality and lack of competition may also favor early pulses vs. slow protracted post-fire tree establishment responses (Agee, 1993); understory vegetation recovery (i.e, competition) was generally very slow in our subalpine sites, even up to 23 years post-fire, however, and did not appear to be a major factor limiting tree establishment (Figure 4D and Table 3). Although our evidence is limited, our results suggest that climate variability and post-fire delayed tree mortality patterns may be less influential processes affecting forest recovery trajectories in cool and mesic forests, than in drier and warmer forests elsewhere (i.e., intermountain western United States). To improve understanding of how post-fire delayed tree mortality affects seed dispersal processes and forest recovery, further work involving multiple lines of evidence are needed to evaluate the relationship between post-fire establishment responses and fire refugia extent, over time, across broader geographic extents and climate-environment gradients (i.e., drier and warmer).
Limitations and Opportunities
Fine-grain quantification of seed availability via high-resolution remote sensing data and high-accuracy imagery classification methods, as illustrated by this study, strengthen our ability test spatial and biotic relationships and predict post-fire ecosystem recovery trajectories. These methods are not without their caveats, however. While highly accurate, supervised imagery classification methods used in this and prior studies (e.g., Coop et al., 2019; Downing et al., 2019; Walker et al., 2019) are non-automated, time consuming, rely on user-objectivity, and may lose accuracy when training samples are drawn from and assessments conducted over large spatial extents with topographically and ecologically heterogenous landscapes (Wulder et al., 2009). Further, high spatial resolution imagery products also entail high data requirements, slowing processing and classification time when the researcher’s aim is to assess large or many landscapes. Thus, while useful for case studies where analysis time and effort is not significantly limited, they are less likely to be used by land managers in their raw form due to limitations associated with accessibility, efficiency, and interpretability. Greater accessibility of these methods to a broader audience, including managers, would be enhanced by automated imagery classification workflows and toolsets programmed for user-friendly statistical (e.g., R Shiny) and/or geographic information system (GIS; ArcGIS) applications.
While moving-window neighborhood based seed availability metrics used in this study and others are significant improvements over simple distance to edge or patch metrics for modelling dispersal processes and tree establishment responses, they do not account for the biotic structure or composition of seed sources in their present form, which strongly influence seed availability dynamics over space and time (e.g., Gill et al., 2020). Current refugia seed availability metrics, like refugia D2WD, could be improved by moving from a binary seed source presence or absence classification to a weighted approach. In the context of this study, pixels first classified as refugia presence could be weighted by either structural attributes (e.g., tree height, basal area, or age) or compositional attributes (e.g., percent species composition). This type of approach would rely on secondary fine-to-moderate resolution spatial data of forest structure or composition, which is becoming increasingly available in the CONUS (e.g., LANDFIRE; LEMMA GNN).
Finally, in this study, we did not consider topographic complexity, prevailing wind patterns, or snowpack dynamics as they relate to dispersal processes. Topographic complexity can enhance or inhibit dispersal processes and additionally interact with wind patterns (Peeler and Smithwick, 2020). Topographic complexity was generally low within our study areas and is more broadly low within high-elevation forests of the Central Cascade range, which may reduce spatial variability in dispersal processes relative to other more topographically heterogenous landscapes. Although not well empirically studied, winter snowpack in our high-elevation study areas may further enhance the dispersal range of select species, whereby seeds dispersed during winter can “skim” past their initial ground-contact point by gliding on snowpack during surface wind gusts (Matlack, 1989; Agee, 1993). Theoretically, the low topographic complexity of our study areas would facilitate improved secondary-dispersal of seeds over snowpack vs. more topographically complex landscapes and especially at lower elevations (i.e., less snow), where seeds are more likely to become trapped by topographic variability and features.
Reforestation
When management objectives support maintaining forested areas at specified stocking levels (tree density and potentially species composition) over space and time, replanting severely burned forests may be a necessary action to mitigate forest losses when local seed source is functionally absent, local seed source species are not desired given timber or restoration needs (e.g., grand fir; Abies grandis), and/or warming climate conditions do not favor natural tree establishment (Coop et al., 2020; Larson et al., 2022). Few spatially-sophisticated tools have been developed to aid land managers in post-fire replanting efforts, although some region-specific web applications exist (e.g., Rodman et al., 2021; Stewart, 2021). Effective seed dispersal distance thresholds (e.g., 100–250 m distance from live forest edge) are commonly used in conjunction with moderate-resolution (30 m) burn severity products (e.g., MTBS burn severity maps) to spatially target burned areas for replanting (Shive et al., 2018; Stevens-Rumann and Morgan, 2019). Moderate-resolution imagery products largely fail to capture fine-grain post-fire tree survival patterns (i.e., fire refugia extent; Walker et al., 2019) that strongly influence tree regeneration responses over space (Coop et al., 2019). Further, results from this study illustrate how site climate and fine-grain biotic and abiotic factors can strongly influence natural tree establishment density over space, especially when seed availability is low. Thus, while easy to calculate and apply, the use of simple distance threshold to live forest edge maps, especially in the absence of local climate and topographic context and data, may lead to negative management consequences, including (1) wasted resources where local site conditions and fine-grain refugia extent, composition, and structure naturally facilitate forest recovery within replanted areas and (2) replanted areas develop overly dense stands when compounded by abundant natural regeneration, effectively increasing stand competition, mortality, spread of disease/insect vectors, and even increased future fire severity (Larson et al., 2022).
As an example, post-fire tree establishment density prediction maps between two climatically and topographically distinct burn perimeters (Figures 6D,E), in this study, illustrate the high variability in tree establishment density responses that can occur over space due to fine-grain patterns of seed availability (Coop et al., 2019; Downing et al., 2019) and local factors (Peeler and Smithwick, 2021). By utilizing and building upon indices of fine-grain seed availability (e.g., refugia D2WD) and incorporating spatially explicit climatic, topographic, and biotic (as available) data, spatially-sophisticated tools can be developed and implemented to help land managers better achieve post-fire forest management goals, minimize costs associated with reforestation, and avoid unintended consequences associated with unintentionally overstocking stands.
Data Availability Statement
The raw data supporting the conclusions of this article will be made available by the authors, without undue reservation.
Author Contributions
SB collected field data, designed the study, conducted data analysis, and wrote the manuscript with oversight, support, and manuscript editing from AH. Both authors contributed to the article and approved the submitted version.
Funding
Funding for this research was provided by the National Science Foundation (NSF awards EAR-1738104 and GSS-1832483).
Conflict of Interest
The authors declare that the research was conducted in the absence of any commercial or financial relationships that could be construed as a potential conflict of interest.
Publisher’s Note
All claims expressed in this article are solely those of the authors and do not necessarily represent those of their affiliated organizations, or those of the publisher, the editors and the reviewers. Any product that may be evaluated in this article, or claim that may be made by its manufacturer, is not guaranteed or endorsed by the publisher.
Acknowledgments
We thank J. Ibarra and B. Michie for their assistance in collecting field data and performing data entry. C. Evers and M. Krawchuk provided helpful feedback that improved the quality and scope of this work. Field site access was graciously provided by the Gifford Pinchot, Mt. Hood, and Willamette National Forests (USFS).
Supplementary Material
The Supplementary Material for this article can be found online at: https://www.frontiersin.org/articles/10.3389/ffgc.2022.890893/full#supplementary-material
References
Abatzoglou, J. T., and Williams, A. P. (2016). Impact of anthropogenic climate change on wildfire across western US forests. Proc. Natl. Acad. Sci. 113, 11770–11775. doi: 10.1073/pnas.1607171113
Agee, J. K., and Smith, L. (1984). Subalpine Tree Reestablishment After Fire in the Olympic Mountains. Ecology 65, 810–819. doi: 10.2307/1938054
Andrus, R. A., Hart, S. J., Tutland, N., and Veblen, T. T. (2021). Future dominance by quaking aspen expected following short-interval, compounded disturbance interaction. Ecosphere 12:1. doi: 10.1016/j.foreco.2012.11.008
Andrus, R. A., Harvey, B. J., Hoffman, A., and Veblen, T. T. (2020). Reproductive maturity and cone abundance vary with tree size and stand basal area for two widely distributed conifers. Ecosphere 11:5. doi: 10.1002/ecs2.3092
Andrus, R. A., Harvey, B. J., Rodman, K. C., Hart, S. J., and Veblen, T. T. (2018). Moisture availability limits subalpine tree establishment. Ecology 99, 567–575. doi: 10.1002/ecy.2134
Baker, W. L. (2009). Fire ecology in Rocky Mountain landscapes. 1st ed. Washington, DC: Island Press.
Bardos, D. C., Guillera-Arroita, G., and Wintle, B. A. (2015). Valid auto-models for spatially autocorrelated occupancy and abundance data. Methods Ecol. Evol. 6, 1137–1149. doi: 10.1111/2041-210x.12402
Blomdahl, E. M., Kolden, C. A., Meddens, A. J. H., and Lutz, J. A. (2019). The importance of small fire refugia in the central Sierra Nevada. California, USA. Forest Ecol. Manag. 432, 1041–1052. doi: 10.1016/j.foreco.2018.10.038
Brown, M. J., Kertis, J., and Huff, M. H. (2013). Natural tree regeneration and coarse woody debris dynamics after a forest fire in the western Cascade Range. Portland, OR: U.S. Department of Agriculture, Forest Service, Pacific Northwest Research Station. doi: 10.2737/PNW-RP-592
Buma, B., Brown, C., Donato, D. C., Fontaine, J. B., and Johnstone, J. F. (2013). The Impacts of Changing Disturbance Regimes on Serotinous Plant Populations and Communities. BioScience 63, 866–876. doi: 10.1525/bio.2013.63.11.5
Burns, R. M., and Honkala, B. H. (1990). Silvics of North America: Volume 1. Conifers. Washington, DC: U.S. Dept. of Agriculture, Forest Service.
Busby, S. U., Moffett, K. B., and Holz, S. (2020). High-severity and short-interval wildfires limit forest recovery in the Central Cascade Range. Ecosphere 11:22. doi: 10.1002/ecs2.3247
Chaney, N. W., Wood, E. F., McBratney, A. B., Hempel, J. W., Nauman, T. W., Brungard, C. W., et al. (2016). POLARIS: A 30-meter probabilistic soil series map of the contiguous United States. Geoderma 274, 54–67. doi: 10.1186/s13104-021-05729-y
Clark, J. S., Fastie, C., Hurtt, G., Jackson, S. T., Johnson, C., King, G. A., et al. (1998). Reid’s Paradox of Rapid Plant Migration. BioScience 48, 13–24. doi: 10.1016/j.jtbi.2005.12.019
Clark, J. S., Silman, M., Kern, R., Macklin, E., and HilleRisLambers, J. (1999). Seed dispersal near and far: patterns across temperate and tropical forests. Ecology 80, 1475–1494. doi: 10.1890/0012-9658(1999)080[1475:sdnafp]2.0.co;2
Congalton, R. G. (1991). A review of assessing the accuracy of classifications of remotely sensed data. Remote Sens. Env. 37, 35–46. doi: 10.1016/0034-4257(91)90048-b
Coop, J. D., DeLory, T. J., Downing, W. M., Haire, S. L., Krawchuk, M. A., Miller, C., et al. (2019). Contributions of fire refugia to resilient ponderosa pine and dry mixed-conifer forest landscapes. Ecosphere 1:7.
Coop, J. D., Parks, S. A., Stevens-Rumann, C. S., Crausbay, S. D., Higuera, P. E., Hurteau, M. D., et al. (2020). Wildfire-Driven Forest Conversion in Western North American Landscapes. BioScience 2020:biaa061. doi: 10.1093/biosci/biaa061
Crase, B., Liedloff, A. C., and Wintle, B. A. (2012). A new method for dealing with residual spatial autocorrelation in species distribution models. Ecography 35, 879–888. doi: 10.1111/j.1600-0587.2011.07138.x
Crisafulli, C. M., Swanson, F. J., Halvorson, J. J., and Clarkson, B. D. (2015). “Volcano Ecology: Disturbance Characteristics and Assembly of Biological Communities,” in The Encyclopedia of Volcanoes, Chap. 73, ed. H. Sigurdsson (Amsterdam: Academic Press), 1265–1284. doi: 10.1016/B978-0-12-385938-9.00073-0
Daly, C., Halbleib, M., Smith, J. I., Gibson, W. P., Doggett, M. K., Taylor, G. H., et al. (2008). Physiographically sensitive mapping of climatological temperature and precipitation across the conterminous United States. Internat. J. Climat. 28, 2031–2064. doi: 10.1002/joc.1688
Davis, K. T., Dobrowski, S. Z., Higuera, P. E., Holden, Z. A., Veblen, T. T., Rother, M. T., et al. (2019). Wildfires and climate change push low-elevation forests across a critical climate threshold for tree regeneration. Proc. Natl. Acad. Sci. 113, 6193–6198. doi: 10.1073/pnas.1815107116
Downing, W. M., Krawchuk, M. A., Meigs, G. W., Haire, S. L., Coop, J. D., Walker, R. B., et al. (2019). Influence of fire refugia spatial pattern on post-fire forest recovery in Oregon’s Blue Mountains. Landscape Ecol. 34:12. doi: 10.1007/s10980-019-00802-1
Eidenshink, J., Schwind, B., Brewer, K., Zhu, Z.-L., Quayle, B., and Howard, S. (2007). A Project for Monitoring Trends in Burn Severity. Fire Ecol. 3, 3–21. doi: 10.4996/fireecology.0301003
Elith, J., Leathwick, J. R., and Hastie, T. (2008). A working guide to boosted regression trees. J. Anim. Ecol. 77, 802–813. doi: 10.1111/j.1365-2656.2008.01390.x
Franklin, J. F., and Dyrness, C. T. (1973). Natural Vegetation of Oregon and Washington. PNW-8: U.S. Washington, DC: Dept. of Agriculture, Forest Service, Pacific Northwest Research Station.
Gergel, D. R., Nijssen, B., Abatzoglou, J. T., Lettenmaier, D. P., and Stumbaugh, M. R. (2017). Effects of climate change on snowpack and fire potential in the western USA. Clim. Chan. 141, 287–299. doi: 10.1007/s10584-017-1899-y
Gill, N. S., Hoecker, T. J., and Turner, M. G. (2020). The propagule doesn’t fall far from the tree, especially after short-interval, high-severity fire. Ecology 33:e03194. doi: 10.1002/ecy.3194
Gill, N. S., Turner, M. G., Brown, C. D., Glassman, S. I., Haire, S. L., Hansen, W. D., et al. (2022). Limitations to Propagule Dispersal Will Constrain Postfire Recovery of Plants and Fungi in Western Coniferous Forests. BioScience XX, 1–18. doi: 10.1093/biosci/biab139
Haire, S. L., and McGarigal, K. (2010). Effects of landscape patterns of fire severity on regenerating ponderosa pine forests (Pinus ponderosa) in New Mexico and Arizona. Landsc. Ecol. 25, 1055–1069. doi: 10.1007/s10980-010-9480-3
Halofsky, J. E., Peterson, D. L., and Harvey, B. J. (2020). Changing wildfire, changing forests: the effects of climate change on fire regimes and vegetation in the Pacific Northwest. Fire Ecol. 16:4.
Halofsky, J. S., Conklin, D. R., Donato, D. C., Halofsky, J. E., and Kim, J. B. (2018). Climate change, wildfire, and vegetation shifts in a high-inertia forest landscape: Western Washington, U.S.A. ed. C. Carcaillet. PLoS One 13:e0209490. doi: 10.1371/journal.pone.0209490
Hart, S. J., Henkelman, J., McLoughlin, P. D., Nielsen, S. E., Truchon-Savard, A., and Johnstone, J. F. (2019). Examining forest resilience to changing fire frequency in a fire-prone region of boreal forest. Glob. Chang. Biol. 25, 869–884. doi: 10.1111/gcb.14550
Harvey, B. J., Donato, D. C., and Turner, M. G. (2016). High and dry: post-fire tree seedling establishment in subalpine forests decreases with post-fire drought and large stand-replacing burn patches. Glob. Ecol. Biogeogr. 25, 655–669. doi: 10.1111/geb.12443
Higuera, P. E., Shuman, B. N., and Wolf, K. D. (2021). Rocky Mountain subalpine forests now burning more than any time in recent millennia. Proc. Natl. Acad. Sci. 118:e2103135118. doi: 10.1073/pnas.2103135118
Hijmans, R. J., Phillips, S., Leathwick, J., and Elith, J. (2021). dismo: Species Distribution Modeling. R package version 1.3-5.
Hood, S. M., and Varner, J. M. (2019). “Post-fire Tree Mortality,” in Encyclopedia of Wildfires and Wildland-Urban Interface (WUI) Fires, ed. S. L. Manzello (Cham: Springer), 1–10. doi: 10.1007/978-3-319-51727-8_252-1
Johnstone, J. F., Allen, C. D., Franklin, J. F., Frelich, L. E., Harvey, B. J., Higuera, P. E., et al. (2016). Changing disturbance regimes, ecological memory, and forest resilience. Front. Ecol. Env. 14, 369–378. doi: 10.1002/ece3.5061
Kemp, K. B., Higuera, P. E., and Morgan, P. (2016). Fire legacies impact conifer regeneration across environmental gradients in the U.S. northern Rockies. Landsc. Ecol. 31, 619–636. doi: 10.1007/s10980-015-0268-3
Kemp, K. B., Higuera, P. E., Morgan, P., and Abatzoglou, J. T. (2019). Climate will increasingly determine post-fire tree regeneration success in low-elevation forests, Northern Rockies, USA. Ecosphere 10:e02568. doi: 10.1002/ecs2.2568
Key, C. H. (2006). Ecological and Sampling Constraints on Defining Landscape Fire Severity. Fire Ecol. 2, 34–59. doi: 10.4996/fireecology.0202034
Keyser, A. R., Krofcheck, D. J., Remy, C. C., Allen, C. D., and Hurteau, M. D. (2020). Simulated Increases in Fire Activity Reinforce Shrub Conversion in a Southwestern US Forest. Ecosystems 23, 1702–1713. doi: 10.1007/s10021-020-00498-4
Kraft, N. J. B., Adler, P. B., Godoy, O., James, E. C., Fuller, S., and Levine, J. M. (2015). Community assembly, coexistence and the environmental filtering metaphor ed. J. Fox. Funct. Ecol. 29, 592–599. doi: 10.1111/1365-2435.12345
Krawchuk, M. A., Meigs, G. W., Cartwright, J. M., Coop, J. D., Davis, R., Holz, A., et al. (2020). Disturbance refugia within mosaics of forest fire, drought, and insect outbreaks. Front. Ecol. Env. 18, 235–244. doi: 10.1002/fee.2190
Kroiss, S. J., and HilleRisLambers, J. (2015). Recruitment limitation of long-lived conifers: implications for climate change responses. Ecology 96, 1286–1297. doi: 10.1890/14-0595.1
Kulakowski, D., Matthews, C., Jarvis, D., and Veblen, T. T. (2013). Compounded disturbances in sub-alpine forests in western Colorado favour future dominance by quaking aspen (Populus tremuloides). J. Veget. Sci. 24, 168–176. doi: 10.1111/j.1654-1103.2012.01437.x
Larson, A. J., Jeronimo, S. M. A., Hessburg, P. F., Lutz, J. A., Povak, N. A., Cansler, C. A., et al. (2022). Tamm review: ecological principles to guide post-fire forest landscape management in the Inland Pacific and Northern Rocky Mountain regions. For. Ecol. Manage. 504:119680.
Leathwick, J., Elith, J., Francis, M., Hastie, T., and Taylor, P. (2006). Variation in demersal fish species richness in the oceans surrounding New Zealand: an analysis using boosted regression trees. Mar. Ecol. Prog. Ser. 321, 267–281. doi: 10.3354/meps321267
Lemmom, P. E. (1956). A Spherical Densiometer for Estimating Forest Overstory Density. For. Sci. 2, 314–320.
Littlefield, C. E., Dobrowski, S. Z., Abatzoglou, J. T., Parks, S. A., and Davis, K. T. (2020). A climatic dipole drives short- and long-term patterns of postfire forest recovery in the western United States. Proc. Natl. Acad. Sci. 117, 29730–29737. doi: 10.1073/pnas.2007434117
Lotan, J. E. (1976). “Cone Serotiny - Fire Relationships in Lodgepole Pine,” in Tall Timbers Fire Ecology Conference Proceedings, (Tallahassee, FL: Tall Timbers Research Center), 267–278.
Lutz, J. A., van Wagtendonk, J. W., and Franklin, J. F. (2010). Climatic water deficit, tree species ranges, and climate change in Yosemite National Park. J. Biogeogr. 37, 936–950. doi: 10.1111/j.1365-2699.2009.02268.x
Matlack, G. R. (1989). Secondary Dispersal of Seed Across Snow in Betula Lenta, a Gap-Colonizing Tree Species. J. Ecol. 77:853. doi: 10.2307/2260990
McCaughey, W., Schmidt, W and Shearer, R. C. (1986). “Seed-Dispersal Characteristics of Conifers in the Inland Mountain West,” In Proceedings-Conifer Tree Seed in the Inland Mountain West Symposium. Ogden?: U.S. Dept. of Agriculture, Forest Service, Intermountain Research Station.
McCune, B., and Keon, D. (2002). Equations for Potential Annual Direct Incident Radiation and Heat Load. J. Veget. Sci. 13, 603–606. doi: 10.1111/j.1654-1103.2002.tb02087.x
Meddens, A. J. H., Kolden, C. A., Lutz, J. A., Smith, A. M. S., Cansler, C. A., Abatzoglou, J. T., et al. (2018). Fire Refugia: what Are They, and Why Do They Matter for Global Change? BioScience 68, 944–954.
Miller, J. D., and Thode, A. E. (2007). Quantifying burn severity in a heterogeneous landscape with a relative version of the delta Normalized Burn Ratio (dNBR). Rem. Sens. Env. 109, 66–80. doi: 10.1016/j.rse.2006.12.006
Minore, D. (1986). Germination, Survival and Early Growth of Conifer Seedlings in Two Habitat Types. Portland, OR: U.S. Department of Agriculture, Forest Service, Pacific Northwest Research Station.
Morelli, T. L., Barrows, C. W., Ramirez, A. R., Cartwright, J. M., Ackerly, D. D., Eaves, T. D., et al. (2020). Climate-change refugia: biodiversity in the slow lane. Front. Ecol. Env. 18, 228–234. doi: 10.1002/fee.2189
OECD (2010). Section 5 - Lodgepole pine (Pinus contorta). In Safety Assessment of Transgenic Organisms. Paris: OECD Publishing.
Parks, S. A., and Abatzoglou, J. T. (2020). Warmer and drier fire seasons contribute to increases in area burned at high severity in western US forests from 1985−2017. Geophys. Res. Lett. 2020:47.
Peeler, J. L., and Smithwick, E. A. H. (2020). Seed source pattern and terrain have scale-dependent effects on post-fire tree recovery. Landsc. Ecol. 35, 1945–1959. doi: 10.1007/s10980-020-01071-z
Peeler, J. L., and Smithwick, E. A. H. (2021). Interactions between landscape and local factors inform spatial action planning in post-fire forest environments. Landsc. Ecol. 36, 3523–3537. doi: 10.1007/s10980-021-01325-4
Qiu, T., Aravena, M.-C., Andrus, R., Ascoli, D., Bergeron, Y., Berretti, R., et al. (2021). Is there tree senescence? The fecundity evidence. Proc. Natl. Acad. Sci. 118:e2106130118. doi: 10.1073/pnas.2106130118
R Core Team (2020). R: A language and environment for statistical computing. Vienna: R Foundation for Statistical Computing.
Reilly, M. J., Dunn, C. J., Meigs, G. W., Spies, T. A., Kennedy, R. E., Bailey, J. D., et al. (2017). Contemporary patterns of fire extent and severity in forests of the Pacific Northwest, USA (1985-2010). Ecosphere 8:e01695. doi: 10.1002/ecs2.1695
Rodman, K. C., Paula, F., Chapman, T. B., Coop, J. D., Edwards, G., Stevens, J. T., et al. (2021). SRM-PLANT: A Decision-Support Tool to Inform Post-fire Planting of Ponderosa Pine and Douglas-fir in the Southern Rocky Mountains. Available online at: https://treesarethebeesknees.users.earthengine.app/view/srme-reforestation-tool. (accessed February 16, 2022).
Rodman, K. C., Veblen, T. T., Chapman, T. B., Rother, M. T., Wion, A. P., and Redmond, M. D. (2020). Limitations to recovery following wildfire in dry forests of southern Colorado and northern New Mexico, USA. Ecol. Appl. 30:20. doi: 10.1002/eap.2001
Schoennagel, T., Turner, M. G., and Romme, W. H. (2003). The influence of fire interval and serotiny on postfire lodgepole pine density in Yellowstone National Park. Ecology 84, 2967–2978. doi: 10.1890/02-0277
Stevens-Rumann, C., and Morgan, P. (2016). Repeated wildfires alter forest recovery of mixed-conifer ecosystems. Ecol. Appl. 26, 1842–1853.
Shive, K. L., Preisler, H. K., Welch, K. R., Safford, H. D., Butz, R. J., O’Hara, K. L., et al. (2018). From the stand-scale to the landscape-scale: predicting the spatial patterns of forest regeneration after disturbance. Ecol. Appl. 28, 1626–1639.
Stevens-Rumann, C. S., Kemp, K. B., Higuera, P. E., Harvey, B. J., Rother, M. T., Donato, D. C., et al. (2018). Evidence for declining forest resilience to wildfires under climate change. Ecol. Lett. 21, 243–252. doi: 10.1111/ele.12889
Stevens-Rumann, C. S., and Morgan, P. (2019). Tree regeneration following wildfires in the western US: a review. Fire Ecol. 15:15.
Stewart, J. A. E. (2021). POSCRPT: Postfire Spatial Conifer Restoration Planning Tool. Available online at: https://stewartecology.shinyapps.io/POSCRPT/. (accessed February 16, 2022).
Stewart, J. A. E., van Mantgem, P. J., Young, D. J. N., Shive, K. L., Preisler, H. K., Das, A. J., et al. (2020). Effects of postfire climate and seed availability on postfire conifer regeneration. Ecol. Appl. 2020:2280. doi: 10.1002/eap.2280
Stovall, A. E. L., Shugart, H., and Yang, X. (2019). Tree height explains mortality risk during an intense drought. Nat. Comm. 10:4385. doi: 10.1038/s41467-019-12380-6
Tepley, A. J., Thomann, E., Veblen, T. T., Perry, G. L. W., Holz, A., Paritsis, J., et al. (2018). Influences of fire-vegetation feedbacks and post-fire recovery rates on forest landscape vulnerability to altered fire regimes. J. Ecol. 106:5.
Tinker, D. B. (1994). Landscape-scale heterogeneity in lodgepole serotiny. Can. J. For. Res. 24, 897–903. doi: 10.1139/x94-118
Turner, M. G., and Gardner, R. H. (2015). Landscape Metrics in Landscape Ecology in Theory and Practice. New York, NY: Springer.
Turner, M. G., and Romme, W. H. (1994). Landscape dynamics in crown fire ecosystems. Landsc. Ecol. 9, 59–77. doi: 10.1007/bf00135079
Urza, A. K., and Sibold, J. S. (2013). Nondestructive Aging of Postfire Seedlings for Four Conifer Species in Northwestern Montana. West. J. Appl. For. 28, 22–29. doi: 10.5849/wjaf.11-014
Urza, A. K., and Sibold, J. S. (2017). Climate and seed availability initiate alternate post-fire trajectories in a lower subalpine forest. J. Veg. Sci. 28, 43–56. doi: 10.1111/jvs.12465
USDA (2008). Conterminous U.S. and Alaska Forest Type Mapping Using Forest Inventory and Analysis Data. Forest Inventory and Analysis (FIA) Program & Geospatial Technology and Applications Center (GTAC). Atlanta, GA: USDA Forest Service.
Walker, R., Coop, J. D., Downing, W. M., Krawchuk, M. A., Malone, S. L., and Meigs, G. W. (2019). How Much Forest Persists Through Fire? High-Resolution Mapping of Tree Cover to Characterize the Abundance and Spatial Pattern of Fire Refugia Across Mosaics of Burn Severity. Forests 10:782. doi: 10.3390/f10090782
Wang, T., Hamann, A., Spittlehouse, D., and Carroll, C. (2016). Locally Downscaled and Spatially Customizable Climate Data for Historical and Future Periods for North America. PLoS One 11, e0156720. doi: 10.1371/journal.pone.0156720
Westerling, A. L. (2006). Warming and Earlier Spring Increase Western U.S. Forest Wildfire Activity. Science 313, 940–943. doi: 10.1126/science.1128834
Keywords: cascade range, post-fire forest recovery, seed availability, fire refugia, seed dispersal, subalpine conifer forest, high-severity wildfire, delayed mortality
Citation: Busby SU and Holz A (2022) Interactions Between Fire Refugia and Climate-Environment Conditions Determine Mesic Subalpine Forest Recovery After Large and Severe Wildfires. Front. For. Glob. Change 5:890893. doi: 10.3389/ffgc.2022.890893
Received: 07 March 2022; Accepted: 26 April 2022;
Published: 20 May 2022.
Edited by:
Robert Michael Scheller, North Carolina State University, United StatesReviewed by:
Daniel Donato, Washington Department of Natural Resources, United StatesCraig Nitschke, University of Melbourne, Australia
Copyright © 2022 Busby and Holz. This is an open-access article distributed under the terms of the Creative Commons Attribution License (CC BY). The use, distribution or reproduction in other forums is permitted, provided the original author(s) and the copyright owner(s) are credited and that the original publication in this journal is cited, in accordance with accepted academic practice. No use, distribution or reproduction is permitted which does not comply with these terms.
*Correspondence: Sebastian U. Busby, sebastian.busby@usda.gov