Response: Where Might We Find Ecologically Intact Communities?
- 1KBA Secretariat, c/o BirdLife International, Cambridge, United Kingdom
- 2Doñana Biological Station, Spanish National Research Council, Seville, Spain
- 3Department of Biological and Environmental Sciences, University of Gothenburg, Gothenburg, Sweden
- 4Gothenburg Global Biodiversity Centre (GGBC), University of Gothenburg, Gothenburg, Sweden
- 5Department of Environmental Science, Faculty of Science, Radboud University Nijmegen, Nijmegen, Netherlands
- 6German Centre for Integrative Biodiversity Research (iDiv) Halle-Jena-Leipzig, Leipzig, Germany
- 7Instituto de Ecología, Universidad Nacional Autónoma de México, Ciudad Universitaria, Mexico City, Mexico
- 8Durrell Institute of Conservation and Ecology, School of Anthropology and Conservation, University of Kent, Canterbury, United Kingdom
- 9School of Biological and Environmental Sciences, Liverpool John Moores University, Liverpool, United Kingdom
- 10Institute for Biodiversity and Ecosystem Dynamics, University of Amsterdam, Amsterdam, Netherlands
- 11Environmental Research Group Oxford Ltd., Department of Zoology, Oxford University, Oxford, United Kingdom
A Commentary on
Where Might We Find Ecologically Intact Communities?
by Plumptre, A. J., Baisero, D., Belote, R. T., Vázquez-Domínguez, E., Faurby, S., Jȩdrzejewski, W., Kiara, H., K, H., Benítez-López, A., Luna-Aranguré, C., Voigt, M., Wich, S., Wint, W., Gallego-Zamorano, J., and Boyd, C. (2021). Front. For. Glob. Change 4:626635. doi: 10.3389/ffgc.2021.626635
We thank the authors of the commentary of our paper “Where might we find ecologically intact communities” (Grantham et al., 2022) for their interest in our paper (Plumptre et al., 2021) and their critique of some of the methods and conclusions. We here respond to their commentary, where many of their points have been well-made, with the aim of advancing discussion of what is meant by ecological integrity or intactness.
Part of the reason for undertaking our study was to provide a scoping of potential Key Biodiversity Areas (KBAs) that might meet Criterion C status defined as sites of “outstanding ecological integrity.” We were interested in assessing what we might obtain if we use a strict interpretation of “wholly intact ecological communities” to mean that no species had been lost since a baseline date of 1,500. Another reason was to encourage the assessment of species composition as part of any assessment of ecological integrity. Our results clearly showed that very few areas would likely contain KBAs with this interpretation and baseline and as a result, we believe that a broader interpretation of intactness will be required, but that still identifies sites of “outstanding ecological integrity.”
There are three main points of criticism by Grantham et al. (2022) of Plumptre et al. (2021): (1) the definition of ecological integrity and that it does not follow the main paradigm of structure-composition-function that has been applied to assessment of ecological integrity; (2) the appropriateness of the methods applied; and (3) the issue of the usefulness of the results for policy and application for real-world conservation.
Definition of Ecological Integrity
We recognize there have been a recent series of papers that have suggested that “ecological integrity” is not a useful concept (Karr et al., 2021; Rohwer and Marris, 2021; Marris and Rohwer, 2022). The main argument by Rohwer and Marris is that integrity is defined in many different ways and that it is clearer to utilize the specific definitions rather than use the term integrity. While we would not necessarily agree that the term integrity should be abandoned, as it facilitates capturing complex ideas, we do believe that there is a value in being clear what we are measuring when we estimate ecological integrity.
Here the main critique appears to be that our paper does not follow the well-established paradigm that ecological integrity should include an assessment of structure, composition and function (Hansen et al., 2021). The authors list a set of studies, which they consider good examples of the measurement of ecological integrity in their first paragraph. We have therefore analyzed these and added one other commonly used measure of Intact Forest Landscapes (Potapov et al., 2017) to assess which ones specifically address Structure, Composition and Function, and summarize our results in Table 1. What is clear is that the majority of studies have used remotely-sensed products to derive impacts on ecosystem structure (human modification of habitat or proxies, like the presence of roads, settlements, human population density, and/or measures of canopy cover, height, or forest extent). A few have addressed composition directly (i.e., compiling species data) using the Biotic Integrity Index which measures changes in species richness and abundance (Scholes and Biggs, 2005) or the BILBI framework that uses species turnover (Mokany et al., 2020). None of the methods specifically targeted measures of ecosystem function. We would argue that only our study has explicitly tried to address aspects of structure, composition and function, in our assessment of global ecological integrity and this is why we identify a much smaller area of the world as “ecologically intact.” We accept that our methods are coarse and could clearly be improved with more accurate local data applied at site scales (e.g., Faber-Langendoen et al., 2016). However, it is still not technologically and logistically possible to derive such accurate data at global scales, particularly for assessing ecosystem function (see Table 1 in Hansen et al., 2021). We would encourage more assessments at this local scale that do include species composition data and measures of ecosystem function where it is possible to obtain data (e.g., Tierney et al., 2009), to advance our knowledge on what is “ecologically intact.”
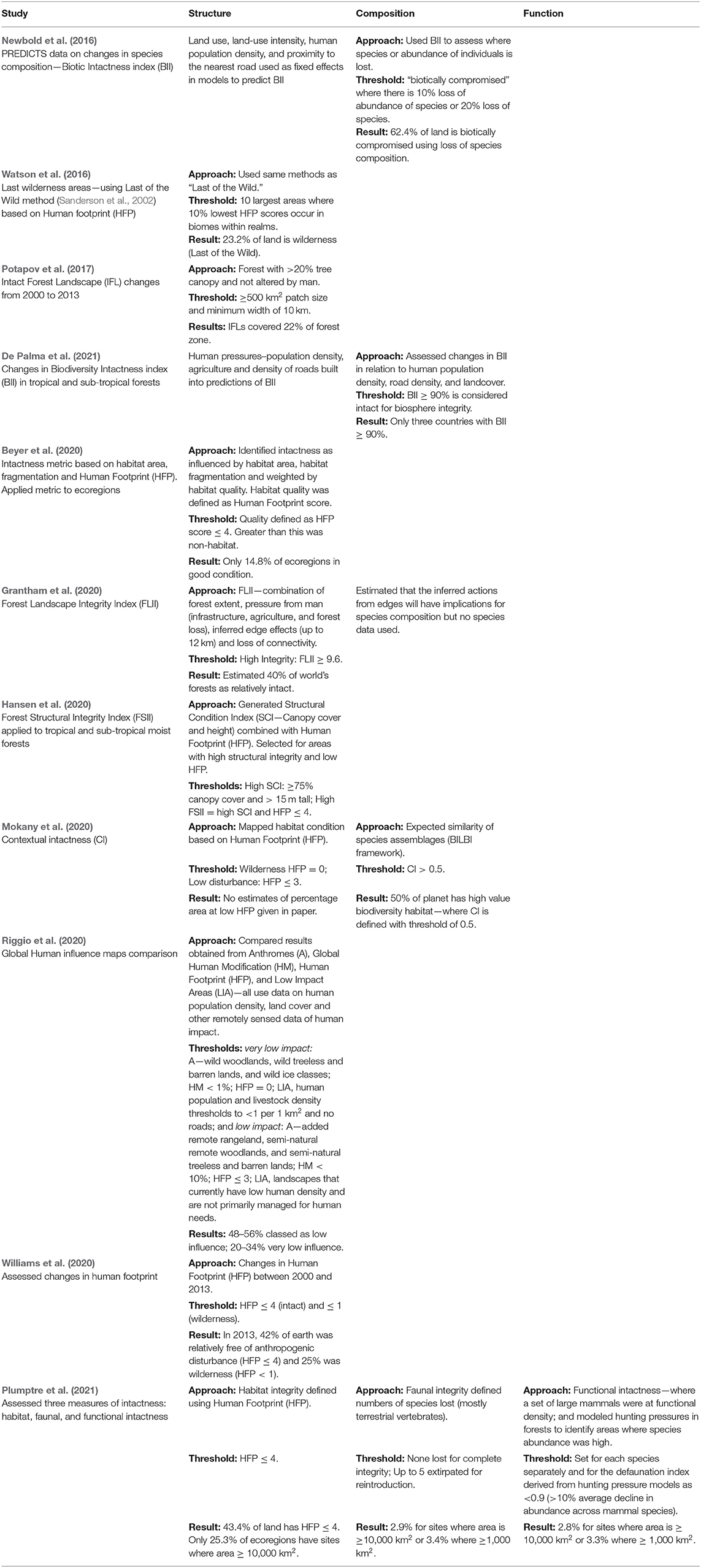
Table 1. Review of global assessments of ecological integrity and the approaches, thresholds, and results obtained in relation to the structure-composition-function paradigm.
A point is made that we identify a site as intact or not in a binary fashion, however, our investigation of these papers shows they all use specific thresholds (listed in Table 1) to separate intact areas from non-intact areas. While we agree with them that intactness is a spectrum, the definition of what is outstanding is necessarily tied to a threshold, with defined “intact areas” representing a binary decision.
Finally, there is the concern that the documented loss of one species in our paper excludes a site as having full ecological integrity. Our methods identified where varying numbers of species had been extirpated but our definition of full ecological integrity was sites with no loss of species. This definition of “outstanding ecological integrity” is admittedly of an extremely high standard but our analysis was dominated by mammal species that have been extirpated. We looked at loss of range across mammals and 39.9% of the range loss is seen in species from 10 to 100 kg, 33.3% in species between 100 and 1,000 kg, and 4.1% in species weighing >1,000 kg. Therefore, 77.3% of the loss encompasses large-bodied species, which regularly serve as ecosystem engineers. The functional roles of large bodied mammals such as elephants (Loxodonta africana and L. cyclotis) have been well-documented (e.g., Laws et al., 1970; Blake et al., 2009; Campos-Arceiz and Blake, 2011; Ripple et al., 2015) as have those of large predators (Smith et al., 2003; Johnson et al., 2007; Ripple et al., 2014). However, scientific evidence also shows that medium and small mammals can also have significant impacts on ecological function, like seed predation and dispersal, with knock-on effects on vegetation dynamics and the carbon cycle (Colman et al., 2014; Mills and Letnic, 2018; Schmitz et al., 2018; Gardner et al., 2019). We therefore believe that loss of such species, in many instances even the loss of one species, may have led to ecological changes at sites and loss of ecological integrity as a result. Sometimes these roles can be replaced by other species but with large mammals, we would maintain that this is harder to achieve.
Appropriateness of Methods
The commentary criticized the use of a threshold value of Human Footprint of ≤ 4. While the focus of the paper was not on measurements of intact structure, we used this threshold because we wanted to scope areas of outstanding ecological integrity and were interested in the combined effects of structure, composition and function. We selected the threshold value based on several of these authors own work because it has been used elsewhere as a threshold for good habitat quality (Beyer et al., 2020) and ecological integrity (Williams et al., 2020). For example, Williams et al. (2020), published 6 months before our paper, states “We used a human footprint threshold of <4 (on a 0–50 scale) to identify where land is considered ecologically intact (below the threshold) or highly modified and thus ecologically degraded (equal to or above the threshold).”
The second criticism was that the reference date of 1,500 CE was arbitrary and not based in science. We selected to use a single date for consistency but note that the KBA Standard (IUCN, 2016) allows for regionally appropriate baseline dates. This date is used as the baseline date by the IUCN Red List of Threatened species for the following reasons: It is a date before the expansion of Europeans into the Americas and also a date before the widespread use of firearms. Extinction rates have also increased substantially since this time (Barnosky et al., 2011; Cowie et al., 2022), although for mammals (and likely many other groups) elevated rates above background extinction rates as a result of human activities were also seen before this time (Andermann et al., 2020). Hence, using 1,500 CE as a baseline reference date falls within a period, which allows for assessment of the current condition of ecosystems relative to their pre-industrial baseline states. We note, however, that this baseline does not mean we necessarily consider the state at 1,500 CE entirely pristine but rather see it as a pragmatic baseline since effectively all changes since this time and some of the changes pre 1,500 CE will be anthropogenic in nature.
We agree that the use of range maps for the species are an approximation but these are the best data available at a global scale that have been validated by experts. They have also been used in many other analyses (e.g., Harfoot et al., 2021 and many others). We would also argue that all global datasets are inaccurate whether these are the range maps we used, the layers that were used to derive the human footprint map, the predicted hunting pressure maps as well as the modeled ranges we used to assess functional intactness. One of the key drivers of the human footprint map is land use change and it is recognized that comparison of land cover maps derived using different methods should not be made (Herold et al., 2008; Sexton et al., 2016; Venter et al., 2016; Estes et al., 2018; Williams et al., 2020). Yet many of the approaches used (Table 1) apply different land cover products when calculating the areas of intactness and as a result are not easily comparable. As we argued in the paper, none of these global datasets are really useful for site identification but can help guide where more detailed investigations should take place to compile local data to develop a more accurate measure of ecological integrity as recommended by Chaplin-Kramer et al. (2022).
Our estimates of functional intactness were based on estimates of reduced species populations from the hunting pressure models in tropical forests, jointly with estimates of low density and less suitable habitat for 16 species/subspecies models considered to have important functional roles. These layers were primarily included as a demonstration of how functional integrity due to species densities might be assessed, but recognize that this can be better assessed at a local scale. We selected wide-ranging species for which species distribution models are available, but for many species these types of models do not exist across their entire range. However, the principle of identifying functional densities of species when selecting sites of ecological integrity, such as Criterion C sites for Key Biodiversity Areas (KBAs), should be strongly considered as part of an assessment of functional integrity. We would argue that again this is easier to apply at a local scale where more will be known or can be measured.
Policy Implications
As we stated, the reason for undertaking our study was to provide a scoping of potential Criterion C KBAs, to assess what we might obtain if we use a strict interpretation of “wholly intact ecological communities.” We therefore agree with Grantham et al. (2022) that such a strict definition is of concern for policy makers who are pushing for ambitious conservation targets under the Global Biodiversity Framework, given how few areas we found were considered ecologically intact. While we think that highlighting the extent of still existing ecologically intact habitat has value in itself, in particular to avoid overly optimistic perceptions caused by the shifting baseline syndrome (Soga and Gaston, 2018), Grantham et al. (2022) mainly see a value when applicable to identifying specific areas for conservation. Clearly, there is a value in conserving large areas of relatively intact habitat, which have been identified by the various approaches used in Table 1 and cover between 22 and 58% of land. Indeed, our paper ended with a statement that we recognized these places should be targets of conservation under the Global Biodiversity Framework. However, we also believe that where we have intact habitat as well as intact fauna since 1,500 CE that these sites are particularly special and are worthy of recognition and protection. The findings of our paper, and the reactions to it, triggered a set of webinars and meetings in the second half of 2021 to reconsider how KBA Criterion C could be interpreted, and in which some of the authors of Grantham et al. (2022) participated. Participants in these events investigated ways in which we might broaden the interpretation to focus on all aspects of structure, composition, and function. Suggestions for how to proceed in relation to species composition advised that some allowance is needed for species turnover, particularly in the face of climate change, and having instead a target of maintaining all trophic levels at a site rather than a species-focused approach. Revised guidance for the application of Criterion C are being developed resulting from what was agreed in these meetings. This will likely identify more Criterion C sites that fall under this broader definition of ecological intactness.
We also showed in our paper that in many of these intact habitats there are opportunities to restore species that undoubtedly have important functional roles. A recent analysis, using similar data to ours, likewise argues that we should be considering the reintroduction of mammals to regain integrity and ecological function (Vynne et al., 2022). Large mammals are frequently the first to disappear and their successful reintroduction not only requires the effective removal of relevant threats, but also supports the re-establishment of associated ecological processes. Due to their spatial and habitat requirements they serve as extremely important indicators. The rewilding movement is growing and having successes in Europe (Fernández et al., 2020), USA (Smith et al., 2003), and Australia (Sweeney et al., 2019); it can be used to improve areas of intact habitat where we know of functional species that have been extirpated. We conceptually disagree with Grantham et al. (2022) that the re-wilding approach is species rather than process focussed. The goal of rewilding is the restoration of ecological processes, which is most easily achieved by reintroduction of extirpated species (Perino et al., 2019). This focus is, for example, seen in the frequent discussion of using functional analogs when the species that used to live at a given site are globally extinct (e.g., Louys et al., 2014; Falcón and Hansen, 2018). In the UN decade of restoration we believe it is more ambitious to not only consider conserving remaining areas of intact habitat but also consider rewilding areas with large mammals and other species that have been extirpated. We agree with Grantham et al. (2022) that conserving the relatively intact areas needed for their introduction is important but that we can achieve more than this and that we should be working together to accomplish both objectives.
Author Contributions
All authors listed have made a substantial, direct, and intellectual contribution to the work and approved it for publication.
Conflict of Interest
GW was employed by Environmental Research Group Oxford Ltd.
The remaining authors declare that the research was conducted in the absence of any commercial or financial relationships that could be construed as a potential conflict of interest.
Publisher's Note
All claims expressed in this article are solely those of the authors and do not necessarily represent those of their affiliated organizations, or those of the publisher, the editors and the reviewers. Any product that may be evaluated in this article, or claim that may be made by its manufacturer, is not guaranteed or endorsed by the publisher.
References
Andermann, T., Faurby, S., Turvey, S. T., Antonelli, A., and Silvestro, D. (2020). The past and future human impact on mammalian diversity. Sci. Adv. 6, eabb2313. doi: 10.1126/sciadv.abb2313
Barnosky, A. D., Matzke, N., Tomiya, S., Wogan, G. O. U., Swartz, B., Quental, T. B., et al. (2011). Has the Earth's sixth mass extinction already arrived? Nature 471, 51–57. doi: 10.1038/nature09678
Beyer, H. L., Venter, O., Grantham, H. S., and Watson, J. E. M. (2020). Substantial losses in ecoregion intactness highlights urgency of globally coordinated action. Conserv. Lett. 13, e12692. doi: 10.1111/conl.12692
Blake, S., Deem, S. L., Mossimbo, E., Maisels, F., and Walsh, P. (2009). Forest elephants: tree planters of the Congo. Biotropica 41, 459–468. doi: 10.1111/j.1744-7429.2009.00512.x
Campos-Arceiz, A., and Blake, S. (2011). Megagardeners of the forest-the role of elephants in seed dispersal. Acta Oecol. 37, 542–553. doi: 10.1016/j.actao.2011.01.014
Chaplin-Kramer, R., Brauman, K. A., Cavender-Bares, J., Díaz, S., Duarte, G. T., Enquist, B. J., et al. (2022). Conservation needs to integrate knowledge across scales. Nat. Ecol. Evol. 6, 118–119. doi: 10.1038/s41559-021-01605-x
Colman, N. J., Gordon, C. E., Crowther, M. S., and Letnic, M. (2014). Lethal control of an apex predator has unintended cascading effects on forest mammal assemblages. Proc. R. Soc. B Biol. Sci. 281, 20133094. doi: 10.1098/rspb.2013.3094
Cowie, R. H., Bouchet, P., and Fontaine, B. (2022). The Sixth Mass Extinction: fact, fiction or speculation?. Biol. Rev. 97, 640–663. doi: 10.1111/brv.12816
De Palma, A. D., Hoskins, A., Gonzalez, R. E., Börger, L., Newbold, T., Sanchez-Ortiz, K., et al. (2021). Annual changes in the Biodiversity Intactness Index in tropical and subtropical forest biomes, 2001962012. Sci. Rep. 11, 20249. doi: 10.1038/s41598-021-98811-1
Estes, L., Chen, P., Debats, S., Evans, T., Ferreira, S., Kuemmerle, T., et al. (2018). A large-area, spatially continuous assessment of land cover map error and its impact on downstream analyses. Glob. Change Biol. 24, 322–337. doi: 10.1111/gcb.13904
Faber-Langendoen, D., Nichols, W., Rocchio, J., Walz, K., and Lemly, J. (2016). An Introduction to Natureserve's Ecological Integrity Assessment Method. Arlington, VA: NatureServe.
Falcón, W., and Hansen, D. M. (2018). Island rewilding with giant tortoises in an era of climate change. Philos. Trans. R. Soc. B Biol. Sci. 373, 20170442. doi: 10.1098/rstb.2017.0442
Fernández, N., Torres, A., Wolf, F., Quintero, L., and Pereira, H. M. (2020). Boosting Ecological Restoration for a Wilder Europe. Halle-Wittenberg: German Centre for Integrative Biodiversity Research (iDiv) and Martin-Luther-Universität.
Gardner, C. J., Bicknell, J. E., Baldwin-Cantello, W., Struebig, M. J., and Davies, Z. G. (2019). Quantifying the impacts of defaunation on natural forest regeneration in a global meta-analysis. Nat. Commun. 10, 1–7. doi: 10.1038/s41467-019-12539-1
Grantham, H. S., Duncan, A., Evans, T. D., Jones, K. R., Beyer, H. L., Schuster, R., et al. (2020). Anthropogenic modification of forests means only 40% of remaining forests have high ecosystem integrity. Nat. Commun. 11, 5978. doi: 10.1038/s41467-020-19493-3
Grantham, H. S., Evans, T., Lieberman, S., Robinson, J. G., Elsen, P. R., Ferrier, S., et al. (2022). Front. For. Glob. Change 5, 730546. doi: 10.3389/ffgc.2022.730546
Hansen, A. J., Burns, P., Ervin, J., Goetz, S. J., Hansen, M., Venter, O., et al. (2020). A policy-driven framework for conserving the best of Earth's remaining moist tropical forests. Nat. Ecol. Evol. 4, 1377–1384. doi: 10.1038/s41559-020-1274-7
Hansen, A. J., Noble, B. P., Veneros, J., East, A., Goetz, S. J., Supples, C., et al. (2021). Toward monitoring forest ecosystem integrity within the post-2020 Global Biodiversity Framework. Conserv. Lett. 14, e12822. doi: 10.1111/conl.12822
Harfoot, M. B. J., Johnston, A., Balmford, A., Burgess, N. D., Butchart, S. H. M., Dias, M. P., et al. (2021). Using the IUCN Red List to map threats to terrestrial vertebrates at global scale. Nat. Ecol. Evol. 5, 1510–1519. doi: 10.1038/s41559-021-01542-9
Herold, M., Mayaux, P., Woodcock, C. E., Baccini, A., and Schmullius, C. (2008). Some challenges in global land cover mapping: an assessment of agreement and accuracy in existing 1 km datasets. Remote Sens. Environ. 112, 2538–2556. doi: 10.1016/j.rse.2007.11.013
IUCN (2016). A Global Standard for the Identification of Key Biodiversity Areas, Version 1.0, 1st Edn. Gland: IUCN. Available online at: https://portals.iucn.org/library/node/46259
Johnson, C. N., Isaac, J. L., and Fisher, D. O. (2007). Rarity of a top predator triggers continent-wide collapse of mammal prey: dingoes and marsupials in Australia. Proc. R. Soc. B Biol. Sci. 274, 341–346. doi: 10.1098/rspb.2006.3711
Karr, J. R., Larsen, E. R., and Chu, E. W. (2021). Ecological integrity is both real and valuable. Conserv. Sci. Pract. (2021) 4, e583. doi: 10.1111/csp2.583
Laws, R. M., Parker, I. S. C., and Johnstone, R. C. B. (1970). Elephants and habitats in north Bunyoro, Uganda. East Afr. Wildl. J. 8, 163–180. doi: 10.1111/j.1365-2028.1970.tb00838.x
Louys, J., Corlett, R. T., Price, G. J., Hawkins, S., and Piper, P. J. (2014). Rewilding the tropics, and other conservation translocations strategies in the tropical Asia-Pacific region. Ecol. Evol. 4, 4380–4398. doi: 10.1002/ece3.1287
Marris, E., and Rohwer, Y. (2022). The concept of ecological integrity may have been useful, but that does not make it real or morally valuable. Conserv. Sci. Pract. 4, e586. doi: 10.1111/csp2.586
Mills, C. H., and Letnic, M. (2018). Reversing functional extinction of mammals prompts a rethink of paradigms about seed fate in arid Australia. R. Soc. Open Sci. 5, 171977. doi: 10.1098/rsos.171977
Mokany, K., Ferrier, S., Harwood, T. D., Ware, C., Di Marco, M., Grantham, H. S., et al. (2020). Reconciling global priorities for conserving biodiversity habitat. Proc. Natl. Acad. Sci. 117, 9906–9911. doi: 10.1073/pnas.1918373117
Newbold, T., Hudson, L. N., Arnell, A. P., Contu, S., De Palma, A., Ferrier, S., et al. (2016). Has land use pushed terrestrial biodiversity beyond the planetary boundary? A global assessment. Science 353, 288–291. doi: 10.1126/science.aaf2201
Perino, A., Pereira, H. M., Navarro, L. M., Fernández, N., Bullock, J. M., Ceau?u, S., et al. (2019). Rewilding complex ecosystems. Science 364, eaav5570. doi: 10.1126/science.aav5570
Plumptre, A. J., Baisero, D., Belote, R. T., Vázquez-Domínguez, E., Faurby, S., J?drzejewski, W., et al. (2021). Where might we find ecologically intact communities? Front. For. Glob. Change 4, 626635. doi: 10.3389/ffgc.2021.626635
Potapov, P., Hansen, M. C., Laestadius, L., Turubanova, S., Yaroshenko, A., Thies, C., et al. (2017). The last frontiers of wilderness: tracking loss of intact forest landscapes from 2000 to 2013. Sci. Adv. 3, e1600821. doi: 10.1126/sciadv.1600821
Riggio, J., Baillie, J. E. M., Brumby, S., Ellis, E., Kennedy, C. M., Oakleaf, J. R., et al. (2020). Global human influence maps reveal clear opportunities in conserving Earth's remaining intact terrestrial ecosystems. Glob. Change Biol. 26, 4344–4356. doi: 10.1111/gcb.15109
Ripple, W. J., Estes, J. A., Beschta, R. L., Wilmers, C. C., Ritchie, E. G., Hebblewhite, M., et al. (2014). Status and ecological effects of the world's largest carnivores. Science 343, 1241484. doi: 10.1126/science.1241484
Ripple, W. J., Newsome, T. M., Wolf, C., Dirzo, R., Everatt, K. T., Galetti, M., et al. (2015). Collapse of the world's largest herbivores. Sci. Adv. 1, e1400103. doi: 10.1126/sciadv.1400103
Rohwer, Y., and Marris, E. (2021). Ecosystem integrity is neither real nor valuable. Conserv. Sci. Pract. 3, e411. doi: 10.1111/csp2.411
Sanderson, E. W., Jaiteh, M., Levy, M. A., Redford, K. H., Wannebo, A. V., and Woolmer, G. (2002). The human footprint and the last of the wild. Bioscience 52, 891–904. doi: 10.1641/0006-3568(2002)052[0891:THFATL]2.0.CO;2
Schmitz, O. J., Wilmers, C. C., Leroux, S. J., Doughty, C. E., Atwood, T. B., Galetti, M., et al. (2018). Animals and the zoogeochemistry of the carbon cycle. Science 362, eaar3213. doi: 10.1126/science.aar3213
Scholes, R., and Biggs, R. (2005). A biodiversity intactness index. Nature 434, 45–49. doi: 10.1038/nature03289
Sexton, J. O., Noojipady, P., Song, X.-P., Feng, M., Song, D.-X., Kim, D.-H., et al. (2016). Conservation policy and the measurement of forests. Nat. Clim. Change 6, 192–196. doi: 10.1038/nclimate2816
Smith, D. W., Peterson, R. O., and Houston, D. B. (2003). Yellowstone after Wolves. Bioscience 53, 330–340. doi: 10.1641/0006-3568(2003)053[0330:YAW]2.0.CO;2
Soga, M., and Gaston, K. J. (2018). Shifting baseline syndrome: causes, consequences, and implications. Front. Ecol. Environ. 16, 222–230. doi: 10.1002/fee.1794
Sweeney, O. F., Turnbull, J., Jones, M., Letnic, M., Newsome, T. M., and Sharp, A. (2019). An Australian perspective on rewilding. Conserv. Biol. 33, 812–820. doi: 10.1111/cobi.13280
Tierney, G. L., Faber-Langendoen, D., Mitchell, B. R., Shriver, W. G., and Gibbs, J. P. (2009). Monitoring and evaluating the ecological integrity of forest ecosystems. Front. Ecol. Environ. 7, 308–316. doi: 10.1890/070176
Venter, O., Sanderson, E., Magrach, A., Allan, J. R., Beher, J., and Jones, K. R. (2016) Sixteen years of change in the global terrestrial human footprint implications for biodiversity conservation. Nat. Commun. 7 12558. doi: 10.1038/ncomms12558.
Vynne, C., Gosling, J., Maney, C., Dinerstein, E., Lee, A. T. L., Burgess, N. D., et al. (2022). An ecoregion-based approach to restoring the world's intact large mammal assemblages. Ecography. doi: 10.1111/ecog.06098. Available online at: https://onlinelibrary.wiley.com/action/showCitFormats?doi=10.1111%2Fecog.06098
Watson, J. E. M., Shanahan, D. F., Di Marco, M., Allan, J., Laurance, W. F., Sanderson, E. W., et al. (2016). Catastrophic declines in wilderness areas undermine global environment targets. Curr. Biol. 26, 2929–2934. doi: 10.1016/j.cub.2016.08.049
Keywords: ecological integrity assessment, Key Biodiversity Areas (KBA), intactness, restoration of integrity, biodiversity
Citation: Plumptre AJ, Baisero D, Benítez-López A, Faurby S, Gallego-Zamorano J, Kühl HS, Luna-Aranguré C, Vázquez-Domínguez E, Voigt M, Wich S and Wint GRW (2022) Response: Where Might We Find Ecologically Intact Communities? Front. For. Glob. Change 5:880353. doi: 10.3389/ffgc.2022.880353
Received: 21 February 2022; Accepted: 11 March 2022;
Published: 05 April 2022.
Edited by:
Barry Alan Gardiner, Institut Européen De La Forêt Cultivée (IEFC), FranceReviewed by:
Iain James Gordon, Australian National University, AustraliaCopyright © 2022 Plumptre, Baisero, Benítez-López, Faurby, Gallego-Zamorano, Kühl, Luna-Aranguré, Vázquez-Domínguez, Voigt, Wich and Wint. This is an open-access article distributed under the terms of the Creative Commons Attribution License (CC BY). The use, distribution or reproduction in other forums is permitted, provided the original author(s) and the copyright owner(s) are credited and that the original publication in this journal is cited, in accordance with accepted academic practice. No use, distribution or reproduction is permitted which does not comply with these terms.
*Correspondence: Andrew J. Plumptre, aplumptre@keybiodiversityareas.org