- 1Ecosystem Science and Management Program, University of Northern British Columbia, Prince George, BC, Canada
- 2Ministry of Forests, Lands, Natural Resource Operations and Rural Development, Victoria, BC, Canada
Densities of naturally regenerated mixtures of lodgepole pine (Pinus contorta Dougl. ex Loud. var. latifolia Engelm.) and Sitka alder [Alnus viridis subsp. sinuata (Regel) Á. Löve & D. Löve] were manipulated in 1995 at a site logged in 1987 in the central interior of British Columbia, Canada. Four levels of alder retention (0, 500, 1,000, and 2,000 clumps/ha) were combined with a uniform lodgepole pine density of 1,000 stems/ha across all treatments. After 25 years, effects of alder retention on lodgepole pine growth consisted of modest reductions in height and diameter increment, and treatment effects on soil nitrogen were confined to the forest floor organic horizons. Nutritional benefits of alder retention for lodgepole pine were not detected after year 6. The operational silvicultural implication of these findings is that only limited intervention to control Sitka alder in similar stands can be justified.
Introduction
Assessing competition between commercial species of coniferous trees and coexisting woody shrub species has great practical importance for silviculture. When the shrub associate has the ability to fix nitrogen (N), which is commonly a limiting nutrient on many boreal and temperate sites, the competitive tradeoffs that need to be considered in decisions to remove or retain this stand component become potentially more complex. In the interior regions of British Columbia (BC), Canada, Sitka alder [Alnus viridis subsp. sinuata (Regel) Á. Löve & D. Löve] is a common actinorhizal N-fixing shrub in understories of lodgepole pine (Pinus contorta Dougl. ex Loud. var. latifolia Engelm.) forests. With its moderate shade tolerance and ability to sprout from established clumps (Haeussler et al., 1990) this species can persist in mature stands, and then rapidly achieve significant cover when the overstory is removed by logging or other disturbances. This prompt site occupancy can be considered as a legacy of the previous stand, and enables considerably more rapid increases in biomass production and presumably N-fixation than would be possible via establishment of new individuals from seed.
Given these attributes, a field experiment designated as Experimental Project 1185 of the BC Ministry of Forests, was established in central BC in 1995 to address several aspects of lodgepole pine – Sitka alder interactions in young stands. With the broad geographical distribution of Sitka alder in interior BC forests, this study was inspired by, and intended to complement, an older field experiment established in 1987 approximately 400 km farther south (Simard, 1990).
Two of the original objectives (Sanborn et al., 2001) will be emphasized in this analysis of results obtained during the initial 25 years of this experiment, specifically to:
– Determine the effects of differing densities of Sitka alder on the long-term growth of a young, naturally regenerated lodgepole pine stand;
– Identify long-term changes in soil nitrogen stocks and availability associated with differing levels of Sitka alder retention.
In addition, other soil properties and foliar nutrient characteristics of lodgepole pine will be considered in relation to Sitka alder retention, as well in terms of change since the inception of the experiment.
Earlier published results for this installation included growth and foliar nutrition of lodgepole pine at 6 and 9 years (Brockley and Sanborn, 2003, 2007) after establishment, nitrogen fixation rate (Sanborn et al., 2002), and litter decomposition (mass loss and element dynamics; Sanborn and Brockley, 2009; Berg et al., 2013, 2017, 2021).
Despite the passage of 27 years since the initiation of this study, there have been few directly relevant additions to the literature on the roles of alder as a shrub component of boreal, sub-boreal and montane forests in North America. Further reports by Simard et al. (2004, 2006) are the only BC contributions, and these emphasized interactions with conifers, and the implications for vegetation management practices. Elsewhere in Canada, studies involving other alder species have emphasized competitive interactions with conifers in northern Alberta (Cortini and Comeau, 2008) and Quebec (Urli et al., 2020), with the latter study documenting differing responses by Pinus and Picea. In boreal Alaska, United States, Wurtz (2000) described establishment conditions and early growth and survival results for mixed plantings of white spruce [Picea glauca (Moench) Voss] and shrubby alders, while Doran et al. (2001) examined photosynthetic responses of white spruce grown in mixtures with green alder [Alnus crispa (Ait.) Pursh], and considered interactions with water stress and N supply. At the margins of the boreal forest and beyond, other Alaska studies have documented the role of shrubby alders in N cycling (Rhoades et al., 2001; Salmon et al., 2019).
Materials and Methods
Study Site
The study site is located approximately 55 km southwest of Prince George, BC, at 53°40′N, 123°39′W within the Stuart Dry Warm variant of the Sub-Boreal Spruce biogeoclimatic zone (SBSdw3; DeLong et al., 1993). The site has a west aspect, with slopes ranging from 5 to 20% and a mean elevation of approximately 1,030 m. Soil parent material consists predominantly of a blanket or veneer of morainal deposits over bedrock, containing 30–40% coarse fragments, and accompanied by surface soil textures ranging from loam to sandy loam. The soil is classified as a Brunisolic Gray Luvisol (Soil Classification Working Group, 1998), equivalent to a Haplocryalf (Soil Survey Staff, 1999), or a Haplic Luvisol (IUSS Working Group WRB, 2015). Additional information about site and soil characteristics is provided by the establishment report (Sanborn et al., 2001) and by Arocena and Sanborn (1999).
The site had been clearcut in 1987 and allowed to regenerate naturally, without site preparation or prescribed burning, to a mixture of lodgepole pine and Sitka alder, with the latter having regenerated densely from pre-existing clumps (Figure 1A). At the time of establishment of the installation in 1995, the average lodgepole pine density was approximately 10,500 stems/ha (average height 1.5 m) and the density of Sitka alder was approximately 4,100 clumps/ha (average height 1.8 m; Sanborn et al., 2001).
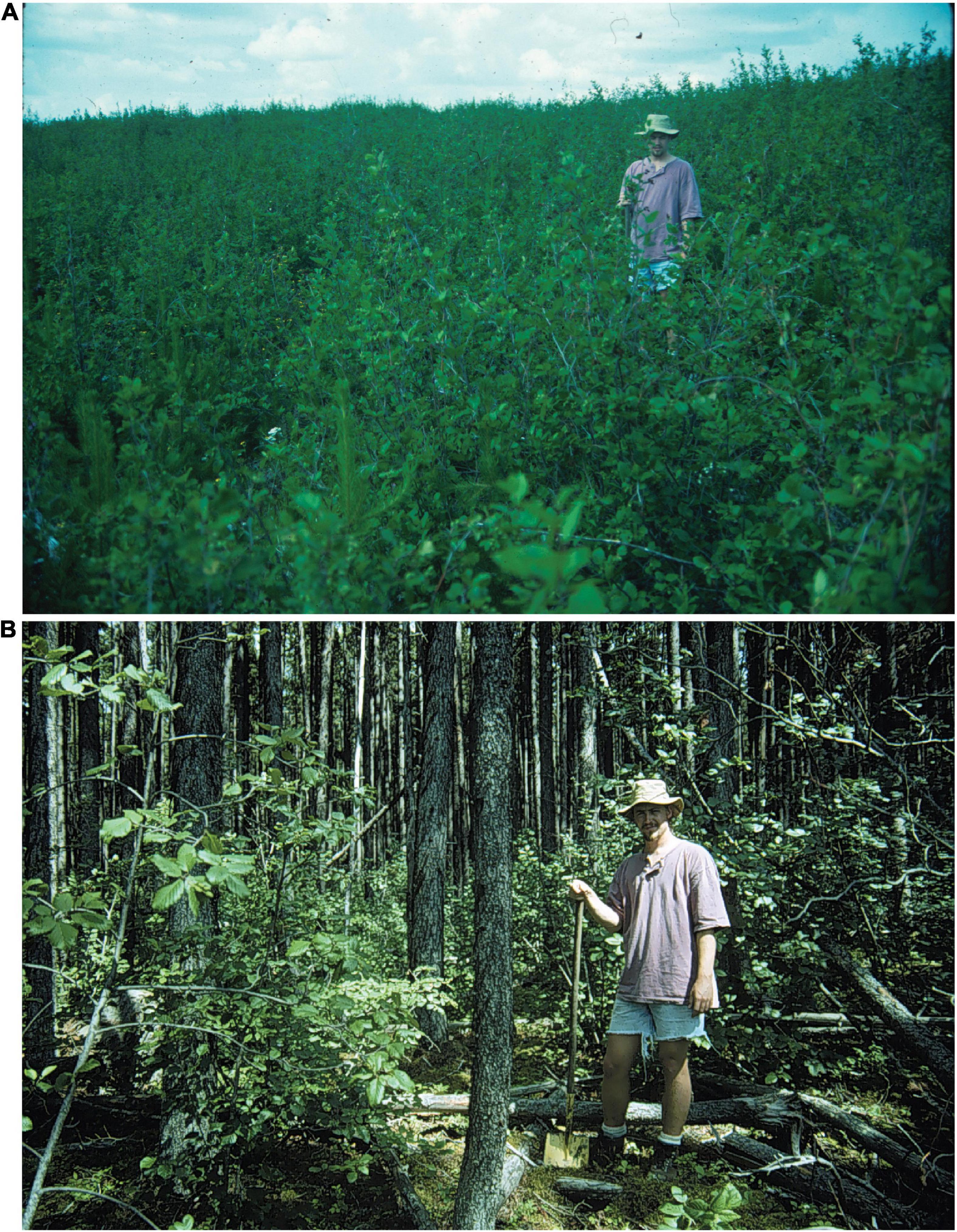
Figure 1. Photographs of stand conditions in 1994, showing (A) dense Sitka alder regeneration at research site, and (B) alder understory below a mature (∼150 year-old) lodgepole pine canopy approximately 100 m east of research site.
Experimental Design
Twelve 0.08 ha rectangular (25.28 m × 31.60 m) plots were established in May 1995 in areas with uniform site conditions and alder cover of approximately 50% and a density of 4,000 clumps/ha. Four alder retention treatments, replicated three times, were randomly assigned to these plots, each with pine thinned to the same density of 1,000 stems/ha: (i) complete removal of alder, (ii) 500 retained clumps/ha, (iii) 1,000 retained clumps/ha, and (iv) 2,000 retained clumps/ha. The pine density was lower than in typical operational stands, and was intended to delay canopy closure so as to maintain a vigorous alder understory in the treatments in which it was retained. Alder control was achieved by manual cutting followed by immediate application of glyphosate herbicide to the cut stems, and was largely successful. A limited repeat herbicide application was made in 1996, with follow-up hand-pulling of any alder seedlings and manual cutting of sprouts as needed in subsequent years. Subject only to natural mortality, the alder treatments maintained their integrity as intended throughout the duration of the experiment. Additional treatment details are provided in the establishment report (Sanborn et al., 2001).
Measurement
Each treatment plot contained an 18.96 m × 18.96 m (0.036 ha) assessment plot with permanently tagged lodgepole pine trees (36 per plot) and alder clumps (0, 18, 36, and 78 per plot, depending on the treatment). A diagram of plot layout and dimensions is provided in Sanborn et al. (2001). Beginning in October 1995, after treatment installation, total height and diameter at breast height (DBH) were recorded for the tagged pine trees, and these measurements were repeated in the autumn at 3-year intervals until year 15 (2010), and at 5-year intervals until year 25 (2020). Measurements of tagged alder clumps were made in the same years and consisted of total height, and crown width (two dimensions at right angles). Alder percent cover was measured by a line intercept survey along permanent transects which ran along the four sides and two diagonals of each assessment plot, giving total transect lengths of 129.5 m in each plot. At 1-m intervals along the transects, points falling beneath alder canopies were tallied and converted to an areal percentage. Alder cover was measured, by the same observer, in the same years as the other properties, except for the addition of 1996 (immediately post-treatment) and the delay of the planned year 20 measurement to 2016.
Soil Sampling and Analysis
Soil sample collection and handling followed the same procedures used at the time of treatment establishment (Sanborn et al., 2001). Samples were collected in July–August, 2020, at 30 random points within each treatment plot, and consisted of forest floor (sampled quantitatively within a 20 cm × 20 cm metal frame) and mineral soil (0–20 cm depth, sampled with a stony soil auger). Forest floor samples were air-dried, and extraneous materials were removed (mineral contaminants, living roots >1 mm diameter, living moss, and herbaceous plants). After this processing, air-dry weight was determined, and a sub-sample of each was oven-dried (105°C, 24 h) to determine moisture content. Unlike in 1995–1996, woody components were not removed from the forest floors for separate weighing and analysis. To enable comparison of forest floor mass and chemical composition between the two samplings, values for the total forest floor characteristics in 1995–1996 were calculated from the archived data for the non-woody and woody forest floor components which had originally been weighed and analyzed separately. Air-dried forest floor samples were ground to <2 mm. Living plant materials were removed from the mineral soil samples, which were air-dried and sieved (2 mm) to remove coarse fragments.
Chemical analyses were performed at the BC Ministry of Environment Analytical Chemistry laboratory and consisted of: total carbon (C), total nitrogen (N), and total sulphur (S) by the combustion method (Kowalenko and Grimmett, 2008; Rutherford et al., 2008; Skjemstad and Baldock, 2008) using a Flash 2000 CHNS combustion analyzer and mineralizable N by a 14-day anaerobic incubation (Curtin and Campbell, 2008), with NH4-N extracted with 1 N KCl and determined by auto-analyzer. Results were reported on an oven-dry basis (105°C, 24 h).
Foliar Analysis
Current-year foliage of lodgepole pine was collected in October 2020 from at least two live branches taken from the upper one third of the live crown of 10 trees in each assessment plot. Samples were frozen after collection, and then oven-dried (70°C, 24 h). An equally weighted composite of the dried foliage was prepared for each plot and ground with an electric coffee grinder prior to analysis. Total C, N, and S were determined by the combustion method (Flash 2000 CHNS combustion analyzer). Other elements were determined by Inductively Coupled Plasma –Mass Spectrometry after HNO3-HCl digestion with a Milestone Ultrawave system. Sulfate-S was extracted with 0.01 N HCl and determined by HPLC ion chromatography, using a PRP-X100 Hamilton column, and phthalate-buffered eluent (Kalra and Maynard, 1991).
Data Analysis
The effects of Sitka alder retention density on the height and crown width development of alder and on the DBH increment, height increment, and foliar nutrient concentration of lodgepole pine were subjected to analysis of variance. The classic model for a completely randomized design was applied to last-year (2020) measurements of foliage chemical properties. Year was incorporated as a split-plot factor for repeated measures model of alder, lodgepole pine and some soil properties measured over the full span of the experiment. A spatial power structure was assumed for the residual correlation (a generalization of a first-order autoregressive structure to handle the unequally spaced years). Overall tests of growth increments were conducted as separate customized multi-row contrasts within the repeated measures models. That is, for each time increment (i.e., span of time between successive measurement periods), each contrast tests by way of an F-test whether the mean change in DBH or height is different for at least one treatment level. Analogously, a contrast was created to test whether the 25-year growth increment (from 1995 to 2000) differed among treatment levels. Model adequacy was assessed graphically by ensuring the distribution of the studentized residuals, conditional on the predicted block effects, was more-or-less Gaussian. Also, using the same residuals we graphically checked for constant variance with respect to treatment levels and fitted observations. For attributes with a statistically significant overall treatment effect, multiple comparisons among all pairs of treatment levels were adjusted using the Holm correction (Holm, 1979) to preserve the familywise type I error rate. All models were fit using the mixed procedure, and multiple comparisons were handled with the multtest procedure in SAS Version 9.4 (SAS Institute Inc, 2019).
Results
Lodgepole Pine Growth
There was a significant treatment effect for DBH that was more-or-less consistent through time (Table 1 and Figure 2). The treatment effect for height changed through time, becoming significant by year 9 and remaining so for all years thereafter (Figure 2, tests not shown). Considering increments at specific time intervals, significant treatment effects were detected for DBH increment only for years 3–6, and for height increment for years 3–6, 15–20, 20–25, and over the complete length of the study (years 0–25). Over the 25 years, pine survival was high, with only 3% cumulative mortality (data not shown).
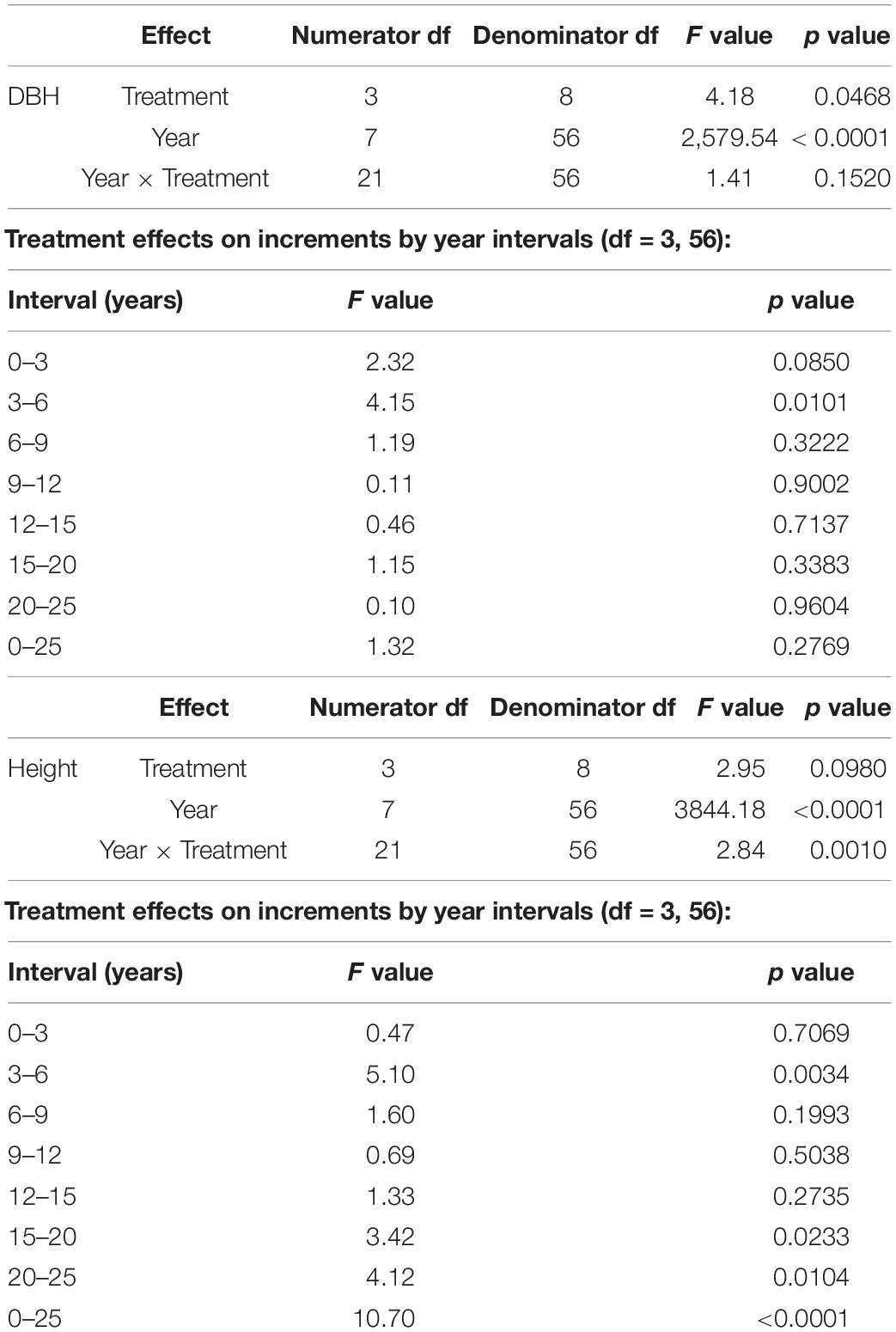
Table 1. ANOVA summary table for lodgepole pine diameter (DBH) and height, showing variance ratios (F), and p values.
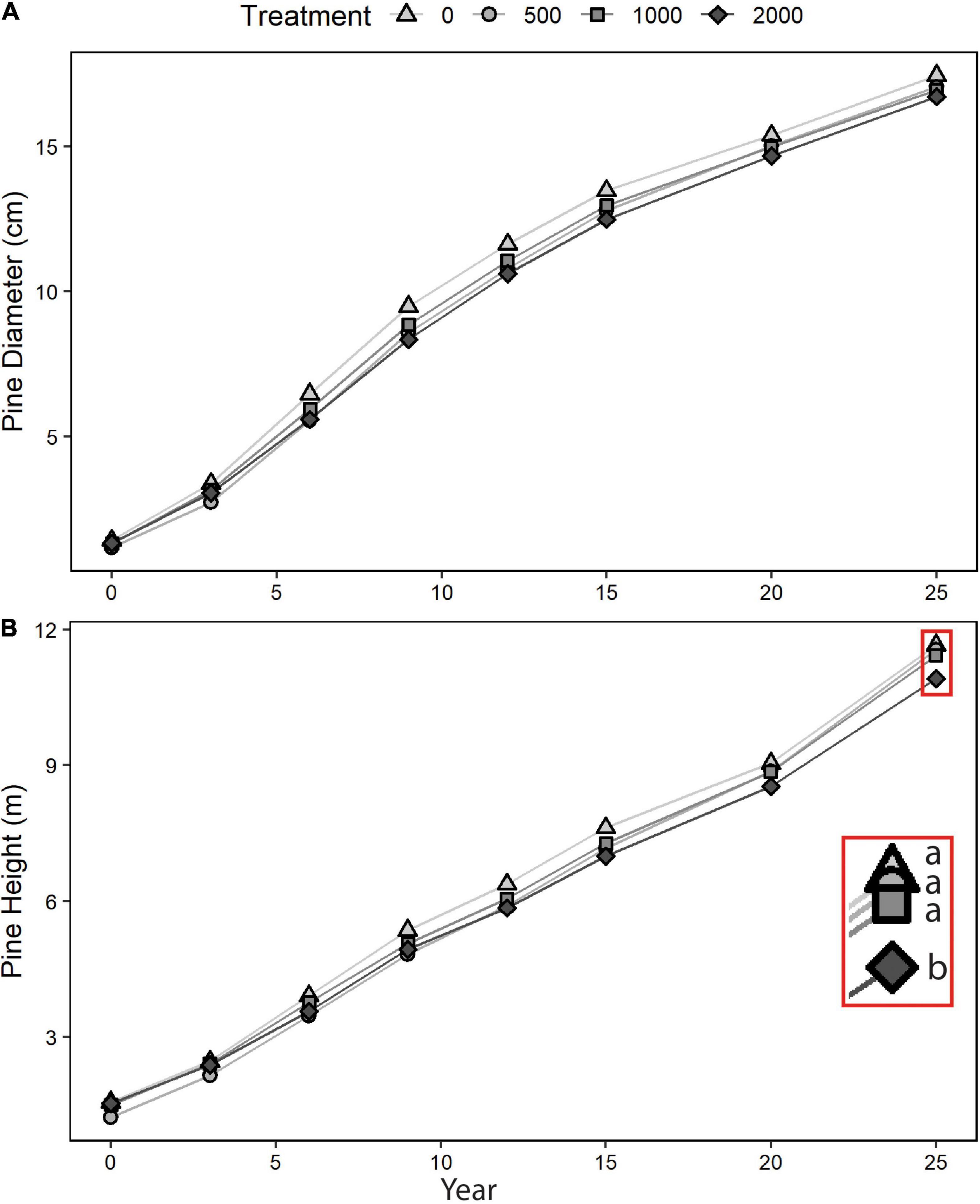
Figure 2. Lodgepole pine growth, 1995–2020: (A) diameter at breast height, and (B) height (treatment means). Different letters (a, b) indicate that year 0–25 increments were significantly different (p < 0.05) between treatments.
Sitka Alder Growth
Cumulative mortality of entire clumps was 18% over 25 years, with the majority occurring after year 15 (data not shown). The key trends observed were a peaking of alder canopy cover and clump dimensions between years 6 and 15 (Figure 3). The apparent convergence in canopy cover and clump width for the two highest alder retention treatments later in experiment (Figure 3) was due to large changes in just one replicate plot of each of those treatments. Clump height increment differed significantly with treatment over the duration of the experiment (years 0–25), and for years 3–6, 6–9, and 12–15 (Table 2). Clump width showed a slightly weaker relationship with density of alder retention (p = 0.0610; Table 2 and Figure 3).
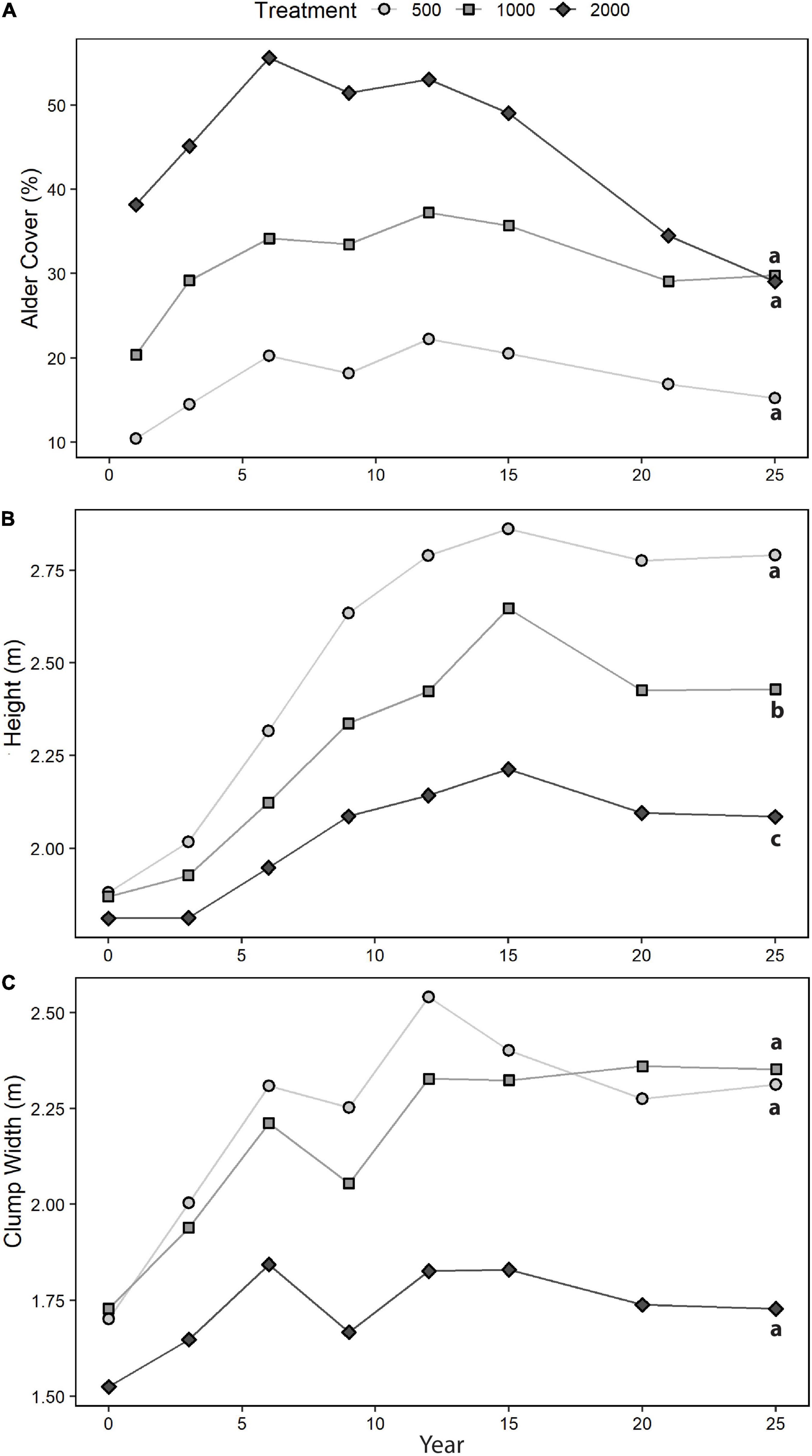
Figure 3. Sitka alder growth, 1995–2020: (A) canopy cover, (B) clump height, and (C) clump width (treatment means). Different letters (a, b, c) indicate that year 0–25 increments (year 1–25 for canopy cover) were significantly different (p < 0.05) between treatments.
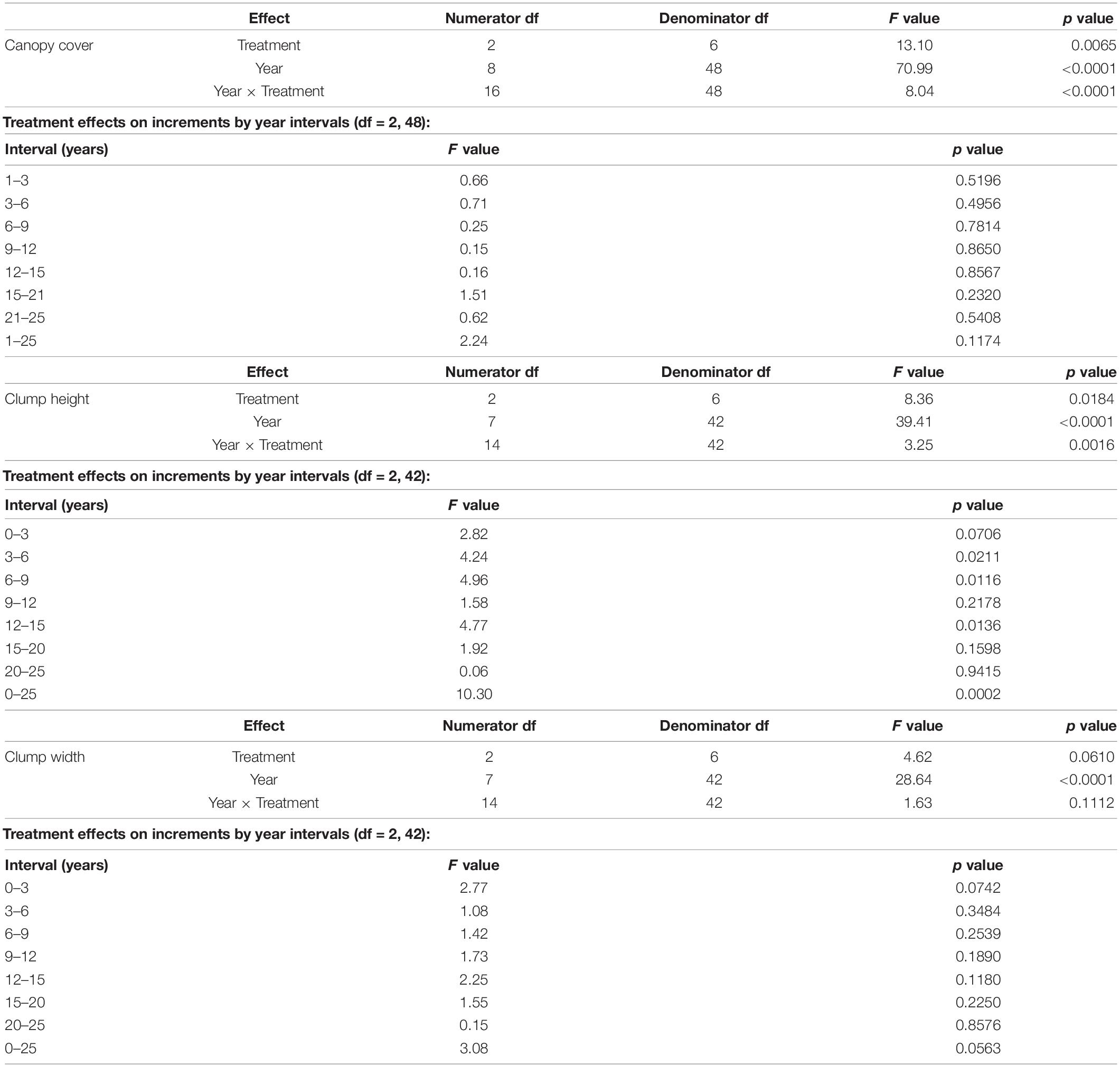
Table 2. ANOVA summary table for Sitka alder canopy cover, clump height, and mean clump width, showing variance ratios (F), and p values.
Soil Properties
For forest floors, total C, total S, mass per unit area (g/m2), and mineralizable N had no significant treatment effect, while for total N the F test for treatment (p = 0.0704) was suggestive of a somewhat stronger relationship with alder retention (Table 3 and Figure 4). For the 0–20 cm mineral soil, treatments with higher alder retention tended to have higher concentrations of total C and N, and mineralizable N; however, these patterns were only suggestive, with F tests of treatment effects coming closest to the threshold of p = 0.05 for total C and N (Table 3 and Figure 5). Comparing the year 25 and initial forest floor properties, the effect of time was significant for total C and S concentrations and forest floor mass (Table 3), with the latter decreasing by ∼40% (Figure 4). For 0–20 cm mineral soil, the effect of time was significant for C and total N, while only suggestive of a relationship (p = 0.0619) for mineralizable N.
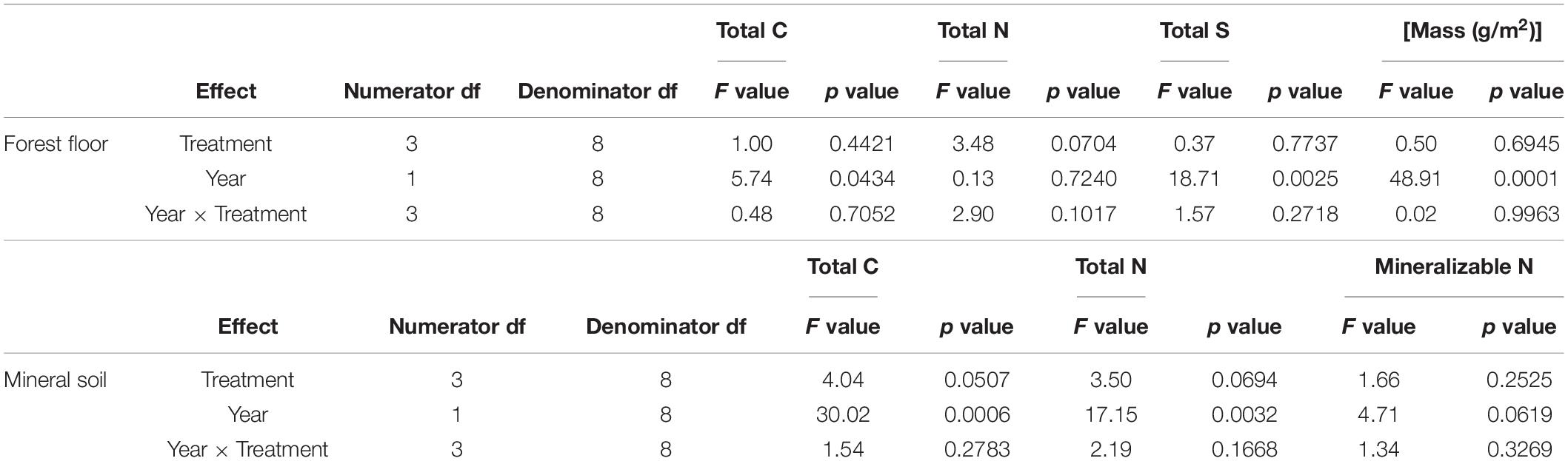
Table 3. ANOVA summary table for forest floor and mineral soil chemical properties (1995/1996–2020).
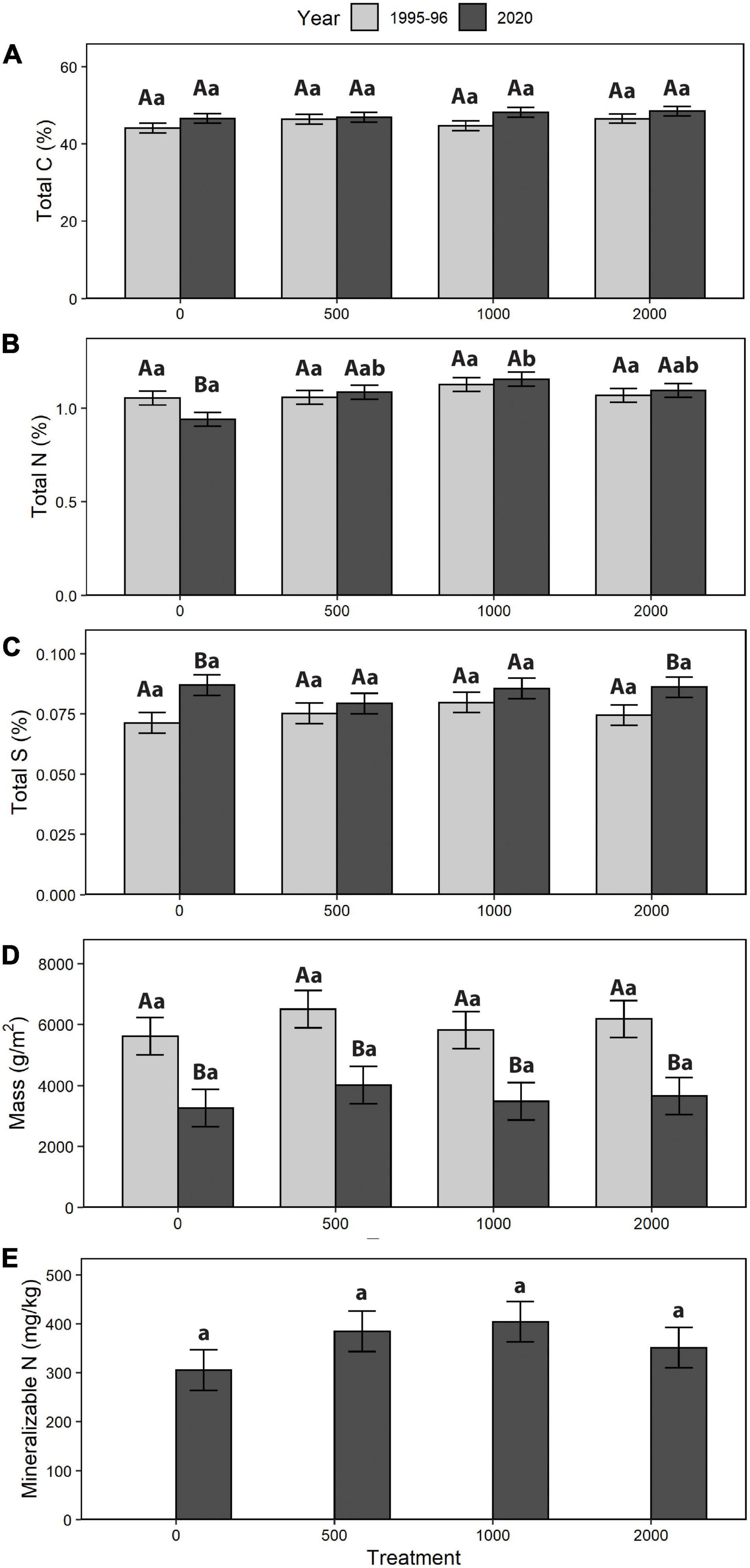
Figure 4. Forest floor change (1995/1996–2020): (A) total carbon (C), (B) total nitrogen (N), (C) total sulphur (S), (D) mass, and (E) mineralizable N (treatment means). Different letters indicate significant differences between years (A, B) or treatment within a year (a, b; p < 0.05). For mineralizable N, comparable data were not available for 1995/1996, so only treatment differences in 2020 were analyzed (F = 1.10, p = 0.4031, df = 3, 8).
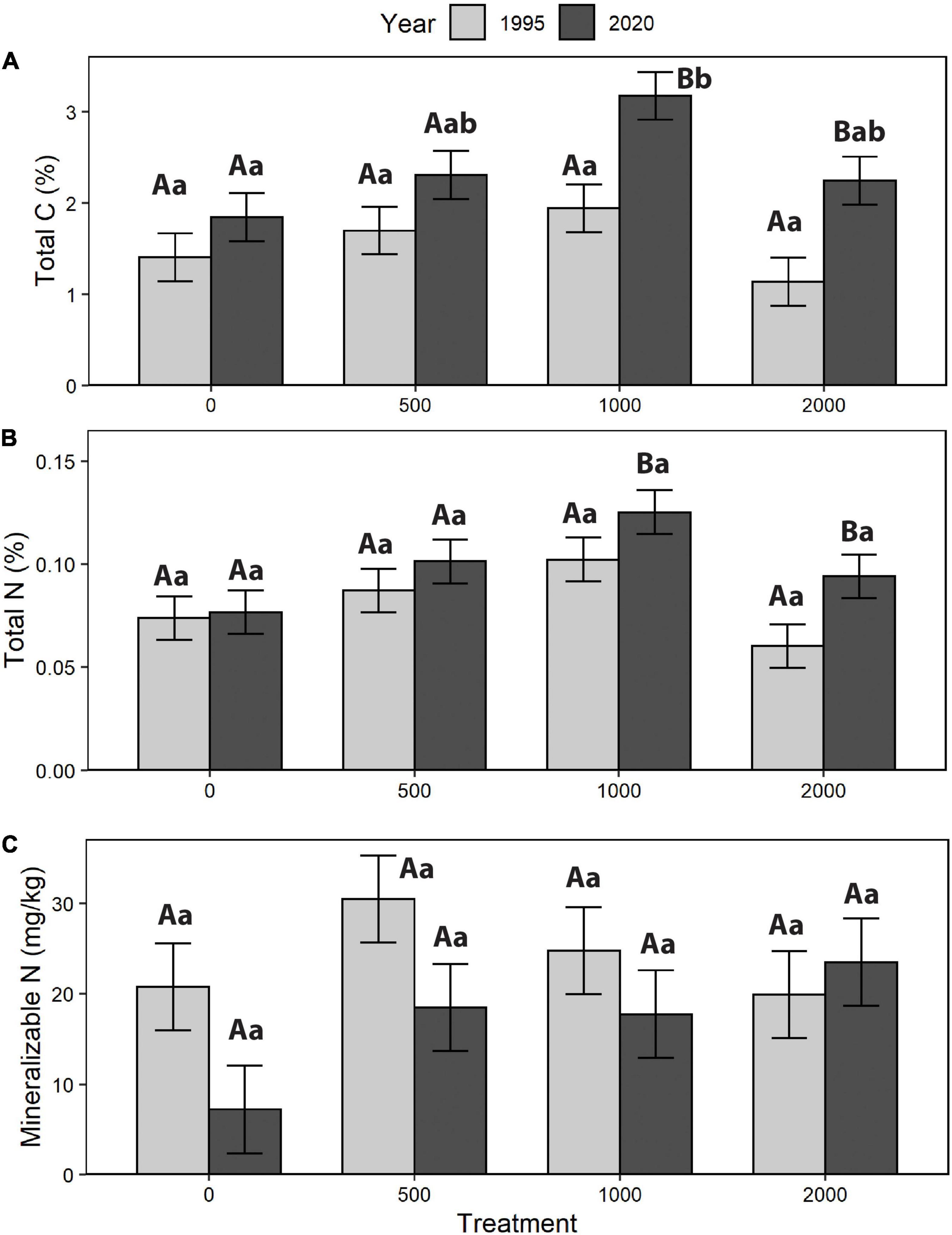
Figure 5. Mineral soil change (1995–2020): (A) total carbon (C), (B) total nitrogen (N), and (C) mineralizable N (treatment means). Different letters indicate significant differences between years (A, B) or treatment within a year (a, b; p < 0.05).
Lodgepole Pine Foliar Chemistry
Of the six major nutrient elements determined (Table 4 and Figure 6), only total S concentrations displayed a significant treatment effect. Treatment effects were not significant for any of the nutrient ratios examined (N:S, N:P, N:K; Table 4 and Figure 7). Boron and sulfate-S concentrations (not shown) were below detection limits for almost all samples.
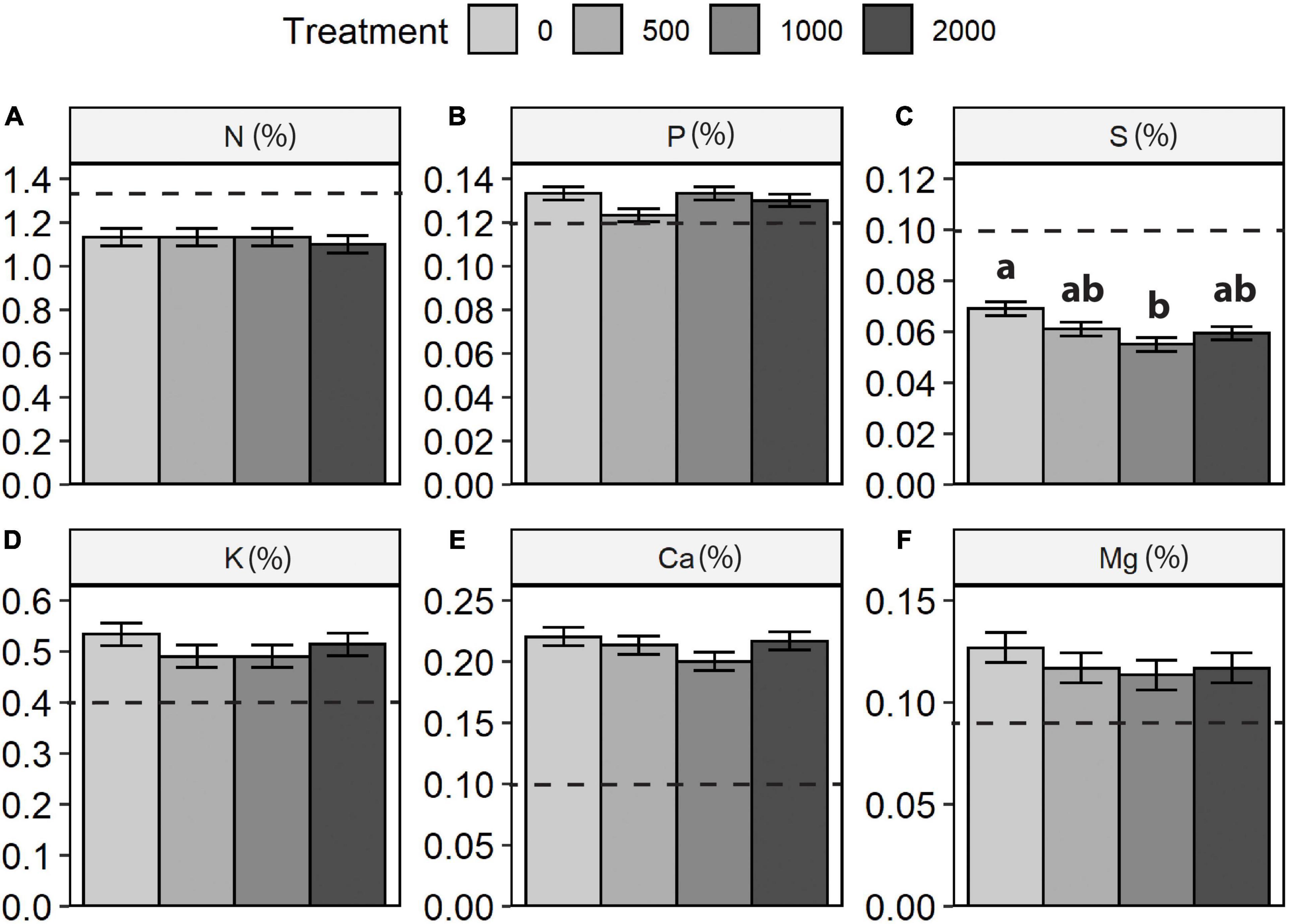
Figure 6. Lodgepole pine foliar nutrient concentrations (2020): (A) nitrogen (N), (B) phosphorus (P), (C) sulphur (S), (D) potassium (K), (E) calcium (Ca), and (F) magnesium (Mg). Dashed horizontal lines indicate minimum adequate concentrations (Brockley, 2001). Where a significant treatment effect occurred (Table 4), different letters (a, b) indicate significant differences between treatments (p < 0.05).
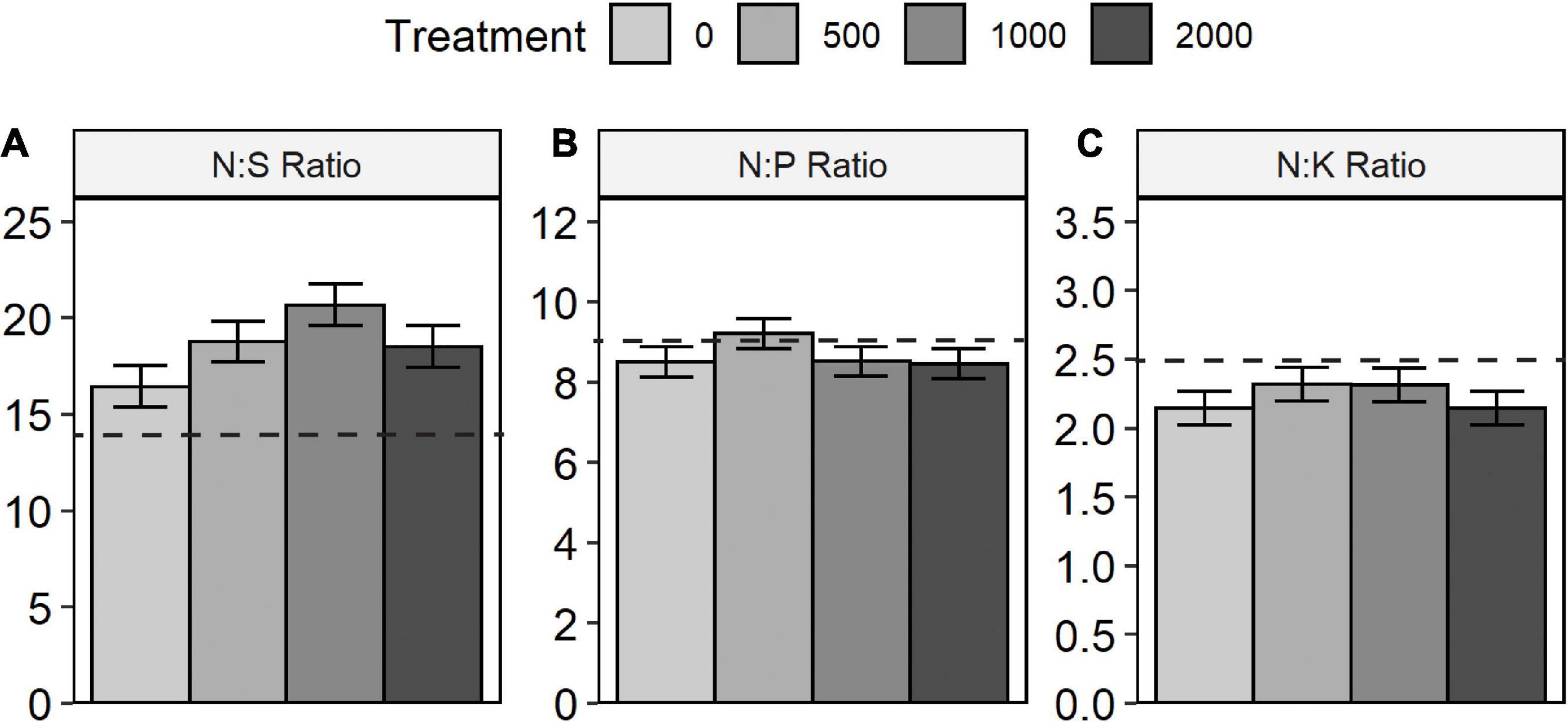
Figure 7. Lodgepole pine foliar nutrient ratios with nitrogen (N): (A) N:S (sulfur), (B) N:P (phosphorus), and (C) N:K (potassium). Values below dashed horizontal lines indicate no deficiency of S, P, or K, respectively, (Brockley, 2001).
Discussion
Growth Responses
Despite the initial overwhelming presence of Sitka alder (>50% cover) at this site in 1994, the experimental manipulations which ranged from complete elimination to retention of approximately 75% of its initial cover have had subtle rather than dramatic effects. In terms of crop tree performance, while there were apparent treatment effects for various time intervals – in effect, a growth reduction for lodgepole pine when more alder was retained – their ultimate biological or economic significance are not yet clear. For example, comparing treatment means at year 9, the difference in pine height from maximum to minimum alder retention was 0.42 m or ∼8% of mean height. By year 25, this separation had increased to 0.74 m but this represented only 6.5% of mean height. Continued monitoring would be needed to determine whether any such small differences persist to the end of the rotation.
Despite some differences in treatment design in the only other similar field experiment conducted in the BC interior, the magnitude of alder removal impacts on lodgepole pine growth in this study appeared to be generally smaller than those detected by year 15 in the stand studied by Simard et al. (2006) in southern BC. In the latter study, lodgepole pine was planted rather than retained from existing natural regeneration, and pine density was greater (1,600 vs. 1,000 stems/ha). Although pine mortality was much higher in the initial years of that study, the saplings in the present study could have been up to 8 years old when the treatments were implemented, so an early wave of seedling mortality could have already run its course.
Although alder biomass was not measured directly, the peaking of canopy cover, clump height, and clump width between years 6 and 15 suggests that biomass of this understory shrub had begun to decline well before the year 25 measurements. Although these three measures may serve as proxies for aboveground biomass, they likely underestimate the degree to which biomass was decreasing, as suggested by observed large losses (estimated at >50%) of living stems in most surviving clumps. In addition, visual comparison of early successional and mature stand conditions suggests a long-term trend to thinner alder canopies (Figure 1). Observations of mature pine stands within ∼10 km of the study site in 1994 (Figure 1) suggested that alder heights under closed pine canopies reach a maximum of approximately 3.0–4.0 m, so more than half of this shrub’s ultimate height growth had already been achieved by year 25 of the study. The apparent decline in alder biomass in the second decade of this experiment likely reflected increased shading combined with episodic moisture stress during any drier-than-usual summers, exacerbated by the sandy textures of surface soils (Sanborn et al., 2001).
Nutrient Dynamics
The difference in N concentration between the treatment with no alder vs. all treatments with alder retention was small: 0.94 vs. 1.11%. Despite such potential gains in the total N stocks, their nutritional significance is unclear since mineralizable N concentrations in both forest floors and mineral soil displayed no treatment effect. Given that mean forest floor mass in 2020 was ∼4 kg/m2, this suggests that the alder retention treatments were accompanied by forest floor N stocks which averaged ∼70 kg/ha higher than in the plots lacking alder. Such a result appears to be of the right order of magnitude when compared to the independent estimate of annual N-fixation by alder of 10–15 kg/ha in the highest retention treatment (2,000 clumps/ha; Sanborn et al., 2002). If such a rate had continued for a decade prior to the apparent peak in alder biomass, and at half that rate subsequently through year 25, it could account for a total input of as much as ∼300 kg N/ha in the highest retention treatment, which would be distributed through the forest floor and other ecosystem compartments (mineral soil, above- and belowground biomass).
Such interpretations become more complex when long-term changes in soil properties over the 25-year interval are considered. Forest floor mass change was much greater than any differences in nutrient concentration and averaged ∼40% loss across all treatments. This result is consistent with other findings of reduction of forest floor mass following harvesting, presumably due to accelerated mineralization and initially reduced litterfall (Nave et al., 2010; James et al., 2021). Since the magnitude of forest floor mass loss was much greater than the small, non-significant change in total N concentration over 25 years, this suggests that the net result was a reduction of forest floor total N stock. As a first approximation, if the average forest floor mass loss of ∼2,400 g/m2, with a N concentration of slightly over 1%, had been due to decomposition, potentially a net amount of ∼250 kg N/ha had been mineralized over 25 years. Since the underlying 0–20 cm mineral soil exhibited only a modest increase in N concentration (along with a larger C increase) over that interval, the mineralized N was incorporated in the biomass of the developing pine stand and/or was leached below 20 cm depth in the mineral soil. Given the dry climate of this area, the former is more likely. The gain in C concentration in the upper mineral soil may reflect belowground biomass inputs by herbaceous vegetation which could have been prolonged at an elevated level by delayed canopy closure.
Our results for mineralizable N as determined by the anaerobic incubation method contrast with those of Simard et al. (2006) who employed aerobic incubation methods, initially in the laboratory and then by the in situ buried bag method. We did not detect any strong treatment effects of alder retention on mineralizable N in either forest floor or 0–20 cm mineral soil, while Simard et al. (2006) found significant differences between treatments with and without alder retention for ammonium- and nitrate-N production during aerobic incubation of mineral soil. Given such ambiguities, it would be useful for follow-up work to compare both approaches to assessing N availability at a single site, using identical soil sampling methods.
The magnitude of alder-derived N contributions as this lodgepole pine stand develops further is uncertain. Previous work (Sanborn et al., 2002) indicated that even under low light conditions in ∼150 year-old pine canopies (18.1% of open sky values), approximately 80–95% of the N in alder tissues had originated from the atmosphere. However, the total amount of N being fixed on an area basis in such stands may be quite small if alder biomass has already plateaued earlier in stand development. Future estimates of alder biomass production using the allometric equation similar that obtained previously (Sanborn et al., 2002) could be combined with resampling and analysis of the heavily 15N-labeled mini-plots established in that earlier work, to estimate N-fixation rates in the main experimental plots.
According to interpretive criteria provided by Brockley (2001), foliar concentrations indicated moderate to severe deficiency of both N and S, while P, K, Ca, and Mg were adequate. Nutrient ratios supported these interpretations, with the N:S ratios giving a further indication of S deficiency (Brockley, 2001), which is not unique to this site but illustrates a regional pattern across the BC interior (Sanborn et al., 2005). Previous foliar analyses had shown that the weakening of treatment-related differences in N nutrition had occurred between years 6 and 9 (Brockley and Sanborn, 2007), and this pattern was maintained at year 25. Such results suggest that any nutritional benefits for lodgepole pine from Sitka alder retention are largely restricted to the first two decades of stand development when the alder shrub understory is experiencing maximum light availability.
Conclusion
A young, naturally regenerated lodgepole pine stand in the central BC interior had inherited a legacy of ∼4,000 clumps/ha of N-fixing Sitka alder from the previous stand. Eight years after logging, this tree-shrub mixture was manipulated to create four levels of alder retention within uniformly thinned pine. Twenty-five years after implementation of these treatments, the initial modest negative impacts of alder retention on pine growth have waned further, and the nutritional benefits of alder indicated by pine foliar nutrient concentrations have not been detected since year 6. Despite robust early estimates of N-fixation rates, the long-term contribution of this source to maintenance of site N stocks remains difficult to quantify from repeated sampling and analysis of forest floors and upper mineral soils. Potential N fluxes resulting from post-harvest decomposition and mineralization of the inherited forest floor may be comparable in magnitude to estimated N inputs from symbiotic fixation in this ecosystem. In view of these caveats, the long-term benefits of alder removal for pine growth at this site remain uncertain, while a conservative approach to managing soil nutrient stocks would favor retention of alder in these ecosystems with only occasional selective interventions to manipulate its density.
Data Availability Statement
The raw data supporting the conclusions of this article will be made available by the authors, without undue reservation.
Author Contributions
PS conducted field work, data compilation, and wrote most of the manuscript. PO conducted the statistical analyses, advised on their interpretation, and contributed to the manuscript. Both authors contributed to the article and approved the submitted version.
Funding
Initial funding was provided by Forest Renewal BC (1996–1999), and subsequently by the research program of the BC Ministry of Forests and its successor ministries.
Conflict of Interest
The authors declare that the research was conducted in the absence of any commercial or financial relationships that could be construed as a potential conflict of interest.
Publisher’s Note
All claims expressed in this article are solely those of the authors and do not necessarily represent those of their affiliated organizations, or those of the publisher, the editors and the reviewers. Any product that may be evaluated in this article, or claim that may be made by its manufacturer, is not guaranteed or endorsed by the publisher.
Acknowledgments
This study would not have happened without the substantial effort by Rob Brockley in choosing the research site, designing and implementing the study design, collecting data, and analyzing the early results. We thank former BC Ministry of Forests colleagues Rick Trowbridge, Anne Macadam and Suzanne Simard for their encouragement of N-fixation research in BC interior forests. We are grateful for the diligent maintenance of E.P. 1185 and data management and sharing by George Harper, Dave Goldie, and Louise de Montigny after Rob Brockley’s retirement. We thank Colin Chisholm Emma Peasgood and Diana Castro for their field assistance in 2020, and the Aleza Lake Research Forest Society for enabling their participation. Brayden Nilson created the graphs.
References
Arocena, J. M., and Sanborn, P. (1999). Mineralogy and genesis of selected soils and their implications for forest management in central and northeastern British Columbia. Can. J. Soil Sci. 79, 571–592. doi: 10.4141/S98-071
Berg, B., Erhagen, B., Johansson, M.-B., Vesterdal, L., Faituri, M., Sanborn, P., et al. (2013). Manganese dynamics in decomposing needle and leaf litter — a synthesis. Can. J. For. Res. 43, 1127–1136. doi: 10.1139/cjfr-2013-0097
Berg, B., Johansson, M.-B., Liu, C., Faituri, M., Sanborn, P., Vesterdal, L., et al. (2017). Calcium in decomposing foliar litter – a synthesis for boreal and temperate coniferous forests. For. Ecol. Manage. 403, 137–144. doi: 10.1016/j.foreco.2017.08.022
Berg, B., Sun, T., Johansson, M.-B., Sanborn, P., Ni, X., Åkerblom, S., et al. (2021). Magnesium dynamics in decomposing foliar litter – A synthesis. Geoderma. 382, 114756. doi: 10.1016/j.geoderma.2020.114756
Brockley, R. (2001). Foliar Sampling Guidelines and Nutrient Interpretive Criteria for Lodgepole Pine: Extension Note 52. Victoria: BC Ministry of Forests, 8.
Brockley, R., and Sanborn, P. (2003). Effects of Sitka alder on the growth and foliar nutrition of young lodgepole pine in the central Interior of British Columbia. Can. J. For. Res. 33, 1761–1771. doi: 10.1139/x03-098
Brockley, R., and Sanborn, P. (2007). Assessing the Effects of Sitka Alder on the Growth and Foliar Nutrition of Young Lodgepole Pine in Central British Columbia (SBSdw3): 9-year Results. Extension Note 79. Victoria: BC Ministry of Forests, 8.
Cortini, F., and Comeau, P. G. (2008). Evaluation of competitive effects of green alder, willow and other tall shrubs on white spruce and lodgepole pine in Northern Alberta. For. Ecol. Manage. 255, 82–91. doi: 10.1016/j.foreco.2007.08.027
Curtin, D., and Campbell, C. A. (2008). “Mineralizable nitrogen,” in Soil Sampling and Methods of Analysis, 2nd Edn, eds M. R. Carter and E. G. Gregorich (Boca Raton, FL: CRC Press), 599–606.
DeLong, C., Tanner, D., and Jull, M. J. (1993). A Field Guide for Site Identification and Interpretation for the Southwest portion of the Prince George Forest Region. Land Management Handbook 24. Victoria: BC Ministry of Forests, 290.
Doran, K., Ruess, R. W., Plumley, F. G., and Wurtz, T. L. (2001). Photosynthetic responses of white spruce saplings (Picea glauca) to controlled density gradients of spruce and green alder (Alnus crispa). Ecoscience 8, 76–88. doi: 10.1080/11956860.2001.11682633
Haeussler, S., Coates, D., and Mather, J. (1990). Autecology of Common Plants in British Columbia: a Literature Review. FRDA Report 158. Victoria: BC Ministry of Forests, 272.
IUSS Working Group WRB (2015). World Reference Base for Soil Resources 2014, update 2015. International Soil Classification System for Naming Soils and Creating Legends for Soil Maps. World Soil Resources Reports No. 106. Rome: Food and Agriculture Organization, 192.
James, J., Page-Dumroese, D., Busse, M., Palik, B., Zhang, J., Eaton, B., et al. (2021). Effects of forest harvesting and biomass removal on soil carbon and nitrogen: two complementary meta-analyses. For. Ecol. Manage. 485:118935. doi: 10.1016/j.foreco.2021.118935
Kalra, Y. P., and Maynard, D. G. (1991). Methods Manual for Forest Soil and Plant Analysis. Inf. Rep. NOR-X-319. Edmonton: Northern Forestry Centre, 116.
Kowalenko, C. G., and Grimmett, M. (2008). “Chemical characterization of soil sulfur,” in Soil Sampling and Methods of Analysis, 2nd Edn, eds M. R. Carter and E. G. Gregorich (Boca Raton, FL: CRC Press), 251–263.
Nave, L. E., Vance, Swanston, C. W., and Curtis, P. S. (2010). Harvest impacts on soil carbon storage in temperate forests. For. Ecol. Manage. 259, 857–866. doi: 10.1016/j.foreco.2009.12.009
Rhoades, C., Oskarsson, H., Binkley, D., and Stottlemeyer, B. (2001). Alder (Alnus crispa) effects on soils in ecosystems of the Agashashok River valley, northwest Alaska. Ecoscience 8, 89–95. doi: 10.1080/11956860.2001.11682634
Rutherford, P. M., McGill, W. B., Arocena, J. M., and Figueiredo. (2008). “Total nitrogen,” in Soil Sampling and Methods of Analysis, 2nd Edn, eds M. R. Carter and E. G. Gregorich (Boca Raton, FL: CRC Press), 239–250.
Salmon, V. G., Breen, A. L., Kumar, J., Lara, M. J., Thornton, P. E., Wullschleger, S. D., et al. (2019). Alder distribution and expansion across a tundra hillslope: implications for local N cycling. Front. Plant Sci. 10:1099. doi: 10.3389/fpls.2019.01099
Sanborn, P., Brockley, R., Mayer, B., Yun, M., and Prietzel, J. (2005). Sulphur Fertilization of Lodgepole Pine: a Stable Isotope Tracer Study (E.P. 886.15): Establishment Report. Technical Report 020. Victoria: BC Ministry of Forests, 50.
Sanborn, P., Preston, C., and Brockley, R. (2001). Effects of Sitka Alder Retention and Removal on the Growth of Young Lodgepole pine in the Central Interior of British Columbia (E.P. 1185). Establishment report. Working Paper 60. Victoria: BC Ministry of Forests, 44.
Sanborn, P., Preston, C., and Brockley, R. (2002). N2-fixation by Sitka alder in a young lodgepole pine stand in central interior British Columbia, Canada. For. Ecol. Manage. 167, 223–231.
Sanborn, P. T., and Brockley, R. P. (2009). Decomposition of pure and mixed foliage litter in a young lodgepole pine-Sitka alder stand in the central interior of British Columbia. Can. J. For. Res. 39, 2257–2262.
Simard, S. (1990). Competition between Sitka Alder and Lodgepole Pine in the Montane Spruce Zone in the Southern Interior of British Columbia. FRDA Report 150. Victoria: BC Ministry of Forests, 26.
Simard, S., Heineman, J. L., Hagerman, S. M., Mather, W. J., and Sachs, D. L. (2004). Manual cutting of Sitka alder-dominated plant communities: effects on conifer growth and plant community structure. W. J. Appl. For. 19, 277–287. doi: 10.1093/wjaf/19.4.277
Simard, S., Radosevich, S. R., Sachs, D. L., and Hagerman, S. M. (2006). Evidence for competition and facilitation tradeoffs: effects of Sitka alder density on pine regeneration and soil productivity. Can. J. For. Res. 36, 1286–1298. doi: 10.1139/x06-040
Skjemstad, J. O., and Baldock, J. O. (2008). “Total and organic carbon,” in Soil Sampling and Methods of Analysis, 2nd Edn, eds M. R. Carter and E. G. Gregorich (Boca Raton, FL: CRC Press), 225–237.
Soil Classification Working Group (1998). The Canadian System of soil Classification: Publ. 1646, 3rd Edn. Ottawa: Agriculture and Agri-Food Canada, 187.
Soil Survey Staff (1999). Soil taxonomy. Agriculture Handbook No. 436. Washington, DC: United States Department of Agriculture, 870.
Urli, M., Thiffault, N., Houle, D., Gauthier, S., and Bergeron, Y. (2020). Role of green alder in boreal conifer growth: competitor or facilitator? FACETS 5, 166–181. doi: 10.1139/facets-2019-0064
Keywords: Sitka alder, lodgepole pine, nitrogen, forest soils, silviculture
Citation: Sanborn P and Ott PK (2022) Tree Growth, Foliar Nutrition, and Soil Properties in Mixtures of Lodgepole Pine and Sitka Alder in the Central Interior of British Columbia, Canada: 25-Year Results. Front. For. Glob. Change 5:867247. doi: 10.3389/ffgc.2022.867247
Received: 31 January 2022; Accepted: 17 May 2022;
Published: 06 June 2022.
Edited by:
Sm’hayetsk Teresa Ryan, University of British Columbia, CanadaReviewed by:
Rongzhou Man, Ontario Ministry of Northern Development, Mines, Natural Resources and Forestry, CanadaBianca Eskelson, University of British Columbia, Canada
Copyright © 2022 Sanborn and Ott. This is an open-access article distributed under the terms of the Creative Commons Attribution License (CC BY). The use, distribution or reproduction in other forums is permitted, provided the original author(s) and the copyright owner(s) are credited and that the original publication in this journal is cited, in accordance with accepted academic practice. No use, distribution or reproduction is permitted which does not comply with these terms.
*Correspondence: Paul Sanborn, UGF1bC5TYW5ib3JuQHVuYmMuY2E=