- 1Department of Geography, Institute of Ecology and Earth Sciences, University of Tartu, Tartu, Estonia
- 2Department of Ecosystem Trace Gas Exchange, Global Change Research Institute of the Czech Academy of Sciences, Brno, Czechia
- 3Research Institute of the Peruvian Amazon, Iquitos, Peru
- 4School of Forestry, National University of the Peruvian Amazon, Iquitos, Peru
- 5Lawrence Berkeley National Laboratory, Berkeley, CA, United States
Tree stems in tropical peat swamp forests are known as considerable methane (CH4) emitters; however, little is known about their carbon dioxide (CO2) and nitrous oxide (N2O) exchange. Differences between species, especially the role of palm stems in the exchange of greenhouse gasses, have remained largely unknown. We measured stem CO2, CH4, and N2O fluxes from the different heights of widely spread aguaje palms (Mauritia flexuosa) and boarwoods (Symphonia globulifera) and the soil beneath the same trees in a Peruvian Amazon palm swamp using a static closed chamber technique from September 2019 to March 2020. The tree stems were the net emitters of CO2 and CH4 but occasionally showed low N2O uptake. We found the highest stem CH4 emissions (average ± SE) from palm stems of the height of 80 cm (1,601 ± 165.9 μg C m–2 h–1), which are more than 300 times greater compared to the highest fluxes from boarwood stems, at the height of 30 cm (5.12 ± 1.27 μg C m–2 h–1). The average soil CH4 flux was 3,618 ± 465 μg C m–2 h–1. Whereas N2O fluxes from the stems were negligible, the average N2O fluxes from soils beneath the same trees were relatively high, ranging from 53.75 ± 24.04 (close to boarwood trees) to 143.4 ± 68.43 (close to palms) μg N m–2 h–1. While roughly upscaling tree-level fluxes to the stand level of 27,732 km2 of palm swamp in the Pastaza-Marañon foreland basin, these forests are net annual emitters of CH4 and N2O (897 Gg C y–1 and 24 Gg N y–1, respectively). These results highlight the necessity to study this kind of ecosystem more intensely.
Introduction
Peatlands are globally important ecosystems covering an area of 463.2 Mha (Leifeld and Menichetti, 2018). However, tropical peatlands, which make up to 11% of the global peatland area (Leifeld and Menichetti, 2018), are the most efficient terrestrial ecosystems for carbon (C) sequestration (Yu et al., 2010). As a long-term global soil organic C stock, they sequester up to 88.6 Gt of C (Page et al., 2011; Gumbricht et al., 2017). Although peatlands are a significant source of atmospheric methane (CH4), vastly altered by anthropogenic pressure (Petrescu et al., 2015), peatlands are the key ecosystems in the climate change mitigation strategy.
The Peruvian Amazon is the home to one of the largest areas of tropical peatlands globally (Page et al., 2011; Draper et al., 2014; Gumbricht et al., 2017). The region is characterized as aguaje palm (Mauritia flexuosa) dominant swamp forest peatland (Draper et al., 2014; Bhomia et al., 2019). The number of studies on the C pools of the Peruvian Amazon peatlands has increased in recent years (Lähteenoja et al., 2009a,b; Draper et al., 2014).
Peatlands influence climate through the uptake and release of greenhouse gasses (GHG) (van Lent et al., 2019). In addition to carbon dioxide (CO2) and CH4, they may also emit or uptake nitrous oxide (N2O). All these gasses are claimed to be the main contributors to global warming.
Carbon dioxide emission from the peatland depends on the balance between plant C fixation through photosynthesis and ecosystem respiration. While CH4 is produced in the anaerobic soil of peatlands by methanogens, CH4 is oxidized in the aerobic zones by methanotrophs, and fixed carbon is released back to the atmosphere as CO2 (Le Mer and Roger, 2001). Meanwhile, N2O production and uptake is a microbial-mediated enzyme-driven process via nitrification (the aerobic oxidation of ammonia to nitrate) and denitrification (the reduction of nitrate to N2O and ultimately to inert N2 under anaerobic conditions) (Syakila and Kroeze, 2011). In tropical peatlands, other pathways can also be significant sources of N2O. In nitrate-limited conditions, these processes can be DNRA (dissimilatory nitrate reduction to ammonium) (Espenberg et al., 2018) or nitrifier denitrification (nitrification in which ammonia is oxidized to nitrite followed by the reduction of nitrite to nitric oxide, N2O and N2) (Hergoualc’h et al., 2020).
A gas pathway from the soil through herbaceous plants, adapted to high soil water content, to the atmosphere is well-studied (Scholander et al., 1955; Henneberg et al., 2012). Plants exposed to permanent or episodic flooding cope with soil anoxia through morphological adaptations such as the formation of adventitious water roots, hypertrophied lenticels and enlarged aerenchyma (Shimamura et al., 2010; Pangala et al., 2013). Trees can take up the soil-produced GHG by their roots and release it by their aboveground tree surfaces into the atmosphere; however, trees’ role in the production and consumption of these has not been thoroughly studied (Machacova et al., 2016; Maier et al., 2018; Barba et al., 2019). Plant stems are particularly efficient conduits of CH4 from the soil because the pathway bypasses highly active methanotrophic bacteria populations at the oxic-anoxic interface under the soil surface (Brune et al., 2000). Palms have adventitious root systems spread broadly throughout the shallow soil to anchor the plant (Steffens and Rasmussen, 2016). The root systems enhance gas exchange between the atmosphere and the soil (Megonigal and Day, 1992), in particular, entry of oxygen to the root zone and exit of soil-generated GHG through stems and aerial roots (pneumatophores) into the atmosphere (van Lent et al., 2019). Several studies have demonstrated that temperate trees adapted to wet soil also facilitate egress of soil-produced CH4 (Gauci et al., 2010; Rice et al., 2010; Machacova et al., 2013; Pangala et al., 2013) via gas transport through aerenchymous tissue, which allows the exchange of gasses between the shoot and the root, and emission to the atmosphere through stem lenticels (Pangala et al., 2014).
Few studies have considered stem CH4 fluxes in tropical trees (Pangala et al., 2017; Welch et al., 2019; Sjögersten et al., 2020; van Haren et al., 2021). For instance, in the Central Amazon basin, Pangala et al. (2017) have found significantly higher CH4 fluxes from mature tree stem than from the soil. However, very little is currently known about the magnitudes and patterns of stem CO2 and N2O fluxes from different plant species, especially the role of Peruvian Amazon dominant palms, which have remained largely unknown. Therefore, this study aimed to estimate GHG fluxes from palm and tree stems and adjacent soil, and roughly upscale the fluxes to the whole aguaje palm (M. flexuosa) covered area in the region.
Based on the results of previous studies, we hypothesize that:
• CH4 and N2O emissions from palm stems are higher than those from hardwood stems.
• CH4 fluxes from palm and tree stems exceed the soil fluxes.
• Soil N2O and CH4 fluxes are higher during the wetter season.
Materials and Methods
Description of the Study Site
The research was conducted in a palm peat swamp (3°50′03′′S, 73°19′08′′W; 101 m a.s.l.), located close to Lake Quistococha and southwest of the city of Iquitos (Department of Loreto, the Peruvian Amazon; Supplementary Figure 1), from September 2019 to March 2020. The regional climate is humid tropical, classified as Af in the Köppen-Geiger system (Peel et al., 2007). The area receives excessive rainfall during the whole year, with a long-term (1999–2019) average annual precipitation of 2,859 mm. The wetter season lasts from December to April and the dryer season from June to October (Yoon and Zeng, 2010). Based on long-term (1999–2019) weather data, monthly average precipitation for wetter and dryer seasons are 295 and 163 mm, respectively. The region’s average annual air temperature is 27.2°C (1999–2019), and the relative air humidity is around 80–90% year-round. All the weather data originate from the Puerto Almendra weather station (6.5 km from the study site), Iquitos, Peru.
Peat deposits up to 6 m thick have been reported by Lähteenoja et al. (2009b). The area has no drainage, and the water table rarely decreases more than 20 cm below the soil surface as Lake Quistococha stabilizes the soil water level in the study site (Kelly et al., 2014; Griffis et al., 2020). The region occasionally floods; Roucoux et al. (2013) mention flooding events in 1998 (30 cm) and 2012 (100 cm). Neighboring peatlands likely receive nutrients from the Amazon River during these annual flooding events. Both minerotrophic (nutrient-rich) and ombrotrophic (nutrient-poor) peatlands have been reported in the region (Lähteenoja et al., 2009a; van Lent et al., 2019). Teh et al. (2017) characterized the Quistococha peat swamp forest as a transition between the minerotrophic and ombrotrophic types.
N Content in Soil
Soil samples were collected close to each chamber (n = 10) at two soil depths: 0–10 and 25–35 cm in September 2019 and March 2020. The soil samples were stored at 5°C and transported to the Estonian University of Life Sciences Laboratory, Estonia, for the chemical analyses. The soil’s average N content (Kjeldahl method) was 30,115 and 25,733 mg/kg in September 2019 and 30,778 and 28,500 mg/kg in March 2020, at a soil depth of 0–10 and 25–35 cm, respectively.
Gas Flux Measurements From Tree Stems and Soil
Stem GHG fluxes were measured from two plant species: aguaje palm (M. flexuosa L.f.) from the Arecaceae family representing palm trees and boarwood (Symphonia globulifera L.f.) representing hardwoods. These species are the most common of their family in the region by the study of Roucoux et al. (2013).
Five replicates per both species (n = 5 per species, in total n = 10) were selected randomly and gas samples were collected using the static closed tree stem chamber method, as described by Schindler et al. (2020). At each measurement level, two chambers were placed on the opposite side of the tree stem, and the installation was done 4 days before the first measurement session in September 2019. The rectangular-shaped chambers were made of transparent plastic containers, including removable airtight lids (Lock & Lock, South Korea). Before the installation, the bottom of the chamber was cut and hot-glued with a neoprene band. The chambers were sealed to the smoothed stem surface with the cord (Supplementary Figure 2) and checked for gas-tightness with a portable CO2 gas analyzer (EGM-5, PP Systems International, Inc., MA, United States).
The chambers were installed at the tree stem’s base, as close to the ground as possible (25–35 cm). In addition, to assess the tree stem vertical flux profile, on three stems per plant species, the fluxes were also studied at the heights of 75–85 and 165–175 cm above the ground (Schindler et al., 2020). During each measurement session from all measurement levels, four gas samples (25 ml) were taken through septa from both closed chambers (12.5 ml from both chambers) as the mixed gas samples in a 60 min interval (a 0–60–120–180 min sequence) and stored in pre-evacuated (0.3 bar) gas-tight glass vials (Labco Limited, Lampeter, United Kingdom). After the sample collection, the chamber remained open till the next measurement session.
Soil GHG fluxes from soils beneath the same trees were measured simultaneously with stem flux measurements using the static closed soil chamber method (Hutchinson and Livingston, 1993). PVC collars of 0.5 m diameter (Supplementary Figure 2) and 0.1 m depth were placed into the peat close to each studied tree (n = 10) 4 days before the first measurement session. For the gas sampling, white opaque PVC 65 L truncated conical chambers were placed into water-filled rings (ensure the airtightness between the collar and chamber) on top of the collars. Gas was sampled from chamber headspace into a pre-evacuated 50 mL glass vial every 20 min during a 1-h session (Soosaar et al., 2011).
All gas samples were transported to the laboratory at the Department of Geography, University of Tartu and gas concentrations were analyzed within 2 weeks using gas chromatography (Shimadzu GC-2014, Shimadzu, Kyoto, Japan) equipped with an electron capture detector for CO2 and N2O and a flame ionization detector for CH4 (Soosaar et al., 2011).
The planned measurement period was a minimum of 1 year. Due to technical issues from October to December 2019 (difficulties with new gas bottles delivery to Iquitos) and the COVID-19 outbreak in spring 2020 (restrictions on internal movement), the field campaign had to be canceled in March 2020. Altogether, nine measurement sessions were conducted: 16, 17, 24, and 25 September 2019, 11 and 25 January, 8 and 22 February, and 4 March 2020.
Data Quality Check and Gas Flux Calculation
The GHG concentration changes in the chamber were calculated from the slope of the least-squares linear regression of the samples’ gas concentrations over time. The adjusted coefficient of determination (R2) value of the linear fit was used to assert each chamber session’s goodness. When the R2 value for CO2 flux was above 0.95, both CH4 and N2O fluxes were accepted regardless of their R2 value.
To compare soil and stems contribution into ecosystem flux, the average stem fluxes across the three heights were up-scaled to a soil surface area for each measurement session using the formula from Machacova et al. (2016). Average values of the parameters used for the calculation were following (Roucoux et al., 2013): (1) aguaje palm – stem diameter 0.293 m, height 21.3 m, and stand density 186 trees per 10,000 m2; (2) boarwood – stem diameter 0.166 m, height 15.1 m, and 62 trees per 10,000 m2, respectively. The average calculated value of stem surface area per soil surface area for palm and boarwood stems were 0.18 and 0.025 m2 m–2, respectively.
Ancillary Measurements
The sampling site was equipped with a 1-m deep observation well (a 50-mm perforated PP-HT pipe wrapped in filter textile) from where the water-table depth during the gas sampling was recorded.
Soil water content was measured at 5 cm with a GS3 sensor connected to a ProCheck handheld reader (Decagon Devices, Pullman, WA, United States), soil temperature was measured at four different depths (10, 20, 30 and 40 cm) with an S0141 four-channel temperature data logger and four PT1000 sensors (COMET SYSTEM, Roznov pod Radhostem, Czechia) next to each studied tree and soil chamber.
Statistical Analyses
The normality of the gas fluxes distribution was checked using the Shapiro–Wilk test and as the data distribution deviated from the normal distribution, non-parametric tests were used. The Kruskal–Wallis one-way ANOVA on ranks test was used to check if tree height influence stems GHG fluxes and Spearman’s Rank-Order Correlation to determine the strength and direction of the relationship between the soil and stem GHG fluxes. Mann–Whitney U test was used to compare the soil flux close to palm and boarwood trees. Statistical analyses were done using Sigmaplot (version 12, Systat Software, San Jose, CA, United States) and Statistica (version 7, Stat Soft Inc., OK, United States) programs. The level of significance of p = 0.05 was accepted in all cases.
Results
Monthly precipitation during the study period differed from the long-term pattern (1999–2019), being slightly lower than typical for the wetter season (January to March; Supplementary Figure 3); however, we follow the traditional climatic division in the hereafter and throughout the text. Soil temperature values at 10–40 cm showed low variation, from 24.2 to 27.8°C.
Variation of Greenhouse Gasses Fluxes From Stems
All stems were the net emitters of CO2 and CH4 but occasionally showed low N2O uptake. Stem CO2 emission of both species varied seasonally and between months. The highest average stem CO2 emissions (±SE) were from the height of 80 cm (57.8 ± 3.81 and 97.1 ± 9.25 mg C m–2 h–1 for palm and boarwood, respectively). Stem CO2 flux was significantly higher from the boarwood stems during the wetter season. Average stem CH4 emissions were significantly (p < 0.05) larger from palm than boarwood for all stem heights. Palms showed the highest emissions at the height of 80 cm (1,601 ± 165.9 μg C m–2 h–1), while boarwood stems had the highest flux values from the lowest measurement level of 30 cm (6.71 ± 1.53 μg C m–2 h–1; Table 1, Figure 1, and Supplementary Figure 4). Palm stem CH4 fluxes showed significant differences between the measurement heights (p < 0.05), while boarwood stem CH4 fluxes showed an insignificant decrease with stem heights. The average CH4 emission from stems was higher in the dryer than during the wetter season (Figure 1). In contrast to the CH4 fluxes, very low and similar stem N2O fluxes were detected in both species and at all measurement levels, ranging from −0.6 to 1.7 μg N m–2 h–1 (Table 1). N2O fluxes from the stems were higher in the dryer season compared to the wetter season (Figure 1).
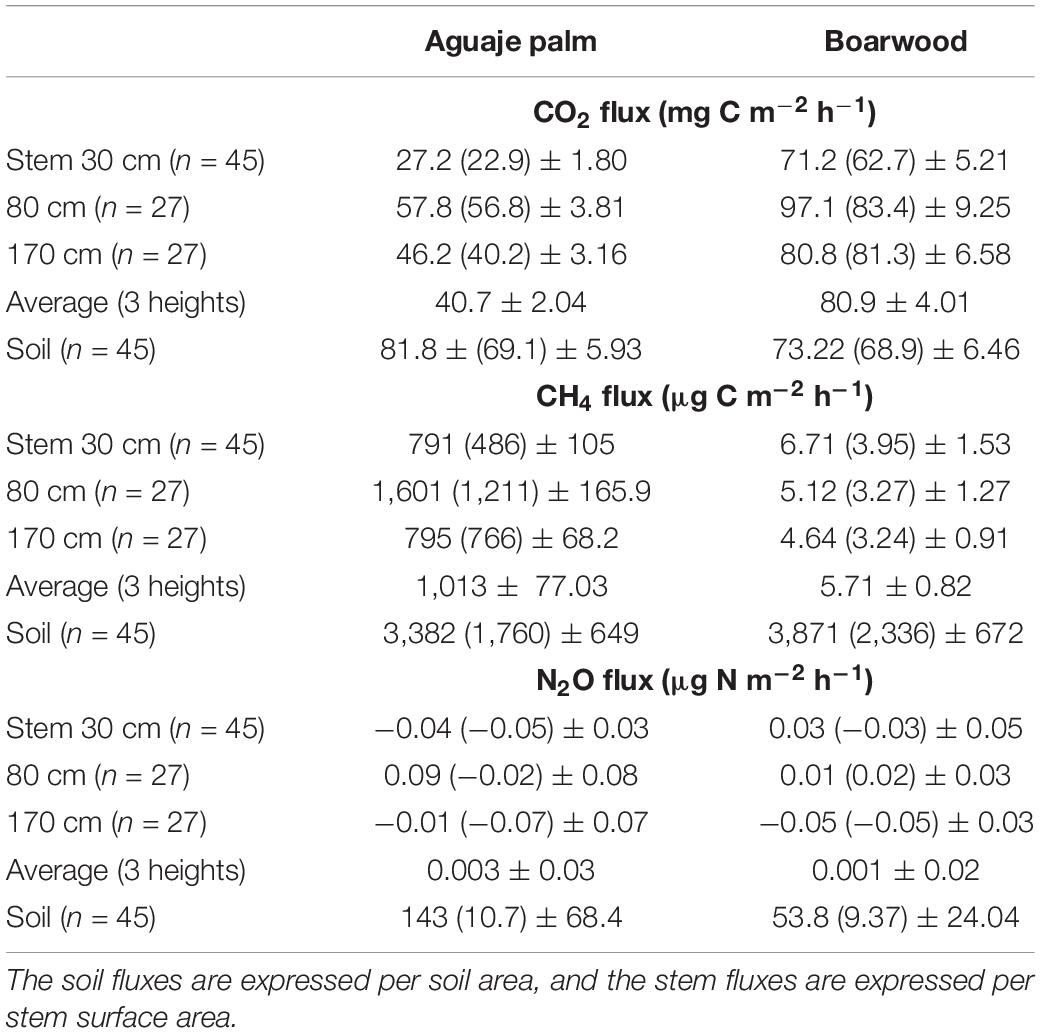
Table 1. Average, median (in parentheses) ± SE stem CO2, CH4, and N2O fluxes from different stem heights and the soil in the Quistococha peat swamp forest.
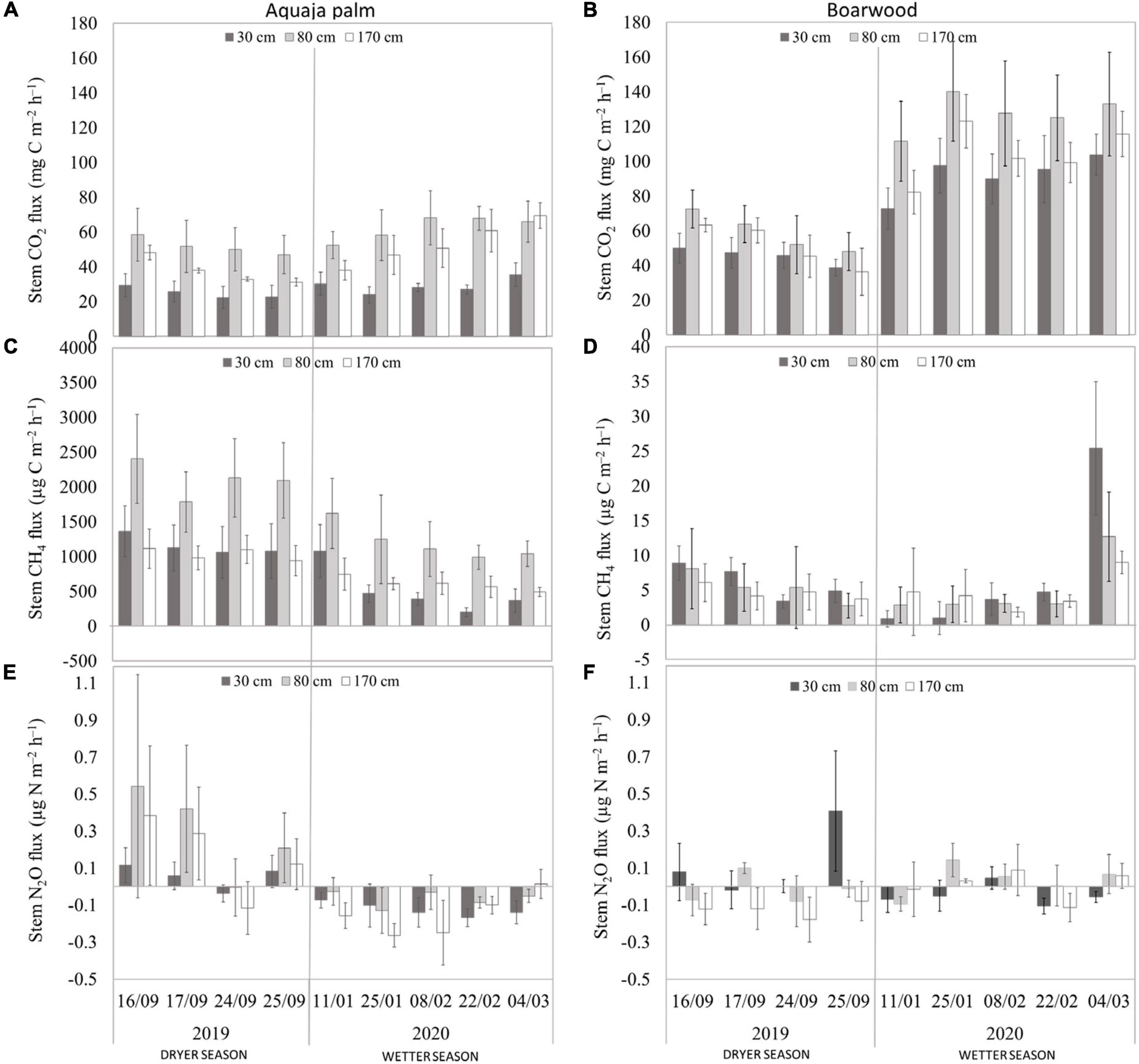
Figure 1. Temporal variation of stem CO2 (A,B), CH4 (C,D), and N2O (E,F) fluxes (session average ± SE) from different heights of aguaje palm (A,C,E) and boarwood (B,D,F) stems. Note that y-axis for stem CH4 (C,D) fluxes have different scales.
Variation of Soil Greenhouse Gasses Fluxes
Average soil CO2 emission (±SE) was 77.2 ± 4.44 mg C m–2 h–1, being the highest at the beginning of September 2019. Soil CH4 fluxes ranged from 220.9 to 19,476 μg C m–2 h–1 with an average (±SE) of 3,618 ± 465 μg C m–2 h–1, being always a net emitter. The highest CH4 emissions were measured at the end of January and in February 2020, while the lowest were in the dryer season, in September 2019 (Figure 2). Soil CH4 emissions near the boarwood were slightly higher than close to the palms (Table 1), but no significant differences were found (Mann–Whitney U test, p < 0.05).
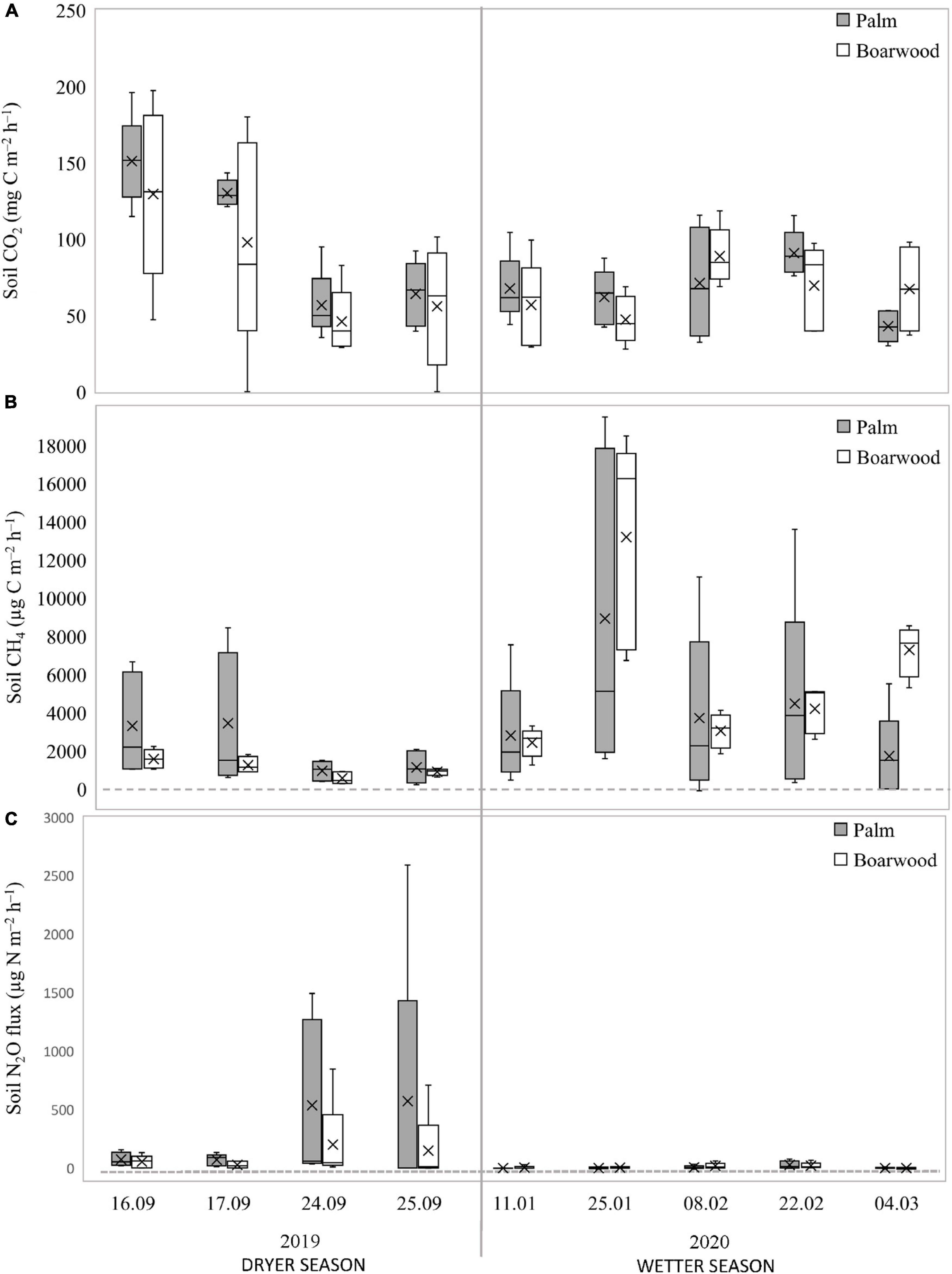
Figure 2. Temporal variation of average soil CO2 (A), CH4 (B), and N2O (C) fluxes. Median (dash line inside the box), average (cross inside the box), 25 and 75% quartiles (lower and higher lines of the box), min and max values (whiskers) are shown.
Soil N2O fluxes had large temporal and spatial variability, ranging from −8.62 to 2,590 μg N m–2 h–1. Average soil N2O fluxes close to the palms (143 ± 68.4 μg N m–2 h–1) exceeded the fluxes next to the boarwoods (53.8 ± 24.04 μg N m–2 h–1; Table 1 and Supplementary Figure 5); however, without detection of significant differences (Mann–Whitney U test, p < 0.05). The highest soil N2O emissions were recorded on 24–25 September 2019, immediately after heavy rainfall, preceded by a dry period of several days. There was no significant correlation between the soil and stem fluxes of CH4 and N2O for both species.
Average net ecosystem CH4 and N2O flux, consisting of flux from palm and boarwood stems (converted to ground area unit under consideration of mean “tree” height and density of both species in the studied forest) and soil, were 3,632 ± 1,015 μg C m–2 h–1 and 107 ± 55 μg N m–2 h–1, respectively. Soil CH4 flux contributes to 95% of the total CH4 emissions (Figure 3). The proportion of stem N2O flux from ecosystem flux was close to zero.
Discussion
The soil CH4 fluxes (average 3,617 μg C m–2 h–1) in the studied Quistococha palm swamp forest were within the same range of fluxes reported from similar studies in Brazilian flooded swamp forests in the Amazon river basin (Pangala et al., 2017) but lower than fluxes from palm swamps in the Southern Peruvian Amazon (Winton et al., 2017). Palm stem CH4 emissions were an order of magnitude lower than the stem CH4 fluxes of various tree and palm species reported by Pangala et al. (2017) and van Haren et al. (2021). The main differences between current and previous studies could be due to the regional soil water regime. The palm swamp forest soil was relatively dry during our study period, with significantly lower precipitation than the long-term average (Supplementary Figure 3). In our study period, the water table varied from −0.125 to +0.08 m (Griffis et al., 2020). In contrast, the water table in the Brazilian floodplain during the flood was up to 8 m higher than during the non-flooding period (Pangala et al., 2017).
At the end of dryer season (September), at a water level above −0.005 m, CH4 fluxes from the palm stems were relatively high compared to the soil fluxes. That was likely caused by the oxidation of CH4 in the aerated upper soil layer and, thus, by egress of CH4 from deeper anoxic soil layers through the aerenchymatous palm roots and stems into the atmosphere (Figures 1, 2). These structures have intercellular (lacunose) spaces, which can serve as transport conduits for soil-produced CH4 into the palm stems and finally into the atmosphere (Supplementary Figure 2; van Lent et al., 2019).
Average N2O soil fluxes measured in our study were 2.5–7 times higher than model-predicted emissions of 21 μg N m–2 h–1 for the Amazon Basin (Guilhen et al., 2020) but still lower than N2O emissions measured by Figueiredo et al. (2019) from floodplain soils in the Brazilian Amazon. In Amazonian palm swamps, no previous studies on N2O stem fluxes are known. Seasonal variations in N2O emissions are most likely correlated with seasonal changes in the water table and soil nitrogen mineralization. In our study, the higher N2O production during the dryer period just after the heavy rainfall could indicate the presence of nitrate or other denitrification product in these soils and the effect of pulsing water level (Pärn et al., 2018).
As CH4 is produced in the anaerobic soil by methanogens, the main factor influencing the soil’s methane production is the groundwater level (Lai, 2009). During our study, the soil CH4 emission significantly increased when the soil surface was flooded in the wetter season (January–March). However, N2O emission showed an opposite trend, being significantly lower during the wetter season than the dryer.
Emissions of CH4 from stems differed by several orders of magnitude among the two studied plant species. This finding supports the results from other studies in temperate and tropical peatland ecosystems, where species-specific differences have been measured (Sjögersten et al., 2020; van Haren et al., 2021). Stem CH4 emissions were significantly (p < 0.05) larger from palms than boarwood. This finding agrees with the study by van Haren et al. (2021). Higher CH4 emissions from the palm stems might be explained by porosity of adventitious roots and stems (Jackson and Armstrong, 1999), tree specific wood density (Pangala et al., 2013) and stem water content (Wang et al., 2017), which significantly affect the gas diffusivity through stem wood.
The mean stem CO2 fluxes from palm and boarwood stems (40.7 and 80.9 mg C m–2 h–1, respectively) in this study were in the same range as presented in overview study by Yang et al. (2016) for tropical forest ecosystems (58 mg C m–2 h–1). Emitted stem CO2 could originate from different sources. A portion of CO2 produced in the soil can be transported from below-ground through the xylem and during the transport diffused to the atmosphere (Teskey et al., 2008). Another part of stem CO2 flux originates from a plant’s growth and maintenance respiration and is released to the atmosphere as autotrophic respiration (Lavigne and Ryan, 1997).
While studying the tree flux profile, we found the peak of GHG fluxes at the 80 cm height of palm stems (Supplementary Figure 4) which is contradictory to other previous studies in temperate and other tropical trees, where stem CH4 flux rates decreased either linearly or exponentially with increasing stem height (Sjögersten et al., 2020; van Haren et al., 2021). In the case of palm trees, it might be explained by an effect of the lowest part of pseudobark. Outside of the central cylinder of the palm stem is a region of sclerified tissue known as the cortex and thin epidermis, which is referred to as the pseudobark (Broschat, 2013). The lowest part of the pseudobark is detached from the cortex and functional stem tissues due to the growth of adventitious roots from the root initialization zone (functional stem tissues) below the bark (Tomlinson, 1990). The GHG flux from the bottommost part of the pseudobark, which could be disconnected from the cortex as the new roots of the palm may force the cortex and pseudobark to split (Broschat, 2013), might be influenced.
The contribution of tree stem CH4 fluxes constituted 4% of the total fluxes of soil and stems, significantly lower than reported in previous mesocosm studies with Alnus glutinosa saplings by Pangala et al. (2014). The share of N2O stem fluxes was negligible in our study.
While roughly upscaling, our CH4 measurements to the 27,732 ± 1,101 km2 of palm swamp in the Pastaza-Marañon foreland basin (Draper et al., 2014) yield 897 Gg C y–1. For N2O, the upscaled estimate is 24 Gg N y–1. Using global warming potentials (100 years, with feedbacks) of 31 for CH4 and 298 for N2O (Myhre et al., 2013), the annual warming effect of these gasses for the Peruvian palm peat swamps is 27.8 and 7.1 Tg CO2 eq y–1, respectively. Nevertheless, this should be taken as an initial and rough estimate. A more spatial and temporal cover is needed for a reliable account. The ongoing increase in droughts will likely increase both N2O and CH4’s role in the radiative balance of the whole Amazon Basin (Abalos et al., 2017; Zemp et al., 2017).
Conclusion
Our study shows that stems of aguaje palm (M. flexuosa), a dominant plant species in West Amazonian peatlands, can emit large amounts of CH4, which is significantly higher than from boarwood (S. globulifera) stems. Thus, our first hypothesis was confirmed. Stem N2O fluxes were low and we found no differences between the species. In contrast to our second hypothesis, the average soil CH4 flux was significantly higher than flux from the stems: 3,617 μg C m–2 h–1 and 193.8 μg C m–2 h–1, respectively. The stem CH4 emission was about a magnitude lower than reported previously for trees in the Amazon floodplain. The CH4 fluxes were higher during the wetter season, while soil N2O showed higher values during the dryer season. We suggest that future studies focus on plant species composition, stem anatomy, stand density, and other key factors that control emissions of CH4 across Amazonian peatland plant species.
Data Availability Statement
The raw data supporting the conclusions of this article will be made available by the authors, without undue reservation.
Ethics Statement
Field studies on plants were performed in accordance with relevant institutional, national, and international guidelines and legislation.
Author Contributions
ÜM, KS, KM, JP, and RN-J: conceptualization. KS, KM, ÜM, JP, TS, and LF-M: methodology. TS, JP, KM, KS, ÜM, JJ-A, JR-M, LF-M, RZ-G, DG-D, and AA-O: investigation (field studies). KS, KM, ÜM, JP, and TS: data validation, quality check, formal analysis, data curation, and writing – original draft preparation. ÜM, KM, KS, LF-M, and WA-M: resources. KS, JP, KM, ÜM, TS, LF-M, RZ-G, and RN-J: writing – review and editing. ÜM, LF-M, RT-E, and WA-M: supervision. KS, JP, KM, ÜM, TS, LF-M, RN-J, TP-G, RT-E, and WA-M: project administration. ÜM, KM, JP, LF-M, WA-M, and TP-G: funding acquisition. All authors have read and agreed to the published version of the manuscript.
Funding
This study was supported by the Ministry of Education and Science of Estonia (grant SF0180127s08), the Estonian Research Council (PRG-352 and MOBERC20), the Czech Science Foundation (17-18112Y), SustES – Adaptation strategies for sustainable ecosystem services and food security under adverse environmental conditions (CZ.02.1.01/0.0/0.0/16_019/0000797), the EU through the European Regional Development Fund (EcolChange and MOBTP101 returning researcher grant by the Mobilitas Pluss program) and LIFE program project “Demonstration of climate change mitigation potential of nutrients rich organic soils in Baltic States and Finland” (LIFE OrgBalt, LIFE18 274CCM/LV/001158).
Conflict of Interest
The authors declare that the research was conducted in the absence of any commercial or financial relationships that could be construed as a potential conflict of interest.
Publisher’s Note
All claims expressed in this article are solely those of the authors and do not necessarily represent those of their affiliated organizations, or those of the publisher, the editors and the reviewers. Any product that may be evaluated in this article, or claim that may be made by its manufacturer, is not guaranteed or endorsed by the publisher.
Acknowledgments
We thank the staff of the U.S. Department of Energy (DOE), U.S. Agency International Development (USAID), U.S. Forest Service, University of Minnesota, University of Missouri, Michigan Tech University, Arizona State University, Oak Ridge National Lab, SilvaCarbon, Dirección de Comercio Exterior, Turismo y Artesanía (DIRCETURA), Gobierno Regional de Loreto (GORE-Loreto), and Parque Turístico Quistococha for economic, managerial and scientific support.
Supplementary Material
The Supplementary Material for this article can be found online at: https://www.frontiersin.org/articles/10.3389/ffgc.2022.849186/full#supplementary-material
Supplementary Figure 1 | Location of the study site at the Lake Quistococha shore.
Supplementary Figure 2 | Chamber profile system on a boarwood stem (A) and an aguaje palm stem (B), a soil collar (C), soil chamber on flooded soil (D), a cross-section of a Mauritia palm stem (E), and a view on Quistococha peat swamp forest (F).
Supplementary Figure 3 | Variation of long-term precipitation and during the study at area (average ± SE). Data originate from the Puerto Almendra weather station, Iquitos, Peru.
Supplementary Figure 4 | Stem CO2 (A,B), CH4 (C,D), and N2O (E,F) fluxes from three stem heights (30, 80, and 170 cm) of aguaje palm (A,C,E) and boarwood (B,D,F) stems. Median (dash line inside the box), average (cross inside the box), 25 and 75% quartiles (lower and higher lines of the box), min and max values (whiskers), and outliers (dots) are shown. Different lowercase letters above the columns indicate significant difference between stem heights (Kruskal–Wallis analysis of variance test, p < 0.05).
Supplementary Figure 5 | Soil CH4 (A) and N2O (B) fluxes in the palm swamp forest. Median (dash line inside the box), average (cross inside the box), 25 and 75% quartiles (lower and higher lines of the box), min and max values (whiskers), and outliers (dots) are shown. No significant differences between adjacent soil to palm and boarwood stems were found (Kruskal–Wallis analysis of variance test, p < 0.05).
References
Abalos, D., Groenigen, J. W., and De Deyn, G. B. (2017). What plant functional traits can reduce nitrous oxide emissions from intensively managed grasslands? Global Chan. Biol. 24, e248–e258. doi: 10.1111/gcb.13827
Barba, J., Bradford, M. A., Brewer, P. E., Bruhn, D., Covey, K., Van Haren, J., et al. (2019). Methane emissions from tree stems: a new frontier in the global carbon cycle. New Phytol. 222, 18–28. doi: 10.1111/nph.15582
Bhomia, R. K., Van Lent, J., Rios, J. M. G., Hergoualc’h, K., Coronado, E. N. H., and Murdiyarso, D. (2019). Impacts of mauritia flexuosa degradation on the carbon stocks of freshwater peatlands in the pastaza-maranon river basin of the peruvian amazon. Mitigat. Adapt. Strate. Global Chan. 24, 645–668.
Broschat, T. K. (2013). Palm Morphology and Anatomy: ENH1212/EP473, 5/2013. EDIS, 2013. Available online at: https://journals.flvc.org/edis/article/view/121032
Brune, A., Frenzel, P., and Cypionka, H. (2000). Life at the oxic-anoxic interface: microbial activities and adaptations. Fems Microbiol. Rev. 24, 691–710. doi: 10.1111/j.1574-6976.2000.tb00567.x
Draper, F. C., Roucoux, K. H., Lawson, I. T., Mitchard, E. T. A., Honorio Coronado, E. N., Lähteenoja, O., et al. (2014). The distribution and amount of carbon in the largest peatland complex in Amazonia. Environ. Res. Lett. 9:124017. doi: 10.1088/1748-9326/9/12/124017
Espenberg, M., Truu, M., Mander, Ü, Kasak, K., Nõlvak, H., Ligi, T., et al. (2018). Differences in microbial community structure and nitrogen cycling in natural and drained tropical peatland soils. Sci. Rep. 8:4742. doi: 10.1038/s41598-018-23032-y
Figueiredo, V., Pangala, S. R., Peacock, M., Gauci, V., Bastviken, D., and Enrich-Prast, A. (2019). Contribution of trees to the N2O budget of Amazon floodplain forest. Geophys. Res. Abstr. 21, EGU2019–EGU18212.
Gauci, V., Gowing, D. J. G., Hornibrook, E. R. C., Davis, J. M., and Dise, N. B. (2010). Woody stem methane emission in mature wetland alder trees. Atmos. Environ. 44, 2157–2160. doi: 10.1016/j.atmosenv.2010.02.034
Griffis, T. J., Roman, D. T., Wood, J. D., Deventer, J., Fachin, L., Rengifo, J., et al. (2020). Hydrometeorological sensitivities of net ecosystem carbon dioxide and methane exchange of an Amazonian palm swamp peatland. Agric. Forest Meteorol. 295:108167. doi: 10.1016/j.agrformet.2020.108167
Guilhen, J., Al Bitar, A., Sauvage, S., Parrens, M., Martinez, J. M., Abril, G., et al. (2020). Denitrification and associated nitrous oxide and carbon dioxide emissions from the Amazonian wetlands. Biogeosciences 17, 4297–4311. doi: 10.5194/bg-17-4297-2020
Gumbricht, T., Roman-Cuesta, R. M., Verchot, L., Herold, M., Wittmann, F., Householder, E., et al. (2017). An expert system model for mapping tropical wetlands and peatlands reveals South America as the largest contributor. Global Change Biol. 23, 3581–3599. doi: 10.1111/gcb.13689
Henneberg, A., Sorrell, B. K., and Brix, H. (2012). Internal methane transport through Juncus effusus: experimental manipulation of morphological barriers to test above- and below-ground diffusion limitation. New Phytol. 196, 799–806. doi: 10.1111/j.1469-8137.2012.04303.x
Hergoualc’h, K., Dezzeo, N., Verchot, L. V., Martius, C., Van Lent, J., Del Aguila-Pasquel, J., et al. (2020). Spatial and temporal variability of soil N2O and CH4 fluxes along a degradation gradient in a palm swamp peat forest in the peruvian Amazon. Global Change Biol. 26, 7198–7216. doi: 10.1111/gcb.15354
Hutchinson, G. L., and Livingston, G. P. (1993). Use of Chamber Systems to Measure Trace Gas Fluxes. Madison: Amer Soc Agronomy.
Jackson, M. B., and Armstrong, W. (1999). Formation of aerenchyma and the processes of plant ventilation in relation to soil flooding and submergence. Plant Biol. 1, 274–287. doi: 10.1111/j.1438-8677.1999.tb00253.x
Kelly, T. J., Baird, A. J., Roucoux, K. H., Baker, T. R., Coronado, E. N. H., Rios, M., et al. (2014). The high hydraulic conductivity of three wooded tropical peat swamps in northeast peru: measurements and implications for hydrological function. Hydrol. Proc. 28, 3373–3387. doi: 10.1002/hyp.9884
Lähteenoja, O., Ruokolainen, K., Schulman, L., and Alvarez, J. (2009a). Amazonian floodplains harbour minerotrophic and ombrotrophic peatlands. Catena 79, 140–145. doi: 10.1016/j.catena.2009.06.006
Lähteenoja, O., Ruokolainen, K., Schulman, L., and Oinonen, M. (2009b). Amazonian peatlands: an ignored C sink and potential source. Global Change Biol. 15, 2311–2320. doi: 10.1111/j.1365-2486.2009.01920.x
Lai, D. Y. F. (2009). Methane dynamics in northern peatlands: a review. Pedosphere 19, 409–421. doi: 10.1016/s1002-0160(09)00003-4
Lavigne, M. B., and Ryan, M. G. (1997). Growth and maintenance respiration rates of aspen, black spruce and jack pine stems at northern and southern BOREAS sites. Tree Physiol. 17, 543–551. doi: 10.1093/treephys/17.8-9.543
Le Mer, J., and Roger, P. (2001). Production, oxidation, emission and consumption of methane by soils: a review. Eur. J. Soil Biol. 37, 25–50. doi: 10.1016/s1164-5563(01)01067-6
Leifeld, J., and Menichetti, L. (2018). The underappreciated potential of peatlands in global climate change mitigation strategies. Nat. Commun. 9:1071. doi: 10.1038/s41467-018-03406-6
Machacova, K., Back, J., Vanhatalo, A., Halmeenmaki, E., Kolari, P., Mammarella, I., et al. (2016). Pinus sylvestris as a missing source of nitrous oxide and methane in boreal forest. Sci. Rep. 6:23410. doi: 10.1038/srep23410
Machacova, K., Papen, H., Kreuzwieser, J., and Rennenberg, H. (2013). Inundation strongly stimulates nitrous oxide emissions from stems of the upland tree Fagus sylvatica and the riparian tree Alnus glutinosa. Plant Soil 364, 287–301. doi: 10.1007/s11104-012-1359-4
Maier, M., Machacova, K., Lang, F., Svobodova, K., and Urban, O. (2018). Combining soil and tree-stem flux measurements and soil gas profiles to understand CH4 pathways in fagus sylvatica forests. J. Plant Nutr. Soil Sci. 181, 31–35. doi: 10.1002/jpln.201600405
Megonigal, J. P., and Day, F. P. (1992). Effects of flooding on root and shoot production of bald cypress in large experimental enclosures. Ecology 73, 1182–1193. doi: 10.2307/1940668
Myhre, G., Shindell, D., BreìOn, F.-M., Collins, W., Fuglestvedt, J., Huang, J., et al. (2013). “Anthropogenic and natural radiative forcing,” in Climate Change 2013: The Physical Science Basis. Contribution of Working Group I to the Fifth Assessment Report of the Intergovernmental Panel on Climate Change, eds T. F. Stocker, D. Qin, G.-K. Plattner, M. Tignor, S. K. Allen, and J. Boschung (Cambridge: Cambridge University Press), 659–740. doi: 10.1017/cbo9781107415324.018
Page, S. E., Rieley, J. O., and Banks, C. J. (2011). Global and regional importance of the tropical peatland carbon pool. Global Change Biol. 17, 798–818. doi: 10.1111/j.1365-2486.2010.02279.x
Pangala, S. R., Enrich-Prast, A., Basso, L. S., Peixoto, R. B., Bastviken, D., Hornibrook, E. R. C., et al. (2017). Large emissions from floodplain trees close the Amazon methane budget. Nature 552, 230–234. doi: 10.1038/nature24639
Pangala, S. R., Gowing, D. J., Hornibrook, E. R. C., and Gauci, V. (2014). Controls on methane emissions from Alnus glutinosa saplings. New Phytol. 201, 887–896. doi: 10.1111/nph.12561
Pangala, S. R., Moore, S., Hornibrook, E. R. C., and Gauci, V. (2013). Trees are major conduits for methane egress from tropical forested wetlands. New Phytol. 197, 524–531. doi: 10.1111/nph.12031
Pärn, J., Verhoeven, J. T. A., Butterbach-Bahl, K., Dise, N. B., Ullah, S., Aasa, A., et al. (2018). Nitrogen-rich organic soils under warm well-drained conditions are global nitrous oxide emission hotspots. Nat. Commun. 9:1135.
Peel, M. C., Finlayson, B. L., and Mcmahon, T. A. (2007). Updated world map of the köppen-geiger climate classification. Hydrol. Earth Syst. Sci. 11, 1633–1644. doi: 10.5194/hess-11-1633-2007
Petrescu, A. M. R., Lohila, A., Tuovinen, J. P., Baldocchi, D. D., Desai, A. R., Roulet, N. T., et al. (2015). The uncertain climate footprint of wetlands under human pressure. Proc. Natl. Acad. Sci. U.S.A. 112, 4594–4599. doi: 10.1073/pnas.1416267112
Rice, A. L., Butenhoff, C. L., Shearer, M. J., Teama, D., Rosenstiel, T. N., and Khalil, M. A. K. (2010). Emissions of anaerobically produced methane by trees. Geophys. Res. Lett. 37:L03807.
Roucoux, K. H., Lawson, I. T., Jones, T. D., Baker, T. R., Coronado, E. N. H., Gosling, W. D., et al. (2013). Vegetation development in an Amazonian peatland. Palaeogeogr. Palaeoclim. Palaeoecol. 374, 242–255. doi: 10.1016/j.palaeo.2013.01.023
Schindler, T., Mander, Ü, Machacova, K., Espenberg, M., Krasnov, D., Escuer-Gatius, J., et al. (2020). Short-term flooding increases CH4 and N2O emissions from trees in a riparian forest soil-stem continuum. Sci. Rep. 10:3204. doi: 10.1038/s41598-020-60058-7
Scholander, P. F., Van Dam, L., and Scholander, S. I. (1955). Gas exchange in the roots of mangroves. Am. J. Bot. 42, 92–98. doi: 10.2307/2438597
Shimamura, S., Yamamoto, R., Nakamura, T., Shimada, S., and Komatsu, S. (2010). Stem hypertrophic lenticels and secondary aerenchyma enable oxygen transport to roots of soybean in flooded soil. Ann. Bot. 106, 277–284. doi: 10.1093/aob/mcq123
Sjögersten, S., Siegenthaler, A., Lopez, O. R., Aplin, P., Turner, B., and Gauci, V. (2020). Methane emissions from tree stems in neotropical peatlands. New Phytol. 225, 769–781. doi: 10.1111/nph.16178
Soosaar, K., Mander, U., Maddison, M., Kanal, A., Kull, A., Lohmus, K., et al. (2011). Dynamics of gaseous nitrogen and carbon fluxes in riparian alder forests. Ecol. Eng. 37, 40–53. doi: 10.1016/j.ecoleng.2010.07.025
Steffens, B., and Rasmussen, A. (2016). The physiology of adventitious roots. Plant Physiol. 170, 603–617. doi: 10.1104/pp.15.01360
Syakila, A., and Kroeze, C. (2011). The global nitrous oxide budget revisited. Greenhouse Gas Measure. Manage. 1, 17–26. doi: 10.3763/ghgmm.2010.0007
Teh, Y. A., Murphy, W. A., Berrio, J. C., Boom, A., and Page, S. E. (2017). Seasonal variability in methane and nitrous oxide fluxes from tropical peatlands in the western Amazon basin. Biogeosciences 14, 3669–3683. doi: 10.5194/bg-14-3669-2017
Teskey, R. O., Saveyn, A., Steppe, K., and Mcguire, M. A. (2008). Origin, fate and significance of CO2 in tree stems. New Phytol. 177, 17–32. doi: 10.1111/j.1469-8137.2007.02286.x
van Haren, J., Brewer, P. E., Kurtzberg, L., Wehr, R. N., Springer, V. L., Espinoza, R. T., et al. (2021). A versatile gas flux chamber reveals high tree stem CH4 emissions in Amazonian peatland. Agric. Forest Meteorol. 307:108504. doi: 10.1016/j.agrformet.2021.108504
van Lent, J., Hergoualc’h, K., Verchot, L., Oenema, O., and Van Groenigen, J. W. (2019). Greenhouse gas emissions along a peat swamp forest degradation gradient in the peruvian amazon: soil moisture and palm roots effects. Mitigat. Adapt. Strat. Global Change 24, 625–643. doi: 10.1007/s11027-018-9796-x
Wang, Z.-P., Han, S.-J., Li, H.-L., Deng, F.-D., Zheng, Y.-H., Liu, H.-F., et al. (2017). Methane production explained largely by water content in the heartwood of living trees in upland forests. J. Geophys. Res. Biogeosci. 122, 2479–2489. doi: 10.1002/2017jg003991
Welch, B., Gauci, V., and Sayer, E. J. (2019). Tree stem bases are sources of CH4 and N2O in a tropical forest on upland soil during the dry to wet season transition. Global Change Biol. 25, 361–372. doi: 10.1111/gcb.14498
Winton, R. S., Flanagan, N., and Richardson, C. J. (2017). Neotropical peatland methane emissions along a vegetation and biogeochemical gradient. PLoS One 12:e0187019. doi: 10.1371/journal.pone.0187019
Yang, J., He, Y., Aubrey, D. P., Zhuang, Q., and Teskey, R. O. (2016). Global patterns and predictors of stem CO2 efflux in forest ecosystems. Global Change Biol. 22, 1433–1444. doi: 10.1111/gcb.13188
Yoon, J.-H., and Zeng, N. (2010). An Atlantic influence on Amazon rainfall. Clim. Dyn. 34, 249–264. doi: 10.1007/s00382-009-0551-6
Yu, Z. C., Loisel, J., Brosseau, D. P., Beilman, D. W., and Hunt, S. J. (2010). Global peatland dynamics since the last glacial maximum. Geophys. Res. Lett. 37:5.
Keywords: aguaje palm, Amazon rainforest, greenhouse gasses, stem profile, tropical peatlands
Citation: Soosaar K, Schindler T, Machacova K, Pärn J, Fachín-Malaverri LM, Rengifo-Marin JE, Alegría-Muñoz W, Jibaja-Aspajo JL, Negron-Juarez R, Zárate-Gómez R, Garay-Dinis DJ, Arista-Oversluijs AG, Tello-Espinoza R, Pacheco-Gómez T and Mander Ü (2022) High Methane Emission From Palm Stems and Nitrous Oxide Emission From the Soil in a Peruvian Amazon Peat Swamp Forest. Front. For. Glob. Change 5:849186. doi: 10.3389/ffgc.2022.849186
Received: 05 January 2022; Accepted: 21 February 2022;
Published: 31 March 2022.
Edited by:
Junbin Zhao, Norwegian Institute of Bioeconomy Research (NIBIO), NorwayReviewed by:
Auldry Chaddy, Sarawak Tropical Peat Research Institute, MalaysiaSetiari Marwanto, Indonesian Agency of Agricultural Research and Development (IAARD), Indonesia
Copyright © 2022 Soosaar, Schindler, Machacova, Pärn, Fachín-Malaverri, Rengifo-Marin, Alegría-Muñoz, Jibaja-Aspajo, Negron-Juarez, Zárate-Gómez, Garay-Dinis, Arista-Oversluijs, Tello-Espinoza, Pacheco-Gómez and Mander. This is an open-access article distributed under the terms of the Creative Commons Attribution License (CC BY). The use, distribution or reproduction in other forums is permitted, provided the original author(s) and the copyright owner(s) are credited and that the original publication in this journal is cited, in accordance with accepted academic practice. No use, distribution or reproduction is permitted which does not comply with these terms.
*Correspondence: Kaido Soosaar, kaido.soosaar@ut.ee