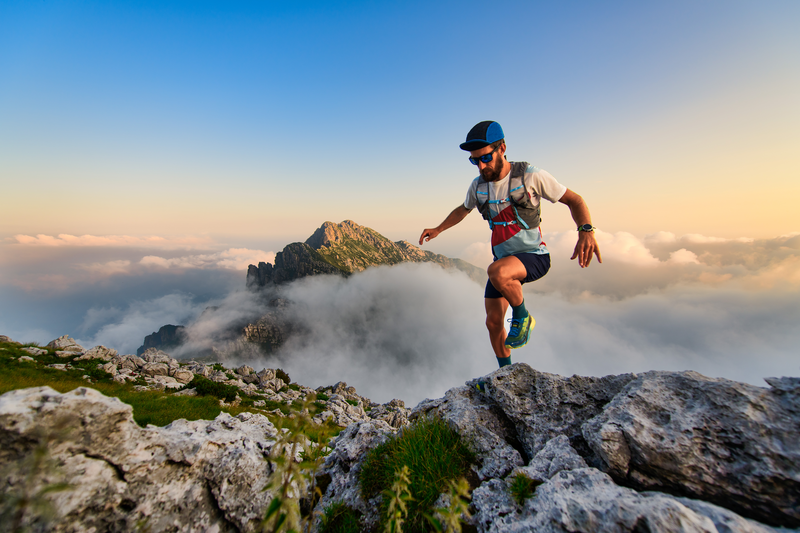
94% of researchers rate our articles as excellent or good
Learn more about the work of our research integrity team to safeguard the quality of each article we publish.
Find out more
ORIGINAL RESEARCH article
Front. For. Glob. Change , 11 May 2022
Sec. Forest Ecophysiology
Volume 5 - 2022 | https://doi.org/10.3389/ffgc.2022.828851
This article is part of the Research Topic Living Far From the Ground: Strategies of Forest Epiphytes View all 10 articles
Background and Aims: Epiphytes are an important component of tropical forests, also they are sensitive to disturbance and deforestation caused by humans, since they depend on their host trees and the micro environmental conditions that these provide. The aim of this study was to analyze the differences in species richness, composition, and vertical distribution of epiphytic angiosperms between areas with natural and disturbed forest at the Northern Coast of Jalisco state, Mexico.
Methods: The presence/absence of epiphytic angiosperms was evaluated in each vertical zone of a selected tree, as well as those present in the understory, both in natural and disturbed sites in three types of vegetation (gallery forest, oak forest, tropical semideciduous forest) with a total of 30 plots of 20 m × 20 m in six sites. Alpha diversity was calculated for each site, as well as species turnover (beta diversity) between habitats. An analysis of variance was performed to determine if there was a significant difference in species richness between sites and, also to compare the height and diameter at breast height (DBH) of the host trees. Multivariate analyzes were used to group the sites according to their floristic composition. Furthermore, a linear regression was performed to detect any relationship between the number of species and the phorophyte structure.
Results: We recorded 45 species, 29 genera and nine families of epiphytic angiosperms. The most diverse families were Bromeliaceae and Orchidaceae and the richest genus was Tillandsia. Although the disturbed sites had more species, a significant difference in richness was not found, except for the disturbed gallery forest. Epiphytic angiosperms presented a high beta diversity, since the sites shared only between 2 and 18% of the recorded species. The inner portion of the canopy (Z3 and Z4) hosted most of the species in all sites and the understory had a high representation of epiphytes except for the disturbed oak forest, where these were absent. A relationship between the DBH and the number of species was found only at the disturbed sites, however, it was highly influenced by the high number of taxa registered in disturbed gallery forest. Therefore, the size of the trees could not be considered a factor in determining the diversity of epiphyte species.
Conclusion: The diversity of epiphytic angiosperm species from the North Coast of Jalisco has not been severely affected by the human disturbance. Most of the species have morphological and physiological adaptations that allow their establishment and survival in adverse climatic conditions. Our results suggest that epiphytic angiosperms cannot be considered as a good indicator for natural or disturbed environments in this region but should be considered in environmental conservation, as they present a high beta diversity.
Currently, there is a great pressure on biodiversity due to anthropic impact, even though factors such as climate change and wildlife trafficking have also negative effects on biological communities, land-use change is considered the principal cause of biodiversity loss and ecosystem degradation (Sala et al., 2000; Wilson et al., 2016). Tropical forests are often the hardest hit, which is particularly serious, because they are containing more than half of the world’s flora, while covering less than 7% of the Earth’s surface (Sodhi et al., 2008; Newbold et al., 2015). Some species are sensitive to environmental changes and can be used as bioindicators, since aspects such as their distribution, abundance, dispersal or reproductive success might indicate the environmental conditions of interest (Holt and Miller, 2011). The level of disturbance and succession in which the forest habitat is found, will determine the plant community’s permanence, as well as the composition and richness (Cuevas-Reyes and Vega-Gutiérrez, 2012).
Epiphytes are principally tropical plants that grow on other plants without being parasitic. This group includes both non-vascular (lichens and bryophytes) and vascular plants, the majority belong to ferns and monocots (e.g., orchids, bromeliads, and aroids) (Benzing, 1990; Zotz, 2016). There exist around 31 311 vascular epiphyte species in the world (ca. 10% of the global flora), of which 28 227 are angiosperms (Zotz et al., 2021). Mexico has a record of 1 813 vascular epiphytes and 84.4% (1 531) are angiosperms (Espejo-Serna et al., 2021). In Jalisco occur around 301 vascular epiphyte species (273 angiosperms) and the state is positioned on sixth place after Chiapas, Oaxaca, Veracruz, Guerrero, and Puebla. These plants are mostly distributed in humid montane forests, which encompass between 30 and 66.6% of their whole richness (Hietz, 2010; Espejo-Serna, 2014); however, they are also an important component in other tropical forests, where they play a crucial role in the community dynamics and constitute habitat and food for many vertebrates and invertebrates (Cruz-Angón et al., 2009; Gotsch et al., 2016; Sabagh et al., 2017). In addition to their floristic and ecological contribution, many epiphyte groups have economic importance, since several species are used as ornamental plants, others have medicinal value and some, especially the bromeliads, are an important element on religious ceremonies (Beutelspacher and Farrera, 2007; Flores-Palacios and Valencia-Díaz, 2007; Haeckel, 2008; Mondragón, 2015; Krömer et al., 2018; Jiménez-López et al., 2019).
Epiphytes are sensible to anthropic disturbance and deforestation, as they depend on their host trees and microenvironmental conditions that these provide (Köster et al., 2009; Larrea and Werner, 2010; Werner et al., 2011; Krömer et al., 2014). Water availability is the most important environmental factor, since it can cause local geographical shifts or species disappearance in certain areas (Benzing, 1998; Zotz and Hietz, 2001), because these plants do not have direct access to water and nutrients from the ground (Gentry and Dodson, 1987; Zotz, 2016). This sensibility has caused a richness decrease in disturbed and secondary forests compared to natural forests in different regions in Mexico (Wolf, 2005; Krömer et al., 2014; Pérez-Peña and Krömer, 2017), as well as in other neotropical countries (Barthlott et al., 2001; Köster et al., 2009, 2013; Werner and Gradstein, 2009). Another factor that influences the distribution, richness, and survival of epiphytes are the characteristics of the phorophytes they are associated with. Their size, diameter, bark texture, and chemical characteristics, as well as water and nutrient availability can positively or negatively affect epiphyte establishment and growth (Mehltreter et al., 2005; Martínez-Meléndez et al., 2008; Jiménez-López et al., 2017). Due to the high vulnerability that epiphytes present to changes in environmental conditions, these plants have been considered as excellent indicators of environmental quality (Turner et al., 1994; Krömer et al., 2014). Epiphytes that depend on mature host trees and have high humidity requirements, will be the most affected by disturbance and microclimatic changes, while epiphytes that are tolerant to high light exposure and drought stress will benefit (e.g., some xeromorphic species of the genus Tillandsia) (Reyes-García et al., 2007; Menini-Neto et al., 2009; Ochoa-López, 2009; Krömer et al., 2021). Therefore, the number of species, the floristic composition, and/or the abundance of epiphytes, can be good indicators of the ecosystem condition, succession status or habitat conservation.
Tropical dry forests can be structurally less complex than humid tropical forests; however, they have a high species turnover (beta diversity) between communities, which is expressed in a high level of endemism (Ceballos et al., 2010). There are few studies on epiphytes from tropical dry forests in Mexico (Aguirre et al., 2010; Cuevas-Reyes and Vega-Gutiérrez, 2012; Trejo-Cruz et al., 2021), which mostly report that disturbance affects species richness according to different land-use types. In a dry forest of Ecuador, less richness in disturbed areas has also been found, but density (number of species per tree) did not vary significantly between the different studied land-use types (Werner and Gradstein, 2009).
The North Coast region of Jalisco has a great plant species diversity and a high level of endemism and includes a part of the most conserved and extensive tropical deciduous and semideciduous forests of Mexico (Arriaga et al., 2000; Vázquez-García et al., 2000). However, these are threatened by land-use changes, like the removal of vegetation for agricultural use, the heavy grazing or periodic fires that prevent the growth of new trees or stop the process of succession, as well as the logging of timber species and the excessive growth of the tourism sector (Ceballos et al., 2010; Morales-Hernández et al., 2016).
According to the available information about human impact on epiphyte diversity, our hypothesis states that, if epiphytic angiosperm richness and its floristic composition are negatively affected by land-use change, then their diversity will decrease in disturbed sites, and the composition will be different between habitats. Therefore, the objective of this study is to observe the differences of the epiphytic angiosperm richness and its floristic composition between areas with natural and disturbed forest at the North Coast of Jalisco state, Mexico.
The study was conducted in the municipality of Cabo Corrientes, located in the western part of Jalisco state, Mexico, between 20° 10′ 55″ and 20° 31′ 00″ latitude N and 105° 10′ 00″ and 105° 41′ 25″ longitude W (Figure 1). Cabo Corrientes belongs to the North Coast region together with the municipalities of Puerto Vallarta and Tomatlán [Comisión Estatal del Agua Jalisco [CEA], 2021] and forms part of the biogeographic provinces Pacific Coast and Sierra Madre del Sur (Morrone et al., 2017). Approximately 53% of the municipality has mountainous terrain, and its elevation ranges from sea level to 1 920 m a.s.l. [Instituto de Información Estadística y Geográfica [IIEG], 2018]. The predominant vegetation type is tropical deciduous forest (TDF), located on the southern coast, followed by tropical semideciduous forest (TSF), which extends its distribution along the north coast (Órgano informativo oficial del municipio de Cabo Corrientes, Jalisco, 2012). On higher elevations there are oak forest (OF) and pine-oak forest (POF) that can sometimes also be found at 300 m a.s.l., associated with tropical species. Besides, there is presence of gallery forest (GF) along rivers, while cloud forest (CF) is restricted to steep slopes in the areas with presence of POF (Rzedowski and McVaugh, 1966). The predominant climate is warm sub-humid with a dry season in winter and a rainy season in summer, and semi-warm semi-humid on the East side (higher elevations). The mean annual temperature is 24.6°C with a maximum of 36°C and minimum of 13°C and the mean annual precipitation is 1 624 mm [Instituto de Información Estadística y Geográfica [IIEG], 2018].
Figure 1. Study area and location of the six sampling sites (NGF = natural gallery forest, DGF = disturbed gallery forest, NOF = natural oak forest, DOF = disturbed oak forest, NTSF = natural tropical sub-deciduous forest, DTSF = disturbed tropical sub-deciduous forest) in Cabo Corrientes, Jalisco, Mexico. (Google Earth Pro, 2020).
Field work was conducted in three types of forest vegetation (GF, OF, TSF), according to the classification of Rzedowski (1978), because the epiphytic angiosperms in this region are better represented in these communities. For each vegetation type two kinds of habitat were chosen, following the criteria proposed by Gómez-Díaz et al. (2017) based on Newbold et al. (2015): (a) natural forest are dominated by mature trees and show no or little signs of logging and other human impacts, with little presence of shrubs and a low canopy opening; and (b) disturbed forest show an evident degree of alteration (selective logging, grazing), a lower presence of mature trees, and a higher percentage of shrubs and grasses. The sampling of epiphytes was based on the method proposed by Krömer and Gradstein (2016), which consists in selecting mature trees with a diameter at breast height (DBH) greater than 10 cm and with a high load of epiphytes. Around the selected tree, a non-permanent 20 m × 20 m plot was established, where also the small trees, shrubs and shoots that constitute the understory were sampled (Figure 2). Five plots were surveyed in each vegetation type (GF, OF, TSF) and habitat (N: natural and D: disturbed) with a total of 30 plots in six study sites (Figure 2) corresponding to an area of 12,000 m2.
Figure 2. Diagram of the plot and view of the sampling sites. (A) NGF = natural gallery forest, (B) DGF = disturbed gallery forest, (C) NOF = natural oak forest, (D) DOF = disturbed oak forest, (E) NTSF = natural tropical sub-deciduous forest, (F) DTSF = disturbed tropical sub-deciduous forest.
We considered true epiphytes or holoepiphytes (that spend their entire life cycle in the canopy without having contact to the ground), primary hemiepiphytes (that begin their cycle in the canopy and then establish root contact with the ground), and secondary hemiepiphytes (that start their life cycle rooted to the ground and then may lose contact while climbing a phorophyte) (Kress, 1986; Benzing, 2012). However, the use of the last term has been questioned by some authors, since there is no evidence that these plants really lose their connection to the ground to pass to an epiphytic state, and thus the use of “nomadic vine” is suggested for all climbing plants that germinate in the ground (Zotz, 2013; Bautista-Bello et al., 2021).
For each selected tree, we recorded the presence/absence of the epiphytic angiosperms located within five vertical zones (Z1: basal part of trunk, 0–2 m high, Z2: upper part of the trunk to the first ramification, Z3: basal part of the large branches, up to the second ramifications, about a third of total branch length, Z4: second third of branch length, Z5: outer third of branch length) according to Johansson (1974). We also noted the presence/absence of all epiphytes in the understory within each 20 m × 20 m subplot. To document the habitat of the epiphytes, the following host tree data were recorded: taxonomic identification (when fertile material was available), height (estimation by observation), and DBH (Krömer and Gradstein, 2016). For a more complete sampling of the epiphytes, the single rope technique was used in several humid montane forest studies (Flores-Palacios and García-Franco, 2001; Krömer et al., 2007a). However, it was not necessary to use this method in our study, due to the simple structure of the phorophyte species (not very dense canopy with trees over 20 m high or trees of low height), which allowed an adequate observation from the ground using binoculars and digital camera.
The species that could not be identified at the sampling site, were collected and herborized; in some cases when epiphytes did not present reproductive structures, live plants were kept in cultivation until flowering, in order to guarantee its correct identification. The collected samples were prepared according to Aguirre-León (1986) and deposited in the Herbario Metropolitano (UAMIZ) of the Universidad Autónoma Metropolitana-Iztapalapa in Mexico City, Mexico. For the circumscription of the epiphyte families, we followed the classification of APG IV (The Angiosperm Phylogeny Group, 2016) and at the species level that of The Plant List (2013) and Tropicos (2020). In the case of some families such as Bromeliaceae, Cactaceae, and Orchidaceae, the studies of some taxonomic specialists were considered (Schuiteman and Chase, 2015; Cruz et al., 2016; Espejo-Serna and López-Ferrari, 2018).
To verify the sampling representativeness, species accumulation curves for the different sampled vegetation types and habitats were made, applying the estimator of potential species richness based on Chao2 presence-absence data using the EstimateS statistical package (Colwell, 2019). Species richness was determined for each vegetation type, habitat, and plot (alpha diversity), as well as the species turnover between vegetation and habitats (beta diversity), using the Sørensen dissimilarity index (Hao et al., 2019). An analysis of variance (ANOVA) was performed to determine if there was a significant difference in species richness between the six sites (NGF, DGF, NOF, DOF, NTSF, DTSF), and to compare the height and the DBH of the hosts. The normality was analyzed with the Skewness and Kurtosis (Omnibus) test and the homogeneity with the F-test. In case of a significant difference, the Tukey-Kramer test was used to compare each site. The significance level used is 0.05, therefore, the null hypothesis (H0) is not rejected if the probability value is equal to or greater than 0.05 (there is no significant difference), and it is rejected if it is less than 0.05 (at least one is significantly different). To determine if there was a relationship between the number of species and the phorophyte structure (height and DBH) a simple linear regression was performed for both natural and disturbed sites. A cluster analysis was performed using the hierarchical, agglomerative method in order to group the sampled sites based on the floristic composition. Regarding the vertical distribution and understory structure, the non-metric multidimensional scaling (NMDS) analysis was applied between each vertical phorophyte zone (Z1-5), and the understory of every sampling site based on Sørensen dissimilarity index. All analyzes were carried out in the statistical program NCSS (2018).
We found 45 species, 29 genera and nine families of epiphytic angiosperms (Supplementary Appendix 1). The most diverse families were Bromeliaceae and Orchidaceae with 17 and 15 species, respectively. The richest genus was Tillandsia with 13 species. The recorded species include 37 true epiphytes, four primary hemiepiphytes, and four secondary hemiepiphytes. None of the species found are registered in the Official Mexican Standard (NOM-059-SEMARNAT-2010); however, 15 species are endemic to Mexico (8 of them exclusive to the Pacific slope), two endemic to western Mexico and two endemic to Jalisco (Supplementary Appendix 1). According to the Chao2 estimator, we observed mostly more than 90% of the estimated species. The lowest numbers of observed species were recorded in NGF with 15 (83%) and in DTSF with 13 (68%) (Figure 3).
Figure 3. Accumulation curves of epiphytic angiosperm species for the six sampling sites (NGF = natural gallery forest, DGF = disturbed gallery forest, NOF = natural oak forest, DOF = disturbed oak forest, NTSF = natural tropical sub-deciduous forest, DTSF = disturbed tropical sub-deciduous forest).
Besides being the most diverse families, Bromeliaceae and Orchidaceae were represented in all sampling sites. Bromeliaceae stands out for high species numbers in NOF (7; 64%) and DTSF (6; 46%), while Orchidaceae dominates in NGF (6; 40%), DGF (10; 43%), and DOF (7; 58%). Araceae represented the highest richness in NTSF (4; 44%) (Figure 4). The remaining families contributed less than 15% in each site. Guarianthe aurantiaca (Bateman) Dressler and W. E. Higgins and Tillandsia schiedeana Steud. were present in five of the six sampling sites, while 49% of the epiphyte species were found in only one site, being DGF the site with the highest number (7) of exclusive species.
Figure 4. Number of species per family in the entire sampled plot (SP), the phorophytes (Ph) and the understory (U) in each sampling site (NGF = natural gallery forest, DGF = disturbed gallery forest, NOF = natural oak forest, DOF = disturbed oak forest, NTSF = natural tropical sub-deciduous forest, DTSF = disturbed tropical sub-deciduous forest).
The DGF harbored most species (23) and the NTSF the lowest number (9) of epiphytes (Figure 5). Although the ANOVA indicated that the richness was significantly different between the six sites (F = 24.6683; p < 0.001), the Tukey-Kramer test showed that the only different site in relation to the number of species was the DGF (p < 0.001; Figure 6).
Figure 5. Families, genera and species in the entire sampled plot (SP), phorophytes (Ph) and understory (U) per sampling site (NGF = natural gallery forest, DGF = disturbed gallery forest, NOF = natural oak forest, DOF = disturbed oak forest, NTSF = natural tropical sub-deciduous forest, DTSF = disturbed tropical sub-deciduous forest).
Figure 6. Variation in number of epiphytic angiosperm species per sampling site (NGF = natural gallery forest, DGF = disturbed gallery forest, NOF = natural oak forest, DOF = disturbed oak forest, NTSF = natural tropical sub-deciduous forest, DTSF = disturbed tropical sub-deciduous forest). Green: no significant difference; Orange: with a significant difference.
The sampled phorophytes were hosting a total of 39 species, 25 genera, and seven families of epiphytic angiosperms (Figure 5 and Supplementary Appendix 2) and showed an average height of 18 ± 5 m and a DBH of 77.4 ± 41.7 cm. The highest tree was located in NTSF measuring 32 m and the smallest was in NOF with 10 m. The widest tree corresponded to the DGF with a DBH of 181.4 cm and the thinnest belonged to the NOF with 29.6 cm (Supplementary Appendix 2). According to the ANOVA, there are significant differences between the height of the trees from the sampling sites (F = 14.0225; p < 0.001), as well as in the DBH (F = 8.3824; p < 0.001) (Figure 7). The simple linear regression model showed that there is no relation between phorophyte height and epiphytic angiosperm richness for both natural (a = 4.7011, b = −0.0569, r2 = 0.1332, p > 0.05) and disturbed sites (a = 12.7123, b = −0.3677, r2 = 0.2095, p > 0.05) (Figure 8). As for DBH, no correlation was found with the number of species in the natural sites (a = 4.1123, b = −0.0079, r2 = 0.0263, p > 0.05), but it was found in the disturbed sites (a = 1.6553, b = 0.0657, r2 = 0.4426, p < 0.05) (Figure 8).
Figure 7. Variation in the height (A) and diameter breast height (DBH) (B) of the phorophytes in each sampling site (NGF = natural gallery forest, DGF = disturbed gallery forest, NOF = natural oak forest, DOF = disturbed oak forest, NTSF = natural tropical sub-deciduous forest, DTSF = disturbed tropical sub-deciduous forest). Sampling sites that do not have the same letter are significantly different.
Figure 8. Simple linear regression between phorophyte height and species richness of epiphytic angiosperms in natural sites (A) and disturbed sites (B). Simple linear regression between phorophyte DBH and species richness of epiphytic angiosperms in natural sites (C) and disturbed sites (D). NGF = natural gallery forest, DGF = disturbed gallery forest, NOF = natural oak forest, DOF = disturbed oak forest, NTSF = natural tropical sub-deciduous forest, DTSF = disturbed tropical sub-deciduous forest.
The vertical distribution of epiphytes was principally concentrated in the zone Z4 with 28 species, while Z1 had the lowest richness with 12. The zones Z3 and Z4 hosted the greatest richness in almost all sampled sites, while Z1 and Z2 had the lowest (Figure 9). Aechmea bracteata, Hylocereus purpusii (Weing.) Britton and Rose, Philodendron scandens Kunth, and Tillandsia jaliscomonticola Matuda were present in all five zones and 26% of the species were exclusive to one zone (Supplementary Appendix 1). On the other hand, the understory had 32 species, 19 genera, and eight families of epiphytic angiosperms. There was a higher richness in the understory than in the phorophytes in the NOF and in the DTSF, but we did not observe any epiphyte species in the DOF understory (Figure 5 and Supplementary Appendix 2). Bromeliads were present in the understory at all sites, except for NTSF where only species of Araceae were found (Figure 4).
Figure 9. Number of species per family in the vertical zones (ZI, Z2, Z3, Z4, Z5) in each sampling site (NGF = natural gallery forest, DGF = disturbed gallery forest, NOF = natural oak forest, DOF = disturbed oak forest, NTSF = natural tropical sub-deciduous forest, DTSF = disturbed tropical sub-deciduous forest).
The sampling sites shared between 2 and 18% of the recorded species in which the DGF and the NOF were least different with a dissimilarity coefficient of 0.68, while the sites with greatest dissimilarity were NTSF with both NOF and DGF, with values of 0.91 and 0.89, respectively (Supplementary Appendix 3). On the other hand, the cluster analysis divided the sites into two main groups: (1) NOF, DOF, and DGF; (2) NTSF, DTSF, and NGF (Figure 10). According to the NMDS analysis, there is a clear difference in the epiphytic species represented in the zones and in the understory in almost all sites (Figure 11). The highest dissimilarity index was between the zones Z3 and Z4 in NOF, as they share 86% of the species, and the zone Z2 in DOF was the most different from all, as it presented only one species, that was also found in zone Z2 in NTSF. The understory from all sites were more similar to the canopy zones than to the trunk area.
Figure 10. Cluster analysis of epiphytic angiosperms floristic composition between the six sampling sites (NGF = natural gallery forest, DGF = disturbed gallery forest, NOF = natural oak forest, DOF = disturbed oak forest, NTSF = natural tropical sub-deciduous forest, DTSF = disturbed tropical sub-deciduous forest).
Figure 11. Ordination site scores in two dimensions generated by non-metric multidimensional scaling (NMDS) using the Sørensen dissimilarity index of epiphytic angiosperms floristic composition per vertical zone (Z1, Z2, Z3, Z4, Z5) and understory (U) in each sampling site (NGF = natural gallery forest, DGF = disturbed gallery forest, NOF = natural oak forest, DOF = disturbed oak forest, NTSF = natural tropical sub-deciduous forest, DTSF = disturbed tropical sub-deciduous forest).
When comparing the number of species obtained in the present study, with the total of angiosperm epiphytes registered for Jalisco (Espejo-Serna et al., 2021), we recorded 16.5% of these in less than one km2 (12,000 m2). There is also a considerable richness compared to that reported in two previous floristic inventories made for the region: Ramírez-Delgadillo and Cupul-Magaña (1999) recorded 29 taxa of epiphytic angiosperms for the municipalities of Bahía de Banderas, Cabo Corrientes, and Puerto Vallarta. On the other hand, Vázquez-García et al. (2000) registered 28 species for the municipality of Cabo Corrientes in their contribution for the North Coast of Jalisco. We obtained more than 80% of the estimated epiphyte species richness for the study area, which indicates that the sampling effort was sufficient.
The high richness of Bromeliaceae and Orchidaceae registered in the area, was an expected result, since both are the richest families among the angiosperm epiphytes at the national and state level in Mexico (Ceja-Romero et al., 2010; Krömer et al., 2020; Miguel-Vázquez et al., 2020; Espejo-Serna et al., 2021). In some local or regional studies carried out in Mexico this pattern has also been found, both in the case of humid forests (Flores-Palacios and García-Franco, 2008; Martínez-Meléndez et al., 2008; Krömer et al., 2013) and dry forests (Wolf, 2005; Trejo-Cruz et al., 2021), with the Orchidaceae family predominating. Other studies have registered a greater number of Bromeliaceae species, as in the case of a dry forest in Chamela, Jalisco (Cuevas-Reyes and Vega-Gutiérrez, 2012), or that of El Cielo Biosphere Reserve, in Tamaulipas (de la Rosa-Manzano et al., 2017). However, in many studies carried out in other Neotropical countries, it has been found that Araceae, including the nomadic vines, occupies the second place especially in lowland rainforests, while in humid montane forests the ferns have a similar richness to that of orchids (Kreft et al., 2004). The dominance of the Araceae in tropical semideciduous forest and its absence in oak forest, is due to the fact that the representatives of this family generally do not inhabit dry and/or temperate forests (Wolf, 2005; Cuevas-Reyes and Vega-Gutiérrez, 2012), and also because their species number tends to decrease with increasing elevations, since the plants are not well adapted, both structurally and physiologically, to arid or cold conditions (Krömer et al., 2005; Acebey and Krömer, 2008).
The Piperaceae family is generally diverse in humid montane forest and tropical evergreen forest (Barthlott et al., 2001; Krömer et al., 2005, 2007b), because the species of the genus Peperomia have a marked preference for warm or temperate areas, but with high humidity conditions (Vergara-Rodríguez et al., 2017), which may also explain the presence of one of its species in the disturbed gallery forest of the study area. The other registered families (Araliaceae, Asteraceae, Cactaceae, Clusiaceae, and Moraceae), most of them present with only one species, had a low diversity, situation that has been reported in other studies (Olmsted and Gómez-Juárez, 1996; Gomez-Escamilla et al., 2019; Krömer et al., 2020; Miguel-Vázquez et al., 2020). It is also important to consider that the presence of the epiphytic habit among its representatives, is lower than in the case of the four main families mentioned above (Araceae, Bromeliaceae, Orchidaceae, Piperaceae), which concentrate 87% of the epiphytic angiosperms in the world (Zotz et al., 2021).
Tillandsia was the richest genus; many of its species present special adaptations (e.g., atmospheric forms, absorbent foliar trichomes, CAM photosynthesis), which allows them to resist and survive in conditions of dry air and high temperatures (Zotz and Andrade, 2002; Benzing, 2012; Krömer et al., 2014), as well as surpassing other species, both in richness and abundance (Wolf, 2005; Cascante-Marín et al., 2006; Hietz et al., 2006; Cuevas-Reyes and Vega-Gutiérrez, 2012). In addition, faster growth rates have been found in seedlings of some Tillandsia species that grow in more exposed sites than those in shady places (Winkler et al., 2005; Flores-Palacios and García-Franco, 2006; Einzmann and Zotz, 2017). This may explain the low presence of species and individuals of the genus in the tropical semideciduous forest, which is the site with the most closed canopy.
In the three types of forest vegetation sampled in the study area, surprisingly the disturbed sites presented a greater number of epiphyte species. This is in contrast to published data (Barthlott et al., 2001; Wolf, 2005; Krömer et al., 2007b,2014; Köster et al., 2009, 2013; Pérez-Peña and Krömer, 2017), where generally the highest specific richness of epiphytes, both in Mexico and in the Neotropics, has been found in natural forests, while few authors found no differences (Hietz-Seifert et al., 1996; Larrea and Werner, 2010), or even reported the opposite situation. Aguirre et al. (2010) recorded a greater richness of epiphytes in disturbed sites in a forest dominated by the palm Sabal mexicana, on the Gulf coast in Veracruz, mainly due to the accumulation of hemiepiphytic species of low abundance (e.g., Ficus spp.) and the presence of accidental epiphytes, although these are not normally considered in studies of this type. Furthermore, Guzmán-Jacob et al. (2020) observed that, along the altitudinal gradient of the Cofre de Perote in Veracruz, richness of epiphytes in a degraded tropical semideciduous forest at the 500 m elevation, was higher than in adjacent old-growth forest.
It should be noted that, in general terms, there was no significant variation among the six sampling sites, except for the DGF. This could indicate that epiphyte richness did not respond significantly to disturbance in dry forests, due to the physiological and morphological pre-adaptations of the mostly drought tolerant species (Werner et al., 2011; Guzmán-Jacob et al., 2020). Some species such as Philodendron warszewiczii Koch and C. D. Bouché, Aechmea bracteata, and Tillandsia jaliscomonticola, had preference for phorophytes from disturbed and open areas, however, they were not exclusive to disturbed sites. Therefore, the changes of dominance in these species, could be used as indicators to determine the effects of anthropic disturbance (Krömer et al., 2014).
Although all the sampling sites presented exclusive species, only nine taxa were restricted to the natural ones; however, most are common and wide-ranging species such as Tillandsia ionantha and Syngonium neglectum. Therefore, the species that can be considered as indicators of environmental quality in this part of the country could not necessarily be used in other regions. The preference for certain type of microenvironments, regardless of whether the site was natural or disturbed, was more notable in the case of some species: for example, Trichocentrum oestlundianum (L. O. Williams) M. W. Chase and N. H. Williams was collected at both habitats in the gallery forest but showed a preference for more closed and humid sites. Some species were also observed to benefit from disturbance, such as several of the genus Tillandsia, which were more abundant in open spaces, or the hemiepiphyte Philodendron warscewiczii that had a wider vertical distribution and also a much higher frequency in the disturbed site (DTSF) (see Supplementary Appendix 1). The changes in dominance of these species could be used to determine effects of anthropic disturbance (Krömer et al., 2014). The greater richness and frequency of the species recorded in the disturbed sites in the study area, could be explained with the hypothesis of intermediate disturbance (Connell, 1978; Kun et al., 2009), which suggests that the presence of intermediate disturbance levels allow the coexistence of many species, more than would exist in the absence of these alterations or in the case of more drastic environmental changes. If the habitat is not highly fragmented, populations can filter through clusters of habitable sites, favoring the spread of a population.
The size (height and DBH) of the phorophytes was highly variable in the six sampling sites, even among the same forest types. The structure of trees in NGF was very similar to those of DTSF, however, the greater richness recorded in the former, may be mainly due to its proximity to a stream. The phorophytes of the disturbed gallery forest were structurally more complex than those of the other sites, offering a greater diversity of ecological niches due to the variety of sizes and positions of their branches, thus presenting pitchforks and nodes, that facilitate colonization by epiphytes (Arévalo and Betancur, 2006), as well as variable spaces, from shady and humid places near the trunk base to lighted and dry areas on the outer part of the crown (Böhnert et al., 2016), which caused the greatest richness of this site. In the case of the oak forest of the study area, all the host trees were of the genus Quercus. The rough bark of their trunks and branches offers a porous substrate with higher humidity due to its high-water retention capacity; this facilitates the anchoring of seeds (Callaway et al., 2002; Malizia, 2003) and makes them ideal phorophytes for the epiphytes in the dry environments of this type of vegetation. The phorophytes of NTSF had the same number of species as those of DTSF (Figure 5), although the average of all trees per site was lower (2.8) compared to the site with disturbed vegetation (3.2), which is probably explained by the presence in this last site of a single phorophyte with many epiphytes, probably due to its proximity to a stream of water (see Supplementary Appendix 2). The scarce presence of epiphytes in the phorophytes of tropical semideciduous forest is a consequence of their structure, since they have long and straight trunks and their branches in the canopy have little surface area for the establishment of epiphytic plants. In addition, the closed canopy of these plant communities, especially in the natural sampling site, did not allow the passage of sunlight, affecting the development of heliophilous species.
There is evidence of a positive relationship between the richness and/or abundance of epiphytic species and the DBH or height of the phorophytes (Hietz and Hietz-Seifert, 1995; Burns and Dawson, 2005; Flores-Palacios and García-Franco, 2006; Ochoa-López, 2009; Gómez-Díaz, 2010; Cuevas-Reyes and Vega-Gutiérrez, 2012; Dislich and Mantovani, 2016; Jiménez-López et al., 2017). However, in the study area, the disturbed sites were the only ones in which this positive relationship was observed, although probably the high number of taxa registered in DGF influenced this result, since both in DOF and DTSF, few species were recorded on trees with greater DBH. In other documented cases, such as that of a temperate forest in the Sierra Norte de Oaxaca (Bautista et al., 2014), and that of a tropical dry forest in the state of Morelos (Vergara-Torres et al., 2010), this relationship between the DHB of the phorophytes and the richness of epiphytes, was also not found. Additionally, it is important to consider that a mature tree does not necessarily have to be large, but rather its age, that is, the time it has been available for the colonization of epiphytes, could be the factor that most influences the species richness. Köster et al. (2011) observed that relatively small, but mature trees, in secondary vegetation derived from a cloud forest, harbored a high number of epiphytic species. Therefore, the structure and the specific identity of the phorophyte, as well as the type of vegetation in which it is found, are the determining factors, rather than the size of the trees.
The greatest epiphyte richness was found inside the canopy (Z4 y Z3), while the lowest was on the trunk (Z1 y Z2) of the trees. The inner part of the canopy has a suitable microenvironment for a successful establishment of many epiphytes, there is abundant organic matter on thick, vertical branches (ter Steege and Cornelissen, 1989; Freiberg, 1996), as well as favorable climatic conditions, such as lower solar incidence and higher humidity, which favors the survival of epiphytes in tropical dry forests. The zone Z5 of the phorophytes in humid montane forest is considered as harsh environment, because it is more exposed to strong insolation, high temperatures, and the action of winds as well as a low air humidity (Krömer et al., 2007a). However, the observed abundance of bromeliads, most of them xeromorphic or atmospheric Tillandsias in the outer part (Z5) of the canopy may be related to the availability of rainwater and fog and the accumulation of dew, conditions that prevail in this zone of the phorophytes in dry environments (Graham and Andrade, 2004; Reyes-García et al., 2007).
The restriction of orchids to the inner zone of the canopy may be due to the conditions required for their seed’s germination and anchorage, since they lack endosperm and need an adequate mycorrhizal association (Mondragón et al., 2007). Even so, some species of this family, such as Prosthechea chacaoensis, presented a wide vertical distribution, due to adaptations to tolerate water stress, such as the presence of pseudobulbs and succulent leaves, which generally store water, as well as CAM metabolism (Zotz, 2004; Krömer et al., 2007b,2014; Kerbauy et al., 2012).
According to Jácome et al. (2004), the members of the Araceae family in the rainforests of the Pacific coast of Colombia, grow preferentially in the first 10 m from the base of the phorophytes, due to the presence of leaves with a thin cuticle and a broader foliar area, which represents a disadvantage compared to the xeric adaptations present in other epiphytes (Benzing, 1990; Mayo et al., 1997). In the study area, the Araceae showed this same pattern, since the hemiepiphytic species of the genera Philodendron and Syngonium were observed mainly in the zones Z1 and Z2 of the phorophytes, while the vertical distribution of the holoepiphyte Anthurium halmoorei extended to higher levels (Z3 and Z4), which is mainly due to the rosette arrangement of its leaves that favors the accumulation of organic matter and rainwater, similar to tank-bromeliads. Probably the most important biotic factors for the establishment of epiphytes in the different zones of the phorophytes, were the microenvironmental characteristics (branch position and surface area, bark type, canopy shape, etc.) that each of them offered and the type of vegetation in which it grew. For example, Tillandsia jaliscomonticola was present in all five zones in the disturbed gallery forest, while in the disturbed oak forest it was found only in zones Z4 and Z5. It has been observed that denser crowns (with more branches) reduce the wind speed and limit the dispersal of anemochore seeds (Victoriano-Romero et al., 2017); this could explain the different vertical distribution of this species in the two sites.
The epiphyte richness of the understory in all sampling sites represented 71% of the total, and six species grew only in this stratum. The environmental conditions of the understory are, in many cases, conducive to the successful establishment of many epiphytes, since there is less solar incidence, grater humidity, and less influence of the wind (Krömer et al., 2007a). The absence of epiphytes in the disturbed oak forest understory, could be due to the alteration of this site by grazing, which has prevented the development of small trees that function as hosts for the epiphytes in this stratum.
The two groups obtained in the cluster analysis represent, in the case of the first one, the sites with species related to the oak forest, and in the second, those that are mainly made up to tropical semideciduous forest species. The taxa that define the first group belong mostly to the Bromeliaceae and Orchidaceae, while the second includes Araceae and other hemiepiphytes such as Araliaceae, Asteraceae, and Moraceae, these four families being exclusive of this second group. This can explain the high beta diversity in the study area. In addition, dry forests can have a higher beta diversity than humid forests (Ceballos et al., 2010); the topographic and climatic conditions present on the Pacific slope create barriers that confine species in a restricted distribution and, therefore, the species composition will change more rapidly from one locality to another (Espinosa and Ocegueda, 2008); Cuevas-Reyes and Vega-Gutiérrez (2012) also report a high dissimilarity in a dry tropical forest with different degrees of disturbance, sharing between 37 and 42.8% of their species. On the other hand, Flores-Palacios and García-Franco (2008) found a high turnover of species among different habitats of a humid montane forest, due to their isolation and the distance at which they were from a permanent source of water. In another study carried out in a montane forest in the Andes of Venezuela, it was also found that the composition of epiphytes was differing more and more with the degree of disturbance, which causes the plants to be exposed to increased insolation (Barthlott et al., 2001).
As in the cluster analysis, a difference can also be observed between each site in the NMDS analysis; however, some zones were very different compared with the ones from the same site, probably due to the presence of species with low abundance like Billbergia pallidiflora Liebm. or because they share few to no species with the other zones. The high similarity between the understory and the inner canopy zones can be explained because the understory also presents environmental conditions similar to those of the inner canopy (Krömer et al., 2007a).
Despite the great capacity that epiphytes present to resist environmental alterations caused by humans in the dry forests of the study area, threats of land use change due to agriculture or tourism, could generate a long-term negative effect. The introduction of cattle or goats implies the simplification of the vegetation structure and provokes the replacement of native species by introduced ones. On the other hand, the removal of vegetation cover for the development of large tourist complexes could generate greater habitat fragmentation, impeding connectivity between epiphyte populations (Fischer and Lindenmayer, 2007). An alternative that has been proposed and carried out for the conservation of epiphytes in other parts of Mexico is the establishment of Environmental Management Units (UMAs), in order to reproduce and propagate some rare or endemic species, either through in vitro laboratories or in a more rustic way (Damon et al., 2005). However, the sustainable use of populations must be based on a management plan for the maintenance of their diversity (Mondragón and Ticktin, 2011; Toledo-Aceves et al., 2014; Francisco-Ventura et al., 2018). In the case of this region, population studies specially of the species with restricted distribution (see Supplementary Appendix 1) would be required before presenting concrete proposals on their management.
The original contributions presented in the study are included in the article/Supplementary Material, further inquiries can be directed to the corresponding author/s.
AF-A, AE-S, and AL-F conducted the field trips and samplings. AF-A analyzed the data and wrote the manuscript. TK contributed with the study design and data analysis. AE-S, AL-F, and TK revised the manuscript. All authors read and approved the final manuscript.
This study was partially supported by the scholarship 634119 awarded by Consejo Nacional de Ciencia y Tecnología (CONACyT).
The authors declare that the research was conducted in the absence of any commercial or financial relationships that could be construed as a potential conflict of interest.
All claims expressed in this article are solely those of the authors and do not necessarily represent those of their affiliated organizations, or those of the publisher, the editors and the reviewers. Any product that may be evaluated in this article, or claim that may be made by its manufacturer, is not guaranteed or endorsed by the publisher.
We would like to thank to the reviewers for their suggestions that allowed us to improve the manuscript. We would also like to thank to Rodrigo Hernández-Cárdenas, Lizetth Jimena Hernández-Barón, Gerardo Contreras-Félix, and Jorge Novoa-Ramos for their help in the field work. The results of this study are part of the Master Thesis of the first author completed at Universidad Autónoma Metropolitana unidad Itztapalapa.
The Supplementary Material for this article can be found online at: https://www.frontiersin.org/articles/10.3389/ffgc.2022.828851/full#supplementary-material
Acebey, A., and Krömer, T. (2008). Diversidad y distribución de Araceae de la Reserva de la Biosfera Los Tuxtlas, Veracruz, México. Rev. Mex. Biodivers. 79, 465–471. doi: 10.22201/ib.20078706e.2008.002.553
Aguirre, A., Guevara, R., García, M., and López, J. C. (2010). Fate of epiphytes on phorophytes with different architectural characteristics along the perturbation gradient of Sabal mexicana forests in Veracruz, Mexico. J. Veg. Sci. 21, 6–15. doi: 10.1111/j.1654-1103.2009.01131.x
Aguirre-León, E. (1986). “Epífitas,” in Manual de herbario: administración y manejo de colecciones técnicas de recolección y preparación de ejemplares botánicos, eds A. Lot and F. Chiang (México: Consejo Nacional de la flora de México, A.C), 113–119.
Arévalo, R., and Betancur, J. (2006). Vertical distribution of vascular epiphytes in four forest types of the Serranía de Chiribiquete, Colombian Guayana. Selbyana 27, 175–185. doi: 10.2307/41760280
Arriaga, L., Espinoza, J. M., Aguilar, C., Martínez, E., Gómez, L., and Loa, E. (2000). Regiones terrestres prioritarias de México. Escala de trabajo 1:1 000 000. México: Comisión Nacional para el Conocimiento y uso de la Biodiversidad.
Barthlott, W., Schmit-Neuerburg, V., Nieder, J., and Engwald, S. (2001). Diversity and abundance of vascular epiphytes: a comparison of secondary vegetation and primary montane rain forest in the Venezuelan Andes. Plant Ecol. 152, 145–156. doi: 10.1023/A:1011483901452
Bautista, J. L., Damon, A., Ochoa-Gaona, S., and Clark-Tapia, R. (2014). Impact of silvicultural methods on vascular epiphytes (ferns, bromeliads and orchids) in a temperate forest in Oaxaca, Mexico. For. Ecol. Manag. 329, 10–20. doi: 10.1016/j.foreco.2014.05.053
Bautista-Bello, A. P., Krömer, T., Acebey, A. R., Weichgrebe, L., and Zotz, G. (2021). Variación biológica en las aráceas trepadoras. Acta Bot. Mex. 128, 1–18. doi: 10.21829/abm128.2021.1819
Benzing, D. H. (1990). Vascular epiphytes, general biology and related biota. New York: Cambridge University Press.
Benzing, D. H. (1998). Vulnerabilities of tropical forests to climate change: the significance of resident epiphytes. Clim. Change 39, 519–540. doi: 10.1023/A:1005312307709
Beutelspacher, C. R., and Farrera, S. O. (2007). Tradición vs. conservación: la “topada” de la Flor. Lacandonia Rev. de Ciencias UNICACH 1, 109–115.
Böhnert, T., Wenzel, A., Altenhövel, C., Beeretz, L., Tjitrosoedirdjo, S. S., Meijide, A., et al. (2016). Effects of land-use change on vascular epiphyte diversity in Sumatra (Indonesia). Biol. Conserv. 202, 20–29. doi: 10.1016/j.biocon.2016.08.008
Burns, K. C., and Dawson, J. (2005). Patterns in the diversity and distribution of epiphytes and vines in a New Zeland forest. Austral Ecol. 30, 883–891. doi: 10.1111/j.1442-9993.2005.01532.x
Callaway, R. M., Reinhart, K. O., Moore, G. W., Moore, D. J., and Pennings, S. C. (2002). Epiphyte host preferences and host traits: mechanisms for species-specific interactions. Oecologia 132, 221–230. doi: 10.1007/s00442-002-0943-3
Cascante-Marín, A., Wolf, J. H., Oostermeijer, J. G. B., den Nijs, J. C. M., Sanahuja, O., and Durán-Apuy, A. (2006). Epiphytic bromeliad communities in secondary and mature forest in a tropical premontane area. Basic Appl. Ecol. 7, 520–532. doi: 10.1016/j.baae.2005.10.005
Ceballos, G., Martínez, L., García, A., Espinoza, E., Bezaury, C. J., and Dirzo, R. (2010). Diversidad, amenazas y áreas prioritarias para la conservación de las selvas secas del Pacífico de México. Ciudad de México, México: Fondo de Cultura Económica y Comisión Nacional para el Conocimiento y Uso de la Biodiversidad.
Ceja-Romero, J., Mendoza-Ruiz, A., Loìpez-Ferrari, A. R., Espejo-Serna, A., Peìrez-Garciìa, B., and Garciìa- Cruz, J. (2010). Las epiìfitas vasculares del estado de Hidalgo, Meìxico: diversidad y distribucioìn. Acta Bot. Mex. 93, 1–39. doi: 10.21829/abm93.2010.274
Colwell, R. (2019). EstimateS: Statistical estimation of species richness and shared species from samples. Versión 9.1.0. Guía de usuario y aplicación publicado. Available Online at: http://viceroy.eeb.uconn.edu/estimates/ (accessed Oct. 3, 2019).
Comisión Estatal del Agua Jalisco [CEA] (2021). Región 09 Costa-Norte. Mexico: Comisión Estatal del Agua de Jalisco
Connell, J. H. (1978). Diversity in tropical rain forest and coral reefs. Science 199, 1302–1310. doi: 10.1126/science.199.4335.1302
Cruz, M. A., Arias, S., and Terrazas, T. (2016). Molecular phylogeny and taxonomy of the genus Disocactus (Cactaceae), based on the DNA sequences of six chloroplast markers. Willdenowia 46, 145–164. doi: 10.3372/wi.46.46112
Cruz-Angón, A., Baena, M. L., and Greenberg, R. (2009). The contribution of epiphytes to the abundance and species richness of canopy insects in a Mexican coffee plantation. J. Trop. Ecol. 25, 453–463. doi: 10.1017/S0266467409990125
Cuevas-Reyes, P., and Vega-Gutiérrez, J. I. (2012). Cambios en la estructura, composición y fenología de plantas epífitas bajo diferentes estadios de sucesión vegetal en un bosque tropical seco. Biológicas 14, 37–44.
Damon, A., Pérez-Soriano, M., and de Lourdes, R. M. (2005). Substrates and fertilization for the rustic cultivation of in vitro propagated native orchids in Soconusco, Chiapas. Renew. Agric. Food Syst. 20, 214–222. doi: 10.1079/RAF2005106
de la Rosa-Manzano, E., Guerra-Pérez, A., Mendieta-Leiva, G., Mora-Olivo, A., Martínez-Ávalos, J. G., and Arellano-Méndez, L. U. (2017). Vascular epiphyte diversity in two forest types of the “El Cielo” Biosphere Reserve, Mexico. Botany 95, 599–610. doi: 10.1139/cjb-2016-0184
Dislich, R., and Mantovani, W. (2016). Vascular epiphyte assemblages in a Brazilian Atlantic Forest fragment: investigating the effect of host tree features. Plant Ecol. 217, 1–12. doi: 10.1007/s11258-015-0553-x
Einzmann, H. J. R., and Zotz, G. (2017). Dispersal and establishment of vascular epiphytes in human-modified landscapes. AoB Plants 9, 1–13. doi: 10.1093/aobpla/plx052
Espejo-Serna, A. (2014). “Las plantas vasculares de los bosques mesófilos de montaña en México,” in Bosques Mesófilos de Montaña de México diversidad, ecología y manejo, eds M. Gual-Díaz and A. Rendón-Correa (México: Comisión Nacional para el Conocimiento y Uso de la Biodiversidad), 189–195.
Espejo-Serna, A., and López-Ferrari, A. R. (2018). La familia Bromeliaceae en México. Bot. Sci. 96, 533–554. doi: 10.17129/botsci.1918
Espejo-Serna, A., López-Ferrari, A. R., Mendoza-Ruiz, A., García-Cruz, J., Ceja-Romero, J., and Pérez-García, B. (2021). Mexican vascular epiphytes: richness and distribution. Phytotaxa 503, 1–124. doi: 10.11646/phytotaxa.503.1.1
Espinosa, O. D., and Ocegueda, C. S. (2008). “El conocimiento biogeográfico de las especies y su regionalización natural,” in Capital Natural de México, vol. I, ed. J. Sarukhan (México: Conocimiento Actual de la Biodiversidad. CONABIO), 33–65.
Fischer, J., and Lindenmayer, D. B. (2007). Landscape modification and habitat fragmentation: a synthesis. Glob. Ecol. Biogeogr. 16, 265–280. doi: 10.1111/j.1466-8238.2007.00287
Flores-Palacios, A., and García-Franco, J. G. (2001). Sampling methods for vascular epiphytes: their effectiveness in recording species richness and frequency. Selbyana 22, 181–191. doi: 10.2307/41760095
Flores-Palacios, A., and García-Franco, J. G. (2006). The relationship between tree size and epiphyte species richness: testing four different hypotheses. J. Biogeogr. 33, 323–330. doi: 10.1111/j.1365-2699.2005.01382.x
Flores-Palacios, A., and García-Franco, J. G. (2008). Habitat isolation changes the beta diversity of the vascular epiphyte community in lower montane forest, Veracruz, Mexico. Biodivers. Conserv. 17, 191–207. doi: 10.1007/s10531-007-9239-6
Flores-Palacios, A., and Valencia-Díaz, S. (2007). Local illegal trade of wild vascular epiphytes reveals unknown diversity. Biol. Conserv. 136, 372–387. doi: 10.1016/j.biocon.2006.12.017
Francisco-Ventura, E., Menchaca-García, R. A., Toledo-Aceves, T., and Krömer, T. (2018). Potencial de aprovechamiento de epífitas vasculares caídas en un bosque mesófilo de montaña de Los Tuxtlas, Veracruz, México. Rev. Mex. Biodivers. 89, 1263–1279. doi: 10.22201/ib.20078706e.2018.4.2390
Freiberg, M. (1996). Spatial distribution of vascular epiphytes on three emergent canopy trees in French Guiana. Biotropica 28, 345–355. doi: 10.2307/2389198
Gentry, A. H., and Dodson, C. H. (1987). Diversity and biogeography of Neotropical vascular epiphytes. Ann. Mo. Bot. Gard. 74, 205–233. doi: 10.2307/2399395
Gómez-Díaz, J. A. (2010). Comparación florística de epífitas vasculares entre un bosque mesófilo de montaña y un acahual en el municipio de Tlalnelhuayocan, Veracruz. Ph.D. thesis. Veracruz (XP): Universidad Veracruzana
Gómez-Díaz, J. A., Krömer, T., Carvajal-Hernández, C. I., Gerold, G., and Heitkamp, F. (2017). Richness and distribution of herbaceous angiosperms along gradients of elevation and forest disturbance in central Veracruz, Mexico. Bot. Sci. 95, 1–22. doi: 10.17129/botsci.859
Gomez-Escamilla, I. N., Espejo-Serna, A., López-Ferrari, A. R., and Krömer, T. (2019). Distribución geográfica de angiospermas epífitas de la región terrestre prioritaria Cerros Negro-Yucaño, Oaxaca, México. Rev. Biol. Trop. 67, 118–131. doi: 10.15517/rbt.v67i1.32726
Google Earth Pro (2020). Descargar Google Earth Pro para PC, Mac o Linux Version 7.3. Available online at: https://www.google.com/intl/es/earth/download/gep/agree.html (accessed January 12, 2022).
Gotsch, S. G., Nadkarni, N., and Amici, A. (2016). The functional roles of epiphytes and arboreal soils in tropical montane cloud forests. J. Trop. Ecol. 32, 455–468. doi: 10.1017/S026646741600033X
Graham, E. A., and Andrade, J. L. (2004). Drought tolerance associated with vertical stratification of two co-occurring epiphytic bromeliads in a tropical dry forest. Am. J. Bot. 91, 699–706. doi: 10.3732/ajb.91.5.699
Guzmán-Jacob, V., Zotz, G., Craven, D., Taylor, A., Krömer, T., Monge-González, M. L., et al. (2020). Effects of forest use intensity on vascular epiphyte diversity along an elevational gradient. Divers. Distrib. 26, 4–15. doi: 10.1111/ddi.12992
Haeckel, I. B. (2008). The “Arco Floral”: ethnobotany of Tillandsia and Dasylirion spp. in a Mexican religious adornment. Econ. Bot. 62, 90–95. doi: 10.1007/s12231-008-9009-8
Hao, M., Corral-Rivas, J. J., González-Elizondo, M. S., Ganeshaiah, K. N., Nava-Miranda, M. G., Zhang, C., et al. (2019). Assessing biological dissimilarities between five forest communities. For. Ecosyst. 6, 1–8. doi: 10.1186/s40663-019-0188-9
Hietz, P. (2010). “Ecology and ecophysiology of epiphytes in tropical montane cloud forests,” in Tropical montane cloud forests. Science for conservation and management, eds L. A. Bruijnzeel, F. N. Scatena, and L. S. Hamilton (Cambridge: Cambridge University Press), 67–76. doi: 10.1017/cbo9780511778384.007
Hietz, P., and Hietz-Seifert, U. (1995). Structure and ecology of epiphyte communities of a cloud forest in central Veracruz, Mexico. J. Veg. Sci. 6, 719–728. doi: 10.2307/3236443
Hietz, P., Buchberger, G., and Winkler, M. (2006). Effect of forest disturbance on abundance and distribution of epiphytic bromeliads and orchids. Ecotropica 12, 103–112.
Hietz-Seifert, U., Hietz, P., and Guevara, S. (1996). Epiphyte vegetation and diversity on remnant trees after forest clearance in southern Veracruz, Mexico. Biol. Conserv. 75, 103–111. doi: 10.1016/0006-3207(95)00071-2
Holt, E. A., and Miller, S. W. (2011). Bioindicators: using Organisms to Measure Environmental Impacts. Nat. Educ. Knowl. 3:8.
Instituto de Información Estadística y Geográfica [IIEG] (2018). Cabo Corrientes: diagnóstico del municipio. Gobierno del Estado de Jalisco. Mexico: Instituto de Información Estadística y Geográfica IIEG, 33.
Jácome, J., Galeano, G., Amaya, M., and Mora, M. (2004). Vertical distribution of epiphytic and hemiepiphytic Araceae in a tropical rain forest in Chocó, Colombia. Selbyana 25, 118–125. doi: 10.2307/41760150
Jiménez-López, D. A., Roblero-Velasco, R. J., Martínez-Meléndez, N., Ocampo, G., and Gallardo-Cruz, J. A. (2017). Relación entre variables del forófito y la riqueza de epífitas vasculares en los Pantanos de Centla, Tabasco, México. Acta Bot. Mex. 121, 125–137. doi: 10.21829/abm121.2017.1179
Jiménez-López, D. A., Solórzano, J. V., Vibrans, H., Espejo-Serna, A., and Peralta-Carreta, C. (2019). Ceremonial use of bromeliads and other vascular epiphytes in cemeteries of two indigenous communities of Las Margaritas, Chiapas, Mexico. Econ. Bot. 73, 127–132. doi: 10.1007/s12231-019-09445-4
Johansson, D. (1974). Ecology of vascular epiphytes in West African rain forest. Acta Phytogeog. Suec. 59, 1–129.
Kerbauy, G. B., Takahashi, C. A., Lopez, A. M., Matsumura, A. T., Hamachi, L., Félix, L. M., et al. (2012). “Crassulacean acid metabolism in epiphytic orchids: current knowledge, future perspectives,” in Applied photosynthesis, ed. M. M. Najafpour (Rijeka: InTech), 81–104.
Köster, N., Friedrich, K., Nieder, J., and Barthlott, W. (2009). Conservation of epiphyte diversity in an Andean landscape transformed by human land use. Conserv. Biol. 25, 911–919. doi: 10.1111/j.1523-1739.2008.01164.x
Köster, N., Kreft, H., Nieder, J., and Barthlott, W. (2013). Range size and climatic niche correlate with the vulnerability of epiphytes to human land use in the tropics. J. Biogeogr. 40, 963–976. doi: 10.1111/jbi.12050
Köster, N., Nieder, J., and Barthlott, W. (2011). Effect of host tree traits on epiphyte diversity in natural and anthropogenic habitats in Ecuador. Biotropica 43, 685–694. doi: 10.1111/j.1744-7429.2011.00759.x
Kreft, H., Köster, N., Küper, W., Nieder, J., and Barthlott, W. (2004). Diversity and biogeography of vascular epiphytes in Western Amazonia, Yasuní, Ecuador. J. Biogeogr. 31, 1463–1476. doi: 10.1111/j.1365-2699.2004.01083.x
Kress, W. J. (1986). The systematic distribution of vascular epiphytes: an update. Selbyana 9, 2–22. doi: 10.1590/s1519-69842009000300027
Krömer, T., Acebey, A. R., Kluge, J., and Kessler, M. (2013). Effects of altitude and climate in determining elevational plant species richness patterns: a case study from Los Tuxtlas, Mexico. Flora 208, 197–210. doi: 10.1016/j.flora.2013.03.003
Krömer, T., Acebey, A., and Toledo-Aceves, T. (2018). “Aprovechamiento de plantas epífitas: implicaciones para su conservación y manejo sustentable,” in De la recolección a los agroecosistemas: soberanía alimentaria y conservación de la biodiversidad, perteneciente a la serie: Hacia la sustentabilidad, eds E. Silva-Rivera, V. Martínez-Valdéz, M. Lascurain, and E. Rodríguez-Luna (Xalapa: Editorial de la Universidad Veracruzana), 175–196.
Krömer, T., and Gradstein, S. R. (2016). “Vascular epiphytes,” in Core standardized method for rapid biological field assessment, ed. T. H. Larsen (Arlington, VA: Conservation International), 25–36.
Krömer, T., Espejo-Serna, A., López-Ferrari, A. R., Acebey, A. R., García-Cruz, J., and Mathieu, G. (2020). Las angiospermas epífitas del estado de Veracruz, México: diversidad y distribución. Rev. Mex. Biodivers. 91:e913415. doi: 10.22201/ib.20078706e.2020.91.3415
Krömer, T., García-Franco, J. G., and Toledo-Aceves, T. (2014). “Epífitas vasculares como bioindicadores de la calidad forestal: impacto antrópico sobre su diversidad y composición,” in Bioindicadores: guardianes de nuestro futuro ambiental, eds C. A. González-Zuarth, A. Vallarino, J. C. Pérez-Jiménez, and A. M. Low-Pfeng (México, D. F. y Campeche: Instituto Nacional de Ecología y Cambio Climático [INECC] – El Colegio de la Frontera Sur [ECOSUR]), 605–623.
Krömer, T., Kessler, M., and Gradstein, S. R. (2007a). Vertical stratification of vascular epiphytes in submontane and montane forest of the Bolivian Andes: the importance of the understory. Plant Ecol. 189, 261–278. doi: 10.1007/s11258-006-9182-8
Krömer, T., Gradstein, S. R., and Acebey, A. (2007b). Diversidad y ecología de epífitas vasculares en bosques montanos primarios y secundarios de Bolivia. Ecol. Boliv. 42, 23–33.
Krömer, T., Kessler, M., Gradstein, S. R., and Acebey, A. (2005). Diversity patterns of vascular epiphytes along an elevational gradient in the Andes. J. Biogeogr. 32, 1799–1809. doi: 10.1111/j.1365-2699.2005.01318.x
Krömer, T., Viccon-Esquivel, J., and Gómez-Díaz, J. A. (2021). “Efectos antrópicos sobre la diversidad de epífitas vasculares y orquídeas en el centro de Veracruz,” in Las orquídeas de Veracruz, eds J. Viccon-Esquivel, M. Castañeda-Zárate, R. Castro-Cortés, and W. Cetzal (Xalapa, Ver: Editorial de la Universidad Veracruzana), 235–252.
Kun, A., Oborny, B., and Dieckmann, U. (2009). Intermediate landscape disturbance maximizes metapopulation density. Lands. Ecol. 24, 1341–1350. doi: 10.1007/s10980-009-9386-0
Larrea, M. L., and Werner, F. A. (2010). Response of vascular epiphyte diversity to different land-use intensities in a neotropical montane wet forest. For. Ecol. Manag. 260, 1950–1955. doi: 10.1016/j.foreco.2010.08.029
Malizia, A. (2003). Host tree preference of vascular epiphytes and climbers in a subtropical montane cloud forest of Northwest Argentina. Selbyana 24, 196–205. doi: 10.2307/41760133
Martínez-Meléndez, N., Pérez-Farrera, M. A., and Flores-Palacios, A. (2008). Estratificación vertical y preferencia de hospedero de las epífitas vasculares de un bosque nublado de Chiapas, México. Rev. Biol. Trop. 56, 2069–2086. doi: 10.15517/rbt.v56i4.5780
Mayo, S. J., Bogner, J., and Boyce, P. C. (1997). The genera of Araceae. London: Royal Botanical Gardens, Kew, 370.
Mehltreter, K., Flores-Palacios, A., and García-Franco, J. G. (2005). Host preferences of low-trunk vascular epiphytes in a cloud forest of Veracruz, Mexico. J. Trop. Ecol. 21, 651–660. doi: 10.1017/S0266467405002683
Menini-Neto, L., Campostrini-Forzza, R., and Zappi, D. (2009). Angiosperm epiphytes as conservation indicators in forest fragments: a case study from southeastern Minas Gerais, Brazil. Biodivers. Conserv. 18, 3785–3807. doi: 10.1007/s10531-009-9679-2
Miguel-Vázquez, M. I., Espejo-Serna, M. A., Ceja-Romero, J., and Cerros-Tlatilpa, R. (2020). Las angiospermas epiìfitas de Puebla: riqueza y distribucioìn. Bot. Sci. 98, 1–65. doi: 10.17129/botsci.2540
Mondragón, D. (2015). La comercialización navideña de bromelias epífitas en la ciudad de Oaxaca, México. Etnobiología 6, 24–28.
Mondragón, D., and Ticktin, T. (2011). Demographic effects of harvesting epiphytic bromeliads and an alternative approach to collection. Conserv. Biol. 25, 797–807. doi: 10.1111/j.1523-1739.2011.01691.x
Mondragón, D., Maldonado, C., and Aguilar-Santelises, R. (2007). Life history and demography of a twig epiphyte: a case study of Erycina crista-galli (Orchidaceae). Selbyana 28, 39–46. doi: 10.2307/41760304
Morales-Hernández, J. L., Carrillo-González, F. M., Farfán-Molina, L. M., and Cornejo-López, V. M. (2016). Cambio de cobertura vegetal en la región de Bahía de Banderas, México. Rev. Colomb. Biotecnol. 18, 7–16. doi: 10.15446/caldasia.v38n1.57831
Morrone, J. J., Escalante, T., and Rodríguez-Tapia, G. (2017). Mexican biogeographic provinces: map and shapefiles. Zootaxa 4277, 277–279. doi: 10.11646/zootaxa.4277.2.8
Newbold, T., Hudson, L. N., Hill, S. L. L., Contu, S., Lysenko, I., Senior, R. A., et al. (2015). Global effects of land use on local terrestrial biodiversity. Nature 520, 45–50. doi: 10.1038/nature14324
Ochoa-López, S. (2009). Influencia de hospederos y condiciones microclimáticas en la distribución de plantas epífitas del género Tillandsia (Bromeliaceae) en la selva baja caducifolia de la región de Chamela-Cuixmala, Jalisco, México. Ph.D. thesis. Ciudad de México: Facultad de Ciencias, UNAM
Olmsted, I., and Gómez-Juárez, M. (1996). Distribution and conservation of epiphyteson the Yucatán Peninsula. Selbyana 17, 58–70.
Órgano informativo oficial del municipio de Cabo Corrientes, Jalisco (2012). Programa municipal de desarrollo urbano. Cabo Corrientes: Gaceta Municipal, 319.
Pérez-Peña, A., and Krömer, T. (2017). “>Qué pueden aportar los acahuales y las plantaciones de cítricos a la conservación de las epífitas vasculares en Los Tuxtlas, Veracruz?,” in Avances y Perspectivas en la Investigación de los Bosques Tropicales y sus Alrededores: la Región de Los Tuxtlas, eds V. H. Reynoso, R. I. Coates, and M. L. Vázquez-Cruz (Ciudad de México: Instituto de Biología, Universidad Nacional Autónoma de México), 569–580.
Ramírez-Delgadillo, R., and Cupul-Magaña, F. (1999). Contribución al conocimiento de la flora de la Bahía de Banderas, Nayarit-Jalisco, México. Ciencia ergo-sum Rev. Cient. Multidis. Univ. Autón. Estad. Méx. 6, 135–146.
Reyes-García, C., Griffiths, H., Rincón, E., and Huante, P. (2007). Niche differentiation in tank and atmospheric epiphytic bromeliads of a seasonally dry forest. Biotropica 40, 168–175. doi: 10.1111/j.1744-7429.2007.00359.x
Rzedowski, J. (1978). Vegetacioìn de Meìxico. Meìxico: Comisioìn Nacional para Conocimiento y Uso de la Biodiversidad, 504.
Rzedowski, J., and McVaugh, R. (1966). La vegetación de Nueva Galicia. Ann Arbor, Michigan, EUA: University of Michigan, 664.
Sabagh, L. T., Barbosa-Ferreria, R., and Duarte-Rocha, C. F. (2017). Host bromeliads and their associated frog species: further considerations on the importance of species interactions for conservation. Symbiosis 73, 201–211. doi: 10.1007/s13199-017-0500-9
Sala, O. E., Chapin, F. S. III, Armesto, J. J., Berlow, E., Bloomfield, J., et al. (2000). Global biodiversity scenarios for the year 2100. Science 287, 1770–1774. doi: 10.1126/science.287.5459.1770
Schuiteman, A., and Chase, M. (2015). A reappraisal of Maxillaria (Orchidaceae). Phytotaxa 225, 1–78. doi: 10.11646/phytotaxa.225.1.1
Sodhi, N. S., Koh, L. P., Peh, K. S.-H., Tan, H. T. W., Chazdon, R. L., Corlett, R. T., et al. (2008). Correlates of extinction proneness in tropical angiosperms. Divers. Distrib. 14, 1–10. doi: 10.1111/j.1472-4642.2007.00398.x
ter Steege, H., and Cornelissen, J. H. C. (1989). Distribution and ecology of vascular epiphytes in lowland rain forest of Guyana. Biotropica 21, 331–339. doi: 10.2307/2388283
The Angiosperm Phylogeny Group (2016). An update of the Angiosperm Phylogeny Group classification for the orders and families of flowering plants: APG IV. Bot. J. Linn. Soc. 181, 1–20. doi: 10.1111/boj.12385
The Plant List (2013). The Plant List Versión 1.1. Available Online at: http://www.theplantlist.org/ (accessed November, 2020).
Toledo-Aceves, T., García-Franco, J. G., and López-Barrera, F. (2014). Bromeliad rain: an opportunity for cloud forest management. For. Ecol. Manag. 329, 129–136. doi: 10.1016/j.foreco.2014.06.022
Trejo-Cruz, I. A., Martínez-Camilo, R., Martínez-Meléndez, N., and Jiménez-López, D. A. (2021). Diversidad de epífitas vasculares en árboles remanentes del género Ficus (Moraceae) es sistemas silvopastoriles del sureste de México. Acta Bot. Mex. 128, 1–20. doi: 10.21829/abm128.2021.1827
Turner, I., Tan, H., Wee, Y., Ibrahim, A., Chew, P., and Corlett, R. (1994). A study of plant species extinction in Singapore: lessons for the conservation of tropical biodiversity. Conserv. Biol. 8, 705–712. doi: 10.1046/j.1523-1739.1994.08030705.x
Vázquez-García, J. A., Reynoso-Dueñas, J. J., Vargas-Rodríguez, Y. L., and Frías-Ureña, H. G. (2000). Jalisco-Costa Norte: patrimonio ecológico, cultural y productivo de México. Libro en versión electrónica. Mexico: Universidad de Guadalajara.
Vergara-Rodríguez, D., Mathieu, G., Samain, M.-S., Armenta-Montero, S., and Krömer, T. (2017). Diversity, distribution and conservation status of Peperomia (Piperaceae) in the state of Veracruz, Mexico. Trop. Conserv. Sci. 10, 1–28. doi: 10.1177/1940082917702383
Vergara-Torres, C. A., Pacheco-Álvarez, M. C., and Flores-Palacios, A. (2010). Host preference and host limitation of vascular epiphytes in a tropical dry forest of central Mexico. J. Trop. Ecol. 26, 563–570. doi: 10.1017/S0266467410000349
Victoriano-Romero, E., Valencia-Díaz, S., Toledo-Hernández, V. H., and Flores-Palacios, A. (2017). Dispersal limitation of Tillandsia species correlates with rain and host structure in a central Mexican tropical dry forest. PLoS One 12:e0171614. doi: 10.1371/journal.pone.0171614
Werner, F. A., and Gradstein, S. R. (2009). Diversity of dry forest epiphytes along a gradient of human disturbance in the tropical Andes. J. Veg. Sci. 20, 59–68. doi: 10.1111/j.1654-1103.2009.05286.x
Werner, F. A., Köster, N., Kessler, M., and Gradstein, S. R. (2011). Is the resilience of epiphyte assemblages to human disturbance a function of local climate? Ecotropica 17, 15–20. doi: 10.5167/uzh-76892
Wilson, M. C., Chen, X. Y., Corlett, R. T., Didham, R. K., Ding, P., Holt, R. D., et al. (2016). Habitat fragmentation and biodiversity conservation: key findings and future challenges. Landsc. Ecol. 31, 219–227. doi: 10.1007/s10980-015-0312-3
Winkler, M., Hülber, K., and Hietz, P. (2005). Effect of canopy position on germination and seedling survival of epiphytic bromeliads in a Mexican humid montane forest. Ann. Bot. 95, 1039–1047. doi: 10.1093/aob/mci115
Wolf, J. H. D. (2005). The response of epiphytes to anthropogenic disturbance of pine-oak forest in the highlands of Chiapas, México. For. Ecol. Manag. 212, 376–393. doi: 10.1016/j.foreco.2005.03.027
Zotz, G. (2004). How prevalent is crassulacean acid metabolism among vascular epiphytes? Oecologia 138, 184–192. doi: 10.1007/s00442-003-1418-x
Zotz, G. (2013). “Hemiepiphyte”: a confusing term and its history. Ann. Bot. 111, 1015–1020. doi: 10.1093/aob/mct085
Zotz, G. (2016). Plants on plants - The biology of vascular epiphytes - Fascinating life sciences. Switzerland: Springer, 283.
Zotz, G., and Andrade, J. (2002). “La ecología y fisiología de las epífitas y hemiepífitas,” in Ecología y conservación de bosques neotropicales, eds M. R. Guariguata and G. H. Kattan (San José: Libro Universitario Regional del Instituto Tecnológico de Costa Rica), 271–296.
Zotz, G., and Hietz, P. (2001). The physiological ecology of vascular epiphytes: current knowledge, open questions. J. Exp. Bot. 52, 2067–2078. doi: 10.1093/jexbot/52.364.2067
Keywords: beta diversity, bromeliads, disturbance, floristic composition, orchids
Citation: Flores-Argüelles A, Espejo-Serna A, López-Ferrari AR and Krömer T (2022) Diversity and Vertical Distribution of Epiphytic Angiosperms, in Natural and Disturbed Forest on the Northern Coast of Jalisco, Mexico. Front. For. Glob. Change 5:828851. doi: 10.3389/ffgc.2022.828851
Received: 04 December 2021; Accepted: 04 April 2022;
Published: 11 May 2022.
Edited by:
Ángel Benítez, Universidad Técnica Particular de Loja, EcuadorCopyright © 2022 Flores-Argüelles, Espejo-Serna, López-Ferrari and Krömer. This is an open-access article distributed under the terms of the Creative Commons Attribution License (CC BY). The use, distribution or reproduction in other forums is permitted, provided the original author(s) and the copyright owner(s) are credited and that the original publication in this journal is cited, in accordance with accepted academic practice. No use, distribution or reproduction is permitted which does not comply with these terms.
*Correspondence: Alejandra Flores-Argüelles, YWZhMjUwMkBnbWFpbC5jb20=
†ORCID: Thorsten Krömer, orcid.org/0000-0002-1398-8172
Disclaimer: All claims expressed in this article are solely those of the authors and do not necessarily represent those of their affiliated organizations, or those of the publisher, the editors and the reviewers. Any product that may be evaluated in this article or claim that may be made by its manufacturer is not guaranteed or endorsed by the publisher.
Research integrity at Frontiers
Learn more about the work of our research integrity team to safeguard the quality of each article we publish.