- 1Department of Biodiversity and Bioecology, School of Sciences, Institute of Natural Sciences and Mathematics, Ural Federal University, Ekaterinburg, Russia
- 2Department of Experimental Biology and Biotechnology, School of Sciences, Institute of Natural Sciences and Mathematics, Ural Federal University, Ekaterinburg, Russia
Diversity of secondary lichen metabolites was studied in epiphytic lichens on six phorophytes—spruce, pine, birch, alder, aspen and poplar in the Middle Urals of Russia. Atranorin, usnic, fumarprotocetraric acid, zeorin, and gyrophoric acid were found in 31, 24, 23, 18, and 14 species, respectively, of 237 taxa collected. Seventy-seven species (i.e., 32% of total species documented) contained no secondary metabolites. Spectra of secondary metabolites of fruticose and foliose lichens varied on different phorophytes, while in crustose species the strong dependence on the tree species was not detected. This is different to the pH dependence of saxicolous lichens where crustose lichens were more susceptible to the rock chemistry. The results of Canonical Correspondence Analysis reveal the affinity of species containing depsides, depsidones or usnic acid to acidic substrata and those lacking secondary metabolites or containing terpenes and antraquinones to the pH-neutral bark. We suppose that phenolic compounds and flavonoids, as chemical constituents of bark, may interact with lichen symbioses and elements in phellem, and similarly to the lichen acids shape the affinity of species to the substrata.
Introduction
Lichens contain from 800 (Elix and Stocker-Wörgötter, 2008) to 1,050 (Stocker-Wörgötter, 2008; Goga et al., 2018) secondary metabolites, or lichen acids, that belong to various groups, including aliphatic acids, antraquinones, phenolic compounds, quinones, pulvinic acid derivatives, steroids, terpenes, and xanthones (Elix, 2014). Some secondary lichen metabolites act as photoprotectors (Legouin et al., 2017; Phinney et al., 2019; Beckett et al., 2021), or cause an ability of species to withstand high temperatures or a prolonged drought (Asplund et al., 2017; Lutsak et al., 2017), others act as allelopathic agents (Giordano et al., 1997), or may alter a permeability of the cell membrane of phycobionts (Lawrey, 1986).
Secondary lichen metabolites play an important role in interaction of lichens with their substrates Hauck and Jürgens, 2008; Hauck et al., 2010). The property as weaker or stronger acids enables lichen substances shape preferences of species to the substrate pH (Bačkor et al., 1997; Hauck and Jürgens, 2008). This action of usnic acid is based on its activity as a protonophore that aids penetration of hydrogen ions to plasmalemma and acidification of protoplasts (Hauck et al., 2009b), the feature that made this metabolite a widely known antimicrobial, antitumor and anti-inflammatory agent (Antonenko et al., 2019). Lichen acids with a lower constant of dissociation such as fumarprotocetraric, perlatolic, or thamnolic may impart lichens a higher acidity tolerance (Hauck et al., 2009a). These chemical properties of secondary lichen metabolites result in differential selection of substrates by lichens containing different lichen acids. Crustose species that specialize in siliceous rock substrates produce more lichen substances than those on calcareous substrates (Spier and Aptroot, 2005) and the abundance of species containing particular chemical constituents depends on the rocky substrate (Paukov et al., 2019). Variation of secondary chemistry is found between species of the same genus growing on different rocks (Favero-Longo et al., 2015).
Rock and bark offer lichens a different set of conditions. Lichens on most rock types interact with substantially higher concentrations of metals (Purvis and Halls, 1996; Favero-Longo et al., 2004). Unpolluted bark has a lower amount of elements (Purvis et al., 2005) but contains variable organic compounds produced by a tree species and is more susceptible to changes caused by airborne pollutants (van Dobben et al., 2001). Both bark and rocks in natural conditions vary from more acidic to neutral or basic, however, bark has lower minimal values and higher variation rates compared to rocks (Skye, 1968; Paukov et al., 2019).
The working hypothesis was that there is an interaction of secondary metabolites in lichens and bark that results in different species composition and abundance of epiphytes on a variety of phorophytes. The aims of the paper were: (1) To evaluate if epiphytic lichens producing different secondary metabolites have distinct affinities to substrates with different pH and (2) to evaluate if organic chemical constituents of phorophyte bark affect the species diversity of epiphytic lichens. We estimated lichen diversity and the variability of secondary metabolites on six different tree species ranging from acid to neutral bark, Siberian spruce (Picea obovata Ledeb.), Scotch pine (Pinus sylvestris L.), common birch (Betula pendula Roth), speckled alder [Alnus incana (L.) Moench], European aspen (Populus tremula L.), and balsam poplar (Populus balsamifera L.) in protected regions of the southern part of Sverdlovsk region (Middle Ural, Russia) and the abundance of lichens in relation to their secondary chemistry on these phorophytes.
Materials and Methods
Study Area
Epiphytic lichens were collected in Pripyshminskiye Bory, Olenyi Ruchyi (Paukov, 2003; Paukov and Teptina, 2013) and Reka Chusovaya (57°16′N, 59°17′E) national parks, and in the vicinity of Dvurechensk settlement (Figure 1). These territories are situated far from sources of atmospheric pollution that prevented possible contamination of the bark by airborne pollutants. The territory of Pripyshminskiye Bory national park belongs to West Siberian plain, hemiboreal small-leaved forests zone. Olenyi Ruchyi and Reka Chusovaya national parks and Dvurechensk belong to the mountain taiga zone. The territory is covered by coniferous forests dominated by Scotch pine and Siberian spruce and secondary forest vegetation with birch and aspen. The climate of the territory is moderately continental, with a cold winter and a warm summer. Average annual temperature varies from –0.7 to + 1.3°C (Ogureeva et al., 2018).
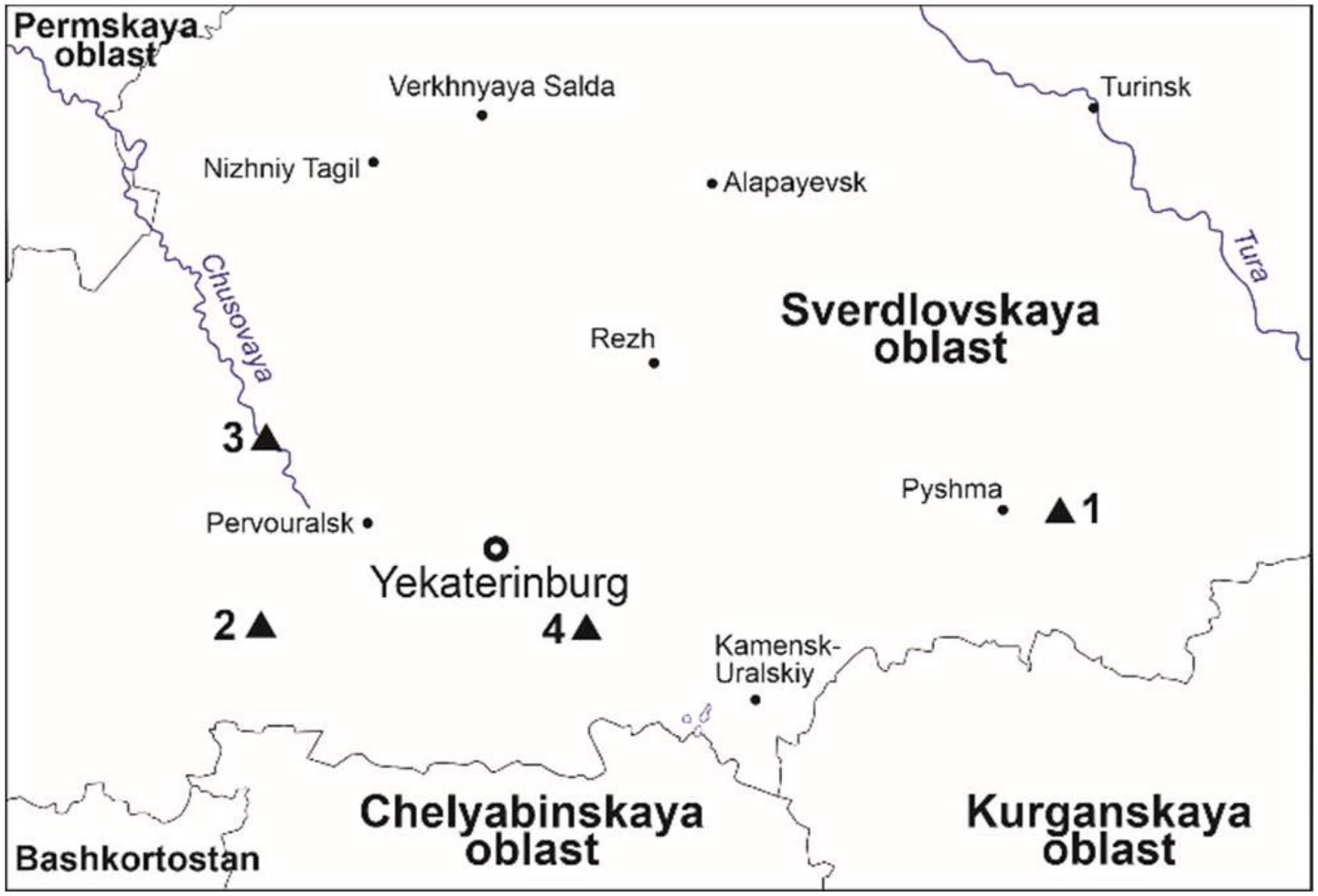
Figure 1. Study area and sampling localities. Modified from maps at d-maps.com. (1) Pripyshminskiye Bory national park, (2) Olenyi Ruchyi national park, (3) Reka Chusovaya national park, (4) Dvurechensk settlement.
Field Survey
The biodiversity of lichens in the protected territories of Middle Urals was studied by a route method (Vassiliyeva, 1959). The routes were defined using vegetation maps so as to visit various forest stands dominated by different phorophytes. Within every stand thorough collecting of epiphytic lichens was undertaken within 10 × 10 m plots. A list of species was compiled for every phorophyte, which was used for comparison the diversity of secondary metabolites of lichens. The most species-rich phorophytes were then selected for recording epiphytic lichen groupings. Epiphytes of pine, birch and poplar were recorded near Dvurechensk settlement (56°36′N, 61°02′E) (Figure 1). The main type of vegetation near Dvurechensk is pine forests with domination of Vaccinium myrtillus L. and Pteridium pinetorum C.N. Page and R.R. Mill. Birch stands are distributed along rivers or as a secondary vegetation after logging. Poplar is an alien species that was widely used in townships of the region some 50–100 years ago. Now it is losing its popularity but remains a very common tree in older parts of settlements. For the characterization of epiphytes on this phorophyte we used poplars in a village of Klyuchi (56°37′N, 61°03′E), 3 km NE from Dvurechensk. Lichens of aspen, spruce, and the rest of birch trees was described in Reka Chusovaya nature park where the dominated vegetation types are spruce forests intermixed with aspen and birch stands as a secondary vegetation. Vertical trees of every phorophyte species with the breast height diameter not less than 40 cm were selected. Epiphyte lichen groupings on alder were not described as this phorophyte has mostly slanted trunks. Thirty trees of poplar, 50—of spruce, 90—of both aspen and birch, and 100—of pine were studied. Every tree was photographed with a superposed 10 × 10 cm quadrate mesh subdivided into 100 1 × 1 cm squares twice, at the base, and at the height of 1.3 m. We followed the approach of preferential sampling (Pennino et al., 2019), describing the most species-rich lichen assemblages and avoiding trees devoid of lichens at either height above the ground. The total number of quadrats used in this study is 720. Bark samples from every tree species were collected together with lichens from the photographed quadrats on the height of 1.3 m to the depth of 2–3 mm. Ten samples for each phorophyte were arbitrarily selected for the determination of pH, and five samples for the determination of phenolic compounds, terpenes, and flavonoids. Care was taken to select the bark populated by lichens as it differs from bare bark in an elevated levels of phenolic compounds (Latkowska et al., 2015).
For determination of species most crustose lichens (genera Lecanora, Lepraria, and others) and foliose-fruticose lichens known for their variable chemistry (Bryoria, Usnea) were studied chromatographically. Specimens of taxa known for their constant chemistry were periodically checked to approve the absence of chemosyndromes in the studied region. Selected thalli of species photographed during the registration of the cover of lichen groupings were taken for the control determination of the composition of secondary metabolites in a laboratory.
Analytical Procedures
Secondary lichen products were analyzed using WinTab software (Lafferty et al., 2021) by applying standard thin-layer chromatography techniques (Culberson and Kristinsson, 1970; Orange et al., 2001) in solvent systems A (toluene:1,4-dioxane:acetic acid, 180:45:5), B (hexane:diethyl ether:formic acid, 140:72:18) and C (toluene:acetic acid, 170:30). For rapid data sorting and operation an electronic table was created where collected species were arranged in rows and phorophytes together with secondary lichen metabolites were arranged in columns. The presence of a species on a particular phorophyte was marked as “1.” Similarly, the presence of a particular metabolite in a lichen species was marked by “1.”
The determination of pH of water extraction was performed following the protocol of Kricke (2002) with some modifications. For this, 2 g of powdered bark samples were poured into 20 mL of distilled water and left for 24 h with periodical shaking. The pH was measured after precipitation of particles with a pH meter (Anion 4100, Novosibirsk, Russia) in ten repetitions for every phorophyte.
Bark chemistry was analyzed using thin layer chromatography (Wagner et al., 1984; Ermoshin et al., 2021) in a solvent system toluene:ethyl acetate:formic acid, 30:18:2. One hundred milligrams of bark of six tree species in 3 repeats each were extracted in 1 mL acetone and 14 mkL of every extract was applied on a chromatographic plate. Quercetin, gallic, salycilic, and ferulic acid solutions (4 mkg in every spot) were used as a reference. Plates were developed in 2% solutions of aluminum chloride, iron chloride, and phosphotungstic acid in ethanol (Sorescu et al., 2018; Shaikh and Patil, 2020). For a quantitative analysis of chemical constituents 120 mg of bark were poured into 1.5 mL of ethanol and extracted at 55°C in an ultrasound chamber (Grad 80–35, Proton LLC, Moscow, Russia) for 35 min. The extract was centrifuged and the extraction from the same bark sample was repeated three times. Supernatants taken from the same bark sample were united and topped to the volume of 6 mL so as to 1 mL corresponded the extract from 20 mg of bark. A total content of phenolic compounds was determined photometrically (Infinite M200pro photometer, Tecan, Grödig, Austria) using Folin-Ciocalteu reagent with addition of a sodium carbonate. The concentration of phenolic compounds in solutions was expressed in relation to gallic acid. Flavonoids were determined photometrically after addition of an aluminum chloride solution and the concentration was expressed in relation to rutin (Larayetan et al., 2019).
Statistical Analyses
Matrices of (a) metabolite occurrence with respect to lichen species, (b) pH and organic compounds in bark of phorophytes, (c) cover of species with different metabolites on every 10 × 10 cm plot were assembled as Microsoft Excel electronic tables. To avoid the influence of species-specific patterns in the distribution of lichens, depending on the parameters of microhabitats, the cover of species containing the same lichen metabolite was summed in every description within 10 × 10 cm plot. If a species contained several lichen metabolites, its cover was counted correspondingly several times for every lichen acid in a thallus. The abundance of lichens containing different secondary metabolites in relation to the content of organic compounds and the pH of bark was analyzed as raw data in CANOCO 5.0 (Šmilauer and Lepš, 2002). The analyses were separately performed for crustose and foliose-fruticose species for comparison. The response data had a gradient of 7.8 and 6.3 SD units long, respectively, so unimodal constrained ordination (CCA) was used. Organic compounds contained in a one phorophyte species only were not included into the CCA analysis. Differences in percentage of species containing various metabolites were analyzed using χ2 criterion and differences of bark pH were estimated using a Mann-Whitney U-test in Statistica 13.0 (Statsoft, Tulsa, United States). Cluster analysis was performed by a single-linkage method in the PAST 3.18 package (Hammer et al., 2001). The data on secondary chemistry and abundance of epiphytic lichens were compared with similar data on saxicolous species (Paukov et al., 2019).
Results
Species and Secondary Metabolite Diversity
During the general inventory 237 lichen species were found inhabiting tree bark in the protected territories of Middle Urals. The species recorded contained 76 lichen metabolites. Of them, six groups of metabolites represented a half of the total diversity: Nine of them belonged to β-orcinol depsidones, eight—to β-orcinol depsides, and eight to pulvinic acid derivatives. The groups of aliphatic acids and orcinol depsidones were both represented by seven metabolites.
Thirty-five secondary lichen metabolites were found in only one species, 21 were present in 5 or more, and six—in 10 or more epiphytic species. The most common among them was atranorin, which was found in 31 species (Table 1). Usnic, fumarprotocetraric acid, zeorin, and gyrophoric acid were found in 24, 23, 18, and 14 species, respectively. Seventy-seven species (i.e., 32% of total species documented) contained no secondary metabolites. Divaricatic, fumarprotocetraric, lecanoric, squamatic and usnic acids are contained in a larger amount of species compared to saxicolous lichens with the same metabolites, and in the saxicolous group antraquinones, gyrophoric, lobaric, norstictic, psoromic, and stictic acids were contained in a relatively larger amount of species compared to epiphytes.
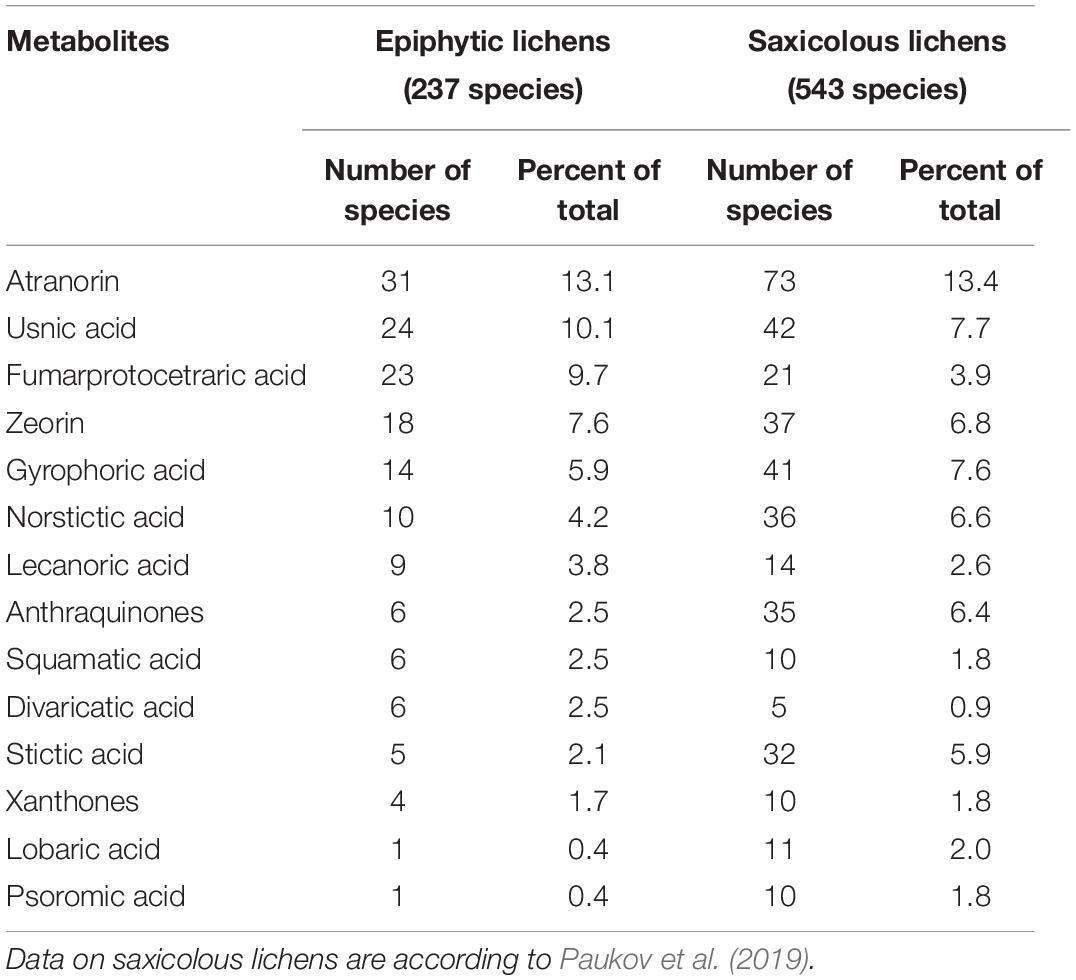
Table 1. The quantity of epiphytic and saxicolous lichen species containing the most frequently recorded secondary metabolites.
Foliose lichens were the most chemically diverse and contained 32 metabolites in 52 species (ratio 0.62), while fruticose lichens had 21 metabolites in 39 taxa (ratio 0.54), and 144 crustose species contained 55 lichen acids (ratio 0.38). Three, 35, and 40 percent of crustose, foliose and fruticose species, respectively, contained no secondary metabolites. Epiphytes are more diverse in lichen metabolites compared to saxicolous species. The latter had metabolite to species ratios of 0.30 for foliose, 0.44 for fruticose, and 0.16 for crustose taxa.
Secondary Metabolite Diversity on Different Phorophytes
The most species-rich substrate was aspen (88 species) followed by birch, alder, spruce and pine (Table 2). The diversity of secondary metabolites did not correlate with the species diversity on a particular tree species but, unlike in the group of saxicolous lichens, the metabolite to species ratio on different phorophytes in epiphytes gradually decreased from spruce to aspen. Similarly, the average number of metabolites in a lichen species decreased in the same direction, the phenomenon that was previously noted also in the group of saxicolous lichens.
The proportion of crustose species without lichen acids on different phorophytes varies but the variability is statistically insignificant (χ2 = 6.8, p = 0.2) (Figure 2A). Lecanoric and vulpinic acids were more common in crustose lichens on conifers and birch (p < 0.05). The only metabolite that increased its percentage in species on aspen was anthraquinone (parietin). No particular trend was seen for other major metabolites, however, zeorin was slightly more abundant in lichens on alder and aspen. This is different to the chemistry of saxicolous crustose lichens where the proportion of species without lichen acids was five times higher on limestone compared to that on quartzite.
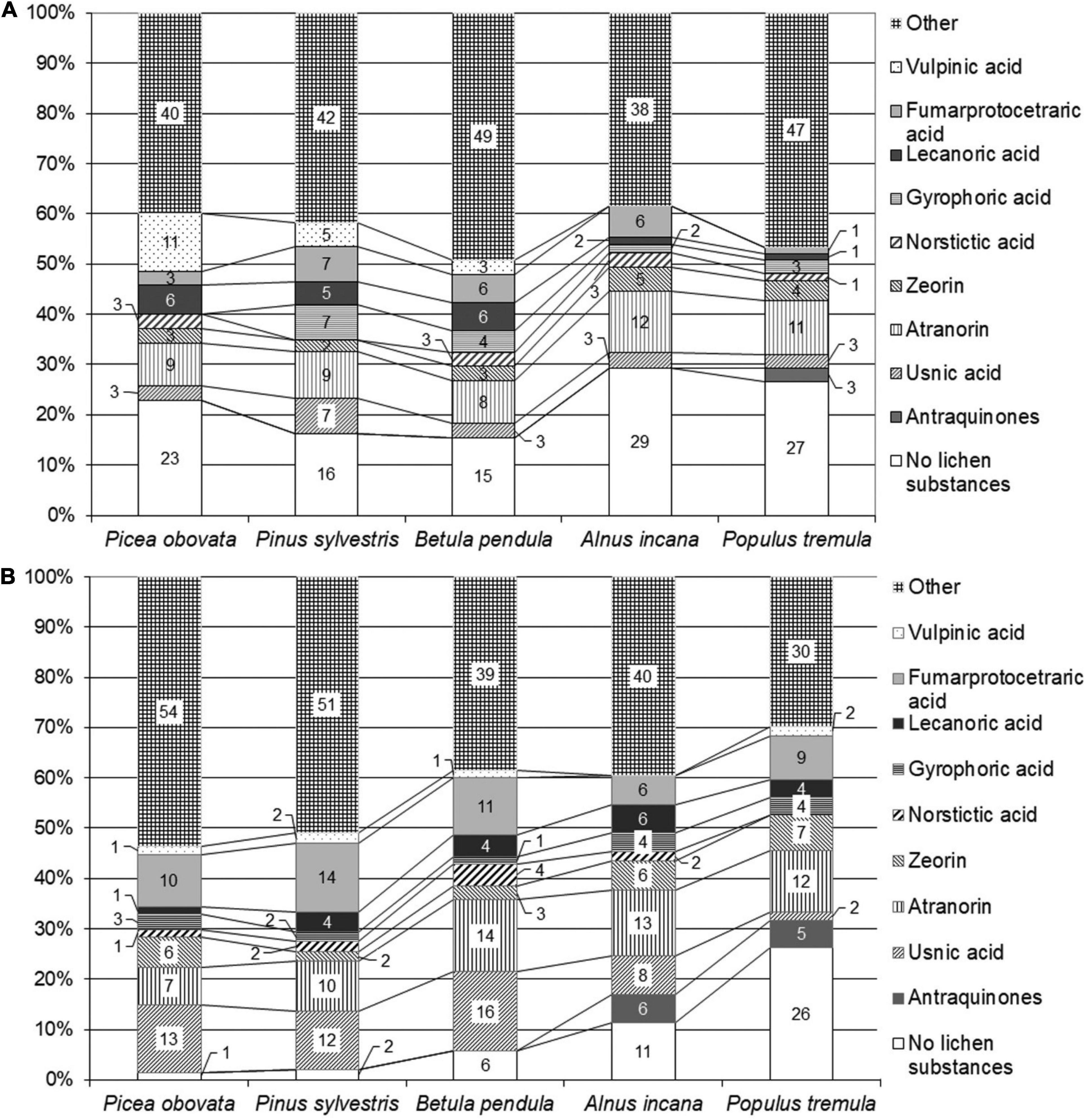
Figure 2. Percentage of lichen species containing major secondary metabolites on different phorophytes. (A) Crustose species, (B) foliose and fruticose species.
Within foliose and fruticose lichens there were 26 percent of species on aspen that contained no secondary metabolites and as low as 1 or 2 percent on coniferous trees (Figure 2B). Species with anthraquinone were the most diverse on aspen (χ2 = 21.9, p = 0.0005) and species with usnic acid were more abundant on conifers and birch (χ2 = 12.05, p = 0.034). Other species that were significantly confined to spruce, pine and birch contained thamnolic, barbatic (χ2 = 18.2, p = 0.002 and 11.2, p = 0.05, respectively), fumarprotocetraric and divaricatic acid, but the dependence of the two latter on the phorophyte species was insignificant. The substrate-dependent variation of secondary metabolites was more pronounced in epiphytic foliose and fruticose compared to saxicolous species as the only statistically supported difference in the latter group was found for species lacking secondary metabolites. Generally, the secondary chemistry of epiphytic foliose and fruticose species is more dependent on the phorophyte as opposed to saxicolous lichens where this dependence is more pronounced in crustose species.
The taxonomic structure of epiphytic lichen groupings is dependent on the species of phorophyte. In crustose lichens the most pronounced differences are found in Caliciaceae family, which is more diverse on conifers, and Ramalinaceae, which is more diverse on deciduous trees (Figure 3A). In both families species growing on pine and spruce contain secondary metabolites and the most taxa found on alder and aspen are lacking “lichen acids.” The taxonomic structure of epiphytic foliose-fruticose species is more simple compared to that of crustose species but the differences between epiphytes of five phorophytes are also visible (Figure 3B). Fruticose Ramalinaceae unlike crustose species belonging this family contain secondary metabolites and found on conifers only. Physciaceae are diverse on aspen and represented by species containing atranorin, zeorin or no lichen substances. As opposed to Physciaceae, Parmeliaceae family, which is rich in secondary metabolites, is more diverse on conifers and birch.
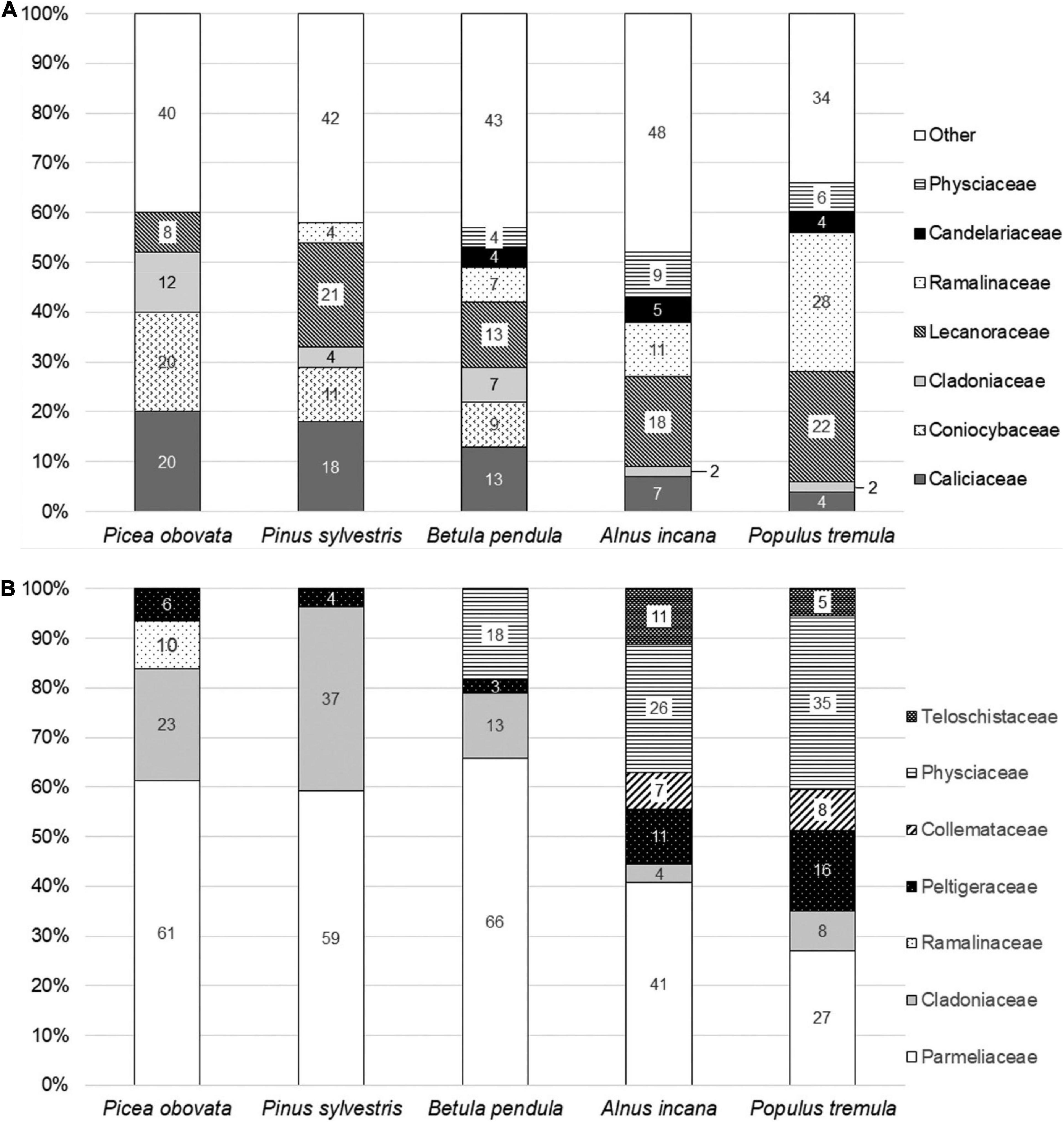
Figure 3. Taxonomic structure of lichen assemblages on five phorophytes. (A) Crustose species, (B) foliose and fruticose species.
Chemical Properties of Bark
Bark of phorophytes varied in chemical composition and pH of water extracts. Thirty-six metabolites belonging to flavonoids, phenolic compounds, quinones, and terpenes were detected chromatographically in acetone extracts. Fifteen of them were found in bark of two or more tree species, and 21 metabolites contained in one species of phorophyte only. Poplar and aspen contained the highest diversity of flavonoids (6 and 7, respectively) (Table 3). Poplar also contained four phenolic compounds followed by pine with three metabolites. Terpenes are the most diverse in birch with eight compounds followed by alder with six metabolites. The chemical composition of bark of studied phorophytes has a low similarity even regarding species belonging to the same family. Pine and spruce have the most similar chemistry (Figure 4).
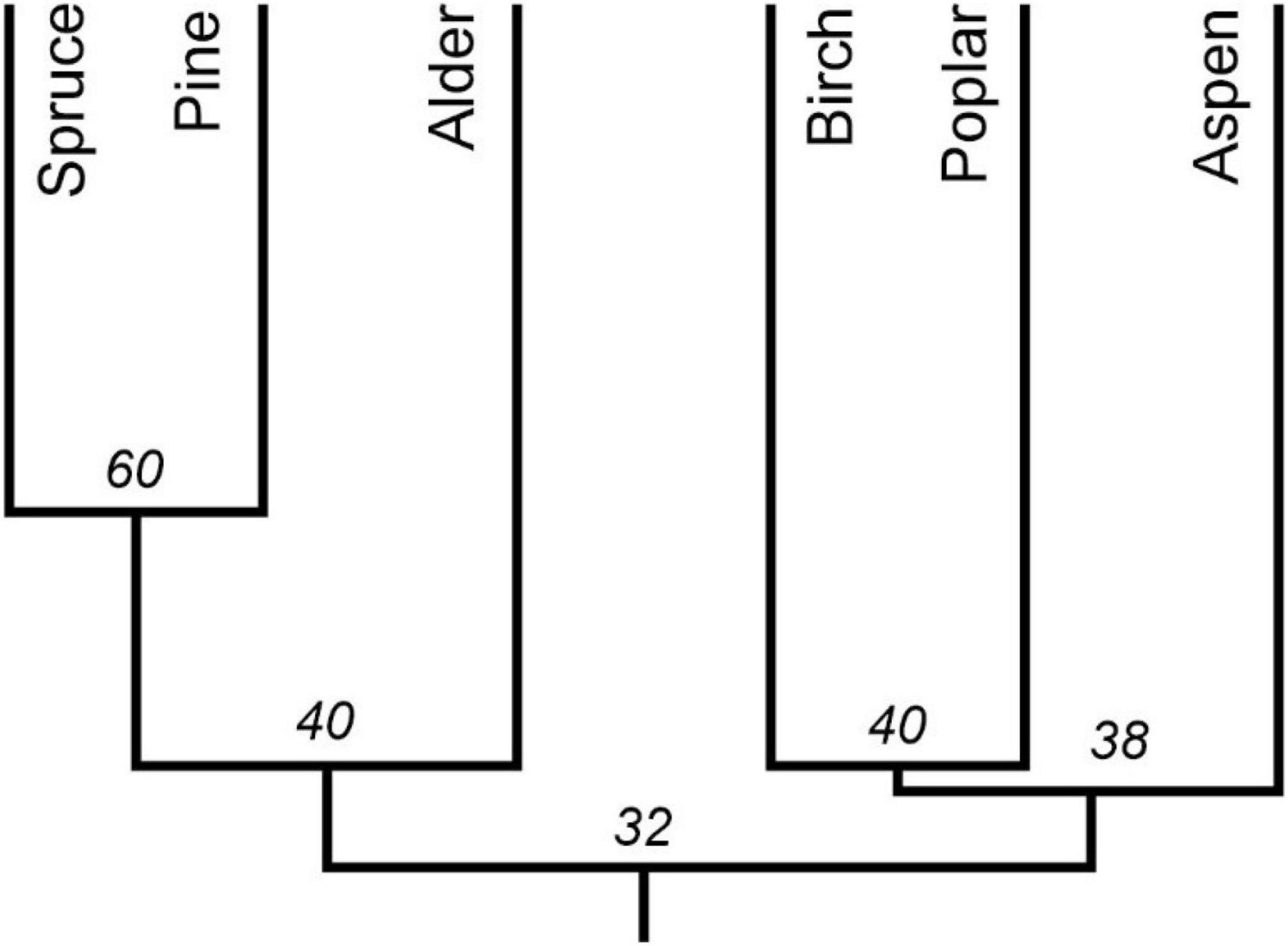
Figure 4. Similarity dendrogram of chemical composition of bark of five phorophytes based on the Sørensen’s coefficient of similarity.
Flavonoids had their highest content in poplar and aspen (44.11–61.86 mg/g) and were the least abundant in alder and birch (1.87–3.51 mg/g) (Table 4). Phenols had the highest concentration in bark of alder (61.06 mg/g); were in a range 44.16–49.24 mg/g in spruce, aspen, and poplar, and had the lowest content in pine and birch (25.21 and 30.62 mg/g, respectively). Bark pH gradually rises from 3.68 in spruce to 6.52 in aspen.

Table 4. pH of samples and a content of phenolic compounds and flavonoids (mg/g) in bark of studied phorophytes.
Secondary Metabolites and Chemistry of Bark
Canonical correspondence analysis (CCA) on relationships between metabolites and the chemical composition of bark showed similar results for crustose and foliose-fruticose species (Figure 5). In both analyses, pH of bark, phenols, flavonoids and two terpenes had high loading on the first axis (63.9% of variance for foliose-fruticose and 34.8% for crustose species) and the second axis (14.7 and 21.7%, for foliose-fruticose and crustose, respectively) reflects a loading of a combination of terpenes as well as flavonoid quercetin with salycilic acid for foliose-fruticose lichens, and phenols with flavonoids for crustose species. The forward-selection procedure implemented in Canoco showed a low impact of the position of lichen groupings (tree base or 1.3 m) compared to other recorded factors.
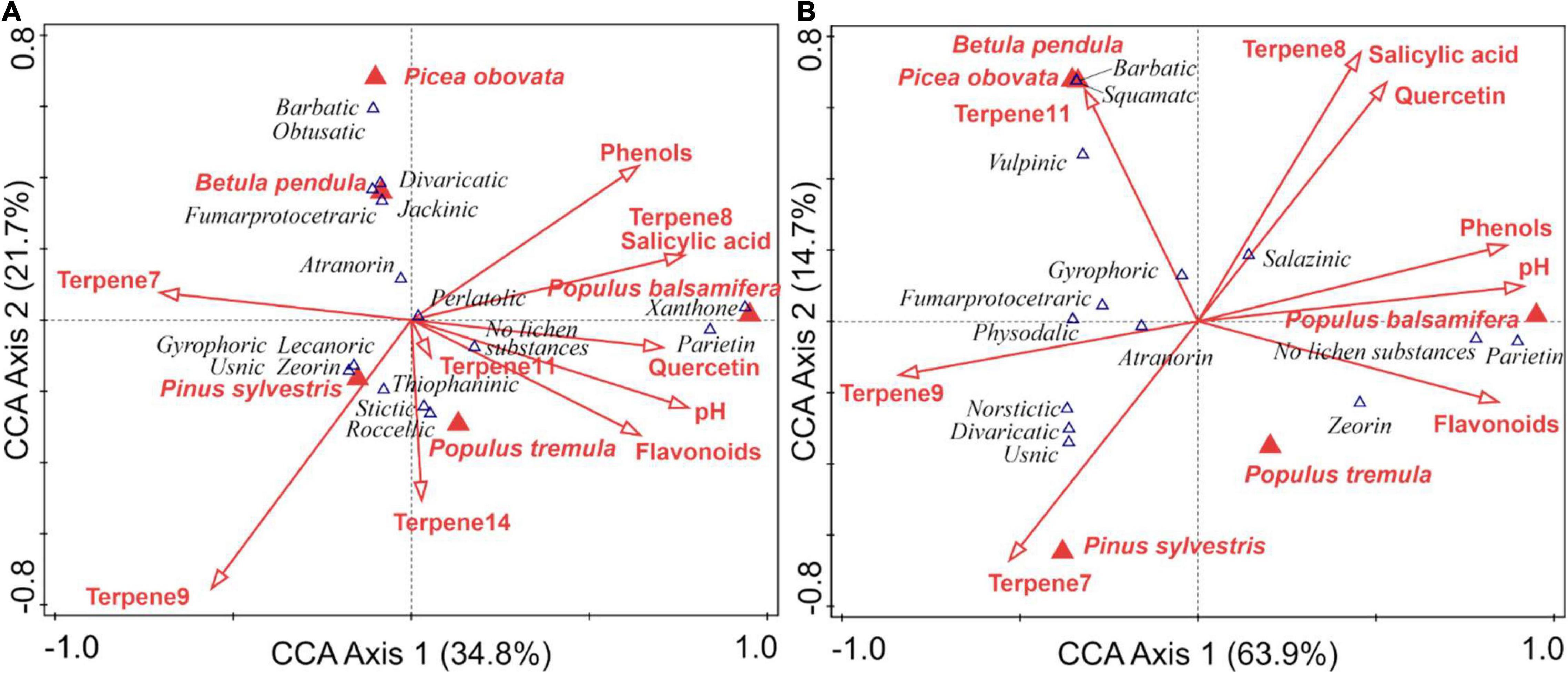
Figure 5. Canonical correspondence analysis (CCA) ordination plot showing the cover of lichens containing different secondary metabolites in relation to phorophytes and their chemical properties. (A) Crustose species, (B) foliose and fruticose species. Bark metabolites found in several phorophytes are shown.
In the CCA, in crustose lichens the species lacking secondary metabolites or with anthraquinones had the highest cover on substrates with higher pH, and content flavonoids and phenols (Figure 5A). A moderate affinity to the substrates with the same characteristics was displayed by species containing stictic, roccellic acids and gangaleoidin, which are more common on aspen compared to poplar. Atranorin, barbatic, divaricatic, fumarprotocetraric, jackinic, and to a lesser extent gyrophoric, lecanoric, usnic acids, and zeorin were associated mostly with conifers and birch with a relatively low pH and a higher content of terpenes.
In foliose-fruticose species, like in the group of crustose lichens, species without secondary metabolites, containing antraquinone parietin, and to a lesser extent β-orcinol depsidone salacinic acid were the most abundant on Populus balsamifera and P. tremula, on substrates with a higher pH and containing higher concentrations of phenolic compounds and flavonoids (Figure 5B). Species containing divaricatic, fumarprotocetraric, norstictic, physodalic, and usnic acid had an affinity to substrates with a low pH and a low concentration of flavonoids and phenolic compounds but containing higher concentrations of terpenes. Fruticose species containing barbatic and squamatic acids were limited to birch and spruce. An aboveground height of lichen groupings performed a little or no effect on the secondary chemistry both for crustose and foliose lichens and was not shown on the graphs.
Generally, both crustose and foliose-fruticose species containing a variety of metabolites were more abundant on substrates with a lower pH, a lower concentration of flavonoids and phenolic compounds and a higher content of terpenes. In both morphological groups only species without secondary metabolites and containing antraquinones are generally confined to a “rich” bark. The former fact is also true for saxicolous species where taxa without “lichen acids” are more abundant on basic substrates and their abundance correlated better with the amount of calcium and strontium, not the pH itself (Paukov et al., 2019).
Discussion
The paper continues our research into the dependence of secondary chemistry of saxicolous lichens on the chemistry of their substrates. Both saxicolous lichens and epiphytes are similar in the relationship of their secondary chemistry to the properties of the substrate. Species growing on rock and bark with a low pH have a more diverse chemistry compared to species on neutral or weakly alkalic substrates that is evident both in metabolite to species ratio and an average number of metabolites in one species. Unlike saxicolous lichens, the dependence of the composition of secondary metabolites on the substrate pH in epiphytes is better visible in foliose-fruticose rather than crustose lichens. A relative amount of species containing gyrophoric, lecanoric, vulpinic acid and antraquinones were dependent on the pH of bark in crustose lichens. In foliose-fruticose species the same was true for species containing no metabolites or usnic acid, and antraquinones. Some metabolites were found in species growing mainly on a particular tree species.
Rocky substrate and bark are different in their chemical properties. The pH values of different kinds of rocks are close to neutral and have a narrower range (6.16 ± 0.06 to 8.18 ± 0.08) compared to that of bark which is more acidic in most phorophytes (3.68 ± 0.10 to 6.52 ± 0.15). Further, the substantial difference between two types of substrates is in the content of metals and transition elements, which is generally much higher in neutral of slightly basic ultramafic rocks compared to unpolluted bark (Bates and Brown, 1981; Rajakaruna et al., 2012; Parzych et al., 2017). Third, bark as opposed to rocky substrates, contains a multitude of secondary metabolites of a non-lichen origin, but are similar to secondary lichen substances, namely terpenes, flavonoids and phenolic compounds.
No rocks with such a low pH were found in the region. The marked shift in pH of bark and rocky substrates causes a rise in a percentage of some metabolites in lichens on a conifer bark. The higher proportion of species containing usnic, thamnolic, and fumarprotocetraric acids (the latter, however, insignificantly) in foliose-fruticose species, vulpinic and lecanoric acids in crustose species on acid bark proves the ability of these components to impart the acidity tolerance to lichens (Hauck and Jürgens, 2008; Hauck et al., 2009a,b). However, provided that pH levels in rock and bark generally do not intersect, the similar tendencies of changes in metabolite spectra in both substrate groups implies that other factors, not only pH, affect the distribution of species containing different metabolites. Additionally, bark with similar pH (aspen and poplar vs. quartzite and granite) has a much higher proportion of species without secondary metabolites.
Lichen secondary metabolites with a high affinity to metals may seemingly play a controversial role. On the one hand, they may chelate elements thus protecting lichens from an excess of toxic metals and preventing them from absorption to the apoplast (Purvis et al., 1987, 1990; Hauck and Huneck, 2007). On the other hand, lichen acids prevent lichens from deficiency of elements (Hauck et al., 2009c). Metabolites that may prevent lichens from excessive metals are those, which are more abundant in saxicolous lichen compared to epiphytes. In our study norstictic, psoromic and stictic acids are components of a higher share of saxicolous lichens. Norstistic and psoromic acids have a high affinity to copper and iron (Purvis et al., 1987) and stictic acid may have similar effect in respect to other metals. Bark pH affects the accumulation rate of metals in lichens (Asplund et al., 2015), and a higher proportion of barbatic, thamnolic and vulpinic acid-containing species on acid bark in our research may also prove the contribution of these metabolites into accumulation of elements. This property of secondary metabolites may have a crucial role for lichens growing on bark compared to most types of rocks as phellem of phorophytes contains lower concentrations of minerals and an ability of “lichen acids” to chelate them at low pH helps lichens attract elements.
The third difference of bark compared to rocks is a presence of organic compounds of a plant origin. As it is shown in the CCA analysis both epiphytic crustose and foliose-fruticose species lacking secondary metabolites and containing antraquinones are more abundant on bark with a higher pH and containing higher concentrations of flavonoids and phenolic compounds. Species containing most of major metabolites are common on a conifer and birch bark with a lower pH and containing more terpenes. Many phenolic compounds and flavonoids are chemically similar to many lichen metabolites in having hydroxyl groups which bear a high affinity to metals, iron and copper (Přemysl et al., 2011; Říha et al., 2014), likewise the group of secondary lichen metabolites. The complex formation is the most active at higher pH (Mira et al., 2002), at the levels, which were found in alder, poplar, and aspen. Higher pH conditions reduce the availability of Fe, Cu (Lucas and Davis, 1961) and a complex formation may increase a bioavailability of these elements. Phenolic compounds have a similar effect as chelators of transition metals and have an ability to quenching of free radical reactions (Foti, 2007; Kulbat, 2016). Thus, the presence of phenols and flavonoids in bark can be a substitution for lichen metabolites such as melanins, lecanoric, and usnic acid (Solhaug and Gauslaa, 1996; Luo et al., 2009; Prokopiev et al., 2018) in prevention thalli from a damage from reactive oxygen species under high UV-B levels. A close contact of crustose epiphytes with their substrates and a variability in an amount of phenols and flavonoids in bark may be a reason for a lower dependence of a secondary chemistry of crustose species on bark pH. Terpenes are used by plants in many ways, including physiological regulation and defense responses (Tholl, 2015). Eighteen species of epiphytic lichens contained terpene zeorin, and fourteen compounds were additionally found in bark. Both in crustose and foliose-fruticose groups species containing zeorin were the least abundant on birch as the most terpene-rich substrate. In lichens zeorin acts as an antiherbivorous substance (Nimis and Skert, 2006; Asplund and Gauslaa, 2010) and other terpenes contained in bark may have a similar protective action on thalli.
Our results show that the secondary chemistry of saxicolous and epiphytic lichens reflects differences of substrate pH in a similar way but the variability of metabolites and distribution of species is refined by a different set of additional parameters, such as metal availability and a presence of organic compounds in bark. This is not the only factor determining distribution of lichens on phorophytes as far as other functional traits like photobiont type, ascomata type and asexual reproduction of mycobionts affect the affinity of lichens to tree species (Łubek et al., 2021). Studies using genera with a variable chemistry and their distribution on different species of trees as well as direct application of bark metabolites on lichens will give additional confirmation on the effect of lichen chemistry as one of the important traits in the selection of substrate by lichen species.
Data Availability Statement
The original contributions presented in the study are included in the article/supplementary material, further inquiries can be directed to the corresponding author/s.
Author Contributions
AP: conceptualization and methodology. AP, AT, AE, EK, and LS: investigation. AP and AT: writing. All authors contributed to the article and approved the submitted version.
Funding
AE was partially supported by the Russian Science Foundation, grant number 22-24-00817.
Conflict of Interest
The authors declare that the research was conducted in the absence of any commercial or financial relationships that could be construed as a potential conflict of interest.
Publisher’s Note
All claims expressed in this article are solely those of the authors and do not necessarily represent those of their affiliated organizations, or those of the publisher, the editors and the reviewers. Any product that may be evaluated in this article, or claim that may be made by its manufacturer, is not guaranteed or endorsed by the publisher.
Acknowledgments
AP and AT are grateful for “Reka Chusovaya” national park and personally for Alexandra Khlopotova and Mikhail Shershnev for their comprehensive assistance and help in the field work. We thank Irina Kiselyova, the head of the department of Experimental Biology and Biotechnology, Ural Federal University for a fruitful discussion. We are grateful to reviewers whose meticolous reading and following recommendations have greatly improved the text.
References
Antonenko, Y. N., Khailova, L. S., Rokitskaya, T. I., Nosikova, E. S., Nazarov, P. A., Luzina, O. A., et al. (2019). Mechanism of action of an old antibiotic revisited: Role of calcium ions in protonophoric activity of usnic acid. Biochim. Biophys. Acta Bioenerg. 1860, 310–316. doi: 10.1016/j.bbabio.2019.01.005
Asplund, J., and Gauslaa, Y. (2010). The gastropod Arion fuscus prefers cyanobacterial to green algal parts of the tripartite lichen Nephroma arcticum due to low chemical defense. Lichenologist 42, 113–117. doi: 10.1017/S0024282909990284
Asplund, J., Ohlson, M., and Gauslaa, Y. (2015). Tree species shape the elemental composition in the lichen Hypogymnia physodes transplanted to pairs of spruce and beech trunks. Fungal Ecol. 16, 1–5. doi: 10.1016/j.funeco.2015.03.006
Asplund, J., Siegenthaler, A., and Gauslaa, Y. (2017). Simulated global warming increases usnic acid but reduces perlatolic acid in the mat-forming terricolous lichen Cladonia stellaris. Lichenologist 49, 269–274. doi: 10.1017/S0024282917000159
Bačkor, M., Gaburjáková, J., Hudák, J., and Ziegler, W. (1997). The biological role of secondary metabolites from lichens. 1. The influence of usnic acid on bimolecular lipid membranes. Acta Fac. Rerum Nat. Univ. Comen. 29, 67–71.
Bates, J. W., and Brown, D. H. (1981). Epiphyte differentiation between Quercus petraea and Fraxinus excelsior trees in a maritime area of South West England. Vegetatio 48, 61–70. doi: 10.1007/BF00117362
Beckett, R. P., Minibayeva, F., Solhaug, K. A., and Roach, T. (2021). Photoprotection in lichens: adaptations of photobionts to high light. Lichenologist 53, 21–33. doi: 10.1017/S0024282920000535
Culberson, C. F., and Kristinsson, H. D. (1970). A standardized method for the identification of lichen products. J. Chromatogr. 46, 85–93. doi: 10.1016/S0021-9673(00)83967-9
Elix, J. A. (2014). A Catalogue of Standardized Chromatographic Data and Biosynthetic Relationships for Lichen Substances, 3rd Edn. Canberra: Elix, J. A.
Elix, J. A., and Stocker-Wörgötter, E. (2008). “Biochemistry and secondary metabolites,” in Lichen Biology, 2nd Edn, ed. T. H. Nash (Cambridge, MA: Cambridge University Press), 104–133. doi: 10.1016/s1360-1385(00)01741-6
Ermoshin, A. A., Kiseleva, I. S., Nikkonen, I. V., Nsengiumva, D. S., Duan, S., Ma, C., et al. (2021). Antioxidant activity and chemical composition of extracts from fruiting bodies of xylotrophic fungi growing on birch. J. Sib. Fed. Univ. Biol. 14, 339–353. doi: 10.17516/1997-1389-0354
Favero-Longo, S. E., Isocrono, D., and Piervittori, R. (2004). Lichens and ultramafic rocks: a review. Lichenologist 36, 391–404.
Favero-Longo, S. E., Matteucci, E., Morando, M., Rolfo, F., Harris, T. B., and Piervittori, R. (2015). Metals and secondary metabolites in saxicolous lichen communities on ultramafic and non-ultramafic rocks of the Western Italian Alps. Aust. J. Bot. 63, 276–291. doi: 10.1071/BT14256
Foti, M. C. (2007). Antioxidant properties of phenols. J. Pharm. Pharmacol. 59, 1673–1685. doi: 10.1211/jpp.59.12.0010
Giordano, S., Basile, A., Lanzetta, R., Corsaro, M. M., Spagnuolo, V., and Castaldo, R. C. (1997). Potential allelochemicals from the lichen Cladonia foliacea and their in vitro effects on the development of mosses. Allelopathy J. 4, 89–100.
Goga, M., Elečko, J., Marcinčinová, M., Ručová, D., Bačkorová, M., and Bačkor, M. (2018). “Lichen metabolites: an overview of some secondary metabolites and their biological potential,” in Co-Evolution of Secondary Metabolites, Reference Series in Phytochemistry, eds J.-M. Mérillon and K. G. Ramawat (Berlin: Springer Nature), 1–36. doi: 10.1007/978-3-319-76887-8_57-1
Hammer, Ø, Harper, D. A. T., and Ryan, P. D. (2001). PAST: paleontological statistics software package for education and data analysis. Palaeont. Electr. 4, 1–9.
Hauck, M., and Huneck, S. (2007). Lichen substances affect metal absorption in Hypogymnia physodes. J. Chem. Ecol. 33, 219–223. doi: 10.1007/s10886-006-9225-6
Hauck, M., and Jürgens, S. R. (2008). Usnic acid controls the acidity tolerance of lichens. Environ. Pollut. 156, 115–122. doi: 10.1016/j.envpol.2007.12.033
Hauck, M., Jürgens, S.-R., Huneck, S., and Leuschner, C. (2009a). High acidity tolerance in lichens with fumarprotocetraric, perlatolic or thamnolic acids is correlated with low pKa1 values of these lichen substances. Environ. Pollut. 157, 2776–2780. doi: 10.1016/j.envpol.2009.04.022
Hauck, M., Jürgens, S.-R., Willenbruch, K., Huneck, S., and Leuschner, C. (2009b). Dissociation and metal-binding characteristics of yellow lichen substances suggest a relationship with site preferences of lichens. Ann. Bot. 103, 13–22. doi: 10.1093/aob/mcn202
Hauck, M., Willenbruch, K., and Leuschner, C. (2009c). Lichen substances prevent lichens from nutrient deficiency. J. Chem. Ecol. 35, 71–73. doi: 10.1007/s10886-008-9584-2
Hauck, M., Jürgens, S. R., and Leuschner, C. (2010). Norstictic acid: correlations between its physico-chemical characteristics and ecological preferences of lichens producing this depsidone. Environ. Exp. Bot. 68, 309–313. doi: 10.1016/j.envexpbot.2010.01.003
Kricke, R. (2002). “Measuring bark pH,” in Monitoring with Lichens – Monitoring Lichens, Nato Science Series. IV. Earth and Environmental Sciences, eds P. L. Nimis, C. Scheidegger, and P. A. Wolseley (Alphen aan den Rijn: Kluwer Academic Publishers), 333–336. doi: 10.1007/978-94-010-0423-7_30
Kulbat, K. (2016). The role of phenolic compounds in plant resistance. Biotechnol. Food Sci. 80, 97–108.
Lafferty, D., Bungartz, F., and Elix, J. A. (2021). Wintab 64bit – a Program Developed at Arizona State University for the Analysis of Secondary Metabolites of Lichens, based on an Original Concept Published by E. Mietzsch, H. T. Lumbsch & J. A. Elix. Help & Resources for the Consortium of Lichen Herbaria. Available online at: https://help.lichenportal.org/index.php/en/resources/metabolites/ (accessed June 12, 2021).
Larayetan, R., Ololade, Z. S., Ogunmola, O. O., and Ladokun, A. (2019). Phytochemical constituents, antioxidant, cytotoxicity, antimicrobial, antitrypanosomal, and antimalarial potentials of the crude extracts of Callistemon citrinus. Evid. Based Complement. Altern. Med. 2019:5410923. doi: 10.1155/2019/5410923
Latkowska, E., Chrapusta, E., Bober, B., Kaminski, A., Adamski, M., and Bialczyk, J. (2015). Allelopathic effects of epiphytic lichen Hypogymnia physodes (L.) Nyl. colonization on the spruce (Picea abies (L.) Karst.) bark. Allelopathy J. 35, 129–138.
Lawrey, J. D. (1986). Biological role of lichen substances. Bryologist 89, 111–122. doi: 10.2307/3242751
Legouin, B., Lohézic-Le Dévéhat, F., Ferron, S., Rouaud, I., Le Pogam, P., Cornevin, L., et al. (2017). Specialized metabolites of the lichen Vulpicida pinastri act as photoprotective agents. Molecules 22:1162. doi: 10.3390/molecules22071162
Łubek, A., Kukwa, M., Jaroszewicz, B., and Czortek, P. (2021). Composition and specialization of the lichen functional traits in a primeval forest – does ecosystem organization level matter? Forests 12:485. doi: 10.3390/f12040485
Lucas, R. E., and Davis, J. F. (1961). Relationships between pH values of organic soils and availabilities of 12 plant nutrients. Soil Sci. 92, 177–182. doi: 10.1097/00010694-196109000-00005
Luo, H., Yamamoto, Y., Kim, J. A., Jung, J. S., Koh, Y. J., and Hur, J.-S. (2009). Lecanoric acid, a secondary lichen substance with antioxidant properties from Umbilicaria antarctica in maritime Antarctica (King George Island). Polar Biol. 32, 1033–1040. doi: 10.1007/s00300-009-0602-9
Lutsak, T., Fernández-Mendoza, F., Nadyeina, O., Şenkardeşler, A., and Printzen, C. (2017). Testing the correlation between norstictic acid content and species evolution in the Cetraria aculeata group in Europe. Lichenologist 49, 39–56. doi: 10.1017/S0024282916000566
Mira, L., Fernandez, M. T., Santos, M., Rocha, R., Florêncio, M. H., and Jennings, K. R. (2002). Interactions of flavonoids with iron and copper ions: a mechanism for their antioxidant activity. Free Radic. Res. 36, 1199–1208. doi: 10.1080/1071576021000016463
Nimis, P. L., and Skert, N. (2006). Lichen chemistry and selective grazing by the coleopteran Lasioderma serricorne. Environ. Exp. Bot. 55, 175–182. doi: 10.1016/j.envexpbot.2004.10.011
Ogureeva, G. N., Leonova, N. B., Buldakova, E. V., Kadetov, N. G., Arkhipova, M. V., Miklyaeva, I. M., et al. (2018). The Biomes of Russia—Map. Moscow: Faculty of Geography Lomonosov Moscow State University.
Orange, A., James, P. W., and White, F. J. (2001). Microchemical Methods for the Identification of Lichens. London: British Lichen Society.
Parzych, A., Mochnacký, S., Sobisz, Z., Kurhaluk, N., and Polláková, N. (2017). Accumulation of heavy metals in needles and bark of Pinus species. Folia. Pol. Ser. A 59, 34–44. doi: 10.1515/ffp-2017-0004
Paukov, A., Teptina, A., Morozova, M., Kruglova, E., Favero-Longo, S. E., Bishop, C., et al. (2019). The effects of edaphic and climatic factors on secondary lichen chemistry: a case study using saxicolous lichens. Diversity 11:94. doi: 10.3390/D11060094
Paukov, A. G. (2003). “Lichens,” in Plants and Fungi of “Pripyshminskiye Bory” Nature Park, ed. V. A. Mukhin (Ekaterinburg: Ekaterinburg Publishing House), 86–92.
Paukov, A. G., and Teptina, A. Y. (2013). Lichens of “Olenji Ruchji” Nature Park (Sverdlovsk Region). Novosti Sist. Nizsh. Rast. 47, 237–252. doi: 10.31111/nsnr/2013.47.237
Pennino, M. G., Paradinas, I., Illian, J. B., Muñoz, F., Bellido, J. M., López-Quílez, A., et al. (2019). Accounting for preferential sampling in species distribution models. Ecol. Evol. 9, 653–663. doi: 10.1002/ece3.4789
Phinney, N. H., Gauslaa, Y., and Solhaug, K. A. (2019). Why chartreuse? The pigment vulpinic acid screens blue light in the lichen Letharia vulpina. Planta 249, 709–718. doi: 10.1007/s00425-018-3034-3
Přemysl, M., Macáková, K., Filipský, T., Zatloukalová, L., Jahodář, L., Bovicelli, P., et al. (2011). In vitro analysis of iron chelating activity of flavonoids. J. Inorg. Biochem. 105, 693–701. doi: 10.1016/j.jinorgbio.2011.02.003
Prokopiev, I. A., Poryadina, L. N., Konoreva, L. A., Chesnokov, S. V., and Shavarda, A. L. (2018). Variation in the composition of secondary metabolites in Flavocetraria lichens from Western Siberia. Russ. J. Ecol. 49, 401–405. doi: 10.1134/S1067413618050107
Purvis, O. W., Chimonides, P. J., Jefferies, T. E., Jones, G. C., Read, H., and Spiro, B. (2005). Investigating biogeochemical signatures in the lichen Parmelia sulcata at Burnham Beeches, Buckinghamshire, England. Lichenologist 37, 329–344. doi: 10.1017/S0024282905015288
Purvis, O. W., Elix, J. A., Broomhead, J. A., and Jones, G. C. (1987). The occurrence of copper norstictic acid in lichens from cupriferous substrata. Lichenologist 19, 193–203. doi: 10.1017/S0024282987000161
Purvis, O. W., Elix, J. A., and Gaul, K. L. (1990). The occurrence of copper-psoromic acid in lichens from cupriferous substrata. Lichenologist 22, 345–354. doi: 10.1017/S002428299000038X
Purvis, O. W., and Halls, C. (1996). A review of lichens in metal-enriched environments. Lichenologist 28, 571–601. doi: 10.1006/lich.1996.0052
Rajakaruna, N., Knudsen, K., Fryday, A. M., O’Dell, R. E., Pope, N., Olday, F. C., et al. (2012). Investigation of the importance of rock chemistry for saxicolous lichen communities of the New Idria serpentinite mass, San Benito County, California, USA. Lichenologist 44, 695–714. doi: 10.1017/S0024282912000205
Říha, M., Karlíčková, J., Filipský, T., Macáková, K., Rocha, L., Bovicelli, P., et al. (2014). In vitro evaluation of copper-chelating properties of flavonoids. RSC Adv. 4, 32628–32638. doi: 10.1039/C4RA04575K
Shaikh, J. R., and Patil, M. K. (2020). Qualitative tests for preliminary phytochemical screening: an overview. Int. J. Chem. Stud. 8, 603–608. doi: 10.22271/chemi.2020.v8.i2i.8834
Skye, E. (1968). Lichens and air pollution. A study of cryptogamic epiphytes and environment in the Stockholm region. Acta Phytogeogr. Suecica 52, 1–123.
Šmilauer, P., and Lepš, J. (2002). Multivariate Analysis of Ecological Data Using CANOCO 5. Cambridge, MA: Cambridge University Press.
Solhaug, K. A., and Gauslaa, Y. (1996). Parietin, a photoprotective secondary product of the lichen Xanthoria parietina. Oecologia 108, 412–418. doi: 10.1007/BF00333715
Sorescu, A.-A., Nuta, A., Ion, R.-M., and Iancu, L. (2018). “Qualitative analysis of phytochemicals from sea buckthorn and gooseberry,” in Phytochemicals – Source of Antioxidants and Role in Disease Prevention, ed. T. Asao (Stoke-on-Trent: Md Asaduzzaman), doi: 10.5772/intechopen.77365
Spier, L., and Aptroot, A. (2005). What is the role of secondary lichen substances in rock-inhabiting crustose lichens? Bull. Br. Lichen Soc. 96, 24–25.
Stocker-Wörgötter, E. (2008). Metabolic diversity of lichen-forming ascomycetous fungi: culturing, polyketide and shikimate metabolite production, and PKS genes. Nat. Prod. Rep. 25, 188–200. doi: 10.1039/b606983p
Tholl, D. (2015). Biosynthesis and biological functions of terpenoids in plants. Adv. Biochem. Eng. Biotechnol. 148, 63–106. doi: 10.1007/10_2014_295
van Dobben, H. F., Wolterbeek, H. T., Wamelink, G. W. W., and Ter Braak, C. J. F. (2001). Relationship between epiphytic lichens, trace elements and gaseous atmospheric pollutants. Environ. Pollut. 112, 163–169. doi: 10.1016/S0269-7491(00)00121-4
Vassiliyeva, L. N. (1959). “Study of mushrooms (macromycetes) as the components of plant communities,” in Field Geobotany, eds E. M. Lavrenko and A. A. Korchagin (Moscow: Academy of Sciences of the USSR Press), 387–398.
Keywords: substrate ecology, phorophyte, flavonoids, terpenes, Middle Urals, CCA analysis
Citation: Paukov A, Teptina A, Ermoshin A, Kruglova E and Shabardina L (2022) The Role of Secondary Metabolites and Bark Chemistry in Shaping Diversity and Abundance of Epiphytic Lichens. Front. For. Glob. Change 5:828211. doi: 10.3389/ffgc.2022.828211
Received: 03 December 2021; Accepted: 26 April 2022;
Published: 13 May 2022.
Edited by:
Ángel Benítez, Universidad Técnica Particular de Loja, EcuadorReviewed by:
Dariusz Kubiak, University of Warmia and Mazury in Olsztyn, PolandPaolo Giordani, University of Genoa, Italy
Copyright © 2022 Paukov, Teptina, Ermoshin, Kruglova and Shabardina. This is an open-access article distributed under the terms of the Creative Commons Attribution License (CC BY). The use, distribution or reproduction in other forums is permitted, provided the original author(s) and the copyright owner(s) are credited and that the original publication in this journal is cited, in accordance with accepted academic practice. No use, distribution or reproduction is permitted which does not comply with these terms.
*Correspondence: Alexander Paukov, alexander.paukov@urfu.ru
†ORCID: Lada Shabardina, orcid.org/0000-0002-8284-0008