- 1Geography, College of Life and Environmental Sciences, University of Exeter, Exeter, United Kingdom
- 2Physics Institute, Fluminense Federal University, Niteroi, Brazil
- 3NEIF Radiocarbon Laboratory, Scottish Universities Environmental Research Centre, East Kilbride, United Kingdom
- 4Estación Biológica Cocha Cashu, Administrador General, San Diego Zoo Global—Perú, Cusco, Peru
- 5School of Geography and Sustainable Development, University of St Andrews, St Andrews, United Kingdom
- 6Remote Sensing Lab, School of Earth, Environment and Society, McMaster University, Hamilton, ON, Canada
- 7Geography, University of Leeds, Leeds, United Kingdom
- 8Scottish Universities Environmental Research Centre (SUERC), University of Glasgow, East Kilbride, United Kingdom
Fire has a historical role in tropical forests related to past climate and ancient land use spanning the Holocene; however, it is unclear from charcoal records how fire varied at different spatiotemporal scales and what sampling strategies are required to determine fire history and their effects. We evaluated fire variation in structurally intact, terra-firme Amazon forests, by intensive soil charcoal sampling from three replicate soil pits in sites in Guyana and northern and southern Peru. We used radiocarbon (14C) measurement to assess (1) locally, how the timing of fires represented in our sample varied across the surface of forest plots and with soil depth, (2) basin-wide, how the age of fires varies across climate and environmental gradients, and (3) how many samples are appropriate when applying the 14C approach to assess the date of last fire. Considering all 14C dates (n = 33), the most recent fires occurred at a similar time at each of the three sites (median ages: 728–851 cal years BP), indicating that in terms of fire disturbance at least, these forests could be considered old-growth. The number of unique fire events ranged from 1 to 4 per pit and from 4 to 6 per site. Based upon our sampling strategy, the N-Peru site—with the highest annual precipitation—had the most fire events. Median fire return intervals varied from 455 to 2,950 cal years BP among sites. Based on available dates, at least three samples (1 from the top of each of 3 pits) are required for the sampling to have a reasonable likelihood of capturing the most recent fire for forests with no history of a recent fire. The maximum fire return interval for two sites was shorter than the time since the last fire, suggesting that over the past ∼800 years these forests have undergone a longer fire-free period than the past 2,000–3,500 years. Our analysis from terra-firme forest soils helps to improve understanding of changes in fire regime, information necessary to evaluate post-fire legacies on modern vegetation and soil and to calibrate models to predict forest response to fire under climate change.
Introduction
Fire is one of the oldest drivers of change in tropical biomes since the rise of sufficient atmospheric oxygen, plant biomass, and ignition sources. The earliest fires that charred stomata-bearing plants date back to the Silurian (440 mya) (Glasspool et al., 2004). Since at least the rise of flammable C4 grasses over the past 4–10 million years (Simon et al., 2009), fire has been a reoccurring feature of tropical savannas (Pivello, 2011), which occur in the most seasonal regions of Amazonia, and where plants have developed growth and anatomical adaptations to fire (Simon and Pennington, 2012). For humid tropical forests, fire history and its impact on biodiversity and carbon storage are less clear. With high annual precipitation and low intra-annual variability, continuous closed canopies that shade understories, rapid decomposition, and few sources of ignition, humid tropical forests have limited conditions for fire ignition and spread. Therefore, they have historically been considered mostly fire-free or fire-resistant systems (Sanford et al., 1985; Hammond and ter Steege, 1998). Understanding fire history and long-term impacts of fire on vegetation and soil are important to estimate drivers of changes in carbon stocks and forest composition, and response to changing climate and fire regimes.
Fires over the past several decades in Amazonia have been widespread and increasing in frequency due to a combination of deforestation, fragmentation, recurrent drought, and human ignition (Aragão et al., 2007). During the 2015/2016 El Niño event with record-breaking drought and temperature anomalies, wet forests in central Amazonia burned (Pontes-Lopes et al., 2021), highlighting how changing climate and land use are interacting to allow forests once considered fire-resistant to burn. Over the past decade, deforestation and burning have resulted in a loss of 71,000 km2 of forest in Amazonia (INPE, 2020). Fire associated with deforestation often spreads into surrounding forests, especially fragmented forests already degraded by selective logging (Cochrane and Laurance, 2002). With fire now widespread in many humid tropical forests, it is unclear how current fire occurrence compares to historical fire regimes and what sampling approaches can be used to understand variability in fire in time and space.
Understanding the fire history of Amazon forests is crucial to estimate how fire has affected soil organic carbon (SOC) pools and forest composition and rates of biomass accumulation following fire. During fire, pyrogenic carbon (PyC) is formed by the incomplete combustion of plant biomass (Bird et al., 2015). Unlike more available SOC such as particulate and humic fractions, PyC is highly resistant to decomposition, with turnover times of centuries to millennia (Glaser et al., 2002; Bird et al., 2015). The most extreme case of PyC enrichment in soil can be found in Anthropogenic Dark Earth (Anthrosols, terra preta) formed by charcoal additions to small-scale agricultural plots from 20 to 350 ha by native Americans in Amazonia (Glaser et al., 2001). In addition to charcoal inputs into terra preta areas, pre-Columbian use of fire may have been associated with other small-scale swidden management and from local campfires. In non-terra preta forests in Amazonia, ancient fires have led to the development of PyC stocks of 2.76 Pg over 0–100 cm soil depth (Koele et al., 2017). Modern conditions are likely to have led to larger and more intense fires in Amazonia than in pre-Columbian times due to interactions between large-scale forest fragmentation through mechanized tree-felling and drought (Aragão et al., 2007).
Following fire, humid Amazon forests can remain a net C source of above-ground C for decades (Silva et al., 2020) and in southeastern Amazonia, the response of forest to fire varies among terra firme and riparian forests (Nogueira et al., 2019). Whether fire can continue to have residual effects on forests over much longer time scales is unclear (Massi et al., 2017). Structurally mature African and Amazon Basin tropical forests have provided a substantial C sink since at least the late twentieth century (Phillips et al., 1998; Lewis et al., 2009; Feldpausch et al., 2016), but the net uptake of C by some Amazon forests has been declining (Brienen et al., 2015; Hubau et al., 2020). Changes in climate and CO2 fertilization could change the rate of C uptake. There is also debate as to whether apparently old-growth forests are recovering from past disturbances such as blow-downs, drought, fire, and pre-Colombian land-use (Gloor et al., 2009; Dull et al., 2010; Espirito-Santo et al., 2014; McMichael et al., 2017; Oliveira et al., 2020; Bush et al., 2021). If apparently old-growth forests are regrowing from fire or other potentially stand-initiating disturbances, then the estimated effects of other drivers such as CO2 fertilization or changes in temperature on forests may be incorrect. Therefore, determining the drivers of change in forest C, including potential regrowth from past fire, is important to predict the longevity of the C sink.
To improve estimates of PyC turnover times and to estimate legacies of ancient fire on contemporary vegetation, a prerequisite is better understanding of time lapsed since the last fire locally, and the fire return interval. Uncertainty in successfully identifying the date of the last fire will have varying levels of importance depending upon the temporal scales over which processes act; e.g., incorrectly identifying the date of the last fire by 100 years could have large effects on estimates of vegetation response to fire since above ground biomass C turnover times average ∼50 years (Galbraith et al., 2013), but would be relatively minor for estimates of PyC turnover since turnover times are orders of magnitude higher.
Long-term fire history and its relation to climate change, vegetation, and soils can be assessed by stratigraphy, anthracology, and radiocarbon dating of charcoal from soil, peat, and lake sediment. Peat and lake sediment samples can be used to develop well-constrained depth-age profiles for those systems but locations with long-term records are not uniformly distributed across Amazonia and so they do not fully represent fire history of forests on terra firme soil in regions where lakes and peat are uncommon (Bush et al., 2007). Charcoal radiocarbon (14C) dating from terra-firme soils provides more extensive opportunities to estimate fire history (Trumbore, 2009) and to link data to vegetation in permanent forest plots to understand potential legacy effects of historical fire on modern forests. However, the lack of well-preserved stratification and the effect of bioturbation makes it difficult to develop fire history records, such as the date of last fire and the fire return interval for an area.
Reported 14C dates of fire vary widely among sites and regions of Amazonia, with few assessments of charcoal in terra firme soils (Piperno and Becker, 1996; Hammond and ter Steege, 1998; McMichael et al., 2012). Understanding this variation and its impacts upon the record that is reflected in Amazonian soil stratigraphies is important for developing sampling and analysis strategies. The earliest fire regime analyses originate from lake cores (e.g., Bush et al., 2007). Many of the lakes in Amazonia, which are more frequent in western Amazonia, are ancient oxbow lakes. The stratigraphies of oxbow lakes can be affected by inputs of minerals and charcoal from distant sources (Clark, 1988), transported fluvially during seasonal flooding. This introduction of material that is not representative of local burning events can obscure the local charcoal record and complicate interpretations. Only recently has soil sampling combined with 14C dating of charcoal shown that tropical humid forests considered old-growth have a long and variable history of fire, with some forests considered old-growth having burned within the last 250 years (Sanford et al., 1985; Goulart et al., 2017; McMichael and Bush, 2019). In a recent compilation of > 850 14C dates for the Amazon region, 25% were sampled from charcoal in soil, with the rest from other sources (e.g., lakes, peat) (McMichael and Bush, 2019). Lowland terra firme soils are the main substrate for the Amazon Basin (Quesada et al., 2011), so the paucity of 14C dates from these soils limits our understanding of fire history and its effects on the forests that represent the majority of Amazonia. Intensively sampling and 14C dating macro charcoal (e.g., ∼ > 1 mm diameter) at the local scale (e.g., 1-hectare) for charcoal from the soil surface and distributed from mineral soil using a terrestrial macro-charcoal approach may be a useful tool to evaluate long-term variation in fire regime (Payette et al., 2012).
Here we use intensive locally replicated 14C dating of soil charcoal to study spatial and temporal variation in fire events in terra firme forests at local scales and across the Amazon Basin and to inform sampling and analytical approaches to determine fire history. Our study had the following main research questions: (1) Locally, how the timing of fires represented in our sample varied across the surface of forest plots and with soil depth (a potential proxy for time), (2) basin-wide, how the age of fires varies across climate and environmental gradients, and (3) how many samples are appropriate when applying the 14C approach to assess the date of last fire and fire chronology. Improving this understanding is essential to conserve and manage tropical forests. The results can inform future sampling and analyses strategies and determine long-term fire histories to better understand the legacy of fire in global tropical forests.
Materials and Methods
Study Sites
Charcoal was sampled from soil from three regions spanning Amazonia in Guyana, northern Peru (N-Peru) and southern Peru (S-Peru) in permanent forest plots with structurally intact vegetation (Feldpausch et al., 2012; Brienen et al., 2015; Figure 1). The sites are all terra-firme, non-seasonally flooded forests and are part of the RAINFOR network, with soil and vegetation data managed at ForestPlots.net (Lopez-Gonzalez et al., 2011; ForestPlots.net et al., 2021). The sites span a climatic and edaphic gradient with annual precipitation and soil fertility increasing in an east to west direction (Quesada et al., 2012; Feldpausch et al., 2016). None of the sites had Anthropogenic Dark Earth (terra preta).
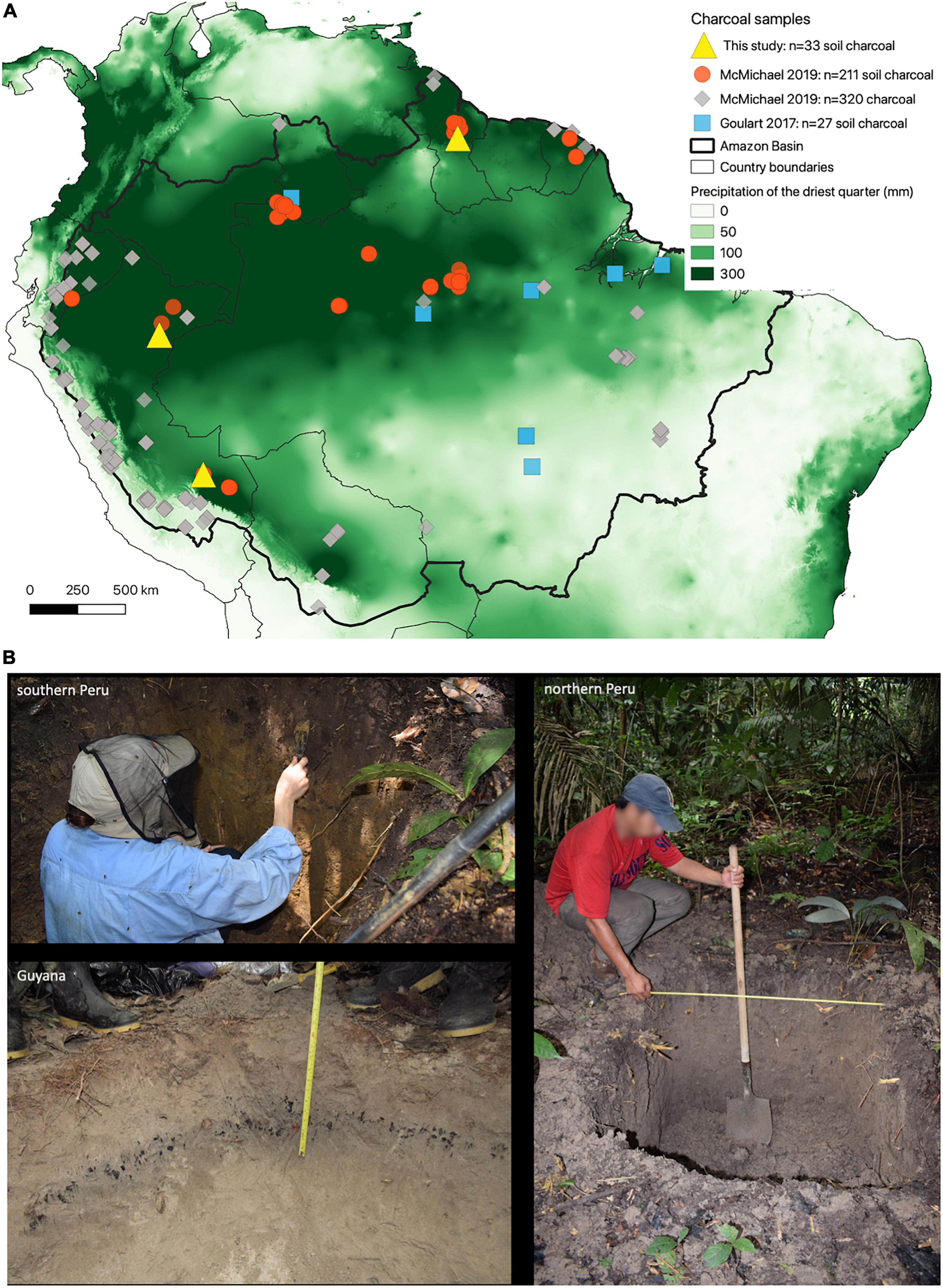
Figure 1. (A) Map showing locations of charcoal samples in Amazonia, and (B) charcoal sampling from terra firme soil pits in southern Peru, northern Peru, and Guyana (clockwise from top left; photo credit: L. Carvalho).
Guyana
The site in Guyana, coded in the RAINFOR network as plot PIB-05, is located at 5.02 latitude, –58.62 longitude in the upper Demerara-Barbice region in closed-canopy humid rainforest dominated by Chlorocardium rodiei (Lauraceae). The site is a control plot for a study assessing the effects of different levels of logging under reduced impact logging. Annual precipitation and temperature are 2,365 mm and 26.5°C, respectively, and the site has a mean annual maximum climatological water deficit (MCWD) of –92.6 mm (Feldpausch et al., 2016; Fick and Hijmans, 2017). The site is located on old, weathered soil from the Tertiary-Quaternary with a maximum geological age of 65 M years and elevation of 103 m above sea level (a.s.l.). Soils are nutrient-poor with low pH (4.03) and clay content (20%) and high sand content (76%) (Quesada et al., 2012).
Northern Peru
The N-Peru site, JEN-11 in RAINFOR, is located at –4.88 latitude and –73.63 longitude in a closed-canopy humid rainforest in the Loreto department. Vegetation is dominated by large trees of the families Lecythidaceae, Sapotaceae, and Chrysobalanaceae (Honorio Coronado et al., 2009). Large anthropogenic fires in the wider region have occurred since the mid-1960s when a colonization project of Peruvian Amazonia opened extended areas of terra firme forest for pastures and cattle raising but the site was not deforested nor affected by these. Annual precipitation and temperature are 2,584 mm and 26.5°C, respectively, and the site has a mean annual MCWD of –30.2 mm. The site is located on old, weathered soil from the Neogene with a maximum geological age of 23.5 M years and elevation of 136 m a.s.l. Soils are nutrient poor with low pH (3.98) and clay content (22%) and 44% sand content.
Southern Peru
The S-Peru site, BAR-01 in RAINFOR, is located at –11.90 latitude and –71.42 longitude in closed-canopy humid rainforest in the Manú National Park in the Madre de Dios department. Vegetation is dominated by Iriartea deltoidea (Arecaceae), one of the hyperdominant species found in Amazonia (ter Steege et al., 2013). The site is remote and uncontacted indigenous nomadic people sporadically access these areas. Annual precipitation and temperature are 2,562 mm and 25.4°C, respectively, and the site has a mean annual MCWD of –194 mm. The site is at an elevation of 345 m a.s.l. and is located on soils from the Quaternary (Dumont et al., 1990).
Charcoal Sampling and 14C Dating
Charcoal fragments were collected at each site in soil profiles from each of three replicate pits separated by 100 m. The pits were located just outside of the boundary of the 1 ha permanent forest plots. To sample macrocharcoal (∼ ≥ 1 mm), soil was carefully excavated in 1 cm depth increments from the pit wall up to 70 cm depth. Macroscopic charcoal was separated from the soil in the field, air-dried and stored in plastic Eppendorf tubes. This size class represents charcoal that would have been produced in situ by local fires (i.e., over hundreds of meters) and not transported to the site as aerosols (e.g., < 10 μm) (Echalar et al., 1998). Convective columns produced by fires could transport charcoal ≤ 200 μm for distances of up to ∼10 km, while larger particles are unlikely to be uplifted and transported (Clark, 1988). We applied the following criteria for 14C dating of four charcoal pieces from each pit by: (i) analyzing the sample nearest the surface from each pit, and (ii) splitting the soil profile into 5 cm depth increments and selecting the next three samples from progressively deeper depth intervals. This resulted in 3–4 samples per pit for a total of 11 samples from three replicate pits for each site. The N-Peru site includes one additional date from 46 cm depth from a fourth soil pit that represents the next depth increment of pit 3 to provide the same sample size across all sites. Samples were prepared for analysis at the Radiocarbon Facility at Universidade Federal Fluminense, Brazil by scraping soil from the surface of charcoal and were 14C dated using Accelerator Mass Spectroscopy (AMS) at the NERC Radiocarbon Facility. Samples were pre-treated at the NERC Facility, United Kingdom using standard acid-base-acid methods (0.5 M HCl at 80°C for 2 h, followed by 0.5 M KOH at 80°C for 2 h, then 0.5 M HCl at 80°C for 2 h). Samples were then combusted to CO2 in sealed quartz tubes before cryogenic purification and conversion to graphite using the Fe/Zn reduction method (Slota et al., 1987). The measured 14C ages (in 14C years BP) were calibrated to calendar years before present (cal yrs BP) using a mixing model of the SHCal20 (for the southern hemisphere; Hogg et al., 2020) and IntCal20 (for the northern hemisphere; Reimer et al., 2020) calibration curves using the OxCal statistical package, ver. 4.4 (Bronk Ramsey, 2009), allowing for tree growth to record anywhere between the two extremes of 100% NH air mass influence and 100% SH air mass influence. Whilst slightly decreasing precision, such an approach should increase the accuracy of the calibrated ages of samples that have grown in equatorial regions and which can experience the influence of both NH and SH air masses at different times throughout the year (Marsh et al., 2018).
Most Recent Fire, Unique Fire Events, and Age-Depth Relationships
To evaluate how 14C dates varied within and between sites, we evaluated differences in 14C dates: (i) By depth within a profile, (ii) among the three pits within a site, and (iii) between sites by region. Differences in 14C dates were assessed using a Chi-square test (χ2) with critical values for a p-value of 0.05 (95% confidence).
Determining the date of the last (most recent) fire is crucial to study fire legacies on modern forest dynamics and composition. We evaluated two hypothetical sample selection scenarios for radiocarbon dating from the 11 14C dated samples per site to assess 14C variability within and among soil pits and how sample selection could be optimized to reduce the number of samples and soil pits required and to minimize costs:
Sampling Strategy 1—Vertical– > Lateral: sampling first down a pit profile within the 5 cm depth intervals and then repeating for each pit for a total of three pits.
Sample Strategy 1—Lateral– > Vertical: sampling first surface samples across three pits and then repeating for the subsequent 5 cm depth interval.
To evaluate the effect of pit selection, we used a resampling approach for each of these strategies to simulate randomly starting sample selection from each of the three pits, i.e., sampling pit 1-->2-->3, 2-->3-->1, 3-->1-->2 progressing from the surface to deepest samples for each subsequent pit sampled. We then evaluated which approach required the fewest samples to identify the most recent fire (i.e., the sample that did not differ statistically from the overall most recent fire for the site). We assessed how many samples (sampling effort) were required to identify, from available dates, the total unique fire events in each site using a bootstrap approach with 500 random resamples selected for each site (Huang et al., 2019). Events were considered unique if they differed based on a Chi-square test (χ2). For the purposes of this analysis, we assume that at least one of our 11 samples from each site represents the most recent fire and acknowledge that it is always possible that additional sampling may reveal a more recent fire.
We compared our 14C dates to published values (Goulart et al., 2017; McMichael and Bush, 2019) to evaluate basin-scale trends in fire. These datasets separate charcoal sampled from soil vs. charcoal sampled from other substrates such as peat and lake cores. Age-depth relationships are important to understand bioturbation and to estimate the potential dates of fires represented in undated charcoal. For each pit, site, and among regions, we evaluated the age-depth relationships using linear regression. Calibrated dates were plotted using the rcarbon package (Crema and Bevan, 2021) and statistical analyses were performed using the R statistical platform (R Core Team, 2021).
Results
Amazon Basin-Scale Variation in 14C Dates
Across all pits in sites in Guyana, S-Peru and N-Peru crossing Amazon Basin-scale climate and environmental gradients, the 33 dates for fires spanned 11,513 years for the soil depths where charcoal was found (minimum = 15 cm; max = 70 cm, median = 30 cm depth) (Table 1). The probability distribution of all calibrated ages showed the two largest peaks occurred at ∼1,000 and 2,600 cal yrs BP (Figure 2). Older fires occurred at ∼7,000 and 11,500 cal years BP. The most recent fires occurred at a similar time across the three regions, at 728, 842, and 851 cal years BP for S-Peru, N-Peru, and Guyana, respectively.
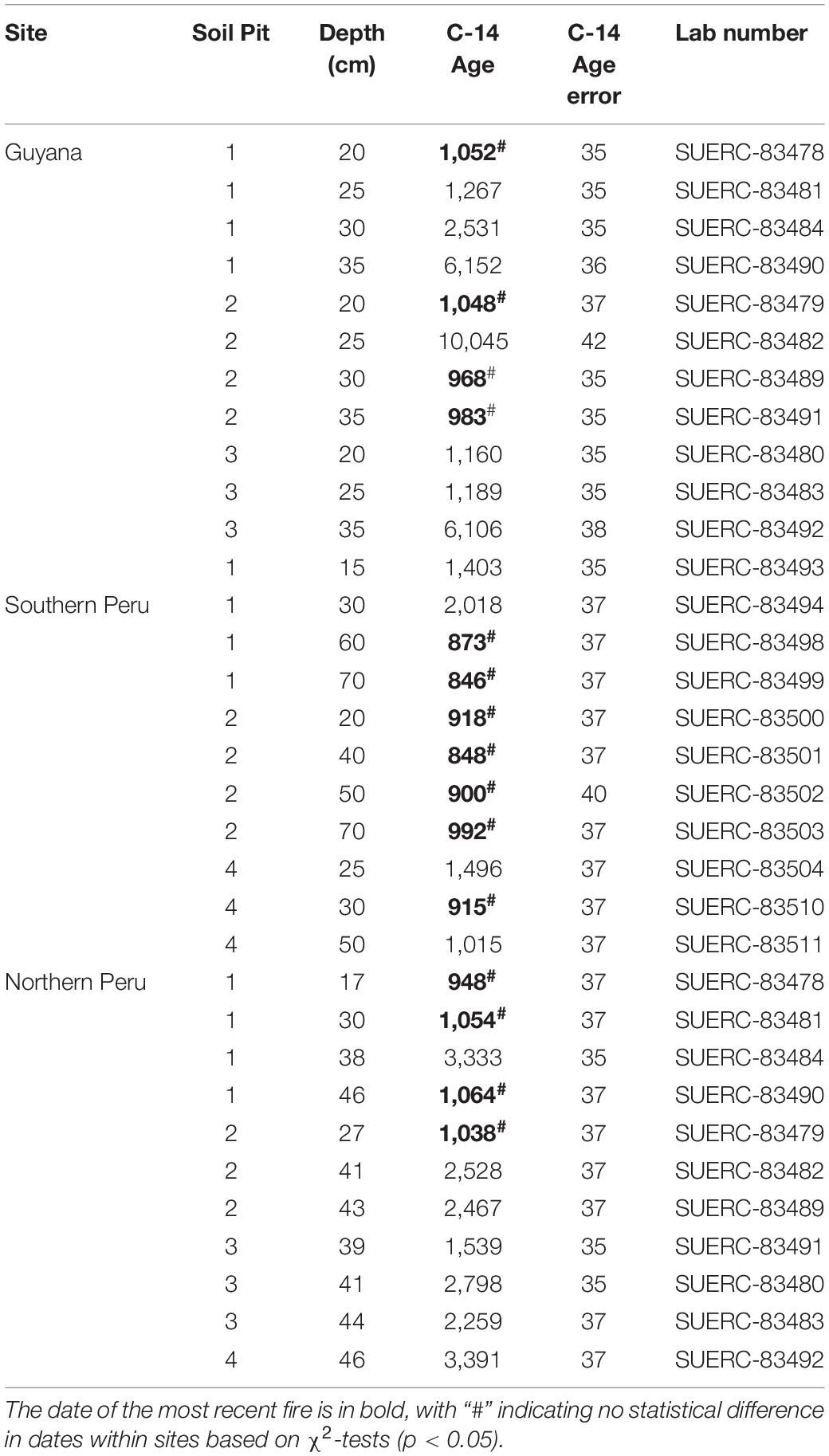
Table 1. Radiocarbon dates for charcoal sampled from terra firme soil in structurally intact forest permanent plots in Guyana, southern Peru, and northern Peru.
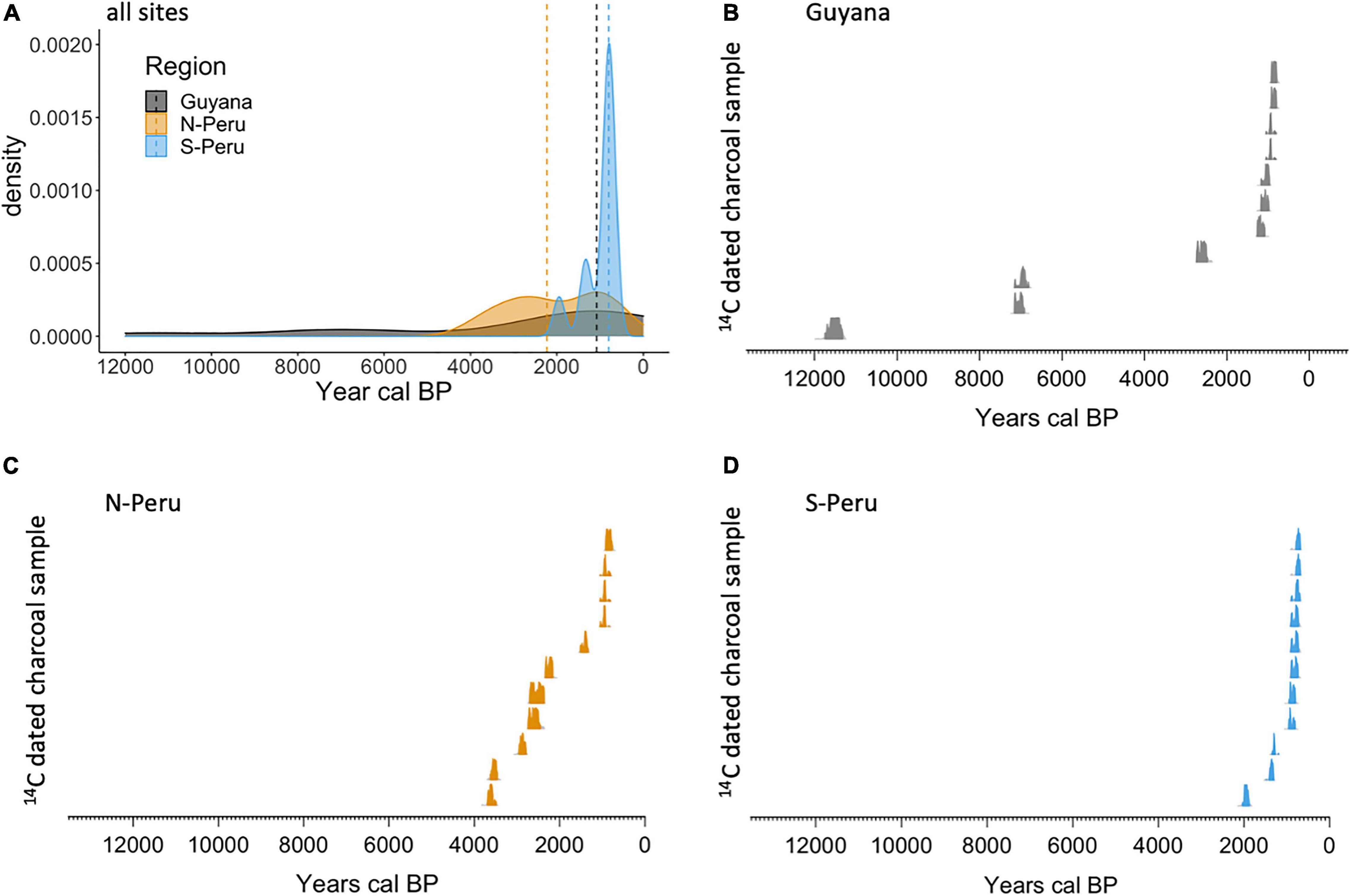
Figure 2. (A) Density distribution for dates (n = 33; cal years BP) by region for charcoal sampled in soil pits in Guyana, N-Peru and S-Peru. Dashed lines indicate median dates among all dates per region. Calibrated 14C dates (cal years BP) for (B) Guyana, (C) northern Peru, and (D) southern Peru; each curve represents a charcoal sample.
Local Lateral and Vertical Variation in 14C Dates
The dates of fire for the three pits at the Guyana site varied between 11,513 and 851 cal years BP, with all three pits exhibiting no statistically significant difference in the 14C date of the most recent fire (p < 0.05; Table 2 and Figure 2). The oldest dates were statistically indistinguishable for two pits, with the oldest date for the third pit ∼4,500 cal years older.

Table 2. Summary of calibrated radiocarbon dates, maximum fire return interval, and the minimum number of unique fire events by pit based on X2 tests for sites in Guyana, northern Peru, and southern Peru.
The S-Peru site had dates that varied between 1,944 and 728 cal yrs BP among the three pits. As with the Guyana site, the three S-Peru pits all had statistically indistinguishable dates for the most recent fire. The oldest pit date varied between 1,944 and 858 cal years BP (p < 0.05).
The 14C dates of fire at the N-Peru site varied between 3,606 and 842 cal years BP among the three pits. Two of the pits gave statistically indistinguishable dates for the most recent fire (p < 0.05), but the most recent fire in the third pit was ∼500 years older. The oldest date was statistically indistinguishable for two pits and ∼1,000 years younger in the third pit.
Unique Fire Events
Across all sites and based on the three to four 14C dates in n = 3 replicate pits, the minimum number of unique fire events ranged from one to four per pit and four to six per site (χ2 analysis, Table 2). Within our dataset and sampling depth, the number of cumulative fire events increased slowly with the number of samples (sampling effort) for Guyana and S-Peru. For N-Peru, there was a greater increase in unique fire events with the number of samples (Figure 3). Given the available evidence (14C data), we cannot prove that sites had more fires than this minimum number, and so our subsequent analyses are based upon the quantification that this is only the minimum number of unique fire events. The site from N-Peru, which apparently had the most fire events, also has the highest annual precipitation. The S-Peru site, which had the fewest unique fire events, has only slightly lower annual precipitation but is more seasonal, with greater climatological water deficits during the dry season.
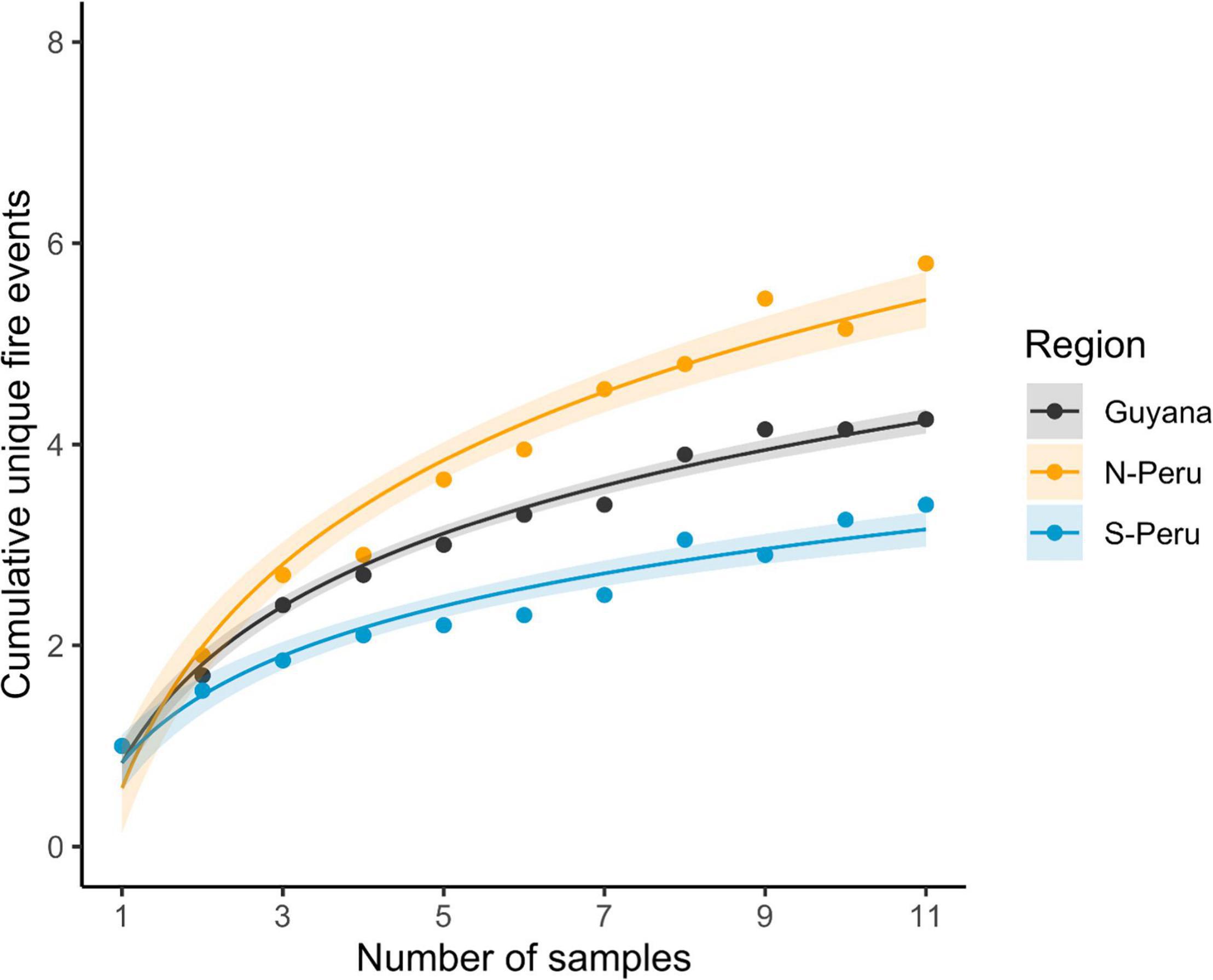
Figure 3. Cumulative number of minimum unique fire events and the number of samples (sampling effort) based on a bootstrap resampling approach from samples from the three pits for each site. The curve represents log-transformed fits and shading represents 95% confidence intervals.
Number of Dates to Estimate the Date of Last Fire
We evaluated two sampling and analysis strategies to evaluate the likelihood of estimating the date of the most recent fire for each site: Strategy (1) Vertical–Lateral: sampling first down a pit profile and then repeating for each pit and Strategy (2) Lateral-Vertical: sampling first surface samples across pits and then repeating for the subsequent sample depth across pits, and for both resampling by starting from each of pits 1, 2, and 3. Under Strategy 1, considering all dates from individual pits from sites, from 3–5 samples (variation representing random resampling from different pits) were required to estimate the most recent fire, i.e., the most recent 14C date not being statistically different from the overall most recent date for the site based on X2 tests. Under Strategy 2, only 2–3 samples were required to estimate the most recent fire, showing this strategy to be most effective by requiring fewer samples and therefore a lower cost.
From the available dates, we also consider the probability for each site of observing the most recent fire, from available dates for each site, based on the number of samples. Under Strategy 1 for Guyana, the probability was 67% when randomly sampling just 1 sample from the available set of measurements and 100% with 4 samples, i.e., up to 4 samples would need to be taken from the depth intervals down the vertical profile (Strategy 1) from any of the 3 pits to sample the most recent fire (100% probability) while if only 1 sample were taken from any of the pits, 2 of the 3 of the samples (67%) would represent the most recent fire. For S-Peru the probability was 67% for 1 sample and 100% for 3 samples. For N-Peru, the probability was 67% for 1 sample and 100% for 5 samples. The probability of observing the most recent fire under Strategy 2 was 67% when randomly sampling just 1 sample and 100% with 2 samples at both Guyana and N-Peru. The probability was 33% for 1 sample and 100% for 3 samples for S-Peru. Therefore, based on available dates, under Strategy 2 at least 3 samples (1 from the top of each of 3 pits) is required if the sampling is to have a reasonable likelihood of capturing the most recent fire for intact forests with no history of a recent fire (or 5 samples under Strategy 1). This analysis assumes that at least one of the dates from each site represents the most recent fire. We acknowledge that additional sampling may result in a more recent date, while also noting that the number of unique fire events is leveling off with increased sampling effort (Figure 3).
Fire Return Interval
Based on the unique fire events for each site, the median fire return interval among sites ranged from 455 cal years for S-Peru to 2,950 cal years for Guyana (Table 1). The shortest return interval was found in S-Peru (115 cal years) and the longest return interval was found at the Guyana site (4,510 cal years). Given that we cannot prove that we have captured all fires at each site, based on our present dataset, these values represent the maximum return period for fires at each site, and could potentially be over-estimates of the time between fires.
Age—Depth Relationships and Macrocharcoal Presence/Absence
Among regions, there was no significant relationship between dates of fire and soil depth. Within sites, only Pit 1 from Guyana and Pit 2 from N-Peru had weak significant age-depth relationships (p < 0.1; Figures 4A,B). Some pits had little variation in dates across deeper (i.e., > 40 cm) portions of the profile (e.g., S-Peru Pit 2). Dates were inverted in profiles, with 4/9 pits having older dates above younger dates. Some dates near the surface were up to 9,000 years older than dates from deeper depths (e.g., Guyana Pit 2, N-Peru Pit 1), demonstrating substantial mixing across the soil profile. Macrocharcoal presence/absence data by soil depth, pit, and site are shown in Figure 4.
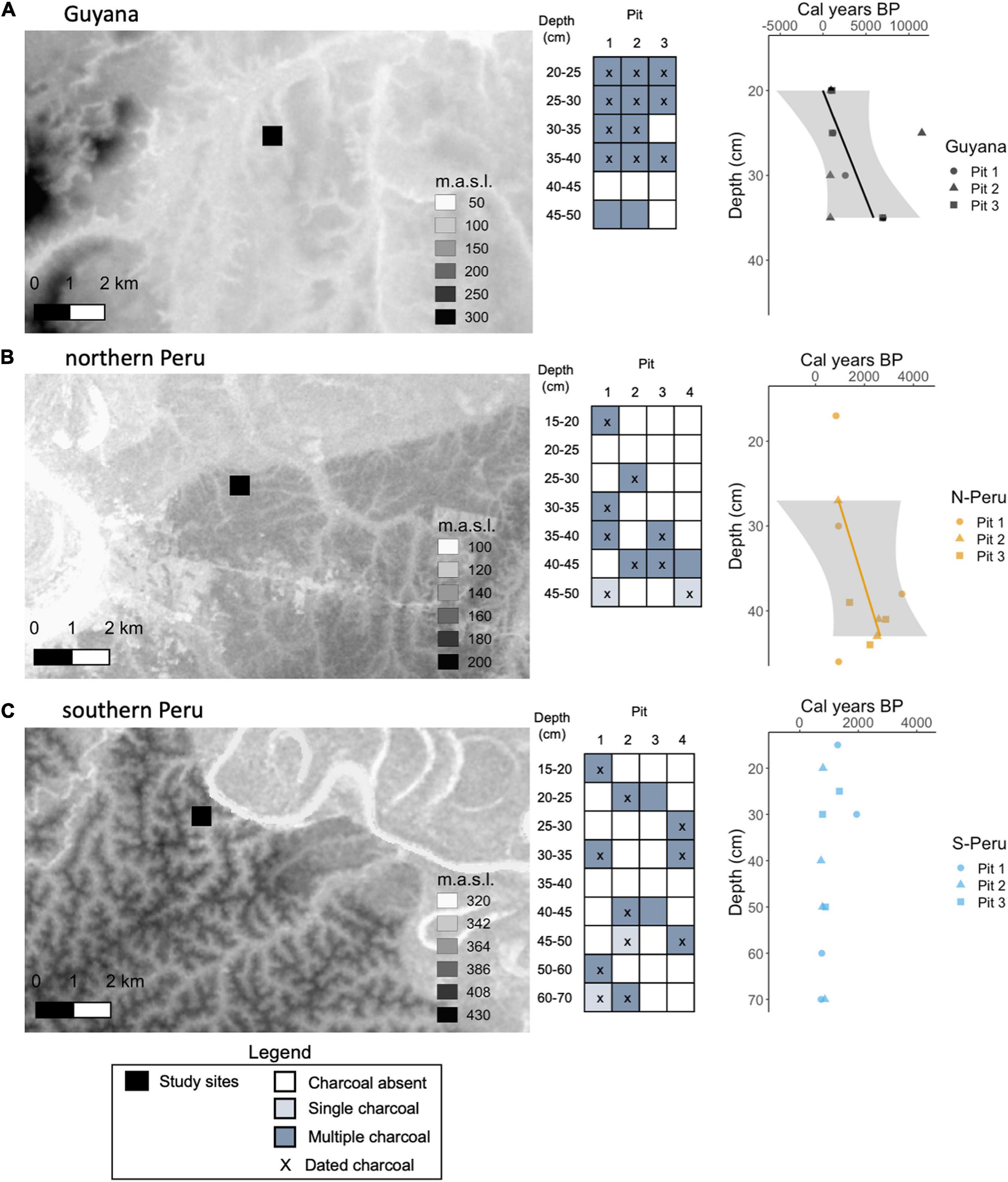
Figure 4. Regional maps of site location (black squares) and elevation (m a.s.l., SRTM DEM). Shaded boxes in the table show macrocharcoal presence/absence by pit and soil depth (see the legend). No macrocharcoal was found above the first reported depth in each table. Bivariate plots of calibrated date (cal yrs BP) and sample soil depth (cm) for (A) Guyana, (B) northern Peru, and (C) southern Peru. Lines represent significant relationships for individual pits (Guyana Pit 1; N-Peru Pit 2; p < 0.1) and shading 95% confidence intervals.
Discussion
Humid Amazonian forests were long considered mostly fire-free systems (Sanford et al., 1985; Pontes-Lopes et al., 2021). Here we show, in one of the most intensively local sampled radiocarbon studies to date of fire in terra-firme forest soils, that fire was rare but recurrent in these systems, with maximum return times of hundreds rather than thousands of years. Our study provides novel information about regional patterns in fire history spanning 11,500 cal yrs BP and, in line with other studies, shows a peak in the number of fire records preceding the Columbian Encounter (Nevle and Bird, 2008). While the sites exhibited 4–6 unique fires, none of the structurally intact forests had fire events more recent than ∼730 cal years BP (1,220 cal AD), indicating that in terms of fire disturbance at least, these forests could be considered old-growth (Willis et al., 2004). Our results add to the growing body of evidence of ancient disturbance by fire and native inhabitants that affected past Amazonian forests (Levis et al., 2017; McMichael and Bush, 2019; Oliveira et al., 2020; Bush et al., 2021).
Amazon Basin Fire Frequency: Regional Patterns, Great Dying, Past Climate
Our results are consistent with other studies from Amazonia showing a higher frequency of dates 500–2,000 cal years BP followed by a decline until modern times (Figure 5). The decline in fire may have been due to the Great Dying caused by the introduction of Old World diseases, where 95% of the native population died (Dull et al., 2010), and which was followed by the Little Ice Age (LIA) global thermal anomaly of about ∼−0.1°C from 1,550 to 1,750 A.D (Nevle and Bird, 2008). Nearly half of our dates occurred 500–1,000 cal years BP, with 18% 1,000–1,500 cal years BP (Figure 5). Non-soil charcoal had a greater fraction occurring earlier in the record, between 2,000 and 3,000 years cal BP, than soil charcoal (McMichael and Bush, 2019). Soil charcoal samples spanning southern, eastern, and northwestern Amazonia showed peaks 500–2,000 cal years BP (Goulart et al., 2017). Other studies reported a decline in the number of fires prior to the Columbian Encounter (Dull et al., 2010; Bush et al., 2021), suggesting events besides the Great Dying may have contributed to an earlier decline in fire, e.g., a wetter climate that reduced fire ignition and spread.
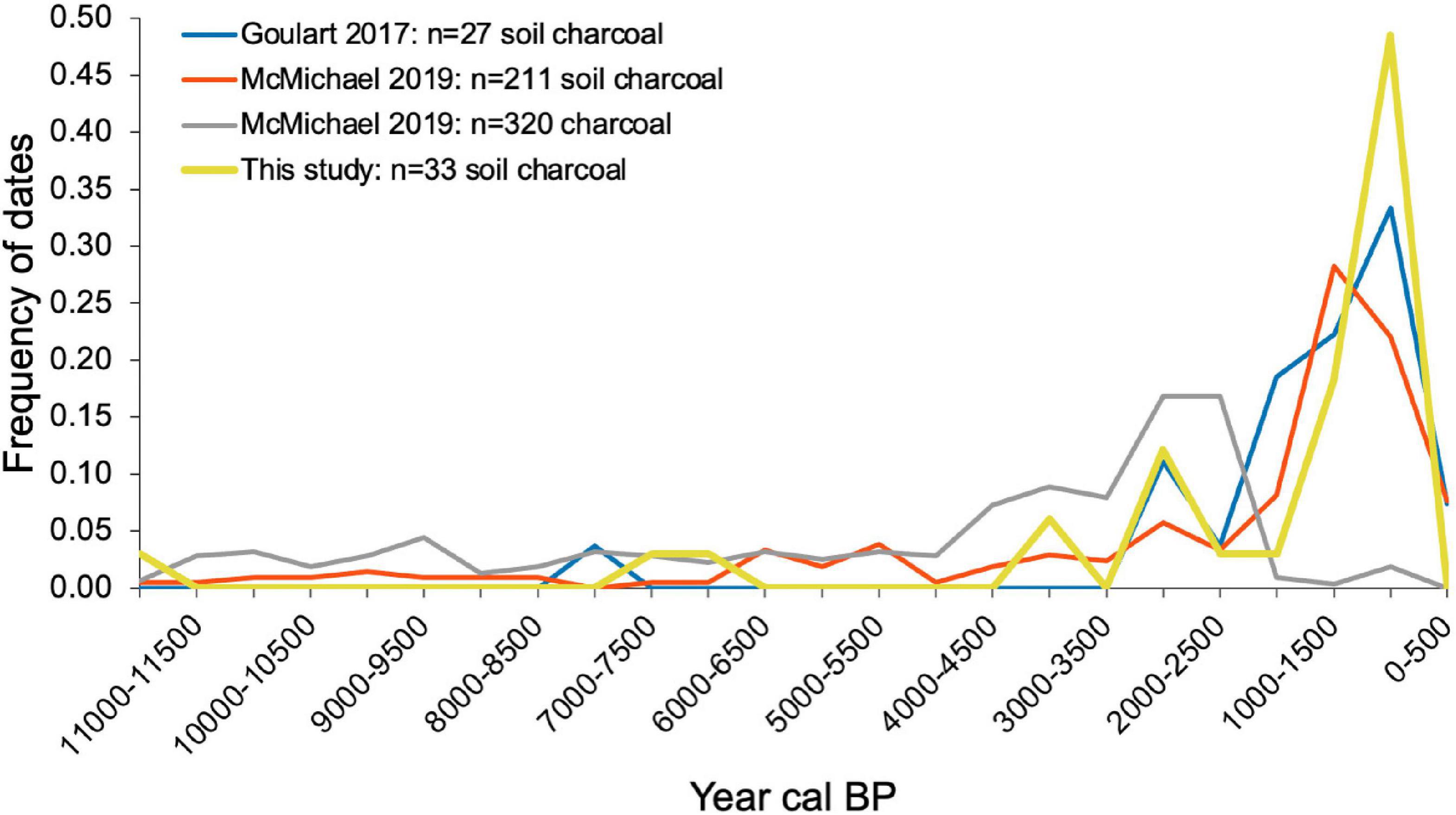
Figure 5. Frequency of dates from charcoal and soil charcoal by years calibrated before present (BP) in fraction of total dates per study from Goulart et al. (2017), compilations by McMichael and Bush, 2019, and this study.
Surprisingly, our wettest site from northern Peru, with 2,584 mm precipitation per year and no dry season (months with < 100 mm precipitation), had the highest number of unique fires. Such closed canopy forests maintain high below-canopy moisture levels that prevent fine fuels such as dead leaves and small branches that carry a fire from reaching flammability levels (e.g., leaf moisture content ≤ 23%) (Ray et al., 2005). An analysis of fire history based on lake cores found that charcoal was less abundant in northwestern Amazonia compared to other regions of Amazonia, with an elevated period of charcoal deposition 2,500–2,000 years ago (Gosling et al., 2021). Based on analyses from modern fires, the northwestern sites are considered too wet to burn in normal years, so that past fires would be associated with severe drought (Aragão et al., 2007; Pontes-Lopes et al., 2021) and ignition by past humans. Although we found no archeological artifacts in the soil or terra preta on the site, the high number of fires in this wet region may be indicative of past human influence in the region (McMichael and Bush, 2019). The charcoal found could have been formed from different types and scales of fire. Seasonally flooded forests have more fertile soil due to nutrient deposition during flooding (Salo et al., 1986), making these areas more suitable for agriculture than terra firme soils. Pre-Columbian land use in the adjacent seasonally flooded areas may have been a source of fire that spread into the higher elevation terra firme forests during drought and may explain the high number of fire events at the N-Peru site. The charcoal may have also been formed by small-scale sub-canopy campfires from hunting parties, i.e., fires that do not necessarily represent burning of live trees.
Fire Chronology and Date of Last Fire
Fire Return Interval
The mean fire return interval for two sites (Table 2) was shorter than the time since the last fire, suggesting that over the past ∼800 years, these forests have undergone a longer fire-free period than over the past 2,000–3,500 years. Where the fire return interval was longer at the Guyana site, the estimate was greater due to several very old dates (e.g., 11,513 cal yrs BP). These data represent the maximum fire return interval and additional sampling may reveal additional fires and shorter fire return intervals.
Date of Last Fire
Three 14C dates per site were sufficient to consistently assess the most recent fire from samples available for a given site. Although the most superficial samples are not always the most recent, within the samples from the same profiles from Peru and Guyana, our intensive sampling suggests ages around 800 cal years BP were the youngest and most likely related to the last fire since no new unique fire events were found after 10 dates for all pits. Strategy 2 that samples first surface soil across the pits and then by depth was most effective to capture the most recent fire; however, this strategy provides less information about fire history compared to Strategy 1. Under Strategy 1, by sampling down the depth profile rather than taking samples first from the surface, there is a greater opportunity to also capture younger dates that were randomly inverted in the soil and key information about fire chronology.
Sampling effort has traditionally focused on radiocarbon dating non-soil charcoal and non-charred material from archeological sites. In one of the largest compilations to date including > 850 14C dates for the Amazon region, 25% of the dates were sampled from charcoal in soil, 40% were from charcoal from other sources (e.g., lakes and peat or not identified as being from soil), and the remaining dates came from a variety of non-charred material such as unburned wood, bone, shell, etc., or from unknown sources (McMichael and Bush, 2019). Of the 540 charcoal and soil charcoal samples, 53% were from lowland (< 1,000 m a.s.l.), with the rest from upland Andean sites (Figure 1). This limits understanding of fire regime (return time, number of fires) for lowland terra firme forests, which represent the majority of Amazonia forests, to allow us to evaluate the role of past fire on forest composition, structure, dynamics.
At the local site scale, there was synchrony in fire events. For example, within a given site each of the 3 pits (separated by 100 m each) generally had the same date of the last fire. While our data and paleo-fire 14C databases provide a greater understanding of fire history, these datasets provide information only for individual sites and therefore have limited capacity to understand fire regime at larger local and regional scales. An analysis of fire history based on lake cores in southwestern Amazonia showed high sub-regional (e.g., 100 km) variability in the charcoal dataset, with a near absence of fire at some sites, while charcoal and vegetation histories indicate other sites were a regional settlement hub for pre-Columbian people (Gosling et al., 2021). To further understand fire regime, we need additional information about the fire area per unit of time to assess fire regime at different spatiotemporal scales (Payette et al., 2012), which requires wider sampling.
Of the several hundred surveyed permanent forest plots across Amazonia used to evaluate biodiversity and forest dynamics (e.g., Brienen et al., 2015; Sullivan et al., 2017), time since the last fire was published for only four plots (McMichael, 2021). Comparison to compiled 14C values (McMichael and Bush, 2019) that were near to our sites allows for assessment of wider-scale local variation. The nearest study in Guyana that sampled soil charcoal, ∼ 30 km north of our site, reported dates of the last fire that were more recent (∼390 cal years BP) than the dates we found (∼850 cal yrs BP). The nearest value for N-Peru, ∼65 km from our site, was slightly older (∼980 cal years BP) than the most recent fire we encountered (∼840 cal years BP). The nearest values for S-Peru, ∼2 km from our site, spanned (∼605–1,388 cal years BP) the most recent fire we encountered (∼725 cal years BP). Considering this regional variation at scales of 2–65 km in the most recent fires, care should be taken when making assumptions about fire history for permanent forest without radiocarbon dates based on plots with radiocarbon dates from the region.
Uncertainty in Estimates
Age Distributions
An aim was to estimate the date of the most recent fire, with sampling focussing on 0–70 cm depth (median 30 cm depth), which would have resulted in a larger number of more recent dates and underrepresented older dates (Figure 2) than sampling deeper depths. Factors such as preservation bias could influence the distribution of dates encountered, e.g., if there is poorer preservation of older charcoal or greater translocation of this material, then more charcoal from younger intervals (i.e., greater frequency of younger char) would be found. Despite this, the oldest dates of the entire dataset were found at 25–35 cm depth and one of the most recent dates at 70 cm depth.
Date Inversion and Age-Depth Relationships
The weak age-depth relationship and date inversion indicate limited utility to develop depth-age relationships from dates from soil pits. Date inversion and weak age-depth relationships can complicate interpretation of the data and require additional sample analysis. In two of the nine pits, the most recent fire event in each pit was found amongst the deeper charcoal fragments. Both of these cases occurred at the S-Peru site, with charcoal representing the most recent fire at 30–60 cm depth and older charcoal found at 15 cm depth. Unlike peat and lake sediment cores, which generally show intact stratigraphies (Swindles et al., 2018), bioturbation in terra firme soils is probably responsible for the lack of correlation between ages and depth.
Date of Last Fire
Our study provides strong evidence that our approach captured the most recent local fire at a given site. In estimating the date of the last fire for these sites, we conclude that 11 dates were sufficient to capture the date of the last fire based on no additional unique fire events encountered after 10 samples for all pits. This assumption could be tested with additional sampling and 14C analysis. We acknowledge that there may have been more recent unsampled fires in these sites. To our knowledge, no other study has sampled at the local intensity for terra-firme forest soils of our study. Therefore, within the limitations of our study, the current results provide the best evidence to date on sample numbers required to determine the local date of the last fire.
In-Built Age
The 14C date of charcoal is related to the year of growth of each tree ring. All 14C dates from charcoal, therefore, contain a bias toward an in-built age when estimating the date of the fire, with the bias larger for older trees. For fires in Amazonia, where fire preferentially kills younger trees, and fires are dominated by surface burning that consumes coarse woody debris and char tree stems rather than crown fires (Cochrane, 2003; Brando et al., 2012), in-built ages would be small and dates probably relate closely to the fire episode. In-built age could be reduced by selecting charred material with a short lifespan such as twigs and seeds.
Fire Legacy on Forests
Fire was an ancient event in our study sites with the most recent dates ∼800 cal years BP, which is well beyond the lifespan of most tropical trees (Laurance et al., 2004). While trees have been estimated using 14C to live to be 1,400 years (Chambers et al., 1998), age determinations using direct measurements of Amazon tree rings result in maximum ages between 400 and 500 years (Worbes and Junk, 1999). At the stand level, the median biomass carbon residence time is much less, ∼50 years (range 23–129 years) for mature tropical forests (Galbraith et al., 2013), since shorter-lived trees overwhelmingly dominate C cycling. This suggests that the ancient fires > 800 cal years BP encountered in our study sites may have had a minimal impact on modern C cycling. While these forests have been considered structurally intact or old-growth, the legacy effects of pre-Colombian land-use of these forests continue to be debated (Levis et al., 2017; McMichael and Bush, 2019; Oliveira et al., 2020; Bush et al., 2021; Gosling et al., 2021). Understanding of current forest C storage and diversity is based on several hundred plots across Amazonia (e.g., Sullivan et al., 2017); however, many forest inventory plots in Amazonia may be disproportionately located in areas having ancient human impacts (McMichael et al., 2017). It may be that ancient fire and historical land use has a greater legacy effect on forest composition (dos Prestes et al., 2020; Oliveira et al., 2020) than C storage and dynamics. Regardless, our study provides an important step toward understanding fire legacy effects on contemporary forests and provides key new radiocarbon datasets for long-term ecological study sites.
Charcoal and Environment
The very old dates (∼7,000 and 11,400 cal years BP) at the Guyana site, relative to the other sites, were at shallower depths than the oldest dates (although significantly younger) at the western Amazonia sites, suggesting environmental and substrate-related factors may have affected the position of charcoal in the soil profile. The Guiana Shield is characterized by a substrate with a geological age at least 42 million years older than western Amazonia (Quesada et al., 2010). These large differences affect the underlying physicochemical properties of the soil, which can affect charcoal protection and persistence (Czimczik and Masiello, 2007). The Guyana site also had a higher sand content (76%) (Quesada et al., 2012) than the other sites, which can improve aeration, reduce water holding capacity, and is associated with lower pyrogenic carbon storage (Koele et al., 2017). There is little information in the literature about the types of fires in tropical forests that produce material that enters the record or whether certain types of charcoal survive intact for longer. In forests in Guyana today, areas with sandy soil burn more, have more dead biomass and up to 10 times more standing litter due to slower decomposition (Hammond and ter Steege, 1998), which may affect the production and quantity of charcoal formed. Following cutting and burning of a forest in central Amazonia, around 2% of pre-burn aboveground C stocks were converted to charcoal (Fearnside et al., 1999). If conversion efficiencies remain consistent for less severe fire (e.g., understory), charcoal production would be small, but the charcoal could persist for millennia (Bird et al., 2015). The terra firme sites from our study are mostly flat and non-seasonally flooded, so that lateral movement and erosion (Bassini and Becker, 1990; Rumpel et al., 2006) of charcoal would be small. The larger charcoal size-class (≥ 1 mm) that we sampled would be unlikely to be uplifted and transported by convection from fires (Clark, 1988). Therefore, the macro charcoal we sampled should be of local origin (hundreds of meters) and represent in situ fires.
It is unlikely that all the charcoal found at our sites is due to lightning-induced fires. In Gabon, a single hollow tree was struck by lightning and burned, with charcoal formed by the burning trunk covering an area of 20 m diameter and by the burning fallen crown an area of 15 × 25 m (Tutin et al., 1996). However, studies from Amazonia, Panama, and Sarawak found that lightning rarely chars trees or results in fires in humid forests (Anderson, 1964; Magnusson et al., 1996; Yanoviak et al., 2020). It is difficult to determine the scale and synchronicity of fire events due to the large uncertainty around radiocarbon dates and without additional sampling around the sites. The synchronized dates of the fires at our sites in pits each separated by 100 m could indicate larger fires beyond single trees or a series of small fires that occurred within the uncertainty range of the radiocarbon dates. Lightning may be a greater source of fire and charcoal in seasonally dry forests where trees suffer greater water stress.
Future Opportunities
Fire history and drivers of its variation across the vast Amazon Basin is still uncertain. Over the coming decades, the Amazon region will likely undergo an increase in temperature, fire, and the extent and frequency of drought (Betts et al., 2015). Our study, with a focus on understanding the age of the most recent fire, provides new understanding for three regions and presents opportunities for future research. This work adds to a growing 14C dataset and extends the record for terra firme soil beyond the work on peat and lakes that are predominately spatially restricted to western Amazonia. Deeper sampling could help to assess more robustly the long-term fire record and the pre-Columbian fire return interval. There are also opportunities to use charcoal to understand changes in vegetation, e.g., anthracological analysis of several charcoal fragments from the same position and depth has revealed different families of trees with similar ages of 14C soil charcoal (Goulart et al., 2017).
Conclusion
Our analysis shows that fire impacted these systems in pre-Columbian times, with a variable fire record spanning the Holocene, marked by a decline in fire leading up to modern times. Ancient fire was occasional but recurrent in these forests, which have been considered too wet to burn naturally. The median values of fire return intervals were a maximum of 450 years, with little variation locally among pits in fire dates. Similar fire events today may have different consequences for forests as climatic conditions are changing. Based on the available dates, there is a high likelihood of estimating the date of the last fire with as few as three dates. The historical fire return interval of hundreds of years, and the absence of fire over the past ∼800 years in these forests, indicates these forests have undergone a longer fire-free period than in the past. In terms of fire disturbance at least, these forests could be considered old-growth, which has important implications for the composition, dynamics, and structure of these forests today and how they respond to future fire.
Data Availability Statement
The original contributions presented in the study are included in the article/supplementary material, further inquiries can be directed to the corresponding author/s.
Author Contributions
TF, LC, and KM conceived the study. TF wrote the manuscript and analyzed the data, with contributions to statistical analysis from LC and RS. LC sampled the charcoal. CFF, MK, and ENHC supported the fieldwork. KM prepared the charcoal samples. PA coordinated radiocarbon dating. All authors contributed to and approved the manuscript.
Funding
This study was supported by funding from the UK Natural Environment Research Council (NERC, NE/N011570/1 and NE/R017980/1) and a radiocarbon dating allocation (allocation 2122.0818) from the NERC-funded NEIF Radiocarbon Laboratory.
Conflict of Interest
The authors declare that the research was conducted in the absence of any commercial or financial relationships that could be construed as a potential conflict of interest.
Publisher’s Note
All claims expressed in this article are solely those of the authors and do not necessarily represent those of their affiliated organizations, or those of the publisher, the editors and the reviewers. Any product that may be evaluated in this article, or claim that may be made by its manufacturer, is not guaranteed or endorsed by the publisher.
Acknowledgments
We thank A.M. Mendoza, M.A. Pedraza Arando, C.D.A. Villacorta for logistical and administrative support for field sampling and Bruna Brandao and Fabiana Couto for assistance with sample preparation. We thank Callum Murray, Josanne Newton, Chris Taylor, and Pauline Gulliver for assistance with radiocarbon measurement and three reviewers for detailed reviews of the manuscript.
References
Anderson, J. A. R. (1964). Observations on climatic damage in peat swamp forest in Sarawak. Commonw. For. Rev. 43, 145–158.
Aragão, L. E. O. C., Malhi, Y., Roman-Cuesta, R. M., Saatchi, S., Anderson, L. O., and Shimabukuro, Y. E. (2007). Spatial patterns and fire response of recent Amazonian droughts. Geophys. Res. Lett. 34:948. doi: 10.1029/2006gl028946
Bassini, F., and Becker, P. (1990). Charcoal’s occurrence in soil depends on topography in terra firme forest near Manaus. Brazil. Biotropica 22, 420–422. doi: 10.2307/2388560
Betts, R. A., Golding, N., Gonzalez, P., Gornall, J., Kahana, R., Kay, G., et al. (2015). Climate and land use change impacts on global terrestrial ecosystems and river flows in the HadGEM2-ES Earth system model using the representative concentration pathways. Biogeosciences 12, 1317–1338. doi: 10.5194/bg-12-1317-2015
Bird, M. I., Wynn, J. G., Saiz, G., Christopher, M., and Wurster Anna, et al. (2015). The pyrogenic carbon cycle. Annu. Rev. Earth Planet. Sci. 43, 273–298. doi: 10.1146/annurev-earth-060614-105038
Brando, P. M., Nepstad, D. C., Balch, J. K., Bolker, B., Christman, M. C., Coe, M., et al. (2012). Fire-induced tree mortality in a neotropical forest: the roles of bark traits, tree size, wood density and fire behavior. Glob Change Bio 18, 630–641. doi: 10.1111/j.1365-2486.2011.02533.x
Brienen, R. J. W., Phillips, O. L., Feldpausch, T. R., Gloor, E., Baker, T. R., Lloyd, J., et al. (2015). Long-term decline of the Amazon carbon sink. Nature 519, 344–348. doi: 10.1038/nature14283
Bronk Ramsey, C. (2009). Bayesian analysis of radiocarbon dates. Radiocarbon 51, 337–360. doi: 10.1017/S0033822200033865
Bush, M. B., Nascimento, M. N., Åkesson, C. M., Cárdenes-Sandí, G. M., Maezumi, S. Y., Behling, H., et al. (2021). Widespread reforestation before European influence on Amazonia. Science 372, 484–487. doi: 10.1126/science.abf3870
Bush, M. B., Silman, M. R., de Toledo, M. B., Listopad, C., Gosling, W. D., Williams, C., et al. (2007). Holocene fire and occupation in Amazonia: records from two lake districts. Philos. Trans. R. Soc. Lond. B Biol. Sci. 362, 209–218. doi: 10.1098/rstb.2006.1980
Chambers, J. Q., Higuchi, N., and Schimel, J. P. (1998). Ancient trees in Amazonia. Nature 391, 135–136. doi: 10.1038/34325
Clark, J. S. (1988). Particle motion and the theory of charcoal analysis: Source area, transport, deposition, and sampling. Quat. Res. 30, 67–80. doi: 10.1016/0033-5894(88)90088-90089
Cochrane, M. A., and Laurance, W. F. (2002). Fire as a large-scale edge effect in Amazonian forests. J. Trop. Ecol. 18, 311–325. doi: 10.1073/pnas.1305499111
Crema, E. R., and Bevan, A. (2021). Inference from large sets of radiocarbon dates: software and methods. Radiocarbon 63, 23–39. doi: 10.1017/RDC.2020.95
Czimczik, C. I., and Masiello, C. A. (2007). Controls on black carbon storage in soils. Glob. Biogeochem. Cycles 21:GB3005. doi: 10.1029/2006gb002798
dos Prestes, N. C. C. S., Massi, K. G., Silva, E. A., Nogueira, D. S., de Oliveira, E. A., Freitag, R., et al. (2020). Fire effects on understory forest regeneration in Southern Amazonia. Front. For. Glob. Change 3:10. doi: 10.3389/ffgc.2020.00010
Dull, R. A., Nevle, R. J., Woods, W. I., Bird, D. K., Avnery, S., and Denevan, W. M. (2010). The Columbian encounter and the little ece age: abrupt land use change, fire, and greenhouse forcing. Ann. Assoc. Am. Geogr. 100, 755–771. doi: 10.1080/00045608.2010.502432
Dumont, J. F., Lamotte, S., and Kahn, F. (1990). Wetland and upland forest ecosystems in Peruvian Amazonia: plant species diversity in the light of some geological and botanical evidence. For. Ecol. Manag. 33, 125–139. doi: 10.1016/0378-1127(90)90188-h
Echalar, F., Artaxo, P., Martins, J. V., Yamasoe, M., Gerab, F., Maenhaut, W., et al. (1998). Long-term monitoring of atmospheric aerosols in the Amazon Basin: Source identification and apportionment. J. Geophys. Res. Atmospheres 103, 31849–31864. doi: 10.1029/98JD01749
Espirito-Santo, F. D. B., Gloor, M., Keller, M., Malhi, Y., Saatchi, S., Nelson, B., et al. (2014). Size and frequency of natural forest disturbances and the Amazon forest carbon balance. Nat. Commun. 5:3434. doi: 10.1038/ncomms4434
Fearnside, P. M., de Alencastro Graça, P. M. L., Leal Filho, N., Rodrigues, F. J. A., and Robinson, J. M. (1999). Tropical forest burning in Brazilian Amazonia: measurement of biomass loading, burning efficiency and charcoal formation at Altamira. Pará. For. Ecol. Manag. 123, 65–79. doi: 10.1016/s0378-1127(99)00016-x
Feldpausch, T. R., Lloyd, J., Lewis, S. L., Brienen, R. J. W., Gloor, M., Mendoza, A. M., et al. (2012). Tree height integrated into pantropical forest biomass estimates. Biogeosciences 9, 3381–3403. doi: 10.5194/bg-9-3381-2012
Feldpausch, T. R., Phillips, O. L., Brienen, R. J. W., Gloor, E., Lloyd, J., Lopez-Gonzalez, G., et al. (2016). Amazon forest response to repeated droughts. Glob. Biogeochem. Cycles 30, 964–982. doi: 10.1002/2015GB005133
Fick, S. E., and Hijmans, R. J. (2017). WorldClim 2: new 1-km spatial resolution climate surfaces for global land areas. Int. J. Climatol. 37, 4302–4315. doi: 10.1002/joc.5086
ForestPlots.net, Blundo, C., Carilla, J., Grau, R., Malizia, A., Malizia, L., et al. (2021). Taking the pulse of Earth’s tropical forests using networks of highly distributed plots. Biol. Conserv. 260:108849. doi: 10.1016/j.biocon.2020.108849
Galbraith, D., Malhi, Y., Affum-Baffoe, K., Castanho, A. D. A., Doughty, C. E., Fisher, R. A., et al. (2013). Residence times of woody biomass in tropical forests. Plant Ecol. Divers. 6, 139–157. doi: 10.1080/17550874.2013.770578
Glaser, B., Haumaier, L., Guggenberger, G., and Zech, W. (2001). The “Terra Preta” phenomenon: a model for sustainable agriculture in the humid tropics. Naturwissenschaften 88, 37–41. doi: 10.1007/s001140000193
Glaser, B., Lehmann, J., and Zech, W. (2002). Ameliorating physical and chemical properties of highly weathered soils in the tropics with charcoal - a review. Biol. Fertil. Soils 35, 219–230. doi: 10.1007/s00374-002-0466-464
Glasspool, I. J., Edwards, D., and Axe, L. (2004). Charcoal in the Silurian as evidence for the earliest wildfire. Geology 32, 381–383. doi: 10.1130/g20363.1
Gloor, M., Phillips, O. L., Lloyd, J. J., Lewis, S. L., Malhi, Y., Baker, T. R., et al. (2009). Does the disturbance hypothesis explain the biomass increase in basin-wide Amazon forest plot data? Glob. Change Biol. 15, 2418–2430. doi: 10.1111/j.1365-2486.2009.01891.x
Gosling, W. D., Maezumi, S. Y., Heijink, B. M., Nascimento, M. N., Raczka, M. F., van der Sande, M. T., et al. (2021). Scarce fire activity in north and north-western Amazonian forests during the last 10,000 years. Plant Ecol. Divers. 14, 143–156. doi: 10.1080/17550874.2021.2008040
Goulart, A. C., Macario, K. D., Scheel-Ybert, R., Alves, E. Q., Bachelet, C., Pereira, B. B., et al. (2017). Charcoal chronology of the Amazon forest: A record of biodiversity preserved by ancient fires. Quat. Geochronol. 41, 180–186. doi: 10.1016/j.quageo.2017.04.005
Hammond, D. S., and ter Steege, H. (1998). Propensity for fire in Guianan rainforests. Conserv. Biol. 12, 944–947. doi: 10.1046/j.1523-1739.1998.012005944.x
Hogg, A. G., Heaton, T. J., Hua, Q., Palmer, J. G., Turney, C. S., Southon, J., et al. (2020). SHCal20 Southern Hemisphere calibration, 0-55,000 years cal BP. Radiocarbon 62, 759–778. doi: 10.1017/rdc.2020.59
Honorio Coronado, E. N., Baker, T. R., Phillips, O. L., Pitman, N. C., Pennington, R. T., Vasquez Martinez, R., et al. (2009). Multi-scale comparisons of tree composition in Amazonian terra firme forests. Biogeosciences 6, 2719–2731. doi: 10.5194/bg-6-2719-2009
Huang, W., Liu, X., González, G., and Zou, X. (2019). Late Holocene fire history and charcoal decay in subtropical dry forests of Puerto Rico. Fire Ecology 15:14.
Hubau, W., Lewis, S. L., Phillips, O. L., Affum-Baffoe, K., Beeckman, H., Cuní-Sanchez, A., et al. (2020). Asynchronous carbon sink saturation in African and Amazonian tropical forests. Nature 579, 80–87. doi: 10.1038/s41586-020-2035-2030
INPE (2020). National Institute of Space Research (INPE) Monitoring of the Brazilian Amazon Forest by Satellite 1999-2020. INPE: São José dos Campos.
Koele, N., Bird, M., Haig, J., Marimon-Junior, B. H., Marimon, B. S., Phillips, O. L., et al. (2017). Amazon Basin forest pyrogenic carbon stocks: first estimate of deep storage. Geoderma 306, 237–243. doi: 10.1016/j.geoderma.2017.07.029
Laurance, W. F., Nascimento, H. E. M., Laurance, S. G., Condit, R., D’Angelo, S., and Andrade, A. (2004). Inferred longevity of Amazonian rainforest trees based on a long-term demographic study. For. Ecol. Manag. 190, 131–143. doi: 10.1016/j.foreco.2003.09.011
Levis, C., Costa, F. R., Bongers, F., Pena-Claros, M., Clement, C. R., Junqueira, A. B., et al. (2017). Persistent effects of pre-Columbian plant domestication on Amazonian forest composition. Science 355, 925–931. doi: 10.1126/science.aal0157
Lewis, S. L., Lopez-Gonzalez, G., Sonke, B., Affum-Baffoe, K., Baker, T. R., Ojo, L. O., et al. (2009). Increasing carbon storage in intact African tropical forests. Nature 457, 1003–1006. doi: 10.1038/nature07771
Lopez-Gonzalez, G., Lewis, S. L., Burkitt, M., and Phillips, O. L. (2011). ForestPlots.net: a web application and research tool to manage and analyse tropical forest plot data. J. Veg. Sci. 22, 610–613. doi: 10.1111/j.1654-1103.2011.01312.x
Magnusson, W. E., Lima, A. P., and De Lima, O. (1996). Group lightning mortality of trees in a Neotropical forest. J. Trop. Ecol. 12, 899–903. doi: 10.1017/s0266467400010166
Marsh, E. J., Bruno, M. C., Fritz, S. C., Baker, P., Capriles, J. M., and Hastorf, C. A. (2018). IntCal, SHCal, or a mixed curve? Choosing a 14C calibration curve for archaeological and paleoenvironmental records from tropical South America. Radiocarbon 60, 925–940. doi: 10.1017/rdc.2018.16
Massi, K. G., Bird, M., Marimon, B. S., Marimon, B. H., Nogueira, D. S., Oliveira, E. A., et al. (2017). Does soil pyrogenic carbon determine plant functional traits in Amazon Basin forests? Plant Ecol. 218, 1047–1062. doi: 10.1007/s11258-017-0751-759
McMichael, C. H., Piperno, D. R., Bush, M. B., Silman, M. R., Zimmerman, A. R., Raczka, M. F., et al. (2012). Sparse pre-columbian human habitation in Western Amazonia. Science 336, 1429–1431. doi: 10.1126/science.1219982
McMichael, C. N. (2021). Ecological legacies of past human activities in Amazonian forests. New Phytol. 229, 2492–2496. doi: 10.1111/nph.16888
McMichael, C. N., and Bush, M. B. (2019). Spatiotemporal patterns of pre-Columbian people in Amazonia. Quat. Res. 92, 53–69. doi: 10.1017/qua.2018.152
McMichael, C. N., Matthews-Bird, F., Farfan-Rios, W., and Feeley, K. J. (2017). Ancient human disturbances may be skewing our understanding of Amazonian forests. Proc. Natl. Acad. Sci. U.S.A. 114, 522–527. doi: 10.1073/pnas.1614577114
Nevle, R. J., and Bird, D. K. (2008). Effects of syn-pandemic fire reduction and reforestation in the tropical Americas on atmospheric CO2 during European conquest. Palaeogeogr. Palaeoclimatol. Palaeoecol. 264, 25–38. doi: 10.1016/j.palaeo.2008.03.008
Nogueira, D. S., Marimon, B. S., Marimon-Junior, B. H., Oliveira, E. A., Morandi, P., Reis, S. M., et al. (2019). Impacts of fire on forest biomass dynamics at the southern amazon edge. Environ. Conserv. 46, 285–292. doi: 10.1017/S0376892919000110
Oliveira, E. A., Marimon-Junior, B. H., Marimon, B. S., Iriarte, J., Morandi, P. S., Maezumi, S. Y., et al. (2020). Legacy of Amazonian Dark Earth soils on forest structure and species composition. Glob. Ecol. Biogeogr. 29, 1458–1473. doi: 10.1111/geb.13116
Payette, S., Delwaide, A., Schaffhauser, A., and Magnan, G. (2012). Calculating long-term fire frequency at the stand scale from charcoal data. Ecosphere 3, 1–16. doi: 10.1890/09-0230.1
Phillips, O. L., Malhi, Y., Higuchi, N., Laurance, W. F., Núñez, P. V., Vásquez, R. M., et al. (1998). Changes in the carbon balance of tropical forests: evidence from long-term plots. Science 282, 439–442. doi: 10.1126/science.282.5388.439
Piperno, D. R., and Becker, P. (1996). Vegetational history of a site in the central Amazon basin derived from phytolith and charcoal records from natural soils. Quat. Res. 45, 202–209. doi: 10.1006/qres.1996.0020
Pivello, V. R. (2011). The use of fire in the cerrado and amazonian rainforests of Brazil: past and present. Fire Ecol. 7, 24–39. doi: 10.4996/fireecology.0701024
Pontes-Lopes, A., Silva, C. V. J., Barlow, J., Rincón, L. M., Campanharo, W. A., Nunes, C. A., et al. (2021). Drought-driven wildfire impacts on structure and dynamics in a wet Central Amazonian forest. Proc. R. Soc. B Biol. Sci. 288:20210094. doi: 10.1098/rspb.2021.0094
Quesada, C. A., Lloyd, J., Anderson, L. O., Fyllas, N. M., Schwarz, M., and Czimczik, C. I. (2011). Soils of Amazonia with particular reference to the RAINFOR sites. Biogeoscience 8, 1415–1440. doi: 10.5194/bg-8-1415-2011
Quesada, C. A., Lloyd, J., Schwarz, M., Patiño, S., Baker, T. R., Czimczik, C., et al. (2010). Variations in chemical and physical properties of Amazon forest soils in relation to their genesis. Biogeoscience 7, 1515–1541. doi: 10.5194/bg-7-1515-2010
Quesada, C. A., Phillips, O. L., Schwarz, M., Czimczik, C. I., Baker, T. R., Patino, S., et al. (2012). Basin-wide variations in Amazon forest structure and function are mediated by both soils and climate. Biogeoscience 9, 2203–2246. doi: 10.5194/Bg-9-2203-2012
R Core Team (2021). R: A Language and Environment for Statistical Computing. Vienna: R Foundation for Statistical Computing.
Ray, D., Nepstad, D., and Moutinho, P. (2005). Micrometeorological and canopy controls of fire susceptibility in a forested Amazon landscape. Ecol. Appl. 15, 1664–1678. doi: 10.1890/05-0404
Reimer, P. J., Austin, W. E., Bard, E., Bayliss, A., Blackwell, P. G., Ramsey, C. B., et al. (2020). The IntCal20 Northern Hemisphere radiocarbon age calibration curve (0-55 cal kBP). Radiocarbon 62, 725–757. doi: 10.1017/rdc.2020.41
Rumpel, C., Chaplot, V., Planchon, O., Bernadou, J., Valentin, C., and Mariotti, A. (2006). Preferential erosion of black carbon on steep slopes with slash and burn agriculture. Catena 65, 30–40. doi: 10.1016/j.catena.2005.09.005
Salo, J., Kalliola, R., Hakkinen, I., Makinen, Y., Niemela, P., Puhakka, M., et al. (1986). River dynamics and the diversity of Amazon lowland forest. Nature 322, 254–258. doi: 10.1038/322254a0
Sanford, R. L., Saldarriaga, J., Clark, K. E., Uhl, C., and Herrera, R. (1985). Amazon rain-forest fires. Science 227, 53–55. doi: 10.1126/science.227.4682.53
Silva, C. V. J., Aragão, L. E. O. C., Young, P. J., Espirito-Santo, F., Berenguer, E., Anderson, L. O., et al. (2020). Estimating the multi-decadal carbon deficit of burned Amazonian forests. Environ. Res. Lett. 15:114023. doi: 10.1088/1748-9326/abb62c
Simon, M. F., and Pennington, T. (2012). Evidence for adaptation to fire regimes in the tropical savannas of the Brazilian Cerrado. Int. J. Plant Sci. 173, 711–723. doi: 10.1086/665973
Simon, M. F., Grether, R., de Queiroz, L. P., Skema, C., Penningtone, R. T., Hughes, C. E., et al. (2009). Recent assembly of the Cerrado, a neotropical plant diversity hotspot, by in situ evolution of adaptations to fire. Proc. Natl. Acad. Sci. U S A. 106:20359. doi: 10.1073/pnas.0903410106
Slota, P. J., Jull, A. T., Linick, T. W., and Toolin, L. J. (1987). Preparation of small samples for 14C accelerator targets by catalytic reduction of CO. Radiocarbon 29, 303–306. doi: 10.1017/S0033822200056988
Sullivan, M. J., Talbot, J., Lewis, S. L., Phillips, O. L., Qie, L., Begne, S. K., et al. (2017). Diversity and carbon storage across the tropical forest biome. Sci. Rep. 7:39102. doi: 10.1038/srep39102
Swindles, G. T., Morris, P. J., Whitney, B., Galloway, J. M., Gałka, M., Gallego-Sala, A., et al. (2018). Ecosystem state shifts during long-term development of an Amazonian peatland. Glob. Change Biol. 24, 738–757. doi: 10.1111/gcb.13950
ter Steege, H., Pitman, N. C. A., Sabatier, D., Baraloto, C., Salomao, R. P., Guevara, J. E., et al. (2013). Hyperdominance in the Amazonian Tree Flora. Science 342:1243092. doi: 10.1126/science.1243092
Trumbore, S. (2009). Radiocarbon and soil carbon dynamics. Annu. Rev. Earth Planet Sci. 37, 47–66. doi: 10.1146/annurev.earth.36.031207.124300
Tutin, C. E., White, L. J., and Mackanga-Missandzou, A. (1996). Lightning strike burns large forest tree in the Lope Reserve. Gabon. Glob. Ecol. Biogeogr. Lett. 5, 36–41. doi: 10.2307/2997469
Willis, K. J., Gillson, L., and Brncic, T. M. (2004). How “virgin” is virgin rainforest? Science 304, 402–403. doi: 10.1126/Science.1093991
Worbes, M., and Junk, W. J. (1999). How old are tropical trees? the persistence of a myth. IAWA J. 20, 255–260. doi: 10.1163/22941932-90000689
Keywords: tropical forest, terra firme, charcoal samples, fire, soil, radiocabon dating, pre-Columbian, Holocene
Citation: Feldpausch TR, Carvalho L, Macario KD, Ascough PL, Flores CF, Coronado ENH, Kalamandeen M, Phillips OL and Staff RA (2022) Forest Fire History in Amazonia Inferred From Intensive Soil Charcoal Sampling and Radiocarbon Dating. Front. For. Glob. Change 5:815438. doi: 10.3389/ffgc.2022.815438
Received: 15 November 2021; Accepted: 28 February 2022;
Published: 03 May 2022.
Edited by:
Nophea Sasaki, Asian Institute of Technology, ThailandReviewed by:
Juan Ernesto Guevara Andino, Field Museum of Natural History, United StatesGiuliano Maselli Locosselli, University of São Paulo, Brazil
William Daniel Gosling, University of Amsterdam, Netherlands
Copyright © 2022 Feldpausch, Carvalho, Macario, Ascough, Flores, Coronado, Kalamandeen, Phillips and Staff. This is an open-access article distributed under the terms of the Creative Commons Attribution License (CC BY). The use, distribution or reproduction in other forums is permitted, provided the original author(s) and the copyright owner(s) are credited and that the original publication in this journal is cited, in accordance with accepted academic practice. No use, distribution or reproduction is permitted which does not comply with these terms.
*Correspondence: Ted R. Feldpausch, dC5yLmZlbGRwYXVzY2hAZXhldGVyLmFjLnVr