- 1Faculty of Forestry, Universitas Gadjah Mada, Yogyakarta, Indonesia
- 2Faculty of Environmental Sciences, Institute of Forest Growth and Computer Sciences, Technische Universität Dresden, Tharandt, Germany
- 3Balai Konservasi Sumberdaya Alam Kalimantan Barat, Pontianak, Indonesia
- 4Department of Mechanical and Industrial Engineering, Faculty of Engineering, Universitas Gadjah Mada, Yogyakarta, Indonesia
- 5Centre for Ecology and Conservation, College of Life and Environmental Sciences, University of Exeter, Exeter, United Kingdom
- 6School of Geography, Geology and the Environment, University of Leicester, Leicester, United Kingdom
Fire is considered a major threat to biodiversity in many habitats and the occurrence of fire has frequently been used to investigate the effectiveness of protected areas. Yet, despite the known importance of tropical peatlands for biodiversity conservation and serious threat that anthropogenically induced fires pose to this ecosystem, the influence of protected area designation on fire occurrence in tropical peatland has been poorly assessed thus far. Our study addresses this knowledge gap through providing a novel assessment of fire patterns from a tropical peatland protected area and surrounding landscape. We investigated the importance of both climatic factors (top-down mechanism) and human interventions (bottom-up mechanism) on fire occurrence through analyzing 20-years (2001–2020) of LANDSAT and Moderate Resolution Imaging Spectrometer (MODIS) images of the Padang Sugihan Wildlife Reserve and a 10-km buffer area surrounding this in Sumatra, Indonesia. Fire density was assessed in relation to road and canal construction. Monthly and annual precipitation was compared between wet and dry years. The reserve was effective in limiting fire compared to surrounding landscapes only in wet years. We revealed that peat fire occurrence in the protected area and buffer zone was not due to climatic factors alone, with distance from canals and roads also contributing toward fire occurrence. Our results suggest that it is essential to address tropical peatland fire processes at a landscape level, particularly at the surroundings of protected areas, in order to increase the effectiveness of fire protection, improve fire risk classification maps, and conserve threatened tropical peatland wildlife such as the Sumatran elephant.
Introduction
Fire has a long historical relationship with humans and, as such, human use of fire has contributed toward shaping landscapes for millennia (Daniau et al., 2010). While fire may be a regular natural event that is important in maintaining the characteristics and properties of some ecosystems (e.g., African savannah/similar: Stocks et al., 1996; Van Wilgen, 2009), in other ecosystems, natural fire events occur only rarely or are absent, and the introduction of fire by humans may therefore create substantial damage from which recovery can be prolonged (e.g., tropical peatlands: Page et al., 2009; Cole et al., 2015). Fires are frequently therefore used as an indicator to evaluate the effectiveness of protected area management (Adeney et al., 2009; Rodríguez et al., 2013). Common approaches to manage fire in protected areas include employing active fire management or prescribed burning to reduce fuel availability for preventing and controlling wildfire as well as managing invasive species (Van Wilgen, 2009), however, this approach is not appropriate for other ecosystems such as peatlands where fire is not a natural event.
Anthropogenic and natural factors lead to different patterns of fire occurrences in relation to the presence/absence of protected area across various ecosystems. For example, fire density was found to be two times higher in non-protected areas than within protected areas in Myanmar (Biswas et al., 2015) and Amazonia (Adeney et al., 2009). In contrast, natural mechanisms caused higher fire density in protected areas than in non-protected areas in West and Central Africa (Grégoire and Simonetti, 2010). More fires occurred in 59% of this area where deforestation rates dropped between 2000 and 2007, because more fuel was available for ignition (Aragao and Shimabukuro, 2010). Here, controlled ignition and active fire management are required to mitigate fuel availability. More generally, the effectiveness of reserves in reducing fire-driven deforestation has been shown to be linked to anthropogenic factors, including reduced road construction and human impact on area occupancy (Adeney et al., 2009) as well as implementing fire-free land management strategies (Aragao and Shimabukuro, 2010).
Fire is a critical issue in contemporary tropical peatland management, especially in Southeast Asia, but rarely occurred in this habitat until the last 3,000 years as anthropogenic factors began to have a greater influence on these peatland areas (Hope et al., 2005; Cole et al., 2015). Peatlands are particularly vital ecosystems in Indonesia, which has the largest peatland area in Southeast Asia, covering 18–20 million hectares, or 10% of the terrestrial area, storing vast quantities of carbon, and significantly contributing to primary sources of wood and livelihood for local people (Miettinen et al., 2013).
In the last two to three decades, fire has become an increasingly critical concern in the tropical peatlands of Southeast Asia and Indonesia in particular, with a drastic shift in peatland dynamics, from being a frequently inundated, moist and therefore fire-resistant ecosystem into an increasingly human-altered, drained and thus fire-prone ecosystem (Langner et al., 2007; Putra et al., 2008; Miettinen and Liew, 2010; Miettinen et al., 2012). This ecosystem change and consequent increase in fire prevalence and severity have important consequences for public health, local economies, carbon emissions, and biodiversity conservation (Page et al., 2004; Harrison et al., 2009; Posa, 2011). The recent fires of 2015 in Indonesia were the most severe since the 1997 megafires and almost the whole Sumatra island was engulfed by smoke, with South Sumatra province holding the second highest number of hotspots amongst the Indonesian provinces during 2015 (Atwood et al., 2016). In Sumatra, the combination of deforestation from adjacent areas (Imron et al., 2011; CIFOR Forests News, 2014), plus fire associated with the construction of roads (Linkie et al., 2004), rapid expansion of palm oil plantations, and land preparation (Field et al., 2009) has significantly increased threats to protected areas and thus for biodiversity conservation, in particular for forest dependent species. Fire in Indonesian peatland has been intensively studied through the use of remote sensing data to predict fire effects (Yeager et al., 2003; Ballhorn et al., 2009; Elvidge et al., 2015), investigate ignition sources (Cattau et al., 2016), causes of fire (Shiodera et al., 2012; Hayasaka et al., 2016), peat hydrology (Jaenicke et al., 2010), fire database management (Field et al., 2015), effect of fire on bio-physical attributes (Yeager et al., 2003; Spessa et al., 2009; Brown et al., 2015), and peat restoration planning (Jaenicke et al., 2010). Nevertheless, studies on the effectiveness of protected areas in reducing tropical peatland wildfire are still limited. This therefore represents an important information gap, given the priority assigned to expanding protected area coverage both globally (Open-Ended Working Group On The Post-2020 Global Biodiversity Framework, 2021) and within tropical peatland nations such as Indonesia (Presiden Republik Indonesia, 2016). In particular, the patterns and causal factors of fire in a protected area might help reveal more information to guide effective tropical peatland fire management, with current studies often overlooking the importance of peatland protected areas on reducing fires in the landscape.
With this in mind, we aim to gain insight into the patterns and causal factors of fires within and surrounding the Padang Sugihan Wildlife Reserve (PSWR), a protected tropical peatland area in South Sumatra, Indonesia, which is dedicated for biodiversity conservation. We observed fire occurrence data over a 15-year period to provide a basis for evaluating the effectiveness of peatland protected areas in reducing fire incidence compared to the surrounding landscape, and in so doing provide insight into the effectiveness of this strategy for reducing the current and potential future impacts of tropical peatland fire on local biodiversity and human communities.
Materials and Methods
The Study Area
Padang Sugihan Wildlife Reserve is a protected area managed by the South Sumatra Natural Resources Conservation Agency (Balai Konservasi Sumber Daya Alam/BKSDA), Ministry of Environment and Forestry, Republic of Indonesia. This reserve aims to protect the remaining Sumatran elephant (Elephas maximus sumatranus) populations in the province. PSWR occupies an area of 881 km2 which is dominated by tropical peatland (Nash and Nash, 1986; Sumsel, 2021). Sugihan River lies to the west of the reserve, while Padang River serves as the border to the east. To the south of the reserve, lies Butung River and a canal has been built to the north (Figure 1). Administrative boundary maps of South Sumatra1 and PSWR maps were used in this study and obtained from BKSDA Sumatera Selatan2 in 2015.
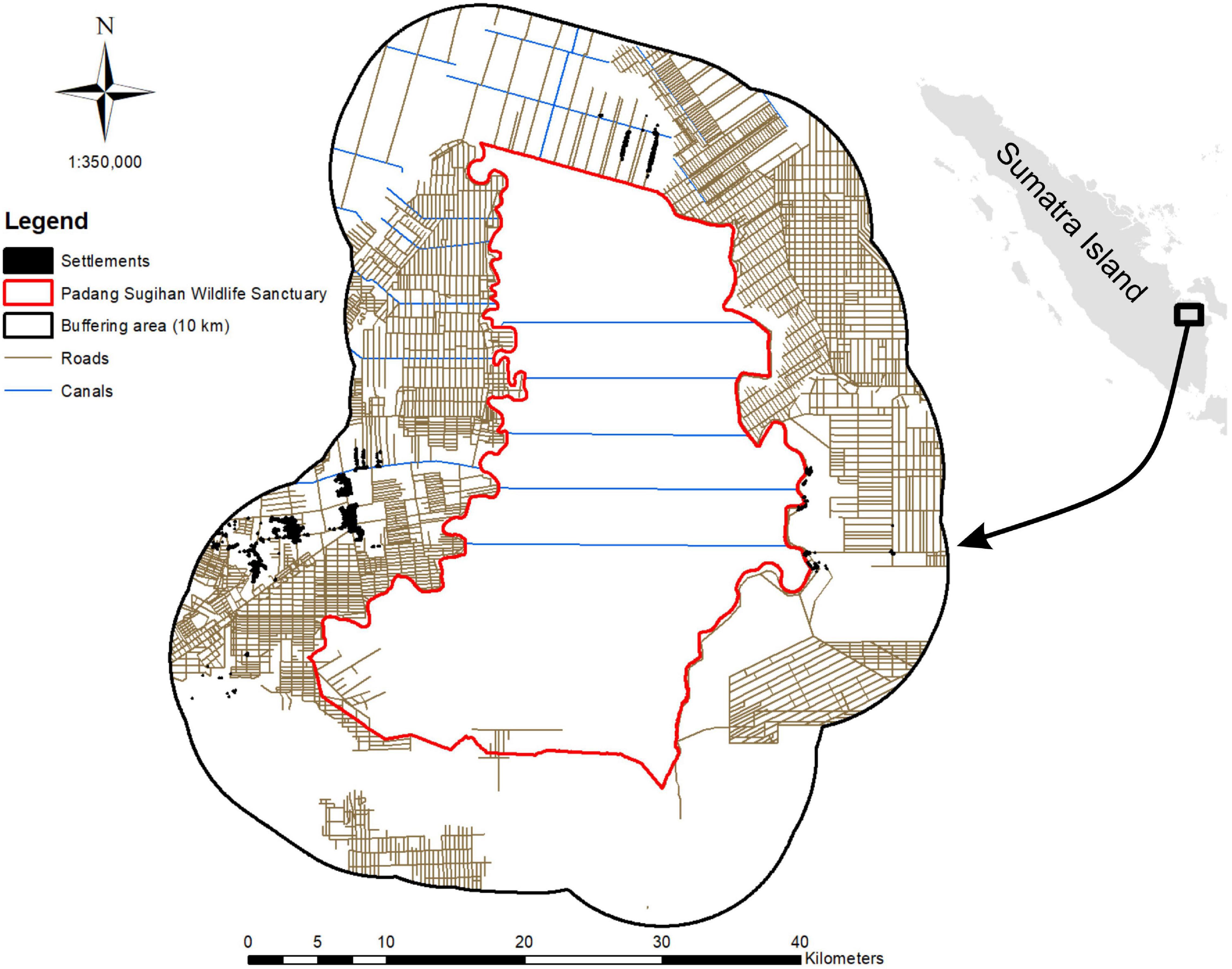
Figure 1. Map of study area as of 2015, showing the canal and road networks within and surrounding Padang Sugihan Wildlife Reserve (PSWR) and the presence of settlements within a 10 km buffer of the reserve.
Fire Detection and Environmental Studies
We used Active Fire data provided by the Moderate Resolution Imaging Spectrometer (MODIS) satellite to document hotspot prevalence over time in the study area.3 MODIS provides the geographical position of fires within 24 h of monitoring (Grégoire and Simonetti, 2010) as well as the 1–2 days return interval which is useful to monitor the change of fire occurrence at any given location (Willis, 2015). We selected MODIS active fire data for the period between 2001 and 2020 with a confidence level of 80%. Since rainfall and thus fire prevalence in Indonesia is heavily influenced by the El Niño Southern Oscillation (ENSO), we categorized the fire data as falling with periods of La Niña, El Niño or Neutral conditions, based on the Oceanic Niño Index (ONI) as per NOAA (2021) following https://ggweather.com/enso/oni.htm.
To compare fire occurrences within and surrounding the reserve, we calculated the number of hotspots within the reserve and within 10 km surrounding its border (Figure 1). A buffer zone of 10 km was chosen because it is equal to the longest distance from the border to the reserve center point (Figure 1) and is a distance commonly used to assess protected area effectiveness (Joppa et al., 2009). The total buffer area was 1,764.417 km2, which combined with the area of the PSWR (878.596 km2), yielded a total study area of 2,643.013 km2. We divided this study area into 1 × 1 km2 grid cells to identify whether fires can occur in the same area, with recurrent incidences of fire in the same cell over the observation period are classified here as a repeat fire.
To understand the impact of the reserve border on fire density, we compared fire frequency within the reserve and within 1 km distance bands in the surrounding buffer zone. We used the buffer function from ArcGIS 10 to measure fire density in each distance interval. The distribution of fire for the period 2001–2020 was calculated using the Kernel Density Estimation method within ArcGIS 10. The period 2001–2020 was important for the region due to a high fire incidence.
To understand the influence of precipitation on fire occurrence, we obtained rainfall data during 2001–2020 from the nearest weather station, at Sultan Badaruddin II Airport4 in Palembang (±45 km from the PSWR). To test the relationship between monthly precipitation and number of rainy days on fire occurrences, we performed Spearman correlation tests using data from fire occurrence months (August, September, and October) across the study period.
The influence of presence of roads and canal systems within the reserve and surrounding areas was ascertained through manually digitizing satellite images from Landsat 7 and Landsat 85 for each year (Table 1). We used www.openstreetmap.org to detect the presence of settlements in the surrounding area of PSWR. We collected land cover data from the Ministry of Environment and Forestry Republic of Indonesia (dan Kehutanan, 2021) and used land cover classifications from LaGro (2005).
To test the potential influence of environmental factors on the probability of fire occurrence, we used the Normalized Density Vegetation Index (NDVI), Enhanced Vegetation Index (EVI), distance from canal (dist-canal), and distance from road (dist-road) within a Maximum Entropy Model (Maxent) to develop a fire-risk probability map (Chen et al., 2015; Kim et al., 2019) for both La Niña and El Niño. We assessed the model performance using the receiver operating characteristic (ROC) curve method, and the models’ outputs were compared based on the area under the ROC curve (AUC). We classified fire risks probability maps into three classes with probability of 0–0.33 (low); 0.34–0.66 (medium) and >0.66 (high).
Results
Land Use Land Cover and Canal-Road Development
The land cover within PSWR is dominated by peatland vegetation/wetland shrubs (81.08%) with a very small proportion of agricultural areas, whereas forest plantation and agricultural areas dominate in the reserve buffer (Table 2). Roads development surrounding the reserve increased substantially during 2001–2015, with a small encroachment of road into the southern part of the reserve visible by 2010, whereas canal construction was steady since 2001, with no significant addition during 2001–2020 (Figure 2).
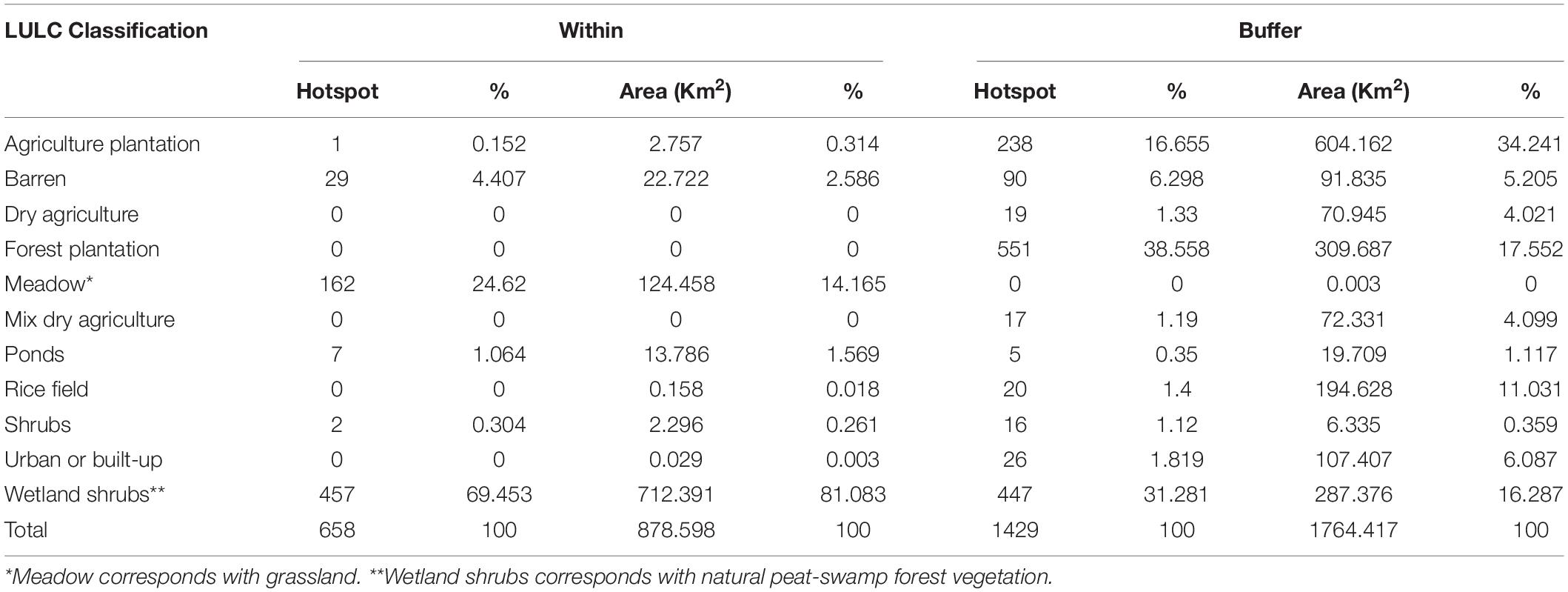
Table 2. Size and proportion of each land use and land cover classification, and number of hotspots from 2001 to 2020, within Padang Sugihan Wildlife Reserve (PSWR) and buffer area in 2021 (Source: dan Kehutanan, 2021).
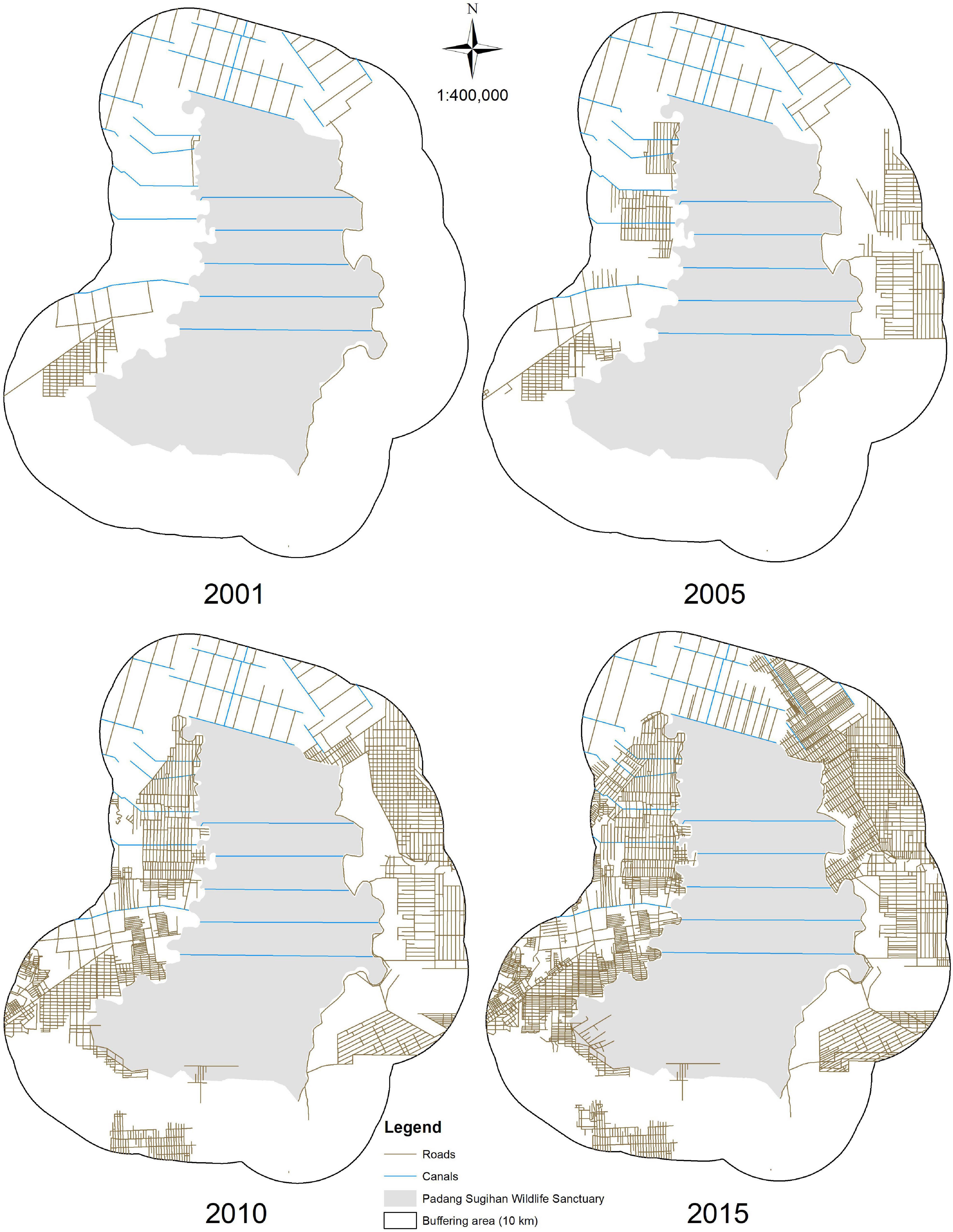
Figure 2. Road and canal development during 2001–2020 surrounding and within PSWR, Sumatra, Indonesia.
Temporal and Spatial Patterns of Fires
As expected, fire occurrence in PSWR and its surrounding buffer area was most frequent during the dry season from July to November (Figure 3). The role of precipitation dynamics on fire emergence was clearly shown, with a delay between the monthly precipitation decrease in July followed by increasing numbers of wildfires from August to September (Figure 3). Only a few anomalies of high fire occurrence were observed during May and December when wet conditions occurred. Fires emerged soon after no rain occurred and up to 37 consecutive dry days, with 8 days as median. The duration of fire events within a 1 × 1 km2 pixel (n = 180) was between 1 and 14 days, with a median of 1 day. A steady reduction in the number of fires occurred as rainfall increases during October to early November (Figure 3). Wetland shrubs which represent natural peat-swamp forest vegetation have high number of fires on both within and surrounding the reserve. While meadow which corresponds with grassland has higher number of fires within the reserve, forest plantation has the highest number of fires among other land use within and in the buffer area during 2001–2020 (Table 2).
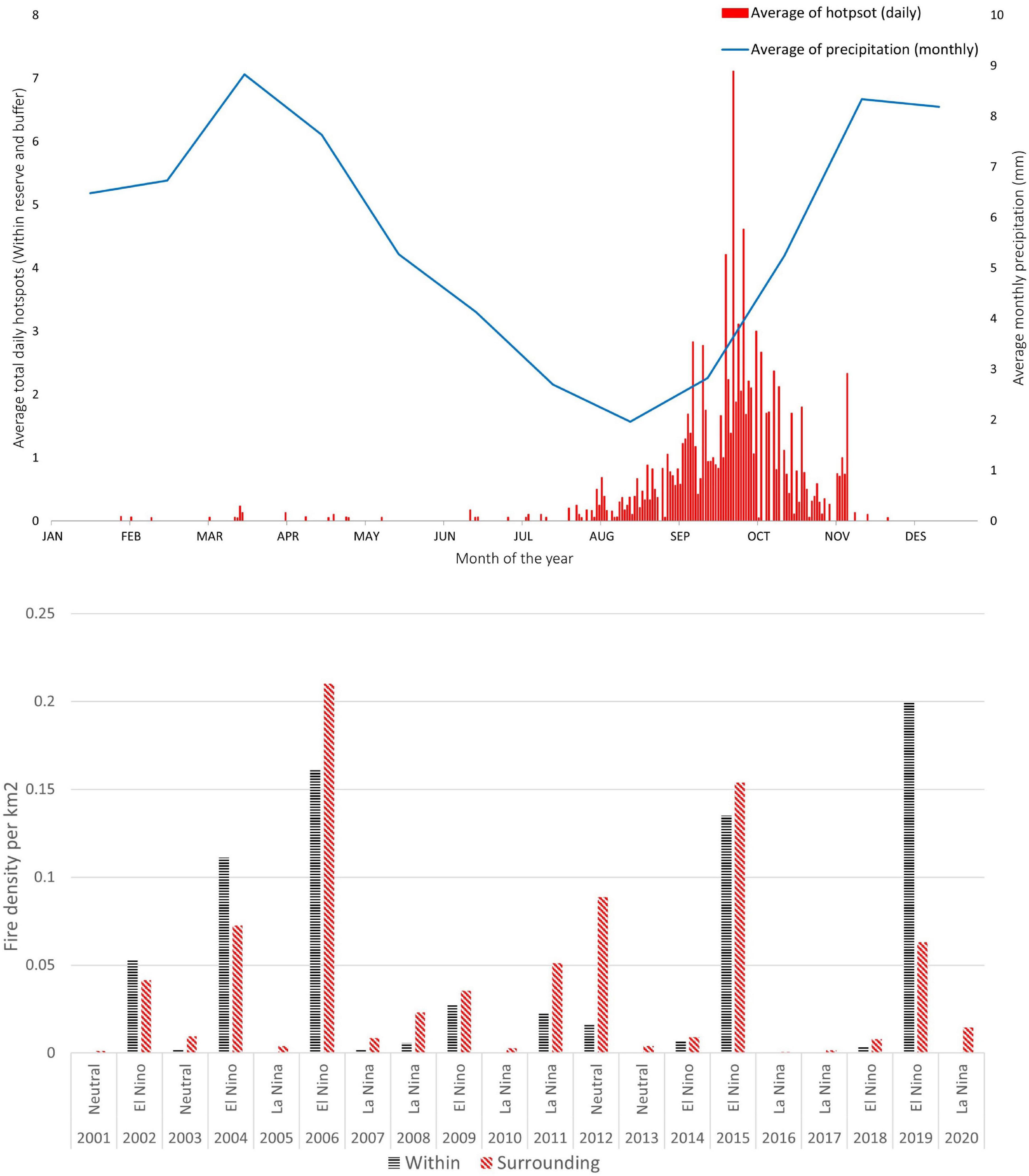
Figure 3. The temporal relationship between the average total daily hotspots (within reserve and buffer) and the monthly average precipitation during 2001–2020 (above); and fire densities within and surrounding the PSWR during 2001–2020, with years classified based on El Niño Southern Oscillation (ENSO) state (below).
Fire density both within and surrounding the reserve shows clear association with ENSO state (Figure 3), with the highest fire incidences all occurring in El Niño years (2006, 2019, 2015, 2004, and 2002), and generally lower prevalence in ENSO neutral and La Niña years (with 2011 and 2012, respectively, being small anomalies for these two states). Fire densities were lower within the PSWR than in the surrounding buffer area except in the three dry El Niño years of 2002, 2004, and 2019. Spearman correlation analysis showed a significant negative relationship between monthly rainfall and fire densities within the reserve, in the buffer and within both areas combined (Table 3).
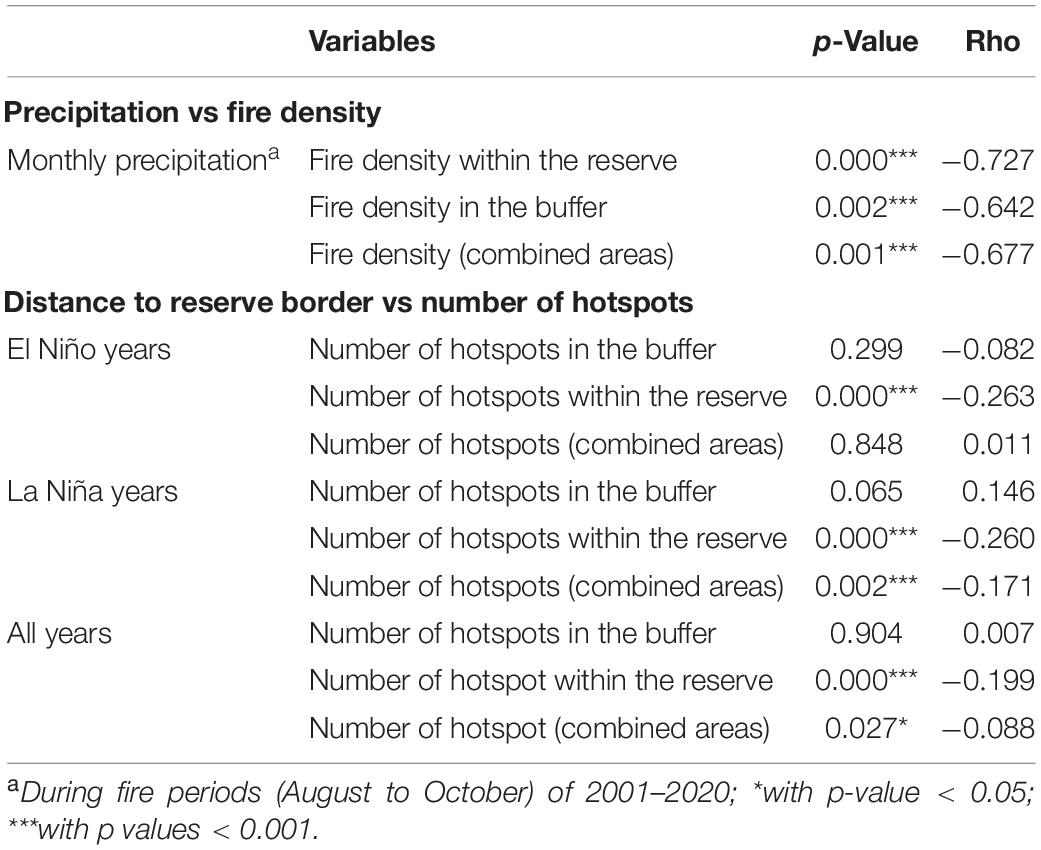
Table 3. Spearman correlation between monthly precipitation during fire periods (August–October) of 2001–2020, distance from border and number of hotspots in the PSWR and surrounding areas of Sumatra, Indonesia.
During the study period, fire generally only occurred once within fire-affected pixels, both within and surrounding PSWR, with fewer grid cells in which fire occurred twice or more, and only a few burnt more than seven times. Fire frequencies were significantly lower within the reserve, where only four repeated fires occurred at most (Figure 4).
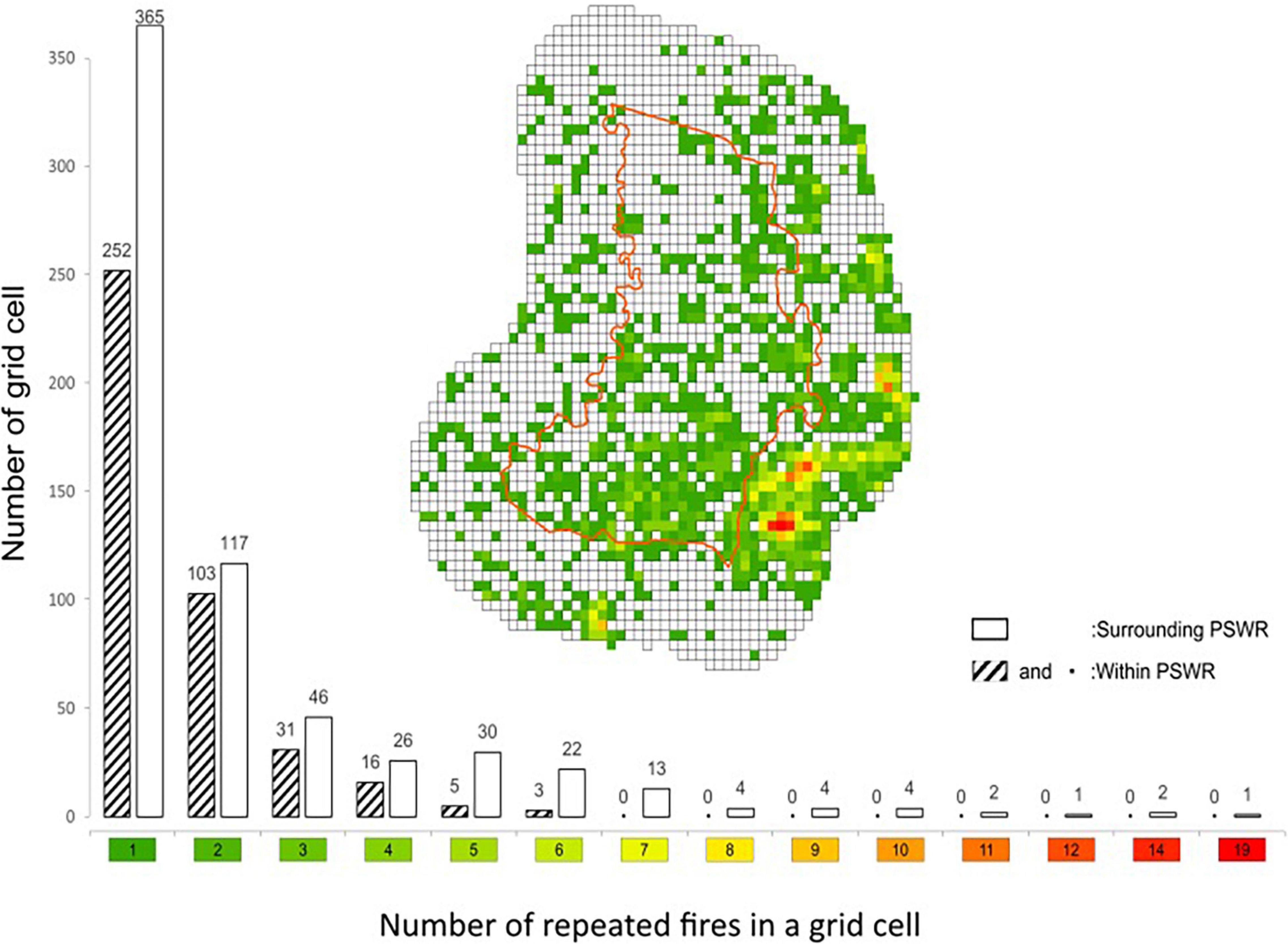
Figure 4. Number of grid cells at each repeated fires grid cells within the Padang Sugihan Reserve and surrounding between 2001 and 2020.
During La Niña years, fires rarely occurred within the protected area, except for a low number in 2003, 2008, 2011, 2012, and 2014. During dry years, fire spread into the reserve. Human disturbance as represented by canal and road systems had a pronounced influence on fire occurrence during both dry and wet years, with a “reverse J” pattern indicating a decrease in fire incidence as the distance from canals and roads increased (Figure 5).
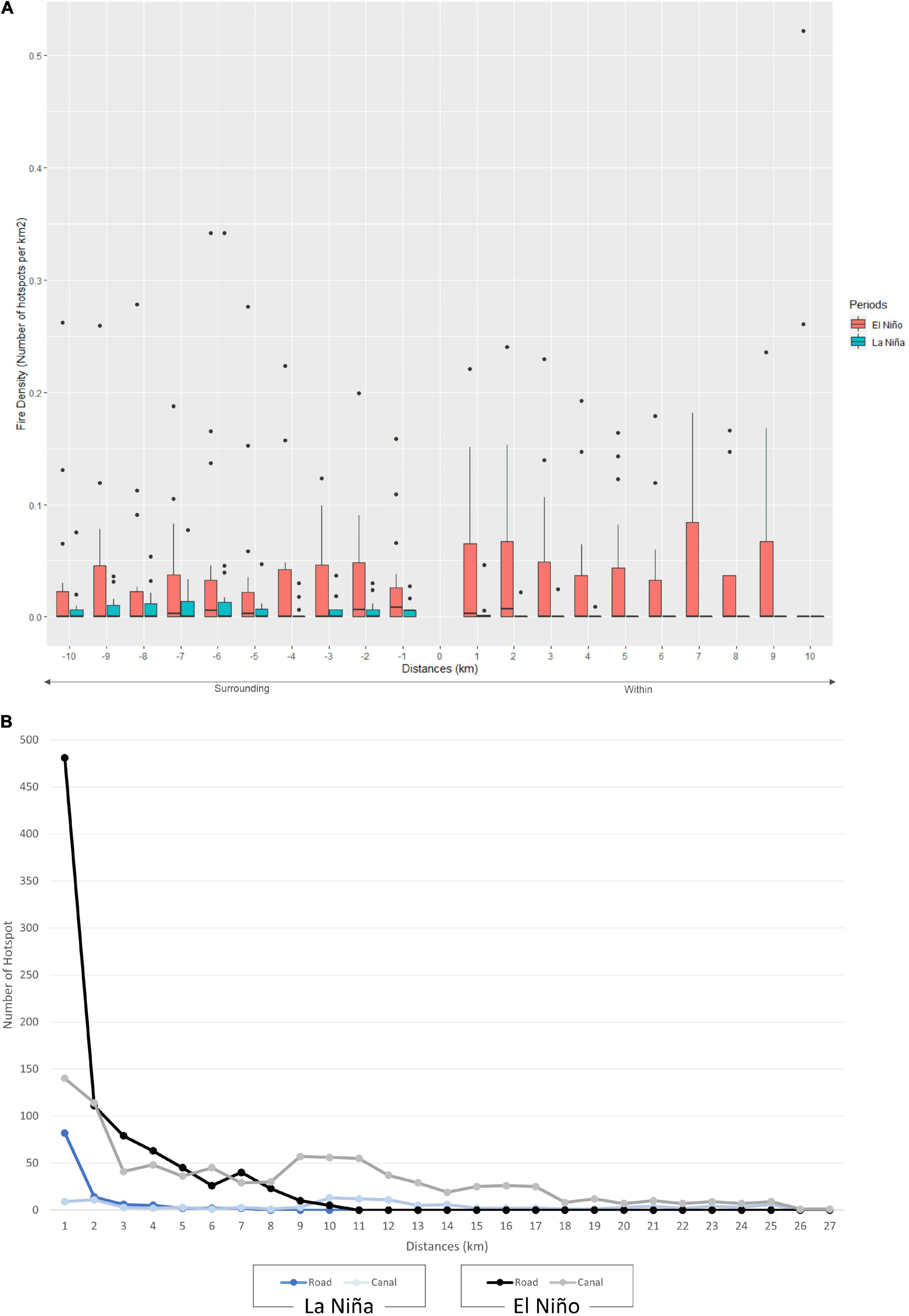
Figure 5. Boxplot of fire density at each distance form border during La Niña and El Niño years within and surrounding the reserve border (A) and frequency of hotspot as function of distance from canals and roads (B).
In the La Niña years, fire density was higher surrounding PSWR (Mann–Whitney U test, U = 176; p < 0.05), whereas in El Niño years fire incidence was not significantly different within the reserve compared to its buffer area (Mann–Whitney U test, U = 158; p > 0.05). Fire density decreased with increasing distance up to 6 km from the reserve border to the core during the wet years, but then the density increases (Figure 5A). Despite the Spearman correlation analysis was significantly showing negative relationship between distance from border within the reserve during La Niña years, it has lower strength (Table 3). Conversely, in El Niño years, no clear pattern was evident, both within the reserve and in the buffer area (Table 3).
During El Niño years, our Maxent model performance was insufficient to predict the presence of fire in the PSWR and surrounding area, while it was acceptable (AUC > 0.7) to predict the presence of fire with distance to canal as the highest contributors to the fire presence during La Niña years (Table 4).
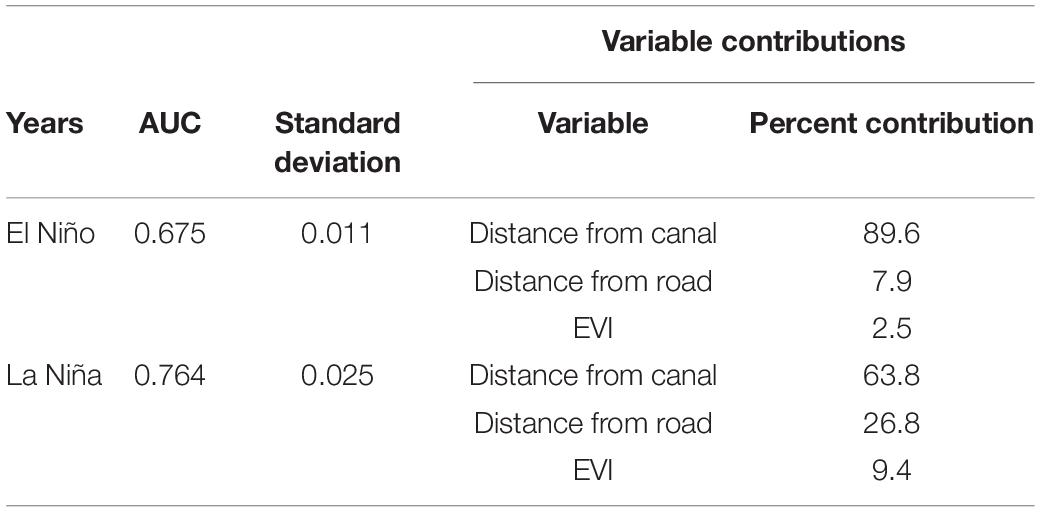
Table 4. Area under the curve (AUC) values, standard deviation and percent contribution of each environmental variables for the probability of fire presence in PSWR and surrounding landscape during El Niño and La Niña years from 2001 to 2020 using the Maximum Entropy Model.
Our predictive Maxent model clearly shows a sudden shift of reduced size of low fire-risks probability class within the PSWR from La Niña years (83.1%) to El Niño years (0.2%). In contrast, a sharp increase was shown from La Niña years to El Niño years for the middle fire-risks probability class (11–63%) and high fire-risks probability (5.7–36.7%) within the PSWR. Whereas during El Niño years, a significant reduction of the area with low fire-risk probability was shown in the surrounding of the reserve, which was compensated higher values for high and medium fire prone probability (Figure 6). Our findings highlight that surrounding of the PSWR is continuously under the threat of fire both during El Niño and La Niña years, while within PSWR can protect some areas during wet years but not – or at least to a much lesser degree – during dry years.
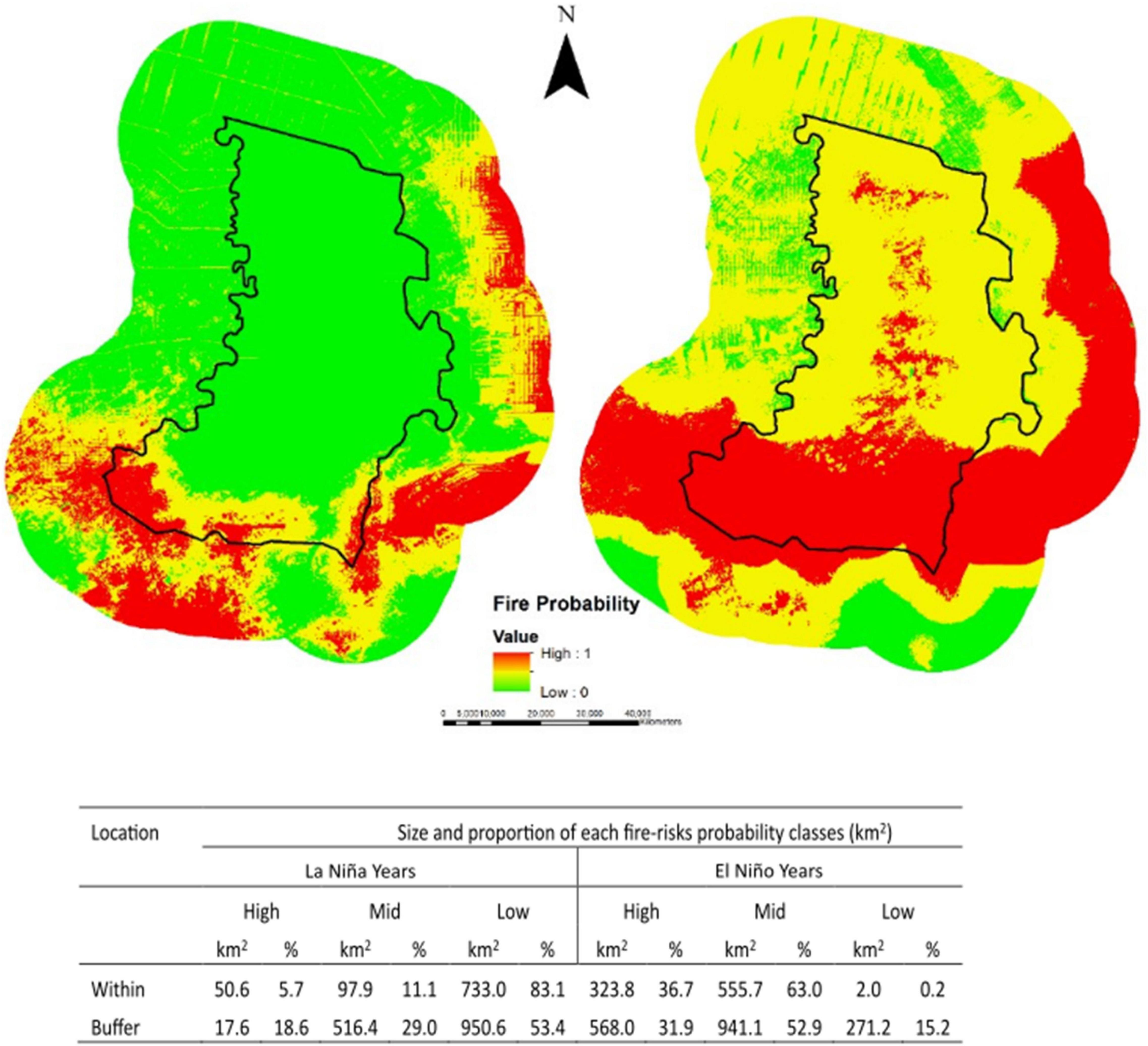
Figure 6. Predicted map of fire-risks probability class for La Niña (left) and El Niño (right) from the Maximum Entropy Model (Maxent) model. The probability of fire occurrence is represented by different colors ranging from red (high) to green (low). The size and proportion of each classes within the PSWR and it’s buffer area during is shown below.
Discussion
Various studies on the effectiveness of protected areas to mitigate damaging wildfires exist, conducted mostly at a large spatial scale (Adeney et al., 2009; Nelson and Chomitz, 2011; Rodríguez et al., 2013; Biswas et al., 2015; Kiely et al., 2021). Our approach to analyzing peat-fire occurrences at a local scale offers new insight into the detailed temporal and spatial patterns of fires within and surrounding a reserve and serves as the first local-specific case for a protected peatland area in Sumatra. Through this, we demonstrate that climatic variation is not sufficient to fully explain fire emergence within and surrounding this protected tropical peatland area, and that both top-down (seasonal variation) and bottom-up (human disturbance) mechanisms have a crucial influence on patterns of peat fires occurrences, and thus the reserve’s effectiveness in reducing fires.
Climatic anomalies such as the ENSO and the Indian Ocean Dipole contribute significantly to fire incidences in Indonesia (Field et al., 2009). We have demonstrated such top-down factors act as a causal explanation of fire occurrences, with clear differences in fire regimes between dry and wet years, which contributes substantially to fire-risks area predictive map development. During La Niña years, the reserve area was seemingly able to limit fire occurrence. However, in El Niño years, the presence of protected area borders as a mean of land-use management was seemingly ineffective in preventing burning events (Moreira et al., 2011), with no significant difference in fire occurrence observed between peatland within the reserve compared to the surrounding buffer area. Therefore, early-warning map development systems should consider the precipitation dynamics in both wet and dry years.
Our findings align with earlier research non-peatland areas (Archibald et al., 2010) indicating that the accumulated rainfall and the length of the dry season influence the annual area burned in a protected area. While at laboratory scales of peatlands, Lin et al. (2020) emphasized the roles of precipitation on extinguishing fire in this ecosystems. From the bottom-up perspective our results show that the distance to both roads and especially canals are important factors determining the occurrence of fire, both in wet and dry years. The construction of canals and roads facilitates human incursion into (protected) areas, and previous studies have demonstrated positive links between both fire occurrence and distance to roads (Sze et al., 2019), and fire frequency and distance to canals (Konecny et al., 2016) in tropical peatlands. In addition to facilitating human access, canals dug in tropical peatlands also lower the peat water level, thus drying the peat and further increasing its vulnerability to fire ignition and spreading (Wösten et al., 2008; Hooijer et al., 2010; Hoscilo et al., 2011; Hayasaka et al., 2014). Canals also have an essential influence on fire during dry years as waterways give access to fire, representing the human-induced activities (Hope et al., 2005; Field et al., 2009) including forest conversion into plantations (Prabowo et al., 2017).
Vegetation indices derived from satellite images have been commonly used for measuring fire fuel availability, which can also be used as a vegetation indicator for its health and susceptibility to fire (Fiorucci and Gaetani, 2007). In our study, during dry years, soil moisture content is expected to be decreased, thus affecting vegetation health: NDVI and EVI values are correspondingly very low and are thus likely to be additional factors affecting the vulnerability of the peat area to fire (Fiorucci and Gaetani, 2007). Yet our study has not explored whether any seasonal changes of NDVI influence the susceptibility to fire due to limited amount of clear satellite images (i.e., less than 10% cloud cover) obtained per-season. Due to this lack of multitemporal NDVI series, we could not ascertain vegetation phenology and thus the complex interactions between vegetation, land use and climate characteristics cannot be explained (Bajocco et al., 2015).
We collected environmental variables which are closest to the same year of the fire (except for EVI), but were unable to obtain and analyze trends in relation to fire occurrence at finer temporal resolutions. Vegetation seasonal variability has been shown to help to identify the primary bioclimatic drivers related to fuel dynamics (Bajocco et al., 2015) and obtaining high-quality resolution of the multi-spatiotemporal image to depict the role of vegetation biomass can provide a substantial contribution to understanding spatial patterns of fire (Palacios-Orueta et al., 2005). Such analyses at finer temporal resolution would therefore be useful in future tropical peatland-protected area studies, through observations of these variables prior to a burning event to aid in determining cause-effect relationships between vegetation indexes and fire occurrences.
The fire observed occurrences showed a delayed response toward reduced precipitation at the onset of the dry season during 2001–2020 in the PSWR. The high fire occurrence during La Niña years in 2008 and 2011 indicates that natural drought processes could not fully explain fires in the reserve. Since fires occurred during the La Nina years, further investigation toward the role of rainfall to reduce fire-risks probability at landscape level, which already done at laboratory level (Lin et al., 2020), will enhance our understanding on this factor. In addition, other factors such as roles of decision of humans to igniting fires during wet years still relevant to be investigated. We were not able to simulate both top and below-ground fire spreading mechanisms, and further exploration of farmers decision-making process to burn land for agricultural conversion is therefore required, as fire is commonly employed as a tool in agricultural-conflict scenarios pertaining to land rights/ownership (Stolle et al., 2003; Cochrane, 2009; Harrison et al., 2009, 2020).
We rarely found repeated fire in an area during the 20-year period, with very few recurrent fires in the same grid cell. Among three main clusters with high repeated fire incidence, we found out that these were dominated by six land use land cover types representing human modified land cover land use types, i.e., agriculture plantation, barren, forest plantation, meadow, shrubs, and wetland shrubs. It is commonly believed that isochronal fire is a function of land conversion into agricultural uses (Hoscilo et al., 2011) and high number of new fires and low number of recurrent fires implies that degradation of the PSWR only occurred recently, as also indicated by the expansion of roads in the area in Figure 2. Commonly fire use by local people only occurs when initially opening/preparing land during the onset of the planting period (Stolle et al., 2003; Cochrane, 2009; Harrison et al., 2009) and therefore cessation of new land conversion may have prevented recurrent fires from being ignited (Weltzin et al., 2003). Our use of 1 × 1 km2 grid cells could over or under estimate of the reoccurring fire phenomenon, and experimenting with different grid cell sizes as well as expanding the observations to a landscape level, involving larger areas might improve the precision of this analysis.
Our observations suggest that canals and roads as a proxy of human presence serve as valuable predictors of fire occurrence in our study area, as human presence has also been found elsewhere (Román-Cuesta and Martínez-Vilalta, 2006; Adeney et al., 2009; Konecny et al., 2016). Although we have shown land cover and land use map as proxy to identify source of fire, however, it requires additional further analysis using more study case to investigate effect of this variable on fire occurrence (Hoscilo et al., 2011; Pereira et al., 2012; Palumbo, 2013). Additionally research should seek to ascertain the motivations of anthropogenic land burning (Mistry and Bizerril, 2011) to aid in understanding the role of humans in dry year fire amplification (Adeney et al., 2009; Field et al., 2009; Spessa et al., 2009).
Previous studies in tropical peatlands have described negative impacts of fire on floral and faunal biodiversity (e.g., Yeager et al., 2003; Thornton et al., 2018; Harrison et al., 2021). Our observation of fire presence within the PSWR during both wet and mostly dry years therefore indicates that the reserve’s biodiversity is under serious threat through fire-induced habitat destruction and isolation within their boundaries. Responses of biodiversity, such as mammals, to and following fire incidences provides insight of the reserve’s effectiveness in maintaining such biodiversity, which was unfortunately beyond the scope of the present study. A particular emphasis on the behavioral response of Sumatran elephants as a flagship species in PSWR, using GPS collaring (Mossbrucker et al., 2016) and population projection studies (Imron et al., 2011) would provide important insight into the effect of fire on endangered species conservation in the reserve and more widely across peatlands and other lowland habitats of Sumatra. Furthermore, population viability analysis for the elephant in the reserve and surrounding area using a modeling approach (Grimm and Wissel, 2004; Hoscilo et al., 2011; Imron, 2011) will provide guidance for management alternatives, which are currently lacking such data.
This study has provided insights on spatial and temporal fire occurrence predictors, including human interference and climatic variations. Nevertheless, knowledge on the detailed mechanism of fire occurrence and its causal factors in this area remains incomplete. Individual/Agent-based modeling (IBM/ABM) is one promising approach to understanding the complex phenomenon of wildfire, as well as predicting fire-risks areas in the future (Berec, 2002; Grimm and Railsback, 2005; Karsai et al., 2016). An ABM was recently developed for tropical peatlands in Sumatra (Widyastuti et al., 2021) and thus could potentially facilitate planning of preventative measures. Further development of such models would be beneficial for applied science development on fire ecology and management. Predictions from such dynamic models, incorporating bottom-up and top-down processes, would allow better understanding of these complex processes, thus providing dynamic information applicable to fire management and mitigation policy development. Since vegetation and social conditions are highly dynamic in tropical peatlands (Cochrane, 2009), using such dynamic models with relatively short temporal scales will enhance predictions derived through this approach.
Implication for Protected Area Management
Protected areas in Sumatra have shown effectiveness in lowering deforestation rates in comparison to adjacent unprotected areas during years of 1990–2000 (Gaveau et al., 2009). However, our study shows that a protected area in a Sumatran peatland ecosystem was not devoid of dry season fires. Our results also indicate that the surrounding landscape becomes critical for the protected areas if adjacent buffer areas cannot contain fire occurrences. The surrounding area is thus likely to be key in mitigating fire, particularly during dry years. In addition, raising awareness of people surrounding the reserve to use fires wisely and practice sustainable peatland management, and supporting the work of Fire-Concerned Community (Masyarakat Peduli Api) in each village surrounding the reserve should be improved through collaboration with local government and private sectors. This will be crucial to the effectiveness of the PSWR to mitigate future fire incidences. In addition, a current measure on closing canals have been done as part of restoration efforts by the Indonesian Peatland and Mangrove Restoration Agency, which will hopefully help decrease future fire prevalence in the area. Our approach on assessing effectiveness of protected areas can be adopted to evaluate the benefit of such hydrological restoration efforts in the future (Kiely et al., 2021).
The PSWR plays a crucial role for biodiversity conservation in Indonesia as habitat for the critically endangered Sumatran Elephant. This subspecies of elephant has a large home range (Mossbrucker et al., 2016) and consequently needs access to various habitat types and a large overall habitat areas. The landscape approach of considering composition and quality of land surrounding a protected area has emerged recently in biodiversity conservation in Sumatra (Imron et al., 2011), and urgent implementation has been recommended. Encouraging the establishment of privately protected areas (Hermawan et al., 2015) and village-based protected areas such as a sacred forest (Silvianingsih et al., 2021) in the area surrounding the reserve may therefore enhance the effectiveness of biodiversity conservation and fire mitigation in the region. In the case of fire incidents within the PSWR, management should ensure safe areas from fire as a wildlife refugia system are present (Wilkin et al., 2016).
Since the PSWR is also surrounded by canal systems and rivers for local transportation, access to the reserve is relatively easy. In light of our findings indicating significant relationships between fire incidence and distance to roads and canals, any proposals to change the reserve into mixed-used protected areas to deal with pressure from agricultural and timber extraction (Nelson and Chomitz, 2011) should therefore be carefully considered. Attention to evaluate the effectiveness of the BKSDA Sumatera Selatan to manage the reserve (Jaenicke et al., 2010; Greve et al., 2011) with particular emphasis on fire management should also be considered, prior to contemplating changing the status of protected areas into other protection types or other land use types. Strengthening the ability of rangers to detect and handle fires when they occur will also enhance the effectiveness of protected areas’ management. Simultaneously peatland restoration using physical approaches such as re-wetting through canal blocking and other methods (Restrepo et al., 2013; Blackham et al., 2014; Ritzema et al., 2014; Lampela et al., 2017) will help reduce future fire incidences.
Data Availability Statement
Publicly available datasets were analyzed in this study, with no new data collected. This data can be found here: https://id.weatherspark.com/h/y/149125/2015/Cuaca-Historis-selama-2015-di-Sultan-Mahmud-Badaruddin-II-Airport-Indonesia#Figures-Rainfall, www.openstreetmap.org, https://balaiksdasumsel.org/, https://tanahair.indonesia.go.id/portal-web, https://earthdata.nasa.gov/earth-observation-data/near-real-time/firms/active-fire-datahttps://landsat.usgs.gov, and https://ggweather.com/enso/oni.htm.
Author Contributions
MI: conceptualizing, designing, writing, and review the manuscript. KW: writing and review the manuscript. DA and SP: data analysis. RS: data collection. WP: data collection and analysis. HS: conceptualizing. BS: conceptualizing and designing. MH: conceptualizing, writing, and proofread. UB: conceptualizing and writing. All authors contributed to the article and approved the submitted version.
Funding
The Lembaga Pengelola Dana Pendidikan (LPDP), the Indonesian Science Fund (DIPI) and UKRI GCRF (Grant No.: NE/T010401/1) provided financial support for this work.
Conflict of Interest
The authors declare that the research was conducted in the absence of any commercial or financial relationships that could be construed as a potential conflict of interest.
Publisher’s Note
All claims expressed in this article are solely those of the authors and do not necessarily represent those of their affiliated organizations, or those of the publisher, the editors and the reviewers. Any product that may be evaluated in this article, or claim that may be made by its manufacturer, is not guaranteed or endorsed by the publisher.
Acknowledgments
Balai Konservasi Sumber Daya Alam (BKSDA) Sumatera Selatan provided the SIMAKSI entry permit to the Padang Sugihan Reserve.
Footnotes
- ^ https://tanahair.indonesia.go.id/portal-web
- ^ https://balaiksdasumsel.org
- ^ https://earthdata.nasa.gov/earth-observation-data/near-real-time/firms/active-fire-data
- ^ https://id.weatherspark.com/h/y/149125/2015/Cuaca-Historis-selama-2015-di-Sultan-Mahmud-Badaruddin-II-Airport-Indonesia#Figures-Rainfall
- ^ https://landsat.usgs.gov/
References
Adeney, J. M., Christensen, N. L Jr., and Pimm, S. L. (2009). Reserves protect against deforestation fires in the Amazon. PLoS One 4:e5014. doi: 10.1371/journal.pone.0005014
Aragao, L. E., and Shimabukuro, Y. E. (2010). The incidence of fire in amazonian forests with implications for REDD. Science 328:1275. doi: 10.1126/science.1186925
Archibald, S., Nickless, A., Govender, N., Scholes, RJ., and Lehsten, V. (2010). Climate and the inter-annual variability of fire in southern Africa: a meta-analysis using long-term field data and satellite-derived burnt area data. Global Ecol. Biogeography 19, 794–809. doi: 10.1111/j.1466-8238.2010.00568.x
Atwood, E. C., Englhart, S., Lorenz, E., Halle, W., Wiedemann, W., Siegert, F., et al. (2016). Detection and characterization of low temperature peat fires during the 2015 fire catastrophe in indonesia using a new high- sensitivity fire monitoring satellite sensor (FireBird). PLoS One 11:e0159410. doi: 10.1371/journal.pone.0159410
Bajocco, S., Dragoz, E., Gitas, I., Smiraglia, D., Salvati, L., Ricotta, C., et al. (2015). Mapping forest fuels through vegetation phenology : the role of coarse- mapping forest fuels through vegetation phenology : the role of coarse-resolution satellite time-series. PLoS One 10:e0119811. doi: 10.1371/journal.pone.0119811
Ballhorn, U., Siegert, F., Mason, M., and Limin, S. (2009). Derivation of burn scar depths and estimation of carbon emissions with LIDAR in Indonesian peatlands. Proc. Natl. Acad. Sci. U S A. 106, 21213–21218. doi: 10.1073/pnas.0906457106
Berec, L. (2002). Techniques of spatially explicit individual-based models: construction, simulation, and mean-field analysis. Ecol. Model. 150, 55–81. doi: 10.1016/s0304-3800(01)00463-x
Biswas, S., Vadrevu, K. P., Lwin, Z. M., Lasko, K., and Justice, C. O. (2015). Factors controlling vegetation fires in protected and non-protected areas of myanmar. PLoS One 10:e0124346. doi: 10.1371/journal.pone.0124346
Blackham, G. V., Webb, E. L., and Corlett, R. T. (2014). Natural regeneration in a degraded tropical peatland, Central Kalimantan, Indonesia : implications for forest restoration. Forest Ecol. Manag. 324, 8–15. doi: 10.1016/j.foreco.2014.03.041
Brown, L. E., Holden, J., Palmer, S. M., Johnston, K., Sorain, J. R., and Grayson, R. (2015). Effects of fire on the hydrology, biogeochemistry, and ecology of peatland river systems. Freshwater Sci. 34, 1406–1425. doi: 10.1086/683426
Cattau, M. E., Harrison, M. E., Shinyo, I., Tungau, S., Uriarte, M., and DeFries, R. (2016) Sources of anthropogenic fire ignitions on the peat-swamp landscape in Kalimantan, Indonesia. Glob. Environ. Change 39, 205–219. doi: 10.1016/j.gloenvcha.2016.05.005
Chen, F., Du, Y., Niu, S., and Zhao, J. (2015). Modeling forest lightning fire occurrence in the daxinganling mountains of Northeastern China with MAXENT. Forests 6, 1422–1438. doi: 10.3390/f6051422
CIFOR Forests News (2014). New Maps Reveal More Complex Picture of Sumatran Fires. Available online at: https://forestsnews.cifor.org/23479/new-maps-reveal-more-complex-picture-of-sumatran-fires?fnl=en (accessed March 19, 2022).
Cochrane, M. A. (2009). Tropical Fire Ecology Climate Change, Landuse and Ecosystem DynamicsBooks i En. Berlin: Springer-Praxis:
Cole, L. E. S., Bhagwat, S. A., and Willis, K. J. (2015). Long-term disturbance dynamics and resilience of tropical peat swamp forests. J. Ecol. 103, 16–30. doi: 10.1111/1365-2745.12329
dan Kehutanan, K. L. H. (2021). SIMONTANA. Available online at: https://nfms.menlhk.go.id/peta (accessed December 1, 2021).
Daniau, A. L., D’Errico, F., and Goñi, M. F. S. (2010). Testing the hypothesis of fire use for ecosystem management by neanderthal and upper palaeolithic modern human populations. PLoS One 5:e9157. doi: 10.1371/journal.pone.0009157
Elvidge, C. D., Zhizhin, M., Hsu, F-C., Baugh, K., Khomarudin, M. R., Vetrita, Y., Sofan, P., et al. (2015). Long-wave infrared identification of smoldering peat fires in Indonesia with nighttime Landsat data. Environ. Res. Lett. 10:065002. doi: 10.1088/1748-9326/10/6/065002
Field, R. D., Spessa, A., Aziz, N., Camia, A., Cantin, A., Carr, R., et al. (2015). Development of a global fire weather database. Nat. Hazards Earth System Sci. 15, 1407–1423. doi: 10.5194/nhess-15-1407-2015
Field, R. D., van der Werf, G. R., and Shen, S. S. P. (2009). Human amplification of drought-induced biomass burning in Indonesia since 1960. Nat. Geosci. 2, 185–188. doi: 10.1038/ngeo443
Fiorucci, P., and Gaetani, F. (2007). Dynamic fire danger mapping from satellite imagery and meteorological forecast data. Earth Interact. 11, 1-17.
Gaveau, D. L. A., Epting, J., Lyne, O., Linkie, M., Kumara, I., Kanninen, M. et al. (2009). Evaluating whether protected areas reduce tropical deforestation in Sumatra. J. Biogeography 36, 2165–2175. doi: 10.1111/j.1365-2699.2009.02147.x
Grégoire, J. M., and Simonetti, D. (2010). Interannual changes of fire activity in the protected areas of the SUN network and other parks and reserves of the West and Central Africa region derived from MODIS observations. Remote Sensing 2, 446–463. doi: 10.3390/rs2020446
Greve, M. Ã., Chown, S. L., Van Rensburg, B. J., Dallimer, M., and Gaston, K. J. (2011). The ecological effectiveness of protected areas : a case study for South African birds. Animal Conservation 14, 295–305. doi: 10.1111/j.1469-1795.2010.00429.x
Grimm, V., and Railsback, S. (2005). Individual-based Modeling and Ecology. Page Individual-based Modeling and Ecology. Princeton, NJ: Princeton University Press
Grimm, V., and Wissel, C. (2004). The intrinsic mean time to extinction: a unifying approach to analysing persistence and viability of populations. Oikos 105, 501–511. doi: 10.1111/j.0030-1299.2004.12606.x
Harrison, M. E., Nasir, D., Healy, W., Kulu, I. P., Husson, S. J., Santiano, P. A., et al. (2021). “The importance of monitoring research in assessing impacts of anthropogenic activities on tropical peatland biodiversity: examples from Central Kalimantan, Indonesia,” in Proceedings of the 16th International Peatland Congress. Peatlands and Peat - Source of Ecosystem Services. Abstract Book: Oral Presentations (Tallinn: International Peat Society), 44–50.
Harrison, M. E., Ottay, J. B., D’Arcy, L. J., Cheyne, S. M., Anggodo Belcher, C., et al. (2020). Tropical forest and peatland conservation in Indonesia : challenges and directions. People Nat. 2, 4–28.
Harrison, M. E., Page, S. E., and Limin, S. H. (2009). The global impact of Indonesian forest fires. Biologist 56, 156–163.
Hayasaka, H., Noguchi, I., Putra, E. I., Yulianti, N., and Vadrevu, K. (2014). Peat-fire-related air pollution in Central Kalimantan, Indonesia. Environ. Pollut. 195:257–266. doi: 10.1016/j.envpol.2014.06.031
Hayasaka, H., Takahashi, H., Limin, S. H., Yulianti, N., and Usup, A. (2016). “Peat Fire occurrence,” in Tropical Peatland Ecosystems, eds M. Osaki and Tsuji, N. (Tokyo: Springer). doi: 10.1007/978-4-431-55681-7_25
Hermawan, M. T. T., Baiquni, M., and Imron, M. A. (2015). Perlindungan ruang jelajah banteng dalam kesenjangan sistem kawasan konservasi di Kabupaten Banyuwangi Propinsi Jawa Timur. J. Ilmu Kehutanan 6, 103–110.
Hooijer, A., Page, S., Canadell, J. G., Silvius, M., Kwadijk, J., Wösten, H. et al. (2010). Current and future CO2 emissions from drained peatlands in Southeast Asia. Biogeosciences 7, 1505–1514. doi: 10.5194/bg-7-1505-2010
Hope, G., Chokkalingam, U., and Anwar, S. (2005). The stratigraphy and fire history of the Kutai Peatlands, Kalimantan, Indonesia. Quaternary Res. 64, 407–417. doi: 10.1016/j.yqres.2005.08.009
Hoscilo, A., Page, S. E., Tansey, K. J., and Rieley, J. O. (2011). Effect of repeated fires on land-cover change on peatland in southern Central Kalimantan, Indonesia, from 1973 to 2005. Int. J. Wildland Fire 20, 578–588. doi: 10.1071/wf10029
Imron, M. A., Herzog, S., and Berger, U. (2011). The influence of agroforestry and other land-use types on the persistence of a Sumatran tiger (Panthera tigris sumatrae) population: an individual-based model approach. Environ. Manag. 48, 276–288. doi: 10.1007/s00267-010-9577-0
Imron, M. A. (2011). An Individual-based Model Approach for the Conservation of the Sumatran tiger Panthera tigris Sumatrae Population in Central Sumatra. Dresden: Technische Universität Dresden
Jaenicke, J., Wösten, H., Budiman, A., and Siegert, F. (2010). Planning hydrological restoration of peatlands in Indonesia to mitigate carbon dioxide emissions. Mitigation Adaptation Strategies Global Change 15, 223–239. doi: 10.1007/s11027-010-9214-5
Joppa, L. N., and Loarie, S. R., and Pimm, S. L. (2009). On population growth near protected areas. PLoS One 4:e4279. doi: 10.1371/journal.pone.0004279
Karsai, I., Roland, B., and Kampis, G. (2016). The effect of fire on an abstract forest ecosystem: an agent based study. Ecol. Complexity 28, 12–23. doi: 10.1016/j.ecocom.2016.09.001
Kiely, L., Spracklen, D. V., Arnold, S. R., Papargyropoulou, E., Conibear, L., Wiedinmyer, C. et al. (2021). Assessing costs of Indonesian fires and the benefits of restoring peatland. Nat. Commun. 12:7044. doi: 10.1038/s41467-021-27353-x
Kim, S. J., Lim, C., Kim, G. S., Lee, J., Geiger, T., Rahmati, O. et al. (2019). Multi-Temporal analysis of forest fire probability using socio-economic and environmental variables. Remote Sensing 11:86. doi: 10.3390/rs11010086
Konecny, K., Ballhorn, U., Navratil, P., Jubanski, J., Page, S. E., Tansey, K. et al. (2016). Variable carbon losses from recurrent fires in drained tropical peatlands. Global Change Biol. 22, 1469–1480. doi: 10.1111/gcb.13186
LaGro, J. A., Jr. (2005). “Land-use classification,” in Encyclopedia of Soils in the Environment D. Hillel ed. (Amsterdam: Elsevier).
Lampela, M., Jauhiainen, J., Sarkkola, S., and Vasander, H. (2017). Promising native tree species for reforestation of degraded tropical peatlands. Forest Ecol. Manag. 394, 52–63. doi: 10.1016/j.foreco.2016.12.004
Langner, A., Miettinen, J., and Siegert, F. (2007). Land cover change 2002-2005 in Borneo and the role of fire derived from MODIS imagery. Global Change Biol. 13, 2329–2340.
Lin, S., Kuen, Y., Xiao, Y., and Huang, X. (2020). Can rain suppress smoldering peat fi re ? Sci. Total Environ. 727:138468. doi: 10.1016/j.scitotenv.2020.138468
Linkie, M., Smith, R. J., and Leader-Williams, N. (2004). Mapping and predicting deforestation patterns in the lowlands of Sumatra. Biodiversity Conservation 13, 1809–1818. doi: 10.1023/b:bioc.0000035867.90891.ea
Miettinen, J., Hooijer, A., Wang, J., Shi, C., and Liew, S. C. (2012). Peatland degradation and conversion sequences and interrelations in Sumatra. Regional Environ. Change 12, 729–737. doi: 10.1007/s10113-012-0290-9
Miettinen, J., and Liew, S. C. (2010). Status of peatland degradation and development in sumatra and kalimantan. Ambio 39, 394–401. doi: 10.1007/s13280-010-0051-2
Miettinen, J., Wang, J., Hooijer, A., and Liew, S. (2013). Peatland conversion and degradation processes in insular Southeast Asia : a case study in Jambi, Indonesia. Land Degradation Dev. 24, 334–341. doi: 10.1002/ldr.1130
Mistry, J., and Bizerril, M. (2011). Why it is important to understand the relationship between people, fire and protected areas. Biodiversidade Bras 1, 40–49.
Moreira, F., Viedma, Q., Arianoutsou, M., Curt, T., Koutsias, N., Rigolot, E., et al. (2011). Landscape - wildfire interactions in southern Europe: implications for landscape management. J. Environ. Manage. 92, 2389–2402. doi: 10.1016/j.jenvman.2011.06.028
Mossbrucker, A. M., Fleming, C. H., Imron, M. A., and Pudyatmoko, S. (2016). AKDEc home range size and habitat selection of Sumatran elephants. Wildlife Res. 43, 566–575. doi: 10.1071/wr16069
Nash, S. V., and Nash, A. D. (1986). The elephants of padang-sugihan, Sumatra. Tigerpaper (FAO/RAPA) 13, 28–29.
Nelson, A., and Chomitz, K. M. (2011). Effectiveness of strict vs. multiple use protected areas in reducing tropical forest fires: a global analysis using matching methods. PLoS One 6:e22722. doi: 10.1371/journal.pone.0022722
Page, S., Hosciło, A., Wösten, H., Jauhiainen, J., Silvius, M., Rieley, J., et al. (2009). Restoration ecology of lowland tropical peatlands in Southeast Asia: current knowledge and future research directions. Ecosystems 12, 888–905. doi: 10.1007/s10021-008-9216-2
Page, S. E., Wust, R. A. J., Rieley, J. O., Shotyk, W., and Limin, S. H. (2004). A record of late pleistocene and holocene carbon accumulation and climate change from an equatorial peat bog (Kalimantan, Indonesia): implications for past, present and future carbon dynamics. J. Quaternary Sci. 19, 625–635. doi: 10.1002/jqs.884
Palacios-Orueta, A., Chuvieco, E., Parra, A., and Carmona-Moreno, C. (2005). Biomass burning emissions: a review of models using remote-sensing data. Environ. Monit. Assess 104, 189–209. doi: 10.1007/s10661-005-1611-y
Palumbo, I. (2013). “The importance of fire ecology in protected areas management,” in Developments in Earth Surface Processes eds P. Palon D. O. Olago and C. T. Omuto, (Kenya : A Natural Outlook Geo-Environmental Resource Hazards)
Pereira, P., Mierauskas, P., Úbeda, X., Mataix-Solera, J., and Cerda, A. (2012). Fire in protected areas - the effect of protection and importanceof fire management. Environ. Res. Eng. Manag. 1, 52–62.
Posa, M. R. C. (2011). Peat swamp forest avifauna of Central Kalimantan, Indonesia: effects of habitat loss and degradation. Biol. Conservation 144, 2548–2556. doi: 10.1016/j.biocon.2011.07.015
Prabowo, D., Maryudi, A., and Senawi Imron, M. A. (2017). Conversion of forests into oil palm plantations in West Kalimantan, Indonesia: insights from actors’ power and its dynamics. Forest Policy Econ. 78:32–39. doi: 10.1016/j.forpol.2017.01.004
Presiden Republik Indonesia (2016). Peraturan Pemerintan Republik Indonesia Nomor 57 Tahun 2016 Tentang Perubahan Atas Peraturan Pemerintah No 71 Tahun 2014 Tentang Perlindungan dan Pengelolaan Ekosistem Gambut. Indonesia: Presiden Republik Indonesia
Putra, E. I., Hayasaka, H., Takahashi, H., and Usup, A. (2008). Recent peat fire activity in the Mega Rice Project area, Central Kalimantan, Indonesia. J. Disaster Res. 3, 334–341. doi: 10.20965/jdr.2008.p0334
Restrepo, M. F., Florez, C. P., Osorio, N. W., and León, J. D. (2013). Passive and active restoration strategies to activate soil biogeochemical nutrient cycles in a degraded tropical dry land. ISRN Soil Sci. 2013:461984.
Ritzema, H., Limin, S., Kusin, K., Jauhiainen, J., and Wösten, H. (2014). Canal blocking strategies for hydrological restoration of degraded tropical peatlands in Central Kalimantan, Indonesia. Catena 114, 11–20. doi: 10.1016/j.catena.2013.10.009
Rodríguez, N., Armenteras, D., and Retana, J. (2013). Effectiveness of protected areas in the colombian andes: deforestation, fire and land-use changes. Regional Environ. Change 13, 423–435. doi: 10.1007/s10113-012-0356-8
Román-Cuesta, R. M., and Martínez-Vilalta, J. (2006). Effectiveness of protected areas in mitigating fire within their boundaries: case study of Chiapas, Mexico. Conservation Biol. 20, 1074–1086. doi: 10.1111/j.1523-1739.2006.00478.x
Shiodera, S., Atikah, T. Setijo Rahajoe, J., Apandi, I., Seino, T., Haraguchi, A., et al. (2012). Impact of peat-fire disturbance to forest structure in tropical peat forest in Central Kalimantan, Indonesia. Hoppou Shinrin Kenkyu 60, 59–62.
Silvianingsih, Y. A., Hairiah, K., Suprayoga, D., and van Noordwijk, M. (2021). Kaleka agroforest in central Kalimantan (Indonesia)_ soil quality, hydrological protection of adjacent peatlands, and sustainability _ enhanced Reader.pdf. Land 10:856. doi: 10.3390/land10080856
Spessa, A., Weber, U., Langner, A., Siegert, F., and Heil, A. (2009). Fire in the vegetation and peatlands of Borneo, 1997-2007 : patterns, drivers and emissions. Assembly 11:8247.
Stocks, B. J., Van Wilgen, B. W., Trollope, W. S. W., McRae, D. J., Mason, J. A., and Weirich, F. PotgieterALFet al. (1996). Fuels and fire behavior dynamics on large-scale savanna fires in Kruger National Park, South Africa. J. Geophys. Res. Atmospheres 101, 23541–23550. doi: 10.1029/95jd01734
Stolle, F., Chomitz, K. M., Lambin, E. F., and Tomich, T. P. (2003). Land use and vegetation fires in Jambi Province, Sumatra, Indonesia. Forest Ecol. Manag. 179, 277–292. doi: 10.1016/s0378-1127(02)00547-9
Sumsel, B. (2021). Kementian Lingkungan Hidup dan Kehutanan Direktorat Jenderal Konservasi Sumberdaya Alam dan Ekosistem Balai Konservasi Sumber Daya Alam Sumatera Selatan. Available online at: http://balaiksdasumsel.org/halaman/detail/padang-sugihan (accessed November 27, 2019).
Thornton, S. A., Dudin, Page, S. E., Upton, C., and Harrison, M. E. (2018). Peatland fish of Sebangau, Borneo: diversity, monitoring and conservation. Mires Peat 22, 1–25.
Van Wilgen, B. W. (2009). The evolution of fire and invasive alien plant management practices in fynbos. South African J. Sci. 105, 335–342.
Weltzin, J. F., Bridgham, S. D., Pastor, J., Chen, J., and Harth, C. (2003). Potential effects of warming and drying on peatland plant community composition. Global Change Biol. 9, 141–151. doi: 10.1046/j.1365-2486.2003.00571.x
Widyastuti, K., Imron, M. A., Pradopo, S. T., Suryatmojo, H., Sopha, B. M., Spessa, A. et al. (2021). PeatFire: an agent-based model to simulate fire ignition and spreading in a tropical peatland ecosystem. Int. J. Wildland Fire 30, 71–89. doi: 10.1071/wf19213
Wilkin, K. M., Ackerly, D. D., and Stephens, S. L. (2016). Climate change refugia, fire ecology and management. Forests 7:77. doi: 10.3390/f7040077
Willis, K. S. (2015). Remote sensing change detection for ecological monitoring in United States protected areas. Biol. Conservation 182, 233–242. doi: 10.1016/j.biocon.2014.12.006
Wösten, J. H. M., Clymans, E., Page, S. E., Rieley, J. O., and Limin, S. H. (2008). Peat-water interrelationships in a tropical peatland ecosystem in Southeast Asia. CATENA 73, 212–224. doi: 10.1016/j.catena.2007.07.010
Keywords: wildlife conservation, peat fires, biodiversity conservation, humans effect, climatic variation, Sumatra
Citation: Imron MA, Widyastuti K, Al Bihad D, Satria RA, Prayoga W, Pradopo ST, Suryatmojo H, Sopha BM, Harrison ME and Berger U (2022) Beyond Climatic Variation: Human Disturbances Alter the Effectiveness of a Protected Area to Reduce Fires in a Tropical Peatland. Front. For. Glob. Change 5:788023. doi: 10.3389/ffgc.2022.788023
Received: 01 October 2021; Accepted: 08 March 2022;
Published: 14 April 2022.
Edited by:
Bastian Bertzky, European Commission, Joint Research Centre (JRC), ItalyReviewed by:
Xinyan Huang, Hong Kong Polytechnic University, Hong Kong SAR, ChinaSoo Chin Liew, National University of Singapore, Singapore
Copyright © 2022 Imron, Widyastuti, Al Bihad, Satria, Prayoga, Pradopo, Suryatmojo, Sopha, Harrison and Berger. This is an open-access article distributed under the terms of the Creative Commons Attribution License (CC BY). The use, distribution or reproduction in other forums is permitted, provided the original author(s) and the copyright owner(s) are credited and that the original publication in this journal is cited, in accordance with accepted academic practice. No use, distribution or reproduction is permitted which does not comply with these terms.
*Correspondence: Muhammad Ali Imron, maimron@ugm.ac.id