- 1Environment Department, Alcoa of Australia Ltd., Huntly Mine, Pinjarra, WA, Australia
- 2Environment and Conservation Sciences, Murdoch University, Perth, WA, Australia
- 3School of Agricultural Policy and Development, Department of Sustainable Land Management and Soil Research Centre for Agri-Environmental Research & Soil Research Centre, University of Reading, Reading, United Kingdom
Despite nutrient enrichment having widely reported negative impacts on biodiversity, fertilizer is routinely applied in post mining restoration to enhance plant growth and establishment. Focusing on surface mine restoration (predominately bauxite and mineral sands), we outline the long-term negative impacts of fertilizer, particularly phosphorus fertilizer, on plant community composition, species richness, fire fuel loads, and belowground impacts on nutrient-cycling. We draw from extensive research in south-western Australia and further afield, noting the geographical coincidence of surface mining, phosphorus impoverished soil and high plant biodiversity. We highlight the trade-offs between rapid plant-growth under fertilisation and the longer-term effects on plant communities and diversity. We note that the initial growth benefits of fertilisation may not persist in water-limited environments: growth of unfertilised forests can eventually match that of fertilised forest, throwing doubt on the premise that fertilisation is necessary at all.
Introduction
Negative impacts of nutrient enrichment on biodiversity in natural and semi-natural ecosystems are widely reported (e.g., Wheeler and Shaw, 1991; Wassen et al., 2005; Isbell et al., 2013). Consequently, for ecosystem restoration of human-modified and degraded landscapes, one of the first considerations is often how to reduce the legacy of nutrient enrichment. Approaches include soil stripping and removal to reduce nitrogen or phosphorus (hereafter N and P, respectively) concentrations (Diaz et al., 2008), applying carbon to reduce soil-N availability (Perry et al., 2010) or P-mining (sensu Schelflout et al., 2015).
However, adding fertilizer to stimulate plant growth is often a key aspect of post mining restoration and is recommended as best practice in policy guidelines (e.g., EPA, 1995; Minerals Council of South Africa, 2007; Tibbett, 2010; DFAT, 2016). Further, many formal closure criteria and regulatory conditions require outcomes such as minimum levels of plant cover (e.g., Kragt et al., 2019; Manero et al., 2021) – and applying fertilizer can help achieve these goals. While well meaning, there is a risk that these guidelines focus too narrowly on short-term outcomes such as cover, with scant consideration to longer-term impacts on plant community assembly.
Using examples largely drawn from restoring bauxite mines in the Jarrah Forest of Western Australia, we outline why fertilizer has been used in mine restoration and how application rates compare with mining related nutrient losses. We further outline the suboptimal outcomes that result from adding P using examples drawn from bauxite and mineral-sands mine restoration. We focus on surface mining of bauxite and mineral sands because the returned soil profile is often intact (e.g., Audet et al., 2013; Standish et al., 2015; Riviera et al., 2021), compared with hard rock mining where novel growth substates such as waste rock and process waste may be used. We acknowledge that the use of soil amendments and fertilizer inputs may be essential for overcoming initial barriers to vegetation establishment on novel substrates (e.g., Doley and Audet, 2013; Bateman et al., 2021). We also focus primarily on P, because P (unlike N) cannot be replaced by biological fixation, is often the most limiting nutrient in highly impoverished soils, and the source of P-input to restored soils only comes from fertilizer inputs (e.g., organic and inorganic fertilizers). Regolith weathering contributes P to soils too, but occurs over such impressively long time scales that it is not usually factored into restoration and management plans.
Surface mining often occurs in nutrient-poor, biodiverse landscapes
Bauxite occurs in ancient landscapes that have undergone significant periods of weathering and in situ leaching. Consequently, we tested whether bauxite and nutrient-deficient soils co-occur using a GIS analysis of the location of bauxite deposits (Mason and Arndt, 1996) combined with the location of ancient and deeply weathered nutrient-deficient soils downloaded from the FAO Soils Portal (i.e., Acrisols, Ferralsols, Lixisols, and Luvisols; FAO, 2012; Tibbett et al., 2019). A layer showing the world’s plant species richness was also added (Barthlott et al., 1999), although it should be noted that to improve visual clarity, we simplified the original 10 zones of increasing richness into three (Figure 1).
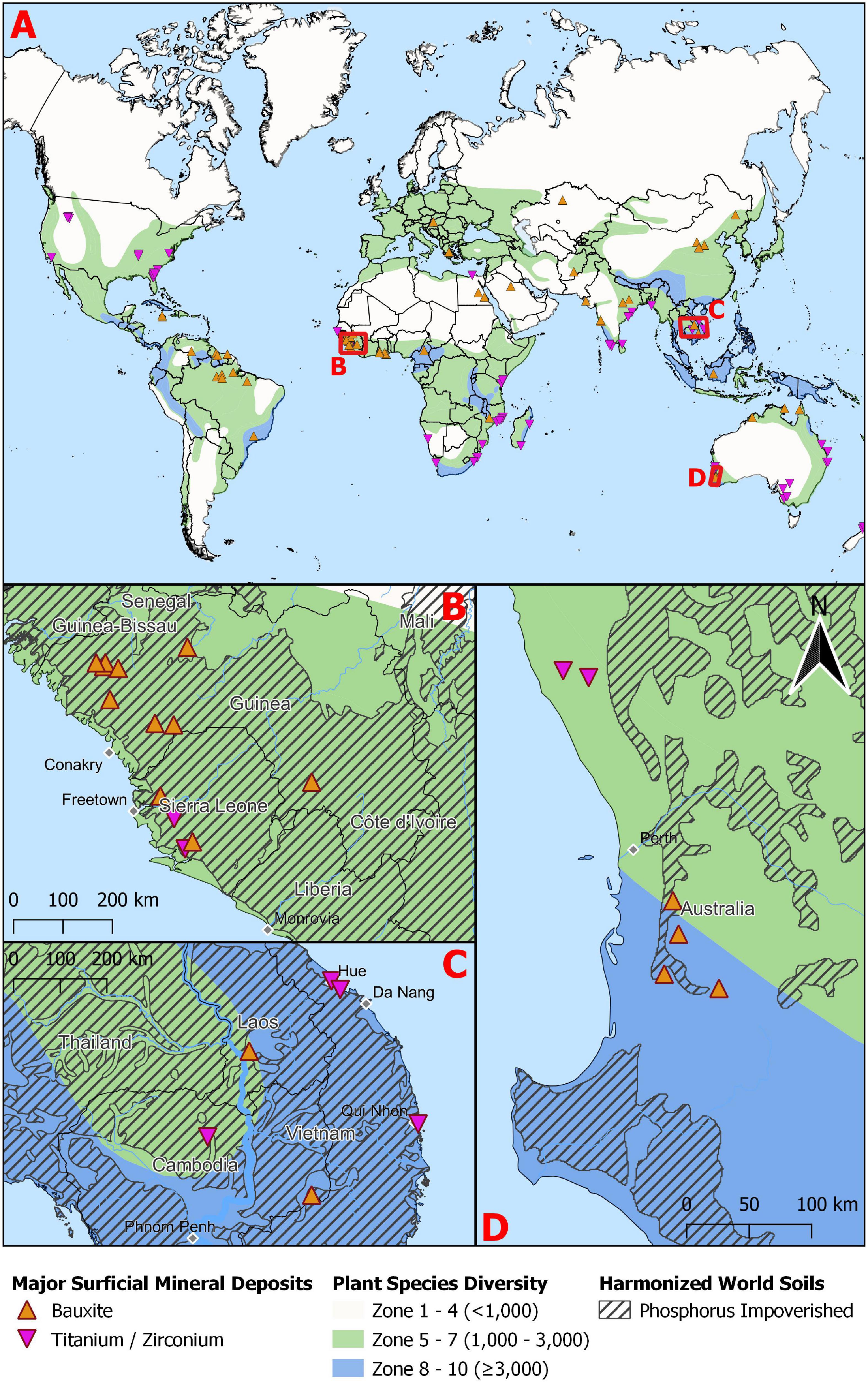
Figure 1. (A) The distribution of global biodiversity hotspots overlaid with bauxite and mineral sands (titanium/zirconium) deposits. Insets B–D are close ups of three regions in (B) West Africa, (C) South East Asia and (D) South West Australia to demonstrate the overlap between biodiversity hotspots, four ancient soil types (Acrisols, Ferralsols, Lixisols, and Luvisols) and bauxite and mineral sands (titanium/zirconium) deposits.
There was a high degree of overlap between bauxite and nutrient-deficient soils. Specifically, 54 of the 62 (87%) bauxite deposits co-occurred with weathered, nutrient impoverished soils (Figures 1B–D). In addition, 81% occurred in regions of moderate (1,000–3,000 species) while 13% occurred in the regions of highest plant diversity (≥3,000 species; Figures 1A–D) reinforcing the link between nutrient-deficient soils and plant biodiversity (Isbell et al., 2013). A congruence of high diversity, a range of species adapted to nutrient-deficient soils and bauxite deposits, highlights the importance of a precautionary approach to applying P-fertilizer to achieve diverse ecological restoration after bauxite mining.
Many mineral sands deposits also occur in biodiversity hotspots (Figure 1) with 67% occurring in regions of moderate diversity (1,000–3,000 species) and 16% in the regions with highest diversity (≥3,000 species; Figures 1A–D). While formed through different processes to bauxite, mineral sands deposits are also likely to be nutrient impoverished. The minerals in minerals sands (i.e., rutile, ilmenite, and zircon) are originally derived from weathered igneous (e.g., granite, basalt) or metamorphic (e.g., schist) rocks, transported in fluvial systems and deposited in beach, lake, or river environments where the minerals are concentrated by wave action. Consequently, mineral sands deposits are often highly permeable, which together with the rapid weathering and leaching of minerals, forms sandy soils of low fertility (e.g., Richard’s Bay, South Africa; Lubke et al., 1996).
In regions with weathered nutrient-deficient soils a significant proportion of species can have specialised adaptations for nutrient acquisition, such as cluster roots and exudation of carboxylates (e.g., Lambers et al., 2008), and/or have conservative growth rates (Daws et al., 2021a). These traits likely contribute to the sensitivity of restored ecosystems in these nutrient-deficient environments to applied-P. In addition, a range of species that occur in P-impoverished soils have been shown, at least in controlled conditions, to exhibit symptoms of toxicity to P when is added at concentrations greater than they would normally experience in native soils (Lambers et al., 2002; Shane et al., 2004; Pang et al., 2010; Williams et al., 2019; Tibbett et al., 2021). Further, the results of Holmes (2001) suggest that direct P-toxicity can occur in a field restoration setting. The potential for direct toxicity of applied-P to impact on restoration outcomes in regions with nutrient-deficient soils suggests a cautious approach to using fertilizer in these environments is appropriate and requires further investigation.
Fertilizer inputs to mine restoration
As part of the strip-mining process, topsoil and overburden are removed, stockpiled and replaced during restoration. Handling large material volumes can result in mixing and redistribution of nutrients across the reconstructed soil profile. Furthermore, removing above-ground vegetation, prior to mining, may reduce the overall nutrient stock leading to the perception that fertilizer inputs are required to “kick-start” nutrient-cycling (e.g., Grant et al., 2007).
For the Jarrah Forest in western Australia, fertilizer use in mine restoration commenced in the early 1980s and was motivated by these goals: (1) re-establishing nutrient reserves in the soil, (2) re-establishing vegetation productivity, especially to maximize tree growth and future timber production, and (3) rapidly stabilizing restored surfaces to minimize erosion (Koch, 1985; Grant et al., 2007). These outcomes were reinforced by “Working Arrangements” with the State Government framed around maximizing productivity and timber production. Similar considerations have also been applied to mine sites elsewhere (e.g., Lawrie, 1985; Petersen, 1985).
Fertilizer-P inputs to Jarrah Forest restoration have ranged from 80 to currently between 20 and 40 kg ha–1 (Standish et al., 2015; Tibbett et al., 2020), exceeding the estimated loss of ca. 14 kg P ha–1 through removing 340–430 tonnes of aboveground vegetation biomass ha–1 (Hingston et al., 1981; John Koch unpublished data). The Jarrah Forest is a tall, closed canopy eucalypt forest. For other restored vegetation types, such as open eucalypt woodland at Gove in Australia’s Northern Territory, the excess P added may be even greater since 25 kg P ha–1 is applied (Spain et al., 2015) while the aboveground biomass removed is only ca. 108 tonnes ha–1 (Chen et al., 2003), although P concentrations in wood are unknown for this site. Bauters et al. (2022) demonstrated that total above-ground P stocks in several neotropical forests range from approx. 20–50 kg ha–1. While these data are rare, and fertilizer rates are often not reported, in some cases it appears excess P is applied for restoration, above what is lost as vegetation cleared for mining (Table 1). This could be especially true of sites where fertilizers are applied despite minimizing P loss by returning cleared vegetation (trunks and branches) to restoration sites (e.g., Barbosa et al., 2022).
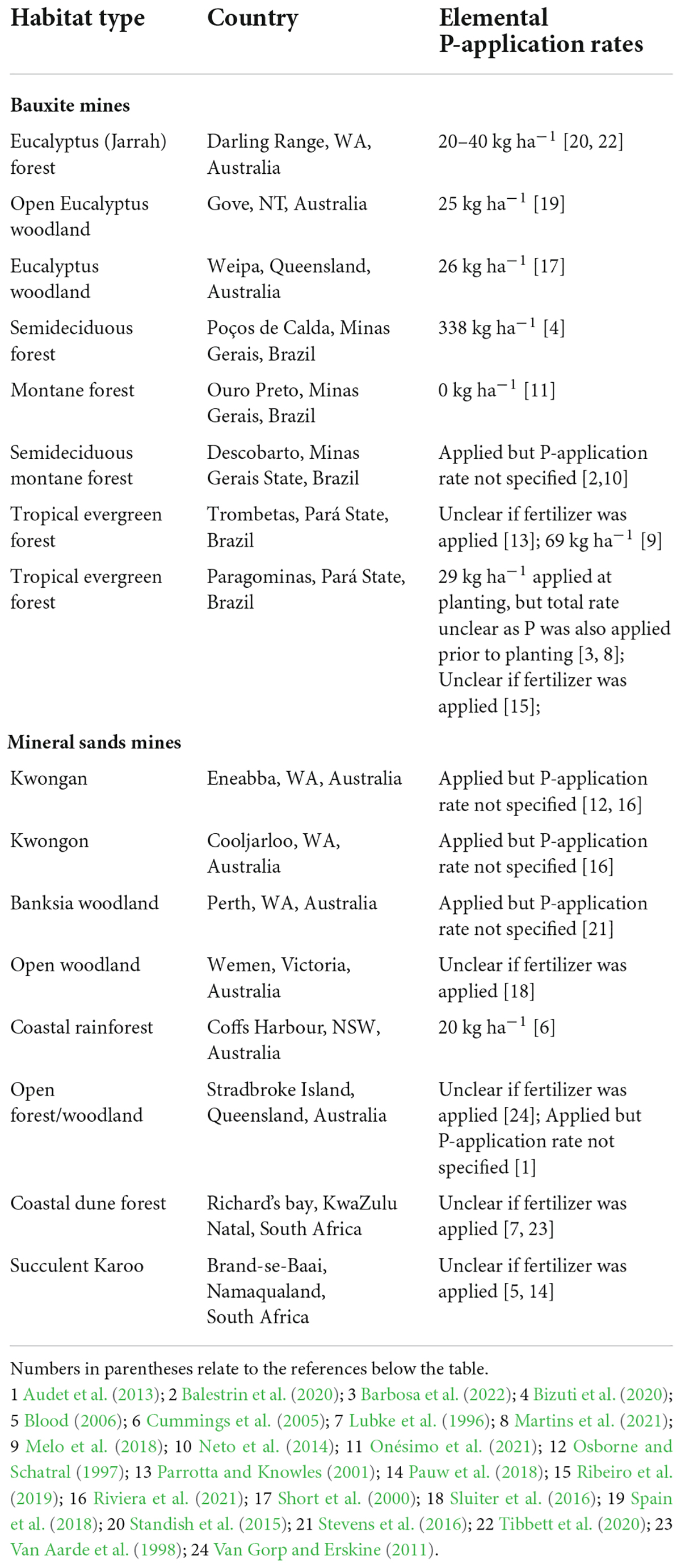
Table 1. Available information on fertilizer-P application rates applied to restored bauxite and mineral sands mines.
Fertilizer inputs to restored Jarrah Forest and at Gove not only represent the likely addition of excess P, but also the addition of P in readily available forms (e.g., diammonium phosphate or superphosphate). In contrast, the P removed pre-mining in biomass has often been sequestered, in wood, for decades to centuries. The conundrum is how to replace this P in a form that is less readily available and “slow release.” One solution may be to use rock phosphate, however, even this can have short-term negative impacts on plant community reestablishment (Tibbett et al., 2020), potentially because a range of species from nutrient-impoverished environments release carboxylates to release P from strongly sorbed forms (Lambers et al., 2002).
Nutrient addition alters soil geochemistry and vegetation responses
A single initial fertilizer application to bauxite mining restoration in the Jarrah Forest can elevate available (bicarbonate extractable) soil P concentrations for more than 20 years (Banning et al., 2008; Daws et al., 2021a) suggesting that applying P at the onset of restoration, may have long-term ongoing impacts on vegetation responses. In addition, at Gove bauxite mine, Spain et al. (2018) demonstrated that labile-P and long-term P concentrations in near-surface soils increase over a 26-year period following an initial P-application suggesting fertilizer-induced alterations to geochemical processes and nutrient-cycling.
Fertilizer addition can benefit weeds in restored systems (Daws et al., 2021a; Holmes, 2001). P can also preferentially benefit species such as N2-fixing legumes (e.g., Acacia spp.). N2-fixing species may benefit when fertilizers containing only P are used (since they themselves are not limited by N-availability) and because of a mismatch in the timeframes of availability of applied N and P. Specifically, applied-N can be lost rapidly from the soil due to leaching or volatilisation (depending on the form of the applied N and soil pH). Therefore, any potential growth benefit of applying N is short-term while P may be available over the longer term. A vigorous growth response of weeds and/or legumes can result in other species being outcompeted leading to reduced understorey diversity (Daws et al., 2015, 2019a, 2021a). These reductions in species richness can be significant. For example, species richness in 20-year-old restored Jarrah Forest sites was reduced between 20 and 25% as the initial fertilizer-P application rate increased from 0 to 80 kg ha–1 (Daws et al., 2019a) and slow-growing resprouter species, that are important for post-fire resilience, were particularly impacted (Daws et al., 2019a,b, 2021a). While these negative impacts of a single, initial application of P-fertilizer on species richness and community composition persist for at least 20 years we are still learning about fertilizer impacts on long(er)-term vegetation trajectories.
The establishment of a dominant legume layer may also result in restored sites being locked into alternate successional pathways (Grant, 2006) at least partly because legumes may produce large quantities of leaf litter and debris that limits seedling emergence and establishment of other species (Tibbett, 2010; Boyes et al., 2011; Le Stradic et al., 2014; Daws et al., 2019a,b). In the Jarrah Forest, vigorous legume establishment, combined with the formation of a prolific soil seed bank can result in the establishment of a “legume-cycle” where legumes rapidly germinate from the soil seed bank, and re-establish dominance, post fire (Grigg and Grant, 2009). Legume dominance has also been reported in restored sites on Stradbroke Island, Australia (Rogers and Mokrzecki, 1984) and at Richard’s Bay in South Africa. At Richard’s Bay, Vachellia karoo (Hayne) Banfi & Galasso dominates some restored sites and can arrest succession for 50 or more years (Boyes et al., 2011). While it is unclear whether the initial establishment and dominance of V. karoo was facilitated by P-addition (Table 1), Van Aarde et al. (1998) reported significantly higher soil-P concentrations in younger restored sites than either older restored sites or unmined reference forest suggesting that fertilizer was applied. Interestingly on Stradbroke Island, a similar pattern has been observed with the N2-fixing species Allocasuarina littoralis (Salisb.) L. A. S. Johnson (Casuarinaceae) dominating some restored sites and supressing other species by producing a dense layer of “needles” (leaf litter) on the soil surface (Audet et al., 2013). Further studies into the dominance of N2-fixing species in restored sites and interactions with fertilizer application would be of value.
The increased growth of P-responsive legumes and production of leaf litter and debris can also increase fire fuel load accumulation and fire risk. For example, an initial application of 80 kg P ha–1 at the outset of restoration resulted in the litter depth (and hence fine fuel loads) in 15-year-old restored sites being increased 3-times compared to unfertilized sites (Daws et al., 2019a). Similarly, at Gove, adding an initial 25 kg P ha–1 resulted in litter depth being elevated by about 3-times in 25-year-old restored sites compared to non-mined sites. This was due to a combination of not only increased litter inputs, but also reduced litter decomposition rates suggesting ongoing impacts on microbial and decomposer communities (Spain et al., 2015).
A vigorous response of legumes to applied-P also increases atmospheric N2-fixation, thereby increasing soil-N pools, which can be a goal of restoration where the intent is to increase N-availability for maximizing tree growth (Koch, 1985; Ward and Koch, 1995). For example, following application of an initial 80 kg P ha–1, N2 fixation rates of up to 207 kg N ha–1 year–1 have been reported in Jarrah Forest restoration (Koch, 1987). However, these rates far exceed fixation rates of 7 kg N ha–1 year–1 reported in unmined forest (Hingston, 1980). In addition, the high rates of N-fixation are associated with a drop of up to one unit in soil pH compared with soils in unmined forest (Ward, 2000). The implications of these changes for soil processes and above-ground vegetation responses are unknown but could be significant and require further research.
In the short-term, using fertilizer in restoration appears beneficial due to increases in both plant cover and growth of individual plants (Daws et al., 2013). Rapid re-establishment of cover may both minimize soil erosion (Ward, 2000) and give the visual impression that restoration efforts have been successful. However, the growth benefits may not persist. For example, in restored Jarrah Forest height and diameter growth of jarrah trees (Eucalyptus marginata Sm.) from 0 to 9 years-of-age increases with applied N or P, but by 20 years-of-age these differences have disappeared (Walters et al., 2021). Similarly, Grigg and Grant (2009) found that restored jarrah stands aged 10–13 years only exhibit a growth response to applied fertilizer when water limitation is temporarily reduced by stand thinning, a response also observed in unmined forest (Stoneman et al., 1997; Daws et al., 2021b). This observation is not unique to jarrah. For example, Campion et al. (2006) demonstrated that 4-year-old Eucalyptus grandis in South Africa was constrained more by water availability than soil nutrient supply. Hence, like the tortoise and the hare, unfertilized jarrah stands catch up eventually– questioning the premise of fertilizing these stands, particularly since they will not be logged until at least 70 years of age (Conservation Commission of Western Australia, 2013).
Discussion
Fertilizer addition may help achieve individual restoration goals, such as maximizing plant cover, but applying fertilizer conflicts with returning biodiverse ecosystems (see Supplementary Figure 1). From a policy perspective reframing targets away from short-term aspects such as growth rates, productivity and cover to focus on longer-term diversity, resilience and community composition may be more appropriate. Since diversity, resilience and productivity are linked in natural ecosystems (Isbell et al., 2015), the increased diversity associated with reducing fertilizer rates may have wider benefits for ecosystem function.
When assessing restoration success long-term outcomes matter. In the short-term fertilizer application to restored Jarrah Forest increases native diversity (by increasing abundance of short-lived ephemerals), plant growth rates and total cover (Daws et al., 2013; Tibbett et al., 2020). While over the longer term, self-sustaining and biodiverse Jarrah Forest ecosystems have been successfully restored (Koch and Hobbs, 2007) there can also be negative impacts on soil biogeochemistry and species composition. Further, any initial growth benefits of applying P – but not the legacy – disappear over time (Spain et al., 2018; Daws et al., 2019a, 2021a). In short, increased initial growth does not mean improved long-term outcomes, not least by upsetting the balance of competitive interactions between species. Consequently, there are further improvements that can be made when pursuing biodiverse outcomes from restoration in nutrient-impoverished environments.
A common theme from the literature is that while fertilizer is typically applied to mine restoration, the precise rates applied are often unclear (Table 1). To understand impacts, it is necessary to know both fertilizer rates and type (e.g., NPK versus P-only and formulation e.g., 9.1% P), as these aspects may affect vegetation reestablishment (Tibbett et al., 2020; Daws et al., 2021a). While we have focused on impacts of P- fertilizer, impacts of N and K fertilizers are likely to be significant in some cases. For example, Tibbett et al. (2020) reported that NPK fertilizers resulted in higher plant diversity than using just P-based fertilizers. While the mechanism(s) behind this response to including N in the fertilizer mix is unclear, applying N may, at least initially, maintain a N:P ratio in the soil more akin to reference forest. It is also possible that including N limits the establishment and competitiveness of N2 fixing species, since nitrate and ammonium addition can depress nodule production in Acacia seedlings (e.g., A. auriculiformis A. Cunn. ex Benth.; Goi et al., 1992). Notably, despite containing the same quantity of P as the P only treatment, NPK fertilizer treatment resulted in significantly lower soil ammonium concentrations, suggesting an impact on atmospheric N2-fixation by legumes (Tibbett et al., 2020). The impact of different fertilizer types on N:P stoichiometry and ecosystem responses requires further research.
These findings are likely applicable to a broad range of bauxite mine restoration beyond the Jarrah Forest because bauxite largely overlaps with nutrient-deficient soils often in biodiverse environments. Since research on Jarrah Forest restoration after bauxite mining is more advanced than restoration after bauxite mining in other regions further studies of fertilizer impacts on responses in a broader range of plant community-types are warranted. In a recent study of bauxite mine restoration in Brazil, Onésimo et al. (2021) ascribed their relative success, compared with earlier studies in the region, to not applying fertilizer, although this remains to be tested experimentally. Mineral sands mining also often occurs in regions with nutrient-poor soils (Section “Surface mining often occurs in nutrient-poor, biodiverse landscapes”) and high plant diversity, and there is emerging evidence that restoration of these environments may also be sensitive to P-application. For kwongan restoration after sand mining in Western Australia, Riviera et al. (2021) showed that P-addition was the management intervention with the largest impact on community composition. More research is needed to understand the generality of these findings.
We propose that P-limitation and moderation of fertilizer-P inputs are important for restoring naturally P-impoverished environments. While the majority of available research comes from the Jarrah Forest of south-western Australia, we view it as the “canary in the coal mine.” The considerable overlap worldwide, among areas of high plant diversity, nutrient-deficient soils and bauxite and mineral sands mining, suggest these findings will not be unique. Ongoing research from elsewhere in the world, such as that emerging from Brazil and South Africa, will be an important test of the generality of the findings for the Jarrah Forest.
Data availability statement
The original contributions presented in the study are included in the article/Supplementary Material, further inquiries can be directed to the corresponding author.
Author contributions
MD wrote the manuscript. All authors contributed to the conceptual development of this manuscript.
Funding
This work was supported by the Building Outstanding Impact Support Programme: Restoring biodiversity to phosphorus sensitive forests grant H&F38, University of Reading, United Kingdom and Research England Grant: Policy change to halt biodiversity loss and restore sustainable ecosystems after mining.
Acknowledgments
We acknowledge the Whadjuk and Pindjarup Noongar, traditional custodians of the unique Jarrah Forest bioregion. Thanks to A. H. Grigg who provided helpful input to earlier versions of this manuscript.
Conflict of interest
MD and CB are paid employees of Alcoa of Australia Ltd.
The remaining authors declare that the research was conducted in the absence of any commercial or financial relationships that could be construed as a potential conflict of interest.
Publisher’s note
All claims expressed in this article are solely those of the authors and do not necessarily represent those of their affiliated organizations, or those of the publisher, the editors and the reviewers. Any product that may be evaluated in this article, or claim that may be made by its manufacturer, is not guaranteed or endorsed by the publisher.
Supplementary material
The Supplementary Material for this article can be found online at: https://www.frontiersin.org/articles/10.3389/ffgc.2022.786305/full#supplementary-material
References
Audet, P., Gravina, A., Glenn, V., McKenna, P., Vickers, H., Gillespie, M., et al. (2013). Structural development of vegetation on rehabilitated North Stradbroke Island: above/belowground feedback may facilitate alternative ecological outcomes. Ecol. Process. 2:20. doi: 10.1186/2192-1709-2-20
Balestrin, D., Martins, S. V., and Villa, P. M. (2020). Brazilian Atlantic forest carbon stocks undergoing active restoration after bauxite mining exploration: an approach based on functional attributes. [Preprint]. doi: 10.21203/rs.3.rs-20764/v1
Banning, N. C., Grant, C. D., Jones, D. L., and Murphy, D. V. (2008). Recovery of soil organic matter, organic matter turnover and nitrogen cycling in a post-mining forest rehabilitation chronosequence. Soil Biol. Biochem. 40, 2021–2031. doi: 10.1016/j.soilbio.2008.04.010
Barbosa, R. S., do Vale, R. S., Schawrtx, G., Martins, W. B. R., Ribeiro, S. S., Rodrigues, J. I. M., et al. (2022). Restoration of degraded areas after bauxite mining in the eastern Amazon: which method to apply? Ecol. Eng. 180:106639. doi: 10.1016/j.ecoleng.2022.106639
Barthlott, W., Biedinger, N., Braun, G., Feig, F., Kier, G., and Mutke, J. (1999). Terminological and methodological aspects of the mapping and analysis of the global biodiversity. Acta Bot. Fennica 162, 103–110.
Bateman, A. M., Erickson, T. E., Merritt, D. J., Veneklaas, E. J., and Muñoz-Rojas, M. (2021). Native plant diversity is a stronger driver for soil quality than inorganic amendments in semi-arid post-mining rehabilitation. Geoderma 394:115001. doi: 10.1016/j.geoderma.2021.115001
Bauters, M., Grau, O., Doetter, S., Heineman, K. D., Dalling, J. W., and Prada, C. M. (2022). Tropical wood stores substantial amounts of nutrients, but we have limited understanding why. Biotropica 54, 596–606. doi: 10.1111/btp.13069
Bizuti, D. T. G., de Marchi Soares, T., Duarte, M. M., Casagrande, J. C., de Souza Moreno, V., and Peinado, F. J. M. (2020). Recovery of soil phosphorus on former bauxite mines through tropical forest restoration. Restor. Ecol. 28, 1237–1246. doi: 10.1111/rec.13194
Blood, J. R. (2006). Monitoring Rehabilitation Success on Namakwa Sands Heavy mineral Mining Operation, Namaqualand, South Africa. Ph.D thesis, South Africa: University of Stellenbosch.
Boyes, L. J., Gunton, R. M., Griffiths, M. E., and Lawes, M. J. (2011). Causes of arrested succession in coastal dune forest. Plant Ecol. 212, 21–32. doi: 10.1007/s11258-010-9798-6
Campion, J. M., Nkosana, M., and Scholes, M. C. (2006). Biomass and N and P pools in above- and below-ground components of an irrigated and fertilised Eucalyptus grandis stand in South Africa. Aust. For. 69, 48–57. doi: 10.1080/00049158.2006.10674987
Chen, X., Hutley, L. B., and Eamus, D. (2003). Carbon balance of a tropical savanna of northern Australia. Oecologia 137, 405–416. doi: 10.1007/s00442-003-1358-5
Conservation Commission of Western Australia. (2013). Forest Management Plan 2014-2023. Perth: Conservation Commission of Western Australia.
Cummings, J., Reid, N., Davies, I., and Grant, C. (2005). Adaptive restoration of sand-mined areas for biological conservation. J. Appl. Ecol. 42, 160–170. doi: 10.1111/j.1365-2664.2005.01003.x
Daws, M. I, Walters, S. J., Harris, R. J., Tibbett, M., Grigg, A. H., Morald, T. K., et al. (2021a). Nutrient enrichment diminishes plant diversity and density, and alters long-term ecological trajectories, in a biodiverse forest restoration. Ecol. Eng. 165:106222. doi: 10.1016/j.ecoleng.2021.106222
Daws, M. I., Standish, R. J., Lambers, H., and Tibbett, M. (2021b). Seeing the forest for the trees: fertiliser increases tree growth but decreases understorey diversity in the Northern Jarrah Forest, southwest Australia. J. Roy. Soc. W.A. 104, 5–9.
Daws, M. I., Grigg, A. H., Tibbett, M., and Standish, R. J. (2019a). Enduring effects of large legumes and phosphorus fertiliser on Jarrah Forest restoration 15 years after bauxite mining. For. Ecol. Manag. 438, 204–214. doi: 10.1016/j.foreco.2019.02.029
Daws, M. I., Grigg, A. H., Standish, R. J., and Tibbett, M. (2019b). “Applied phosphorus has long term impacts on vegetation responses in restored Jarrah Forest,” in Proceedings of the 13th International Conference on Mine Closure, eds A. B. Fourie and M. Tibbett (Perth: Australian Centre for Geomechanics), 693–704. doi: 10.36487/ACG_rep/1915_55_Daws
Daws, M. I., Standish, R. J., Koch, J. M., and Morald, T. K. (2013). Nitrogen and phosphorus fertiliser regime affect Jarrah Forest restoration after bauxite mining in Western Australia. Appl. Veg. Sci. 16, 610–618. doi: 10.1111/avsc.12046
Daws, M. I., Standish, R. J., Koch, J. M., Morald, T. K., Tibbett, M., and Hobbs, R. J. (2015). Phosphorus fertilisation and large legume species affect Jarrah Forest restoration after bauxite mining. For. Ecol. Manage. 354, 10–17. doi: 10.1016/j.foreco.2015.07.003
DFAT. (2016). Mine Rehabilitation: Leading Practice Sustainable Development Program for the Mining Industry. Department of Foreign Affairs and Trade. Canberra, Australia.: Commonwealth Government of Australia.
Diaz, A., Green, I., and Tibbett, M. (2008). Re-creation of heathland on improved pasture using topsoil removal and sulphur amendments: edaphic drivers and impacts on ericoid mycorrhizas. Biol. Conserv. 141, 1628–1635. doi: 10.1016/j.biocon.2008.04.006
Doley, D., and Audet, P. (2013). Adopting novel ecosystems as suitable rehabilitation alternatives for former mine sites. Ecol. Process. 2:22. doi: 10.1186/2192-1709-2-22
EPA. (1995). Rehabilitation and Revegetation. Best Practice Environmental Management in Mining. Canberra, Australia: Commonwealth of Australia.
FAO (2012). Harmonized World Soil Database v 1.2. http://www.fao.org/soilsportal/soil-survey/soil-maps-and-databases/harmonized-world-soil-database-v12/en/ (accessed August 24, 2021).
Goi, S. R., Sprent, J. I., James, E. K., and Jacob-Neto, J. (1992). influence of nitrogen form and concentration on the nitrogen fixation of Acacia auriculiformis. Symbiosis 14, 115–122.
Grant, C. D. (2006). State-and-transition successional model for bauxite mining rehabilitation in the Jarrah Forest of Western Australia. Restor. Ecol. 14, 28–37. doi: 10.1111/j.1526-100X.2006.00102.x
Grant, C. D., Ward, S. C., and Morley, S. C. (2007). Return of ecosystem function to restored bauxite mines in Western Australia. Restor. Ecol. 15:S94–S103. doi: 10.1111/j.1526-100X.2007.00297.x
Grigg, A. H., and Grant, C. D. (2009). Overstorey growth response to thinning, burning and fertiliser in 10–13-year-old rehabilitated jarrah (Eucalyptus marginata) forest after bauxite mining in south-western Australia. Aust. For. 72, 80–86. doi: 10.1080/00049158.2009.10676293
Hingston, F. J. (1980). “Nitrogen in litter and soils with reference to the Jarrah Forest ecosystem,” in Managing the Nitrogen economises of Natural and Man-Made Ecosystems, eds R. A. Rummery and F. J. Hingston (Perth: CSIRO), 229–249.
Hingston, F. J., Dimmock, G. M., and Turton, A. G. (1981). Nutrient distribution in a Jarrah (Eucalyptus marginata Donn ex sm.) ecosystem in south-west Western Australia. For. Ecol. Manag. 3, 183–207. doi: 10.1016/0378-1127(80)90015-8
Holmes, P. M. (2001). Shrubland restoration following woody alien invasion and mining: effects of topsoil depth, seed source, and fertilizer addition. Restor. Ecol. 9, 71–84. doi: 10.1046/j.1526-100x.2001.009001071.x
Isbell, F., Craven, D., Connolly, J., Schmid, B., Beierkuhnlein, C., Bezemer, T. M., et al. (2015). Biodiversity increases the resistance of ecosystem productivity to climate extremes. Nature 526, 574–577. doi: 10.1038/nature15374
Isbell, F., Reich, P. B., Tilman, D., Hobbie, S. E., Polasky, S., and Binder, S. (2013). Nutrient enrichment, biodiversity loss, and consequent declines in ecosystem productivity. Proc. Natl. Acad. Sci. U.S.A. 110, 11911–11916. doi: 10.1073/pnas.1310880110
Koch, J. M. (1985). “Tree nutrition research at Alcoa’s Darling Range operations,” in Proceedings of the North Australian Mine Rehabilitation Workshop No. 9, Weipa, ed. J. W. Lawrie (Weipa: Comalco), 77–94.
Koch, J. M. (1987). Nitrogen accumulation in a rehabilitated bauxite mined area in the Darling Range. Western Australia. Aust. Forestry Res. 17, 59–72.
Koch, J. M., and Hobbs, R. J. (2007). Synthesis: is Alcoa successfully restoring a Jarrah Forest ecosystem after bauxite mining in Western Australia? Restor. Ecol. 15:S137–S144. doi: 10.1111/j.1526-100X.2007.00301.x
Kragt, M. E., Lison, C., Manero, A., and Hawkins, J. (2019). “Mine site rehabilitation conditions in Western Australia,” in Proceedings of the 13th International Conference on Mine Closure, eds A. B. Fourie and M. Tibbett (Perth: Australian Centre for Geomechanics), 95–110. doi: 10.36487/ACG_rep/1915_09_Kragt
Lambers, H., Juniper, D., Cawthray, G. R., Veneklaas, E. J., and Martinez-Ferri, E. (2002). The pattern of carboxylate exudation in Banksia grandis (Proteaceae) is affected by the form of phosphate added to the soil. Plant Soil 238, 111–122. doi: 10.1023/A:1014289121672
Lambers, H., Raven, J. A., Shaver, G. R., and Smith, S. E. (2008). Plant nutrient-acquisition strategies change with soil age. Trends Ecol. Evol. 23, 95–103. doi: 10.1016/j.tree.2007.10.008
Lawrie, J. W. (1985). “The development of the regeneration programme at Weipa,” in Proceedings of the North Australian Mine Rehabilitation Workshop No. 9, Weipa, ed. J. W. Lawrie (Weipa: Comalco), 7–32.
Le Stradic, S., Buisson, E., Negreiros, D., Campagne, P., and Fernandes, G. W. (2014). The role of native woody species in the restoration of Campos Rupestres in quarries. Appl. Veg. Sci. 17, 109–120. doi: 10.1111/avsc.12058
Lubke, R. A., Avis, A. M., and Moll, J. B. (1996). Post-mining rehabilitation of coastal sand dunes in Zululand. South Africa. Landscape Urban Plan. 34, 335–345. doi: 10.1016/0169-2046(95)00227-8
Manero, A., Standish, R., and Young, R. (2021). Mine completion criteria defined by best-practice: a global meta-analysis and Western Australian case studies. J. Environ. Manag. 282:111912. doi: 10.1016/j.jenvman.2020.111912
Martins, W. B. R., Schwartz, G., Ribeiro, S. S., Ferreira, G. C., de Souza Barbosa, R., de Paula, M. T., et al. (2021). Ecosystem restoration after bauxite mining: favorable indicators for Technosols construction and soil management using liming and subsoiling. New Forest. 52, 971–994. doi: 10.1007/s11056-021-09834-5
Mason, G. T., and Arndt, R. E. (1996). Mineral Resources Data System (MRDS): USGS Data Series 20. https://doi.org/10.3133/ds20 (accessed August 24, 2021).
Melo, V. F., Batista, A. H., Barbosa, J. Z., Barbeiro, L., Gomes, R., and Dultra, M. T. M. (2018). Soil quality and reforestation of the Brazil nut tree (Bertholletia excelsa Bonpl.) after laterite-type bauxite mining in the Brazilian Amazon forest. Ecol. Eng. 124, 111–118. doi: 10.1016/j.ecoleng.2018.10.016
Minerals Council of South Africa. (2007). Guidelines for the Rehabilitation of Mine Land. Coaltech: Chamber of Mines of South Africa.
Neto, A. M., Martins, S. V., de Almeida Silva, K., Lopes, A. T., and de Abreu Demolinari, R. (2014). Natural regeneration in a restored bauxite mine in southeast Brazil [Regeneración natural en mina de bauxita restaurada en el sureste de Brasil]. Bosque 35, 377–389. doi: 10.4067/S0717-92002014000300012
Onésimo, C. M. G., Dias, D. D., Beirão, M. V., Kozovits, A. R., and Messias, M. C. T. B. (2021). Ecological succession in areas degraded by bauxite mining indicates successful use of topsoil. Restor. Ecol. 29:e13303. doi: 10.1111/rec.13303
Osborne, J. M., and Schatral, A. (1997). Late autumn and early winter sowings increase survival of native plant species in rehabilitated mine sites in the Western Australian Kwongan. Int. J. Surface Min. Reclam. Environ. 11, 95–103. doi: 10.1080/09208119708944067
Pang, J. Y., Tibbett, M., Denton, M. D., Lambers, H., Siddique, K. H. M., Bolland, M. D. A., et al. (2010). Variation in seedling growth of 11 perennial legumes in response to phosphorus supply. Plant Soil 328, 133–143. doi: 10.1007/s11104-009-0088-9
Parrotta, J. A., and Knowles, O. H. (2001). Restoring tropical forests on lands mined for bauxite: Examples from the Brazilian Amazon. Ecol. Eng. 17, 219–239. doi: 10.1016/S0925-8574(00)00141-5
Pauw, M. J., Esler, K. J., and Le Maitre, D. C. (2018). Assessing the success of experimental rehabilitation on a coastal mineral sands mine in Namaqualand, South Africa. Ecosyst. Process. Rehab. 35, 363–373. doi: 10.2989/10220119.2018.1526823
Perry, L. G., Blumenthal, D. M., Monaco, T. A., Paschke, M. W., and Redente, E. F. (2010). Immobilizing nitrogen to control plant invasion. Oecologia 163, 13–24. doi: 10.1007/s00442-010-1580-x
Petersen, A. (1985). “Assessment of the success of rehabilitation in Queensland,” in Proceedings of the North Australian Mine Rehabilitation Workshop No. 9, Weipa, ed. J. W. Lawrie (Weipa: Comalco), 255–263.
Ribeiro, S. S., de Assis Oliveira, F., Ferreira, G. C., Santos, D. E., and Cruz, D. C. (2019). Forest restoration evaluation through indicators in areas of bauxite mining. Floresta e Ambiente 26:e20170812. doi: 10.1007/s11356-020-10626-1
Riviera, F., Renton, M., Dobrowolski, M. P., Veneklaas, E. J., and Mucina, L. (2021). Patterns and drivers of structure, diversity, and composition in species-rich shrublands restored after mining. Rest. Ecol. 29:e13360. doi: 10.1111/rec.13360
Rogers, R. W., and Mokrzecki, S. (1984). “Succession following sand mining of high dunes, North Stradbroke Island,” in Focus on Stradbroke. New information on North Stradbroke Island and surrounding areas 1974 to 1984, eds R. J. Coleman, J. Covacevich, and P. Davie (QLD, Australia: Boolarong, Brisbane), 260–266.
Schelflout, S., De Schrijver, A., De Bolle, S., De Gelder, L., Demey, A., Du Pré, T., et al. (2015). Phosphorous mining for ecological restoration on former agricultural land. Rest. Ecol. 23, 842–851. doi: 10.2134/jeq2015.08.0422
Shane, M. W., Szota, C., and Lambers, H. (2004). A root trait accounting for the extreme phosphorus sensitivity of Hakea prostrata (Proteaceae). J. Exp. Bot. 27, 991–1004. doi: 10.1111/j.1365-3040.2004.01204.x
Short, T. A., Menzies, N. W., and Mulligan, D. R. (2000). Mining disturbance alters phosphorus fractions in northern Australian soils. Aust. J. Soil Res. 38, 411–421. doi: 10.1071/SR99033
Sluiter, I. R. K., Schweitzer, A., and MacNally, R. (2016). Spinifex–mallee revegetation: implications for restoration after mineral-sands mining in the Murray–Darling Basin. Aust. J. Bot. 64, 547–554. doi: 10.1071/BT15265
Spain, A. V., Tibbett, M., Hinz, D. A., Ludwig, J. A., and Tongway, D. J. (2015). “The mining-restoration system and ecosystem development following bauxite mining in a biodiverse environment of the seasonally dry tropics, Northern Territory, Australia,” in Mining in Ecologically Sensitive Landscapes, ed. M. Tibbett (Australia: CSIRO Publishing), 159–227.
Spain, A. V., Tibbett, M., Ridd, M., and McLaren, T. I. (2018). Phosphorus dynamics in a tropical forest soil restored after strip mining. Plant Soil 427, 105–123. doi: 10.1007/s11104-018-3668-8
Standish, R. J., Daws, M. I., Gove, A. D., Didham, R. K., Grigg, A. H., Koch, J. M., et al. (2015). Long-term data suggest jarrah-forest establishment at restored mine sites is resistant to climate variability. J. Ecol. 103, 78–89. doi: 10.1111/1365-2745.12301
Stevens, J. C., Rokich, D. P., Newton, V. J., Barrett, R. L., and Dixon, K. W. (2016). Banksia Woodlands: A Restoration Guide for the Swan Coastal Plain. Perth, Aus.: UWA publishing.
Stoneman, G. L., Crombie, D. S., Whitford, K., Hingston, F. J., Giles, R., Portlock, C. C., et al. (1997). Growth and water relations of Eucalyptus marginata (jarrah) stands in response to thinning and fertilization. Tree Phys. 16, 267–227. doi: 10.1093/treephys/17.4.267
Tibbett, M. (2010). “Large-scale mine site restoration of Australian eucalypt forests after bauxite mining: soil management and ecosystem development,” in Ecology of Industrial Pollution, eds L. C. Batty and K. Hallberg (Cambridge, UK: Cambridge University Press), 309–326. doi: 10.1017/CBO9780511805561.016
Tibbett, M., Daws, M. I., George, S. J., and Ryan, M. H. (2020). The where, when and what of phosphorus fertilisation for seedling establishment in a biodiverse Jarrah Forest restoration after bauxite mining in Western Australia. Ecol. Eng. 153:105907. doi: 10.1016/j.ecoleng.2020.105907
Tibbett, M., Daws, M. I., and Ryan, M. H. (2021). Phosphorus uptake and toxicity is delimited by mycorrhizal symbiosis in P-sensitive Eucalyptus marginata but not in P-tolerant Acacia celastrifolia. bioRxiv [Preprint]. doi: 10.1101/2021.09.28.462111
Tibbett, M., O’Connor, R., and Daws, M. I. (2019). “Too much of a good thing: phosphorus over-fertilisation in rehabilitated landscapes of high biodiversity value,” in Proceedings of the 13th International Conference on Mine Closure, eds A. B. Fourie and M. Tibbett (Perth: Australian Centre for Geomechanics), 651–665. doi: 10.36487/ACG_rep/1915_52_Tibbett
Van Aarde, R. J., Smit, A.-M., and Claassens, A. S. (1998). Soil Characteristics of rehabilitating and unmined coastal dunes at Richards Bay, KwaZulu-Natal, South Africa. Restor. Ecol. 6, 102–110. doi: 10.1046/j.1526-100x.1998.06113.x
Van Gorp, L., and Erskine, P. D. (2011). The influence of topsoil management on Stradbroke Island sand mine rehabilitation: implications for ecosystem recovery. Proc. Roy. Soc. Queensland 117, 377–389.
Walters, S. J., Harris, R. J., Daws, M. I., Gillett, M. J., Richardson, C. G., Tibbett, M., et al. (2021). The benefits of fertiliser application on tree growth are transient in restored Jarrah Forest. Trees For. People 5:100112. doi: 10.1016/j.tfp.2021.100112
Ward, S. C. (2000). Soil development on rehabilitated bauxite mines in south-west Australia. Aust. J. Soil Res. 38, 453–464. doi: 10.1071/SR99032
Ward, S. C., and Koch, J. M. (1995). Early growth of jarrah (Eucalyptus marginata Donn ex Smith) on rehabilitated bauxite minesites in south-west Australia. Aust. Forest. 58, 65–71. doi: 10.1080/00049158.1995.10674646
Wassen, M. J., Venterink, H. O., Lapshina, E. D., and Tanneberger, F. (2005). Endangered plants persist under phosphorus limitation. Nature 437, 547–550. doi: 10.1038/nature03950
Wheeler, B. D., and Shaw, S. C. (1991). Aboveground crop mass and species richness of the principal types of herbaceous rich-fen vegetation of lowland England and Wales. J. Ecol. 79, 285–301. doi: 10.2307/2260713
Keywords: diversity, legume, nitrogen, nutrients, rehabilitation
Citation: Daws MI, Blackburn C, Standish RJ and Tibbett M (2022) Canary in the coal mine: Lessons from the Jarrah Forest suggest long-term negative effects of phosphorus fertilizer on biodiverse restoration after surface mining. Front. For. Glob. Change 5:786305. doi: 10.3389/ffgc.2022.786305
Received: 30 September 2021; Accepted: 05 July 2022;
Published: 25 July 2022.
Edited by:
Jean-François Bastin, University of Liège, BelgiumReviewed by:
Panayiotis G. Dimitrakopoulos, University of the Aegean, GreeceSambieni Kouagou Raoul Sambieni, University of Liège, Belgium
Copyright © 2022 Daws, Blackburn, Standish and Tibbett. This is an open-access article distributed under the terms of the Creative Commons Attribution License (CC BY). The use, distribution or reproduction in other forums is permitted, provided the original author(s) and the copyright owner(s) are credited and that the original publication in this journal is cited, in accordance with accepted academic practice. No use, distribution or reproduction is permitted which does not comply with these terms.
*Correspondence: Matthew I. Daws, bWlkYXdzQHlhaG9vLmNvbQ==