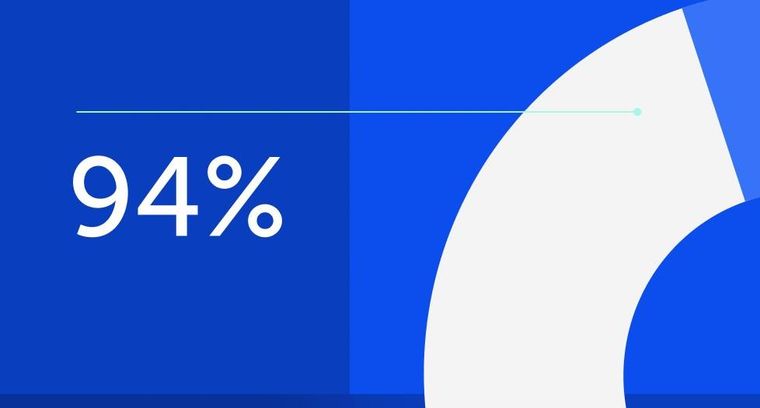
94% of researchers rate our articles as excellent or good
Learn more about the work of our research integrity team to safeguard the quality of each article we publish.
Find out more
PERSPECTIVE article
Front. For. Glob. Change, 11 July 2022
Sec. People and Forests
Volume 5 - 2022 | https://doi.org/10.3389/ffgc.2022.785740
This article is part of the Research TopicClimate-Smart Solutions for Tropical Mountain EnvironmentsView all 6 articles
We are of the opinion that environmental policies that are based on scientific knowledge at the time they are established need to be revisited in terms of the current knowledge and the effectiveness of these policies in protecting or promoting a particular ecosystem service. Here we use the first Swiss Federal Forest Law (1876) as a case example, which was established to protect mountain forests as a natural means of protection against natural hazards, particularly floods. We briefly summarize the current relevant scientific knowledge on (i) reasons for reforestation in mountains and how the law may have contributed, (ii) forest effects on hydrological regimes and their protection service against floods, and (iii) other watershed changes affecting both reforestation and the forest-runoff interaction. We then present insights from a case study on the Upper Rhone catchment, which lead us to develop a methodological approach based on interdisciplinary collaboration among social and natural sciences to gain the needed data to answer the question of whether a forest protection law can serve as a means of flood protection. Specifically, we found that a data interpolation method is key to answering this question given data are at different scales and resolutions and suggest modeling to fill gaps. Such methods and collaborations are key for basing environmental laws and policies in current scientific knowledge and effectively manage ecosystems and their services.
Environmental policies have the purpose to protect ecosystems in their structure and function to maintain the ecosystem services they provide. However, these policies are rarely revisited to investigate whether their assumptions agree with current scientific understanding, nor whether their implementation served to protect the targeted ecosystem services (McEldowney and McEldowney, 2011; Luo et al., 2015). The environmental policy of interest for this perspective is the first Swiss Federal Forest Law. It was established in 1876 following catastrophic flood events to preserve and restore the protective service of mountain forests against natural disasters, such as floods, by prohibiting clear-cutting and an excessive use of forests (Schuler, 2007). In the 19th century, wood was a critical resource for people in mountain areas and forests were often used as pastures within their agricultural production system (Stuber and Bürgi, 2001). The law was one of the factors contributing to a transition from net deforestation to net reforestation in the Swiss Alps and is believed to have substantially contributed to flood protection, defined herein as the prevention of loss of life and against infrastructure and economic damages (Mather and Fairbairn, 2000; Reynard et al., 2001). However, whether reforestation was due to the law or other socio-economic changes and to what extent reforestation contributed to flood protection remains unclear.
The aim, assumption(s), and effects of the Forest Law need to be revisited to understand its role in reforestation and flood protection. The first question, based on the aim to conserve and expand forests, is whether, why and where the law triggered reforestation. The second question, based on the assumed protective role of forests, is whether and under what conditions forests influence flood occurrence. The third question, and the most critical regarding the aims of the law, combines the answers from questions one and two to answer whether any potential law-related reforestation served to minimize flood risks. While forests were assumed to protect against floods in the late 19th century (e.g., Landolt, 1862; Culmann, 1865), in Switzerland and countries with similar laws such as France and Austria (Weiss, 2001; Ford, 2004), the actual effects of these laws on flood risk have not been systematically investigated. Yet, their investigation is critical to better understand the protection services of mountain forests and to adapt related environmental policies accordingly.
Answering these questions is not the aim of this perspective but rather we explore the approach required, namely an interdisciplinary collaboration combining insights from social and natural sciences. On the one hand, historical data on socioeconomic changes affecting forest cover simultaneously to the Forest Law are necessary to determine whether the law can be considered as the reason of reforestation (e.g., Loran et al., 2017). On the other hand, the scientific basis of forest-runoff interactions must be considered to link any potential forest cover changes to floods (e.g., Eisenbies et al., 2007; Kirchner et al., 2020). Also, other factors that can alter the alleged influence of forests on floods need investigation (e.g., climatic and anthropogenic changes) (Calder, 2007). Finally, these social and natural science aspects need to be combined interdisciplinarily to draw conclusions on the links from law to reforestation to hydrological regimes and floods.
In this perspective, we present a conceptual and methodological framework to evaluate the importance of legal forest protection on flood occurrence based on insights gained from a case study in the Upper Rhone catchment. Specifically, we (i) elaborate on the law in relation to mountain forest cover changes and society in Switzerland; (ii) synthesize the current understanding of forest effects on flood risk reduction; (iii) explore additional factors potentially altering forest-runoff interactions, and (iv) suggest a methodological approach to evaluate the effects of forest protection on flood occurrence and present example results on the data for the sub-catchments of the Upper Rhone River (Switzerland). We conclude that studying the impacts of the Forest Law on flood protection can be evaluated and similar evaluation could enhance environmental policies regarding their provision of ecosystem services.
The Swiss Federal Forest Law (1876) was instituted to protect mountain forests to increase their protection against natural hazards. It was the first environmental measure at the federal level in Switzerland. Its origin were the disastrous floods of 1868 and accompanying landslides that caused the deaths of 50 people and damages of 14 million Swiss francs (Pfister, 2002). The Swiss Forestry Society had already alerted the Swiss government in 1856 about the alleged negative effects of deforestation on flood control. These warnings were later supported by the expert reports of Landolt (1862) and Culmann (1865). The general belief among experts and politicians at the time was that Alpine forests were diminishing due to cutting by the mountain populations (Blotnitzki, 1869). Thus, the crucial role of forests in the management of natural risks was added to the argument in favor of securing a sustainable yield. A commission of experts was appointed by the Federal Council to determine the causes of the floods of 1868 and the measures to be taken to better deal with future floods. It determined that heavy precipitation and snow melting due to the warm foehn wind were the main reason for the disaster. However, the commission’s report also suggested that mountain forest cover may play a significant role in reducing the flood risk (Summermatter, 2005). Thus, the law was created to foster reforestation with the aim to increase the protection services of forests against natural hazards.
Before industrialization, increases in populations often led to a decrease in forest cover due to the need for wood in construction and heating, and of land for agriculture and pasture (Peltier, 2005; Figure 1; point 6). In Switzerland, the demand of wood increased after 1800 and inhabitants made heavy use of wood resources, resulting in a decrease in forested area (Schuler, 2007) which in turn affected soil characteristics important for the water cycle (e.g., soil organic content, soil storage capacity; see Section “Forest – Catchment Hydrology Interactions”). The mountain population was generally not very cooperative with following the forest law initially, as highlighted by cases of illegally cutting and selling wood (Journal et Feuille d’avis du Valais, 1906). Similarly, the interpretation of reforestation projects and forest cover change requires the consideration of additional factors, such as difficulties that arose due to a lack of seedlings (SCR, 1883), conflicts with the local communities (SCR, 1881), a lack of forestry personnel (SCR, 1880), and the generally slow action taken by the cantonal and municipal authorities to implement the law-related subsidies of the Confederation (L’Ami du peuple, 1906). However, industrialization, the migration of inhabitants from rural mountain areas to cities, and the development of fossil fuels reduced the need for wood and forests were able to recover. These changes led to an increase in forest cover from around 20% in 1850 to 30% at the beginning of the 21st century (Loran et al., 2016). As industrialization and the timing of the Forest Law coincided, methods are needed to disentangle how much of the realized reforestation can be attributed to the latter.
Figure 1. Conceptual representation of the different factors influencing catchment-scale runoff and how they might affect catchment hydrology. The focus is on the effect of forests on catchment hyrology.
The impact of forest cover on the hydrological regime has long been subject to scientific and political debate (Andréassian, 2004; Calder and Aylward, 2006). All agree that vegetation cover influences the effects of rainfall events (Fercher et al., 2018), whereby three regulating mechanisms can be distinguished (Figure 1; points 2–4): (i) increased water infiltration into soils (e.g., Gonzalez-Sosa et al., 2010; Neris et al., 2012); (ii) increased water storage capacity in soils (e.g., Ferreira et al., 2004; Nijzink et al., 2016); and (iii) reduced water flow velocity via increased evapotranspiration and flow path lengths through the catchment (Bradshaw et al., 2007; Moos et al., 2018). These mechanisms are often linked as a higher porosity of the soil with more meso- and macropores due to tree roots allows for higher/faster and deeper infiltration (Beven and Germann, 2013; Vereecken et al., 2016), while tree roots can also extract water from deeper soil horizons compared to other vegetation cover (Moore and Heilman, 2011). The interception of precipitation by leaves increases the flow path lengths of water thus decreasing the amount of water for infiltration at a point in time, and evaporation during dry periods removes up to 5–20 mm of precipitation that could otherwise enter the stream (Wahren et al., 2012). These effects, however, strongly depend on the season and the type and structure of vegetation (Anderson, 1976; Figure 1: 1). Furthermore, only few catchment scale studies on forest effects have been conducted (e.g., Jones and Grant, 1996; Storck et al., 1998; Guillemette et al., 2005), demonstrating that deforestation could reduce soil infiltration and shorten flow pathways, thus increasing runoff. In addition, trees stabilize slopes as they reinforce the soil with their root network and reduce soil pore water pressure (Bathurst et al., 2010; Schwarz et al., 2012). Consequently, forests can reduce the sediment availability in torrents and thus the risk of debris flows (Cannon et al., 2010; Seebald et al., 2019). However, as most studies on the forests’ influence on floods have been conducted at local scales, their conclusions cannot be easily applied to other places without appropriate extrapolation methods.
Increasing scales to the catchment also increases the variability of climates and terrain that need to be accounted for and may potentially obscure a causal link between forests and floods (Calder, 2007; van Dijk et al., 2009; Alewell and Bebi, 2011). For example, the forest effect on floods strongly depends on soil characteristics. Soils with low water storage capacity are only altered marginally by roots, thus forest effects on hydrology can only be small (Badoux et al., 2006). It is often argued that the effects of forests are negligible at large scales and for extreme precipitation events (Calder, 2007; van Dijk et al., 2009; Wahren et al., 2012). During extreme precipitation events infiltration rates are generally too low to cope with the amount of water arriving, or soil storage capacity may be exceeded. Thus, areas with few but intense precipitation events may profit less from forests than areas with longer and more moderate rainfall events (Viglione et al., 2016). The extrapolation methods to the catchment scale need to include such cumulative forest effects moving downstream, by for example using process-based models.
The hydrological regime of a mountain catchment depends on many factors, some of which potentially exert a stronger control on catchment hydrology than forests (Chang and Franczyk, 2008; Li et al., 2009). They include geologic setting and climate variation (Figure 1: points 5 and 9). Structural geology affects hydrology as flow paths tend to develop along predefined structures (e.g., Howard, 1967; Kühni and Pfiffner, 2001), and runoff generation depends on altitude and orientation of the land-surface (Molnar et al., 2007; Roy et al., 2015). Climate varies in space, mainly as a function of latitude, elevation, topography, global circulation patterns, and time, with recent climate change being an important aspect for the evaluation of a centuries-old environmental law (Figure 1; point 5). Since 1876, Swiss mean annual temperatures decreased by about 0.5°C in the late 19th century with a subsequent increase of about 2°C until today. Over the same period, average annual precipitation showed no significant trend in Switzerland (Brönnimann et al., 2014). Nevertheless, increasing temperatures led to an increase in runoff in glaciated catchments (Pellicciotti et al., 2010). Also, over 90% of Swiss weather stations show a positive trend in intensity and frequency of heavy precipitation events from 1901 to 2014 (Scherrer et al., 2016). These climate trends affect the hydrology but may also alter the structure and function of forests.
Factors beyond the natural environment can impact catchment hydrology, such as hydropower production, touristic infrastructure, and natural hazard mitigation (Verbunt et al., 2005; Figure 1; points 7 and 8). In Switzerland, the onset of modern hydropower generation took place in the late 19th century. Between 1945 and 1970, the country experienced a period of expansion with the opening of several storage plants in high alpine terrain (SFOE, 2020). As a result, downstream hydrology was altered drastically, with a reduction of flows except when releases from the storage plants occur. Verbunt et al. (2005) found a clear influence of water releases and storages by hydropower stations on the hydrology of the Rhine basin. The analysis further revealed a significant, but localized, impact of urbanization and afforestation. Urbanization in the Swiss Alps, as related to mass tourism, started to develop in the middle of the 19th century (Reichen, 2013). This required the regulation of torrents to expand and protect alpine settlements and infrastructure (Figure 1; points 7 and 8). First attempts of river regulation in Switzerland date back to the 18th century with large-scale interventions commencing in the 19th and 20th century (Woolsey et al., 2005). In the Swiss Alps, 61% of the rivers are in a (near-)natural ecomorphological condition, as are 82% of the rivers above 2,000 m (Zeh Weissmann et al., 2009). However, obstructions such as weirs and dams are widespread in Switzerland and can influence the hydrological regime and sediment transport capacity. All these factors, individually or jointly, can have far reaching influences on a (sub-)catchment’s hydrology, the forest cover and structure, their interaction, and thus flood occurrence and prevention. An evaluation approach thus needs to allow such factors to be incorporated.
Based on an interdisciplinary case study in the Upper Rhone catchment in Switzerland, we developed a potential methodological approach allowing for the evaluation of the Swiss Federal Forest Law as a means for flood protection (Figure 2). The proposed framework is based on a multi-step process consisting of the following parts: (i) the determination of the net effect of the forest law on forest cover; (ii) the determination of the importance of forest cover on hydrological regimes and flood occurrence under varying climate conditions; and (iii), by combining (i) and (ii), the net effect of the forest law on hydrological regimes and flood occurrence.
Figure 2. Proposed methodological approach to answer the research question regarding the effectiveness of flood protection via the protection of mountain forests.
We included a variety of data from different sources, using methods from social and natural sciences. We focused on hydrological (discharge, water level) and meteorological (precipitation, humidity, wind, temperature) data from sensor stations maintained by municipal, cantonal or federal agencies, topographical data (e.g., digital elevation model) and land cover data using historical maps, orthophotos, and photography. For historical data we accessed archival newspapers and the Valais State archives (Table 1). These data provided the database (Figure 2; lilac), which then serves as input for modeling (Figure 2; tangerine). Historical and current forest cover and hydrological data rarely have the same temporal and spatial resolution, requiring methods for inter- and extrapolation adapted to the data types. By processing collected data directly or indirectly (Figure 2; tangerine) intermediate results can be created, namely the conditions of forest and hydrological regimes at different points in space and time, in relation to climate conditions (Figure 2; green). However, hydrological models generally incorporate forest cover and structure insufficiently or at coarse scales (e.g., Adams et al., 2010; Moos et al., 2018; Speich et al., 2020). Therefore, the development of an adapted forest-hydrology model (Figure 3; blue), depending on the forest state and the climate and catchment conditions, is necessary to realistically reconstruct hydrographs (e.g., Kuczera, 1987; Scheidl et al., 2020). Such a hydrological model will need to appropriately represent forest effects, which is rarely done (but see Zemokost, which includes vegetation cover type, Fercher et al., 2018), by, for example, considering canopy cover, roots, and evapotranspiration (Figure 1). Additionally, the model will need to address changes to the hydrological cycle via anthropogenic modifications to separate their effects from that of the forest where possible. An appropriate model could then indicate whether forest effects are also relevant during extreme climate events, which are generally those leading to most damages on infrastructure and society (e.g., Kelly et al., 2016). We could imagine the model then being parametrized based on different forest cover, climate conditions, and catchment characteristics, and simulated with actual and hypothetical forest cover and climate conditions under a factorial design (e.g., current and historical forest cover crossed with historical and current climate, respectively) (e.g., Li et al., 2012; Buechel et al., 2022). The sensitivity analyses of the model parameters as well as the multivariate analyses of input variables and the resulting hydrographs will provide critical information on forest-hydrological regime-flood relations (e.g., Bouaziz et al., 2022). Model sensitivity combined with multivariate statistical analysis will serve to disentangle the complex interactions among forest policy, forest cover change, climate variables, catchment characteristics, and anthropogenic alterations in influencing hydrological regimes and flood occurrence. Combining the degree of forest cover attributable to the forest law and forest-runoff analyses will allow us to answer the initially proposed question of whether an environmental law protecting forests can also reduce flood risk.
Figure 3. Data types and availability of the case study conducted in the Upper Rhone catchment. Panel (A) shows the canton Valais in relation to Switzerland. Panel (B) shows the delineated regions, with specific details on the five regions selected for further study. Panel (C) shows examples of data types available. For data sources please see the Supplementary Material.
In order to assess whether the required input data for the proposed approach are available, we divided the Upper Rhone catchment into 46 regions based on sub-catchments (Figures 3A,B). We then focused in depth data collection on five regions: Vouvry, Val d’Illiez, Lötschental, Val de Bagnes, and Zermattertal (Figure 3B). We found at least some relevant information for 44 out of the 46 regions (e.g., forest data or weather, hydrometric, or hydropower stations), though at times limited in scope (space and/or time). Only 19 sub-catchments had hydrometric stations that provided some type of flow rate (e.g., stage, discharge, velocity). Even the five most intensely investigated regions differed in the spatial and temporal resolution of the data (Table 2 and Figure 3B). Some have detailed flow rates for decades (e.g., Val de Bagnes), others are more limited (e.g., Vouvry), while documentation on forest cover is available over different periods and resolutions (e.g., Figure 3C). In general, data from these five sub-catchments are valuable and informative, but unfortunately present challenges.
Many reforestation projects are reported; between 1870 and 1980, there were 435 reforestation projects in Valais (Archival Documents, 2004). Not all projects, however, state the reason for, and success of, the reforestation. Aerial and terrestrial photography provide a data record of the forest cover change, but methods need to be developed to fill in spatial and temporal data gaps (i.e., see Section “Proposed Methodological Approach”).
Challenges in determining flood occurrence and risk are many-fold. Flow rates, and thus the ability to determine flood frequency, are only measured continuously/daily for a maximum of 46 years, starting a hundred years after the inception of the Forest Law. Newspaper articles provide some information on prior flood occurrence (data not presented), but the severity and definition of flood is unclear. A confounding factor evident in our five regions was that of hydropower installations. They can completely change the hydrology of a catchment, likely altering the influence the forest may exert on the hydrological regimes.
The results of our Upper Rhone catchment case study indicate that the input data needed to determine whether the Forest Law affected flood regimes (Figure 2) are available, but their collection and interpretation requires an interdisciplinary approach enabling a holistic study of the forest’s effects on hydrological regimes along changing social-ecological conditions. The information we presented indicates that data are not distributed evenly in space or time, but that catchments exist where enough data are available to develop models to fill data gaps. As our case study only covered a small portion of the Swiss mountain region with a specific climate, these models need to allow for the variation in driving climate variables and their interactions with forest ecosystems and hydrology. However, we posit that enough data can be found throughout Switzerland and potentially other countries with similar laws to evaluate the effects of mountain forest protection on flood risk using our methodological approach (Figure 2).
Our experiences made it clear that the team needed to undertake such research should include at least an environmental historian, a geographer, a forest scientist/engineer, and a hydrologist. There are two main parts to the overarching question, the first being how much did the forest law contribute to forest cover change. The answer to this question lies within the realm of interdisciplinary social sciences. The second question is what the effect of the forest is on hydrological regimes and flood behavior. The answer hereto lies within the realm of interdisciplinary natural sciences. We think that the answer to the overarching question raised at the beginning of the manuscript draws on the combination of social and natural science findings. We found it important that each discipline would have the opportunity to address discipline-specific questions within the framework of the interdisciplinary team. At the same time, all team members needed to be kept apprised of progress in all disciplines to adapt to new insights and not lose sight of the overarching, interdisciplinary research goal. Key requirements for success are that (i) the research team is interdisciplinary and interactions among disciplines are properly planned and monitored, (ii) the team is given enough time and resources for data collection on a large geographical scope and for frequent interactions, and (iii) the data collected and modeled will be combined with statistical analyses of hydrological data in relation to forest state change attributed to the law. Only a joint methodological and interdisciplinary approach can draw a clear conclusion on the effectiveness of the first Swiss Federal Forest Law and its role in flood protection.
In this perspective we posit that science can play a crucial role in monitoring and quantifying the potential effects of environmental policies on the provision of ecosystem services. We offer important insights on the complex interactions of an environmental law’s (assumed) scientific basis, its impacts on the environment, as well as the evaluation of its efficacy. In Alpine countries, the establishment of forest laws preventing deforestation in mountain areas has generally been regarded as a great success, although the scientific debate on their benefits remains. Thus, it is essential to enhance the current scientific basis of the Forest Law’s effects on the provisioning of flood protection services, determine the efficacy of the law in affecting reforestation and use models to link forest, climate, and hydrology. Findings based on our proposed interdisciplinary approach will not only be relevant for Alpine countries, but other mountain regions, such as the Himalaya or Taiwan, where similar conflicts exist due to the strong dependence of upland communities on wooden resources (e.g., Cheng et al., 2002; Chen et al., 2004; Wasson et al., 2008).
Our study emphasizes the necessity of a true interdisciplinary approach, combining a variety of methods from natural and social sciences (cf. Otero et al., 2020; Reynard et al., 2020). This allows for consideration and combination of a variety of data sources as well as different temporal and spatial scales relevant for assessing the impacts of forest changes on flood risk. The interdisciplinary framework we developed and presented here can further serve as an example for other ecosystem services, where similar questions on the effects of environmental practices and policies arise. Only a regular scientific questioning of the assumptions and purposes of environmental laws and policies can guarantee an effective management of ecosystem services.
The original contributions presented in this study are included in the article/Supplementary Material, further inquiries can be directed to the corresponding author/s.
JR, CM, AE, and GP obtained the funding for AG and GL to conduct the on-the-ground data collection for the feasibility study. All authors wrote sections of the manuscript, led by JR and CM who then joined and unified them. All authors contributed to the ideas in this manuscript through discussion during the feasibility study and provided multiple rounds of extensive comments on the entire manuscript.
Open access funding provided by University of Lausanne.
The authors declare that the research was conducted in the absence of any commercial or financial relationships that could be construed as a potential conflict of interest.
All claims expressed in this article are solely those of the authors and do not necessarily represent those of their affiliated organizations, or those of the publisher, the editors and the reviewers. Any product that may be evaluated in this article, or claim that may be made by its manufacturer, is not guaranteed or endorsed by the publisher.
We thank the University of Lausanne’s Interdisciplinary Centre for Mountain Research seed funding program for allowing us to explore the feasibility of answering the postulated research questions.
The Supplementary Material for this article can be found online at: https://www.frontiersin.org/articles/10.3389/ffgc.2022.785740/full#supplementary-material
Adams, M., Markart, G., and Stary, U. (2010). A comparison of hydrological models for forest management and climate change. How can existing models capture and reproduce hydrological effects of forests?. Innsbruck: Natural Hazards and Landscapes.
Alewell, C., and Bebi, P. (2011). “Forest development in the European Alps and potential consequences on hydrological regime,” in Forest management and the water cycle: An ecosystem-based approach, M. Bredemeier, S. Cohen, D. L. Godbold, E. Lode, V. Pichler, and P. Schleppi (Dordrecht: Springer).
Anderson, T. W. (1976). Evapotranspiration losses from flood-plain areas in central Arizona. Collins: US Geological Survey. doi: 10.3133/ofr76864
Andréassian, V. (2004). Waters and forests: from historical controversy to scientific debate. J. Hydrol. 291, 1–27. doi: 10.1016/j.jhydrol.2003.12.015
Archival Documents (2004). Identified via. Available online at: https://scopequery.vs.ch/suchinfo.aspx (accessed June 25, 2021).
Badoux, A., Jeisy, M., Kienholz, H., Lüscher, P., Weingartner, R., Witzig, J., et al. (2006). Influence of storm damage on the runoff generation in two sub-catchments of the Sperbelgraben, Swiss Emmental. Eur. J. For. Res. 125, 27–41. doi: 10.1007/s10342-005-0102-6
Bathurst, J. C., Bovolo, C. I., and Cisneros, F. (2010). Modelling the effect of forest cover on shallow landslides at the river basin scale. Ecol. Eng. 36, 317–327. doi: 10.1016/j.ecoleng.2009.05.001
Beven, K. J., and Germann, P. F. (2013). Macropores and water flow in soils revisited. Water Resour. Res. 49, 3071–3092. doi: 10.1002/wrcr.20156
Blotnitzki, L. (1869). Rapport au Département de l’Intérieur de la Confédération suisse sur les inondations de l’an 1868 en Valais. Geneva: Médiathèque Valais de Sion.
Bouaziz, L. J. E., Aalbers, E. E., Weerts, A. H., Hegnauer, M., Buiteveld, H., Lammersen, R., et al. (2022). Ecosystem adaptation to climate change: The sensitivity of hydrological predictions to time-dynamic model parameters. Hydrol. Earth Syst. Sci. 26, 1295–1318. doi: 10.5194/hess-26-1295-2022
Bradshaw, C. J. A., Sodhi, N. S., Peh, K. S.-H., and Brook, B. W. (2007). Global evidence that deforestation amplifies flood risk and severity in the developing word. Glob. Change Biol. 13, 2379–2395. doi: 10.1111/j.1365-2486.2007.01446.x
Brönnimann, S., Appenzeller, C., Croci-Maspoli, M., Fuhrer, J., Grosjean, M., Hohmann, R., et al. (2014). Climate change in Switzerland: a review of physical, institutional, and political aspects. WIRE’s Clim. Change 5, 461–481. doi: 10.1002/wcc.280
Buechel, M., Slater, L., and Dadson, S. (2022). Hydrological impact of widespread afforestation in Great Britain using a large ensemble of modelled scenarios. Commun. Earth Environ. 3:6. doi: 10.1038/s43247-021-00334-0
Calder, I. R. (2007). Forests and water - ensuring forest benefits outweigh water costs. For. Ecol. Manage. 25, 110–120. doi: 10.1016/j.foreco.2007.06.015
Calder, I. R., and Aylward, B. (2006). Forests and floods. Moving to an evidence-based approach to watershed and integrated flood management. Water Int. 31, 87–99. doi: 10.1080/02508060608691918
Cannon, S. H., Gartner, J. E., Rupert, M. G., Michael, J. A., Rea, A. H., and Parrett, C. (2010). Predicting the probability and volume of post-wildfire debris flows in the intermountain western United States. Geol. Soc. Am. Bull. 122, 127–144. doi: 10.1130/B26459.1
Chang, H., and Franczyk, J. (2008). Climate change, land-use change, and foods: toward an integrated assessment. Geogr. Compass 2, 1549–1579. doi: 10.1111/j.1749-8198.2008.00136.x
Chen, C. T. A., Liu, J. T., and Tsuang, B. J. (2004). Island-based catchment—The Taiwan example. Reg. Environ. Change 4, 39–48. doi: 10.1007/s10113-003-0058-3
Cheng, J. D., Lin, L. L., and Lu, H. S. (2002). Influences of forests on water flows from headwater watersheds in Taiwan. For. Ecol. Manage. 165, 11–28. doi: 10.1016/S0378-1127(01)00626-0
Culmann, C. (1865). Rapport au Conseil fédéral sur les torrents des Alpes suisses inspectés en 1858, 1859, 1860 et 1863. Lausanne: Imprimerie Corbaz & Comp.
Eisenbies, M. H., Aust, W. M., Burger, J. A., and Adams, M. B. (2007). Forest operations, extreme flooding events, and considerations for hydrologic modeling in the Appalachians—a review. For. Ecol. Manage. 242, 77–98. doi: 10.1016/j.foreco.2007.01.051
Fercher, M., Mueller, M. H., and Alaoui, A. (2018). Modelling the impact of land use changes on peak discharge in the Urseren Valley, Central Swiss Alps. Catena 163, 321–331. doi: 10.1016/j.catena.2017.12.032
Ferreira, S. J. F., Luizão, F. J., Ross, S., Biot, M. Y., and Mello-Ivo, W. M. P. (2004). Soil water storage in an upland forest after selective logging in Central Amazonia. Rev. Bras. Ciên. Solo 28, 59–66. doi: 10.1590/S0100-06832004000100006
Ford, C. (2004). Nature, culture and conservation in France and her colonies 1840-1940. Past Present 183, 173–198. doi: 10.1093/past/183.1.173
Gonzalez-Sosa, E., Braud, I., Dehotin, J., Lassabatère, L., Angulo-Jaramillo, R., Lagouy, M., et al. (2010). Impact of land use on the hydraulic properties of the topsoil in a small French catchment. Hydrol. Process. 24, 2382–2399. doi: 10.1002/hyp.7640
Guillemette, F., Plamondon, A., Prévost, P. M., and Lévesque, D. (2005). Rainfall generated stormflow response to clearcutting a boreal forest: peak flow comparison with 50 world-wide basin studies. J. Hydrol. 302, 137–153. doi: 10.1016/j.jhydrol.2004.06.043
Howard, A. D. (1967). Drainage analysis in geologic interpretation: a summation. AAPG Bull. 51, 2246–2259. doi: 10.1306/5D25C26D-16C1-11D7-8645000102C1865D
Jones, J. A., and Grant, G. E. (1996). Peak flow responses to clear-cutting and roads in small and large basins, western cascades, Oregon. Water Resour. Res. 32, 959–974. doi: 10.1029/95WR03493
Journal et Feuille d’avis du Valais (1906). N°103. Available online at: https://www.e-newspaperarchives.ch (accessed June 25, 2021).
Kelly, C. N., McGuire, K. J., Miniat, C. F., and Vose, J. M. (2016). Streamflow response to increasing precipitation extremes altered by forest management. Geophys. Res. Lett. 43, 3727–3736. doi: 10.1002/2016GL068058
Kirchner, J. W., Berghuijs, W., Allen, R., Allen, T. S., Hrachowitz, S. T. M., Hut, R., et al. (2020). Streamflow response to forest management. Nature 578, E12–E15. doi: 10.1038/s41586-020-1940-6
Kuczera, G. (1987). Prediction of water yield reductions following a bushfire in ash-mixed species eucalypt forest. J. Hydrol. 94, 215–236. doi: 10.1016/0022-1694(87)90054-0
Kühni, A., and Pfiffner, O. A. (2001). Drainage patterns and tectonic forcing: a model study for the Swiss Alps. Basin Res. 13, 169–197. doi: 10.1046/j.1365-2117.2001.00146.x
L’Ami du peuple (1906). N°63. Available online at: https://www.e-newspaperarchives.ch (accessed June 25, 2021).
Landolt, E. (1862). Rapport au Conseil fédéral sur les forêts des hautes montagnes de la Suisse inspectées dans les années 1858, 1859 et 1860. Lausanne: Imprimerie Corbaz & Comp.
Li, H., Zhang, Y., Vaze, J., and Wang, B. (2012). Separating effects of vegetation change and climate variability using hydrological modelling and sensitivity-based approaches. J. Hydrol. 42, 403–418. doi: 10.1016/j.jhydrol.2011.12.033
Li, Z., Liu, W., Zhang, X., and Zheng, F. (2009). Impacts of land use change and climate variability on hydrology in an agricultural catchment on the Loess Plateau of China. J. Hydrol. 377, 35–42. doi: 10.1016/j.jhydrol.2009.08.007
Loran, C., Ginzler, C., and Bürgi, M. (2016). Evaluating forest transition based on a multi-scale approach: forest area dynamics in Switzerland 1850–2000. Reg. Environ. Change 16, 1807–1818. doi: 10.1007/s10113-015-0911-1
Loran, C., Munteanu, C., Verburg, P., Schmatz, H., Bürgi, D. R. M., and Zimmermann, N. E. (2017). Long-term change in drivers of forest cover expansion: an analysis for Switzerland (1850-2000). Reg. Environ. Change 17, 2223–2235. doi: 10.1007/s10113-017-1148-y
Luo, P., He, B., Takara, K., Xiong, Y., Nover, E. D., Duan, W., et al. (2015). Historical assessment of Chinese and Japanese flood management policies and implications for managing future floods. Environ. Sci. Policy 48, 265–277. doi: 10.1016/j.envsci.2014.12.015
Mather, A., and Fairbairn, J. (2000). From floods to reforestation: the forest transition in Switzerland. Environ. History 6, 399–421. doi: 10.3197/096734000129342352
McEldowney, J., and McEldowney, S. (2011). Science and environmental law: collaboration across the double helix. Environ. Law Rev. 13, 169–198. doi: 10.1350/enlr.2011.13.3.128
Molnar, P., Anderson, R. S., and Anderson, S. P. (2007). Tectonics, fracturing of rock, and erosion. J. Geophys. Res. 112:F03014. doi: 10.1029/2005JF000433
Moore, G. W., and Heilman, J. L. (2011). Proposed principles governing how vegetation changes affect transpiration. Ecohydrology 4, 351–358. doi: 10.1002/eco.232
Moos, C., Bebi, P., Schwarz, M., Stoffel, M., Sudmeier-Rieux, K., and Dorren, L. (2018). Ecosystem-based disaster risk reduction in mountains. Earth Sci. Rev. 177, 497–513. doi: 10.1016/j.earscirev.2017.12.011
Neris, J., Jiménez, C., Fuentes, J., Morillas, G., and Tejedor, M. (2012). Vegetation and land-use effects on soil properties and water infiltration of Andisols in Tenerife (Canary Islands, Spain). Catena 98, 55–62. doi: 10.1016/j.catena.2012.06.006
Nijzink, R., Hutton, C., Pechlivanidis, I., Capell, R., Arheimer, B., Freer, J., et al. (2016). The evolution of root-zone moisture capacities after deforestation: a step towards hydrological predictions under change? Hydrol. Earth Syst. Sci. 20, 4775–4799. doi: 10.5194/hess-20-4775-2016
Otero, I., Darbellay, F., Reynard, E., Hetényi, G., Perga, M.-E., Rüegg, J., et al. (2020). Designing inter- and transdisciplinary research on mountains: what place for the unexpected? Mt. Res. Dev. 40, D10–D20. doi: 10.1659/MRD-JOURNAL-D-20-00036.1
Pellicciotti, F., Bauder, A., and Parola, M. (2010). Effect of glaciers on streamflow trends in the Swiss Alps. Water Resour. Res. 46:W10522. doi: 10.1029/2009WR009039
Peltier, A. (2005). La gestion des risques naturels dans les montagnes d’Europe occidentale. Etude comparative du Valais (Suisse), de la Vallée d’Aoste (Italie) et des Hautes-Pyrénées (France). Available online at: https://tel.archives-ouvertes.fr/tel-00078352 (accessed June 25, 2021).
Pfister, C. (2002). Le jour d’après: surmonter les catastrophes naturelles: le cas de la Suisse entre 1500 et 2000. Stuttgart: Haupt.
Reynard, E., Otero, I., and Clivaz, M. (2020). The Interdisciplinary Centre for Mountain Research (CIRM): fostering transdisciplinarity for transformation research in mountains. Mt. Res. Dev. 40, 1–3. doi: 10.1659/MRD-JOURNAL-D-20-00051.1
Reichen, Q. (2013). Tourismus: Historisches Lexikon der Schweiz (HLS). Available online at: https://hls-dhs-dss.ch/de/articles/015795/2008-11-25 (accessed June 25, 2021).
Reynard, E., Mauch, C., and Thorens, A. (2001). “Développement historique des régimes institutionnels de l’eau en Suisse entre 1870 et 2000,” in Régimes institutionnels de ressources naturelles: analyse comparée du sol, de l’eau et de la forêt, eds I. Kissling-Naf, P. Knoepfel, and F. Varone (Bâle: Helbing and Lichtenhaln).
Roy, S. G., Koons, P., Upton, O. P., and Tucker, G. E. (2015). The influence of crustal strength fields on the patterns and rates of fluvial incision. J. Geophys. Res. 120, 275–299. doi: 10.1002/2014JF003281
Scheidl, C., Heiser, M., Kamper, S., Thaler, T., Klebinder, K., Nagl, F., et al. (2020). The influence of climate change and canopy disturbances on landslide susceptibility in headwater catchments. Sci. Total Environ. 742:140588. doi: 10.1016/j.scitotenv.2020.140588
Scherrer, S. C., Fischer, E., Posselt, M. R., Liniger, M., Croci-Maspoli, A. M., and Knutti, R. (2016). Emerging trends in heavy precipitation and hot temperature extremes in Switzerland. J. Geophys. Res. 121, 2626–2637. doi: 10.1002/2015JD024634
Schuler, A. (2007). Lois sur les forêts. Available online at: https://hls-dhs-dss.ch/articles/013802/2007-08-17/
Schwarz, M., Cohen, D., and Or, D. (2012). Spatial characterization of root reinforcement at stand scale: theory and case study. Geomorphology 17, 190–200. doi: 10.1016/j.geomorph.2012.05.020
Seebald, J., Senf, C., Heiser, M., Scheidl, C., Pflugmacher, D., and Seidl, R. (2019). The effects of forest cover and disturbance on torrential hazards: Large-scale evidence from the Eastern Alps. Environ. Res. Lett. 14:114032. doi: 10.1088/1748-9326/ab4937
SFOE (2020). Swiss Federal Office of Energy. Available online at: https://web.archive.org/web/20180813065300/http://www.bfe.admin.ch/themen/00490/00491/index.html?lang=en (accessed Oct. 1, 2020)
Speich, M. J. R., Zappa, M., Scherstjanoi, M., and Lischke, H. (2020). FORests and HYdrology under climate change in Switzerland v1.0: a spatially distributed model combining hydrology and forest dynamics. Geosci. Model Dev. 13, 537–564. doi: 10.5194/gmd-13-537-2020
Storck, P., Bowling, L., Wetherbee, P., and Lettenmaier, D. (1998). Application of a GIS-based distributed hydrology model for prediction of forest harvest effects on peak stream flow in the Pacific Northwest. Hydrol. Process. 12, 889–904. doi: 10.1002/(SICI)1099-1085(199805)12:6<889::AID-HYP661>3.0.CO;2-P
Stuber, M., and Bürgi, M. (2001). Agrarische Waldnutzungen in der Schweiz 1800-1950. Waldweide, Waldheu, Nadel- und Laubfutter. Schweizerische Z. Forstwesen 152, 490–508. doi: 10.3188/szf.2001.0490
Summermatter, S. (2005). Die Überschwemmungen von 1868 in der Schweiz: unmittelbare Reaktion und längerfristige Prävention mit näherer Betrachtung des Kantons Wallis. Nordhausen: Verlag Traugott Bautz GmbH.
van Dijk, A., van Noordwijk, M., Calder, I., Bruijnzel, S., Schellekens, J., and Chappell, N. A. (2009). Forest-flood relation still tenuous - comment on ‘global evidence that deforestation amplifies flood risk and severity in the developing world’ by C.J.A, Bradshaw, N.S. Sodi, K.S.-H. Peh and B.W. Brook. Glob. Change Biol. 15, 110–115. doi: 10.1111/j.1365-2486.2008.01708.x
Verbunt, M., Zwaaftink, M. G., and Gurtz, J. J. (2005). The hydrologic impact of land cover changes and hydropower stations in the Alpine Rhine basin. Ecol. Model. 187, 71–84. doi: 10.1016/j.ecolmodel.2005.01.027
Vereecken, H., Schnepf, A., Hopmans, J., Javaux, W. M., Or, D., Roose, T., et al. (2016). Modeling soil processes: review, key challenges, and new perspectives. Vadose Zone J. 15:vzj2015.09.0131. doi: 10.2136/vzj2015.09.0131
Viglione, A., Merz, B., Viet Dung, N., Parajka, J., Nester, T., and Blöschl, G. (2016). Attribution of regional flood changes based on scaling fingerprints. Water Resour. Res. 52, 5322–5340. doi: 10.1002/2016WR019036
Wahren, A., Schwärzel, K., and Feger, K.-H. (2012). Potentials and limitations of natural flood retention by forested land in headwater catchments: evidence from experimental and model studies. J. Flood Risk Manage. 5, 321–335. doi: 10.1111/j.1753-318X.2012.01152.x
Wasson, R. J., Juyal, N., Jaiswal, M., McCulloch, M., Sarin, M., Jain, M. V., et al. (2008). The mountain-lowland debate: Deforestation and sediment transport in the upper Ganga catchment. J. Environ. Manage. 88, 53–61. doi: 10.1016/j.jenvman.2007.01.046
Weiss, G. (2001). Mountain forest policy in Austria: a historical policy analysis on regulating a natural resource. Environ. History 335–355.
Woolsey, S., Weber, C., Gonser, T., Hoehn, E., Hostmann, M., Junker, B., et al. (2005). Handbuch für die Erfolgskontrolle bei Fliessgewässerrevitalisierungen. Publikation des Rhone-Thur Projektes. Dübendorf: Eawag.
Keywords: environmental law, ecosystem service, forest protection and management, flood protection and management, forest-hydrology interaction, forest-society interaction
Citation: Rüegg J, Moos C, Gentile A, Luisier G, Elsig A, Prasicek G and Otero I (2022) An Approach to Evaluate Mountain Forest Protection and Management as a Means for Flood Mitigation. Front. For. Glob. Change 5:785740. doi: 10.3389/ffgc.2022.785740
Received: 29 September 2021; Accepted: 22 June 2022;
Published: 11 July 2022.
Edited by:
Andrea Pieroni, University of Gastronomic Sciences, ItalyReviewed by:
Taehee Hwang, Indiana University Bloomington, United StatesCopyright © 2022 Rüegg, Moos, Gentile, Luisier, Elsig, Prasicek and Otero. This is an open-access article distributed under the terms of the Creative Commons Attribution License (CC BY). The use, distribution or reproduction in other forums is permitted, provided the original author(s) and the copyright owner(s) are credited and that the original publication in this journal is cited, in accordance with accepted academic practice. No use, distribution or reproduction is permitted which does not comply with these terms.
*Correspondence: Janine Rüegg, amFuaW5lLnJ1ZWdnQHVuaWwuY2g=
†Present address: Alexandre Elsig, College of Humanities, Swiss Federal Institute of Technology (EPFL), Lausanne, Switzerland
Disclaimer: All claims expressed in this article are solely those of the authors and do not necessarily represent those of their affiliated organizations, or those of the publisher, the editors and the reviewers. Any product that may be evaluated in this article or claim that may be made by its manufacturer is not guaranteed or endorsed by the publisher.
Research integrity at Frontiers
Learn more about the work of our research integrity team to safeguard the quality of each article we publish.