- 1Instituto de Ecología Regional, Universidad Nacional de Tucumán-Consejo Nacional de Investigaciones Científicas y Técnicas, Tucumán, Argentina
- 2Facultad de Ciencias Naturales e Instituto Miguel Lillo, Universidad Nacional de Tucumán, Tucumán, Argentina
Most studies on forest transition (FT) have focused on temporal patterns of forest cover across whole countries or regions, without much consideration of the local spatio-temporal heterogeneity or the species composition of new forests. We hypothesize that peri-urban forest transitions are driven by processes associated with urban-based economies and functioning, which define spatial characteristics of new forests. We analyzed the patterns of peri-urban forest expansion detected during the past three decades in the Lules watershed, a montane area of ca. 100,000 ha around San Miguel de Tucumán (SMT), an urban center of ca. one million inhabitants in the Andean foothills of subtropical Argentina. New forests (4,600 ha) were observed through the watershed, but they were more frequent at short distances (1–15 km) from the urban and second home residential areas. New forests nearby SMT are characterized by higher plant diversity largely due to a higher proportion of exotic species. In some cases, these exotic species dominated new forests diminishing stand-level biodiversity. Second-home residential areas, a byproduct of the urban centers in intermontane valleys, replicate the spatial patterns of exotic-dominated forest transition observed around SMT. We argue that peri-urban FT provides an early characterization of the future patterns of spontaneous forest transition with increased presence of globalized species which may locally increase species richness. We use the case study to discuss emerging research lines and to emphasize the importance of urban-centered land use policies (e.g., associated to urban-based uses such as recreation or watershed conservation) as key targets for promoting new forests more beneficial for future generations.
Introduction
New land uses and human activities generate rapid changes in ecosystems. Although the expansion of croplands over natural systems is one of the most evident land cover changes, many developed countries experienced a reversal toward reforestation (“forest transition” Mather, 1992; Redo et al., 2012). The woody cover expansion is an ongoing process registered in many regions around the world (Rudel et al., 2005) and although deforestation is the dominant trend in developing countries, recovery of forests has also been observed in Latin America and Asia (Ashraf et al., 2017; Nanni et al., 2019). Forest transitions have been studied mainly at continental/country scales, without much distinctinction of local heterogeneity and species composition.
At a local scale, large urban centers may influence forest expansion (Grau et al., 2003; Parés-Ramos et al., 2008; Gutierrez Angonese and Grau., 2012; Salvati et al., 2017). Several cities have developed programs to increase the provision of ecosystem services and reduce the negative impacts of the expansion of built areas, resulting in the reforestation of peri-urban areas (Salvati et al., 2017). There is also a spontaneous process of agricultural abandonment as a consequence of socioeconomic changes leading to forest recovery that are more intense near urban areas (Grau et al., 2008).
In addition, it is frequent that around prosperous cities, rural systems are transformed into areas of second homes or exurbias (Shaw et al., 2020; Golding, 2021). These new developments remain connected and depend on the main urbanizations (e.g., roads, economic activity, governance, cultural influence) forming a complex system that may promote forest recovery. These settlements are usually driven by urban residents looking for greater contact with nature. Newcomers modify the local economic system favoring a transition from agriculture-livestock toward tourism, services and real estate economies, which may accelerate the process of land abandonment. One of the drivers of second homes development or exurbanization is the positive appraisal of nature by newcomers, which leads to landscape design, introduction of ornamental exotic species or forest protection in rural landscapes. The proximity of abandoned land to urbanized areas may influence the species composition of new forests favoring the arrival and dispersal of exotic plant species from home gardens. Exotic species may integrate with the local flora and some of them become naturalized or even invasive (Dehnen Schmutz et al., 2007; Montti et al., 2017).
San Miguel de Tucuman (SMT) is the largest city in northwestern Argentina (ca. one million inhabitants), and the fifth of the country, located in the foothills between subtropical montane systems and the plains. The development of the sugar industry associated with sugarcane plantations since the 19th century, determined an early deforestation of plain areas and the development of a prosperous city. Recently high-income urban populations began to move to semi-natural, well-connected areas where marginal economic activities have been outcompeted by mechanized agriculture in the plains. During the past decades, peri-urban forest transition associated with environmental and socio-economic processes have been documented (Grau et al., 1997, 2008, 2010; Carilla et al., 2006; Gutierrez Angonese and Grau, 2014; Malizia et al., 2017; Jimenez et al., 2021). Permanent plots of secondary forests located close to SMT have been monitored for more than 30 years, which allow analyzing the impact of the forest dynamics on biodiversity and biomass storage (Grau et al., 1997; Malizia et al., 2017; Ceballos et al., 2021). In addition to field studies, transitions have been monitored using satellite images since 1970, and the association of forest expansion with multiple biophysical and socio-economic variables was analyzed (Gutierrez Angonese and Grau, 2014; Jimenez et al., 2021).
In this paper we analyze spontaneous peri-urban forest transition spatial patterns based on field surveys and remote sensing data and explore the role of accessibility on the introduction of exotic species in a subtropical mountain watershed. Specifically, we analyze (1) The association between naturally regenerated forest and accessibility to urban centers and (2) The impact of proximity to urban areas on the percentage of exotic plant species at different scales.
Materials and Methods
Study Area
We focused on the Lules watershed (26°37′56″– 27°01′00″S and 65°29 07″–65°15′69″W), a 100,000 ha (Figure 1) mountain watershed in Tucumán Province, northwestern Argentina. In the lowlands of this watershed (i.e., below 600 masl) most natural vegetation has been replaced by agriculture and urban areas, this sector includes part of the city of SMT, due there is no possibility of reforestation in this sector, lower basin was not included in forest transition analysis. The middle-upper watershed ranges from 600 to 4,500 masl and covers an area of approximately 80,000 ha. This includes built-up areas in mountain valleys that are frequently used as second homes (Grau, 2011; Viollaz and Casares, 2017; Jimenez et al., 2021), such as Villa Nougués (ca. 1350 masl), Anta Muerta (ca. 1,200 masl), La Sala (ca.1,100 masl) and El Siambón (ca.1,200 masl; Figure 1). Villa nougués, founded in 1899, is the oldest second home area in the watershed. Anta Muerta, El Siambón and La Sala are localities historically oriented to livestock ranching and horticultural cultivation. Anta Muerta and El Siambón have reoriented their economy toward tourism since the 1970s and La Sala has recently been reoriented to real estate development (since ca. 2001).
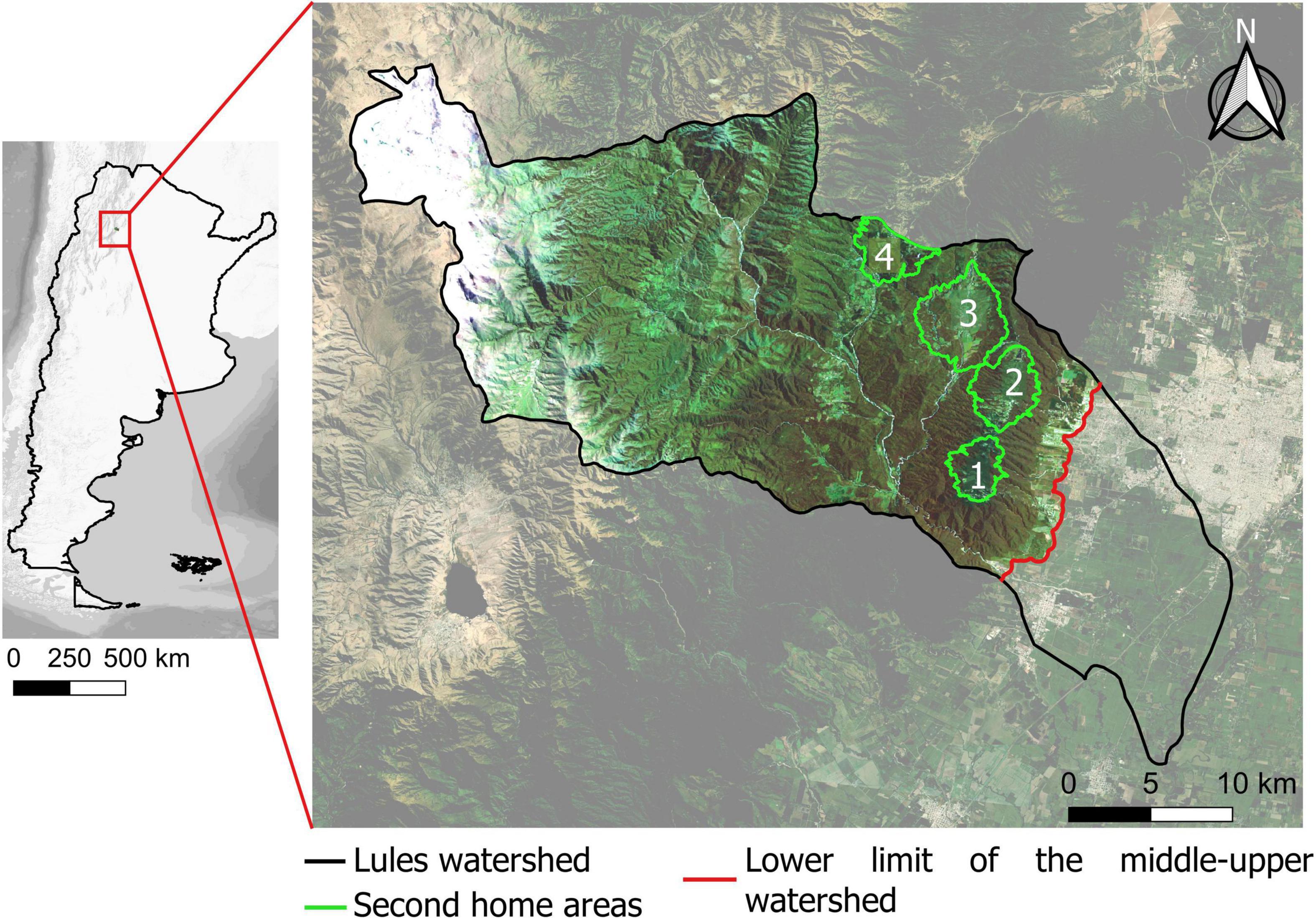
Figure 1. Middle-upper Lules watershed. Second home areas localization: (1) Villa Nougués; (2) Anta Muerta; (3) La Sala; (4) El Siambón.
In the study area the climate is subtropical with rainfall concentrated between October and March. Mean annual precipitation varies in the altitudinal gradient between 300 and 2,300 mm and the mean annual temperature in the lowlands is 21 ± 6°C (Malizia et al., 2012; Lomáscolo et al., 2014).
Most of the watershed is covered with subtropical montane forest (also known as Yungas), which can be divided into altitudinal vegetation belts. The Lower Montane forest (600–1,600 masl) includes canopy species such as Blepharocalyx salicifolius, Ocotea porphyria, and Pisonia zapallo, while the subcanopy is dominated by Eugenia uniflora, Piper tucumanum, and Allophylus edulis (Grau, 2002; Malizia and Grau., 2006). In this altitudinal gradient there are secondary forest patches dominated by exotic tree species such as Ligustrum lucidum or Gleditsia triacanthos (Ceballos et al., 2020; Jimenez et al., 2021), registered as invasive species in several regions of Argentina (Fernandez et al., 2017, 2020). Vegetation in the Upper Montane forest (1,600–2,300 masl) is dominated by Alnus acuminata, Podocarpus parlatorei, and species of Mirtaceae family alternating with grassland and shrublands (Carilla and Grau, 2011; Malizia et al., 2012). Above the treeline, up to 3,800 masl, vegetation is dominated by high grasslands used for livestock ranching.
Methodology
This study was carried out at different spatial scales. The analysis of forest expansion using satellite data was developed in the middle-upper watershed which hosts most of the natural vegetation and where land with potential for reforestation remains available. On other hand, the analysis of exotic species was carried out in the whole watershed and at plot level, combining free access databases (e.g., herbaria) and own field data (Supplementary Figure 1).
Forest Transition
Data Set
Land cover classifications were based on Landsat TM images; Landsat missions provides images of the Earth’s surface since 1972 with a temporal resolution of 16 days and a spatial resolution of 30 m.1 We selected images from Landsat 5 ETM sensor and Landsat 8 OLI/TIRS sensor to cover the period from 1989 to 2017. Landsat 5 images contain 4 visible and near-infrared bands, 2 short-wave infrared bands and one thermal infrared band. Landsat 8 images contain 5 visible and near-infrared bands, 2 short-wave infrared and one thermal infrared (see text footnote 1).
All the images are available in the Surface Reflectance Collection (SR) of Google Earth Engine, which are radiometrically and atmospherically corrected to surface reflectance (methods for surface reflectance estimation are available at https://developers.google.com/earth-engine/guides/landsat). Original Landsat images are supplied by the United States Geological Survey Earth Resources Observation and Science Data Center (USGS).
We filtered images that had less than 20% cloud coverage. For every year we considered all the available images to calculate the median of each band during the wet (October to May) and dry (June to September) periods, except for 2002, when no images were available.
The information of the Blue, Green, Red, near infrared (NIR), and shortwave infrared SWIR (median of the wet and dry period) bands were used to classify different land covers. We also included a digital elevation map (SRTM-DEM) and NDVI of the dry period.
Land Cover Maps
To create land cover maps for each year from 1989 to 2017 we used the random forest classifier (RF). RF is a potent machine learning classifier achieving high precision to differentiate land cover classes (Talukdar et al., 2020). RF works with an assembly of decision trees, each tree votes for a class and the most voted class becomes the model’s prediction for each pixel (Pal, 2005).
The supervised classifier requests a training data set to predict land cover classes (Pal, 2005). We defined six land cover classes a priori: forest, cropland, shrubland, pasture/grassland, bare soil, and urban area. We sampled 12,633 pixels belonging to the different land covers in 2017 using field data and high-resolution images from Google Earth Pro. The sampled points were used in the classification of all the years (from 1989 to 2017) to parameterize RF classifier: 7,941 as training data (70%) and 4,692 pixels were used to validate the classification (30%).
We executed RF in the geospatial processing service Google earth engine. Our classification was based on the 12 spectral bands previously mentioned, we included bands from the dry and wet seasons to improve the discrimination of similar land cover classes because it represents the phenological patterns of vegetation (Rodriguez-Galiano et al., 2012). The bands were standardized to allow interanual comparisons.
We evaluated the accuracy of classifications by estimating the Kappa value and we discarded the classifications corresponding to the years 1993, 1997, and 2012 because they had low precision. The remaining classifications had a kappa value greater than 80, which is considered as a strong agreement (Congalton, 2001). As pasture/grassland was difficult to discriminate from croplands in the middle watershed we combined both classes. Natural grasslands are present only in the upper watershed, so the class that grouped pasture/grasslands and croplands in the middle watershed correspond to anthropogenic uses (farmland). These land cover maps allowed us to quantify forest recovery through the years from the whole middle-upper watershed and for each second home area (Villa Nougués, Anta Muerta, La Sala, and El Siambón). We considered that forest expansion (forested pixel) occurs when a pixel transitions from non-forest land (e.g., crops, pastures, shrubs, bare soil) to forest class in land cover maps of consecutive years.
Accessibility Map
We created an accessibility map from SMT and second home localities (starting points). This map represents the time (minutes) that it takes to arrive by vehicle and walking (beyond road reach) from starting points to any point of the watershed. To elaborate the accessibility maps we performed a cost distance analysis using ArcGis. This analysis requires a raster with the starting points and a cost map as inputs.
First, we calculated the time (minutes) from starting points traveling by roads using the maximum allowed speed as a cost map. Then, we estimated the walking time from roads (for computational simplicity we used 338 points evenly distributed along roads as starting points) to each pixel in the study area. The walking cost map was estimated using Tobler’s hiking function, which allows estimating the hiking speed taking into account the slope of the terrain (Tobler, 1993). Accessibility, modeled with this function, has previously been used as an indicator of the influence of human settlements on land cover changes (Teferi et al., 2013; Navarro et al., 2020).
Once we obtained accessibility maps for each starting point, the rasters were overlapped and we kept the lowest value of accessibility for each pixel (i.e., less arrival time means the pixel is more accessible from SMT and second home localities, Figure 2).
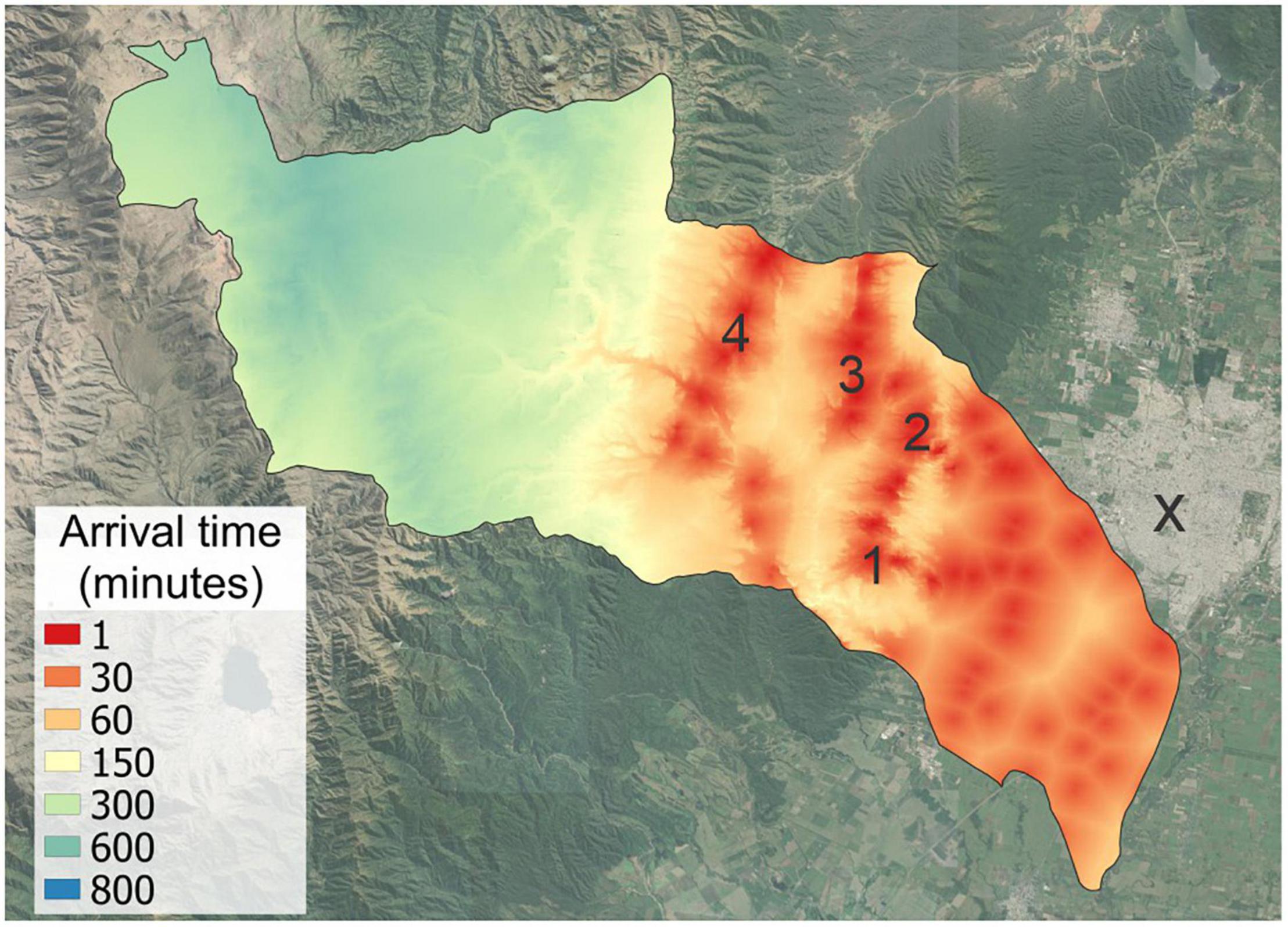
Figure 2. Accessibility from SMT (“X”) and second home localities: (1) Villa Nougués; (2) Anta Muerta; (3) La Sala; (4) El Siambón. This map represents the time that it takes to arrive by vehicle and walking (beyond road reach) from urban sites to any point of the watershed.
Forest Transition Around SMT and Second Home Localities
We quantified the density of pixels with new forests (naturally regenerated forest) and land with potential for reforestation (croplands, pasture/grasslands, shrublands) for each year (post 1990) in the middle-upper watershed and around the second home localities.
To reduce the probability of overestimating land cover transitions, we applied an additional filter. When a pixel classified as “non-forest” in the year i was “forest” in years i-1 and i + 1, we reclassified it as “forest”. In the same way, if a pixel was classified as “forest” in the year i and as “non-forest” in years i – 1 and i + 1, we reclassified it as non-forest. For this analysis we eliminated the first (1989) and last classifications (2017) since they could not be compared with years i – 1 and i + 1, respectively.
We considered the pixels that were forests in 1990 as a reference, and we counted the new forests per year starting in 1991. We analyzed the forest transition pattern over the years and at different distances from SMT using a multiple ring buffer with an equidistance of 1 km. This information was used to elaborate a heat map of forest pixel density. The heat map allows us to visualize the magnitude of density of new forests (pixels/km2) in a color-shaded graph with two dimensions (time and space) (Wilkinson and Friendly, 2009). In addition, we quantified forest recovery around the four second home localities. We delimited the area of influence of second home localities using the accessibility maps from the starting points in these localities, taking the pixels that were less than 60 min away (Figure 1). This threshold was defined considering low mobility cost and avoiding overlap between areas.
Forest Transition and Proximity to Urban Centers
To analyze the association between new naturally regenerated forest and proximity to urban areas we elaborated a map of forest gain between 1991 and 2016. We masked the pixels that were forests in 1990 (that is, areas without reforestation possibility) and we obtained a binomial map of reforestation (1 = forest expansion, 0 = non-forest expansion). For this analysis we used the accessibility map (from SMT and second home localities) as a predictor variable together with a digital elevation map (SRTM-DEM, resolution 30 m) and a slope map estimated from the SRTM-DEM (in degrees). Finally, we performed a generalized linear model (glm) between forest expansion and accessibility, elevation and slope using data between 600 and 3,000 masl. Although we used regression analyses, which are usually used in causal studies, as we did not perform experimental studies, we could not determine causal relationship, but rather spatial associations between variables. We used a binomial distribution and logit as a link function in R (R Development Core Team, 2021).
Exotic Species in Proximity to Urban Centers
We analyzed the association between exotic plant presence and distance to urban centers in the whole Lules watershed at watershed and plot scales (Supplementary Figure 1).
We divided the Lules watershed into quadrants of 2.5 km × 2.5 km. For each quadrant we collected species presence points (i.e., localities where a species was recorded) from different sources including Global Biodiversity Information Facility (GBIF), herbaria, forest permanent plots and floristic inventories (Ayup et al., 2014; Pero et al., 2015, Fernandez et al., 2016; Ceballos, 2019). We considered only quadrants with three or more species presence points, which cover an elevation gradient from 396 to 2,090 masl. We collected data from 1,466 species presence points including trees, shrubs, herbs, lianas, and epiphytes that belonged to 321 plant species, 73 of which were exotic. For each quadrant, we obtained (1) the percentage of exotic plants using the data of species presence points, (2) an accessibility value using the access map from SMT and second home localities, and (3) the elevation of the centroid using a digital elevation model.
At the plot scale, we used tree data from 22 forest plots distributed in the area (i.e.,17 were permanent plots and five were plots with a single vegetation inventory). Permanent plots are part of the Red Subtropical de Parcelas Permanentes (RedSPP2) that provides data of long-term plot monitoring of subtropical montane forests of Argentina. Plots were established in secondary and mature forests with different abundances of exotic tree species. We used data from vegetation surveys performed between 2016 and 2017 in 1.6-ha plot, 6.1-ha plots, and 15 plots between 0.12 and 0.48 ha. For each plot, we used the abundance of exotic and native trees to calculate the percentage of exotic tree individuals. For the centroid of each plot, we extracted data from a digital elevation map (SRTM-DEM) and from the accessibility map from SMT and second home localities.
In addition to their differences in scale, the watershed level considered a longer elevation gradient and different plant life forms, while the plot level considered only trees and a shorter gradient due to the distribution of the plots. However, the plot level included abundance data, while the watershed level considered only occurrence data (number of exotic species present and 0 when no exotic species were recorded, Supplementary Table 5). At both scales, the association between exotic plant presence with accessibility from urban areas or second home localities were analyzed using generalized linear models in R (R Development Core Team, 2021). Exotic plant presence was represented as the percentage of exotic plant species for the watershed scale, and the percentage of exotic tree individuals for the plot scale.
Results
In 2016 Lules watershed had 50,970 ha of forests (they covered 60% of the middle-upper watershed area) with an annual gross forest gain of 0.9% (400 ± 170 ha/year) between 1991 and 2016 (Figure 3). Because some areas had also experienced deforestation, the result was a net increase of 4,600 ha in 25 years (Supplementary Table 1).
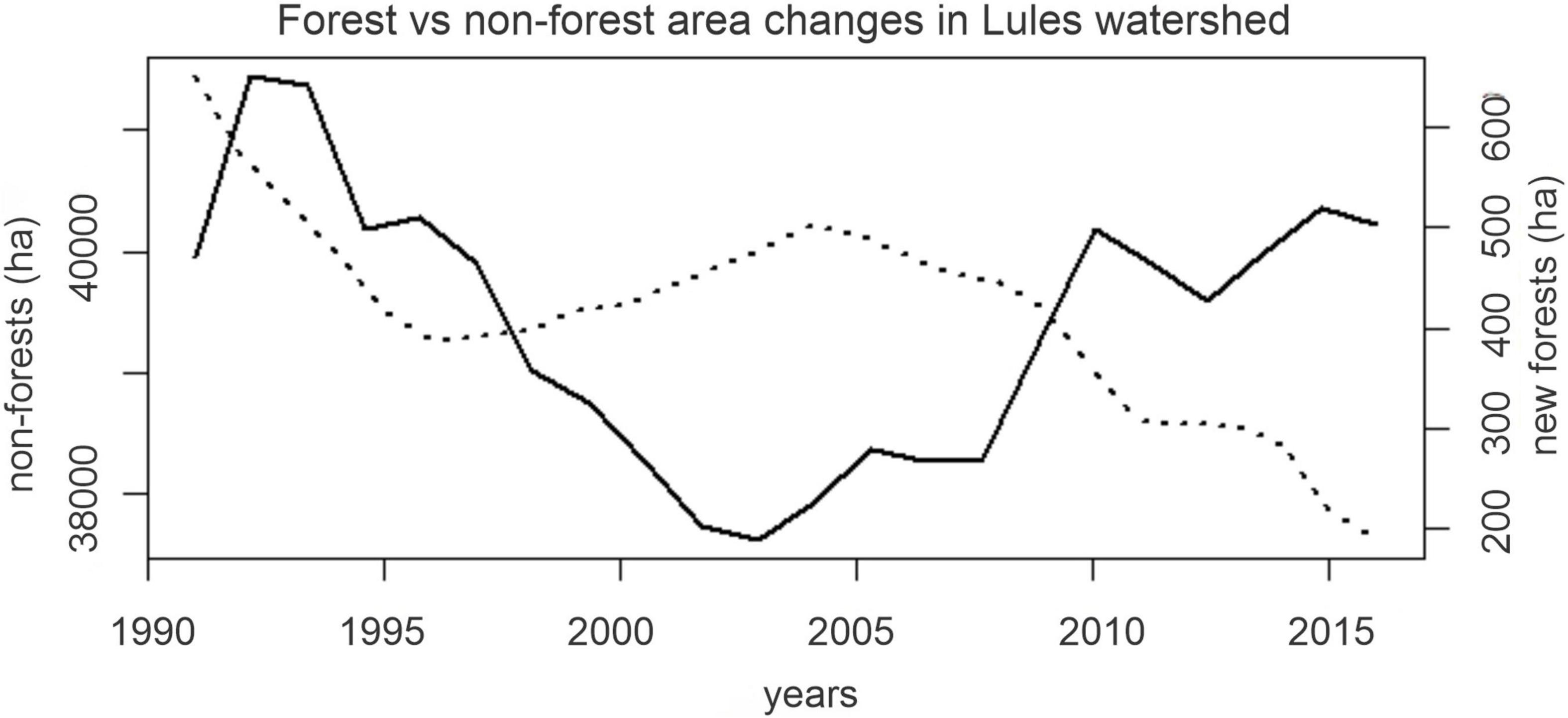
Figure 3. Gross annual forest gain (solid line) and existing non-forest areas (cropland, shrubland and grasslands) (dotted line) in the middle-upper Lules watershed.
According to the heatmap a disproportionately large share of the reforestation occurred in the vicinity of second home localities, mainly over farmland, and the remainder occurred in remote areas (20–30 km from SMT) in the ecotone between forests and high-altitude grasslands/shrublands (∼2,400 masl) concentrated at the beginning (until 1999) and at the end of the study period (after 2009) (Figure 4).
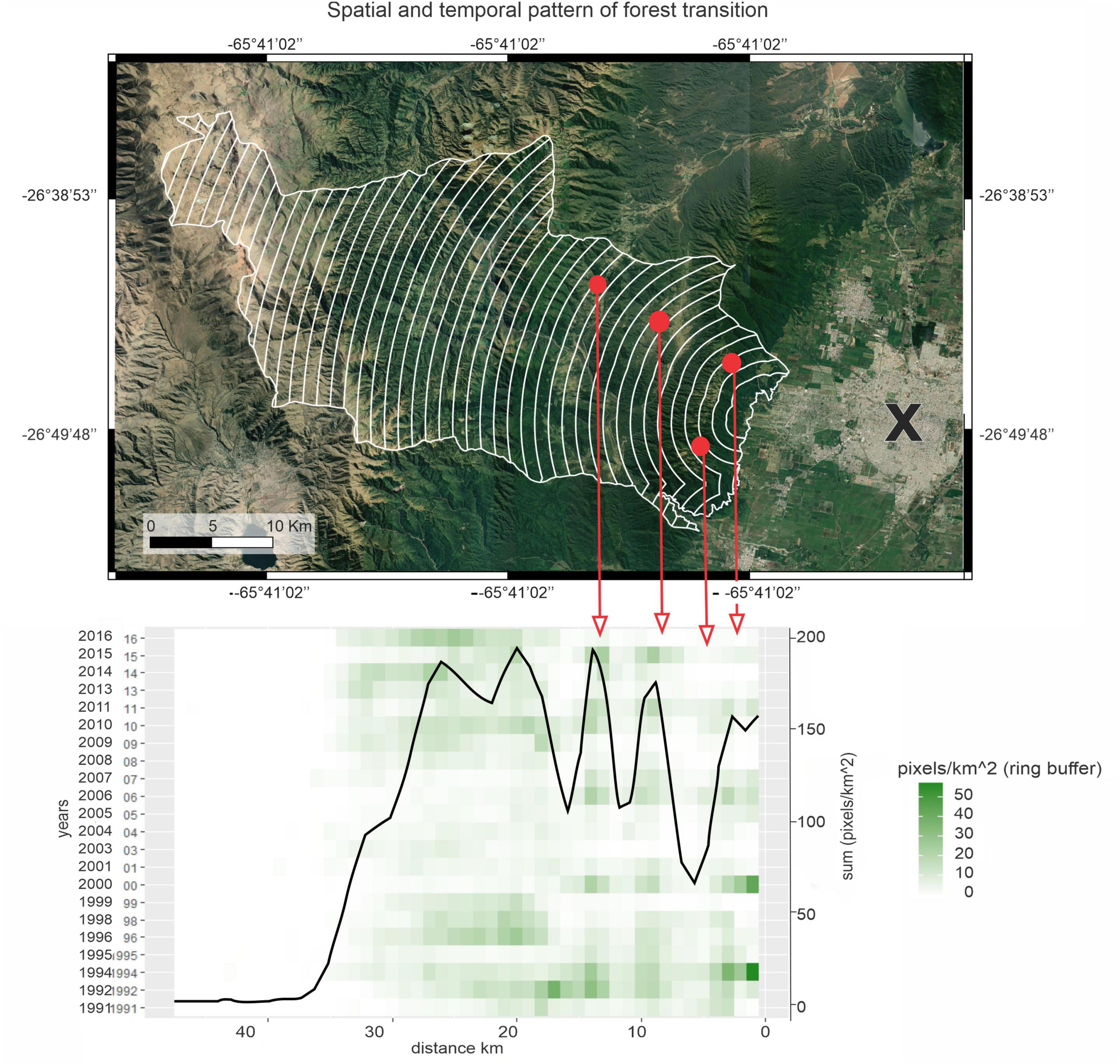
Figure 4. The white lines in the upper map represent linear distances from SMT estimated through concentric 1km rings, red points represent second home areas, and the “X” indicates SMT ubication (26°49′47″S and 65°12′’51″W). The heatmap (below) illustrates the proportion annual forest gain in relation to the distance to SMT, darker cells indicate increased forest gain (pixels/km2). Black line represents the sum of data obtained for each distance class to SMT (total of forested pixels/km2, right y- axis), over the 24-year study period. The peaks of the black line (areas that gained most forests) coincide with the location of the second home areas (indicated with the red arrows).
In the middle watershed (from 600 to 1,500 masl) there were 5,494 ha of non-forest lands in 1991. Of the total land available around second home localities, 30% was reforested (575 ha) while in the neighboring areas only 23% (849 ha) gained new forests (Table 1 and Supplementary Tables 2, 3). A large proportion of new forests in second home areas were recorded at the beginning of the study period (Figure 5).
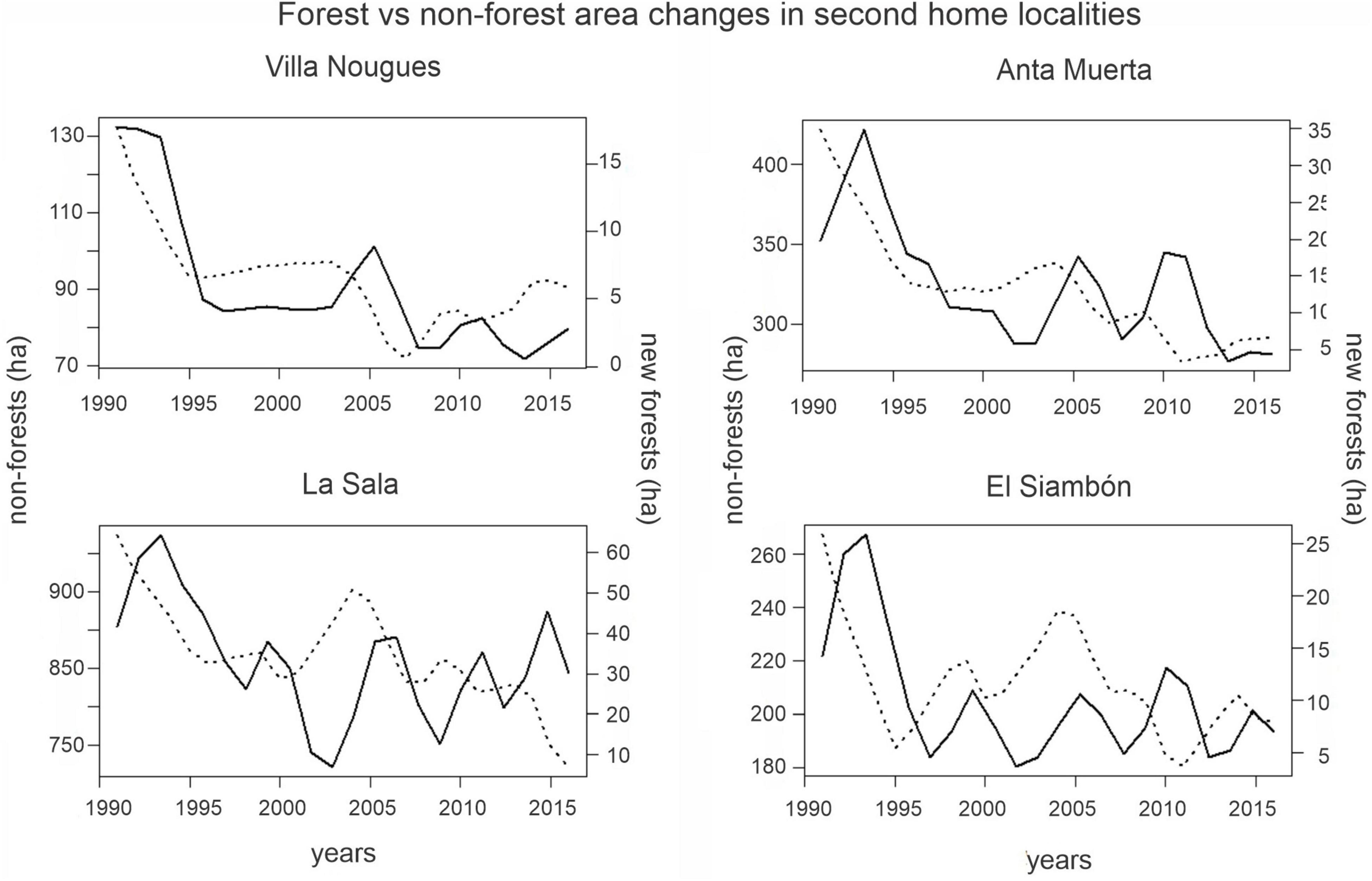
Figure 5. Gross forest gain (solid line) and non-forest areas: cropland, shrubland, and grasslands (dotted line) in the surroundings of second home localities.
Forest expansion showed a negative association with accessibility (arrival time) from SMT and second home localities (–0.0026, p < 0.001, Supplementary Table 4). According to our results, considering an average altitude and slope (2,000 masl and 20°, respectively), the probability of reforestation is maximum (22–25%) in areas that are less than 60 min from SMT and second home localities, while this value decreases in areas located more than 300 min (<13%). Both topographic variables included in the model had a significant effect on forest expansion; the probability of reforestation was higher in accessible areas of medium elevations (between 1,000 and 1,700 masl) and steeper slopes (Supplementary Table 4).
At watershed scale, the percentage of exotic plant species (0–100%) was negatively related to accessibility (arrival time) (estimate = –0.31, t = –5.57, p < 0.001) and elevation (estimate = –0.19, t = –4.72, p < 0.001). In other words, the percentage of exotic species was higher in accessible areas at lower elevations, generally in areas located less than 50 min from SMT and second home localities and less than 1,500 masl (Figure 6). At a plot scale, the percentage of exotic tree individuals (0–44%) was negatively related to accessibility (estimate = –3.91, t = –7.68, p < 0.001) but it was not related to elevation (estimate = –0.81, t = –0.91, p = 0.33). Compared to the watershed level, the percentage of exotic tree individuals was higher in plots located at more accessible areas (i.e., less than 40 min from SMT and second home localities), and lower elevations (i.e., less than 900 masl; Figure 6).
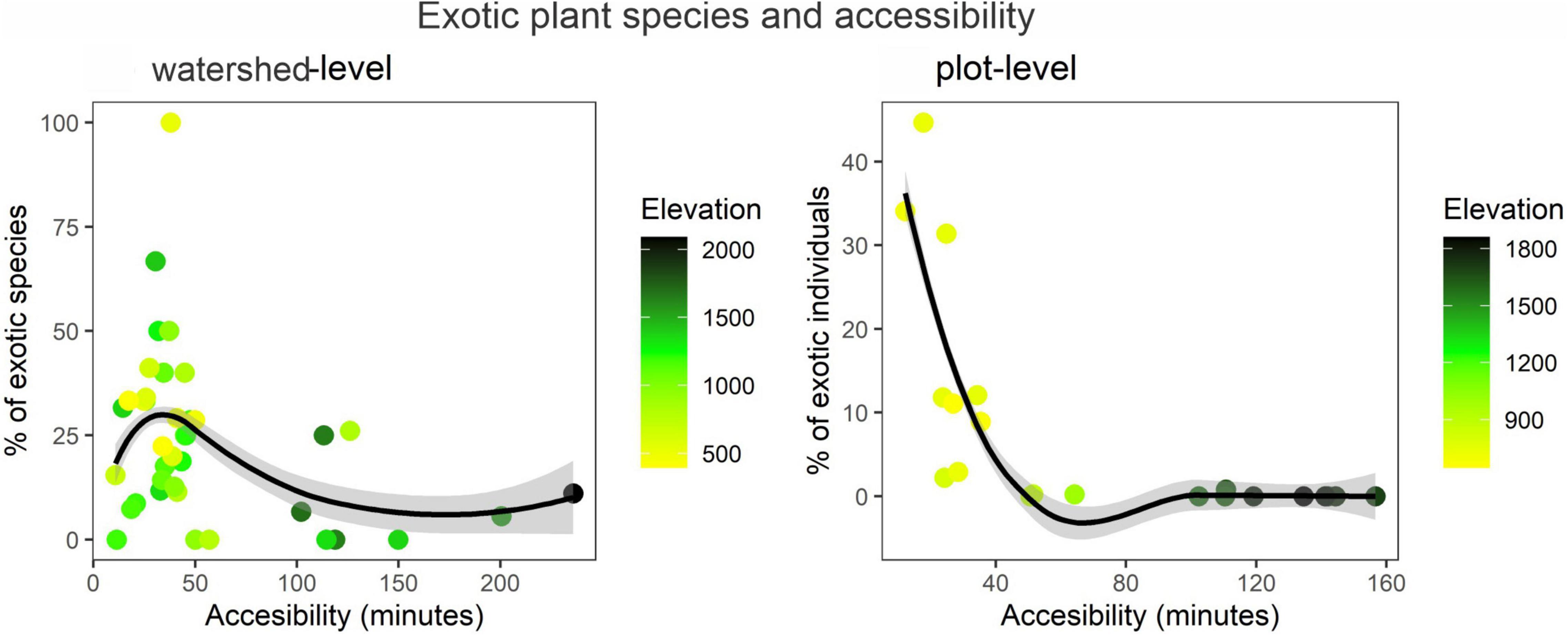
Figure 6. Variation of the percentage of exotic plants at watershed (left) and plot levels (right). Each point represents a quadrant of 2.5 km × 2.5 km.
Discussion
The present study shows the spatial and temporal variability of the spontaneous forest transition in the Lules watershed. Tree establishment is especially sensitive to topography (Supplementary Table 4). Steep slopes and intermediate elevations favor the recovery and persistence of forests. It is likely that steep slopes favor forest persistence by preventing agricultural use while climatic suitability favors forest establishments at middle elevation (Crk et al., 2009; da Silva et al., 2016). However urban and peri-urban settlements also influence the forest transition process through two alternative trajectories: (1) traditional forest transition pathway mediated by outmigration, which is more frequent in remote areas where residents need to migrate to access social amenities (e.g., education, health) abandoning farming activities (Rudel et al., 2020); (2) forest transition by exurban development, a process that occurs in areas connected to prosperous cities, where residents combine the accessibility to public services with the amenities of natural environments (Taylor and Hurley, 2016; Jimenez et al., 2022). Our results reveal that these two trajectories are occurring in the Lules watershed, reflected in the two peaks of forest expansion; near SMT (up to 15 km) overlapping with the second home localities, and in remote areas (between 20 and 30 km from SMT) corresponding to the traditional forest transition. This implies differences in forest recovery timing and forest composition (which in turn affects habitat quality and ecosystem services). At the scale of the whole watershed, more remote areas experience a gradual forest expansion process (Figure 4) while the surroundings of second home localities had a marked increase in forest area before 2000 followed by reforestation pulses as the use of non-forested land dis-intensified, a process that slows down over time as the system saturates (Figures 4, 5). This temporal difference in the forest transition process could also be associated with the trajectories mentioned above. In the less accessible areas, forest transition by rural out-migration and climatic factors are combined. Forests expanded over areas with decreasing population (i.e., accompanied by a reducing agricultural labor and increasing land availability; Jimenez et al., 2021) and also over high elevation grasslands where tree establishment is driven by suitable climate conditions (e.g., A. acuminata, Aráoz and Grau, 2010). On other hand, the land cover change observed around the second home localities may support the theory of forest transition mediated by counterurbanization (Jimenez et al., 2022). The repopulation and low-density urbanization of rural landscapes connected to prosperous cities lead to an economic restructuring and agricultural/livestock uses are replaced by services sector (e.g., tourism, Woods, 2005; Abrams et al., 2012). In this context the land is not abandoned but the new uses are reoriented to the provision of natural amenities to meet the demands of new inhabitants and visitors (Woods, 2005; Abrams et al., 2012). In these areas reforestation may occur due to the creation of protected areas, landscape design and agricultural dis-intensification because real estate and tourism development outcompete farming. In addition, the second home areas analyzed in this study are located in low montane forests where less restrictive climatic conditions facilitate the colonization by surrounding vegetation (Entrocassi et al., 2020).
We also found that in accessible areas (near urbanizations and second home areas) the proportion of exotic species was higher (Figure 5). This may be due to the influence of the city and second home developments, that regulates connections and flows between people and surrounding natural habitats that lead to the introduction and dispersal of exotic species (Montti et al., 2017). Many of these exotic species are usually herbs and trees present in gardens, urban forests, and peri-urban orchards that act as propagule sources for exotic plants that disperse near urbanizations. In some cases, these species integrate within the native plant communities (naturalization) and in others they become invaders and they may even dominate new forests. In the Lules watershed the focal points of invasion by exotic species, such as L. lucidum and G. triacanthos were usually observed close to urban areas (Fernandez et al., 2017; Montti et al., 2017, Fernandez et al., 2020).
Introduction of exotic plants may lead to biodiversity increase but also regional or global biotic homogenization and formation of novel forests with structural and functional characteristics that modify the original ecosystem (Hobbs et al., 2009). At larger scales (e.g., region, landscape), exotic plants add to the local flora and increase species richness (Ellis et al., 2012). For example, this happens in heterogeneous landscapes that include both patches dominated by native and exotic species. However, in some cases local biodiversity (e.g., within a stand) may decrease due to the monopolization of resources by the invader species. Some exotic species may form monodominant forest, as G. triacanthos and L. lucidum in the middle watershed (Malizia et al., 2017; Ceballos et al., 2020; Jimenez et al., 2021) inhibiting the growth of native species and reducing local biodiversity (Ayup et al., 2014; Malizia et al., 2017). This kind of forest, dominated by exotic species, often establishes in abandoned croplands or pastureland with proximate seed sources, a situation that is likely to happen around second home areas. However, not all exotic species registered in the watershed behave as invasive, contributing to increased richness even at small scales (Thomas and Palmer, 2015). The plasticity of some invader species permits them to colonize different environments around the world, implying a homogenization of regional or global biota. L. lucidum is an example of this kind of species (Fernandez et al., 2020). In the long term, this species may monopolize resources preventing the recolonization of species with more restrained distributions.
The process that we record and describe here (Forest transition mediated by counterurbanization) implies local land cover changes that are replicated in rural areas with natural and social amenities (Taylor and Hurley, 2016). Several studies have registered an increase of exurban and suburban development around the globe, mainly in Europe and North America, and predict that this land use is going to increase in the future (Schneider and Woodcock, 2008; Golding and Winkler, 2020). This process may represent an opportunity for forest recovery but is also associated with trade-offs between ecosystem services and biodiversity and emerging social conflicts with local people (e.g., restriction of traditional land uses as livestock grazing or activities as hunting that do not coincide with the idyllic rural lifestyle that urbanites expect) an adequate understanding and management (Abrams et al., 2012; Taylor and Hurley, 2016; Jimenez et al., 2022).
Conclusion
Our study depicts how accessibility by urbanites, interacting with topography, modulates the environmental effects of urban areas on natural montane ecosystems through time. The presence of urban and peri-urban centers seems to favor the process of land use dis-intensification in montane areas favoring forest recovery. This recovery is time and distance dependent. In the first stages forest recovers in areas adjacent to urban centers, and it gradually moves to less accessible areas. The presence of satellite urbanized areas where second home are owned by urban people function as a leap-frog expansion of urban lifestyle accelerating forest recovery in their surroundings. Urban areas also constitute sources of exotic species that change local species composition and thus may result in FT dominated in part by a new type of forests. Thus, species diversity increases in peri-urban areas and in some instances new species dominate local patches generating novel and unexpected environmental effects. As low-density peri-urban areas are expanding and increasing around the world, the spontaneous forest transition, with a significant participation of non-native species occurring around these types of urbanizations will play a key role in the creation and functioning of novel ecosystems of the Anthropocene.
Data Availability Statement
The original contributions presented in the study are included in the article/Supplementary Material, further inquiries can be directed to the corresponding author.
Author Contributions
HG, EA, and YJ contributed to the conception and design of the study. CB, JC, and AM provided data from permanent plots. YJ and SC performed the statistical analysis. YJ wrote the first draft of the manuscript. SC wrote sections of the manuscript. All authors contributed to manuscript revision, read, and approved the submitted version.
Funding
This research was funded by grants PUE N°023.GRAU “El antropoceno en el noroeste Argentino: uso del territorio, nuevos ecosistemas, servicios ambientales, forzantes globales y gobernanza regional” and PICT 2018 03881 “Transición de la megaherbivoría en el gradiente altitudinal del noroeste Argentino: patrones, mecanismos causales, consecuencias ecológicas y escenarios para la optimización del territorio” to HG.
Conflict of Interest
The authors declare that the research was conducted in the absence of any commercial or financial relationships that could be construed as a potential conflict of interest.
Publisher’s Note
All claims expressed in this article are solely those of the authors and do not necessarily represent those of their affiliated organizations, or those of the publisher, the editors and the reviewers. Any product that may be evaluated in this article, or claim that may be made by its manufacturer, is not guaranteed or endorsed by the publisher.
Supplementary Material
The Supplementary Material for this article can be found online at: https://www.frontiersin.org/articles/10.3389/ffgc.2022.761957/full#supplementary-material
Footnotes
- ^ https://www.usgs.gov/landsat-missions
- ^ https://ier.conicet.gov.ar/red-subtropical-de-parcelas-permanentes-redspp/
References
Abrams, J. B., Gosnell, H., Gill, N. J., and Klepeis, P. J. (2012). Re-creating the rural, reconstructing nature: an international literature review of the environmental implications of amenity migration. Conserv. soc. 10, 270–284. doi: 10.4103/0972-4923.101837
Aráoz, E., and Grau, H. R. (2010). Fire-mediated forest encroachment in response to climatic and land-use change in subtropical andean treelines. Ecosystems 13, 992–1005. doi: 10.1007/s10021-010-9369-7
Ashraf, J. R., Pandey, R., and De Jong, W. (2017). Assessment of bio-physical, social and economic drivers for forest transition in Asia-Pacific region. For. Policy Econ. 76, 35–44. doi: 10.1016/j.forpol.2016.07.008
Ayup, M. M., Montti, L., Aragón, R., and Grau, H. R. (2014). Invasion of Ligustrum lucidum (Oleaceae) in the southern yungas: Changes in habitat properties and decline in bird diversity. Acta oecologica 54, 72–81. doi: 10.1016/j.actao.2013.03.006
Carilla, J., and Grau, H. R. (2011). Successional patterns of subtropical montane forest from northwestern Argentina. Bosque 32, 97–111. doi: 10.4067/S0717-92002011000200001
Carilla, J., Grau, H. R., and Malizia, A. (2006). “Patterns of forest recovery in grazing fields in the subtropical mountains of northwest Argentina,” in Land Use Change and Mountain Biodiversity, 1st Edn. (Boca Raton: CRC Press), 263–274. doi: 10.1201/9781420002874.ch19
Ceballos, S. J. (2019). Dinámica de las comunidades de lianas y epífitas en bosques sucesionales de las Yungas australes (Sierra de San Javier, Tucumán, Argentina). Ph.D. Thesis. San Miguel de Tucumán: Universidad Nacional de Tucumán.
Ceballos, S. J., Blundo, C., Malizia, A., Osinaga Acosta, O., and Carilla, J. (2021). Dynamics of tree mortality in subtropical montane forests of Northwestern Argentina. For. Ecol. Manag. 497:119528. doi: 10.1016/j.foreco.2021.119528
Ceballos, S. J., Jimenez, Y., and Fernandez, R. (2020). Estructura de los bosques de Gleditsia triacanthos en función de la edad (valle de la sala, Tucumán, Argentina). Ecol. Austral 30, 251–259. doi: 10.25260/EA.20.30.2.0.1083
Congalton, R. G. (2001). Accuracy assessment and validation of remotely sensed and other spatial information. Int. J. Wildland Fire 10, 321–328. doi: 10.1071/WF01031
Crk, T., Uriarte, M., Corsi, F., and Flynn, D. (2009). Forest recovery in a tropical landscape: what is the relative importance of biophysical, socioeconomic, and landscape variables? Landsc. Ecol. 24, 629–642. doi: 10.1007/s10980-009-9338-8
da Silva, R. F., Batistella, M., and Moran, E. F. (2016). Drivers of land change: Human-environment interactions and the Atlantic forest transition in the Paraíba Valley. Brazil. Land Use Policy 58, 133–144. doi: 10.1016/j.landusepol.2016.07.021
Dehnen Schmutz, K., Touza, J., Perrings, C., and Williamson, M. (2007). The horticultural trade and ornamental plant invasions in Britain. Conserv. Biol. 21, 224–231. doi: 10.1111/j.1523-1739.2006.00538.x
Ellis, E. C., Antill, E. C., and Kreft, H. (2012). All is not loss: plant biodiversity in the Anthropocene. PLoS One 7:e30535. doi: 10.1371/journal.pone.0030535
Entrocassi, G. S., Gavilán, R. G., and Mata, D. S. (2020). Subtropical Mountain Forests of Las Yungas: Vegetation and Bioclimate. New York:NY: Springer, doi: 10.1007/978-3-030-25521-3
Fernandez, R. D., Ceballos, S. J., Aragón, R., Malizia, A., Montti, L., Whitworth-Hulse, J. I., et al. (2020). A global review of Ligustrum lucidum (OLEACEAE) invasion. Bot. Rev. 86, 93–118. doi: 10.1007/s12229-020-09228-w
Fernandez, R. D., Ceballos, S. J., González Achem, A. L., Hidalgo, M. D. V., and Fernández, H. (2016). Quality and conservation of riparian forest in a mountain subtropical basin of Argentina. Int. J. Ecol. 2016, 10. doi: 10.1155/2016/4842165
Fernandez, R. D., Ceballos, S. J., Malizia, A., and Aragón, R. (2017). Gleditsia triacanthos (Fabaceae) in Argentina: a review of its invasion. Aus. J. Bot. 65, 203–213. doi: 10.1071/BT16147
Golding, S. A. (2021). World System satellites: place-making and inequality during the 1990s rural migration rebound. J. Rural Stud. 83, 226–235. doi: 10.1016/j.jrurstud.2020.11.007
Golding, S. A., and Winkler, R. L. (2020). Tracking urbanization and exurbs: migration across the rural–urban continuum, 1990–2016. Popul. Res. policy Rev. 39, 835–859. doi: 10.1007/s11113-020-09611-w
Grau, H. R. (2002). Scale-dependent relationships between treefalls and species richness in a neotropical montane forest. Ecology 83, 2591–2601. doi: 10.1890/0012-9658(2002)083[2591:sdrbta]2.0.co;2
Grau, H. R. (2011). Ecología de una interfase natural-urbana: la sierra de San Javier y el Gran San Miguel de Tucumán. San Miguel de Tucumán: Editorial Edunt.
Grau, H. R., Aide, T. M., Zimmerman, J. K., Thomlinson, J. R., Helmer, E., and Zou, X. (2003). The ecological consequences of socioeconomic and land-use changes in postagriculture Puerto Rico. BioScience 53, 1159–1168. doi: 10.1641/0006-3568(2003)053[1159:tecosa]2.0.co;2
Grau, H. R., Arturi, M. F., Brown, A. D., and Aceñolaza, P. G. (1997). Floristic and structural patterns along a chronosequence of secondary forest succession in Argentinean subtropical montane forests. For. Ecol. Manag. 95, 161–171. doi: 10.1016/S0378-1127(97)00010-8
Grau, H. R., Hernández, M. E., Gutierrez, J., Gasparri, N. I., Casavecchia, M. C., Flores-Ivaldi, E. E., et al. (2008). A peri-urban neotropical forest transition and its consequences for environmental services. Ecol. Soc. 13, 35.
Grau, H. R., Paolini, L., Malizia, A., and Carilla, J. (2010). Distribución, estructura y dinámica de los bosques de la Sierra de San Javier. Ecología de una transición natural urbana: El Gran San Miguel de Tucumán y la Sierra de San Javier. San Miguel de Tucumán, Argentina: Editorial de la Universidad Nacional de Tucumán, 33–48.
Gutierrez Angonese, J. G., and Grau, H. R. (2014). Assessment of swaps and persistence in land cover changes in a subtropical periurban region, NW Argentina. Landscape Urban Plan. 127, 83–93. doi: 10.1016/j.landurbplan.2014.01.021
Hobbs, R. J., Higgs, E., and Harris, J. A. (2009). Novel ecosystems: implications for conservation and restoration. Trends Ecol. Evol. 24, 599–605. doi: 10.1016/j.tree.2009.05.012
Jimenez, Y. G., Aráoz, E., Fernandez, R. D., Nanni, S., Ovejero, R., Paolini, L., et al. (2022). Counterurbanization: a neglected pathway of forest transition. Ambio 51, 823–835. doi: 10.1007/s13280-021-01632-9
Jimenez, Y. G., Aráoz, E., Grau, H. R., and Paolini, L. (2021). Linking forest transition, plant invasion and forest succession theories: socioeconomic drivers and composition of new subtropical andean forests. Landsc. Ecol. 36, 1161–1176. doi: 10.1007/s10980-021-01192-z
Lomáscolo, T., Grau, A., and Brown, A. (2014). Guía Visual de Las Áreas Protegidas De Tucumán. San Miguel de Tucumán: Argentina: Ediciones del Subtrópico,
Malizia, A., Osinaga-Acosta, O., Powell, P. A., and Aragón, R. (2017). Invasion of Ligustrum lucidum (oleaceae) in subtropical secondary forests of NW Argentina: declining growth rates of abundant native tree species. J. Veg. Sci. 28, 1240–1249. doi: 10.1111/jvs.12572
Malizia, L., Pacheco, S., Blundo, C., and Brown, A. D. (2012). Caracterización altitudinal, uso y conservación de las yungas subtropicales de Argentina. Ecosistemas 21, 53–73.
Montti, L., Carrillo, V. P., Gutiérrez-Angonese, J., Gasparri, N. I., Aragón, R., and Grau, H. R. (2017). The role of bioclimatic features, landscape configuration and historical land use in the invasion of an asian tree in subtropical Argentina. Landsc. Ecol. 32, 2167–2185. doi: 10.1007/s10980-017-0563-2
Nanni, A. S., Sloan, S., Aide, T. M., Graesser, J., Edwards, D., and Grau, H. R. (2019). The neotropical reforestation hotspots: a biophysical and socioeconomic typology of contemporary forest expansion. Glob. Environ. Change 54, 148–159. doi: 10.1016/j.gloenvcha.2018.12.001
Navarro, C. J., Izquierdo, A. E., Aráoz, E., Foguet, J., and Grau, H. R. (2020). Rewilding of large herbivore communities in high elevation Puna: geographic segregation and no evidence of positive effects on peatland productivity. Reg. Environ. Change 20, 1–11. doi: 10.1007/s10113-020-01704-8
Pal, M. (2005). Random forest classifier for remote sensing classification. Int. J. Remote Sens. 26, 217–222. doi: 10.1080/01431160412331269698
Parés-Ramos, I. K., Gould, W. A., and Aide, T. M. (2008). Agricultural abandonment, suburban growth, and forest expansion in puerto rico between 1991 and 2000. Ecol. Soc. 13:1.
Pero, E. J., Busnelli, J., and Juliá, J. P. (2015). Cambios en la cobertura vegetal y mapeo de un área protegida del no argentino. Lilloa 52, 70–81.
Redo, D. J., Grau, H. R., Aide, T. M., and Clark, M. L. (2012). Asymmetric forest transition driven by the interaction of socioeconomic development and environmental heterogeneity in Central America. Proc. Natl. Acad. Sci. 109, 8839–8844. doi: 10.1073/pnas.1201664109
Rodriguez-Galiano, V. F., Ghimire, B., Rogan, J., Chica-Olmo, M., and Rigol-Sanchez, J. P. (2012). An assessment of the effectiveness of a random forest classifier for land-cover classification. ISPRS J. photogramm. Remote sens. 67, 93–104. doi: 10.1016/j.isprsjprs.2011.11.002
Rudel, T. K., Coomes, O. T., Moran, E., Achard, F., Angelsen, A., Xu, J., et al. (2005). Forest transitions: towards a global understanding of land use change. Glob. Environ. change 15, 23–31. doi: 10.1016/j.gloenvcha.2004.11.001
Rudel, T. K., Meyfroidt, P., Chazdon, R., Bongers, F., Sloan, S., Grau, H. R., et al. (2020). Whither the forest transition? Climate change, policy responses, and redistributed forests in the twenty-first century. Ambio 49, 74–84. doi: 10.1007/s13280-018-01143-0
Salvati, L., Ranalli, F., Carlucci, M., Ippolito, A., Ferrara, A., and Corona, P. (2017). Forest and the city: a multivariate analysis of peri-urban forest land cover patterns in 283 European metropolitan areas. Ecol. Indic. 73, 369–377. doi: 10.1016/j.ecolind.2016.09.025
Schneider, A., and Woodcock, C. E. (2008). Compact, dispersed, fragmented, extensive? a comparison of urban growth in twenty-five global cities using remotely sensed data, pattern metrics and census information. Urban Stud. 45, 659–692. doi: 10.1177/0042098007087340
Shaw, B. J., van Vliet, J., and Verburg, P. H. (2020). The peri-urbanization of Europe: a systematic review of a multifaceted process. Landsc. Urban Plan. 196:103733. doi: 10.1016/j.landurbplan.2019.103733
Talukdar, S., Singha, P., Mahato, S., Pal, S., Liou, Y. A., and Rahman, A. (2020). Land-use land-cover classification by machine learning classifiers for satellite observations–a review. Remote Sens. 12, 1135. doi: 10.3390/rs12071135
Taylor, L. E., and Hurley, P. T. (2016). Introduction: the broad contours of exurban landscape change. In a comparative political ecology of exurbia. New York:NY: Springer, 1–29. doi: 10.1007/978-3-319-29462-9
Teferi, E., Bewket, W., Uhlenbrook, S., and Wenninger, J. (2013). Understanding recent land use and land cover dynamics in the source region of the upper blue nile, ethiopia: spatially explicit statistical modeling of systematic transitions. Agric. Ecosyst. Environ. 165, 98–117. doi: 10.1016/j.agee.2012.11.007
Thomas, C. D., and Palmer, G. (2015). Non-native plants add to the British flora without negative consequences for native diversity. Proc. Natl. Acad. Sci. 112, 4387–4392. doi: 10.1073/pnas.1423995112
Tobler, W. (1993). Three Presentations On Geographical Analysis And Modeling: Non-Isotropic Geographic Modeling; Speculations On The Geometry Of Geography; and Global Spatial Analysis. Technical Report 93-1. Santa Barbara, CA: National Center for Geographic Information and Analysis.
Viollaz, M. C., and Casares, M. (2017). Desarrollo Sustentable De Los Valles De Raco Y El Siambón. Sustainable Development Of The Valleys Of Raco and El Siambón. V Workshop De La Red Iberoamericana De Observación Territorial, Vi Seminario Internacional De Ordenamiento Territorial. Argentina: Universidad Nacional de Cuyo, Mendoza.
Wilkinson, L., and Friendly, M. (2009). The history of the cluster heat map. Am. Stat. 63, 179–184. doi: 10.1198/tas.2009.0033
Keywords: land use change, exurbanziation, exotic plants, second home, accessibility
Citation: Jimenez YG, Ceballos SJ, Aráoz E, Blundo C, Carilla J, Malizia A and Grau HR (2022) Spatial, Temporal and Ecological Patterns of Peri-Urban Forest Transitions. An Example From Subtropical Argentina. Front. For. Glob. Change 5:761957. doi: 10.3389/ffgc.2022.761957
Received: 20 August 2021; Accepted: 11 April 2022;
Published: 29 April 2022.
Edited by:
Patrick O. Waeber, ETH Zürich, SwitzerlandReviewed by:
Didit Okta Pribadi, Indonesian Institute of Sciences, IndonesiaWendy Y. Chen, The University of Hong Kong, Hong Kong SAR, China
Copyright © 2022 Jimenez, Ceballos, Aráoz, Blundo, Carilla, Malizia and Grau. This is an open-access article distributed under the terms of the Creative Commons Attribution License (CC BY). The use, distribution or reproduction in other forums is permitted, provided the original author(s) and the copyright owner(s) are credited and that the original publication in this journal is cited, in accordance with accepted academic practice. No use, distribution or reproduction is permitted which does not comply with these terms.
*Correspondence: Yohana G. Jimenez, eW9oYW5hamltZW5lejI0MDZAZ21haWwuY29t