- 1Faculty of Resources and Environment, Baotou Teachers’ College, Inner Mongolia University of Science and Technology, Baotou, China
- 2Key Laboratory of Ecohydrology of Inland River Basin, Northwest Institute of Eco-Environment and Resources, Chinese Academy of Sciences, Lanzhou, China
- 3University of Chinese Academy of Sciences, Beijing, China
Hippophae rhamnoides is widely known for its important ecological, economic, and social benefits. It is known as the pioneer plant of soil and water conservation, with homology in food and medicine. With the climate warming in recent years, the numbers of this species and countries with this plant have decreased steadily. H. r. sinensis and H. r. turkestanica have the widest distribution area in China, which account for more than 90% of the total national Hippophae rhamnoides resources. We firstly screened the presence data and downscale the environment variables (climate and soil) by correlation analysis. Secondly, based on the 232 occurrence data of H. r. sinensis and 10 environmental variables, the 73 occurrence data of H. r. turkestanica and 11 environmental variables, we simulated and predicted their suitable habitats in China, both at the current time and in the 2050S (2041–2060), and analyzed the dominant factors effecting its distribution by using MaxEnt. Finally, we studied the habitat variations and centroid migrations of these subspecies under future climate scenarios using the spatial analysis function of ArcGIS. The results indicated that the area of suitable habitat for H. r. sinensis is much larger than that of H. r. trkestanica in China. The suitable habitat of H. r. sinensis is concentrated in the middle and upper reaches of the Yellow River, mainly distributed in Shaanxi, Shanxi, Sichuan, Qinghai, Gansu, Ningxia, Tibet, and Inner Mongolia, and that of H. r. trkestanica is mainly distributed in Xinjiang and Tibet. The former is mainly affected by bio13 (precipitation of the wettest month), bio11 (mean temperature of the coldest quarte) and bio3 (Isothermality), and the latter is mainly affected by bio13 (precipitation of the wettest month), bio2 (mean diurnal range) and bio15 (precipitation seasonality), and the former is also more stable in the face of future climate change. They are more susceptible to climate than soil in their survival. Although, the two subspecies tend to expand and migrate toward lower latitude under future climate scenarios, there are some differences. H. r. sinensis will migrate westward, while H. r. trkestanica will migrate eastward as a whole. They have a high stability of suitable habitat and are not at risk of extinction in the future. The study’s findings help to clarify the resource reserve of Hippophae rhamnoides L. in China, which will help to guide the protection of wild resources and to popularize artificial planting in suitable areas, and provides scientific basis for the protection of ecological environment.
1. Introduction
Global climate change has become the most important environmental issue we have ever faced, and which is considered one of the major threats to global biodiversity in the 21st Century (Dawson, 2011; Zhao et al., 2021). Climate change affects significantly the growth, reproduction and habitat variation of species, plant phenology, and even the ecosystem stability, both directly and indirectly (Pielke et al., 1998). Previous researches have demonstrated that the habitat area of many species will be significantly reduced, the suitable habitat will migrate to higher latitudes and higher altitudes with climate warming, and some rare plants will face the risk of extinction (Lenoir et al., 2008; Bellard et al., 2012). Therefore, species geographical distribution, migration of suitable habitats and biodiversity conservation under climate change have become hot topics in botany, geography and ecology research in recent years (Guo et al., 2016; Yi et al., 2016; Guan et al., 2018; Ab Lah et al., 2021). In particular, those species that are both economically and ecologically valuable have attracted great interest from scholars. Numerous studies have shown that they exhibit very different adaptive capacities and with heterogeneous trends of their niches in variations under future climate scenarios (Hu et al., 2015; Guan et al., 2018; Hamid et al., 2019).
Hippophae rhamnoides Linn. (Elaeagnaceae Juss.), also known as sea buckthorn, is a flowering shrub or small tree. It first appeared form the Eastern Himalayas to the Hengduan Mountains, and which has a tendency to grow in the arid, semi-arid and high mountainous ecosystems of Eurasia, including China, Russia, Mongolia, India, Finland, France, Nepal, Iran, Pakistan, Afghanistan, Pakistan, Kazakhstan, Bhutan, Britain, Germany, Norway, and Sweden (GBIF, 2020; Ui Haq et al., 2021). This genus contains seven species and eleven subspecies around the world (Swenson and Bartish, 2002). However, as the climate has warmed in recent years, the numbers of this species and countries with this plant have decreased steadily (Pundir et al., 2021). Hippophae rhamnoides has attracted much attention from scholars in different fields all around the world because of its high ecological, economic, and medicinal value (Suryakumar and Gupta, 2011; Zielinska and Nowak, 2017; Pundir et al., 2021). Studies on Hippophae rhamnoides have mainly focused on its biochemical characteristics, genetic structure, pharmacological actions, germplasm resources, and phylogeography (Zielinska and Nowak, 2017; Pundir et al., 2021; Ui Haq et al., 2021). However, little attention has been paid to its geographical distribution and its response to climate change. H. rhamnoides. sinensis and H. rhamnoides. turkestanica have the widest distribution area in China, especially H. r. sinensis, they account for more than 90% of the total national Hippophae rhamnoides resources. Understanding the suitable habitat changes and migration of these two subspecies under climate change not only provides theoretical support for the protection of wild species and artificial planting, but also provides a scientific basis for protecting the ecological environment in China.
Species distribution models (SDMs), also known as ecological niche models (ENM), which mainly use environmental data and species distribution data (presence and absence) to estimate ecological niche requirements of species that reflecting in probabilistic form the degree of species habitat preference, thus reflecting the distribution of suitable habitats for species in the selected spatial and temporal range (Guisan and Thuiller, 2007; Elith et al., 2011). SDMs include GAM, GLM, DOMAIN, BIOMAPPER, BIOClIM, CLIMEX, MAXENT, and so on (Carpenter et al., 1993; Hirzel and Guisan, 2002). Although a variety of models are available for predicting geographic distribution, each model has its own advantages and disadvantages. For example, DOMAIN, GLM, GAM, which cannot handle qualitative environmental variables, and their precision is strongly influenced by the sample size (Carpenter et al., 1993; Guisan et al., 2002). Maximum entropy (MaxEnt) model provides higher predictive accuracy with incomplete data and small sample sizes, and performance well (Elith et al., 2006; Ghareghan et al., 2020). The input species data can be presence-only data and the MaxEnt simulation results can directly generate spatial habitat suitability maps (Phillips and Dudik, 2008; Elith et al., 2011). Therefore, MaxEnt is not only commonly used for the determination of the geographical distribution of plant species (Guan et al., 2018; Li et al., 2020), but also widely used in other fields, such as identifying suitable habitat for animals (Su et al., 2021), biological invasion (Gallagher et al., 2010), urban geography and archeology (Muttaqin et al., 2019), space-generating capacity of natural hazards (Javidan et al., 2021), and energy suitability distribution (Tekin et al., 2021).
In order to understand the effects of climate change on the geographic distribution of H. r. sinensis and H. r. turkestanica, we used the distribution data and environmental variables to produce the current habitat suitability map and predict future potential habitat distribution for these two subspecies under current climate and different future climate change scenarios by using MaxEnt. Meanwhile, we analyzed their dominant environmental factors affect the spatial distribution, variations, and migration of suitable habitats under future climate change. Our results will provide theoretical support for the artificial planting, protection and sustainable utilization of Hippophae rhamnoides.
2. Materials and methods
2.1. Data sources
2.1.1. Occurrence data
The occurrence data of H. r. sinensis and H. r. turkestanica in China were obtained from the Global Biodiversity Information Facility (GBIF)1, the National Specimen Information Infrastructure (NSII),2 the Chinese Virtual Herbarium (CVH)3, and the China National Knowledge Infrastructure (CNKI).4 For occurrence data, we first removed the invalid and wrong data, then performed spatial thinning in which we created a grid of 5 × 5 km cells and randomly selected a single point from each cell to remove the sampling bias and spatial autocorrelation by using ENMTools. Finally, 232 occurrence data of H. r. sinensis and 73 occurrence data of H. r. turkestanica were obtained to build the model (Figure 1).
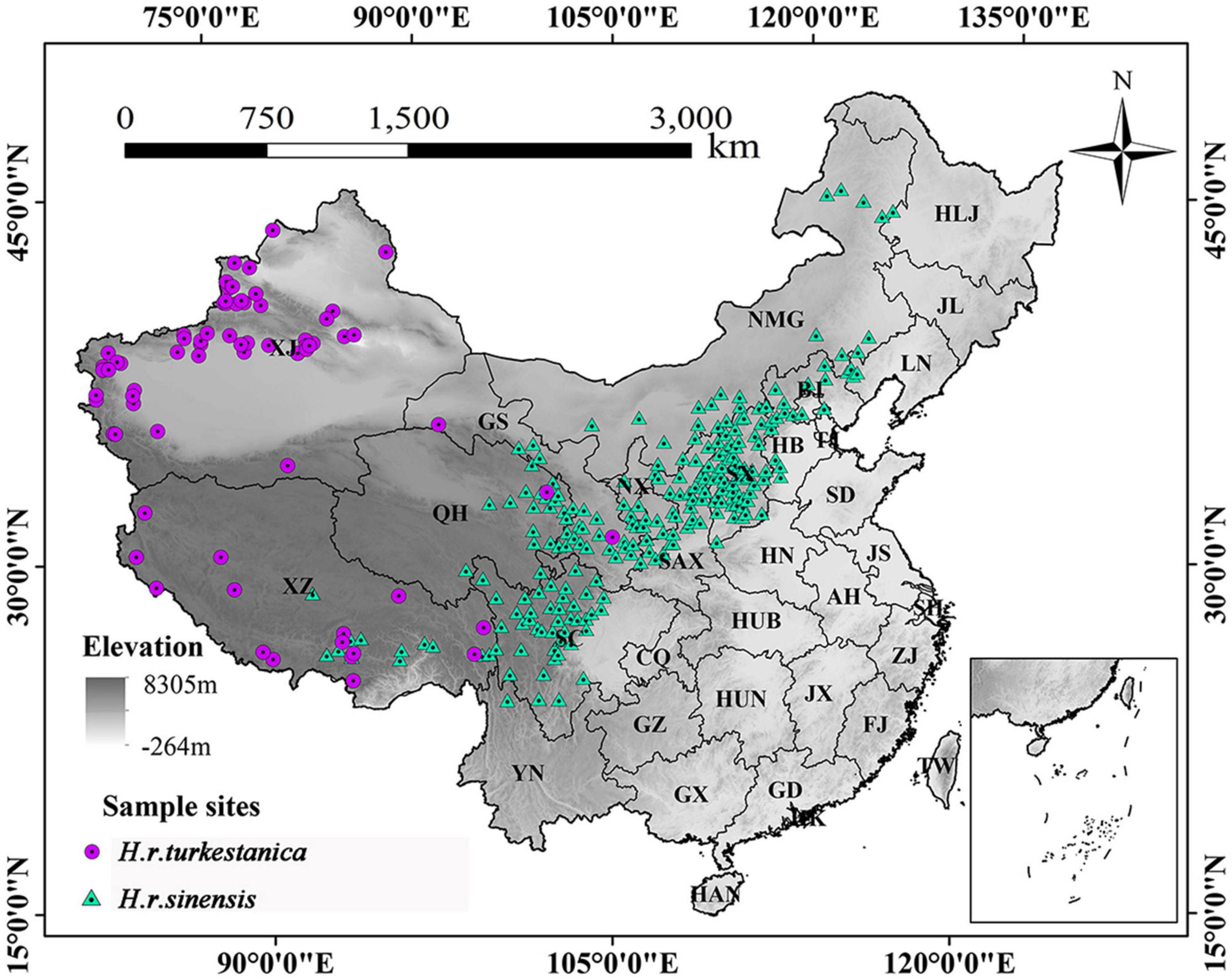
Figure 1. Sample sites of H. r. sinensis and H. r. turkestanica populations in China. Provinces names are abbreviated, XJ, Xinjiang; XZ, Xizang (Tibet); QH, Qinghai; GS, Gansu; NX, Ningxia; NMG, Inner Mongolia; SC, Sichuan; CQ, Chongqing; SAX, Shaanxi; SX, Shanxi; HN, Henan; HUB, Hubei; HUN, Hunan; SD, Shandong; BJ, Beijing; TJ, Tianjin; LN, Liaoning; HB, Hebei; JS, Jiangsu; AH, Anhui; ZJ, Zhejiang; GX, Guangxi; GD, Guangdong; YN, Yunnan; JX, Jiangxi; FJ, Fujian; GZ, Guizhou; SH, Shanghai; HLJ, Heilongjiang; JL, Jilin; HAN, Hainan; MAC, Maco; HK, Hongkong; and TW, Taiwan.
2.1.2. Environmental variables
Bioclimatic variables are of biological significance in determining the environmental niche of species (Yi et al., 2016). A total of 36 environmental variables were selected from two types (19 bioclimatic variables and 17 soil variables) in this study. These bioclimatic variables were downloaded from WorldClim version2.1 (Fick and Hijmans, 2017),5 the resolution is 2.5 arc-min (∼ 5 km spatial resolution at the equator). The current bioclimatic variables were derived from the monthly temperature and rainfall values of meteorological stations around the world from 1970 to 2000, which is a popular dataset used in species distribution modeling and related ecological modeling techniques (Zhao et al., 2021). The future bioclimatic variables of the 2050s (2041–2060) were simulated by Beijing Climate Center Climate System Model version 2 (BCC-CSM2-MR) for representative concentration pathway (SSP1-2.6, SSP2-4.5, SSP3-7.0, and SSP5-8.5) (Fischer et al., 2005; Zhao et al., 2021), this model is reliable in simulating precipitation and temperature in China (Wu et al., 2019). Soil data were obtained from the Harmonized World Soil Database (HWSD), which is 1 km spatial resolution. The above data were processed by ArcGIS with uniform geographic coordinate system and spatial resolution (5 km × 5 km).
Environmental variables are characterized by complexity, comprehensiveness, and correlation. Thus, we screened the environmental variables before the simulation to reduce the collinearity between variables, and improve model accuracy. First, the jackknife test was used to remove environmental variables with contribution rate 0 (Worthington et al., 2016). Second, the correlation analysis was performed in ENMTools, we removed one variable with high correlation (|r| ≥ 0.80), which contributed less variable in the jackknife test (Wei et al., 2018). Ultimately, 10 variables of H. r. sinensis and 11 variables of H. r. turkestanica were selected (Table 1).
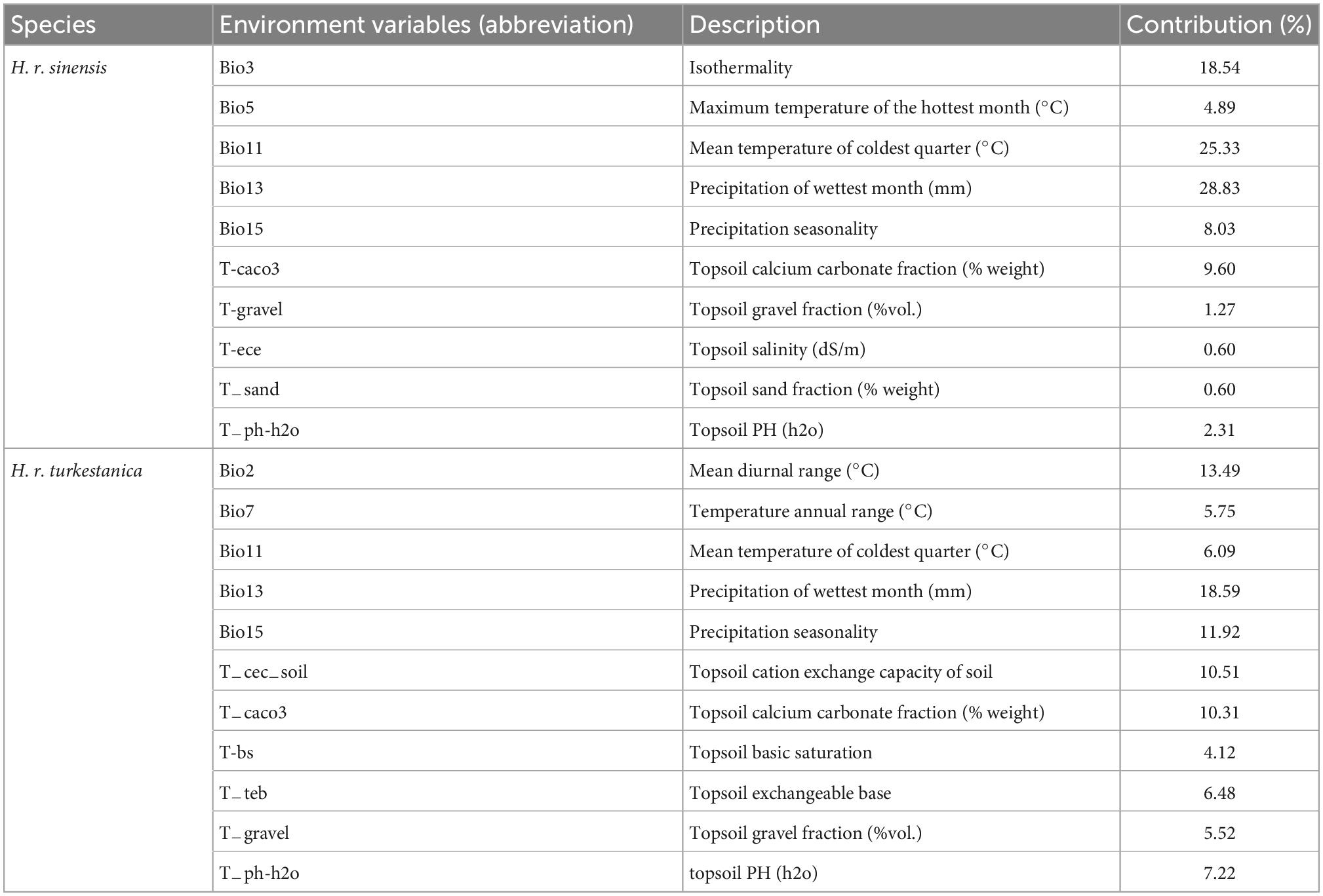
Table 1. Environment variables used for modeling the habitat suitability distribution of H. r. sinensis and H. r. turkestanica and variables’ contribution.
2.2. Methods
2.2.1. Model operation and evaluation index of model accuracy
The 232 occurrence data of H. r. sinensis and 10 environmental variables were imported into MaxEnt 3.4.1 for modeling operation; for the 73 occurrence data of H. r. turkestanica and 11 environmental variables data, we performed the same operation. In order to get the probability of species appearing close to a normal distribution and verify the accuracy of the simulation, we randomly selected 75% of occurrence data as the training set to establish models, and the remaining 25% was used as the testing set (Guo et al., 2016). The contribution rate of each variable to the species distribution was calculated by using the jackknife test. Furthermore, a threshold rule of 10 percentile training presence was applied. We used the area under the receiver operating characteristic (ROC) curve (AUC) as the evaluation indicator of the accuracy of the MaxEnt model because the AUC is not affected by the choice of threshold (Peterson et al., 2008). The ROC curve is a graphical method that uses multiple thresholds to show the relationship between false positive ratio (one minus the-specificity) and sensitivity (Zhang et al., 2020; Fang et al., 2021). The AUC values range between 0 and 1. An AUC value of <0.5 indicates that the model fails to describe reality, 0.5 indicates pure guessing, 0.5–0.6 indicates failure, 0.6–0.7 indicates poor performance, 0.7–0.8 indicates fair performance, 0.8–0.9 indicates good performance, and 0.9–1.0 indicates excellent performance (Swets, 1988).
2.2.2. Suitable habitat grade classification and spatial variation
The range of each raster value was 0–1, which represents the fitness probability of the species in the study area. The habitat suitability predictions were classified into three grades based on the Jenks algorithm. There grades include unsuitable habitat, low suitable habitat, and suitable habitat.
With climate change, species’ habitats may change. To better study the habitat variations of H. r. sinensis and H. r. turkestanica under future climate scenarios, we converted their probability distribution maps into binary maps for unsuitable habitat and suitable habitat. The future and current binary maps were calculated in the grid calculator in ArcGIS, then we generated the spatial change map of the two subspecies under different climate scenarios in the future. Four types of spatial variations were generated (Table 2).
The centroid is an important index used to describe the spatial distribution of species. Its changes can reveal the spatial aggregation and migration of species in a certain period of time. The determination of the suitable habitats’ centroid to be done in ArcGIS. First, maps should be converted into vectors and the points representing the unsuitable habitat should be filtered out. Subsequently, the raster centroid was simulated and converted into a point. Finally, the coordinates of the suitable habitats’ centroid were calculated. In this way, we were able to use the centroid change under different climate scenarios to indicate the spatial pattern change of Hippophae rhamnoides L.
3. Results
3.1. Model accuracy evaluation and variables’ contribution
After the environmental variables were selected and the model simulation, the mean AUC values of H. r. sinensis and H. r. turkestanica were 0.927 (Figure 2A), and 0.969 (Figure 2B), and the AUC standard deviation were 0.004 and 0.009, respectively.
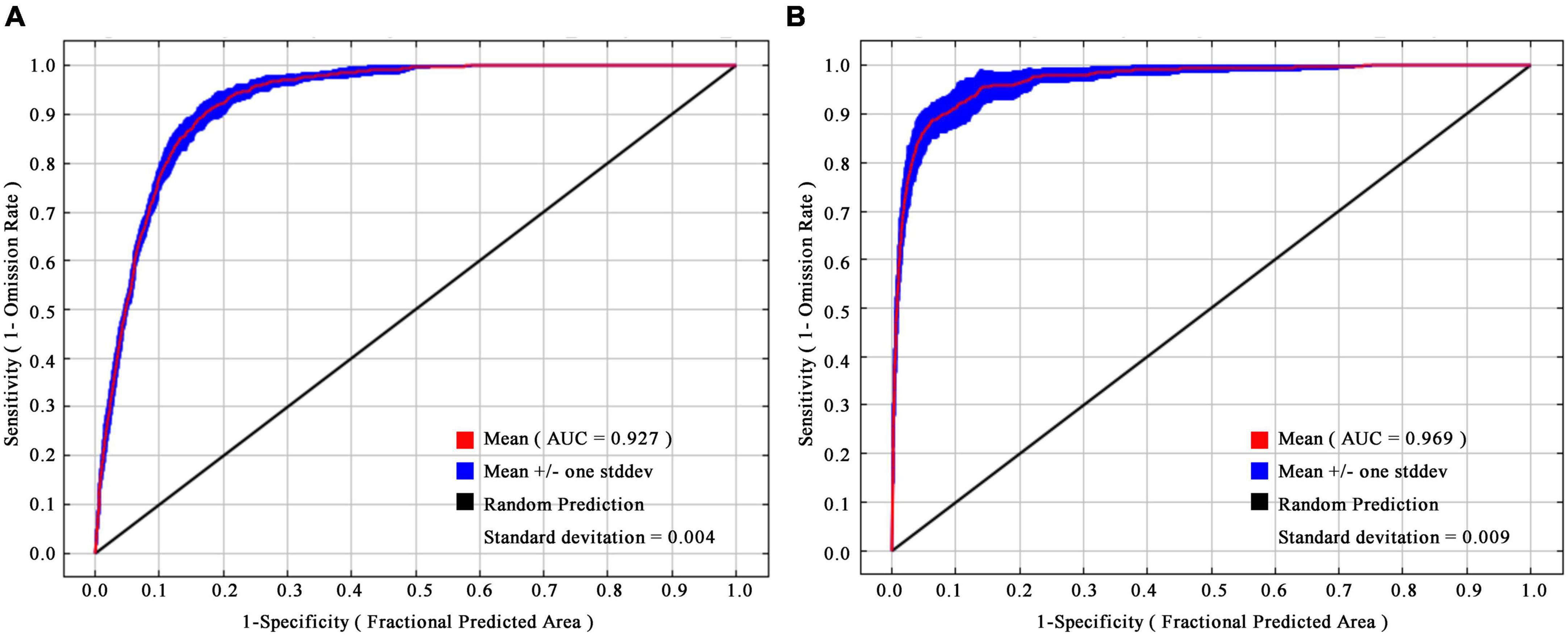
Figure 2. Receiver operating characteristic (ROC) curves of (A) H.r. sinensis and (B) H. r. turkestanica.
The contribution rate of each environmental variable is shown in Table 1. And they are average value over replicate runs. For H. r. sinensis, the top five environmental variables contributing to species distribution were precipitation of the wettest month (bio13, 28.83% contribution rate), mean temperature of the coldest quarter (bio11, 25.33%), isothermality (bio3, 18.54%), topsoil calcium carbonate fraction (t-caco3, 9.60%) and precipitation seasonality (bio15, 8.03%), their cumulative contribution was 90.33%. For H. r. turkestanica, the top six environmental variables contributing to species distribution were precipitation of the wettest month (bio13, 18.59% contribution rate), mean diurnal range (bio2, 13.49%), precipitation seasonality (bio15, 11.92%), topsoil cation exchange capacity of soil (t-cec-soil, 10.51%), topsoil calcium carbonate fraction (t-caco3, 10.31%), and topsoil PH (h2o) (t-ph-h2o, 7.22%), their cumulative contribution was 72.04%.
According to the response curves of the dominant environmental variables, for H. r. sinensis, we found that the highest habitat suitability was obtained when bio13 is 87–135 mm, bio 11 is −9.35 to −0.37°C; bio 3 is 28.3–31.9 and 41.5–45.3 (Figures 3A–C). When a location has a value above or below this value, its suitability decreases rapidly. And the relationship between habitat suitability and bio13 showed a positive skewness distribution, with the estimated peak value of 106 mm. For H. r. turkestanica, we found that the three key environmental variables affecting its distribution, which are bio13, bio2, and bio15, with values of 0–57 mm, 12.6–14.9°C, and 12.1–79.5, respectively (Figures 3D–F). Its habitat suitability is positively skewed with bio13 when values are higher or lower than the peak, which is 3.5 mm. We found that it has a narrow niche overall in bio13, its adaptability becomes poor beyond this range, and has a large adaptation range in bio15, and the probability of species growth decreases with increasing the value of bio15.
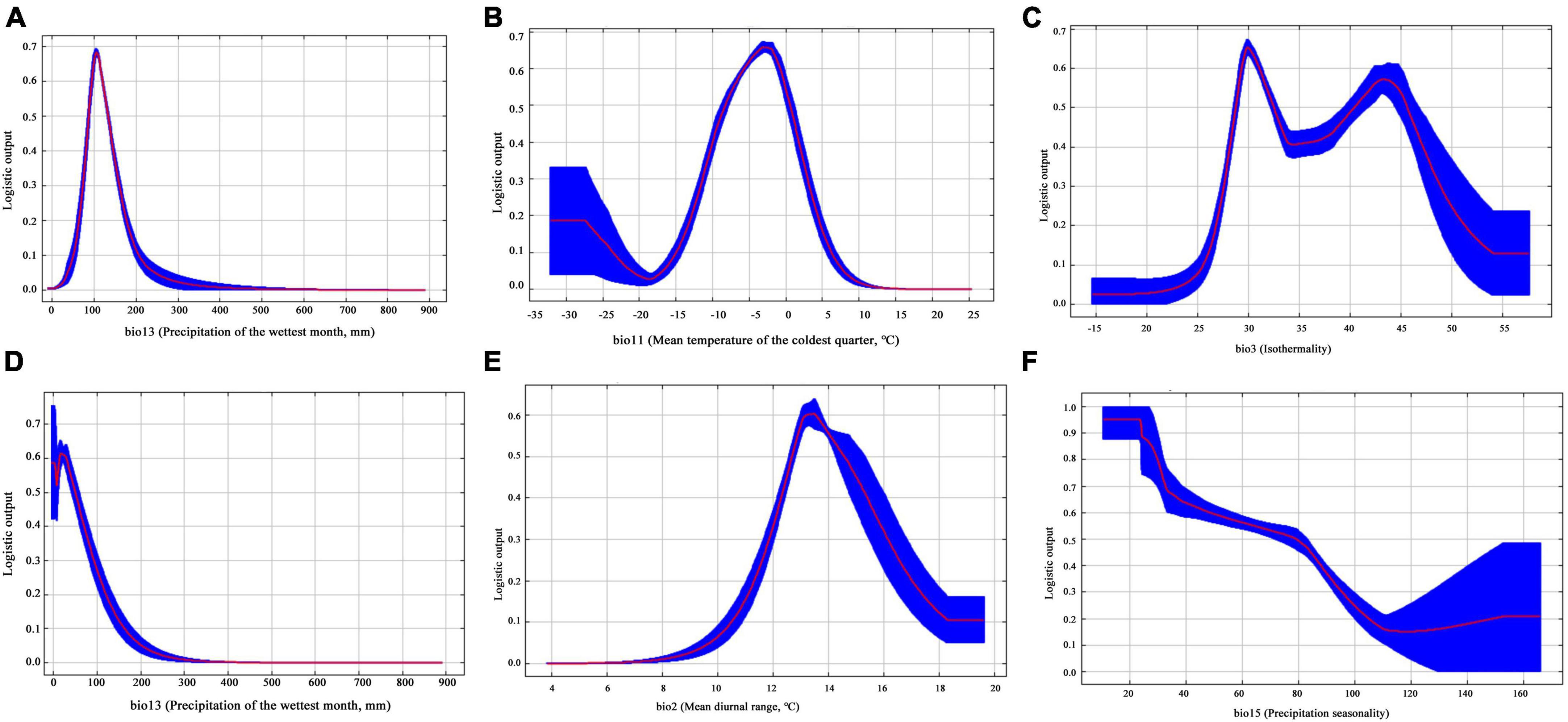
Figure 3. Response curves of probability of presence of (A–C) H. r. sinensis and (D–F) H. r. turkestanica.
3.2. Habitat suitability of H. r. sinensis and H. r. turkestanica under the current and future climatic scenarios
The distribution maps of the suitable habitat of H. r. sinensis and H. r. turkestanica under the current and future climatic scenarios are shown in Figures 4, 5, respectively. The results showed that the suitable area of H. r. sinensis is 883.19 × 103 km2 under current climatic scenario, accounts for 9.20% of China (Table 3), which mainly located in the Loess Plateau and the marginal area from southeast to northeast of the Qinghai-Tibet Plateau. A belt is formed from southwest to northeast, including most areas of Shanxi, central and northern Shaanxi, southwestern Sichuan, Ningxia, southern and Eastern Gansu, Northeastern Qinghai, central Inner Mongolia, and SouthEastern Tibet (Figure 4A). The low-suitability area is approximately 1444.01 × 103 km2, accounting for 15.04% of China. The suitable habitat area of H. r. turkestanica is 227.15 × 103 km2, accounting for 2.37% of China (Table 3), which mainly located around the Tarim Basin and Jungar Basin in Xinjing, western Ali, Shigatse and Qamdo in Tibet, Haixi Mongolian and Tibetan Autonomous Prefecture in Qinghai; Ganzi and Aba prefectures in Sichuan, and parts of Gansu and Ningxia (Figure 5A). The low-suitability area is approximately 1172.39 × 103 km2, accounting for 12.21% of the study area. The low suitable habitat is distributed at the periphery of the suitable habitat.
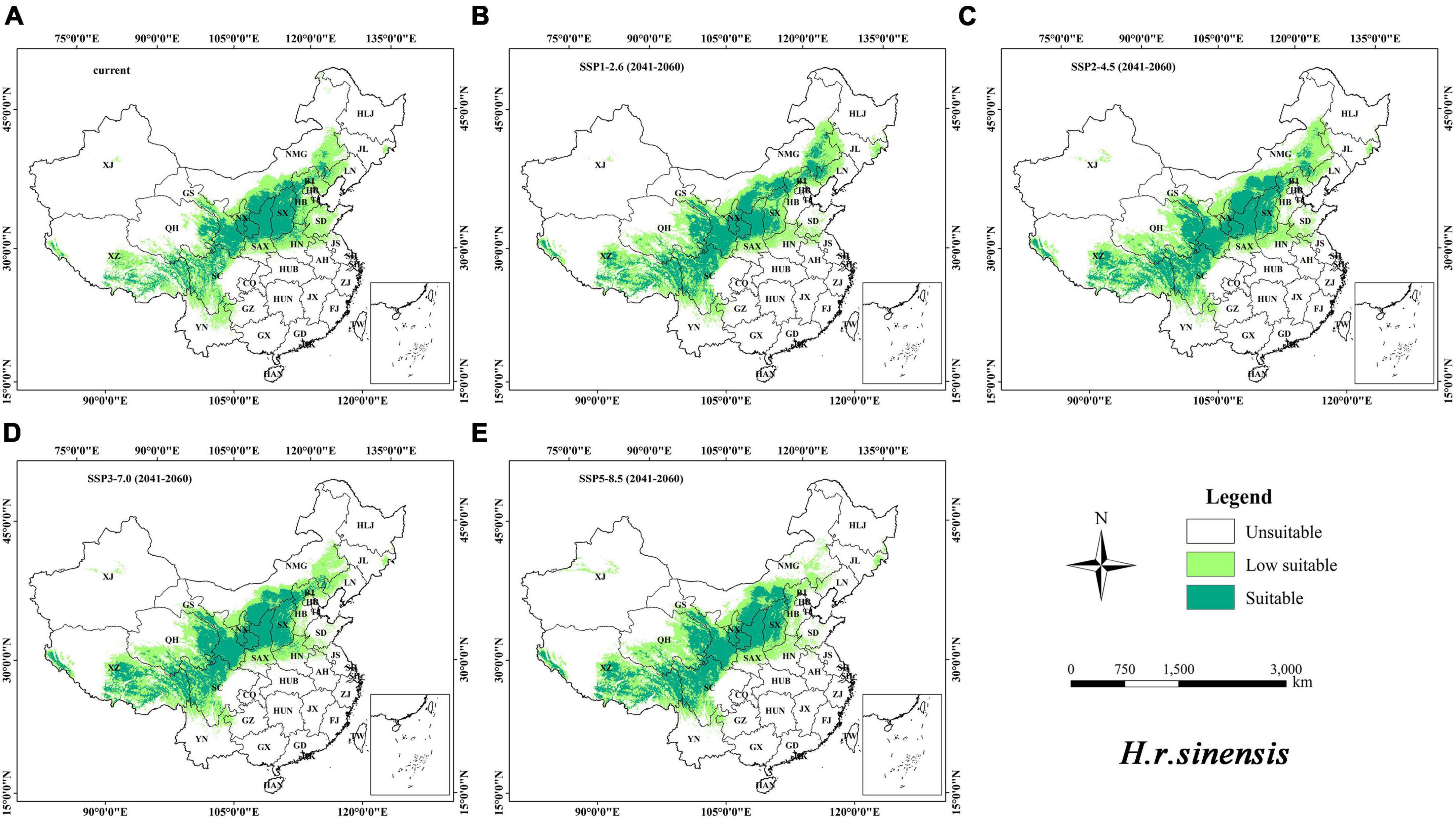
Figure 4. Distribution of the suitable habitat of H. r. sinensis in China under different climate change scenarios. (A) current; (B–E) SSP1-2.6, SSP2-4.5, SSP3-7.0, and SSP5-8.5, in 2050S, respectively.
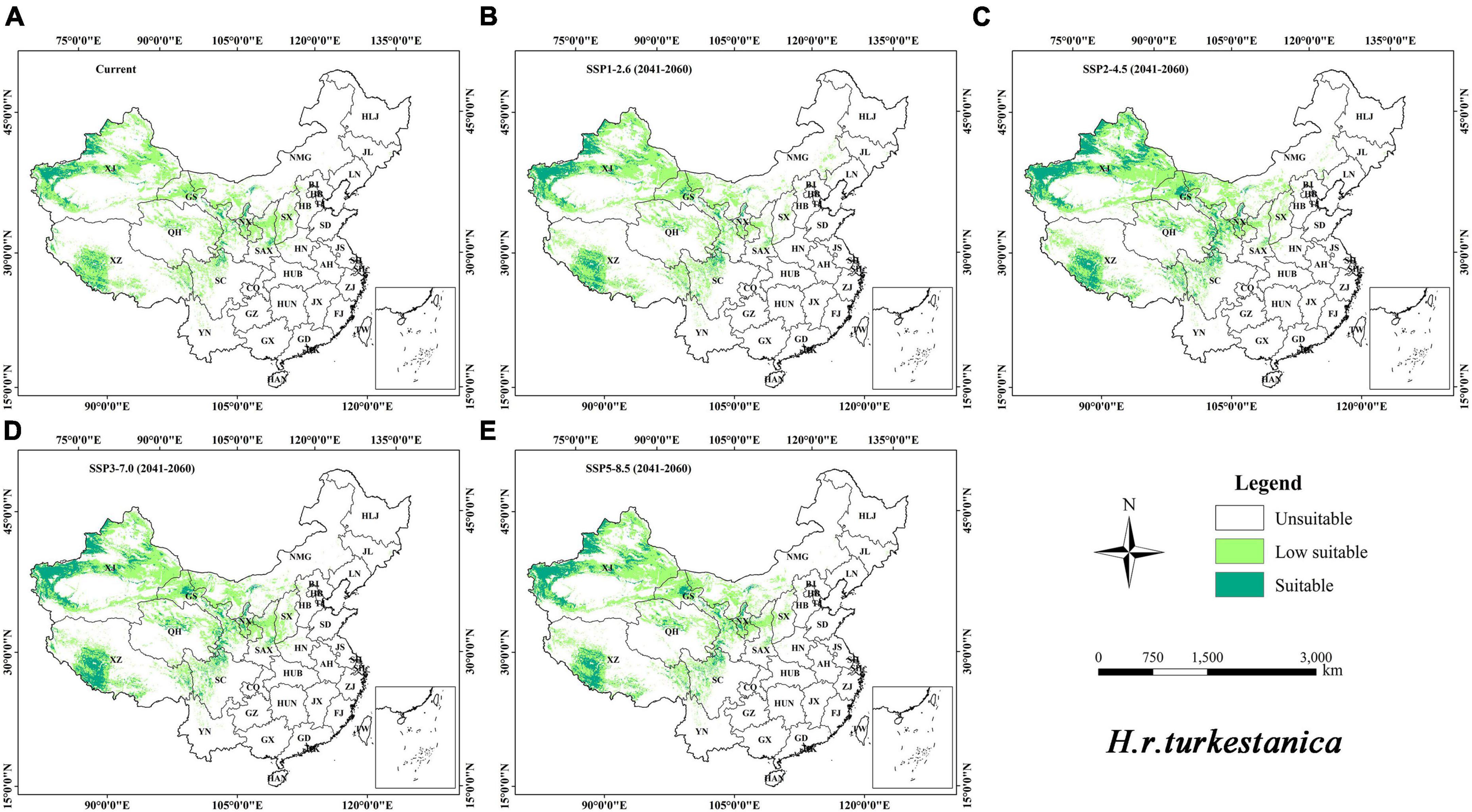
Figure 5. Distribution of the suitable habitat of H. r. turkestanica in China under different climate change scenarios. (A) current; (B–E) SSP1-2.6, SSP2-4.5, SSP3-7.0, and SSP5-8.5, in 2050S, respectively.
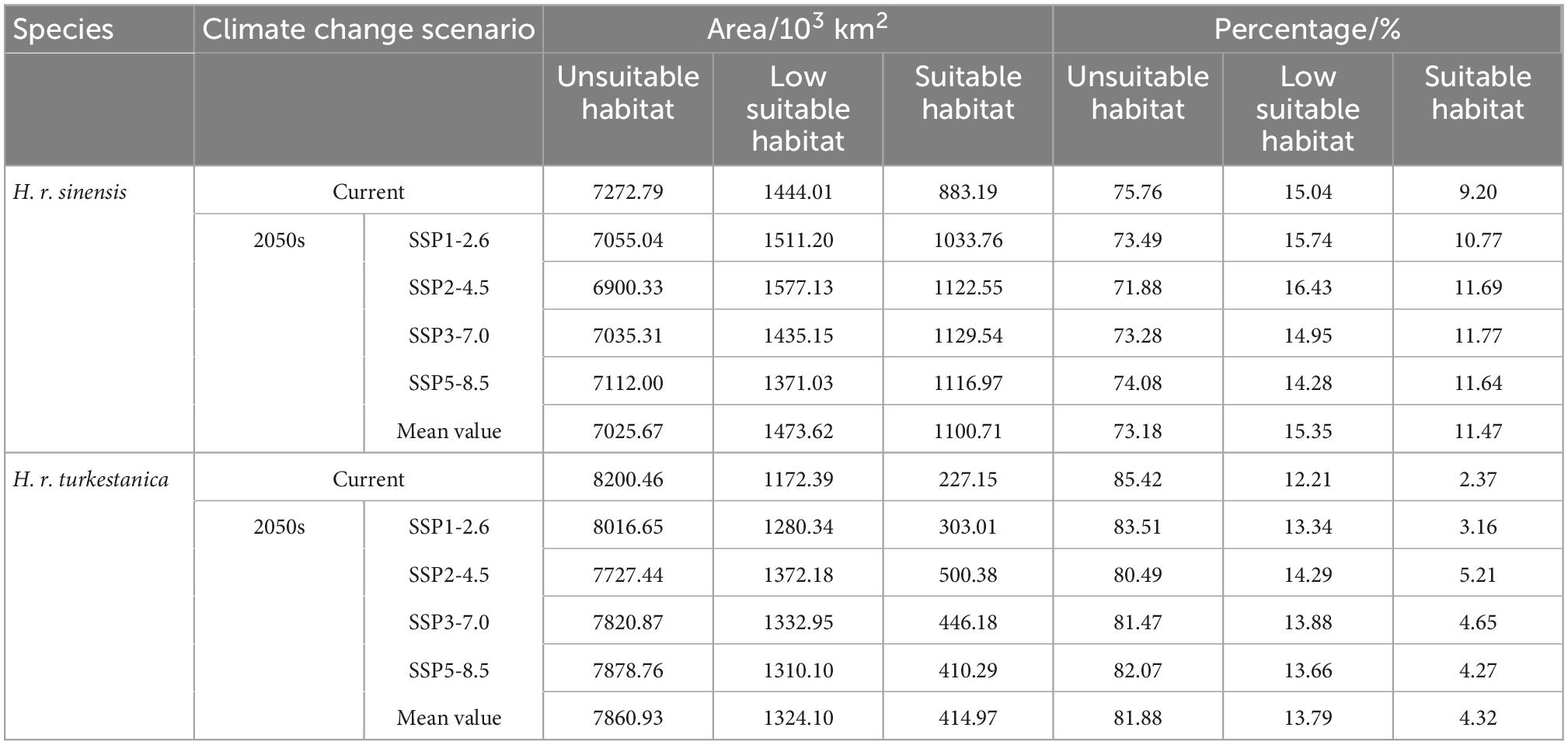
Table 3. Area and percentage of the suitable habitat of these two subspecies belonging to class three under the different climatic scenarios.
Under the different climatic scenarios in the 2050s, although the suitable habitat distribution of these two subspecies is similar to the current distribution (Figures 4B–E, 5B–E), they showed different increasing trends in the area of suitable habitat. For H. r. sinensis, the area of suitable habitat increased to 1033.76 × 103, 1122.55 × 103, 1129.54 × 103, and 1116.97 × 103 km2 under the climatic scenarios of SSP1-2.6, SSP2-4.5, SSP3-7.0, and SSP5-8.5, respectively. The average suitable habitat area is 1100.71 × 103 km2, accounting for 11.47% of the study area, an increase of 217.52 × 103 km2 from current. For H. r. turkestanica, the area of suitable habitat increased to 303.01 × 103, 500.38 × 103, 446.18 × 103, and 410.29 × 103 km2, and the average suitable habitat area is 414.97 × 103 km2, accounting for 4.32% of the study area. Their unsuitable habitat areas also will be reduced (Table 3).
3.3. Spatial pattern changes in the suitable habitat under the different climatic scenarios
Under the different future climate scenarios, significant dynamic changes occur for both H. r. sinensis and H. r. turkestanica compared to the current climatic scenario. For H. r. sinensis, degraded suitable habitat is mainly distributed in Hebei, Shangdong and Henan. At the same time, the suitability of some areas in Eastern inner Mongolia will experience a decrease significantly under the SSP5-8.5. The increased suitable habitat is mainly distributed in Tibet, Qinghai, and Inner Mongolia, and with the increase of emission concentration, a belt area is formed in Inner Mongolia from southeast of Alxa left banner to southwest of Hailar (Figures 6A–D). The increased suitable habitat of H. r. turkestanica is mainly distributed around the Jungar Basin, Altay and Tacheng, the Tianshan Mountains, and Turpan Basin in Xinjiang, central Changdu in Tibet, Western and central of Inner Mongolia, Jiuquan in Gansu, southwest of Sichuan in the future. Under SSP2-4.5, the area of suitable habitat increased the most, and mainly in Xinjiang. These are the main loss areas of suitable habitat, such as Xifeng in Gansu, Yulin and Yanan in Shaanxi, and the southern of Shanxi (Figures 6E–H).
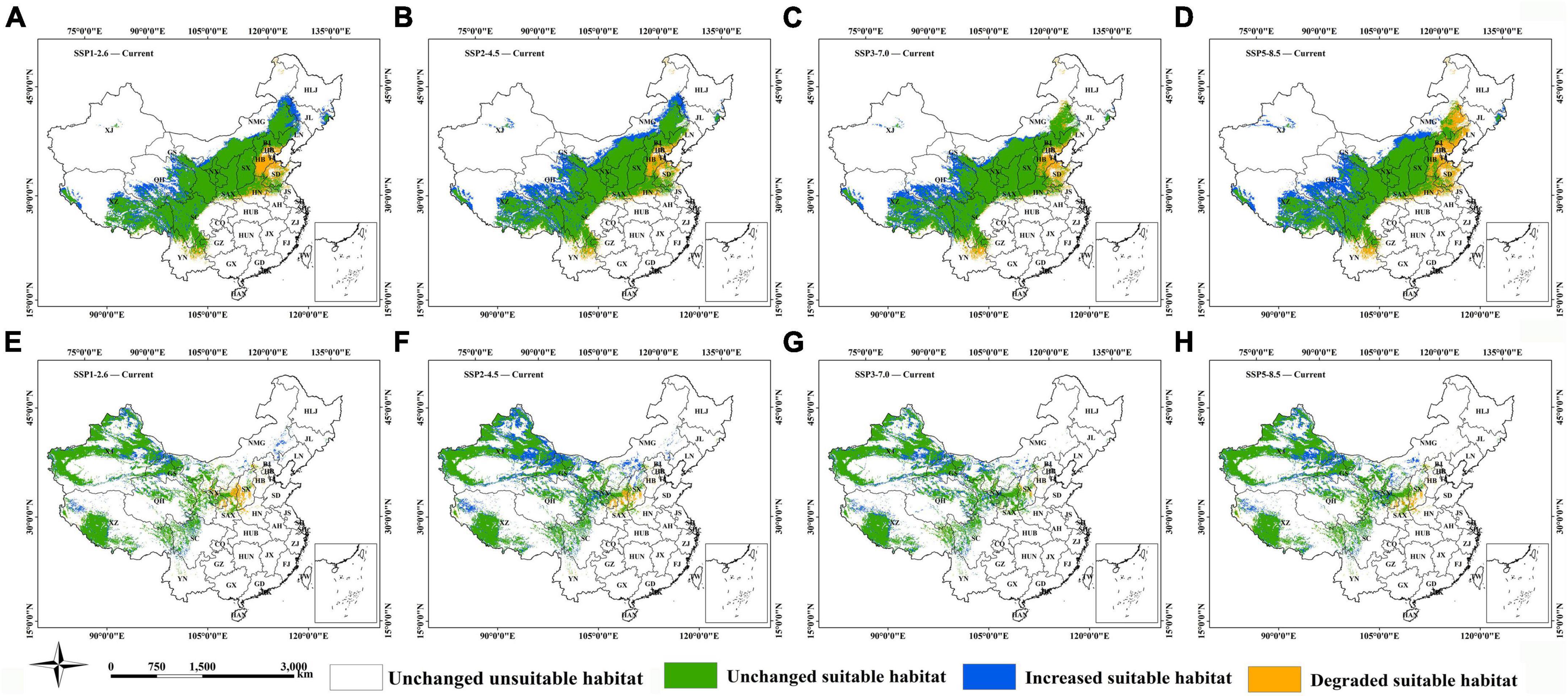
Figure 6. Changes in the habitat suitable for (A–D) H. r. sinensis and (E–H) H. r. turkestanica under the different climatic scenarios.
The habitats suitable for these two subspecies are relatively stable. The percentage of the stable suitable habitat for H. r. sinensis is slightly higher than that for H. r. turkestanica: The average value of the former is 79.06%, and the value of latter is 76.90% (Table 4). The results showed that the change in habitat area of the two subspecies from one species are slightly different under the different scenarios. The stability of suitable habitat for H. r. sinensis will show a slight decrease trend as the concentration of gas emissions increases. While H. r. turkestanica will be the least stable under SSP2-4.5, and the most stable under SSP1-2.6.
Figure 7 shows that the straight-line direction and distance of geographic migration of the suitable habitat centroids are heterogeneous. Currently, the centroid of suitable habitat is located in Guyuan county in southern Ningxia. While the centroids will gradually migrate westward with increasing emission concentrations under different future climate scenarios, and are distributed in Dingxi county, Linxia county and Xiahe county in Southeastern Gansu, respectively (Figure 7A). Overall, H. r. sinensis may migrate to the lower latitudes in the future. The centroids of H. r. turkestanica are located in Qiemo county, southeast Xinjiang. Currently, the centroid is located at 85°43′41″E, 38°21′26″N. Except for the centroid of the SSP2-4.5 scenario, the centroids will migrate to the higher latitude, the others migrate to the lower latitude (Figure 7B). The main migration direction will toward Eastern, and the magnitude of migration is greater under SSP2-4.5 and SSP3-7.0.
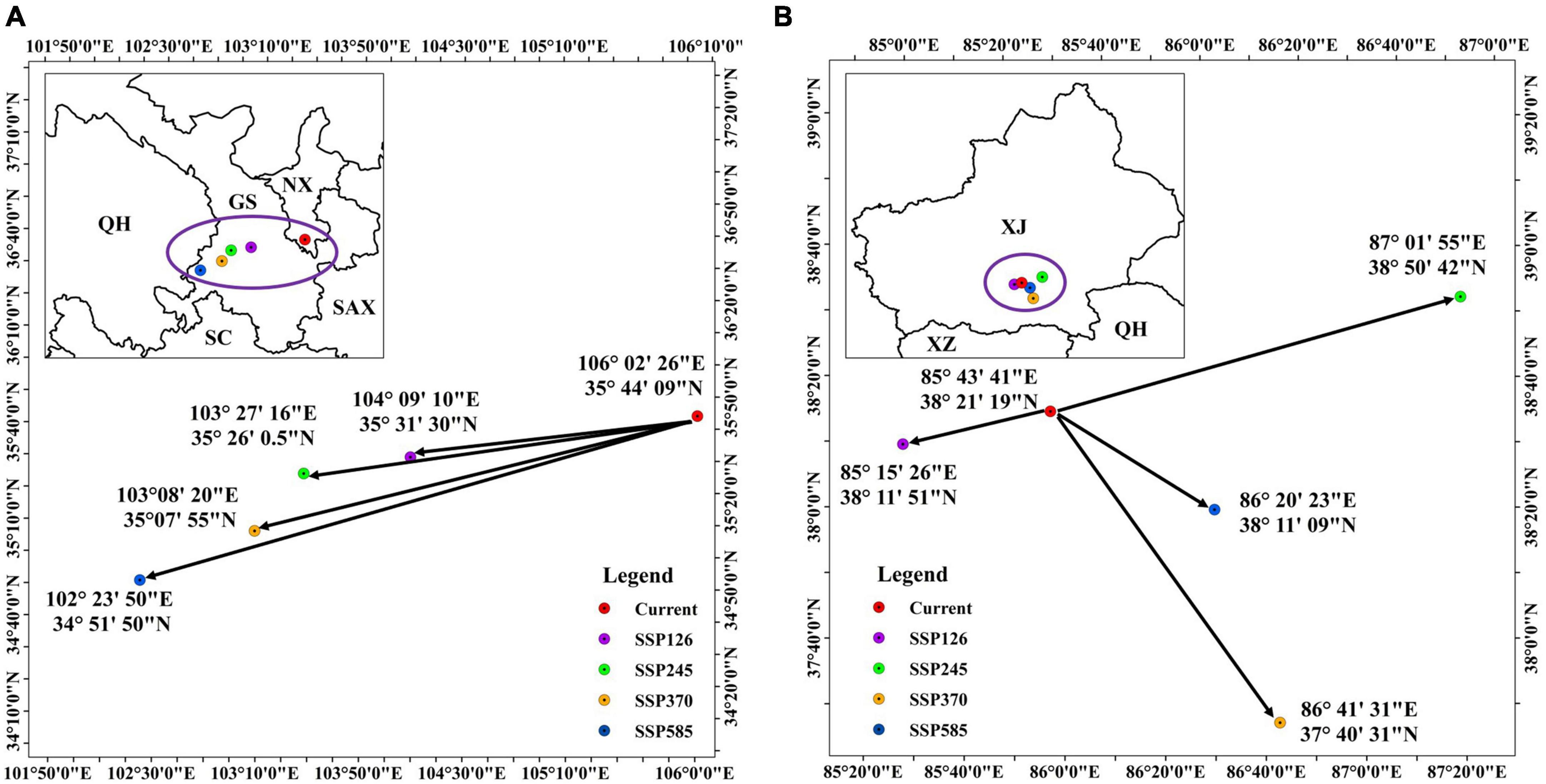
Figure 7. The centroid migration of suitable habitats of (A) H. r. sinensis and (B) H. r. turkestanica.
4. Discussion
4.1. Model accuracy and main environment variables
Although many methods can be used for understanding a species’ ecological niche distribution and for predicting the potential suitable habitat, the single model is still widely used. Among the SDM studies, MaxEnt is more effective in simulating the habitat suitability of plant species (Cobben et al., 2015; Ghareghan et al., 2020; Ab Lah et al., 2021). So, we chose this method for our study. The results showed that MaxEnt model can effectively simulate and predict the habitats suitable of H. r. sinensis and H. r. turkestanica. Therefore, we believed that the conclusions of this study are reliable. MaxEnt also was used to study the suitable distribution of H. r. sinensis by Li and Huang, who showed that the model achieved good simulation accuracy (Li et al., 2015; Huang et al., 2018). Despite MaxEnt having many advantages, some scholars think that a single model can produce over-fitting, and an integrated model may reduce the uncertainty of model fitting (Battini et al., 2019). The ensemble model (EM) strategy is widely used for studying the habitat distribution of species because of its higher accuracy, and better universality and fitting (Guo et al., 2019; Zhao et al., 2021). Moreover, MaxEnt is only applicable when considering abiotic factors; its applicability to areas strongly affected by human activities needs to be further verified. In future studies, other factors potentially affecting the distribution of species, such as human activities, biological interactions, diffusion constraints, and terrain variables (e g., landform classes, hill shade, heat load index, and compound topographic index) (Driver et al., 2020), should be more comprehensively considered. In this way, the results will be more accurate and the suitable habitat may be more fragmented.
Climatic elements have more influence on the growth and survival of these two subspecies of Hippophae rhamnoides than soil elements, especially H. r. sinensis. Bio13, bio11, and bio3 are the most important factors affecting the distribution of H. r. sinensis in this study. H. r. sinensis is affected by precipitation and temperature, and is less affected by soil, so it can be grown in meadow soil, chernozem, sandy soil and even in half-stone, sandstone, and sandy soil areas. The annual precipitation has a significant effect on its distribution: the 400 mm contour is the dividing line of the distribution area of H. r. sinensis on the Qinghai-Tibet Plateau. The Yunnan-Guizhou Plateau is unsuitable for its growth because its precipitation is too much, which is beyond the suitable range, so it does not extend to this area, which contradicts the research conclusion of Li et al. (2015). Whereas, Huang found that the precipitation of the coldest quarter has an important effect (Huang et al., 2018), it did not have significant effect in our study. This difference may be due to the different environmental variables selected and the future climate variables from different climate models. Precipitation and temperature are still the dominant factors affecting the geographical distribution of H. r. turkestanica. Its range of suitable habitat is narrower than that of H. r. sinensis, but its requirement for bio 13 is lower than that of H. r. sinensis, which may be the reason why the area of habitat suitable for H. r. turkestanica smaller than that of H. r. sinensis. H. r. turkestanica grows more easily under extreme drought conditions than H. r. sinensis.
4.2. Distribution of currently suitable habitats
Under the current climatic scenario, the habitat suitable for H. r. sinensis is concentrated in the middle and upper reaches of the Yellow River, the Loess Plateau, and the Northeastern to Southeastern margin of the Qinghai-Tibet Plateau, from the northeast of Hengduan Mountains to Taihang Mountains, north of Qinling Mountains and south of Yinshan Mountains, and it is distributed in a belt from Southwest to Northeast (Figure 4 and Table 3). The results are similar to that of Li’ results, but there are some differences in the area of suitable habitat, probably due to the number of selected sampling points and the suitability classification criteria applied (Li et al., 2015). H. r. sinensis likes sunlight and has certain water resources requirements, and distributes on banks of rivers, mountains, and valleys, and grows well in sparse forest land (Fischer et al., 2005). According to the difference in the climate and vegetation zonality in different growing areas of H. r. sinensis in China, four typical distribution areas can be identified: the Western Sichuan Plateau and Qinba Mountain distribution area, the Southern Loess Plateau distribution area, the central and northern Loess Plateau and Mu Us sandy land and arsenic sandstone distribution area, and the Qinghai Plateau distribution area (Dai et al., 2011). This subspecies can grow in the arid and barren Loess Plateau and arsenic sandstone areas due to its strong root germination and rich rhizobia, which can continuously improve the soil texture through nitrogen fixation. As a primitive group of Hippophae, Hippophae rhamnoides originated from the Qinghai-Tibet Plateau, some scholars have speculated that it may have spread from northeast to northwest and north China along the Hengduan Mountains. In the mid-1960s, H. r. sinensis invaded Mu Us sandy land through birds, and occupied the habitat with good water conditions in the area, and rapidly diffused to the slope and top of sand dunes, then formed a local unique ecological barrier. In recent years, H. r. sinensis has cultivated in Heilongjiang, Jilin, Shandong, Henan, and Beijing.
The suitable habitat area of H. r. turkestanica accounts for 2.37% of the total territory of China, and which is mainly distributed in Xinjiang, Tibet, and Gansu (Figure 5 and Table 3). A small amount of artificial cultivation occurs in Qinghai, Ningxia, and Inner Mongolia. It is often found in River Valley terraces, floodplains, and open hillsides. There are banded natural communities in the Tahe River Basin of Southern Xinjiang, along the Hotan River, bortara River Valley, both sides of the river in the Ali Region of Tibet, and Danghe river of Gansu. In addition, it shows drought resistance and barren resistance, and can grow in saline alkali beach and desert. For example, natural H. r. turkestanica grows in Taklimakan Desert. The two subspecies do not have high soil requirements, but some researchers showed that the growth of H. r. turkestanica is not as good as that of H. r. sinensis under the arsenic sandstone conditions.
4.3. Spatial changes in suitable habitats under future climate scenarios
Different regions have already experienced climate change and will continue in the future. Climate change significantly affects species survival. Species responses to climate change are heterogeneous under different climatic scenarios (Dyderski et al., 2018). Numerous studies have shown that climate change will not harm all species and the range of suitable habitat for most species will decrease and a few will expand. There is no general tendency for even closely related plants in the respond to climate change (Kolanowska et al., 2017). For the two subspecies considered in this study, the predicted range of suitable habitat under different climate scenarios in the future is basically consistent with the currently suitable habitat range, but shows significant dynamic changes. Their suitable habitat showed an increasing trend in the future climate scenario. But, the suitable habitat range, the direction and distance of centroid migration of these subspecies were heterogeneous (Figures 5–7). The increased suitable habitat of H. r. sinensis are mainly in Tibet, Qinghai, Gansu and Inner Mongolia, which are formed a belt in Inner Mongolia. The centroids of suitable habitat will move to the lower latitudes in the future and gradually migrate westward with increasing emission concentrations. H. r. turkestanica’s change also showed the same trend under the four climatic scenarios in the future, and its suitable range will increase. The centroids will move to the Eastern and lower latitude overall. With global warming, the area of arid and semi-arid areas will gradually increase (Huang et al., 2016). This may also be the reason for the expansion of suitable habitat of H. r. turkestanica in the future. Nevertheless, many studies had shown that species will move to places that are suitable for them, usually migrate to higher elevations and higher latitudes with climate warming (Li et al., 2013; Bezeng et al., 2017; Dyderski et al., 2018). The findings of this study do not support the view. The question of whether the response of subspecies to climate change can reflect the characteristics of the whole species needs to be further explored.
As a drought-tolerant plant with low requirement for natural environment, great adaptability, and with ecological, economic and social values, they are excellent species vigorously promoted for cultivation in China. In recent years, they have been successfully planted on a large scale on different types of degraded land, and the area of planted forests has been increasing year by year. However, extensive mortality has occurred in many artificial planting areas, mainly due to blind site selection and seed selection. For example, the central region of Inner Mongolia is only suitable for Hippophae rhamnoides subsp. mongolica, while Hippophae thibetana Schlechtend. and Hippophae gyantsensis (Rousi) Y.S. Lian cannot survive. Increased suitable habitat can be used as future artificial planting areas. The ecological niche of H. r. sinensis is wider than that of H. r. turkestanica, and more artificially planted, which has played a strong ecological role in the loess plateau, desert, watershed and other ecologically fragile zones and soil erosion areas. For most species, the suitable habitats are generally stable and will not face the risk of extinction with climate warming, exception of endangered species. Similarly, these two subspecies are not at risk of extinction in the future, and H. r. turkestanica is more vulnerable to climate change than H. r. sinensis. This is good news for the future planting of Hippophae rhamnoides Linn. in China. After 7–8 years of planting, Hippophae rhamnoides Linn. can form a plant community with more than 80% coverage, which greatly improves the local soil and water conservation capacity, increases soil fertility, improves biodiversity and stabilizes the ecosystem. The variation of suitable habitats of H. r. sinensis and H. r. turkestanica under different climatic scenarios can provide theoretical guidance and data support for the conservation of wild resources and the delineation of artificially expanded planting areas.
5. Conclusion
In this study, we successfully modeled the habitat suitability of H. r. sinensis and H. r. turkestanica under the current and future climatic change scenarios using MaxEnt. Our results showed that they prefer to grow in arid and semi-arid areas. H. r. sinensis is mainly affected by bio13, bio11, and bio3. H. r. turkestanica is mainly affected by bio13, bio2, and bio15, and is more suitable for growing in the extreme arid area, due to its requirement of annual precipitation is lower than that of H. r. sinensis. Although the two subspecies’ suitable habitat distribution have obvious differences, the changing trends are similar. The area of suitable habitat tends to expand, and the centroids will migrate to lower latitudes under future climate scenarios. Their distribution could also be affected by the river, so the modeling result could be improved adding this variable in the future. Hippophae rhamnoides Linn., as a multi-purpose tree species, plays an important role in the protection and restoration of ecological environment in China. Therefore, this study is helpful to the protection of wild resources and the expansion of artificial cultivation, and provides a scientific reference for eco-environmental managers.
Data availability statement
The original contributions presented in this study are included in the article/supplementary material, further inquiries can be directed to the corresponding authors.
Author contributions
X-HH: conceptualization, data curation, methodology, formal analysis, roles/writing—original draft, and writing—review and editing. J-HS: conceptualization, data curation, formal analysis, funding acquisition, and methodology. LZ: conceptualization, methodology, and funding support. D-MZ: data curation, formal analysis, and figures production. C-YZ and BJ: data curation and formal analysis. C-LW: visualization; JQ and X-LZ: data curation. All authors contributed to the article and approved the submitted version.
Funding
This study was supported by the Major Science and Technology Project in Inner Mongolia Autonomous region of China (no. Zdzx2018057), the Innovation Cross Team Project of Chinese Academy of Sciences, CAS (no. JCTD-2019-19), Transformation Projects of Scientific and Technological Achievements in Inner Mongolia Autonomous region of China (no. 2021CG0046), Science and Technology Research Project of Colleges and Universities in Inner Mongolia Autonomous Region (no. NJZY21034), and the National Natural Science Foundation of China (no. 42001038).
Conflict of interest
The authors declare that the research was conducted in the absence of any commercial or financial relationships that could be construed as a potential conflict of interest.
Publisher’s note
All claims expressed in this article are solely those of the authors and do not necessarily represent those of their affiliated organizations, or those of the publisher, the editors and the reviewers. Any product that may be evaluated in this article, or claim that may be made by its manufacturer, is not guaranteed or endorsed by the publisher.
Footnotes
- ^ https://www.gbif.org
- ^ http://www.nsii.org.cn/2017/
- ^ https://www.cvh.ac.cn
- ^ https://www.cnki.net
- ^ http://www.worldclim.org
References
Ab Lah, N. Z., Yusop, Z., Hashim, M., Salim, J. M., and Numata, S. (2021). Predicting the habitat suitability of melaleuca cajuputi based on the maxent species distribution model. Forests 12:1449. doi: 10.3390/f12111449
Battini, N., Farias, N., Belen Giachetti, C., Schwindt, E., and Bortolus, A. (2019). Staying ahead of invaders: Using species distribution modeling to predict alien species’ potential niche shifts. Mar. Ecol. Prog. Ser. 612, 127–140. doi: 10.3354/meps12878
Bellard, C., Bertelsmeier, C., Leadley, P., Thuiller, W., and Courchamp, F. (2012). Impacts of climate change on the future of biodiversity. Ecol. Lett. 15, 365–377. doi: 10.1111/j.1461-0248.2011.01736.x
Bezeng, B. S., Morales-Castilla, I., van der Bank, M., Yessoufou, K., and Daru, B. H. (2017). Climate change may reduce the spread of non-native species. Ecosphere 8:e01694. doi: 10.1002/ecs2.1694
Carpenter, G., Gillison, A. N., and Winter. (1993). Domain: A flexible modeling procedure for mapping potential distributions of plants and animals. Biodiv. Conserv. 2, 667–680. doi: 10.1007/BF00051966
Cobben, M. M. P., van Treuren, R., Castaneda-Alvarez, N. P., Khoury, C. K., and Kik, C. (2015). Robustness and accuracy of Maxent niche modelling for Lactuca species distributions in light of collecting expeditions. Plant Genet. Resour. Charact. Utiliz. 13, 153–161. doi: 10.1017/S1479262114000847
Dai, G., Li, G., Li, T., Li, S., and Xiao, Z. (2011). Effects of water conditions on the growth and population stability of hippophae rhamnoides subsp sinensis. J. Northw. Forest. College 26, 1–8.
Dawson, T. P. (2011). Beyond predictions: Biodiversity conservation in a changing climate. Science 332:664. doi: 10.1126/science.1200303
Driver, S., Schalk, C. M., Unger, D., and Kulhavy, D. (2020). Species distribution modeling for arid adapted habitat specialists in Zion National Park. Electr. Theses Dissert. 12:354.
Dyderski, M. K., Paz, S., Frelich, L. E., and Jagodzinski, A. M. (2018). How much does climate change threaten European forest tree species distributions? Glob. Change Biol. 24, 1150–1163. doi: 10.1111/gcb.13925
Elith, J., Graham, C. H., Anderson, R. P., Dudik, M., and Ferrier, S. (2006). Novel methods improve prediction of species’ distributions from occurrence data. Ecography 29, 129–151. doi: 10.1111/j.2006.0906-7590.04596.x
Elith, J., Phillips, S. J., Hastie, T., Dudik, M., and Chee, Y. E. (2011). A statistical explanation of MaxEnt for ecologists. Diver. Distrib. 17, 43–57. doi: 10.1111/j.1472-4642.2010.00725.x
Fang, Y., Zhang, X., Wei, H., Wang, D., and Chen, R. (2021). Predicting the invasive trend of exotic plants in China based on the ensemble model under climate change: A case for three invasive plants of Asteraceae. Sci. Total Environ. 756:143841 doi: 10.1016/j.scitotenv.2020.143841
Fick, S. E., and Hijmans, R. J. (2017). WorldClim 2: New 1-km spatial resolution climate surfaces for global land areas. Int. J. Climatol. 37, 4302–4315. doi: 10.1002/joc.5086
Fischer, G., Shah, M., Tubiello, F. N., and van Velhuizen, H. (2005). Socio-economic and climate change impacts on agriculture: An integrated assessment, 1990-2080. Philosoph. Transact. Roy. Soc. B Biol. Sci. 360, 2067–2083. doi: 10.1098/rstb.2005.1744
Gallagher, R. V., Hughes, L., Leishman, M. R., and Wilson, P. D. (2010). Predicted impact of exotic vines on an endangered ecological community under future climate change. Biol. Invas. 12, 4049–4063. doi: 10.1007/s10530-010-9814-8
GBIF (2020). Available online from: https://www.gbif.org/species/3039284 [accessed on 19,April 2020].
Ghareghan, F., Ghanbarian, G., Pourghasemi, H. R., and Safaeian, R. (2020). rediction of habitat suitability of Morina persica L. species using artificial intelligence techniques. Ecol. Indic. 112:106096. doi: 10.1016/j.ecolind.2020.106096
Guan, X., Shi, W., and Cao, K. (2018). Effect of climate change in future on geographical distribution of widespread quercus acutissima and analysis of dominant climatic factors. J. Trop. Subtr. Bot. 26, 661–668.
Guisan, A., Edwards, T. C., and Hastie, T. (2002). Generalized linear and generalized additive models in studies of species distributions: Setting the scene. Ecol. Model. 157, 89–100. doi: 10.1016/S0304-3800(02)00204-1
Guisan, A., and Thuiller, W. (2007). Predicting species distribution: Offering more than simple habitat models. Ecol. Lett. 10:435. doi: 10.1111/j.1461-0248.2007.01044.x
Guo, Y., Li, X., Zhao, Z., and Nawaz, Z. (2019). Predicting the impacts of climate change, soils and vegetation types on the geographic distribution of Polyporus umbellatus in China. Sci. Total Environ. 648, 1–11. doi: 10.1016/j.scitotenv.2018.07.465
Guo, Y., Wei, H., Lu, C., Gao, B., and Gu, W. (2016). Predictions of potential geographical distribution and quality of Schisandra sphenanthera under climate change. Peerj 4:e2554. doi: 10.7717/peerj.2554
Hamid, M., Khuroo, A. A., and Charles, B. (2019). Impact of climate change on the distribution range and niche dynamics of Himalayan birch, a typical treeline species in Himalayas. Biodiv. Conserv. 28, 2345–2370. doi: 10.1007/s10531-018-1641-8
Hirzel, A., and Guisan, A. (2002). Which is the optimal sampling strategy for habitat suitability modelling. Ecol. Model. 157, 331–341. doi: 10.1016/S0304-3800(02)00203-X
Hu, X. -G., Jin, Y., Wang, X. -R., Mao, J. -F., and Li, Y. (2015). Predicting impacts of future climate change on the distribution of the widespread conifer platycladus orientalis. PLoS One 10:e0132326. doi: 10.1371/journal.pone.0132326
Huang, J., Ji, M., Xie, Y., Wang, S., and He, Y. (2016). Global semi-arid climate change over last 60 years. Clim. Dynam. 46, 1131–1150. doi: 10.1007/s00382-015-2636-8
Huang, J., Li, G., Li, J., Zhang, X., and Yan, M. (2018). Projecting the range shifts in climatically suitable habitat for Chinese sea buckthorn under climate change scenarios. Forests 9, 1–11. doi: 10.3390/f9010009
Javidan, N., Kavian, A., Pourghasemi, H. R., Conoscenti, C., and Jafarian, Z. (2021). Evaluation of multi-hazard map produced using MaxEnt machine learning technique. Scientif. Rep. 11:6496. doi: 10.1038/s41598-021-85862-7
Kolanowska, M., Kras, M., Lipinska, M., Mystkowska, K., and Szlachetko, D. L. (2017). Global warming not so harmful for all plants - response of holomycotrophic orchid species for the future climate change scientific. Reports 7:12704. doi: 10.1038/s41598-017-13088-7
Lenoir, J., Gégout, J. C., and Marquet, P. A. (2008). A significant upward shift in plant species optimum elevation during the 20th century. Science 320, 1768–1771. doi: 10.1126/science.1156831
Li, G. Q., Du, S., and Guo, K. (2015). Evaluation of Limiting climatic factors and simulation of a climatically suitable habitat for Chinese Sea Buckthorn. PLoS One 10:e0131659. doi: 10.1371/journal.pone.0131659
Li, J., Fan, G., and He, Y. (2020). Predicting the current and future distribution of three Coptis herbs in China under climate change conditions, using the MaxEnt model and chemical analysis. Sci. Total Environ. 698:134141. doi: 10.1016/j.scitotenv.2019.134141
Li, X., Tian, H., Wang, Y., Li, R., and Song, Z. (2013). Vulnerability of 208 endemic or endangered species in China to the effects of climate change. Reg. Environ. Change 13, 843–852. doi: 10.1007/s10113-012-0344-z
Muttaqin, L. A., Murti, S. H., and Susilo, B. (2019). MaxEnt (Maximum entropy) model for predicting prehistoric cave sites in karst area of gunung sewu, gunung kidul, yogyakarta. Sixth Geoinform. Sci. Symp. 2019:11311. doi: 10.1117/12.2543522
Peterson, A. T., Papes, M., and Soberon, J. (2008). Rethinking receiver operating characteristic analysis applications in ecological niche modeling. Ecol. Model. 213, 63–72. doi: 10.1016/j.ecolmodel.2007.11.008
Phillips, S. J., and Dudik, M. (2008). Modeling of species distributions with Maxent: New extensions and a comprehensive evaluation. Ecography 31, 161–175. doi: 10.1111/j.0906-7590.2008.5203.x
Pielke, R. A., Avissar, R., Raupach, M., Dolman, A. J., and Zeng, X. B. (1998). Interactions between the atmosphere and terrestrial ecosystems: Influence on weather and climate. Glob. Change Biol. 4, 461–475. doi: 10.1046/j.1365-2486.1998.t01-1-00176.x
Pundir, S., Garg, P., Dviwedi, A., Ali, A., and Kapoor, V. K. (2021). Ethnomedicinal uses, phytochemistry and dermatological effects of Hippophae rhamnoides L.: A review. J. Ethnopharmacol. 266:113434. doi: 10.1016/j.jep.2020.113434
Su, H., Bista, M., and Li, M. (2021). Mapping habitat suitability for Asiatic black bear and red panda in Makalu Barun National Park of Nepal from Maxent and GARP models. Scientif. Rep. 11:14135. doi: 10.1038/s41598-021-93540-x
Suryakumar, G., and Gupta, A. (2011). Medicinal and therapeutic potential of Sea buckthorn (Hippophae rhamnoides L.). J. Ethnopharmacol. 138, 268–278. doi: 10.1016/j.jep.2011.09.024
Swenson, U., and Bartish, I. V. (2002). Taxonomic synopsis of Hippophae (Elaeagnaceae). Nordic J. Bot. 22, 369–374. doi: 10.1111/j.1756-1051.2002.tb01386.x
Swets, J. A. (1988). Measuring the accuracy of diagnostic systems. Science 240, 1285–1293. doi: 10.1126/science.3287615
Tekin, S., Guner, E. D., Cilek, A., and Unal Cilek, M. (2021). Selection of renewable energy systems sites using the MaxEnt model in the Eastern Mediterranean region in Turkey. Environ. Sci. Pollut. Res. 28, 51405–51424. doi: 10.1007/s11356-021-13760-6
Ui Haq, S. A., Mir, M. A., Lone, S. M., Banoo, A., and Shafi, F. (2021). Explicating genetic diversity based on its characterization and determination of antioxidant potential in sea buckthorn (Hippophae spp.). Mole. Biol. Rep. 49, 3839–3847. doi: 10.1007/s11033-021-06619-z
Wei, B., Wang, R., Hou, K., Wang, X., and Wu, W. (2018). Predicting the current and future cultivation regions of Carthamus tinctorius L. Using MaxEnt model under climate change in China. Glob. Ecol. Conservat. 16:e00477 doi: 10.1016/j.gecco.2018.e00477
Worthington, T. A., Zhang, T., Logue, D. R., Mittelstet, A. R., and Brewer, S. K. (2016). Landscape and flow metrics affecting the distribution of a federally-threatened fish: Improving management, model fit, and model transferability. Ecol. Model. 342, 1–18. doi: 10.1016/j.ecolmodel.2016.09.016
Wu, T. W., Lu, Y. X., Fang, Y. J., Xin, X. G., Li, L., and Li, W. P. (2019). The Beijing Climate Center Climate System Model (BCC-CSM): The main progress from CMIP5 to CMIP6. Geosci. Model. Dev. 12, 1573–1600.
Yi, Y., Cheng, X., Yang, Z., and Zhang, S. (2016). Maxent modeling for predicting the potential distribution of endangered medicinal plant (H. Riparia Lour) in Yunnan, China. Ecol. Engin. 92, 260–269. doi: 10.1016/j.ecoleng.2016.04.010
Zhang, X., Wei, H., Zhao, Z., Liu, J., and Zhang, Q. (2020). The Global Potential Distribution of Invasive Plants: Anredera cordifolia under Climate Change and Human Activity Based on Random Forest Models. Sustainability 12:1419. doi: 10.3390/su12041491
Zhao, Z., Guo, Y., Zhu, F., and Jiang, Y. (2021). Prediction of the impact of climate change on fast-growing timber trees in China. Forest Ecol. Manag. 501:119653 doi: 10.1016/j.foreco.2021.119653
Keywords: environmental variables, climate change scenarios, maximum entropy (MaxEnt) model, suitable habitat, centroid migration
Citation: He X-h, Si J-h, Zhu L, Zhou D-m, Zhao C-y, Jia B, Wang C-l, Qin J and Zhu X-l (2023) Modeling habitat suitability of Hippophae rhamnoides L. using MaxEnt under climate change in China: A case study of H. r. sinensis and H. r. turkestanica. Front. For. Glob. Change 5:1095784. doi: 10.3389/ffgc.2022.1095784
Received: 11 November 2022; Accepted: 28 December 2022;
Published: 11 January 2023.
Edited by:
YanLong Guo, Institute of Tibetan Plateau Research (CAS), ChinaReviewed by:
Biao Zhang, Institute of Geographic Sciences and Natural Resources Research (CAS), ChinaRuonan Li, Research Center for Eco-Environmental Sciences (CAS), China
Copyright © 2023 He, Si, Zhu, Zhou, Zhao, Jia, Wang, Qin and Zhu. This is an open-access article distributed under the terms of the Creative Commons Attribution License (CC BY). The use, distribution or reproduction in other forums is permitted, provided the original author(s) and the copyright owner(s) are credited and that the original publication in this journal is cited, in accordance with accepted academic practice. No use, distribution or reproduction is permitted which does not comply with these terms.
*Correspondence: Jian-hua Si, amlhbmh1YXNAbHpiLmFjLmNu; Li-Zhu,
MTU2NDcyMjkyNDRAMTI2LmNvbQ==