- 1Escuela Técnica Superior de Ingeniería de Montes, Forestal y del Medio Natural, Departamento de Sistemas y Recursos Naturales, Universidad Politécnica de Madrid, Madrid, Spain
- 2Kaudal, Edificio NANOtec, San Cristóbal de La Laguna, Spain
- 3Estación Experimental de Zonas Áridas, Consejo Superior de Investigaciones Científicas, Almería, Spain
- 4Department of Forestry and Natural Resources, Hardwood Tree Improvement and Regeneration Center, Purdue University, West Lafayette, IN, United States
Forest restoration is challenging in arid and semiarid lands. Research has identified ecotechnologies that may alleviate stressful conditions of planted seedlings, but studies are often limited to the first few years of regeneration establishment. Over 20 years, we tested the effects of tree shelters (mesh- and tube-shelters) and competition suppression (two types of synthetic mulch and manual weeding) on soil water content and development of Pinus halepensis on an arid site in southeastern Spain. Competition suppression increased soil water content at shallow depths only using a polyethylene sheet, with no effect on survival. Tree shelters had a much greater effect than competition suppression on tree responses. Survival was mostly affected by shelter type, with lower survival in tube-shelters compared to mesh-shelters and non-protected seedlings; differences began after the first year, but became more pronounced following an intense drought at 3 years. Survival for pines protected by mesh-shelters was significantly higher than for non-protected seedlings beginning at 6 years, and mortality did not stabilize until 15 years. After 20 years, survival ranked in order of mesh-shelters (57.5%), non-sheltered (46%), and tube-shelters (29.5%). Effects of shelter type on growth response over time followed an opposite pattern, with differences declining when pines outgrew the height of the shelter after 3 years. The response of pines to tube-shelters could be explained by the microenvironmal differences among vertical protection and its effects on a shade intolerant species such as Pinus halepensis: survival depletion is provoked probably by a reduction in root growth under the shaded conditions of tubes. On the other side, growth and morphology response could be partially explained by differences in light, but response of pines in mesh also points towards a reduction of wind pressure that precludes stem growth in thickness. Competition suppression had no effect on pine growth. Lack of response to main effects of competition suppression treatments can be attributed to the minimum changes of soil water content to mulching or weeding under arid conditions. Thus, 20 years after planting, tube-shelters and competition suppression treatments were relatively ineffective at improving survival or growth of P. halepensis. Mesh-shelters are recommended as the most effective protection method for restoration under arid site conditions.
1. Introduction
Forest restoration by afforestation is an effective way of reducing degradation of soils and increasing the provision of ecosystem services (Löf et al., 2019; Castro et al., 2021). However, restoration initiatives are often challenged by poor performance of planted seedlings, particularly in harsh or arid environments where abiotic and biotic limiting factors constrain survival and growth. Shallow soil and poor fertility (particularly low organic matter and N content, Delgado-Baquerizo et al., 2013), predation (Castro et al., 2015), and especially the extensive and intense summer drought of drylands precludes establishment, causing significant failures and subsequent economic loss (Vallejo et al., 2012) that may deter private or public land owners to invest in restoration (Cortina et al., 2011). Limitations imposed by these factors can be alleviated by employing suitable restoration techniques. Most ecotechnological tools commonly used in arid environments have focused on deterring seedling predation or improving microsite conditions and resource availability (Piñeiro et al., 2013; Muñoz-Rengifo et al., 2020). The implementation of these ecotechnologies is particularly important in highly degraded areas with low retention of water and nutrients (Vallejo et al., 2012), which generally experience more extreme and harsh summers (IPCC, 2014) and require continuous improvements in planting techniques (Chirino et al., 2009; Valdecantos et al., 2014; Jacobs et al., 2015).
Among the ecotechnologies used to improve forest restoration success are tubes for tree sheltering and mulching. Solid-walled tree shelters (hereafter, referred to as tube-shelters) represent one of the most widespread cultural practices in reforestation programs in Mediterranean climates over the past 20 years (Oliet et al., 2019). Along with protecting seedlings from animal predation, research conducted in the Mediterranean has demonstrated significant changes in microclimatic conditions (Del Campo et al., 2006; Bergez and Dupraz, 2009; Puértolas et al., 2010; Oliet et al., 2019) that alter growth and patterns of biomass allocation (Oliet and Jacobs, 2007; Vázquez de Castro et al., 2014; Oliet et al., 2021). These changes are overall positive for survival and growth within dry areas (Piñeiro et al., 2013), although the effect is clearly species-specific (Puértolas et al., 2010; Padilla et al., 2011; Oliet et al., 2016, 2019). Along with tree shelters, mesh-walled tree shelters (hereafter, referred to as mesh-shelters) have also been extensively used to prevent browsing (Taylor et al., 2006; Thyroff et al., 2022). Unlike tube-shelters, mesh-shelters minimally affect microclimatic conditions around the seedling relative to ambient (non-sheltered) conditions (Close et al., 2009; Padilla et al., 2011; Oliet et al., 2019). Similar to tube-shelters, however, mechanical restrictions to lateral growth and branching, as well as to stem bending by wind can influence growth patterns of the tree (Coutand et al., 2008; Devine and Harrington, 2011), with effects persisting over time (Abe, 2022). Simultaneous evaluation of mesh-shelters alongside tube-shelters is needed to help identify the most adequate protection system in afforestation.
Groundcover or mulching is another ecotechnology used to improve restoration success. This technique involves covering the soil around the seedlings with an opaque layer that impedes weeds from germinating and establishing (Coello et al., 2018a). The application of mulch is an effective measure also to reduce the kinetic energy of raindrops, avoid soil crusting, promote water infiltration or reduce soil temperature oscillation (Santana et al., 2014). In addition, mulch can reduce evaporation (Barajas-Guzmán et al., 2006) and contribute to controlling plant competition by hampering the establishment of neighboring vegetation (Chirino et al., 2009; Coello et al., 2018a). Types of mulch are numerous: natural mulches like forest litter and logging debris can be shredded on site; placing stones around the seedling is a traditional practice in dry areas (Jiménez et al., 2017); manufactured mulches, such as polyethylene sheets or fiber mats, have desirable properties including higher durability (Haywood, 1999) and/or different degrees of permeability (Coello et al., 2018a). For instance, impermeable polyethylene sheets can reduce evaporation after rainfall (Andrews, 2002), with positive effects on survival (Barajas-Guzmán et al., 2006). However, the extent to which these improvements affect planting performance of seedlings is highly species and site-specific. Additionally, mulch can be an efficient alternative to mechanical control if herbicides are strongly regulated (Ammer et al., 2011; González-Tokman et al., 2017). In tropical or temperate areas or in former arable lands, vegetative competition is intense and weed control is one of the most important silvicultural practices for establishing forest plantations (Barajas-Guzmán et al., 2006; Hytönen and Jylhä, 2010; Coello et al., 2018b). However, in dry and degraded areas, seed banks are scarce (Maestre and Cortina, 2004), and the benefits of mulch can be due to effects other than competition suppression, such as evaporation reduction or water infiltration enhancement (Haywood, 1999; Santana et al., 2014). In particular, the trade-off effect between reducing soil evaporation and absorbing water from rainfall may be highly dependent on the mulch material and climatic conditions (Andrews, 2002). In dry Mediterranean areas, impermeable polyethylene sheets may potentially reduce seedling water availability under the low rainfall events that constitute the most common source of precipitation (Mayor et al., 2011). However, the number of studies examining the effect of mulch type on soil moisture dynamics during plantation establishment in dry areas are very scarce (but see Jiménez et al., 2017).
In dry (arid or semi-arid) Mediterranean conditions, the establishment phase during the wet season when roots grow in depth to avoid dry upper horizons in the dry season, is critical for survival (Padilla and Pugnaire, 2007; Villar-Salvador et al., 2012). Because most mortality occurs during the first summer following planting, ecotechnologies in dry areas are tested mainly during the first year of planting (Vallejo et al., 2012; Piñeiro et al., 2013). However, the effects of management at planting can diverge beyond the first summer as treatment responses often shift over time or with meteorological conditions (Oliet et al., 2009). Thus, long-term studies are especially important to help identify treatment-related variation with time and ascertain sustained effects (Bautista and Alloza, 2009). For instance, we still have a poor knowledge of the effect of tree shelters on growth and mechanical stability of the tree at mid- to long-term in harsh areas or with slow growing species (but see Dupraz, 1997; Oliet et al., 2005, 2016; Jacobs, 2011). This information is needed to support decisions such as type of shelter (mesh- vs. tube-shelters) or time to remove the shelter. Additionally, although some studies have compared planting performance of Mediterranean species under solid- vs. mesh-walled shelters (Close et al., 2002, 2009; Puértolas et al., 2010; Padilla et al., 2011; Vázquez de Castro et al., 2014; Oliet et al., 2019) none of them followed planted seedling responses beyond the first establishment year. Furthermore, mulching effects on survival and growth can change after the first year of planting as mulch properties such as degradation degree or permeability vary with time depending on the material forming the mulch (Jiménez et al., 2013). Prior studies such as Haywood (1999) and Coello et al. (2018b) have evaluated changes in mulch properties over 3 years, but these studies were conducted in temperate areas. We are aware of only one study under Mediterranean conditions that evaluated mulch type over a relatively long duration (9 years), although in this case only natural mulches were tested (Jiménez et al., 2013).
Analyzing long term responses of mulching and tree shelters combined will provide additional useful information toward development of cost-effective management in dry area plantations (Bautista and Alloza, 2009). Although some studies in the Mediterranean have included the combined effect of weed control and tree shelters on tree establishment (Chaar et al., 2008; Mechergui et al., 2013, 2019; Ceacero et al., 2014), none have monitored long-term responses. Longer-term studies (>10 years) are necessary to fully evaluate the outcome of management practices in semi-arid areas (Cortina et al., 2011).
In this study, we evaluated the mid- to long-term effects (20 years) of tree shelters (mesh- and solid-walled) and competition suppression (two types of synthetic mulches with different permeability and manual weeding) combined on the development of a Pinus halepensis Mill (Aleppo pine) plantation in a Mediterranean arid area of Southeast Spain. Aleppo pine is a shade intolerant early successional species (Niinemets and Valladares, 2006) that is commonly used for afforestation in the arid lands of the Mediterranean basin (Maestre and Cortina, 2004). Due to its high resistance to aridity, this species plays a critical role in the restoration of degraded lands and abandoned croplands in this region (Ne’eman and Trabaud, 2000). Seedling survival and growth response to treatments were evaluated, along with soil moisture during the first years to disentangle the relationships between treatments and plant response. The objectives of the study were (1) evaluate tree shelters and mulch types under this environment (2) analyze potential interactions between time and treatments at mid to long-term and (3) provide a rationale for the management of ecotechnologies for restoration of dry areas. We hypothesized that (1) pine trees will perform (survival and growth) better when unprotected; (2) mulch effects will depend on the material but will not differ from manual weeding; and (3) there will be a long-term effect of treatments beyond the establishment phase.
2. Materials and methods
2.1. Planting site and experimental design
The planting site was in the southeast of Almería province, Southeast of Spain (2°0′W, 36°58′N, elevation 230 m), on a hillside with a moderate slope and SE aspect. The previous stand was a 20-year-old P. halepensis plantation on terraces destroyed by a 1994 forest fire. The natural vegetation is typical of degraded areas from the arid to semiarid Mediterranean region, dominated by thyme (Thymus spp.) and alpha grass (Stipa tenacissima L.) (Ruiz de la Torre, 1990) with scattered mastic (Pistacia lentiscus L.) and wild olive trees (Olea europaea var. sylvestris). The soil profile is rocky, of the unit calcaric regosol (FAO), and developed on calcareous parent material (MAPA, 1989). The climate is arid Mediterranean, with extremely hot and dry summers. Average annual rainfall and mean temperature of the area are 190 mm and 17.9°C, respectively (Spanish Agency of Meteorology, 2021).
Planting was conducted on 22 November 1995, in mechanically opened pits 0.95 m × 0.95 m × 0.95 m upon the 3.8-m wide terraces of the old burned plantation. Two staggered rows of pits were opened per terrace. Pits were 1.95 m apart. Container grown seedlings of P. halepensis (seeds collected in local provenance 2°30′ W, 37°30′ N, elevation 600–800 m, ES15′ Bética meridional’ Alía et al., 2009), were raised in a local nursery in 230 ml containers (75 containers per tray; density of 308 m–2) filled with a mixture of 80:20 (percent volume) moss peat:vermiculite and fertilized with 5 g.l–1 of Osmocote Plus 15-8-11 (Scotts, Netherlands). After 1 year of culture, pre-planted seedlings were 36.3 ± 1.3 cm height, 3.3 ± 0.1 mm root collar diameter, 3.6 ± 0.2 and 1.4 ± 0.1 g shoot and root weight, respectively, and 18.7 ± 0.8 mg⋅g–1 shoot N concentration (n = 28).
Treatments were arranged in a two-factor (tree shelter × competition suppression) experiment. Three levels of tree shelter (no protection, mesh- and solid-walled tube shelters) and four competition suppression levels (no weeding, two types of synthetic mulching and manual weeding) were fully crossed to a total of twelve treatments. Manual weeding with a hoe was conducted annually in spring from planting until 2002 around the plant on an area equal to the planting pit (0.95 m × 0.95 m). Two types of mulch were tested: impermeable plastic (polyethylene film) sheet 0.82 m × 0.82 m green color upper side (Tubex Co., South Wales, UK) and permeable coconut fiber mat 0.70 m × 0.75 m (Projar SA, Valencia, Spain). Mulches were placed on soil with the seedling in the central hole and fixed with stones on corners to avoid wind blowing. Tube-shelters were an unventilated brown, translucent, circular, twin-walled polypropylene tube 0.6 m tall × 0.11 m wide (Tubex ®, Fiberweb Geosynthetics Ltd., UK) anchored by an iron stick. Average light transmission coefficient of the tube-shelter is 45% (Oliet et al., 2003). Mesh-walled tree shelters consisted of a 60 cm tall × 15 cm wide cylindrical blue polyethylene net with mesh holes 0.8 cm × 0.8 cm (Redplanton, Projar SA, Valencia, Spain), anchored by two bamboo sticks. The light transmission coefficient was 83% (Vázquez de Castro et al., 2014). Neither shelter types were removed during the study period. The 12 treatments were arranged as a randomized complete block design with five replications. The experimental unit consisted of a row of 25 seedlings. Experimental units in a block were placed on two to four consecutive terraces, and blocks were arranged along the slope. A total of 1,500 seedlings were planted, 125 seedlings per treatment.
2.2. Measurements
In January of 1996 (first measurement), 1997, 1998, 1999, 2001, 2003, or February 2009 and 2016, seedlings were measured for survival, height (h), and groundline stem diameter (GSD). Stem volume was calculated using the formula for a cone: 0.2618 (GSD2 × h). In addition, during 2016 measurement, two orthogonal diameters of the crown vertical projection were taken, and projected crown area of the tree was calculated by averaging both diameters. Precipitation from planting to 2002 was recorded with an automatic pluviograph (DATA-RAIN 128 Geonica SA, Spain) installed on the planting site. From 2003 to the end of the study, rainfall was predicted from database Chelsa (Climatologies at high resolution for the earth’s land surface areas, Karger et al., 2017). Annual rainfall from 1996 to 2016 averaged 168.5 mm, with an extremely dry year (78.2 mm) in 1998 (Supplementary Figure 1).
Soil moisture was monitored during 3 years following planting with a time-domain reflectometry (TDR) equipment (Trase System, Soil Moisture Equipment Corp., USA). One permanent station per weeding treatment was randomly installed in each block, summing up to 4 (weeding treatments) × 5 (blocks) = 20 permanent stations in total throughout the plantation. Each station consisted of three pairs of stainless-steel connected rods of 15, 30, and 60 cm length inserted in the soil around one planted seedling on a 10-cm radius circumference. Measurements were recorded monthly during the first year after planting (1996), every 2 months during 1997, every 3 months in 1998, and every 4 months in 1999, 2000, and 2001 with a final measurement conducted at the beginning of 2003. Each individual value corresponds to the average of the three single values compiled instantaneously to stabilize the measure. Given that each value corresponds to the average of volumetric soil water content (VWC, θ) in the entire profile of the rods, θ at 15–30 and 30–60 cm depth at each station were calculated from readings at 0–15, 0–30, and 0–60 cm of the correspondent station as follows:
To determine water content at wilting point (–1500 kPa, Carminati et al., 2017), five samples (one per block) were taken from a TDR station at the three depths. Gravimetric water content at wilting point was determined with the Soilmoisture system (Santa Barbara, CA, USA). Volumetric water content at this point was calculated as the product of later magnitude and soil bulk density of each sample. Volumetric water content at wilting point for each depth was 11.2 ± 1.4% (0–15 cm), 12.2 ± 2.0% (15–30 cm), and 10.4 ± 1.5% (30–60 cm).
2.3. Data analysis
Soil VWC data were analyzed over the measurement period for each depth using repeated measures one-way ANOVA with competition suppression as a between subject factor and date as the within subject factor. Standard error of the whole studied period for VWC was calculated from average data from each date (mean of the five replicated stations). Survival data analysis from the planting experiment for each year was conducted by a generalized lineal model based upon a binomial error distribution with a logit link function. Full model included tree shelter (TS), competition suppression (CS), TS × CS interaction and experimental block as predictors. Growth data (height, basal stem diameter, slenderness quotient, stem volume and projected crown area) analysis for each year was conducted by a linear mix model. In this model the fixed effects were tree shelter, competition suppression and their interactions, while random effects were block, row (nested in block, tree shelter, and competition suppression) and individual tree (nested in block, tree shelter, competition suppression, and row) (Sit, 1995). In cases where ANOVA treatment effects were significant (P < 0.05), statistical differences among factor levels and treatments were identified using Fisher’s protected least significant difference (LSD) test, adjusting the overall α level to 0.05 by Bonferroni’s correction. SPSS Statistical Package version 26.00 (SPSS Inc., Chicago, IL, USA) was employed for data analysis.
3. Results
3.1. Soil water content during first three years of establishment
Volumetric soil water content during the first 3 years was significantly affected by competition suppression treatments only at the shallow (0–15 cm) depth [F(3,16) = 4.517, P = 0.018]. On average, VWC at 0–15 cm under polyethylene sheet was maximum (7.0 ± 0.9%) and significantly higher than minimum soil VWC under coconut fiber mat (5.9 ± 0.8%). Values of average VWC for the rest of the treatments did not differ from polyethylene or fiber mulches. Across seasons, VWC at 0–15 under polyethylene film was maximum after a rainy period, when soil water reached a maximum. However, during dry seasons, differences in VWC among treatments dissipated (Figure 1A).
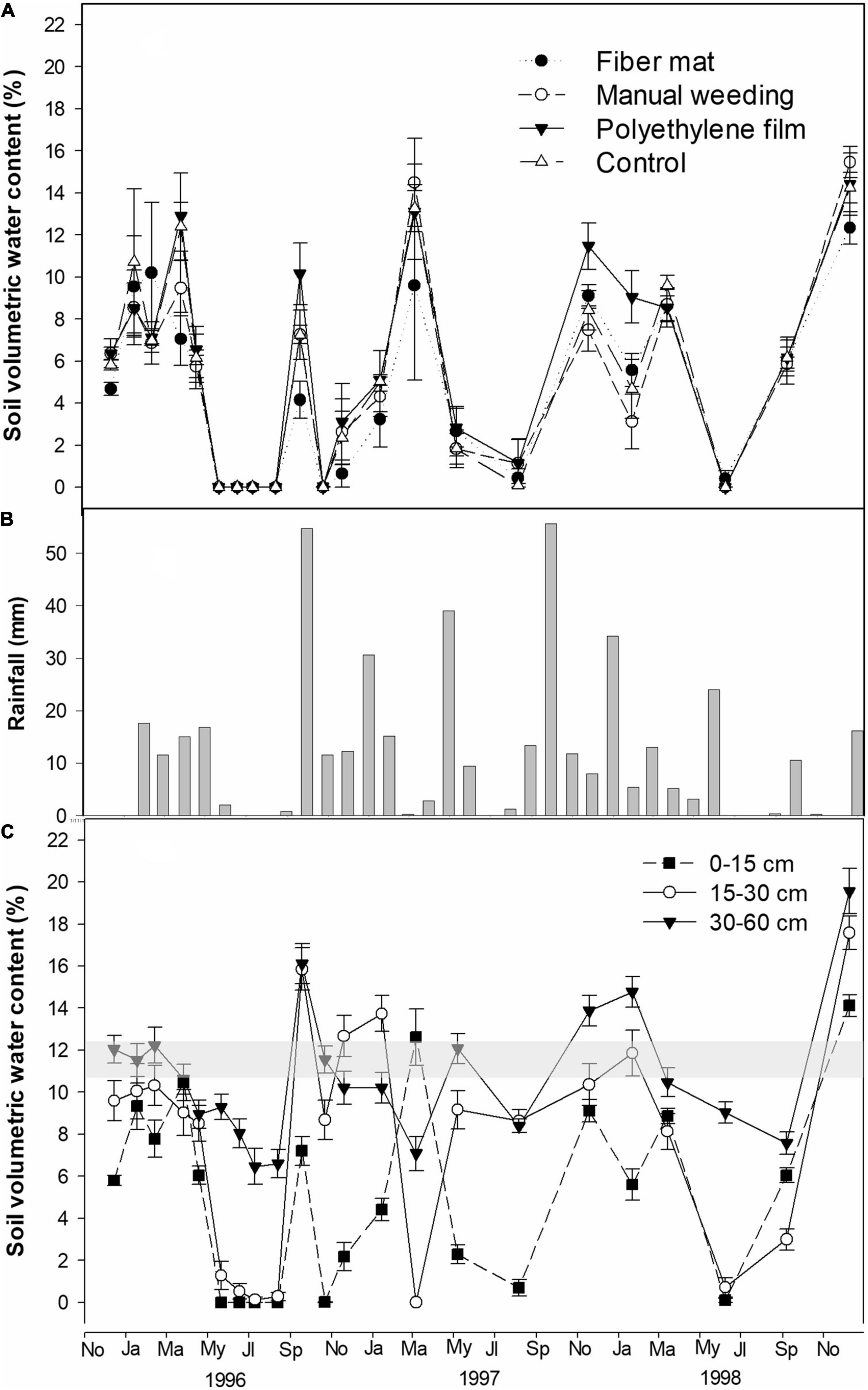
Figure 1. Soil volumetric water content ± SE (VWC) and rainfall during the first 3 years of the study period (from planting in November 1995 to the end of 1998): (A) VWC at 0–15 cm as affected by competition suppression treatments (n = 5); (B) monthly rainfall (mm); (C) VWC at three depths averaged by treatments (n = 20). In sub-figure (C) wilting point range is shaded (see section “Materials and methods” for details).
The response of VWC was different by seasons along the soil profile. In general, VWC was minimum at 0–15 cm, followed by 15–30 cm depth, with values that dropped to 0 in summer months (Figure 1C) when precipitation was null (Figure 1B). This was especially apparent during the first year after planting (1996) when the soil water level from 0 to 30 cm was below the wilting point since time of planting, while from 30 to 60 cm soil water was maintained around the wilting point from planting to April (Figure 1C). In general, soil VWC of deepest horizons (30–60 cm) was more stable with seasons, with values above 6% even during the dry summer of 1996. Year 1997 was more humid (Figure 1B), while 1998 was a very dry year, with almost no rain from May to November with the exception of 10.6 mm that fell in September. This resulted in minimum values of soil VWC from July to September not only at 0–15 cm, but also at 15–30 cm. As a result, during the first 3 years after planting, soil water was below the wilting point for 0–15 cm and 15–30 cm depths, and kept above this value for 30–60 cm depth along several months of fall and winter of the studied period (Figure 1C). No significant interactions between time and competition suppression factors on soil humidity were found across the studied period for any soil depth.
3.2. Survival during 20 years following planting
Survival of P. halepensis seedlings dropped strongly during the first year after planting to an average of 78.2% across factors in early 1997. This decrease in survival was primarily affected by levels of tree shelters, with a lower survival for pines in tube-shelters (Figure 2A) that boosted significant differences with no shelter or mesh-shelter after 1 year of planting (Table 1). However, a significant tree shelter × competition suppression interaction appeared during the first year, showing reduced survival for manually weeded and non-protected pines (Table 1 and Supplementary Figure 2). Survival barely dropped during 1997 (in average 1.5% for all treatments), while a new intense drop in survival occurred during 1998. In this year, and similar to 1996, this descent was more pronounced for pines protected with solid-walled tube-shelters, reaching values of 54.6 ± 2.3% survival at early 1999, while those protected with mesh-shelters or non-protected showed significantly (Table 1) higher survival (average survival of the latter two treatments was 73.0 ± 2.0% at the beginning of 1999, Figure 2). Tree shelter × competition suppression interaction became more significant during 1998 (see increases in χ2Wald from 1998 to 1999 in Table 1), due to a higher reduction in survival for manually weeded and non-protected pines, but also for control (non-weeded) pines in mesh (Supplementary Figure 2). During the next 2 years (1999 and 2000) survival continued dropping at a higher rate for trees in tube-shelters (16.9% in 2 years) than that of those protected with meshes (7.9%), although in these 2 years the mortality rate of non-protected (13.0%) seedlings was higher than that of pines in mesh. The latter was due to the more intense drop in survival of non-protected and manually weeded pines, along with a more intense survival decline for non-weeded pines in mesh (significant interaction, Table 1 and Supplementary Figure 2). From 2001 to 2009, survival dropped at a lower annual rate for all treatments, although non-protected trees died faster (13.5% for the whole period of 8 years) than pines in mesh (8.6%) or tube-shelters (7.7%). The pattern of interactions between the studied factors remained constant during these 8 years (data not shown). From 2009 and beyond, survival stabilized, and no significant mortality occurred between this year and 2016 for any treatment (Figure 2A). As a result, 20 years after planting, survival of mesh-protected pines was maximum (57.5 ± 2.3%) and almost double that of solid-walled tree shelters (29.5 ± 2.1%), leaving survival of non-sheltered seedlings within an intermediate range (46.0 ± 2.3%, Figure 2A). However, these figures must be assessed considering the aforementioned tree shelter × competition suppression interaction (Table 1). This significant effect was mainly due to two interacting responses: on one hand, manually weeded and non-protected pines survival (29.3 ± 4.1%) was lower after 20 years than remaining non-protected pines (average 52.0 ± 4.5%, Supplementary Figure 2, January 2016); on the other hand, non-weeded and mesh protected pines survival after 20 years (40.6 ± 4.4%) was lower than remaining trees in mesh, which in fact had the highest levels of survival among the treatments tested (average 62.8 ± 4.3%, Supplementary Figure 2, January 2016).
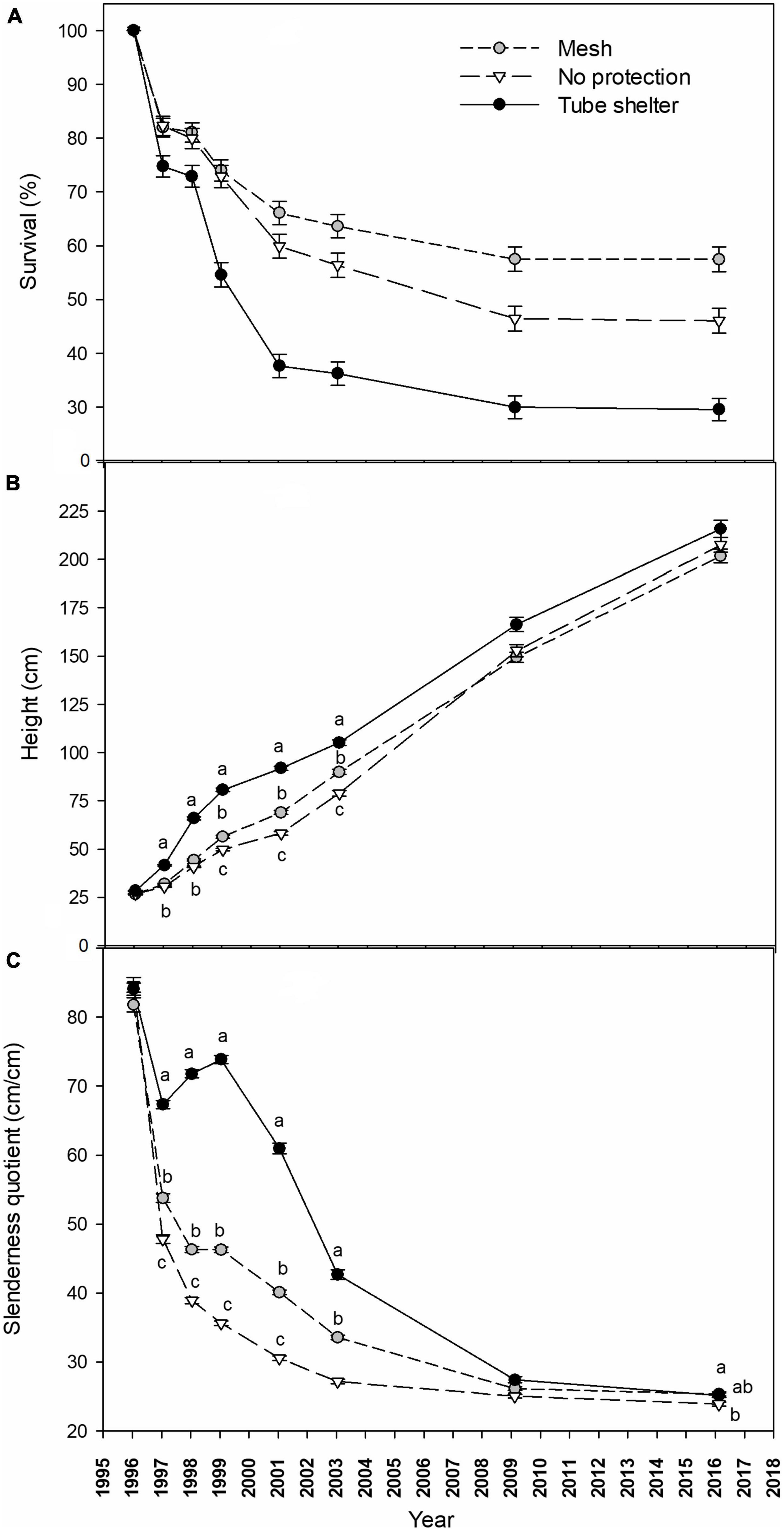
Figure 2. Survival [(A) %], height [(B) cm] and slenderness quotient [(C) cm/cm] of a Pinus halepensis plantation during a 20-year period as affected by tree shelter treatments. Depicted values are mean ± SE. Within a date, values with different lowercase letters differ significantly among tree shelter treatments according to Fisher’s protected least significant difference test, α = 0.05. In sub-figure (A) interactions with competition suppression factor preclude showing differences by tree shelters (see Supplementary Figure 2 for details).
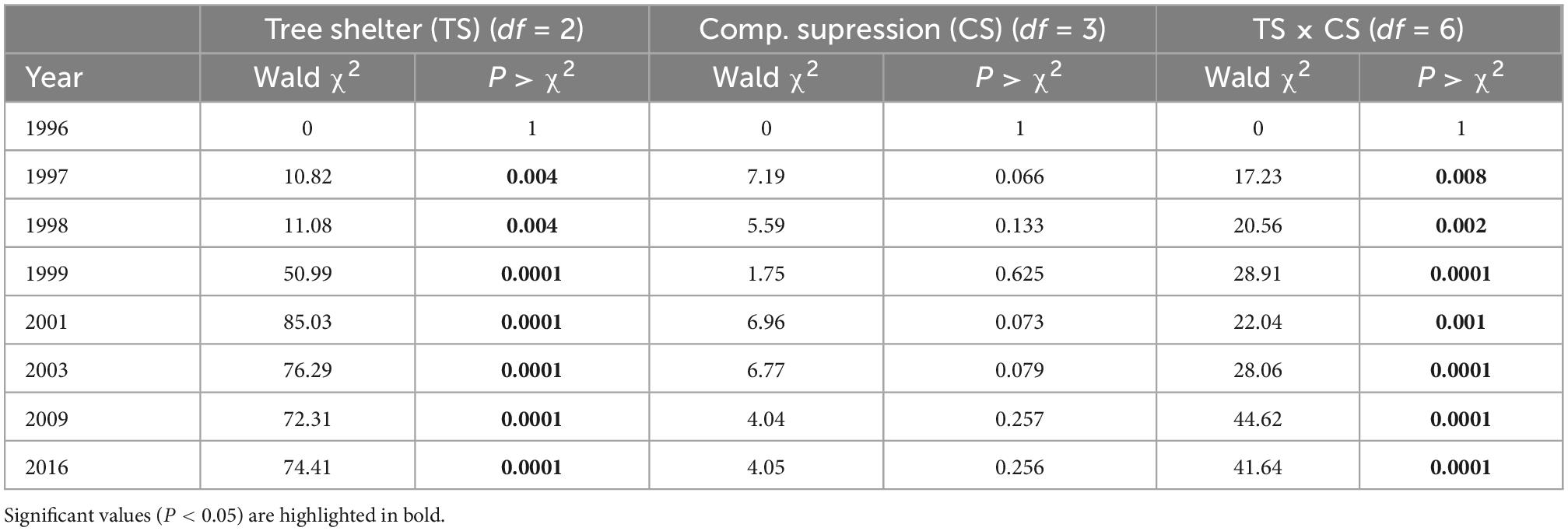
Table 1. Contrasts of the generalized linear model effects for survival along 20 years of Pinus halepensis planted in a factorial experiment with tree protection (no shelter, mesh, and solid tube) and four competition suppression (control, manual weeding, polyethylene mulch, and fiber mat) levels.
3.3. Growth and morphology along 20 years following plantation
Height growth of pines along the 20 years period was not affected by competition suppression or by interactions between both factors (with the exception of an interaction 6 years after planting, Table 2). However, shelter type affected height beginning the first year after planting: in January 1997, height of trees in tube-shelters (41.6 ± 0.5 cm) was significantly higher than that of trees in mesh ones (31.9 ± 0.3 cm) or non-protected (30.5 ± 0.3 cm, Figure 2B and Table 2). During the second year of planting (1997) seedlings in tube-shelter grew at a much faster rate in height (24.2 cm⋅year–1) than pines in mesh (12.4 cm⋅year–1) or non-protected (10.4 cm⋅year–1). As a result, in January 1998 pines in tubes reached 65.9 ± 0.8 cm height on average, with 63% of pines growing out of the height of the shelter (60 cm), and differences with other two levels of protection being more significant (see F increase between 1997 and 1998 in Table 2). During 1998, height growth rate of sheltered pines in tubes dropped to 14.8 cm⋅year–1, but was still higher than that of mesh (12.1 cm) or non-protected (8.9 cm) seedlings. In January 1999, mean height of meshed pines was first time significantly higher (6.6 cm) than that of non-protected ones, although both were still significantly lower than the height of pines in tubes (80.7 ± 1.0 cm, Figure 2B). From 1999 to 2003 height growth rate in solid wall tubes dropped to minimum values (6.1 cm year–1) compared to non-protected (7.3 cm year–1) seedlings or those protected with mesh-shelters (8.4 cm⋅year–1), although significant differences in height among the three treatments still persisted. During the next 6 years (2003–2009) the trend in height growth of non-protected seedlings reversed, and there were no longer differences among protection (Table 2). In 2016, 20 years after planting, height of pines in tube-shelters (215.7 ± 4.5 cm) were 13.9 cm taller than those in mesh (Figure 2B), but these differences were even less significant (Table 2) than in 2009. Interaction between main factors for height only occurred in 2001 (Table 2). At this time, non-protected and manually weeded pines were the shortest among the other treatments of this factor level, while this did not occur for the rest of non-protected × competition suppression treatments (data not shown). The same interactive pattern, although only marginally significant, occurred from 1999 to 2009 (Table 2).
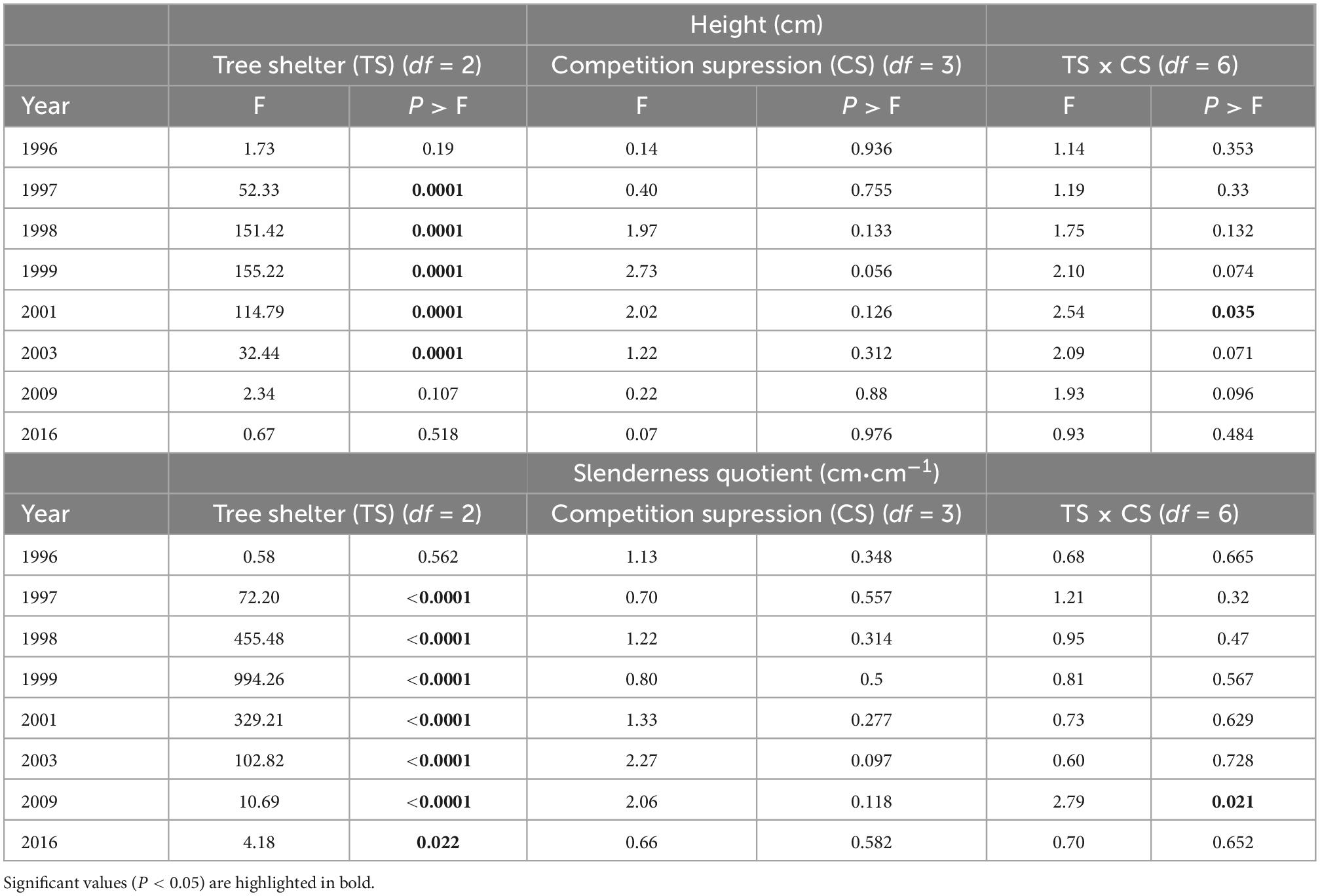
Table 2. Results from ANOVA (Snedecor F and associated probability P) test for height and sturdiness quotient along 20 years of P. halepensis planted in a factorial experiment with tree protection (no shelter, mesh, and tube-shelter) and four competition suppression (control, manual weeding, polyethylene film, and coconut mulches) levels.
As per height, basal stem diameter growth of pines along the 20 years period was not affected by competition suppression. In addition, interactions between main factors were not present in the studied years (data not shown). Only shelter type affected diameter growth pattern during the first 5 years (Supplementary Table 1). Interestingly, differences were significant just 2 months after planting (January 1996), with diameter of pine stems in tube-shelters being significantly greater than those of non-protected (Supplementary Table 1). One year later, in January 1997, trends changed, and non-protected seedlings had the largest diameter, followed by trees in shelters and in meshes, with basal diameters marginally (but significantly) smaller. This trend of non-protected pines having the largest diameter continued from January 1998 and beyond, although from this year trees in meshes (9.7 ± 0.11 mm) placed in a midway between un-protected (10.9 ± 0.16 mm) and trees in tube-shelters (9.2 ± 0.10 mm) with differences being significant for diameters of pines in meshes or tube-shelters and non-protected. During 1999 and 2000 this tendency continued and, in January 2001, basal stem diameter of pines in tube-shelters was still significantly smaller than non-protected trees, with pines in mesh (17.6 ± 0.30 mm), being not significantly different from any of them. After 2001, basal stem diameter was similar, and differences among treatments were no longer significant. Interestingly, after 14 years (February 2009), trends changed, as trees in tube-shelters had the greatest stem diameter (Supplementary Table 1), which continued for the next 7 years until February 2016.
Similar to height, slenderness quotient of pines along the 20 years period was not affected by competition suppression nor interactions between factors (except for an interaction 14 years after planting, Table 2). However, the effect of shelter type persisted across the study period except for the first 2 months after planting (January 1996). During 1996, all treatments experienced a sound reduction of slenderness, with this reduction being much more intense for non-protected seedlings, whose rate (36.9 cm⋅cm–1⋅year–1) more than doubled that of seedlings in tubes (16.8 cm⋅cm–1⋅year–1, Figure 2C). During 1997, slenderness quotient of pines in mesh and non-protected continued dropping but this trend reversed in pines in tube-shelters, as they showed an increasing pattern (Figure 2C). In 1998 the increasing trend of slenderness of pines in tube-shelters continued, leading to maximum values of 73.8 ± 0.6 cm⋅cm–1 in January 1999. During the same year, pines in meshes did not vary in their sturdiness quotient, while non-protected pines followed a continuous reduction at a diminishing rate that persisted over the whole studied period (Figure 2C). During 1999 and 2000, slenderness of trees in tube-shelters experienced a new dramatic change from increasing to a rapid decreasing rate of 6.4 cm⋅cm–1⋅year–1. This rate even accelerated for the following 2 years (2001 and 2002) to 9.1 cm⋅cm–1⋅year–1, while trees in meshes or non-protected showed a less intense reduction (3.3 or 1.7 cm⋅cm–1⋅year–1, respectively). From January 2003 to the end of studied period, slenderness values converged for all treatments. As a result, differences between pines in tube-shelters and for non-protected trees changed from 15.5 cm⋅cm–1⋅in 2003 to 1.3 cm⋅cm–1 in 2016 (Figure 2C). These small differences were still significant (Table 2).
Stem volume was not affected by competition suppression nor the interaction between this and shelter type factors. Only the shelter type main factor was significant along the first 2 years out of the whole 20 years period (see Supplementary Table 1). As early as 2 months after planting (January 1996), stem volume of trees in tube-shelters (0.92 ± 0.02 cm3) was significantly larger than that of non-protected pines (0.76 ± 0.02 cm3), with meshed pines being intermediate. During the next 2 years after planting (1996 and 1997), pines in tubes shelters continued to exhibit maximum volume values as compared to non-protected plants or seedlings in mesh. After January 1998, differences in stem volume among types of shelters were not significantly different, with a similar pattern among treatments persisting. At the end of the studied period (2016), trees in tube-shelters still accounted for a major bole volume compared to that of non-protected and meshed pines although differences were not significant (Supplementary Table 1). Area of the projected canopy in 2016 (3.3 ± 0.07 m2 in average) was not significantly different among treatments (ANOVA data not shown).
4. Discussion
4.1. Soil water content along the soil profile
Overall soil water dynamics and spatial distribution during the first 3 years showed a known pattern for semiarid areas: cycles of dry and wet periods that follow rainfall events; and a strong gradient of water increase by depth. This has also been observed in similar studies under Mediterranean arid to semiarid conditions (Padilla and Pugnaire, 2007; Jiménez et al., 2017). In our study, absolute values were lower, probably due to the reduced rainfall logged in our experimental area. As a result, soil humidity remained under the wilting point for long periods, especially from 0 to 30 cm. From 30 to 60 cm, soil water was below the wilting point mostly in summer, with some water still present even in this season. Although we did not measure VWC below 60 cm, we expect higher values beyond this depth, according to the typical gradient shown in other studies (Pinto et al., 2016).
The effects of weeding and mulching on soil profile water content during the first 3 years was weak: only the polyethylene sheet increased soil water content and only at shallow (0–15 cm) horizons, with no effect of weeding. Despite literature showing positive effects of weeding on soil water content (Cuesta et al., 2010a; Ammer et al., 2011 and references therein), these improvements have been related to competition suppression that reduces transpiration of spontaneous vegetation (Cuesta et al., 2010b; Pinto et al., 2012). The particularly hard conditions of arid areas as per our study precludes seed bank recovery following soil preparation disturbance (Coello et al., 2018a). In our study, spontaneous vegetation was nearly absent in control (not weeded) spots around the planted tree during the first 3 years (personal observation). Thus, no significant effect of weeding should be expected. Apart from effects on vegetation emergence, mulching exerts additional effects on soil water dynamics on the first layers of the soil profile (Jiménez et al., 2017). In our study, the positive effect of polyethylene sheets at 0–15 cm could be explained by a reduction in water evaporation that occurs under polyethylene coverage as a result of the large amount of solar radiation reflected by the sheets, as evidenced by results from Barajas-Guzmán et al. (2006) with this material. Other studies conducted under similar arid conditions of the Mediterranean have also reported positive effect of rock fragments as a mulching system on soil water content above 20 cm (Jiménez et al., 2017). In our study, we did not find any effect of fiber mat mulch at any depth. Lack of beneficial impact on solar radiation reflection and on spontaneous vegetation inhibition could explain the absence of fiber mat effects on soil water content as compared to polyethylene or weeding treatments. Other factors could explain the observed differences between materials: the fiber mat does not preclude evaporation as much as a plastic layer, and the size of the polyethylene sheet (6,724 cm2) was almost 30% larger than the fiber mat (5,250 cm2). Contrary to our study, other trials under Mediterranean arid to semiarid conditions found significant and positive effects of straw mulch on water content at 20 cm and beyond (Jiménez et al., 2017) and of different mulching techniques on water content at 20–40 cm (Coello et al., 2018a). The effect of mulching on soil water dynamics apparently follows a complex pattern with interacting factors such as intensity of rainfall events, total precipitation, mulch size, type of material with regard to evaporation-infiltration dynamics, as well as abundance and type of weeds (herbaceous vs. woody) playing key roles (Valdecantos et al., 2014; Coello et al., 2018b; Luna et al., 2018).
4.2. Survival response: Effect of shelters and interactions with soil water dynamics and competition suppression
Overall, tree shelter type had the most significant effect on survival and growth of P. halepensis under our experimental conditions. However, some interactions occurred between factors along the 20-year study period. Survival was maximized for trees in mesh, followed by unprotected pines and was lowest for tubes. High mortality of Aleppo pines protected with tube-shelters could be attributed to the shade intolerance of this species. Additionally, stem volume of pines in tube-shelters was significantly higher during the first 2 years, suggesting preferential allocation to shoot growth at the expense of root growth (but see next Section about potential effect of temperature on aboveground growth). In past studies, root growth of Aleppo pine was reduced under the effect of tube-shelters with identical or similar light transmission than the tested tube in our experiment, while this effect was null for shade tolerant species (Puértolas et al., 2010; Vázquez de Castro et al., 2014; Oliet et al., 2021). The reduction of root growth rate is lethal in plantations under arid conditions, as they cannot reach soil depths where water is still available. In a similar study with Aleppo pine, survival during the first summer after planting was clearly reduced if rooting depth did not reach 30 cm (Padilla et al., 2007). Our study shows that during the first years of establishment, water in the soil profile is mostly available from 30 to 60 cm, but rarely available from 15 to 30 cm and very infrequently in the shallowest 0 to 15 cm horizon (Figure 1). Surviving pines must therefore have reached root depths below 30 cm before the onset of the first summer drought. Interestingly, the intense reductions in the third- and fourth-year survival of pines in tube-shelters after the strong drought of the second year (Figure 2A) supports the hypothesis that this response was probably caused by a reduction in root growth of pines in tubes. A similar reduction in survival was observed in a simultaneous experiment conducted at the same area testing seedling quality effects (Oliet et al., 2009), and other studies have shown survival reductions of shade intolerant species in tree shelters under Mediterranean conditions (Oliet et al., 2003; Padilla et al., 2011). Despite these trends are likely to be site or year-specific (e.g., Barberá et al., 2005), under the current context of aridification and extreme climatic events, which emphasizes the necessity and utility of these long-term works to detect effects of subsequent intense droughts. Although temperatures within shelters were assumed to be higher than ambient (Bergez and Dupraz, 2009; Puértolas et al., 2010), we do not believe that this affected survival of P. halepensis in tube-shelters in our study, due to the high thermal-tolerance of this species to high temperatures (Methy et al., 1997).
The effect of competition suppression on survival was very weak, and only occurred by an interaction with shelter type. Two different interactive effects were found, with reduced survival for non-protected and manually weeded pines and for mesh-protected non-weeded pines (Table 1 and Supplementary Figure 2). We were unable to develop a solid explanation for these results, although we can hypothesize that manual weeding with a hoe could have wounded trees at higher rate if they were non-protected. And mesh could play a protective role against this effect, to the extent of being major interactive cause of differences between meshes and non-protection in survival; in fact, mesh and un-protected pines did not differ in survival for the rest of the treatment combinations (Supplementary Figure 2), what excludes a browsing damage as a potential cause of mortality in non-protected seedlings. Apart from this effect, no significant influence of competition suppression on survival was found. This could be expected, because differences in soil moisture among treatments was very small during the first 3 years after planting. Additionally, they only occurred at 0–15 cm, where water availability is mostly under the wilting point and no root abundance of pines is expected. Studies under arid and semiarid Mediterranean conditions are not very conclusive regarding the effect of mulching on survival, with some studies showing positive effects after 9 years (Jiménez et al., 2013) or weak or null effects under shorter durations (Chaar et al., 2008; Soliveres, 2012; Jiménez et al., 2017; Coello et al., 2018a). Weeding was shown to affect soil water content and survival of this species where herbaceous vegetation is abundant (Cuesta et al., 2010b), but this was not the case in our study (see previous section). Therefore, our study suggests that the use of mulching or weeding in arid areas is an inefficient investment.
Although the mortality rate of plantations is usually highest during the first year in dry ecosystems (Close et al., 2005; Padilla et al., 2009), our study confirms that monitoring survival for more than the first summer is key to detecting cumulative effects that can improve discrimination of some planting treatments: in our case, mortality rate was still much higher for tube-sheltered pines in the fourth year of planting. As a result, survival of sheltered pines was significantly depleted, suggesting that the use of this technology for protecting Aleppo pine is not recommended. Interestingly, another study in Mediterranean semiarid area shows an additional drop in survival after the fourth year of planting for control trees as compared to mulched seedlings (Jiménez et al., 2013). Survival in meshes, however, was identical of that of unprotected pines until the sixth year of planting, and differences diverged significantly thereafter. Additionally, long-term monitoring of survival in this study confirms that mortality is only stabilized after more than 15 years, although differences between protection types remained relatively consistent since the fifth to sixth year. This response underlines the necessity of monitoring restoration to detect potential long-term signals of treatments such as tree-shelters.
4.3. Growth response: Shelter protection and competition suppression
Long-term period analyses help to reveal the dynamics of tree morphology, as well as whether and when differences among tested treatments vanish. Across the 20-year study period we confirmed that, similar to survival, the main effects on growth are determined by vertical protection treatments. In general, solid wall tube-shelters stimulate height and reduce basal stem diameter growth during the first years after planting, which results in dramatic changes in tree morphology (measured as slenderness quotient) during the first years after planting. Changes in slenderness are influenced by the conditions within shelters. Maximum differences among treatments in slenderness quotient were found 3 years after planting (Figure 2C), when most of the pines began to exceed the shelter height. A similar response was found in an experiment using the same tube-shelters with Acacia salicina (Oliet et al., 2016). Tree slenderness can serve as a measure of morphological balance between allocation to primary vs. secondary growth, with those trees with low slenderness values being the most sensitive to stem breakage (Ancelin et al., 2004). Although it is difficult to estimate the slenderness quotient that optimizes stability to young trees, trees growing without protection are the most resistant to bending, and the slenderness quotient values of 73.8 ± 0.6 cm⋅cm–1 reached within tubes 3 years after planting may prevent the ability to remove shelters without causing damage to the seedlings. After the third year of maximum differences, allometry began to converge with non-protected pines, although this convergence took different times among variables: five and 8–13 years for basal stem diameter and height, respectively, with slenderness values being very similar from 14 years of planting in absolute terms, despite differences in slenderness kept statistically significant after 20 years (Figure 2). This result, along with lack of differences in crown projected area among treatments, suggests that after 20 years, tree morphology was similar irrespective of vertical protection applied at planting. Protected trees will attain maximum stability when they reach slenderness convergence with unprotected pines. The period to convergence is species and site specific, with trees requiring a long period to converge on sites as per our study where plants grow slowly (Potter, 1991; Barberá et al., 2005; Jacobs, 2011; Mechergui et al., 2019). Irrigation planting experiments with tube-shelters suggest an earlier slenderness convergence under watering conditions in parallel to faster growth (Oliet et al., 2005, 2016). In temperate forests with fast growing deciduous species, convergence takes 3 to 4 years even within 1.2-m tree shelters (Johansson, 2004).
Tree morphology and allocation dynamics of protected seedlings along time is a complex process that results from the interaction of several factors. Those interactions can be disentangled with an approach based on long-term studies. On one side, light environment within the shelter stimulates a shade avoidance syndrome that includes the promotion of a stem elongation response. This response is boosted mainly by a reduction in red:far red (R:FR) ratio (Smith and Whitelam, 1997) but recent reviews suggests that a reduction of light intensity itself also fosters a similar response (Ballaré and Pierik, 2017). In our study, the brown color of the tree shelter wall transmitted a neutral light, with an R:FR ratio close to open canopy (pers. observ). Therefore, the stimulus for height growth of protected pines in our study may come from the 55% reduction of light radiation. This could explain, among other reasons, why height growth rates of pines in shelters decreased after the third year, when they had outgrown of the top of the shelter. Similar responses were found in a Mediterranean oak (McCreary and Tecklin, 2001). Light environment could also explain the observed reduction in basal stem diameter growth within shelters, as this allocation effect is also part of the shade avoidance syndrome (Ballaré and Pierik, 2017). The reduction on diameter growth in tree-shelters has been consistently reported in many experiments (Navarro-Cerrillo et al., 2005; Devine and Harrington, 2008; Mechergui et al., 2019), some of them with P. halepensis (Vázquez de Castro et al., 2014; Oliet et al., 2021). However, basal stem diameter of tube-sheltered seedlings was not significantly different from those pines protected with meshes, whose foliage was under open light conditions most of the time. Thus, the light environment cannot be considered the main cause of reduced diameter growth for pines in tubes. Solid tubes and meshes would impair the dynamic pressure of the strong winds of the area during first years that would promote the classic tapering stem form (Coutand et al., 2008). Once pines emerged from tubes and meshes (from third year and beyond), crowns widen and wind pressure increases, promoting the thickening of basal stem. In addition, height of pines in mesh is significantly larger than that of non-protected pines during the first 8–13 years (Figure 2B), suggesting that there is a stimulus for growth not explained by light reduction but by an allocative response to the restriction on stem movement, as suggested by other results (Dupraz, 1997; McCreary and Tecklin, 2001; Thyroff et al., 2022). As response in tubes and meshes is similar in stem diameter growth, we hypothesize that this mechanic influence is more important than the photomorphogenic effect of light attenuation for the diameter growth depletion. Additionally, physical restrictions to branch growth both within solid tube-shelter and mesh could explain the reduction in stem thickness, as it has been suggested in other studies (Devine and Harrington, 2008). Other factors that help to differentiate the microenvironments around trees in meshes or solid tubes, such as radiation directionality (direct with sunflecks vs. diffuse, respectively) or wind exposure (Oliet et al., 2019), could also affect dynamics of growth but more research is needed to assess these effects.
The trophic effect of the shelter is not as clear as the allocative one. Based on the results of stem volume, which is strongly correlated with shoot biomass (South et al., 2001), shoot growth of P. halepensis within tube-shelters was significantly higher, occurring as soon as 2 months after planting, and this effect persisted for two more years despite the shade intolerance of Aleppo pine. This striking short-term effect was also observed in a similar study with A. salicina (Oliet et al., 2005) and can be associated with the higher temperature inside this Tubex ® type of shelter (Puértolas et al., 2010), which acted to extend the growing season. After the second year, most pines grew out of the shelter, avoiding the temperature growth promotion effect. Additionally, high mortality rates due to reduction of root exploitation capacity depicts a highly stressful scenario where shoot growth of surviving pines would clearly be reduced.
In this study, the effect of mulching or manual weeding on growth was mostly null. Only an interactive effect between both factors shows that non-protected, manually weeded pines grew less in height than with the other three types of weed suppression treatments during some years of the study. This result is in accordance with survival, revealing a poor vegetative condition for this treatment. Other studies in semiarid and dry Mediterranean conditions show significant effect of mulch on growth, but this is explained by a variation of soil VWC that was not found in our study (Pardos et al., 2015; Jiménez et al., 2017).
5. Conclusion
We demonstrated the importance of long-term analyses of ecotechnologies for forest restoration of arid Mediterranean sites. In our study with Pinus halepensis, mortality stabilized after 9–15 years, although differences among protection types remained relatively consistent after 5 or 6 years. We continued to detect a strong signal of protection type on survival after 20 years with ranking in order of mesh-shelters, non-sheltered, and tube-shelters. Growth differences were primarily observed during the establishment period, with shading in tube-shelters stimulating height growth in shade-intolerant P. halepensis, and reduction of stem movement from wind likely reducing diameter growth. However, growth differences subsided after 3 years once trees emerged from shelters and this effect would likely occur earlier in more mesic regions with faster tree growth. Operationally, our results showed no benefit from competition suppression treatments and a detrimental effect of the use of tube-shelters. Thus, mesh-shelters alone are recommended as the most effective protection method for restoration of P. halepensis under arid site conditions, especially if browsing damages are expected. We suspect that these results may be applicable to other forest tree species for restoration on similar arid Mediterranean sites, although further research should help to confirm this expectation.
Data availability statement
The raw data supporting the conclusions of this article will be made available by the authors, without undue reservation.
Author contributions
JO: conceptualization, data curation, formal analysis, funding acquisition, investigation, methodology, project administration, supervision, validation, visualization, and writing – original draft and review and editing. RP: conceptualization, investigation, methodology, project administration, supervision, validation, and writing – review and editing. FA: investigation, methodology, and supervision. DJ: investigation, supervision, validation, visualization, and writing – review and editing. All authors contributed to the article and approved the submitted version.
Funding
We gratefully acknowledge the financial support of the National Institute for Agriculture and Food Technology and Research (INIA, Spanish Department of Science and Innovation) through projects (SC-94111 and OT98-001), the Technical University of Madrid Research Program, and the Comunidad de Madrid Research Program through REMEDINAL-P2018/EMT-4338 Project.
Acknowledgments
We thank technical support of Almudena Martin, José Rodríguez, Pepa Aroca, and Milagros Serrano. The comments of two reviewers substantially improved this manuscript.
Conflict of interest
RP was currently employed by Kaudal.
The remaining authors declare that the research was conducted in the absence of any commercial or financial relationships that could be construed as a potential conflict of interest.
Publisher’s note
All claims expressed in this article are solely those of the authors and do not necessarily represent those of their affiliated organizations, or those of the publisher, the editors and the reviewers. Any product that may be evaluated in this article, or claim that may be made by its manufacturer, is not guaranteed or endorsed by the publisher.
Supplementary material
The Supplementary Material for this article can be found online at: https://www.frontiersin.org/articles/10.3389/ffgc.2022.1092703/full#supplementary-material
References
Abe, T. (2022). Effects of treeshelter on seedling performance: A meta-analysis. J. For. Res. 27, 171–181. doi: 10.1080/13416979.2021.1992700
Alía, R., García del Barrio, J. M., Iglesias, S., Mancha, J. A., de Miguel y del Ángel, J., Nicolás, J. L., et al. (2009). Regiones de procedencia de especies forestales españolas. Madrid: Organismo Autónomo Parques Nacionales, 363.
Ammer, C., Balandier, P., Betsen, N. S., Coll, L., and Löf, M. (2011). Forest vegetation management under debate: An introduction. Eur. J. For. Res. 130, 1–5.
Ancelin, P., Courbaud, B., and Fourcaud, T. (2004). Development of an individual tree-based mechanical model to predict wind damage within forest stands. For. Ecol. Manag. 203, 101–121. doi: 10.1016/j.foreco.2004.07.067
Andrews, G. (2002). Mulch a growth control mechanism. Comb. Proc. Int. Plant Propag. Soc. 52, 63–71.
Ballaré, C., and Pierik, R. (2017). The shade-avoidance syndrome: Multiple signals and ecological consequences. Plant Cell Environ. 40, 2530–2543. doi: 10.1111/pce.12914
Barajas-Guzmán, M., Campo, J., and Barradas, V. L. (2006). Soil water, nutrient availability and sapling survival under organic and polyethylene mulch in seasonally dry tropical forests. Plant Soil 287, 347–357. doi: 10.1007/s11104-006-9082-7
Barberá, G. G., Martinez-Fernandez, F., Alvarez-Rogel, J., Albaladejo, J., and Castillo, V. (2005). Short- and intermediate-term effects of site and plant preparation techniques on reforestation of a Mediterranean semiarid ecosystem with Pinus halepensis Mill. New For. 29, 177–198. doi: 10.1007/s11056-005-0248-6
Bautista, S., and Alloza, J. A. (2009). “Evaluation of forest restoration projects,” in Land restoration to combat desertification. Innovative approaches, quality control and project evaluation, eds S. Bautista, V. Aronson, and V. Vallejo (Valencia: Fundación Centro de Estudios Ambientales del Mediterráneo), 47–72.
Bergez, J. E., and Dupraz, Z. C. (2009). Radiation and thermal microclimate in tree shelter. Agric. For. Meteorol. 149, 179–186. doi: 10.1016/j.agrformet.2008.08.003
Carminati, A., Benard, P., Ahmed, M. A., and Zarebanadkouki, M. (2017). Liquid bridges at the root-soil interface. Plant Soil 417, 1–15. doi: 10.1007/s11104-017-3227-8
Castro, J., Leverkus, A. B., and Fuster, F. (2015). A new device to foster oak forest restoration via seed sowing. New For. 46, 919–929. doi: 10.1007/s11056-015-9478-4
Castro, J., Morales-Rueda, F., Navarro, F. B., Löf, M., Vacchiano, G., and Alcaraz-Segura, D. (2021). Precision restoration: A necessary approach to foster forest recovery in the 21st century. Restor. Ecol. 29:e13421. doi: 10.1111/rec.13421
Ceacero, C. J., Navarro-Cerrillo, R. M., Díazhernández, J. L., and Del Campo, A. (2014). Is tree shelter protection an effective complement to weed competition management in improving the morphophysiological response of holm oak planted seedlings? iForest 7, 289–299. doi: 10.3832/ifor1126-007
Chaar, H., Mechergui, T., Khouaja, A., and Abid, H. (2008). Effects of treeshelters and polyethylene mulch sheets on survival and growth of cork oak (Quercus suber L.) seedlings planted in northwestern Tunisia. For. Ecol. Manag. 256, 722–731. doi: 10.1016/j.foreco.2008.05.027
Chirino, E., Vilagrosa, A., Cortina, J., Valdecantos, A., Fuentes, D., Trubat, R., et al. (2009). “Ecological restoration in degraded drylands: The need to improve the seedling quality and site conditions in the field,” in Forest management, ed. S. Grossberg (New York, NY: Nova Publisher), 85–158.
Close, D. C., Beadle, C. L., and Brown, P. H. (2005). The physiological basis of containerised tree seedling ‘transplant shock’: A review. Aust. For. 68, 112–120. doi: 10.1080/00049158.2005.10674954
Close, D. C., Beadle, C. L., Holz, G. K., and Brown, P. H. (2002). Effect of shadecloth tree shelters on cold-induced photoinhibition, foliar anthocyanin and growth of Eucalyptus globulus and E. nitens seedlings during establishment. Aust. J. Bot. 50, 15–20. doi: 10.1071/BT01038
Close, D. C., Ruthrof, K. X., Turner, S., Rokich, D. P., and Dixon, K. W. (2009). Ecophysiology of species with distinct leaf morphologies: Effects of plastic and shadecloth tree guards. Restor. Ecol. 17, 33–41. doi: 10.1111/j.1526-100X.2007.00330.x
Coello, J., Ameztegui, A., Piqué, M., Rovira, P., and Fuentes, C. (2018a). Innovative soil conditioners and groundcovers for forest restoration in semiarid conditions in northeast Spain. Ecol. Eng. 118, 52–65. doi: 10.1016/j.ecoleng.2018.04.015
Coello, J., Piqué, M., Rovira, P., Fuentes, C., and Ameztegui, A. (2018b). Combining innovative mulches and soil conditioners in mountain afforestation with ash (Fraxinus excelsior L.) in the Pyrenees (NE Spain). For. Syst. 27:e017. doi: 10.5424/fs/2018273-13540
Cortina, J., Amat, B., Castillo, V., Fuentes, D., Maestre, F. T., Padilla, F. M., et al. (2011). The restoration of vegetation cover in the semi-arid Iberian southeast. J. Arid Environ. 75, 1377–1384. doi: 10.1016/j.jaridenv.2011.08.003
Coutand, C., Dupraz, C., Jaouen, G., Ploquin, S., and Adam, B. (2008). Mechanical stimuli regulates the allocation of biomass in trees: Demonstration with young Prunus avium trees. Ann. Bot. 101, 1421–1432. doi: 10.1093/aob/mcn054
Cuesta, B., Villar-Salvador, P., Puértolas, J., Rey Benayas, J. M., and Michalet, R. (2010a). Facilitation of Quercus ilex in Mediterranean shrubland is explained by both direct and indirect interactions mediated by herbs. J. Ecol. 98, 687–696. doi: 10.1111/j.1365-2745.2010.01655.x
Cuesta, B., Villar-Salvador, P., Puértolas, J., Jacobs, D. F., and Rey Benayas, J. M. (2010b). Why do large, nitrogen rich seedlings better resist stressful transplanting conditions? A physiological analysis in two functionally contrasting Mediterranean forest species. For. Ecol. Manag. 260, 71–78. doi: 10.1016/j.foreco.2010.04.002
Del Campo, A., Navarro, R. M., Aguililla, A., and Gonzalez, E. (2006). Effect of tree shelter design on water condensation and run-off and its potential benefit for reforestation establishment in semiarid climates. For. Ecol. Manag. 235, 107–115. doi: 10.1016/j.foreco.2006.08.003
Delgado-Baquerizo, M., Maestre, F., Gallardo, A., Bowker, M., Wallenstein, M., Quero, J., et al. (2013). Decoupling of soil nutrient cycles as a function of aridity in global drylands. Nature 502, 672–676. doi: 10.1038/nature12670
Devine, W. D., and Harrington, T. B. (2011). Aboveground growth interactions of paired conifer seedlings in close proximity. New For. 41, 163–178. doi: 10.1007/s11056-010-9218-8
Devine, W., and Harrington, C. A. (2008). Influence of four tree shelter types on microclimate and seedling performance of Oregon white oak and Western Redcedar. General Technical Report PNW-GTR 576. Portland, OR: Pacific NorthWest Research Station. doi: 10.2737/PNW-RP-576
Dupraz, C. (1997). Les protections de plants à effet de serre. Première partie: Ce qu’en pensent les arbres. Rev. For. Fr. 49, 417–432.
González-Tokman, D. M., Barradas, V. L., Boege, K., Domínguez, C. A., del-Val, E., Saucedo, E., et al. (2017). Performance of 11 tree species under different management treatments in restoration plantings in a tropical dry forest. Restor. Ecol. 26, 642–649. doi: 10.1111/rec.12617
Haywood, J. D. (1999). Durability of selected mulches, their ability to control weeds, and influence growth of loblolly pine seedlings. New For. 18, 263–276. doi: 10.1023/A:1006699910149
Hytönen, J., and Jylhä, P. (2010). Long-term response of weed control intensity on scots pine on survival, growth and nutrition on former arable land. Eur. J. For. Res. 130, 91–98. doi: 10.1007/s10342-010-0371-6
IPCC (2014). Climate change 2014: Impacts, adaptation, and vulnerability. Part A: Global and sectoral aspects. Page contribution of working group II to the fifth assessment report of the intergovernmental panel on climate change. New York, NY: Cambridge University Press.
Jacobs, D. F. (2011). Reforestation of a salvage-logged high-elevation clearcut: Engelman spruce seedling response to tree shelters after 11 growing seasons. West. J. Appl. For. 26, 53–56. doi: 10.1093/wjaf/26.2.53
Jacobs, D. F., Oliet, J. A., Aronson, J., Bolte, A., Bullock, J. M., Donoso, P. J., et al. (2015). Restoring forests: What constitutes success in the twenty first century? New For. 46, 601–614. doi: 10.1007/s11056-015-9513-5
Jiménez, M. N., Fernández-Ondoño, E., Ripoll, M. A., Castro-Rodríguez, J., Huntsinger, L., and Bruno Navarro, F. (2013). Stones and organic mulches improve the Quercus ilex L. afforestation success under Mediterranean climatic conditions. Land Degrad. Dev. 27, 357–365. doi: 10.1002/ldr.2250
Jiménez, M. N., Pinto, J. R., Ripoll, M. A., Sánchez-Miranda, A., and Navarro, F. B. (2017). Impact of straw and rock-fragment mulches on soil moisture and early growth of holm oaks in a semiarid area. Catena 152, 198–206. doi: 10.1016/j.catena.2017.01.021
Johansson, T. (2004). Changes in stem taper for birch plants growing in tree shelters. New For. 27, 13–24. doi: 10.1023/A:1025021926765
Karger, D. N., Conrad, O., Böhner, J., Kawohl, T., Kreft, H., Soria-Auza, R. W., et al. (2017). Climatologies at high resolution for the Earth land surface areas. Sci. Data 4:170122. doi: 10.1038/sdata.2017.122
Löf, M., Madsen, P., Metslaid, M., Witzell, J., and Jacobs, D. F. (2019). Restoring forests: Regeneration and ecosystem function for the future. New For. 50, 139–151. doi: 10.1007/s11056-019-09713-0
Luna, L., Vignozzi, N., Miralles, I., and Solé-Benet, A. (2018). Organic amendments and mulches modify soil porosity and infiltration in semiarid mine soils. Land Degrad. Dev. 29, 1019–1030.
Maestre, F. T., and Cortina, J. (2004). Are Pinus halepensis plantations useful as a restoration tool in semiarid Mediterranean areas? For. Ecol. Manag. 198, 303–317. doi: 10.1002/ldr.2830
MAPA (1989). Mapa de suelos. Escala 1:100.000. Carboneras (1046) ICONA. Granada: Universidad de Granada.
Mayor, A. G., Bautista, S., and Bellot, J. (2011). Scale-dependent variation in runoff and sediment yield in a semiarid Mediterranean catchment. J. Hydrol. 397, 128–135. doi: 10.1016/j.jhydrol.2010.11.039
McCreary, D. D., and Tecklin, J. (2001). The effects of different sizes of tree shelters on blue oak (Quercus douglasii) growth. West. J. Appl. For. 16, 153–158. doi: 10.1093/wjaf/16.4.153
Mechergui, T., Pardos, M., and Jacobs, D. F. (2019). Influence of mulching and tree shelters on 4-year survival and growth of zeen oak (Quercus canariensis) seedlings. J. For. Res. 30, 129–141. doi: 10.1007/s11676-018-0606-9
Mechergui, T., Pardos, M., Boussaidi, N., Hasnaoui, B., and Jacobs, D. F. (2013). Development of cork oak (Quercus suber L.) seedlings in response to tree shelters and mulching in northwestern Tunisia. J. For. Res. 24, 193–204. doi: 10.1007/s11676-013-0345-x
Methy, M., Gillon, D., and Houssard, C. (1997). Temperature-induced changes of photosystem II activity in Quercus ilex and Pinus halepensis. Can. J. For. Res. 27, 31–38.
Muñoz-Rengifo, J., Chirino, E., Cerdán, V., Martínez, J., Fosado, O., and Vilagrosa, A. (2020). Using field and nursery treatments to establish Quercus suber seedlings in Mediterranean degraded shrubland. iForests 13, 114–123. doi: 10.3832/ifor3095-013
Navarro-Cerrillo, R. M., Fragueiro, B., Ceaceros, C., Del Campo, A., and De Prado, R. (2005). Establishment of Quercus ilex L. subsp. ballota [Desf.] Samp. using different weed control strategies in southern Spain. Ecol. Eng. 25, 332–342. doi: 10.1016/j.ecoleng.2005.06.002
Ne’eman, G., and Trabaud, L. (eds). (2000). Ecology, biogeography and management of Pinus halepensis and P. brutia forest ecosystems in the Mediterranean Basin. Leiden: Backhuys Publishers, 407.
Niinemets, U., and Valladares, F. (2006). Tolerance to shade, drought, and waterlogging of temperate northern hemisphere trees and shrubs. Ecol. Monogr. 76, 521–547. doi: 10.1890/0012-9615
Oliet, J. A., and Jacobs, D. F. (2007). Microclimatic conditions and plant morpho-physiological development within a tree shelter environment during establishment of Quercus ilex seedlings. Agric. For. Meteorol. 144, 58–72. doi: 10.1016/j.agrformet.2007.01.012
Oliet, J. A., Blasco, R., Valenzuela, P., Melero de Blas, M., and Puértolas, J. (2019). Should we use meshes or solid tube shelters when planting in Mediterranean semiarid environments? New For. 50, 267–282. doi: 10.1007/s11056-018-9659-z
Oliet, J. A., Planelles, R., Artero, F., and Domingo-Santos, J. M. (2016). Establishing Acacia salicina under dry Mediterranean conditions: The effects of nursery fertilization and tree shelters on a mid-term experiment with saline irrigation. Cien. Inv. Agr. 43, 385–396. doi: 10.4067/S0718-16202016000100007
Oliet, J. A., Planelles, R., Artero, F., Valverde, R., Jacobs, D. F., and Segura, M. L. (2009). Field performance of Pinus halepensis planted in Mediterranean arid conditions: Relative influence of seedling morphology and mineral nutrition. New For. 37, 313–331. doi: 10.1007/s11056-008-9126-3
Oliet, J. A., Puértolas, J., Valenzuela, P., and Vázquez de Castro, P. (2021). Light transmissivity of tree shelters interacts with site environment and species ecophysiology to determine outplanting performance in Mediterranean climates. Land 10:753. doi: 10.3390/land10070753
Oliet, J., Navarro, R., and Contreras, O. (2003). Evaluación de la aplicación de mejoradores y tubos en repoblaciones forestales. Córdoba: Consejería de Medio Ambiente de la Junta de Andalucía, 234.
Oliet, J., Planelles, R., Artero, F., and Jacobs, D. F. (2005). Nursery fertilization and tree shelters affect long-term field response of Acacia salicina Lindl. planted in Mediterranean semiarid conditions. For. Ecol. Manag. 215, 339–351. doi: 10.1016/j.foreco.2005.05.024
Padilla, F. M., Miranda, J., and Pugnaire, F. I. (2007). Early root growth plasticity in seedlings of three Mediterranean woody species. Plant Soil 296, 103–113. doi: 10.1007/s11104-007-9294-5
Padilla, F. M., Miranda, J. D., Ortega, R., Hervás, M., Sánchez, J., and Pugnaire, F. I. (2011). Does shelter enhance early seedling survival in dry environments? A test with eight Mediterranean species. Appl. Veg. Sci. 14, 31–39. doi: 10.1111/j.1654-109X.2010.01094.x
Padilla, F. M., Ortega, R., Sánchez, J., and Pugnaire, F. (2009). Rethinking species selection for restoration of arid shurblands. Basic Appl. Ecol. 10, 640–647. doi: 10.1016/j.baae.2009.03.003
Padilla, F. M., and Pugnaire, F. I. (2007). Rooting depth and soil moisture control Mediterranean woody seedling survival during drought. Funct. Ecol. 21, 489–495. doi: 10.1111/j.1365-2435.2007.01267.x
Pardos, M., Calama, R., Mayoral, C., Madrigal, G., and Sánchez-Gómez, M. (2015). Addressing post-transplant summer water stress in Pinus pinea and Quercus ilex seedlings. iForest 8, 348–358. doi: 10.3832/ifor1256-007
Piñeiro, J., Maestre, F. T., Bartolomé, L., and Valdecantos, A. (2013). Ecotechnology as a tool for restoring degraded drylands: A meta-analysis of field experiments. Ecol. Eng. 61, 133–144.
Pinto, J. R., Marshall, J. D., Dumroese, R. K., Davis, A. S., and Cobo, D. R. (2016). Seedling establishment and physiological responses to temporal and spatial soil moisture changes. New For. 47, 223–241. doi: 10.1007/s11056-015-9511-7
Pinto, J. R., Marshall, J. D., Dumroese, R. K., Davis, S., and Cobos, D. R. (2012). Photosynthetic response, carbon isotopic composition, survival, and growth of three stock types under water stress enhanced by vegetative competition. Can. J. For. Res. 42, 333–344. doi: 10.1139/x11-189
Puértolas, J., Oliet, J. A., Jacobs, D. F., Benito, L. F., and Peñuelas, J. L. (2010). Is light the key factor for success of tube shelters in forest restoration plantings under Mediterranean climates? For. Ecol. Manag. 260, 610–617. doi: 10.1016/j.foreco.2010.05.017
Ruiz de la Torre, J. (1990). Mapaforestal de españa. Hoja 6-11 (almería). Memoria. Madrid: MAPA-ICONA.
Santana, V. M., Baeza, M. J., and Alday, J. G. (2014). Mulch application as post-fire rehabilitation treatment does not affect vegetation recovery in ecosystems dominated by obligate seeders. Ecol. Eng. 71, 80–86. doi: 10.1016/j.ecoleng.2014.07.037
Sit, V. (1995). Analyzing ANOVA designs (biometrics information handbook No. 5). Working paper 07/1995. Victoria, BC: Research Branch British Columbia Ministry of Forests, 61.
Smith, H., and Whitelam, G. C. (1997). The shade avoidance syndrome: Multiple responses mediated by multiple phytochromes. Plant Cell Environ. 20, 840–844. doi: 10.1046/j.1365-3040.1997.d01-104.x
Soliveres, S., Monerris, J., and Cortina, J. (2012). Irrigation, organic fertilization and species successional stage modulate the response of woody seedlings to herbaceous competition in a semi-arid quarry restoration. Appl. Veg. Sci. 15, 175–186. doi: 10.1111/j.1654-109X.2011.01163.x
South, D. B., Rakestraw, J. L., and Lowerts, G. A. (2001). Early gains from planting large diameter seedlings and intensive management are additive for loblolly pine. New For. 22, 97–110. doi: 10.1023/A:1012097924355
Spanish Agency of Meteorology (2021). Web view of the climatic Atlas. Available online at: http://agroclimap.aemet.es/ (accessed June 15, 2021).
Taylor, T. S., Loewenstein, E. F., and Chappelka, A. H. (2006). Effect of animal browse protection and fertilizer application on the establishment of planted Nuttall oak seedlings. New For. 32, 133–143. doi: 10.1007/s11056-005-4167-3
Thyroff, E. C., Burney, O. T., Oliet, J. A., Redick, C. H., and Jacobs, D. F. (2022). Toward identifying alternatives to fencing for forest restoration: Tube shelters outperform mesh shelters for deer browse protection of live oak, Quercus virginiana. Land 11:966. doi: 10.3390/land11070966
Valdecantos, A., Fuentes, D., Smanis, A., Llovet, J., Luna-Morcillo, L., and Bautista, S. (2014). Effectiveness of low-cost planting techniques for improving water availability to olea Europaea seedlings in degraded drylands. Restor. Ecol. 22, 327–335. doi: 10.1111/rec.12076
Vallejo, R. V., Smanis, A., Chirino, E., Fuentes, D., Valdecantos, A., and Vilagrosa, A. (2012). Perspectives in dryland restoration: Approaches for climate change adaptation. New For. 43, 561–579. doi: 10.1007/s11056-012-9325-9
Vázquez de Castro, A., Oliet, J. A., Puértolas, J., and Jacobs, D. F. (2014). Light transmissivity of tube shelters affects root growth and biomass allocation of Quercus ilex L. and Pinus halepensis Mill. Ann. For. Sci. 71, 91–99. doi: 10.1007/s13595-013-0335-3
Villar-Salvador, P., Puértolas, J., Cuesta, B., Peñuelas, J. L., Uscola, M., Heredia-Guerrero, N., et al. (2012). Increase in size and nitrogen concentration enhances seedling survival in Mediterranean plantations. Insights from an ecophysiological conceptual model of plant survival. New For. 43, 755–770. doi: 10.1007/s11056-012-9328-6
Keywords: arid lands, tree shelters, Aleppo pine, forest restoration, weeding, mulch
Citation: Oliet JA, Planelles R, Artero F and Jacobs DF (2023) Mesh-shelters provide more effective long-term protection than tube-shelters or mulching for restoration of Pinus halepensis in a Mediterranean arid ecosystem. Front. For. Glob. Change 5:1092703. doi: 10.3389/ffgc.2022.1092703
Received: 08 November 2022; Accepted: 12 December 2022;
Published: 05 January 2023.
Edited by:
Burenjargal Otgonsuren, Mongolian University of Life Sciences, MongoliaReviewed by:
Jose Climent, Instituto Nacional de Investigación y Tecnología Agroalimentaria (INIA), SpainRoque Rodríguez-Soalleiro, University of Santiago de Compostela, Spain
Copyright © 2023 Oliet, Planelles, Artero and Jacobs. This is an open-access article distributed under the terms of the Creative Commons Attribution License (CC BY). The use, distribution or reproduction in other forums is permitted, provided the original author(s) and the copyright owner(s) are credited and that the original publication in this journal is cited, in accordance with accepted academic practice. No use, distribution or reproduction is permitted which does not comply with these terms.
*Correspondence: Juan A. Oliet, ✉ anVhbi5vbGlldEB1cG0uZXM=