- 1Zhejiang Tiantong Forest Ecosystem National Observation and Research Station, School of Ecological and Environmental Sciences, East China Normal University, Shanghai, China
- 2Laboratory of Forest Ecology, School of Life Sciences, Nanjing University, Nanjing, China
- 3Institute of Ecology and Evolution and Oeschger Centre for Climate Change Research, University of Bern, Bern, Switzerland
- 4College of Horticulture and Forestry Science, Huazhong Agricultural University, Wuhan, China
- 5Key Laboratory of Sustainable Forest Ecosystem Management-Ministry of Education, Center for Ecological Research, School of Forestry, Northeast Forestry University, Harbin, China
Tropical forests continue to suffer from various kinds of disturbances in the Anthropocene. An immediate impact of disturbances on forest ecosystems is the creation of numerous large and small canopy gaps, which dramatically affect forest structure and function. Yet, we know little about the effect of canopy gaps on forest successional trajectory. More specifically, the responses of seedlings from different successional stages to increased light intensity under large and small canopy gaps in understory remain unclear. In this study, dominant tree seedlings from early-, mid-, and late-successional stages were selected, respectively from a tropical montane forest in Hainan Island, China to study their growth rate, biomass and traits. Our results showed that the light condition under small canopy gaps (SG, 10–15% of full sunlight) and large canopy gaps (LG, 40–50% of full sunlight) induced greater increment of relative growth rates for seedlings from early- and mid-successional stages relative to that in late-successional stage. Both SG and LG also significantly increased photosynthesis rate, leaf area (LA), light saturation point (LSP), root mass ratio (RMR) and root: shoot ratio, but decreased specific leaf area (SLA) of seedlings across successional stages. Tree seedlings from the early-successional stage displayed the greatest decrease in leaf mass ratio, increase in LA, LSP, and RMR, in comparison to those from mid- and late- successional stages. Light condition and SLA were the most important factors for seedlings’ relative growth rate across successional stages. SLA connected the interaction between the light condition and successional stage on seedlings’ growth, thereby jointly explaining the 93% variation of seedlings’ growth, combining with area-based light saturated rate of CO2 assimilation. Our study highlights the distinct effect of disturbance-induced canopy gaps on seedling regeneration in the understory in tropical forest due to the variation of light intensity. We suspect that the seedlings from late-successional stage will recover relatively slow after disturbances causing canopy losses, which can have detrimental impacts on structure feature and successional trajectory in tropical forest, as well as forest-based ecosystem services.
Introduction
Tropical forests harbor over one half of global biodiversity on land, and play a crucial role in terrestrial carbon cycle (Malhi and Marthews, 2013; Behera et al., 2022). It is crucial to understand the sustainability of functions and services in tropical forest for ecosystem management in context of global change (Lohbeck et al., 2015). In the Anthropocene, deforestation and climate changes continue to exert tremendous stress on ecosystems (Broadbent et al., 2008; Thakur et al., 2022), inducing detrimental consequences for tropical forest diversity and associated ecosystem functions (Emanuel, 2005; Miller et al., 2011; Olivero-Lora et al., 2022). Several such disturbances, like drought, heat waves, disease outbreaks, and extreme weather events (e.g., typhoon) induce tree mortality and heavy defoliation, causing canopy senescence and resulting in numerous canopy gaps (Corona-Lozada et al., 2019; Thakur et al., 2022). As a double-edged sword, disturbance-induced formation of canopy gaps cause more opportunity for germination from the soil seed bank, as well as huge loss of tree biomass (Barlow and Peres, 2004). With the formation of canopy gaps, the environment at forest understory correspondingly shifts, e.g., the enhanced available light and nutrient release from decomposition of dead trees, affecting the seedlings’ growth and the process of forest regeneration and succession after these disturbances are over (Prescott, 2002; Lin et al., 2003). Understanding the growth and survival of tree seedlings under canopy gaps at the understory is key for predicting forest resilience and also to help shed insights on the ongoing debate about the role of canopy gaps for maintaining forest’s diversity and function (Muscolo et al., 2014; Wang and Lin, 2019; Xi et al., 2019).
In the understory, the nursery for young seedlings in tropical forests, the availability of light and nutrient determine seedlings’ growth, survivorship and competition, driving forest regeneration and succession (Trauernicht et al., 2006; Mazzochini and Camargo, 2020). The formation of canopy gaps are generally considered to have positive effect on seedlings’ growth, due to the alleviated limitation of light availability (Alvarez-Clare and Avalos, 2007; Westerband and Horvitz, 2015). However, what species of tree are recruited and be more stimulated by increased light, becomes a critical determinant for the forest recovery and subsequent trajectory of natural regeneration after disturbance, in the understory of tropical forest (Matsuo et al., 2021; Tourville et al., 2022; Wang et al., 2022).
For example, tree seedlings of species from different successional stages (e.g., early-, mid-, and late- stage) exhibit distinct survival, growth rates, and susceptibility to photoinhibition in understory habitats, due to their respective physiological and growth traits (Kitao et al., 2000; Martinez-Garza et al., 2005). In general, germination of early-successional tree species occur in tree-fall gaps, characterized by a high mortality rates and high inherent growth rates (Souza and Válio, 2003). Conversely, species germinated from the later successional stages (e.g., mid- and late-successional species) or shade-tolerant species germinated in relative deep shade, exhibit high survival and lower relative growth rates to adapt the potential nutrient limitation (Kneeshaw and Bergeron, 1998; Kitao et al., 2000). The distinct optimal illumination condition among species from different successional stages is one of the main factors that determines demography of the understory seedlings’ community (Pollastrini et al., 2022). Thus, in facing with suddenly enhanced light intensity under canopy gaps after disturbance, seedlings of species from different successional stages might exhibit diverse response in growth (Hogan et al., 2022).
Besides of inherent difference in characteristics of germination and growth, species from different successional stages also represent differences in leaf traits (e.g., specific leaf area, SLA) and resource allocation strategies (e.g., pattern of biomass partitioning), which in turn regulate their ability to obtain above–(e.g., light and CO2) and below-ground resources (e.g., nutrients and water) (Davidson et al., 2002; Toledo-Aceves and Swaine, 2008). Relative to the seedlings from the late-successional stage, that from early-successional stage generally have shorter leaf life-span, higher SLA, lesser investments to defense compounds and structures, and smaller root: shoot ratio (Reich et al., 1992; Antos and Halpern, 1997; Batuwatta and Singhakumara, 2014). These different traits among species from diverse successional stage control their growth rates in forest, regulating species’ competitiveness and understory biodiversity together with forest succession (Hu et al., 2018; Tsai et al., 2018). Whether the seedlings from different successional stages, display diverse response in leaf-scale photosynthetic and/or individual morphological traits, to enhanced light intensity or not, become a crucial issue (Kitajima, 1994; Wang et al., 2022). Especially in the context of more frequent natural or anthropogenic disturbance in tropical forest, the formation of canopy gaps would affect future forest succession due to the distinct growth responses among different seedlings (Zong et al., 2018; Li et al., 2021).
In this study, we selected the dominant species from early-, mid-, and late-successional stages at the understory layer from a tropical forest in Hainan Island, which lies on the northern edge of Asian tropical rain forest as an important biodiversity hotspot in China (Li, 2002). The frequency and intensity of natural or anthropogenic disturbances, e.g., typhoon and selective logging, have increased tremendously in this district, resulting in a higher frequency of canopy gaps in tropical forest (Ding et al., 2017; Yang et al., 2017). The effects of canopy gap-induced increasing light intensity on seedlings’ growth were hypothesized to be different among species from early-, mid-, and late-successional stages, consequently regulating the future recovery and successional trajectory for tropical forest after disturbances (Olivero-Lora et al., 2022). Thus, the relative growth rates of seedlings under manipulated three light conditions, i.e., light intensity in ambient understory of tropical forest (Control, 0–5% of full light), that in small (SG, 10–15% of full sunlight, to simulate the canopy opening caused by snapped branches and defoliation) and large canopy gap (LG, 40–50% of full sunlight, to simulate the canopy opening caused by tree fall and selective logging) (Valladares et al., 2000; Yang et al., 2017), were investigated together with their photosynthetic properties (e.g., light-saturated rate of CO2 assimilation and apparent quantum efficiency) and resource allocation strategy (e.g., root: shoot ratio and leaf area ratio). Here, we seek to answer the following questions: (1) how does the increased light intensity due to canopy gaps, respectively affect relative growth rate of seedlings for tree species from early-, mid-, and late-successional stages of tropical forests; (2) what are the key properties of tree seedlings regulating their growth responses to enhanced light conditions?
Materials and methods
Experimental design
In order to probe the effects of light condition altered by canopy gaps on the growth of understory tree seedlings in tropical forest, six native tree species from early- [Dolichandrone cauda-felina (Hance) Benth. et Hook. F. and Radermachera hainanensis Merr.], mid- [Syzygium cumini (L.) Skeels and Sterculia lanceolata Cav.], and late- successional stages (Dillenia turbinate Finet et Gagnep. and Cryptocarya chinensis Hance) were selected from Jianfengling tropical montane rainforest (E 108°46′–109°45′, N 18°23′–18°50′, Hainan, China) (Li, 2002; Fang et al., 2004; Sheng et al., 2012). The seeds for each species were collected from the understory layer under five parent trees during seed dispersal stage in Jianfengling natural reserve and germinated in shallow trays filled with forest topsoil (Yang et al., 2011). The parent trees were selected in habitat without canopy opening to avoid the effect of disturbance on selected seeds. After growing in a nursery with 5% full sunlight, 1 month-old tree seedlings for each species with 20 replicates were removed to three shade-houses from June. The three shade–houses were covered with plastic shade nets of different thicknesses to achieve 0–5% (as control, i.e., the light condition in tropical forest understory without disturbance) (Capers and Chazdon, 2004), 10–15% (SG, as light condition under small canopy gaps caused by heavy defoliation or broken branches) and 40–50% of full sunlight (LG, as light condition under large canopy gaps caused by fallen tree) (Clark et al., 1996; Tobita et al., 2010; Yang et al., 2017), respectively. All the seedlings were received natural rainfall, and sprayed monthly with 100 ml solution of NPK (ammonium, phosphate, and potassium) compound fertilizer (2 g l–1) and a fungicide solution (50% Carbendazim, Pesticide Technology Development Co., Ltd., Wuhan Scarlett, China) twice during the experiment in order to control fungal infections. In order to calculate the seedlings’ relative growth rates for each species under Control, SG and LG treatments, the averaged initial biomass parameters (e.g., stem height, dry mass in leaves, stem and root) of each species were measured based ten additional replicates.
Measurements of photosynthetic, morphological properties, and relative growth rate
From July to October, the photosynthetic properties of five seedlings per species under three light treatments were measured from 09:00 to 11:30 a.m. under clear skies, by taking on five fully expanded leaves per plant. Light response curves were generated with a Li-6400 portable photosynthesis system (Li-Cor Inc., Lincoln, NE, USA) using the “Light Curve” automatic program (Yang et al., 2008). Leaves were allowed 10 min to acclimate to light intensity changes before measurements at light levels of 2,000, 1,500, 1,000, 500, 200, 100, 50, 20, 10, and 0 μmol m–2⋅s–1. Ambient temperature ranged from 24 to 28°C, and leaf chamber temperature was about 25°C. The leaf chamber environment was maintained at 370 mmol m–2 s–1 CO2, 28 ± 2°C leaf temperature and 65 ± 5% relative air humidity in the measuring chamber, respectively. Light response curves (A/PAR) were fit using non-rectangular hyperbola least square curve fitting procedure (Equation 1, Eq. 1; Lambers et al., 1998):
where AQE is the apparent quantum efficiency; PAR is photosynthetic available radiation; Pmax (μmol CO2 m–2 s–1) is the light-saturated rate of CO2 assimilation; Rd (μmol CO2 m–2 s–1) is the dark respiration rate; and k is the convexity or curvature factor. The light compensation point (LCP) was determined to be the light intensity on the light curve where the rate of photosynthesis exactly matches the rate of cellular respiration. The light saturation point (LSP) was calculated by the same equation, considering that LSP is the value when net photosynthetic rate (Pn) reaches 90% of Pmax (Quero et al., 2006).
After 12 months of experimental duration in shade houses to create canopy conditions caused by disturbances, 10 seedlings per species from three treatments of light conditions (i.e., Control, SG, and LG) were harvested for relative growth rate in mass (RGRm, Eq. 2). Before being dried at 72°C in a forced air oven for 48 h for dry mass measurement, seedlings were separated into roots, stems and leaves, and washed for morphological characteristics determination, including specific leaf area (SLA, Eq. 3), leaf mass ratio (LMR, Eq. 4), stem mass ratio (SMR, Eq. 5), root mass ratio (RMR, Eq. 6), and leaf area ratio (LAR, Eq. 7).
where W2 and W1 are the final and initial total dry weights per plant; and T2–T1 is the growth time interval (i.e., 12 months, Yang et al., 2011).
where leaf total area of each seedling was measured using a Li-3000 leaf area meter (Li-Cor Inc., Lincoln, NE, USA, An et al., 2010).
Data analysis
The mean effect size of light condition changed by SG and LG on morphological and photosynthetic properties on tree seedlings from early-, mid-, and late-successional stages were calculated as Eq. 8–12 (Hedges et al., 1999):
where, i is the number of species of tree seedlings from early-, mid-, and late-successional stages; and nSG or LG, and nCK, SSG or LG and SCKare the mean value, number of replication and standard deviation of morphological and photosynthetic properties for tree seedlings in SG or LG, and control treatment, respectively. A significant mean effect size of light condition changed by SG and LG on morphological and photosynthetic properties was considered only when the 95% confidence interval (CI) did not overlap with zero (Zhou et al., 2014).
The importance of morphological and photosynthetic properties on seedlings’ RGRm was expressed as %IncMSE (percent increase in mean squared error) using the package “RandomForest” (version 4.6-12, Liaw and Wiener, 2002) in R (R Core Team, 2015). The relationships among predictors with RGRm were analyzed by Pearson correlation analysis. The effect of light conditions (Control, SG, and LG) and successional stage (early-, mid-, and late-) on the variables were examined by analysis of variance (ANOVA). The path analysis was performed using the “lavaan” package (version 0.6-12, Rosseel, 2010) in R to examine the effect of light conditions and successional stages on RGRm through morphological and photosynthetic properties.
Results
Response of seedlings’ relative growth rates to increased light intensity
In the control groups with ambient light intensity, seedlings from different successional stages exhibited significant differences in the relative growth rate (RGRm, p < 0.0001), with the lowest values of –8.61 (± 0.41 standard error, s.e.) mg g–1d–1 at early-successional stage and the highest value of 5.61 (± 0.80 s.e.) mg g–1d–1 at late-successional stage (Supplementary Table 1 and Figures 1A–D). Relative to control, both SG and LG induced significant increment of RGRm for seedlings (P < 0.05, Supplementary Table 1 and Figure 1E). Improved light condition caused greater enhancement of growth for seedlings from both early- and mid-successional stage than that from the late-one, that SG and LG increased RGRm of seedlings from both early- and mid-successional stage significantly, but not always of the seedling RGRm of late- successional tree species (Figures 1A–C). Canopy gaps could affect the structure of understory community due to these distinct positive effects of increased light intensity on relative growth rates among seedlings from different successional stages (Figure 1).
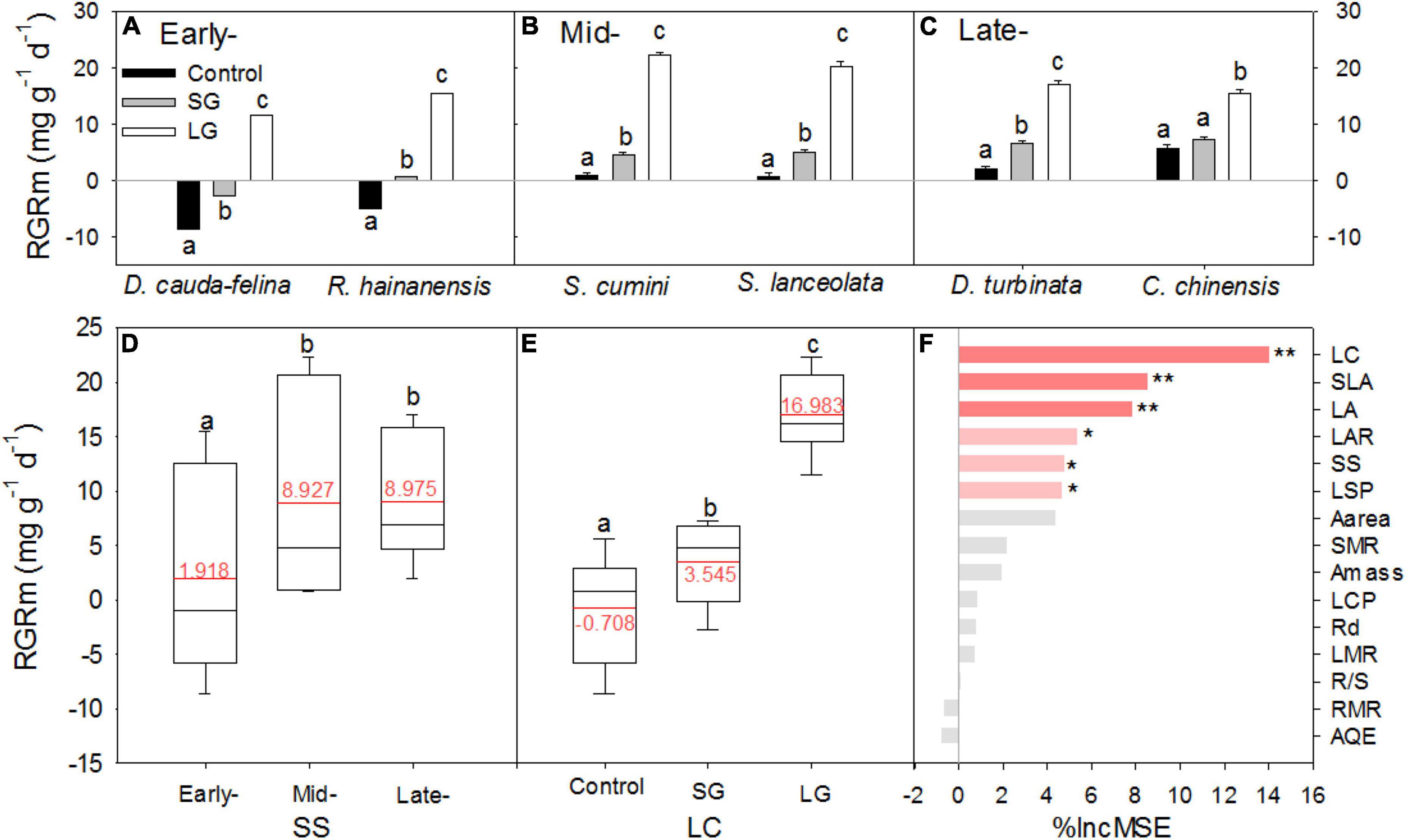
Figure 1. Relative mass growth rate [RGRm, (A–D)] for tree seedlings at early– (A,D), mid- (B,D) and late-successional stage (C,D), and in light conditions of Control, SG and LG (E), and the importance of predictor variables for RGRm in random forest modelling (F). Control, 0–5% of full sunlight; SG: small canopy gaps, 10–15% of full sunlight; LG: large canopy gaps, 40–50% of full sunlight. The red lines and figures in plots (D,E) are the mean value of RGRm in each group. LC, SLA, LA, LAR, SS, LSP, LCP, Aarea, Amass, SMR, Rd, LMR, R/S, RMR, and AQE in panel (F) were abbreviations of light condition, specific leaf area, leaf area, leaf area ratio, successional stage, light saturation and compensation point, area- and mass- based light saturated rate of CO2 assimilation, stem mass ratio, dark respiration, leaf mass ratio, root: shoot ratio, root mass ratio, and apparent quantum efficiency, respectively. In random forest modeling, “1,” “2,” and “3” were assigned to treatments of Control, SG and LG; and species at early-, mid-, and late-successional stage, respectively. %IncMSE is the per cent increase in mean squared error. Error bars and the different letters in plots (A–E) are standard error (n = 25) and significant difference at P < 0.05, respectively. Symbols ** and * in plot (F) indicates statistical significance at P < 0.01 and 0.05, respectively.
Response of seedlings’ photosynthetic and morphological properties to increased light intensity
Improved light intensity (i.e., in SG and LG) caused higher photosynthesis rates (Pn) relative to that in control, inducing 24.6–212.1, 35.0–107.8, and 17.8–50.2% increase of mass- and area-based light-saturated rate of CO2 assimilation (Amass and Aarea) for species at early-, mid-, and late-successional stage, respectively (P < 0.05, Figures 2A–F). Leaf area (LA) and light saturation point (LSP) in both SG and LG were greater than that in control (P < 0.05, Supplementary Tables 1, 2), while the pattern of specific leaf area (SLA) was contrary (Figures 2G–I and Supplementary Table 2). Increased light intensity induced larger positive effects on both LA and LSP for seedlings from early-successional stage than that from mid- and late-ones (Figure 2). Relative to SG, LG induced greater effect on seedlings’ photosynthetic properties, especially for species from early-successional stage (Figure 2 and Supplementary Table 1). Both dark respiration (Rd) and light compensation point (LCP) displayed positive response to LG (P < 0.05) but not to SG (P > 0.05, Figures 2G–I, and Supplementary Table 2).
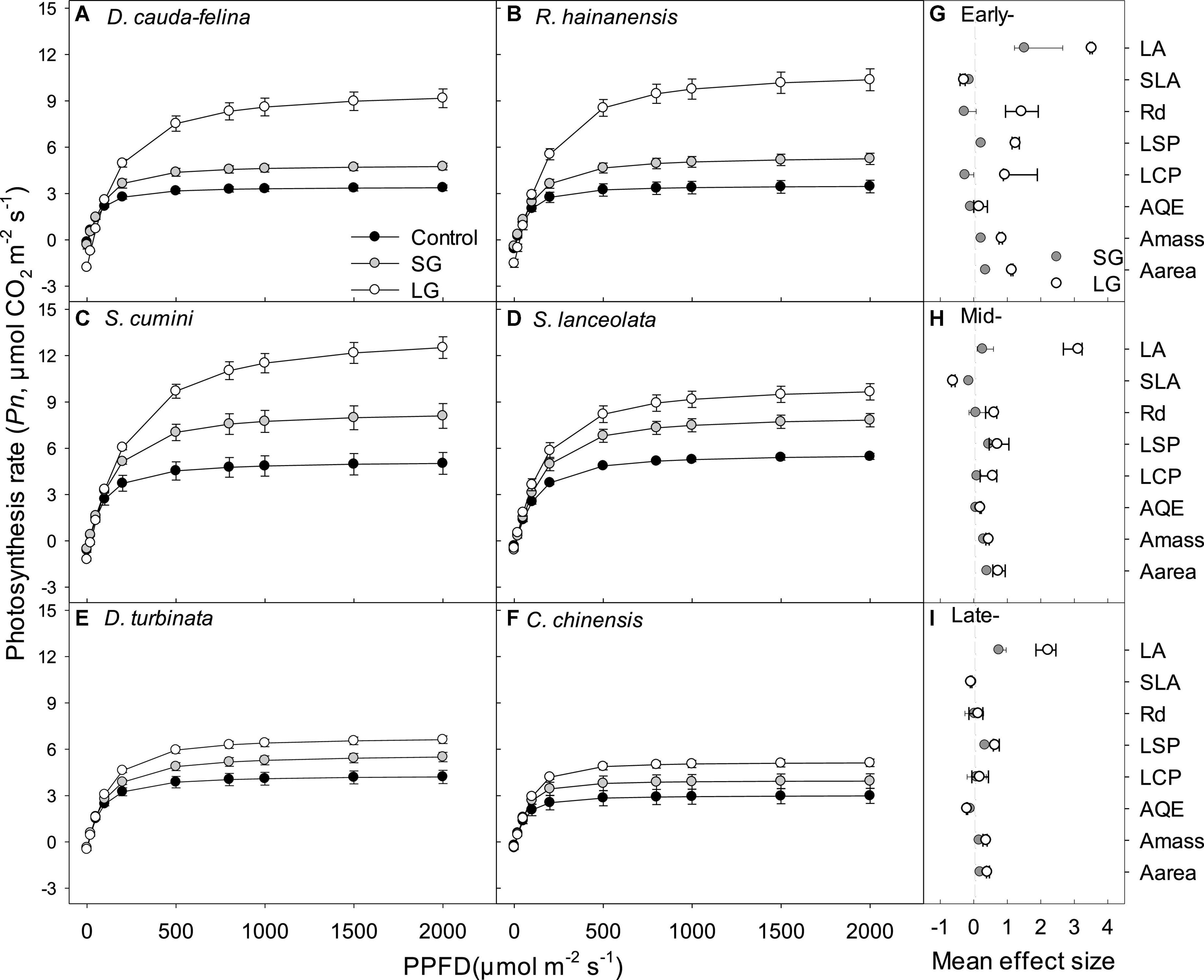
Figure 2. Photosynthetic light response curves (A–F) for seedlings grown in light conditions under Control, SG and LG, and the mean effect size (G–I) of SG and LG on leaf area (LA), specific leaf area (SLA), dark respiration (Rd), light saturation point (LSP), light compensation point (LCP), apparent quantum efficiency (AQE), mass-, and area- based light saturated rate of CO2 assimilation (Amass and Aarea) for seedlings at early- (G), mid- (H) and late-successional stage (I). Control: 0–5% of full sunlight; SG: small canopy gaps, 10–15% of full sunlight; LG: large canopy gaps, 40–50% of full sunlight. Light curves were fitted by non-linear regression using non-rectangular hyperbola least square curve fitting procedure (Lambers et al., 1998). Data points in plots (A–F) and (G–I) represent mean ± s.e. [standard error, n = 25 (5 plants × 5 leaves)] and mean ± CI (the 95% confidence interval), respectively. If the CI in plots (G–I) did not overlap with zero (the gray dotted line), a significant mean effect of SG and/or LG was considered (P < 0.05).
Both SG and LG caused positive effect on root: shoot ratio (R/S) and root mass ratio (RMR) for seedlings from all three successional stages, while only LG significantly decreased seedlings’ leaf area ratio (LAR) due to the dependence on both light condition and successional stages (P < 0.05, Figures 2D–F and Supplementary Tables 1, 3). Species at early-successional stage displayed the greatest decrease of leaf mass ratio (LMR) and increase of RMR in comparison with that at mid- and late-successional stage, especially for those at late- stage, seedlings displayed no significant decrease of LMR under both SG and LG (P < 0.05, Figure 2F and Supplementary Figure 1). Seedlings from early-stage displayed greater positive responses of both photosynthetic and morphological properties to enhanced light intensity, suggesting a larger advantage in growth in early-stage tree species over mid- and late-successional tree species (Figures 2, 3).
Regulation of successional stage on RGRm’s response to increased light condition
Among the predictor variables, we confirmed that light condition was the most important factor for seedlings’ RGRm, followed by SLA, LA, LAR, successional stage, and LSP (Figure 1F). Light condition increased LA, RMR, R/S, Rd, LCP, LSP and Aarea, but decreased SLA (Figures 2, 3, 5), and displayed significant interactions with successional stage on SLA, Rd, LCP and Aarea (P = 0.021, 0.014, 0.012 and 0.048, Supplementary Table 1). RGRm displayed positive correlations with Aarea, LA, LSP and R/S (R2 = 0.51, 0.39, 0.46, and 0.24, respectively, P < 0.05, Figures 4A–C, F), while exhibited negative relationship with SLA and LAR (R2 = 0.52 and 0.45, P < 0.01, Figures 4D, E). The positive effects of improved light condition on RGRm included direct and indirect pathways (through SLA and Aarea, Figure 5). The regulation of species’ successional stage on RGRm was through the path of SLA. Light condition, Aarea and SLA all had direct path to affect RGRm, and jointly explained 93% of seedlings’ RGRm in total (P = 0.319, Figure 5). As one of crucial traits of plants, SLA represented the important regulation of successional stages on seedlings’ RGRm in responding to enhanced light intensity after disturbance-induced canopy gaps (Figures 1F, 5).
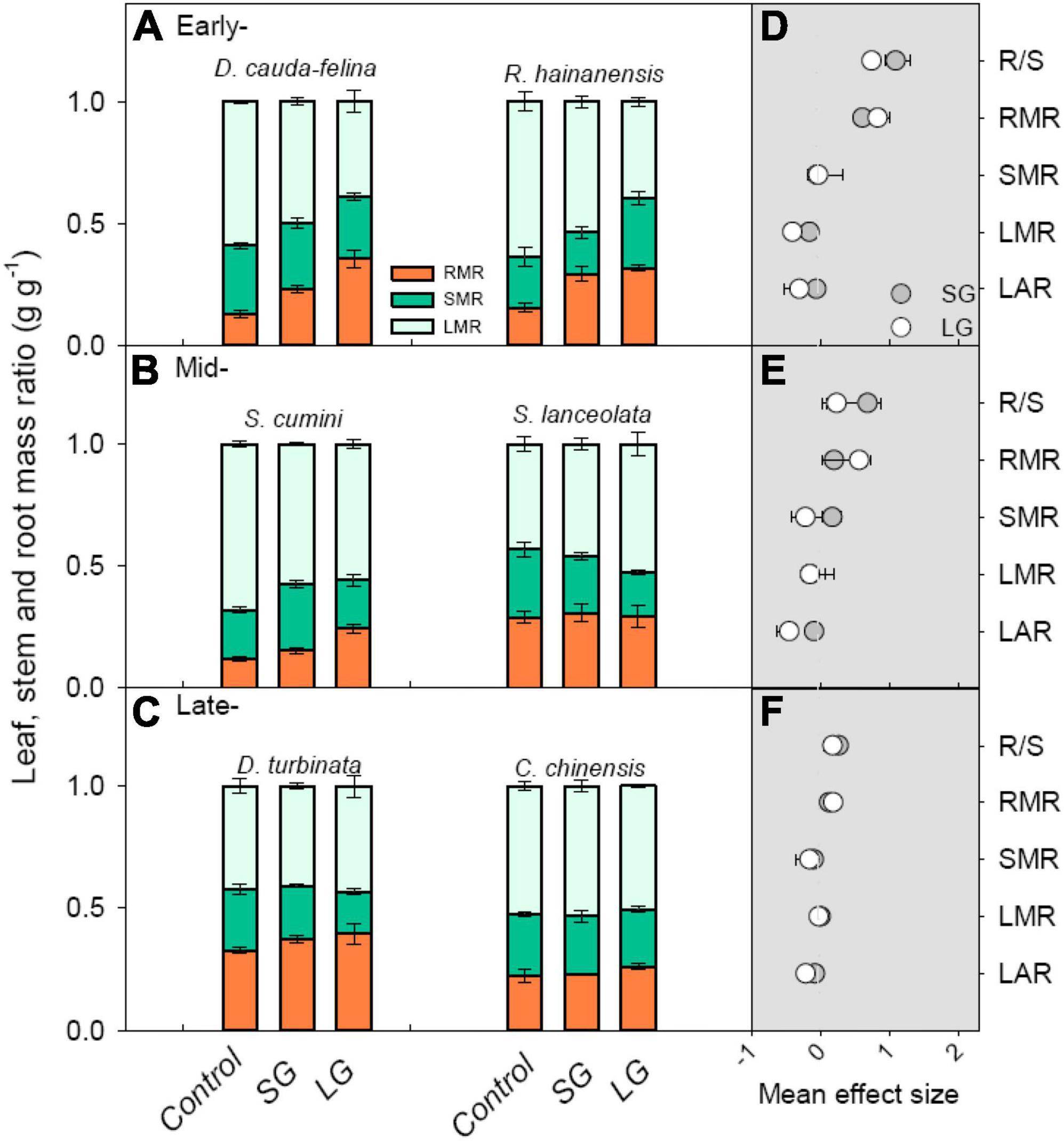
Figure 3. Leaf, stem and root mass ratio [LMR, SMR, and RMR, (A–C)] of seedlings in Control, SG, and LG, and the mean effect size (D–F) of SG and LG on root: shoot ratio (R/S), root, stem, and leaf mass ratio (RMR, SMR, and LMR), and leaf area ratio (LAR) for tree seedlings at early- (D), mid- (E), late-successional stage (F). Control, 0–5% of full sunlight; SG: small canopy gaps, 10–15% of full sunlight; LG: large canopy gaps, 40–50% of full sunlight. The bars in plots (A–C) and (D–F) represent standard error (n = 5 plants) and 95% confidence interval (CI), respectively. If the CI in plots (D–F) did not overlap with zero (the gray dotted line), a significant mean effect of SG and/or LG was considered (P < 0.05).
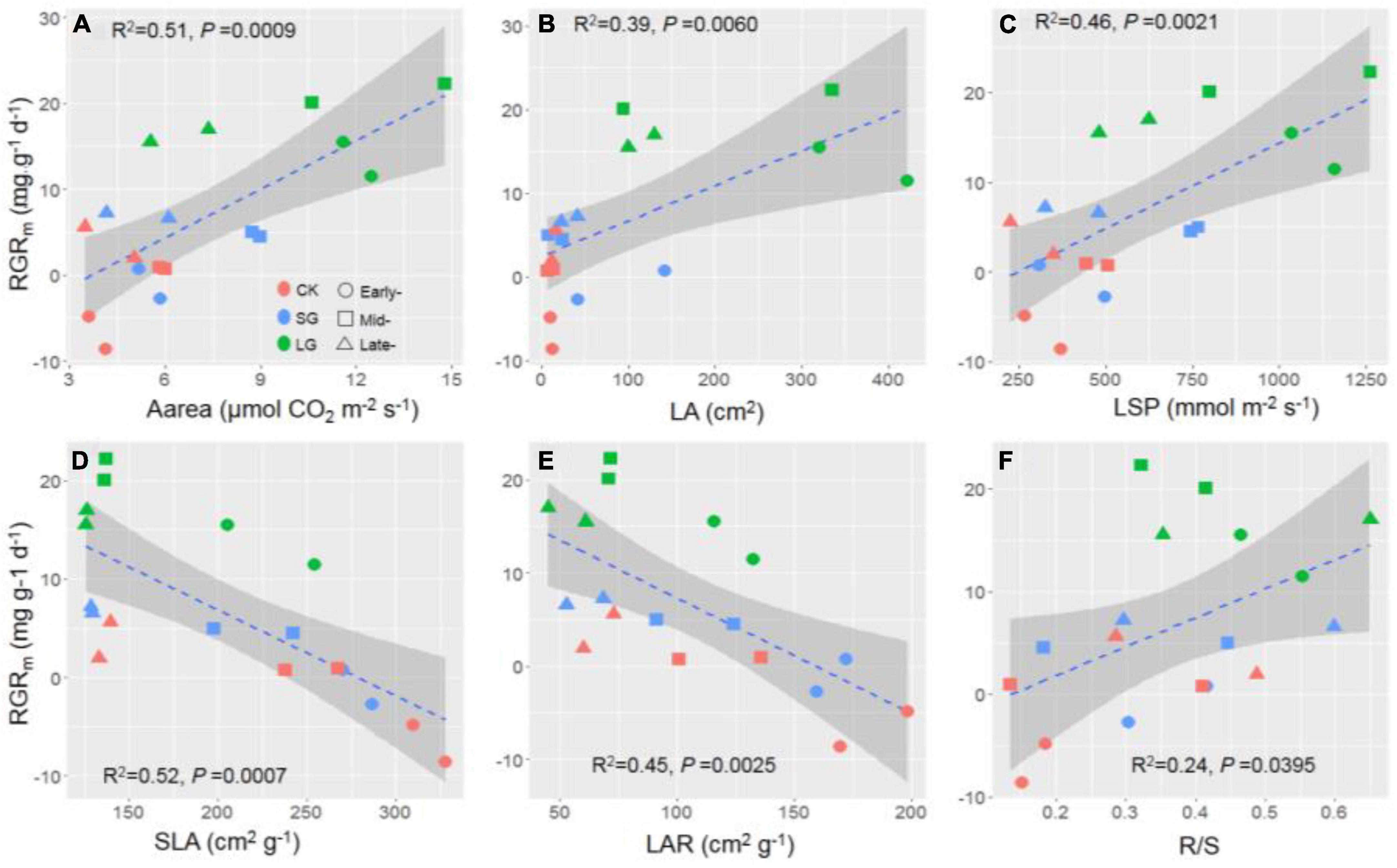
Figure 4. The relationship between relative mass growth rate (RGRm) with area- based light saturated rate of CO2 assimilation [Aarea, (A)], leaf area [LA, (B)], light saturation point [LSP, (C)], specific leaf area [SLA, (D)], leaf area ratio [LAR, (E)], and root: shoot ratio [R/S, (F)]. The gray shadow represents the 95% confidence interval for the estimates.
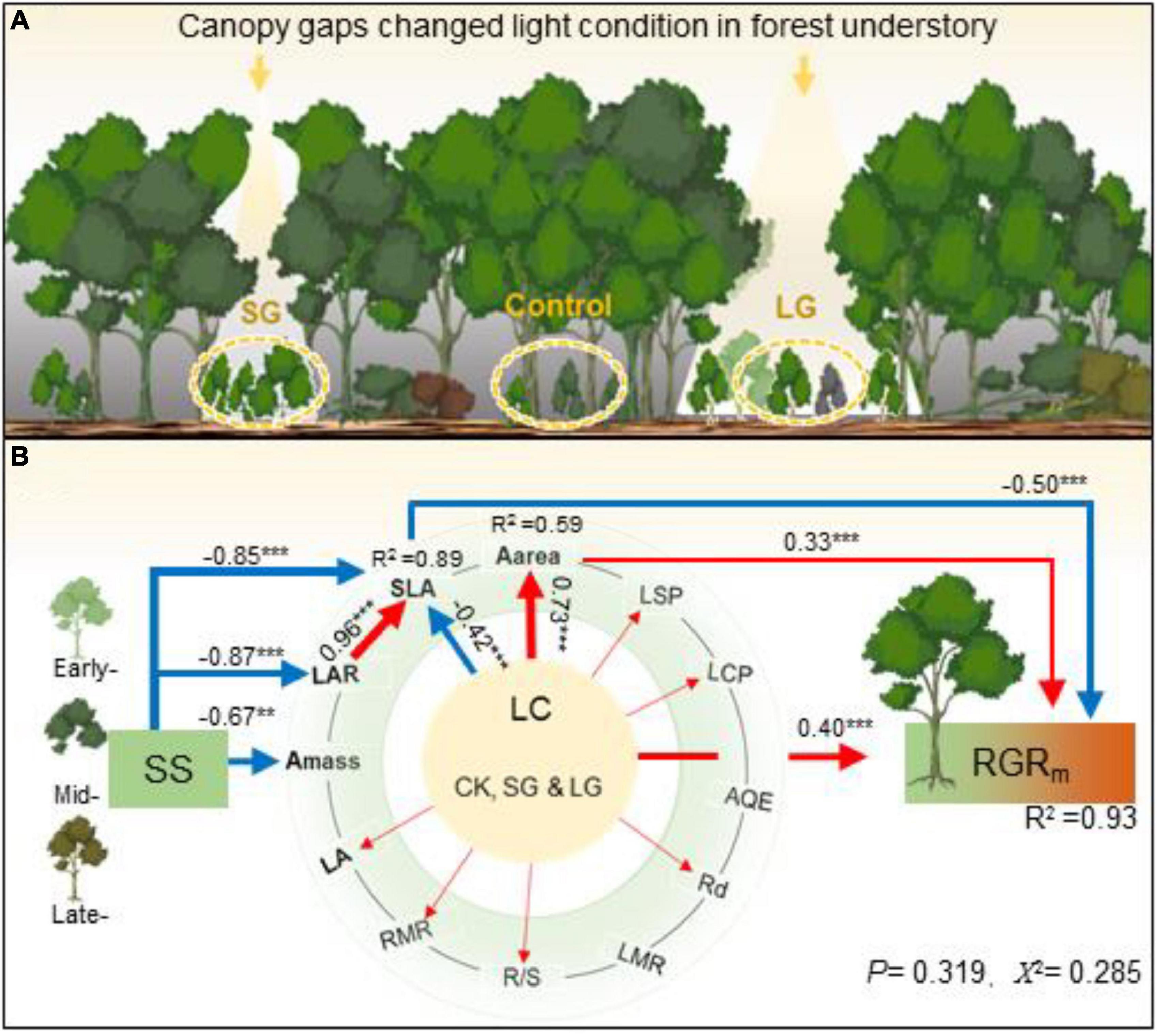
Figure 5. Illustration of disturbance-caused canopy gaps’ impact on light condition (LC, i.e., Control, SG, and LG) in forest understory (A), and their effects on seedlings’ relative mass growth rate [RGRm, (B)] for tree species at different successional stage (SS, i.e., early-, mid-, and late- successional stage) based on the path analysis. Control, 0–5% of full sunlight; SG: small canopy gaps, 10–15% of full sunlight; LG: large canopy gaps, 40–50% of full sunlight. In path analysis, “1,” “2”, and “3” were assigned to treatments of Control, SG and LG; and species at early-, mid-, and late-successional stage, respectively. The red and blue arrows indicate significantly positive and negative effects of light condition (LC), successional stage (SS), leaf area (LA), specific leaf area (SLA), dark respiration (Rd), light saturation point (LSP), light compensation point (LCP), apparent quantum efficiency (AQE), mass-, and area- based light saturated rate of CO2 assimilation (Amass and Aarea), leaf mass ratio (LMR), root mass ratio (RMR) and root: shoot ratio (R/S) on RGRm (P < 0.05), respectively. Symbols **, *** indicate statistical significance at P < 0.01 and 0.001. In Chi-square test for the path model, the P-value (0.319, >0.05) calculated from one-tailed test indicated that model fit had been established.
Discussion
Changes in the canopy structure and resource availability due to various anthropogenic activities continue to alter forest regeneration and ecosystem functioning. Here, we tested whether the increased light availability (and intensity) due to various size of canopy gaps created from anthropogenic activities differentially affect the growth rates of tree seedlings from different successional stages, which are crucial for predicting future forest recovery and successional trajectory. The results agreed with our hypothesis that the light condition under small canopy gaps (SG, 10–15% of full sunlight) and large canopy gaps (LG, 40–50% of full sunlight) induced greater increment of relative growth rates for seedlings from early- and mid-successional stages relative to that in late- successional stage.
Effect of light condition under canopy gap on growth of seedlings at different successional stages
Canopy opening readily alleviate the light limitation for understory plants in a forest (Lin et al., 2003; Lee et al., 2017). In our study, seedlings grown with light intensity to mimic small (SG) and large canopy gap (LG) displayed significantly higher RGRm than that with ambient light intensity (Control, P < 0.05, Figure 1 and Supplementary Table 1). Moreover, we found that the development of leaf area (LA, Figures 2, 4) was one of the most important mechanisms to improve photosynthetic assimilation for plant individuals at enhanced light availability (Evans and Poorter, 2001; Tang et al., 2021). The formation of canopy gaps was verified to be beneficial for the understory regeneration in Jianfengling tropical montane rainforest (Feldmann et al., 2020), without considering the impacts on the direction of forest succession.
Exposed to increased light intensity, seedlings at early successional stage with a negative RGRm in ambient light condition, exhibited the greatest improvement in their RGRm (Figure 1), altering the survival rate, competition and dynamics of seedling community in the tropical forest understory subsequently (Valladares et al., 2000). In contrast, relative to seedlings from early- and mid- successional stage, those from the late-successional stage displayed a lower increment in RGRm at increased light intensity (Figure 1), potentially confirming to the narrow niche breadth of species from the late-successional stage (Parrish and Bazzaz, 1982; Carscadden et al., 2020). Therefore, the canopy gaps due to defoliation (with the equivalent light intensity in SG) and tree fall (with the equivalent light intensity in LG) would trigger the changes of understory productivity, biodiversity and successional trajectory in disturbed patches, altering the structural heterogeneity in tropical forest (Takafumi et al., 2010; Lee et al., 2017).
Effect of light condition under canopy gap on photosynthetic properties of seedling at different successional stages
The different response of RGRm among seedlings from three successional stages could be firstly reflected by corresponding changes in leaf-scale photosynthetic properties (Figures 1, 2, 4), which is the foundation for regulating plant carbon assimilation to take the advantage from enhanced light due to temporary canopy gaps (Kneeshaw and Bergeron, 1998; Yao et al., 2015). Especially for the greatest improvement of RGRm for seedlings at early successional stage could be ascribed to their changes in photosynthetic properties, e.g., a significant enhancement of light saturated point (LSP, Figures 2, 4). We suspect that greater LSP may have benefited the seedlings to achieve a higher saturated photosynthetic rate, such as the light–demanding species from the early stage of succession (Ackerly, 1996; Qi et al., 2004). Especially for light condition in LG, seedlings from early-successional stage displayed the largest improvement in both mass- and area-based light-saturated rate of CO2 assimilation (Amass and Aarea, Figure 2), thus the more light penetrated into forest understory the more advantage would be found for seedlings from early-successional stage (Baul et al., 2022). Beside changes in LSP, light compensation point (LCP) and dark respiration (Rd) in seedlings from early successional stages may also have enhanced due to increased growth respiration driven by accelerated development of leaf and total biomass (Figures 1, 2; Marcelis et al., 1998; Inoue et al., 2022). Furthermore, seedlings from early- and mid- successional stages declined their specific leaf area (SLA, Figure 2) as a response to improved light conditions, especially in LG treatment, while that from the late- with an original successional stage lower SLA had no significant changes in SLA (Figure 2 and Supplementary Table 3), displaying the evolutionary conservatism in this functional trait among these plant species (Schweizer et al., 2013; Letcher et al., 2015). The intensity and range of disturbance would be crucial for species composition and successional process in understory patches in tropical forest, the larger canopy gap might brought more advantage for seedlings from early- and mid-successional stages (Yamashita et al., 2000; Liu B. et al., 2012).
Different from other photosynthetic properties with positive or negative responses, the apparent quantum yields (AQE) didn’t change even for the seedlings from the early-successional stage (Figure 2 and Supplementary Table 1), which agreed with the correlated results in comparing AQE between shade-tolerant species (i.e., from the late-successional stage) and pioneer species (i.e., from the early-successional stage) previous studies (e.g., Ramos and Grace, 1990; Marenco et al., 2001; Tsvuura et al., 2010).
Effect of light condition under canopy gap on morphological properties in seedlings at different successional stages
Apart from the regulation in leaf-scale photosynthesis properties, seedlings would also alter allocation strategy of photosynthates to the organs acquiring the resource that strongly limits seedling growth (McCarthy and Enquist, 2007; Zhou et al., 2020). In this study, the sensitivity of root mass ratio was greater than that of leaf and stem mass ratio under improved light intensity (Figure 3; Poorter and Nagel, 2000). The adequate root development was considered to be crucial for improving seedlings’ survival in forest understory, since more belowground sources, e.g., nutrients and water, could support seedlings with an accelerated growth and a greater competitiveness in understory community (Landhausser and Lieffers, 2001; Myers and Kitajima, 2007). Correspondingly, the vertical biomass allocations between below- and above- ground biomass, reflected in root: shoot ratio (R/S) in these seedlings were altered by improved light availability (Figure 3; Poorter and Nagel, 2000). However, the significant positive response of R/S to both SG and LG exhibiting an increasing trend from late- to early-successional stage (Figure 3), that the seedlings from early- and mid- successional stages displayed a greater morphological plasticity than that from late- stage (Yan et al., 2006). The greater increment of R/S for seedlings from early- and mid- successional stages would be more beneficial for root nutrient absorption, particularly for phosphorus capture, in tropical montane forests, such as our study site (Liu et al., 2010, Liu F. et al., 2012). Thus, seedlings from early- and mid- could successional stages take advantage of the transitory opportunity of improved light condition caused by anthropogenic disturbances, regulating seedlings’ niche-partitioning and successional process in the understory of tropical forest (Dupuy and Chazdon, 2006; Yang et al., 2011).
In contrast to the positive correlation between R/S and RGRm, leaf area ratio (LAR) exhibited a negative relationship with RGRm (Figure 4). Under higher light intensity, per unit leaf area or shoot mass could provide greater productivity for plant individual than ambient light condition in understory (Kong et al., 2016), that seedlings with lower LAR and higher R/S potentially had a sufficient functions of above-ground organs, e.g., adequate photosynthetic capacity under increased light intensity (Shafiq et al., 2021). Therefore, canopy gap-induced greater light intensity could drive the trade-off between above- and below-ground biomass allocation, regulating morphological properties of seedlings for better resource acquisition and growing (Freschet et al., 2013; Baez and Homeier, 2018).
The dependence of seedlings’ RGRm on successional stage in responding to increased light condition under canopy gap
Our results show that the seedlings in the understory from different successional stages are characterized by diverse strategies in responding to improved light condition caused by canopy gap after disturbance (Figures 1–3). In ambient light condition of understory, seedlings from the late-successional stage had a higher RGRm, ensuring a greater competitiveness relative to seedlings from early- and mid-successional stages in the understory tree community (Figure 2; Gao et al., 2016). However, greater light availability due to canopy gaps provided a potential opportunity for the seedlings from early- or mid- successional stages to offset the competitive advantage of late-successional plants (Abbas et al., 2020).
Seedlings’ RGRm in the understory of tropical forest performed a dependence on the light condition, while the successional stage of species displayed a significant regulation on changes of RGRm through effects on Amass, LAR and SLA (Figure 5). Thereinto, SLA was a critical traits connected the effects of light condition and successional stage on seedlings’ growth, and playing the most important role for RGRm after light condition (Figures 1F, 5; Baez and Homeier, 2018). For different seedlings, interspecific functional trait differentiation had been indicated to mainly associate with SLA, that at early-successional stage are generally characterized by a higher SLA relative to that at mid- and late- stages (Supplementary Table 3; Wang et al., 2012). Light condition and successional stage displayed a significant interaction on seedlings’ SLA, jointly affected seedlings’ RGRm in the understory of tropical forest (Supplementary Table 1 and Figure 5; Kitao et al., 2000). Under the more frequency and intensity disturbances, the advantage of seedlings from late-successional stage would be weaken much more than that from early- and mid-successional stages, slowing down the process of forest forward succession.
Conclusion
Natural and anthropogenic disturbance induced canopy gaps affecting the forest structure and function. Increased light intensity under canopy gaps were verified to accelerate the relative growth rate (RGRm) of seedlings, improving the understory productivity in Jianfengling tropical rainforest. Relative to the seedlings from late- successional stages, early- and mid-successional stage seedlings displayed stronger positive response of RGRm due to the higher improvement of leaf area, light saturation point, mass- or area-based light saturated rates of CO2 assimilation, root mass ratio and root: shoot ratio, as well as the decrement of specific leaf area and leaf area ratio. Light condition and SLA were the most important factors for seedlings’ relative growth rate across successional stages. The formation of canopy gaps would open up opportunities for the seedlings of tree species from the early- and mid-successional stages, being beneficial for species diversity and habitat heterogeneity, while go against the forward successional process due to the depressed advantage of species from late-successional stage in tropical forest. Especially, in the context of global climate change, facing higher frequency and intensity of natural disturbance (i.e., typhoon and forest fire), whether the succession of tropical forest would be redirected by too many big scale canopy gaps should depend on both disturbance per se and forest stability.
Data availability statement
The original contributions presented in this study are included in the article/Supplementary material, further inquiries can be directed to the corresponding authors.
Author contributions
LZ completed the experiment and analyzed the data with substantial contributions from MT, WY, and SA. LZ, WY, and SA conceived, designed, and oversaw the study. LZ wrote the manuscript with substantial contribution from MT. ZJ and YH did the statistical analysis with suggestions from LZ. MT, SA, and XZ commented on the manuscript. All authors contributed to the article and approved the submitted version.
Funding
This research was funded by the National Natural Science Foundation of China (Grant Nos. 32071593, 31600352, and 31930072), Shanghai Key Lab for Urban Ecological Processes and Eco-Restoration (SHUES2021B01), and the Fundamental Research Funds for the Central Universities. MT acknowledges the support from the Swiss State Secretariat for Education, Research and lnnovation (SERI) under contract number M822.00029.
Acknowledgments
We acknowledge the staff of the Jianfengling Experimental Station (Research Institute of Tropical Forestry, Chinese Academy of Forestry) for their field assistance.
Conflict of interest
The authors declare that the research was conducted in the absence of any commercial or financial relationships that could be construed as a potential conflict of interest.
Publisher’s note
All claims expressed in this article are solely those of the authors and do not necessarily represent those of their affiliated organizations, or those of the publisher, the editors and the reviewers. Any product that may be evaluated in this article, or claim that may be made by its manufacturer, is not guaranteed or endorsed by the publisher.
Supplementary material
The Supplementary Material for this article can be found online at: https://www.frontiersin.org/articles/10.3389/ffgc.2022.1088291/full#supplementary-material
References
Abbas, S., Nichol, J. E., Fischer, G. A., Wong, M. S., and Irteza, S. M. (2020). Impact assessment of a super-typhoon on Hong Kong’s secondary vegetation and recommendations for restoration of resilience in the forest succession. Agric. For. Meteorol. 280:107784. doi: 10.1016/j.agrformet.2019.107784
Ackerly, D. D. (1996). “Canopy structure and dynamics: Integration of growth processes in tropical pioneer trees,” in Tropical forest plant ecophysiology, eds S. Strauss-Debenedetti, F. A. Bazzaz, S. S. Mulkey, R. L. Chazdon, and A. P. Smith (New York, NY: Springer), 619–658. doi: 10.1007/978-1-4613-1163-8_21
Alvarez-Clare, S., and Avalos, G. (2007). Light interception efficiency of the understory palm Calyptrogyne ghiesbreghtiana under deep shade conditions. Ecotropica 13, 57–65.
An, D., Li, M., and Zhang, L. (2010). Measurement of tomato leaf area using computer image processing technology. Sens. Lett. 8, 56–60. doi: 10.1166/sl.2010.1201
Antos, J. A., and Halpern, C. B. (1997). Root system differences among species: Implications for early successional changes in forests of Western Oregon. Am. Midl. Nat. 138, 97–108. doi: 10.2307/2426658
Baez, S., and Homeier, J. (2018). Functional traits determine tree growth and ecosystem productivity of a tropical montane forest: Insights from a long-term nutrient manipulation experiment. Glob. Change Biol. 24, 399–409. doi: 10.1111/gcb.13905
Barlow, J., and Peres, C. A. (2004). Ecological responses to El Nino-induced surface fires in central Brazilian Amazonia: Management implications for flammable tropical forests. Philos. Trans. R. Soc. B Biol. Sci. 359, 367–380. doi: 10.1098/rstb.2003.1423
Batuwatta, S., and Singhakumara, B. (2014). “Sun-shade plasticity in leaf traits of early and late-successional tree species in a low land rain forest South West Sri Lanka,” in Proceedings of the 2014 international forestry and environment symposium, Sigiriya. doi: 10.31357/fesympo.v18i0.1864
Baul, T. K., Chowdhury, A. I., Uddin, M., Hasan, M. K., Nandi, R., Nath, T. K., et al. (2022). Evaluating gap characteristics and their effects on regeneration in Sitapahar forest reserve, Bangladesh. Eur. J. For. Res. doi: 10.1007/s10342-022-01502-3
Behera, S. K., Mishra, S., Sahu, N., Manika, N., Singh, S. N., Anto, S., et al. (2022). Assessment of carbon sequestration potential of tropical tree species for urban forestry in India. Ecol. Eng. 181:106692. doi: 10.1016/j.ecoleng.2022.106692
Broadbent, E. N., Asner, G. P., Keller, M., Knapp, D. E., Oliveira, P. J. C., and Silva, J. N. (2008). Forest fragmentation and edge effects from deforestation and selective logging in the Brazilian Amazon. Biol. Conserv. 141, 1745–1757. doi: 10.1016/j.biocon.2008.04.024
Capers, R. S., and Chazdon, R. L. (2004). Rapid assessment of understory light availability in a wet tropical forest. Agric. For. Meteorol. 123, 177–185. doi: 10.1016/j.agrformet.2003.12.009
Carscadden, K. A., Emery, N. C., Arnillas, C. A., Cadotte, M. W., Afkhami, M. E., Gravel, D., et al. (2020). Niche breadth: Causes and consequences for ecology, evolution, and conservation. Q. Rev. Biol. 95, 179–214. doi: 10.1086/710388
Clark, D. B., Clark, D. A., Rich, P. M., Weiss, S., and Oberbauer, S. F. (1996). Landscape scale evaluation of understory light and canopy structure: Methods and application in a neotropical lowland rain forest. Can. J. For. Res. 26, 747–757. doi: 10.1139/x26-084
Corona-Lozada, M. C., Morin, S., and Choler, P. (2019). Drought offsets the positive effect of summer heat waves on the canopy greenness of mountain grasslands. Agric. For. Meteorol. 276-277:107617. doi: 10.1016/j.agrformet.2019.107617
Davidson, R., Mauffette, Y., and Gagnon, D. (2002). Light requirements of seedlings: A method for selecting tropical trees for plantation forestry. Basic Appl. Ecol. 3, 209–220. doi: 10.1078/1439-1791-00098
Ding, Y., Zang, R., Lu, X., and Huang, J. (2017). The impacts of selective logging and clear-cutting on woody plant diversity after 40years of natural recovery in a tropical montane rain forest, south China. Sci. Total Environ. 579, 1683–1691. doi: 10.1016/j.scitotenv.2016.11.185
Dupuy, J. M., and Chazdon, R. L. (2006). Effects of vegetation cover on seedling and sapling dynamics in secondary tropical wet forests in Costa Rica. J. Trop. Ecol. 22, 65–76. doi: 10.1017/S0266467405002890
Emanuel, K. (2005). Increasing destructiveness of tropical cyclones over the past 30 years. Nature 436, 686–688. doi: 10.1038/nature03906
Evans, J. R., and Poorter, H. (2001). Photosynthetic acclimation of plants to growth irradiance: The relative importance of specific leaf area and nitrogen partitioning in maximizing carbon gain. Plant Cell Environ. 24, 755–767. doi: 10.1046/j.1365-3040.2001.00724.x
Fang, J., Li, Y., Zhu, B., Liu, G., and Zhou, G. (2004). Community structures and species richness in the montane rain forest of Jianfengling, Hainan Island, China. Chin. Biodivers. 12, 29–43.
Feldmann, E., Glatthorn, J., Ammer, C., and Leuschner, C. (2020). Regeneration dynamics following the formation of understory gaps in a Slovakian beech virgin forest. Forests 11:585. doi: 10.3390/f11050585
Freschet, G. T., Bellingham, P. J., Lyver, P. O. B., Bonner, K. I., and Wardle, D. A. (2013). Plasticity in above- and belowground resource acquisition traits in response to single and multiple environmental factors in three tree species. Ecol. Evol. 3, 1065–1078. doi: 10.1002/ece3.520
Gao, Y., Du, F., Wang, Y., Wang, Y., Li, W., and Shi, H. (2016). Interspecific competition of six succession series species with different soil moisture treatments in loess hilly region. Res. Soil Water Conserv. 23, 192–197.
Hedges, L. V., Gurevitch, J., and Curtis, P. S. (1999). The meta-analysis of response ratios in experimental ecology. Ecology 80, 1150–1156. doi: 10.1890/0012-9658(1999)080[1150:TMAORR]2.0.CO;2
Hogan, J. A., Sharpe, J. M., Van Beusekom, A., Stankavich, S., Carmona, S. M., Bithorn, J. E., et al. (2022). Solar radiation and soil moisture drive tropical forest understory responses to experimental and natural hurricanes. Ecosphere 13:11543. doi: 10.1002/ecs2.4150
Hu, M., Liu, Y., Sun, Z., Zhang, K., Liu, Y., Miao, R., et al. (2018). Fire rather than nitrogen addition affects understory plant communities in the short term in a coniferous-broadleaf mixed forest. Ecol. Evol. 8, 8135–8148. doi: 10.1002/ece3.4263
Inoue, T., Akaji, Y., and Noguchi, K. (2022). Distinct responses of growth and respiration to growth temperatures in two mangrove species. Ann. Bot. 129, 15–28. doi: 10.1093/aob/mcab117
Kitajima, K. (1994). Relative importance of photosynthetic traits and allocation patterns as correlates of seedling shade tolerance of 13 tropical trees. Oecologia 98, 419–428. doi: 10.1007/BF00324232
Kitao, M., Lei, T. T., Koike, T., Tobita, H., and Maruyama, Y. (2000). Susceptibility to photoinhibition of three deciduous broadleaf tree species with different successional traits raised under various light regimes. Plant Cell Environ. 23, 81–89. doi: 10.1046/j.1365-3040.2000.00528.x
Kneeshaw, D. D., and Bergeron, Y. (1998). Canopy gap characteristics and tree replacement in the southeastern boreal forest. Ecology 79, 783–794. doi: 10.1890/0012-9658(1998)079[0783:CGCATR]2.0.CO;2
Kong, D.-X., Li, Y.-Q., Wang, M.-L., Bai, M., Zou, R., Tang, H., et al. (2016). Effects of light intensity on leaf photosynthetic characteristics, chloroplast structure, and alkaloid content of Mahonia bodinieri (Gagnep.) Laferr. Acta Physiol. Plant. 38:120. doi: 10.1007/s11738-016-2147-1
Lambers, H., Chapin, F. S., and Pons, T. L. (1998). Plant physiological ecology. New York, NY: Springer-Verlag. doi: 10.1007/978-1-4757-2855-2
Landhausser, S. M., and Lieffers, V. J. (2001). Photosynthesis and carbon allocation of six boreal tree species grown in understory and open conditions. Tree Physiol. 21, 243–250. doi: 10.1093/treephys/21.4.243
Lee, C. M., Kwon, T.-S., and Cheon, K. (2017). Response of ground beetles (Coleoptera: Carabidae) to forest gaps formed by a typhoon in a red pine forest at Gwangneung Forest, Republic of Korea. J. Forestry Res. 28, 173–181. doi: 10.1007/s11676-016-0291-5
Letcher, S. G., Lasky, J. R., Chazdon, R. L., Norden, N., Joseph Wright, S., Meave, J. A., et al. (2015). Environmental gradients and the evolution of successional habitat specialization: A test case with 14 Neotropical forest sites. J. Ecol. 103, 1276–1290. doi: 10.1111/1365-2745.12435
Li, Y. (2002). “Tropical forests and biodiversity of Jianfengling region,” in Research and conservation of tropical forest and the biodiversity. A special reference to Jianfengling, Hainan Island, eds Y. Li, B. Chen, and G. Zhou (Beijing: China Forestry Publishing House), 44–133.
Li, Y., Mwangi, B., Zhou, S., Liu, S., Zhang, Q., Liu, J., et al. (2021). Effects of typhoon Mangkhut on a monsoon evergreen broad-leaved forest community in Dinghushan nature reserve, lower subtropical China. Front. Ecol. Evol. 9:692155. doi: 10.3389/fevo.2021.692155
Liaw, A., and Wiener, M. (2002). Classification and regression by random forest. R News 2, 18–22. Available online at: https://cogns.northwestern.edu/cbmg/LiawAndWiener2002.pdf
Lin, T.-C., Hamburg, S. P., Hsia, Y.-J., Lin, T.-T., King, H.-B., Wang, L.-J., et al. (2003). Influence of typhoon disturbances on the understory light regime and stand dynamics of a subtropical rain forest in northeastern Taiwan. J. For. Res. 8, 139–145. doi: 10.1007/s10310-002-0019-6
Liu, B., Pan, L., and Xue, L. (2012). A review of the effect of typhoon on forests. Acta Ecol. Sin. 32, 1596–1605. doi: 10.5846/stxb201012231832
Liu, F., Zhang, M., Yang, W., Liu, Y., Wang, W., Zheng, J., et al. (2012). Leaf functional traits and trait relationships of tropical woody vegetation in relation to successional stage: Shifts in understory and canopy layers. Ecoscience 19, 198–208. doi: 10.2980/19-3-3499
Liu, F., Yang, W., Zhang, M., Liu, Y., Zheng, J., Wang, W., et al. (2010). Does strategy of resource acquisition in tropical woody species vary with life form, leaf texture, and canopy gradient? Eur. J. For. Res. 129, 1093–1108. doi: 10.1007/s10342-010-0394-z
Lohbeck, M., Poorter, L., Martinez-Ramos, M., and Bongers, F. (2015). Biomass is the main driver of changes in ecosystem process rates during tropical forest succession. Ecology 96, 1242–1252. doi: 10.1890/14-0472.1
Malhi, Y., and Marthews, T. R. (2013). “Tropical forests:: Carbon, climate and biodiversity,” in Law, tropical forests and carbon: The Case of REDD+, eds C. MacKenzie, C. McDermott, and R. Lyster (Cambridge: Cambridge University Press), 26–43. doi: 10.1017/CBO9781139236904.004
Marcelis, L. F. M., Heuvelink, E., and Goudriaan, J. (1998). Modelling biomass production and yield of horticultural crops: A review. Sci. Hortic. 74, 83–111. doi: 10.1016/S0304-4238(98)00083-1
Marenco, R. A., Gonçalves, J. F. D. C., and Vieira, G. (2001). Leaf gas exchange and carbohydrates in tropical trees differing in successional status in two light environments in central Amazonia. Tree Physiol. 21, 1311–1318. doi: 10.1093/treephys/21.18.1311
Martinez-Garza, C., Pena, V., Ricker, M., Campos, A., and Howe, H. F. (2005). Restoring tropical biodiversity: Leaf traits predict growth and survival of late-successional trees in early-successional environments. For. Ecol. Manage. 217, 365–379. doi: 10.1016/j.foreco.2005.07.001
Matsuo, T., Martinez-Ramos, M., Bongers, F., van der Sande, M. T., and Poorter, L. (2021). Forest structure drives changes in light heterogeneity during tropical secondary forest succession. J. Ecol. 109, 2871–2884. doi: 10.1111/1365-2745.13680
Mazzochini, G. G., and Camargo, J. L. C. (2020). Understory plant interactions along a successional gradient in Central Amazon. Plant Soil 450, 81–92. doi: 10.1007/s11104-019-04100-2
McCarthy, M. C., and Enquist, B. J. (2007). Consistency between an allometric approach and optimal partitioning theory in global patterns of plant biomass allocation. Funct. Ecol. 21, 713–720. doi: 10.1111/j.1365-2435.2007.01276.x
Miller, A. D., Roxburgh, S. H., and Shea, K. (2011). How frequency and intensity shape diversity-disturbance relationships. Proc. Natl. Acad. Sci. U.S.A. 108, 5643–5648. doi: 10.1073/pnas.1018594108
Muscolo, A., Bagnato, S., Sidari, M., and Mercurio, R. (2014). A review of the roles of forest canopy gaps. J. Forestry Res. 25, 725–736. doi: 10.1007/s11676-014-0521-7
Myers, J. A., and Kitajima, K. (2007). Carbohydrate storage enhances seedling shade and stress tolerance in a neotropical forest. J. Ecol. 95, 383–395. doi: 10.1111/j.1365-2745.2006.01207.x
Olivero-Lora, S., Rojas-Sandoval, J., Melendez-Ackerman, E. J., and Orengo-Rolon, J. L. (2022). Hurricane driven changes in vegetation structure and ecosystem services in tropical urban yards: A study case in San Juan, Puerto Rico. Urban Ecosyst. 25, 1431–1444. doi: 10.1007/s11252-022-01236-5
Parrish, J. A. D., and Bazzaz, F. A. (1982). Niche responses of early and late successional tree seedlings on three resource gradients. Bull. Torrey Bot. Club 109, 451–456. doi: 10.2307/2996486
Pollastrini, M., Brüggeman, W., Fotelli, M., and Bussotti, F. (2022). Downregulation of PSI regulates photosynthesis in early successional tree species. Evidence from a field survey across European forests. J. Photochem. Photobiol. 12:100145. doi: 10.1016/j.jpap.2022.100145
Poorter, H., and Nagel, O. (2000). The role of biomass allocation in the growth response of plants to different levels of light, CO2, nutrients and water: A quantitative review. Aust. J. Plant Physiol. 27, 595–607. doi: 10.1071/PP99173
Prescott, C. E. (2002). The influence of the forest canopy on nutrient cycling. Tree Physiol. 22, 1193–1200. doi: 10.1093/treephys/22.15-16.1193
Qi, X., Cao, K.-F., and Feng, Y.-L. (2004). Photosynthetic acclimation to different growth light environments in seedlings of three tropical rainforest Syzygium species. Zhiwu Shengtai Xuebao 28, 31–38. doi: 10.17521/cjpe.2004.0005
Quero, J. L., Villar, R., Marañón, T., and Zamora, R. (2006). Interactions of drought and shade effects on seedlings of four Quercus species: Physiological and structural leaf responses. New Phytol. 170, 819–834. doi: 10.1111/j.1469-8137.2006.01713.x
R Core Team (2015). R: A language and environment for statistical computing. Vienna: R Foundation for Statistical Computing.
Ramos, G., and Grace, J. (1990). The effects of shade on the gas exchange of seedlings of four tropical trees from Mexico. Funct. Ecol. 4, 667–677. doi: 10.2307/2389735
Reich, P. B., Walters, M. B., and Ellsworth, D. S. (1992). Leaf life-span in relation to leaf, plant, and stand characteristics among diverse ecosystems. Ecol. Monogr. 62, 365–392. doi: 10.2307/2937116
Rosseel, Y. (2010). lavaan: An R package for structural equation modeling and more Version 0.5-10 (BETA). Ghent: Ghent University.
Schweizer, D., Gilbert, G. S., and Holl, K. D. (2013). Phylogenetic ecology applied to enrichment planting of tropical native tree species. For. Ecol. Manage. 297, 57–66. doi: 10.1016/j.foreco.2013.02.012
Shafiq, I., Hussain, S., Raza, M. A., Iqbal, N., Ahsan Asghar, M., Raza, A., et al. (2021). Crop photosynthetic response to light quality and light intensity. J. Integr. Agric. 20, 4–23. doi: 10.1016/S2095-3119(20)63227-0
Sheng, D.-Y., Zhuang, X.-Y., Xu, H., Li, Y.-D., Hu, Y.-H., Shi, L.-L., et al. (2012). Community structure of endemic woody plants in tropical montane rainforest of Jianfengling, Hainan Island, China. Chin. J. Plant Ecol. 36, 935–947. doi: 10.3724/SP.J.1258.2012.00935
Souza, R. P., and Válio, I. F. (2003). Seedling growth of fifteen Brazilian tropical tree species differing in successional status. Braz. J. Bot. 26, 35–47. doi: 10.1590/S0100-84042003000100005
Takafumi, H., Kawase, S., Nakamura, M., and Hiura, T. (2010). Herbivory in canopy gaps created by a typhoon varies by understory plant leaf phenology. Ecol. Entomol. 35, 576–585. doi: 10.1111/j.1365-2311.2010.01216.x
Tang, J., Sun, B., Cheng, R., Shi, Z., Luo, D., Liu, S., et al. (2021). The effect of low irradiance on leaf nitrogen allocation and mesophyll conductance to CO2 in seedlings of four tree species in subtropical China. Plants 10:2213. doi: 10.3390/plants10102213
Thakur, M. P., Risch, A. C., and van der Putten, W. H. (2022). Biotic responses to climate extremes in terrestrial ecosystems. iScience 25:104559. doi: 10.1016/j.isci.2022.104559
Tobita, H., Utsugi, H., Kitao, M., Kayama, M., Uemura, A., Kitaoka, S., et al. (2010). Variation in photoinhibition among Sasa senanensis, Quercus mongolica, and Acer mono in the understory of a deciduous broad-leaved forest exposed to canopy gaps caused by typhoons. Trees 24, 307–319. doi: 10.1007/s00468-009-0400-6
Toledo-Aceves, T., and Swaine, M. D. (2008). Biomass allocation and photosynthetic responses of lianas and pioneer tree seedlings to light. Acta Oecol. 34, 38–49. doi: 10.1016/j.actao.2008.03.003
Tourville, J. C., Wason, J. W., and Dovciak, M. (2022). Canopy gaps facilitate upslope shifts in montane conifers but not in temperate deciduous trees in the Northeastern United States. J. Ecol. 110, 2870–2882. doi: 10.1111/1365-2745.13993
Trauernicht, C., Ticktin, T., and Herrera, G. L. (2006). Cultivation of non-timber forest products alters understory light availability in a humid tropical forest in Mexico. Biotropica 38, 428–436. doi: 10.1111/j.1744-7429.2006.00143.x
Tsai, H.-C., Chiang, J.-M., McEwan, R. W., and Lin, T.-C. (2018). Decadal effects of thinning on understory light environments and plant community structure in a subtropical forest. Ecosphere 9:e02464. doi: 10.1002/ecs2.2464
Tsvuura, Z., Griffiths, M. E., Gunton, R. M., Franks, P. J., and Lawes, M. J. (2010). Ecological filtering by a dominant herb selects for shade tolerance in the tree seedling community of coastal dune forest. Oecologia 164, 861–870. doi: 10.1007/s00442-010-1711-4
Valladares, F., Wright, S. J., Lasso, E., Kitajima, K., and Pearcy, R. W. (2000). Plastic phenotypic response to light of 16 congeneric shrubs from a Panamanian rainforest. Ecology 81, 1925–1936. doi: 10.1890/0012-9658(2000)081[1925:PPRTLO]2.0.CO;2
Wang, L., and Lin, T.-C. (2019). Forests affected by frequent and intense typhoons challenge the intermediate disturbance hypothesis. Biotropica 51, 797–801. doi: 10.1111/btp.12711
Wang, Y., Tao, J., Liu, J., and He, Z. (2012). Response of leaf functional traits to different light regimes in an evergreen broad-leaved forest in the Jinyun Mountain. Sci. Silvae Sin. 48, 23–29.
Wang, Z., Jiang, L., Gao, J., Qing, S., Pan, C., Wu, Y., et al. (2022). The influence of microhabitat factors on the regeneration and species composition of understory woody plants in Pinus tabuliformis plantations on the Loess Plateau. For. Ecol. Manage. 509:120080. doi: 10.1016/j.foreco.2022.120080
Westerband, A. C., and Horvitz, C. C. (2015). Interactions between plant size and canopy openness influence vital rates and life-history tradeoffs in two neotropical understory herbs. Am. J. Bot. 102, 1290–1299. doi: 10.3732/ajb.1500041
Xi, W., Peet, R. K., Lee, M. T., and Urban, D. L. (2019). Hurricane disturbances, tree diversity, and succession in North Carolina Piedmont forests, USA. J. Forestry Res. 30, 219–231. doi: 10.1007/s11676-018-0813-4
Yamashita, N., Ishida, A., Kushima, H., and Tanaka, N. (2000). Acclimation to sudden increase in light favoring an invasive over native trees in subtropical islands, Japan. Oecologia 125, 412–419. doi: 10.1007/s004420000475
Yan, E.-R., Wang, X.-H., and Huang, J.-J. (2006). Shifts in plant nutrient use strategies under secondary forest succession. Plant Soil 289, 187–197. doi: 10.1007/s11104-006-9128-x
Yang, H., Liu, S., Cao, K., Wang, J., Li, Y., and Xu, H. (2017). Characteristics of typhoon disturbed gaps in an old-growth tropical montane rainforest in Hainan Island, China. J. Forestry Res. 28, 1231–1239. doi: 10.1007/s11676-017-0402-y
Yang, H. B., An, S. Q., Sung, O. J., Shi, Z. M., She, X. S., Sun, Q. Y., et al. (2008). Seasonal variation and correlation with environmental factors of photosynthesis and water use efficiency of Juglans regia and Ziziphus jujuba. J. Integr. Plant Biol. 50, 210–220. doi: 10.1111/j.1744-7909.2007.00391.x
Yang, W., Liu, F., Zhou, L., Zhang, S., and An, S. (2011). Trade-offs between growth and survival of non-pioneer light-demanding tree seedlings in tropical forest of Hainan Island, China. J. Trop. Ecol. 27, 611–620. doi: 10.1017/S0266467411000435
Yao, A.-W., Chiang, J.-M., McEwan, R., and Lin, T.-C. (2015). The effect of typhoon-related defoliation on the ecology of gap dynamics in a subtropical rain forest of Taiwan. J. Veg. Sci. 26, 145–154. doi: 10.1111/jvs.12217
Zhou, L., Hong, Y., Li, C., Lu, C., He, Y., Shao, J., et al. (2020). Responses of biomass allocation to multi-factor global change: A global synthesis. Agric. Ecosyst. Environ. 304:107115. doi: 10.1016/j.agee.2020.107115
Zhou, L., Zhou, X., Zhang, B., Lu, M., Luo, Y., Liu, L., et al. (2014). Different responses of soil respiration and its components to nitrogen addition among biomes: A meta-analysis. Glob. Change Biol. 20, 2332–2343. doi: 10.1111/gcb.12490
Keywords: light condition, successional stage, seedling growth, photosynthetic properties, biomass allocation
Citation: Zhou L, Thakur MP, Jia Z, Hong Y, Yang W, An S and Zhou X (2023) Light effects on seedling growth in simulated forest canopy gaps vary across species from different successional stages. Front. For. Glob. Change 5:1088291. doi: 10.3389/ffgc.2022.1088291
Received: 03 November 2022; Accepted: 30 December 2022;
Published: 11 January 2023.
Edited by:
Uttam Kumar Sahoo, Mizoram University, IndiaReviewed by:
Qing-Wei Wang, Institute of Applied Ecology (CAS), ChinaBhupendra Singh, VCSG Uttarakhand University, India
Copyright © 2023 Zhou, Thakur, Jia, Hong, Yang, An and Zhou. This is an open-access article distributed under the terms of the Creative Commons Attribution License (CC BY). The use, distribution or reproduction in other forums is permitted, provided the original author(s) and the copyright owner(s) are credited and that the original publication in this journal is cited, in accordance with accepted academic practice. No use, distribution or reproduction is permitted which does not comply with these terms.
*Correspondence: Wenjie Yang, eWFuZ3dlbmppZUBtYWlsLmh6YXUuZWR1LmNu; Shuqing An,
YW5zaHFAbmp1LmVkdS5jbg==