- 1Natural Resources Canada, Canadian Forest Service, Laurentian Forestry Centre, Québec, QC, Canada
- 2Department of Plant, Soil and Microbial Sciences, Michigan State University, East Lansing, MI, United States
- 3Department of Forestry, Michigan State University, East Lansing, MI, United States
Oak wilt is a vascular disease of oak trees caused by the fungus Bretziella fagacearum. Once infected, trees may die in a few weeks. Although the disease is currently only found in the United States, it has been reported within just a few hundred meters of the Canada–USA border. To limit the establishment and spread of oak wilt in Canada, the development of an on-site, quick and reliable method to detect B. fagacearum is critical. In this study, we developed and validated a new qPCR TaqMan® assay that can detect B. fagacearum in a laboratory setting with great specificity and sensitivity. Using this test as a reference, we also developed and validated a new DETECTR assay that can detect B. fagacearum under 1 h from a variety of environmental samples, such as mycelium mats and insect vectors, using minimal laboratory equipment. While there are still some limitations to the sensitivity of this assay, we believe that its ease of use, flexibility and accuracy will provide an essential tool in efforts to reduce the spread of oak wilt.
1 Introduction
Oak wilt is a vascular disease of oak trees (Quercus spp.) caused by the fungus Bretziella fagacearum, previously known as Ceratocystis fagacearum (Beer et al., 2017). First described in the 1940’s (Henry, 1944; Bretz, 1953), disease symptoms appear after the pathogen enters the sapwood and disrupts the xylem vessels, therefore preventing water and nutrient transport (French and Stienstra, 1980). The affected trees eventually die, sometimes within just a few months of infection. For instance, red oaks (section Lobatae) are highly susceptible to the disease and can die in a matter of weeks, forever altering urban landscapes, plantations, and natural ecosystems (Gibbs and French, 1980).
Dispersion of B. fagacearum to healthy trees happens by underground or aboveground transmission. Underground transmission involves the movement of B. fagacearum through naturally occurring root grafts and accounts for short distance spread and the creation of expanding oak wilt pockets. The connected xylem vessels of the root grafts allow the fungus to travel from an infected tree to adjacent healthy oaks. Aboveground transmission occurs mainly through spores dissemination via insect vectors, mostly by Nitidulidae species (sap beetles) such as Colopterus truncatus and Carpophilus sayi (Cease and Juzwik, 2001). Sap beetles are attracted to fragrant sporulating fungal mats formed on bark cracks on the trunk of oak wilt killed trees or by mat bearing firewood (Juzwik et al., 2008). They can later transmit spores to wounded healthy trees, up to 600 meters away within a single year (Shelstad et al., 1991).
Currently, oak wilt disease is only found in Texas, midwestern and eastern states in the United States (USDA-Forest Service Northern Research Station, and Forest Health Protection, 2019), where it was potentially introduced many years ago (Juzwik et al., 2008). However, there has been a continuous northward spread of the disease, with an increasing number of infected counties being reported at the Canada–USA border (Jensen-Tracy et al., 2009).
Of the 200 oak species existing in the world, only 10 can be found in Canada, mainly located in Ontario and Eastern Canada (Lacoursière, 2015). They include species from red oaks, white oaks (section Quercus) and chestnut oaks (section Quercus), all of whom are susceptible to B. fagacearum infections to various levels (Gibbs and French, 1980; OWTAC, 2019).
As climate change progresses, insect vectors could possibly carry the fungus across the border and eventually spread to all of Eastern Canada, an area now climatically suitable for B. fagacearum and numerous potential vectors. In fact, the climate in southern Ontario has already been found appropriate for B. fagacearum, C. truncatus, and C. sayi (Pedlar et al., 2020). Additionally, despite the regulatory measures already in place, oak wilt could enter Canada through the transportation of contaminated logs (CFIA, 2020). The spread of oak wilt to Canada may lead to large-scale mortality of urban trees, the devastation of natural ecosystems and could cripple the oak log industry, with estimated economic losses of CDN$ 400 million (Pedlar et al., 2020).
Since it is not possible to cure infected trees, management of oak wilt is focused on preventing additional spread and involves the establishment of root graft barriers and trench inserts, removal of potential spore-producing trees, preventive fungicide injections, a ban on firewood transportation between different areas and restricted oak tree wounding activities to certain periods of the year (O’Brien et al., 2000; Wilson, 2001; Koch et al., 2010). The European Union is also tightening its restrictions when trading oak logs with bark from the USA (EFSA et al., 2020). For these efforts to be successful, rapid confirmation of infection is critical.
Diseased trees can tentatively be identified due to wilted leaves and defoliation. Unfortunately, those early symptoms are common among other abiotic and biotic issues, such as oak leaf blister and drought (Natural Resources Canada [NRCAN], 2015; Moore et al., 2022). Therefore, lab-based identification of B. fagacearum from symptomatic plant samples is required to confirm oak wilt. The need for a lab confirmation results in delays to the implementation of mitigating measures and increased associated costs. B. fagacearum may be present in logs or firewood from oak wilt killed trees, and may also persist in the root system of cut down diseased trees for several years and go unnoticed (Juzwik et al., 2008). The need to develop an on-site, quick and reliable method to detect B. fagacearum is now becoming more and more pressing in order to limit the establishment and spread of oak wilt.
Quantitative real-time PCR (qPCR) has become the gold standard in pathogen detection in many fields, including invasive alien tree pathogens, facilitating the detection of infinitesimal amounts of target DNA within a large quantity of environmental material (Heid et al., 1996). However, while qPCR is highly specific and sensitive, it is time-consuming, costly and requires specialized equipment, i.e., real-time PCR machine and technical expertise. Efforts have been made to miniaturize this piece of equipment, and various companies have commercialized portable real-time PCR instruments like the Franklin™ by Biomeme (Biomeme, Philadelphia, PA, USA), facilitating point-of-care detection. In a recent study, the Franklin™ machine, was shown to reliably detect forest pests and pathogens such as Sphaerulina musiva, Phytophthora ramorum, Lymantria dispar, and Cronartium sp. in the field (Capron et al., 2020). Other studies have also demonstrated on-site molecular detection using a variety of portable machines and sample types (Brown et al., 2020; Zowawi et al., 2021). These portable machines are robust, allow multiplexing and can also use previously developed lab-validated assays (Capron et al., 2020). However, with a price around US $10 0000, the cost of the portable real-time PCR machine can hamper its deployment.
In recent years, a novel technique called recombinase polymerase amplification (RPA) was developed, which can exponentially amplify a DNA target with a sensitivity, specificity, and a reliability comparable to that of a standard PCR (Piepenburg et al., 2006). The RPA reaction exploits the activity of recombinase proteins that bind to oligonucleotide primers and scan the double stranded DNA for complementary sequence. The recombinase then inserts the primers via strand exchange, and a single-strand DNA binding protein binds to the displaced strand to stabilize the loop. A polymerase can then initiate polymerization from the primers if the target sequence is present. Companies like TwistDx have packaged RPA reactions in very convenient lyophilized tubes, making the technology even more accessible (TwistDx, Cambridge, UK). RPA is fast and works at a constant low temperature, two features making this technology highly desirable for outdoor deployment since the use of a simple thermal block can now be considered. RPA has been used for the rapid detection of viruses (Euler et al., 2012a; Boyle et al., 2013; Yang et al., 2017), bacteria (Euler et al., 2012b; Ahmed et al., 2014) and fungi (Ahmed et al., 2015; Karakkat et al., 2018).
It has recently been shown that RPA can be combined with CRISPR/Cas technologies leading to different detection methods. DNA endonuclease-targeted CRISPR trans reporter (DETECTR) is one of these new detection methods. DETECTR uses RPA enzymes combined with Cas12a enzyme from the clustered regularly interspaced short palindromic repeats (CRISPR) platform (Chen et al., 2018). This innovative detection tool exploits the collateral trans-cleavage activity of an activated Cas12a, which will, upon recognition of a dsDNA target sequence, cleave ssDNA non-specifically at multiple random sites. By adding a modified ssDNA reporter in the reaction, a positive signal, i.e., the presence of the original target DNA sequence, can be detected by various methods, such as lateral flow assays or fluorometry (Chen et al., 2018). DETECTR assays have already been used in the detection and/or diagnosis of various pathogens and cancer (Arora et al., 2020; Zhang et al., 2020), most notably for the betacoronavirus severe acute respiratory syndrome (SARS)-CoV-2 (Broughton et al., 2020; Ding et al., 2020; Lucia et al., 2020). Similar assays have also been recently tested, to a lesser extent, on bacterial and viral plant diseases (Luo et al., 2021; Singh et al., 2022).
This study therefore aimed at developing and validating a DETECTR assay targeting the internal transcribed spacer (ITS) gene of B. fagacearum. The specificity and sensitivity of this detection test were evaluated and compared to a newly designed qPCR TaqMan® assay for a variety of environmental sample types, namely mycelial mats and insect samples. A panel of participants was also used to optimize the DETECTR assay for on-site deployment. We hope that future implementation of this detection test with phytosanitary inspectors and other end users will effectively limit the spread of oak wilt in Canada and the USA.
2 Materials and methods
2.1 Isolates, DNA extraction and sample preparation
DNA from B. fagacearum and closely related species was obtained from a previously published study (Lamarche et al., 2015) and is listed in Supplementary Table 1. To confirm the species identity of all samples, the ITS gene, recognized as the universal DNA barcode for fungi (Schoch et al., 2012), was amplified and sequenced. A genus-specific SYBR Green-based qPCR assay (Table 1) was designed in a conserved region of the ITS gene to perform an absolute quantification (Rutledge, 2011) of all the strains and to standardize their concentration when necessary.
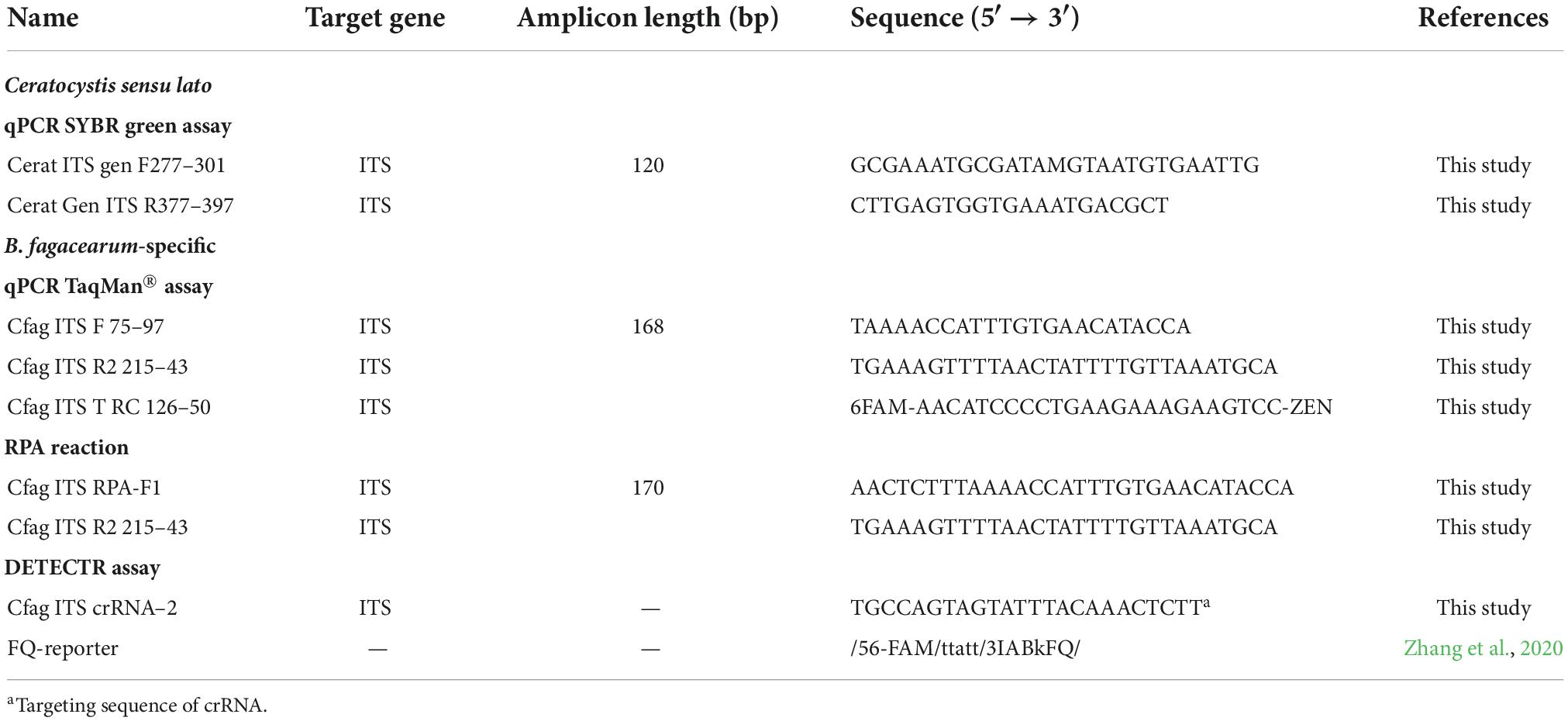
Table 1. Primers and probe used for genus-specific qPCR SYBRGreen, species-specific qPCR TaqMan®, and DETECTR assays.
Fresh cultures of B. fagacearum were also isolated from wood tissue of various infected Quercus spp. in the Lower Peninsula of Michigan in 2020. Isolates were grown on acidified potato dextrose agar (PDA; Difco™, Sparks, MD, USA) for 2 weeks at room temperature, and hyphal-tip purified. One mL of Lactic acid, 85% (JT Baker, Phillipsburg, NJ, USA) was added into 1L of PDA. Mycelium was then harvested, frozen in liquid nitrogen, and ground with a mortar and pestle. Subsequent DNA extraction followed the protocol described before (Parada-Rojas and Quesada-Ocampo, 2018). All samples were quantified using the SYBR Green assay and are listed in Supplementary Table 1.
2.2 Novel target-specific TaqMan®-based real-time PCR assay design
To benchmark the DETECTR assay, we first developed a TaqMan®-based real-time PCR assay. A total of 37 published ITS sequences for B. fagacearum and closely related species were aligned (Supplementary File 2). A set of primers and a probe were then designed in regions showing B. fagacearum-specific nucleotide polymorphisms. All primers were designed using previously described criteria and amplifications were conducted under the conditions described in Lamarche et al. (2015; Table 1). Positive (using B. fagacearum DNA from pure culture isolate C520, Supplementary Table 1) and negative (no DNA template) controls were included in all qPCR runs.
2.3 Novel target-specific DETECTR assay design
The DETECTR assay was performed using RPA to amplify fungal DNA with the TwistAmp Basic Kit (TwistDx, Cambridge, UK), and LbaCas12a (New England Biolabs, MA, USA) for the DETECTR part of the assay. RPA primers (Integrated DNA Technologies Inc., Coralville, IA, USA) were adapted from the TaqMan® qPCR assay following the TwistAmp Basic kit manual and are listed in Table 1. Amplification was achieved with a few modifications to the manufacturer’s instructions. Briefly, each reaction was prepared using 29.5 μL of TwistAmp Rehydration buffer, 2.75 μL of forward and reverse primers (10 μM) and 10.8 μL of nuclease-free water. The mix was used to resuspend the reaction pellets provided with the TwistAmp Basic kit. Then, 1 μL of DNA template and 3.2 μL of 280 mM magnesium acetate were added in a final volume of 50 μL. Tubes were quickly vortexed and incubated at 37°C for 20 min, followed by inactivation at 65°C for 10 min.
The LbaCas12a (Cas12a from Lachnospiraceae bacterium ND2006) trans-cleavage assay was performed similarly to those previously described (Chen et al., 2018; Zhang et al., 2020). Cas12a targeting sequence was selected according to the presence of PAM for Lba Cas12a (TTTV) (Zetsche et al., 2015). Guide RNA and ssDNA FQ-reporter are listed in Table 1 and were designed following recommendations from NEB (New England Biolabs, MA, USA) as well as previous studies (Zetsche et al., 2015; Zhang et al., 2020). Both were ordered from Integrated DNA Technology (IDT Inc., Coralville, IA, USA). To detect the DNA target via fluorescence, Cas12a digestion reactions were prepared using 1X NEBuffer 2.1, 50 nM of LbaCas12a, 50 nM guide RNA and 250 nM FQ-reporter in a final volume of 60 μL. The mix was pre-incubated at room temperature for 10 min. Then, 6 μL of unpurified RPA product was added to the reaction and transferred to a 96-well black optical-bottom plate (Thermo Fisher Scientific, MA, USA). Fluorescence was measured at 37°C every 5 min for 1 h (excitation: 485 nm, emission: 535 nm) using the Fluoroskan Ascent plate reader (Labsystems).
2.4 Validating the specificity and sensitivity of the qPCR TaqMan® assay
To validate the specificity of the newly designed qPCR TaqMan® for B. fagacearum, we ran the assay in triplicates on 31 B. fagacearum strains from pure cultures as well as a panel of closely related species (section “DNA from pure cultures,” Supplementary Table 1). Closely related species’ DNA samples were standardized to approximately 5,000 ITS copies with the SYBR Green assay. Since high DNA concentrations are known to sometimes inhibit amplification, we wanted to ensure that, if samples were not detected, it would be due to the specificity of the assay, and not because of potential inhibition.
In order to evaluate the sensitivity of the qPCR TaqMan® assay, we built standard curves using serial dilutions of B. fagacearum ITS synthetic DNA target sequence, and calculated the limit of detection (LOD), which is the smallest amount of target DNA that can be detected 95% of the time with the assay (Bustin et al., 2009). Briefly, 10-fold serial dilutions of B. fagacearum ITS copies were made from a gBlocks™ gene fragment (IDT Inc., Coralville, IA, USA) comprising a segment of the B. fagacearum ITS sequence, encompassing the two TaqMan® assay primers (Table 1) (ref GenBank: KC305152.1). The ITS concentration of each dilution was calculated using the TaqMan® primers and SYBR Green reagents. The qPCR TaqMan® assay was then run in triplicates for each dilution and a standard curve was obtained by plotting the Ct values against the log value of the number of ITS copies. The LOD was determined by running 20 replicates of the smallest dilution of the standard curve giving 3 positive results.
2.5 Efficiency of two DNA extractions methods using the qPCR TaqMan® assay
To assess the efficiency of two DNA extraction methods, standard curves were also built using DNA extracted with 2 protocols from serial dilutions of B. fagacearum conidia. A conidial suspension was prepared by growing isolate MIFCC41 on acidified PDA for 2 weeks at room temperature (Chahal et al., 2019). Conidia were harvested by flooding pure cultures with 70% ethanol, followed by two filtrations using a double layer of Miracloth (Miracloth, EMD Millipore, Billerica, MA, USA). The concentration of conidia in the suspension was determined using a hemocytometer (6.18 × 107 conidia per mL). Conidia were plated onto acidified PDA to confirm their non-viability, sedimented by centrifugation, and then sent to the Tanguay Lab at the Laurentian Forestry Centre, Canada. Conidia were then resuspended in 1 mL of sterile distilled water, disaggregated by repeatedly pushing them through a 24-gauge needle, and counted with a hemocytometer. Concentration was adjusted to 10E6 conidia per mL, and the stock solution was diluted following a series of 10-fold dilutions in water, down to 1000 conidia per mL. Aliquots of 10 μL from each concentration were dried using a SpeedVac, and then extracted using two different methods. First, to speed-up and facilitate the on-site detection process, samples were immersed in 100 μL of the QuickExtract™ Plant DNA Extraction Solution (Lucigen, WI, USA) and were immediately heated to 65°C for 6 min, followed by 98°C for 2 min. Extracted samples were stored at −20°C until use. As a comparison, we also extracted DNA from the conidia dilutions using the QIAamp DNA micro kit (Qiagen, Valencia, CA, USA) according to the manufacturer’s recommendations. The qPCR TaqMan® assay was run in triplicates with both sets of conidia dilutions and standard curves were obtained by plotting the Ct values against the log value of the number of conidia.
2.6 Performance of the DETECTR and TaqMan® qPCR assays on environmental samples
We ran the DETECTR along with the qPCR TaqMan® (in triplicates) on the same samples to see if the source of the material would impact the performance of the assay. The list of all environmental samples, and their origins, used to validate both of our new assays, is shown in Supplementary Table 1. DNA from B. fagacearum mycelial mat samples, which ranged from development stages 0 to 5 (Chahal et al., 2021) was extracted with 200 μL of QuickExtract™ Plant DNA Extraction Solution (Lucigen, Wisconsin, USA). DNA samples from various insect vectors carrying B. fagacearum spores (Carpophilus sayi, Epuraea corticina, Glischrochilus sanguinolentus) were obtained from Lamarche et al. (2015). DNA from other insect samples not carrying B. fagacearum spores was also included to ensure the specificity of both tests (Bergeron et al., 2019).
2.7 Adapting DETECTR protocol for on-site deployment
In order to facilitate the on-site deployment of this DETECTR assay, a few modifications were made to simplify its use outside of laboratory settings (Figure 1). Most notably, each step of the protocol was carried out in a microtube of a specific color, incubation times were reduced to the minimum and reactions were lyophilized when possible. The DNA extraction step using Lucigen buffer was the same as described above, except that only 100 μL of buffer was prealiquoted in red microtubes. The RPA reaction components (TwistAmp Rehydration buffer, forward and reverse primers, MgOAc and ddH2O) were aliquoted in green microtubes with the addition of 5% trehalose. Cas12a digestion reactions were assembled in blue microtubes with a few modifications: reactions were prepared in a final volume of 50 μl, FQ-reporter concentration was increased to 500 nM and 5% of trehalose was added. The RPA and Cas12a reactions were lyophilized overnight at −50°C in a FreeZone 2.5 Liter freeze-dryer (Labconco, Kansas City, MO, USA) and stored at −20°C until use.
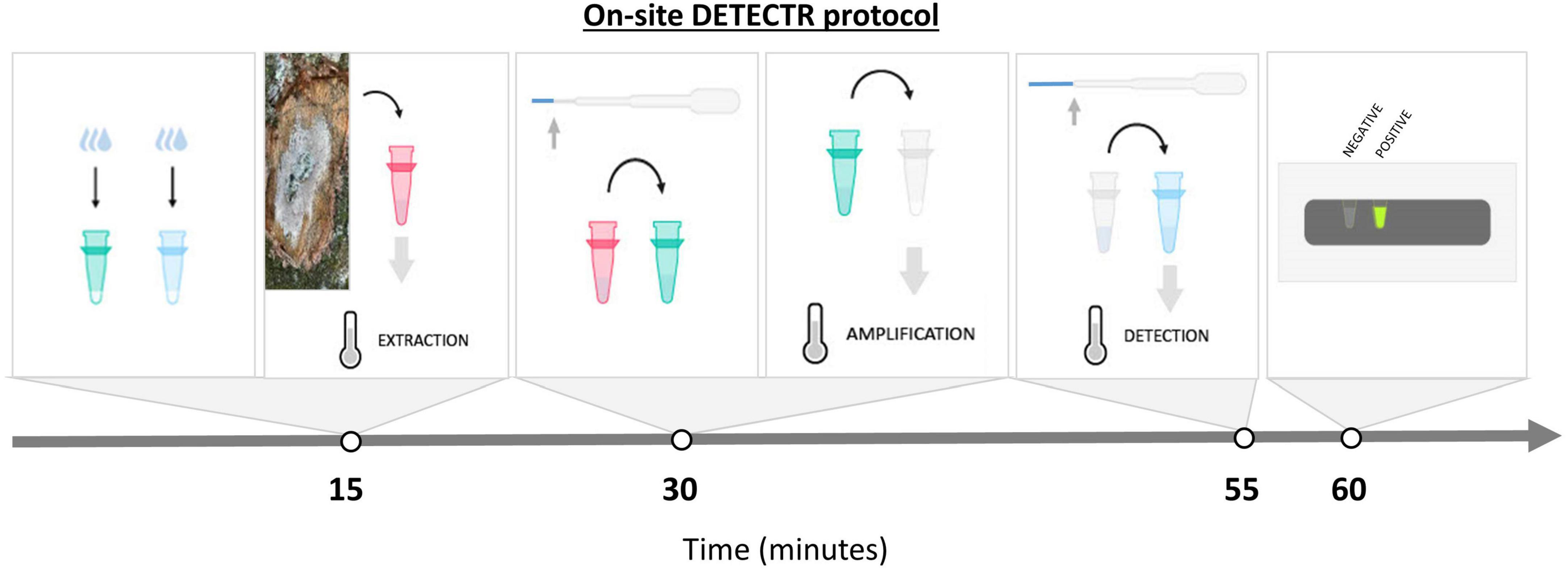
Figure 1. Summary of the new and simplified on-site DETECTR assay. Amplification of target DNA by RPA, and detection by trans-cleavage release of fluorescence from the ssDNA FQ-reporter following CRISPR/CAS12a recognition of the target DNA. Each step is carried out in a specific colored microtube. All liquid handling is done via a simple transfer pipette. Reagents are either stable at room temperature or have been previously lyophilized to facilitate the deployment of the test. The equipment needed to perform the test is minimal and easy to carry into the field. A complete description of the protocol is available in Supplementary File 3.
Just before use, each of the RPA and Cas12a lyophilized reactions tubes were rehydrated with water (approx. 48 μL, or 3 drops) using a 1.5 mL extended fine tip transfer pipette (Samco™ Fine Tip Transfer Pipettes, 231PK, Thermo Fisher Scientific, MA, USA). A small piece of mycelium mat was inserted in red microtubes containing the QuickExtract™ buffer, and samples were then incubated at 65°C for 6 min, followed by 98°C for 2 min in a portable mini16 Thermal cycler (miniPCR Bio, Cambridge, MA, USA). Using the same transfer pipette, the extracted DNA was diluted about 10-fold when transferred to the green microtubes. The entire content of the green microtubes was then used to rehydrate the RPA reaction pellets provided with the TwistAmp Basic Kit (TwistDx, Cambridge, UK). Samples were incubated at 37°C for 10 min without further inactivation. Part of the RPA reactions (approx. 8 μL) was then transferred to the blue microtubes. Samples were incubated at 37°C for 20 min, and fluorescence was visualized with the P51™ Molecular Fluorescence Viewer (miniPCR Bio, Cambridge, MA, USA). Each sample’s fluorescence was compared to that of the negative control, which was run along the assay. The complete protocol for the on-site DETECTR assay is illustrated in Figure 1 and listed in (Supplementary File 3).
2.8 Human trial to validate the simplified DETECTR assay
To evaluate the level of difficulty in implementing the DETECTR on-site assay with non-laboratory end users, we organized a trial consisting of 22 volunteers with various backgrounds (from non-scientific to basic/advanced laboratory skills). Each participant was given a detailed version of the on-site protocol to read beforehand (Supplementary File 3), from DNA extraction to fluorescence read-out. A demonstration as well as clarifications were offered on the day of the trial. A total of 3 randomized samples (positive fungal mats or negative fungi) as well as a negative (no template) DNA control were given to each volunteer. Participants were told to use the on-site DETECTR assay to detect the presence of B. fagacearum in their samples. Various parameters were investigated, including time to completion and accuracy of results. Suggested improvements and recommendations from participants were also considered when designing the final written version of the simplified on-site protocol (Supplementary File 3).
2.9 Statistical analysis
Analysis was conducted using R (R Core Team, 2014), RStudio (R Studio Team, 2018). The standard curves, that represent the relationships between the outcome (Ct values) and the predictors (either ITS copies or the number of extracted conidia) were evaluated by simple regression using the lm function in the R statistical package.
Performance of the DETECTR assay was compared to the qPCR reference by constructing confusion matrices for each type of samples. The python package pycm (Haghighi et al., 2018) was used to generate the tables and calculate the accuracy. The statistical comparison was performed using the mcnemar function of the statsmodels package (Seabold and Perktold, 2010) with default parameters.
3 Results
3.1 Design and validation of a new B. fagacearum gold standard qPCR TaqMan® assay
We first designed a new qPCR TaqMan® assay that could specifically detect B. fagacearum but also act as a reference for our new molecular DETECTR assay. Using closely related species listed in Supplementary Table 1 (see section “DNA from pure cultures”), our data shows amplification only for B. fagacearum DNA. There was no amplification of any sister species isolates, making this new qPCR TaqMan® assay 100% specific in discriminating B. fagacearum among the other closely related species selected here. Of note, some very highly concentrated DNA samples from pure cultures had to be diluted first to be detectable, most probably due to the presence of inhibitors or a DNA concentration too high. Nonetheless, amplification results were proportional to the amount of DNA present in the samples.
We then assessed the sensitivity of the qPCR TaqMan® assay and calculated its LOD. First, we ran the assay in triplicates on serial dilutions of the gBlocks™ ITS gene fragments. The standard curve obtained by plotting the Ct values against the log value of the number of ITS copies shows a square correction coefficient (R2) of 0.98 and a LOD of 2 ITS copies (Figure 2A). The LOD was determined by re-running 20 times the last dilution that gave 3 positive detection results, giving a positive detection 18/20 runs. In all cases, Ct values were proportional to the amount of template DNA used for the qPCR reactions.
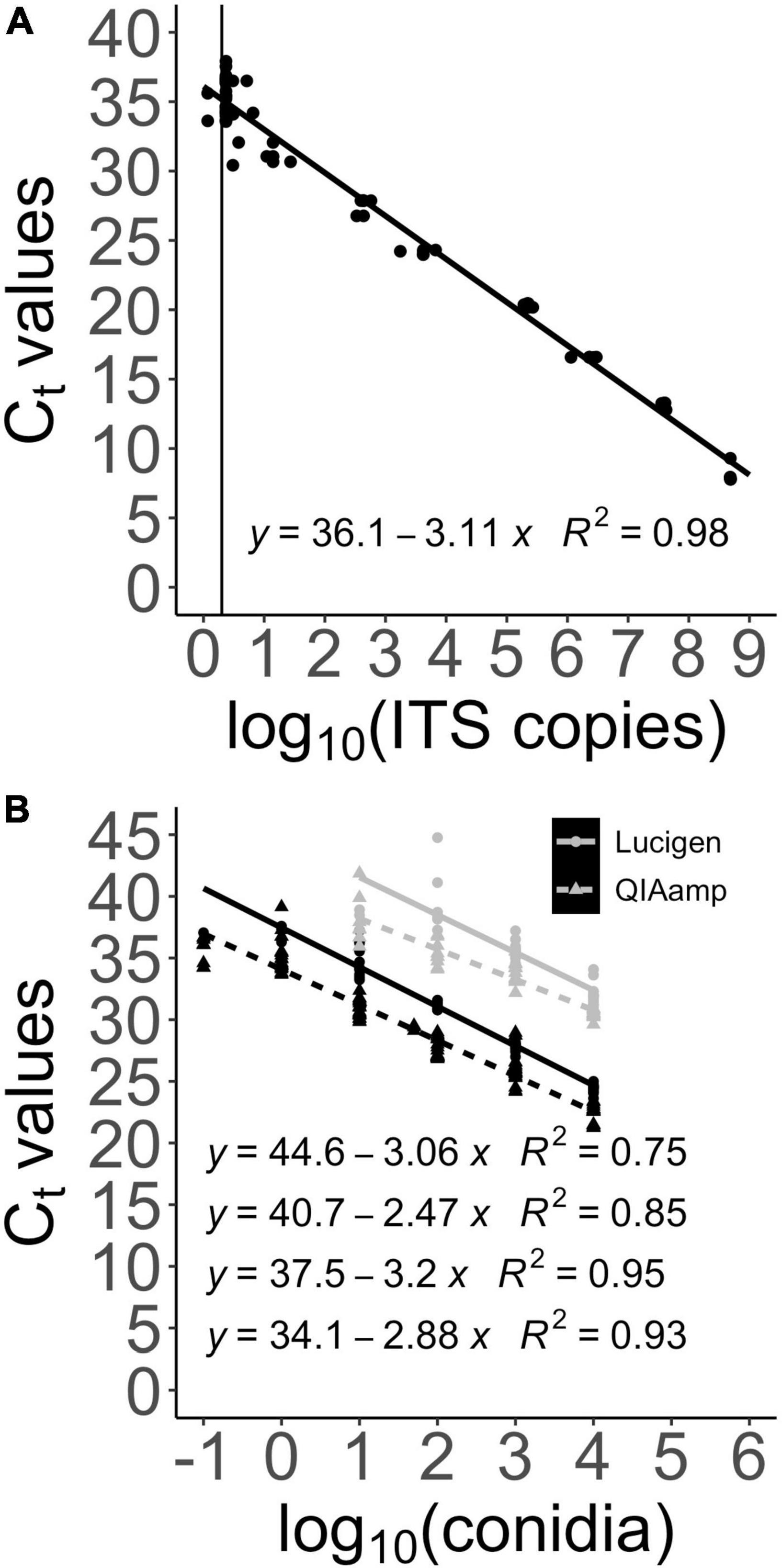
Figure 2. Sensitivity of the new qPCR TaqMan® detection test against B. fagacearum and comparison of DNA extraction methods. Ct values obtained from running the qPCR TaqMan® assay are plotted against the log value of the number of ITS copies (gBlocks™ gene fragment) and conidia. Equations and coefficients R2 are displayed on the graphs. (A) LOD of the newly designed qPCR TaqMan® assay on ITS copies (gBlocks™ gene fragment) is 2 ITS copies. (B) Comparison of the new qPCR TaqMan® detection test (black) and the old qPCR TaqMan® designed in the TEF region (gray) to detect B. fagacearum DNA extracted from various amount of conidia using the Lucigen (circle points) and QIAamp (triangle points) procedures.
3.2 Comparison of DNA extraction methods using the qPCR TaqMan® assay and a previously designed assay
The assay was also run on two series of dilutions of conidia DNA extracted with the QIAamp and Lucigen DNA extraction kits. Figure 2B shows the standard curves obtained, with R2 ranging from 0.93 to 0.95. The difference between the two DNA extraction methods is clearly showed by a 3.4 Ct shift upward with the Lucigen method, making it about 10 times less sensitive than the QIAamp method, but easier to deploy.
In parallel, another goal when designing the B. fagacearum ITS qPCR TaqMan® assay was to replace the previous qPCR detection assay that our laboratory had developed and published in 2015 (Lamarche et al., 2015), which, while being specific, lacked in sensitivity. The previous detection assay targeted the transcription elongation factor 1-a (TEF) gene instead of the ITS region. Figure 2B shows a comparison between the two detection assays using the two DNA extraction methods above mentioned. As seen clearly on the graph, results confirm that our new B. fagacearum qPCR assay allowed us to gain a downshift of 6.6 Ct (with the QIAamp extraction), which corresponds to a 97-fold increase in sensitivity when run on conidia dilutions. This result was expected since TEF is a single copy gene, while the ITS is multi-copy. The increase in sensitivity suggests that the B. fagacearum genome possesses about 100 copies of the nuclear ribosomal DNA.
3.3 Design and validation of a new molecular DETECTR assay
The specificity of the DETECTR assay was evaluated with the same sister species isolates used with the qPCR TaqMan® assay (see “DNA from pure cultures,” Supplementary Table 1). This new molecular test is not meant to be quantitative and therefore only determines if a sample is “positive” or “negative.” As for our qPCR TaqMan® assay, the DETECTR test only gave a positive result for B. fagacearum, whereas every other closely related species came up negative following a fluorescence reading on a plate reader. The new DETECTR test is therefore 100% specific for B. fagacearum according to the panel of species tested.
To estimate the sensitivity of this non-quantitative test, we ran it on various B. fagacearum sample types that could be naturally found in the environment, for instance, fungal mats and insect vectors known to carry spores of the pathogen (see samples in Supplementary Table 1). We also investigated the conidia dilution set extracted with the Qiagen method, as well as DNA samples from pure cultures (Supplementary Table 1). Figure 3 represents a scatter plot graph comparing the sensitivities of the qPCR TaqMan® and DETECTR assays for all sample types combined. Results show that the DETECTR assay does not span the same detection range as that of the reference qPCR TaqMan® assay. As described by Bustin et al. (2009), sensitivity refers to the minimum number of copies in a sample that can be measured accurately with an assay, and it is typically expressed as the LOD. While we didn’t run the DETECTR assay on predefined amounts of copies as we did with the qPCR TaqMan® assay to determine the LOD, plotting all the data from this study as shown in Figure 3 suggests that the DETECTR test is roughly a 1000 times less sensitive than the qPCR TaqMan® assay. As described above, LOD is only dependant on the target copy number, which we were able to obtain for all types of samples. Sample type and DNA preparation will, however, impact the amount of target copies being present in a sample.
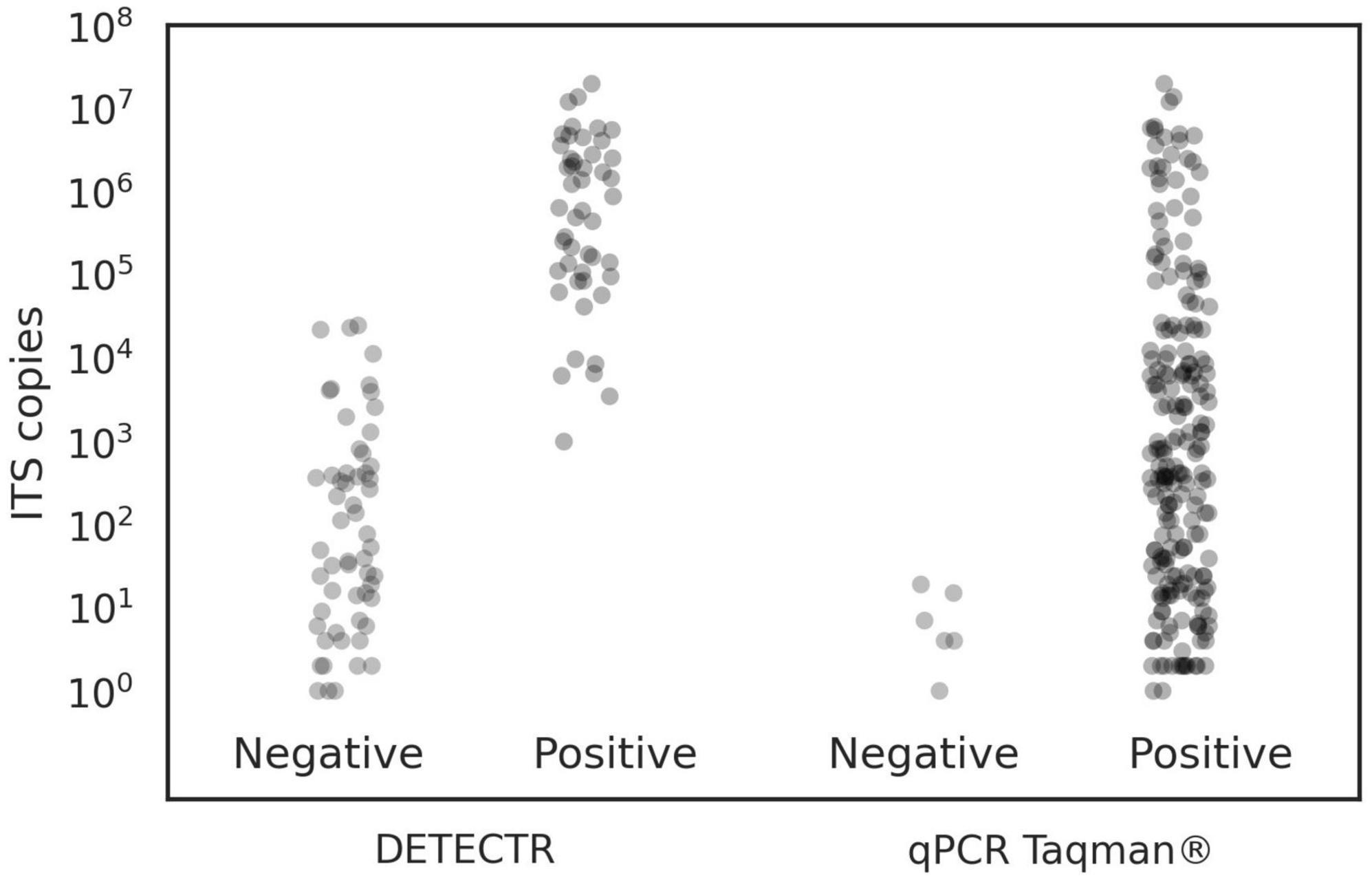
Figure 3. Comparison of the qPCR TaqMan® and DETECTR assays sensitivity when detecting various sample types. The scatter plot depicted here suggests that the lower detection limit of the DETECTR test is much higher than that of the qPCR TaqMan®.
In order to further validate and compare our new DETECTR assay to the qPCR reference, we looked into their respective detection results of these samples. Supplementary Table 1 shows results obtained with both tests for the same sample, and Table 2 summarizes the outcome of the comparison using a confusion matrix. Results were exactly the same for both assays when investigating DNA from pure cultures and DNA from insect vectors, with positive and negative results matching perfectly, giving an accuracy of 1 in both circumstances. In a confusion table, the accuracy represents the number of all correct predictions divided by the total number of the dataset. Therefore, the best possible case is an accuracy of 1 and indeed shows here that DETECTR results for pure DNA cultures and insects thoroughly match the qPCR “reference” results. However, while the qPCR TaqMan® assay detected a positive signal for every mycelial mat tested, the DETECTR assay missed 5 of the late-stage samples, therefore giving 5 false-negative results. This slightly lowered the accuracy of the test to 0.84375, but with a negligible difference (McNemar’s exact test, P = 0.0625). Those mats were also late-stage mats (stages 4A and 5), with the expectation that extracted DNA amount and quality would be lower than fresh mats. SYBR Green quantification of those samples indeed confirmed that very few ITS copies were present. Hence, the DETECTR test can still be considered as a reliable assay when applied to young and fresh mycelial mats.
3.4 Assessing how user friendly the on-site assay is and improving it using a panel of participants
In order to facilitate the deployment of the new DETECTR assay designed here, a few modifications were made to the original protocol, as described in section “2.6 Performance of the DETECTR and TaqMan® qPCR assays on environmental samples.” To ensure that every end user with any experience background could handle this novel on-site detection assay, we ran a trial combining 22 participants with or without previous laboratory experience. The average time to completion was 66.6 min, where people with a scientific background fared slightly better regarding the time taken to perform the test. Accuracy of results obtained by these beta participants compared to the qPCR TaqMan® results are shown in the bottom part of the confusion matrix (Table 2). The accuracy of the DETECTR assay was calculated at just above 80% when compared to the qPCR reference. However, a total of 12 false-negative and one false-positive results (cross contamination from a manipulation) were recorded, lowering the P-value of the McNemar test below 0.05.
The trial was also used to gather feedback from participants in order to make the on-site DETECTR test more user-friendly and easily deployable. Their suggestions and comments strongly shaped the final version of the protocol presented in (Supplementary File 3). The factors and challenges most commonly pointed out by the participants were the wording and nomenclature of the protocol (i.e., replace technical terms like “microtube, resuspend, incubation,” etc. with non-scientific terms) and the manipulation of the transfer pipette. All participants agreed that performing the protocol a second time would be much easier and that mistakes would most certainly be avoided then.
4 Discussion
In this study, we report a new field detection assay using DETECTR technology that can confirm the presence of B. fagacearum on-site within 1 h. We first developed and validated a traditional qPCR TaqMan® assay that could detect specifically B. fagacearum and act as a reference when testing the new assay. We then designed and optimized the new DETECTR assay and benchmarked it to our gold standard qPCR TaqMan® assay. In addition, we used a panel of participants from various scientific backgrounds to estimate the ease of deployment of the DETECTR test with different users.
We strongly believe that biosurveillance of invasive forest pests would benefit from on-site DNA testing capability, hence the need to develop deployable, quick and reliable detection tests. Unfortunately, rapid detection is currently hampered by the requirement to send samples to a laboratory for confirmation. With the continued spread of oak wilt in the USA and concern over its introduction into Canada, rapid, on-site detection of B. fagacearum is critical.
The qPCR TaqMan® assay developed here is specific to B. fagacearum, with no cross-amplification, and is more sensitive than our previously reported assay (Lamarche et al., 2015). Unlike the nested PCR reported by Yang and Juzwik (2017), this assay is quantitative, allowing an estimation of the biomass load of the target species in given samples, and does not require a post amplification step to visualize the results. McLaughlin et al. (2022) recently published a very interesting study where Yang’s double-nested PCR successfully detected the oak wilt fungus from insects trapped in various locations in New York State, US. However, as mentioned before, this method not only requires sorting the content of the traps beforehand, it also needs more time than our qPCR TaqMan® assay to obtain results. Nonetheless, while this test is highly sensitive and very accurate, it can either be performed in laboratory settings or deployed using expensive and fragile equipment.
The on-site DETECTR assay designed here could detect DNA purified from axenic cultures of B. fagacearum with the same specificity as the qPCR TaqMan® assay. We successfully validated the DETECTR assay on environmental samples of mycelial mats, both fresh and older ones, as well as insect vectors. Although the sensitivity of the DETECTR assay was not as good as that of the qPCR TaqMan® test for very old mycelium mats, we still believe it can act as a useful tool to deploy and screen apparent symptoms in the field. Indeed, the current best way to identify B. fagacearum on insects is a timely culture-based method requiring 2–4 weeks before B. fagacearum can be confirmed. And while confirmation of B. fagacearum is straightforward from fresh mats (the only diagnostic sign), it can be difficult from older, decayed mats, also requiring laborious culture work and microscopy. Moreover, since mycelial mats develop once the tree has been killed from a B. fagacearum infection, earlier detection in still living plant tissues such as wood and wilted twigs would increase the usefulness of the test and reduce disease spread and tree loss. Work is already underway in our laboratory to assess this possibility.
Additionally, results from insect vectors and serial dilution of spores clearly showed a relationship between spore loads and detection results. We found that target pathogen DNA concentrations that are lower than 10E4 copies most often yield false negatives detection results when using the DETECTR assay. Efforts to increase the quantity and quality of the DNA extracted might help circumvent this limitation. However, most protocols are lengthy, requiring more time than the Lucigen extraction method used here, and necessitate bulky laboratory equipment, such as a vortex or a centrifuge, which cannot be easily deployed out in the field. A recent study showed that B. fagacearum DNA could be detected from oak wilt infected wood chips using a simple alkaline DNA extraction procedure (Moore et al., 2022). The compatibility of other simple and deployable protocols reported for the DNA extraction of pathogens from symptomatic plants (Capron et al., 2020; Paul et al., 2020) with the DETECTR approach developed here is also being tested in our laboratory.
To facilitate the ease and speed of use and reduce costs of the DETECTR test, we simplified the whole process by using color-coded tubes, dehydrated pre-packed reagents, and disposable transfer pipets along with an affordable battery-powered heating block and a simple blue light box (see Supplementary File 3). The resulting kit is easily usable in any environment harboring a small flat surface to work on.
The panel of beta participants that had never performed this assay before, the majority of whom lacked laboratory experience, were able to use the kits and correctly identify 80% of samples as either a positive B. fagacearum target specimen or as a negative non-target specimen. These results suggest that by providing minimal training to phytosanitary inspectors, they could achieve very accurate on-site detection of the oak wilt pathogen. Previous investigators streamlined detection assays for on-site detection by end users (Capron et al., 2020). However, this study is one of the rare examples where the accuracy of a test is determined with a panel of untrained and inexperienced participants. We found that comments received from the panel improved the assay and the related protocol, mostly by simplifying the wording for non-scientific people and adding a few extra explanations.
Streamlining the assay by reducing the number of steps required would further facilitate the ease of use and time taken, but also potentially increase accuracy (less steps leading to fewer mistakes). Currently, the RPA amplification and Cas12a cleavage of the fluorescent ssDNA probe are being carried sequentially in two different tubes. However, recent publications have successfully combined the reagents of these two reactions into a single tube and performed the two reactions simultaneously (Chen et al., 2020; de Puig et al., 2021). We tested a one-step reaction with pure DNA according to Chen et al. (2020) protocol. In this preliminary work, the fluorescent signal was very weak and required an increased amount of DNA template as well as longer incubation times, which makes this method less suitable for a rapid on-site detection at this time (data not shown).
One major benefit of the DETECTR based detection is the ability to easily design assay components. In fact, in the present paper, primer F1, used for the RPA, consisted of a longer version of the primer used in the TaqMan® assay (primer extended of a few bases in 5′), primer R2 was the same for both assays, and the gRNA was straightforwardly designed based on PAM site within the amplified region. Therefore, we believe that relatively little effort would be required to implement DETECTR assays from available TaqMan® assays containing discriminatory nucleotides. This is especially true if primers contain target specific SNPs, and the sequence amplified contains PAM sites with target specific polymorphisms in the 3′ end of the crRNA. On the other hand, and unlike TaqMan® technology, multiplexing multiple assays into one reaction tube is not possible due to the unspecific ssDNA trans-nuclease activity of the RNP complex upon binding to the target crRNA site.
In conclusion, we report in this study the development and validation of a new DETECTR assay detecting the presence of B. fagacearum in mycelial mats and insect vectors, both common environmental sample types. This reliable and cost-effective test has been optimized to be rapidly deployed on-site. We expect that training front line people such as wood industry workers and phytosanitary inspectors on how to use the DETECTR assay in the field will help prevent the establishment of oak wilt in Canada and reduce its spread in the USA. The qPCR TaqMan® assay designed in parallel is reliable, has a high sensitivity and can confidently be used to confirm results in a laboratory setting.
Data availability statement
The datasets presented in this study can be found in online repositories. The names of the repository/repositories and accession number(s) can be found in the article/Supplementary material.
Author contributions
PT, M-KG, and ÉB designed the study. DS performed the bioinformatics analyses. AP, ÉB, and M-KG performed the lab experiments. KC collected the field material. PT and ÉB conducted the statistical analyses. M-KG and PT drafted the initial manuscript with contributions from ÉB, AP, KC, and MS. All authors contributed to the article and approved the submitted version.
Funding
Findings for this project were received through the Natural Resources Canada Expanding Market Opportunities and Pest Risk Management programs. KC was funded through the Michigan Invasive Species Grant Program, Michigan State University Project GREEEN and Forrest Strong endowments. Both KC and MS were supported by HATCH project MICL02505 from the USDA National Institute of Food and Agriculture.
Acknowledgments
We would like to thank all 22 participants of the trial conducted to optimize the on-site DETECTR protocol.
Conflict of interest
The authors declare that the research was conducted in the absence of any commercial or financial relationships that could be construed as a potential conflict of interest.
Publisher’s note
All claims expressed in this article are solely those of the authors and do not necessarily represent those of their affiliated organizations, or those of the publisher, the editors and the reviewers. Any product that may be evaluated in this article, or claim that may be made by its manufacturer, is not guaranteed or endorsed by the publisher.
Supplementary material
The Supplementary Material for this article can be found online at: https://www.frontiersin.org/articles/10.3389/ffgc.2022.1068135/full#supplementary-material
References
Ahmed, A., Linden van der, H., and Hartskeerl, R. A. (2014). Development of a recombinase polymerase amplification assay for the detection of pathogenic leptospira. Int. J. Environ. Res. 11, 4953–4964. doi: 10.3390/ijerph110504953
Ahmed, S. A., Sande, W. W. J., van de, Desnos-Ollivier, M., Fahal, A. H., Mhmoud, N. A., et al. (2015). Application of isothermal amplification techniques for identification of madurella mycetomatis, the prevalent agent of human mycetoma. J. Clin. Microbiol. 53, 3280–3285. doi: 10.1128/jcm.01544-15
Arora, R., Gupta, K., Vijaykumar, A., and Krishna, S. (2020). DETECTing merkel cell polyomavirus in merkel tumors. Front. Mol. Biosci. 7:10. doi: 10.3389/fmolb.2020.00010
Beer, Z. W., de, Marincowitz, S., Duong, T. A., and Wingfield, M. J. (2017). Bretziella, a new genus to accommodate the oak wilt fungus, Ceratocystis fagacearum (Microascales, Ascomycota). Mycokeys 27, 1–19. doi: 10.3897/mycokeys.27.20657
Bergeron, M.-J., Feau, N., Stewart, D., Tanguay, P., and Hamelin, R. C. (2019). Genome-enhanced detection and identification of fungal pathogens responsible for pine and poplar rust diseases. PLoS One 14:e210952. doi: 10.1371/journal.pone.0210952
Boyle, D. S., Lehman, D. A., Lillis, L., Peterson, D., Singhal, M., Armes, N., et al. (2013). Rapid detection of HIV-1 Proviral DNA for early infant diagnosis using recombinase polymerase amplification. mBio 4, e135–e113. doi: 10.1128/mbio.00135-13
Broughton, J. P., Deng, X., Yu, G., Fasching, C. L., Servellita, V., Singh, J., et al. (2020). CRISPR–Cas12-based detection of SARS-CoV-2. Nat. Biotechnol. 38, 870–874. doi: 10.1038/s41587-020-0513-4
Brown, A. T., McAloose, D., Calle, P. P., Auer, A., Posautz, A., Slavinski, S., et al. (2020). Development and validation of a portable, point-of-care canine distemper virus qPCR test. PLoS One 15:e0232044. doi: 10.1371/journal.pone.0232044
Bustin, S. A., Benes, V., Garson, J. A., Hellemans, J., Huggett, J., Kubista, M., et al. (2009). The MIQE guidelines: Minimum information for publication of quantitative Real-Time PCR experiments. Clin. Chem. 55, 611–622. doi: 10.1373/clinchem.2008.112797
Capron, A., Stewart, D., Hrywkiw, K., Allen, K., Feau, N., Bilodeau, G., et al. (2020). In situ processing and efficient environmental detection (iSPEED) of tree pests and pathogens using point-of-use real-time PCR. PLoS One 15:e0226863. doi: 10.1371/journal.pone.0226863
Cease, K. R., and Juzwik, J. (2001). Predominant nitidulid species (Coleoptera: Nitidulidae) associated with spring oak wilt mats in Minnesota. Can. J. For. Res. 31, 635–643. doi: 10.1139/x00-201
CFIA (2020). D-99-03: Phytosanitary import requirements to prevent the entry of oak wilt disease (Bretziella fagacearum (Bretz) Hunt) from the Continental United States. Available online at: https://inspection.canada.ca/plant-health/invasive-species/directives/forest-products/d-99-03/eng/1323852753311/1323852875523 (accessed April 11, 2022).
Chahal, K., Morris, O. R., McCullough, D. G., Cregg, B., and Sakalidis, M. L. (2021). Sporulation timing of the invasive oak wilt fungus, Bretziella fagacearum in Michigan, (Abstr.). Phytopathology 111:S2.51. doi: 10.1094/PHYTO111-10-S2.1
Chahal, K., Morris, O., McCullough, D. G., and Sakalidis, M. L. (2019). Biology, epidemiology and detection of oak wilt in Michigan. (Abstr). Phytopathology 109:S2.33. doi: 10.1094/PHYTO-109-10-S2.1
Chen, J. S., Ma, E., Harrington, L. B., Costa, M. D., Tian, X., Palefsky, J. M., et al. (2018). CRISPR-Cas12a target binding unleashes indiscriminate single-stranded DNase activity. Science 360, 436–439. doi: 10.1126/science.aar6245
Chen, Y., Mei, Y., Zhao, X., and Jiang, X. (2020). Reagents-loaded, automated assay that integrates recombinase-aided amplification and Cas12a nucleic acid detection for a point-of-care test. Anal. Chem. 92, 14846–14852. doi: 10.1021/acs.analchem.0c03883
de Puig, H., A., Lee, R., Najjar, D., Tan, X., Soeknsen, L., Angenent-Mari, N., et al. (2021). Minimally instrumented SHERLOCK (miSHERLOCK) for CRISPR-based point-of-care diagnosis of SARS-CoV-2 and emerging variants. Sci. Adv. 7:eabh2944. doi: 10.1126/sciadv.abh2944
Ding, X., Yin, K., Li, Z., Lalla, R. V., Ballesteros, E., Sfeir, M. M., et al. (2020). Ultrasensitive and visual detection of SARS-CoV-2 using all-in-one dual CRISPR-Cas12a assay. Nat. Commun. 11:4711. doi: 10.1038/s41467-020-18575-6
EFSA, P., On, P. H., Bragard, C., Dehnen-Schmutz, K., Serio, F. D., Jacques, M., et al. (2020). Commodity risk assessment of oak logs with bark from the US for the oak wilt pathogen Bretziella fagacearum under an integrated systems approach. EFSA J. 18:e06352. doi: 10.2903/j.efsa.2020.6352
Euler, M., Wang, Y., Nentwich, O., Piepenburg, O., Hufert, F. T., and Weidmann, M. (2012a). Recombinase polymerase amplification assay for rapid detection of rift valley fever virus. J. Clin. Virol. 54, 308–312. doi: 10.1016/j.jcv.2012.05.006
Euler, M., Wang, Y., Otto, P., Tomaso, H., Escudero, R., Anda, P., et al. (2012b). Recombinase polymerase amplification assay for rapid detection of Francisella tularensis. J. Clin. Microbiol. 50, 2234–2238. doi: 10.1128/jcm.06504-11
Gibbs, J. N., and French, D. W. (1980). The transmission of oak wilt. St Paul, MN: U.S. Department of Agriculture.
Haghighi, S., Jasemi, M., Hessabi, S., and Zolanvari, A. (2018). PyCM: Multiclass confusion matrix library in Python. J. Open Source Softw. 3:729. doi: 10.21105/joss.00729
Heid, C. A., Stevens, J., Livak, K. J., and Williams, P. M. (1996). Real time quantitative PCR. Genome Res. 6, 986–994. doi: 10.1101/gr.6.10.986
Jensen-Tracy, S., Kenaley, S., Hudler, G., Harrington, T., and Logue, C. (2009). First report of the oak wilt fungus, ceratocystis fagacearum, in New York state. Plant Dis. 93, 428–428. doi: 10.1094/pdis-93-4-0428b
Juzwik, J., Harrington, T. C., MacDonald, W. L., and Appel, D. N. (2008). The Origin of Ceratocystis fagacearum, the Oak wilt fungus. Annu. Rev. Phytopathol. 46, 13–26. doi: 10.1146/annurev.phyto.45.062806.094406
Karakkat, B. B., Hockemeyer, K., Franchett, M., Olson, M., Mullenberg, C., and Koch, P. L. (2018). Detection of root-infecting fungi on cool-season turfgrasses using loop-mediated isothermal amplification and recombinase polymerase amplification. J. Microbiol. Meth. 151, 90–98. doi: 10.1016/j.mimet.2018.06.011
Koch, K. A., Quiram, G. L., and Venette, R. C. (2010). A review of oak wilt management: A summary of treatment options and their efficacy. Urban For. Urban Green. 9, 1–8. doi: 10.1016/j.ufug.2009.11.004
Lacoursière, E. (2015). Oak, the Canadian encyclopedia. Available online at: https://www.thecanadianencyclopedia.ca/en/article/oak (accessed June 15, 2022).
Lamarche, J., Potvin, A., Pelletier, G., Stewart, D., Feau, N., Alayon, D. I. O., et al. (2015). Molecular detection of 10 of the most unwanted alien forest pathogens in Canada using real-time PCR. PLoS One 10:e0134265. doi: 10.1371/journal.pone.0134265
Lucia, C., Federico, P.-B., and Alejandra, G. C. (2020). An ultrasensitive, rapid, and portable coronavirus SARS-CoV-2 sequence detection method based on CRISPR-Cas12. bioRxiv [Preprint]. doi: 10.1101/2020.02.29.971127
Luo, M., Meng, F.-Z., Tan, Q., Yin, W.-X., and Luo, C.-X. (2021). Recombinase polymerase amplification/Cas12a-Based Identification of Xanthomonas arboricola pv. pruni on peach. Front. Plant Sci. 12:740177. doi: 10.3389/fpls.2021.740177
McLaughlin, K., Snover-Clift, K., Somers, L., Cancelliere, J., and Cole, R. (2022). Early detection of the oak wilt fungus (Bretziella fagacearum) using trapped nitidulid beetle vectors. For. Pathol. 52. doi: 10.1111/efp.12767
Moore, M. J., Juzwik, J., Saiapina, O., Ahmed, S., Yang, A., and Abbas, A. (2022). Use of sodium hydroxide DNA extraction methods for nested PCR detection of Bretziella fagacearum in the sapwood of Oak Species in Minnesota. Plant Health Prog. 23, 132–139. doi: 10.1094/php-03-21-0057-rs
Natural Resources Canada [NRCAN] (2015). Red oak. Available online at: https://tidcf.nrcan.gc.ca/en/trees/factsheet/66 (accessed July 21, 2022).
O’Brien, J., Mielke, M., Starkey, D., and Juzwik, J. (2000). How to identify, prevent and control oak wilt. St-Paul, MN: USDA Forest Service.
OWTAC (2019). Oak wilt response framework for Canada. Available online at: https://inspection.canada.ca/plant-health/invasive-species/plant-diseases/oak-wilt/response-framework/eng/1563898431188/1563898479048 (accessed July 21, 2022).
Parada-Rojas, C. H., and Quesada-Ocampo, L. M. (2018). Analysis of microsatellites from transcriptome sequences of Phytophthora capsici and applications for population studies. Sci. Rep. 8:5194. doi: 10.1038/s41598-018-23438-8
Paul, R., Ostermann, E., and Wei, Q. (2020). Advances in point-of-care nucleic acid extraction technologies for rapid diagnosis of human and plant diseases. Biosens. Bioelectron. 169, 112592–112592. doi: 10.1016/j.bios.2020.112592
Pedlar, J. H., McKenney, D. W., Hope, E., Reed, S., and Sweeney, J. (2020). Assessing the climate suitability and potential economic impacts of Oak wilt in Canada. Sci. Rep. 10:19391. doi: 10.1038/s41598-020-75549-w
Piepenburg, O., Williams, C. H., Stemple, D. L., and Armes, N. A. (2006). DNA detection using recombination proteins. PLoS Biol. 4:e204. doi: 10.1371/journal.pbio.0040204
Rutledge, R. G. (2011). A Java program for LRE-based real-time qPCR that enables large-scale absolute quantification. PLoS One 6:e17636. doi: 10.1371/journal.pone.0017636
Schoch, C. L., Seifert, K. A., Huhndorf, S., Robert, V., Spouge, J. L., Levesque, C. A., et al. (2012). Nuclear ribosomal internal transcribed spacer (ITS) region as a universal DNA barcode marker for Fungi. Proc. Natl. Acad. Sci. U.S.A 109, 6241–6246.
Seabold, S., and Perktold, J. (2010). “Econometric and statistical modeling with Python skipper seabold 1 1,” in Proceedings of the 9th python in science conference, Austin, TX.
Shelstad, D., Queen, L., French, D., and Fitzpatrick, D. (1991). Describing the spread of Oak Wilt using a geographic information system. Arboric. J. 17, 192–199. doi: 10.48044/jauf.1991.047
Singh, M., Bindal, G., Misra, C. S., and Rath, D. (2022). The era of Cas12 and Cas13 CRISPR-based disease diagnosis. Crit. Rev. Microbiol. 48, 714–729. doi: 10.1080/1040841x.2021.2025041
USDA-Forest Service Northern Research Station, and Forest Health Protection (2019). Alien forest pest explorer—Species map. Available online at: https://www.nrs.fs.fed.us/tools/afpe/maps (accessed April 11, 2022).
Wilson, A. D. (2001). Oak Wilt, a potential threat to southern and western oak forests. J. For. 99, 4–11.
Yang, A., and Juzwik, J. (2017). Use of nested and real-time PCR for the detection of ceratocystis fagacearum in the sapwood of diseased oak Species in Minnesota. Plant Dis. 101, 480–486. doi: 10.1094/pdis-07-16-0990-re
Yang, Y., Qin, X., Song, Y., Zhang, W., Hu, G., Dou, Y., et al. (2017). Development of real-time and lateral flow strip reverse transcription recombinase polymerase Amplification assays for rapid detection of peste des petits ruminants virus. Virol. J. 14:24. doi: 10.1186/s12985-017-0688-6
Zetsche, B., Gootenberg, J. S., Abudayyeh, O. O., Slaymaker, I. M., Makarova, K. S., Essletzbichler, P., et al. (2015). Cpf1 is a single RNA-Guided endonuclease of a class 2 CRISPR-Cas system. Cell 163, 759–771. doi: 10.1016/j.cell.2015.09.038
Zhang, Y., Zhang, Y., Zhang, Y., and Xie, K. (2020). Evaluation of CRISPR/Cas12a-based DNA detection for fast pathogen diagnosis and GMO test in rice. Mol. Breed. 40, 1–12. doi: 10.1007/s11032-019-1092-2
Keywords: DETECTR, molecular detection, oak wilt, Bretziella fagacearum, TaqMan® assay
Citation: Bourgault É, Gauthier M-K, Potvin A, Stewart D, Chahal K, Sakalidis ML and Tanguay P (2022) Benchmarking a fast and simple on-site detection assay for the oak wilt pathogen Bretziella fagacearum. Front. For. Glob. Change 5:1068135. doi: 10.3389/ffgc.2022.1068135
Received: 12 October 2022; Accepted: 30 November 2022;
Published: 15 December 2022.
Edited by:
Benoit Marçais, INRA Centre Nancy-Lorraine, FranceReviewed by:
Jaime Aguayo, Agence Nationale de Sécurité Sanitaire de l’Alimentation, de l’Environnement et du Travail (ANSES), FranceLuisa Ghelardini, University of Florence, Italy
Copyright © 2022 Bourgault, Gauthier, Potvin, Stewart, Chahal, Sakalidis and Tanguay. This is an open-access article distributed under the terms of the Creative Commons Attribution License (CC BY). The use, distribution or reproduction in other forums is permitted, provided the original author(s) and the copyright owner(s) are credited and that the original publication in this journal is cited, in accordance with accepted academic practice. No use, distribution or reproduction is permitted which does not comply with these terms.
*Correspondence: Philippe Tanguay, UGhpbGlwcGUuVGFuZ3VheUBucmNhbi1ybmNhbi5nYy5jYQ==
†These authors have contributed equally to this work and share first authorship