- 1Yale School of the Environment, Yale University, New Haven, CT, United States
- 2The New York Botanical Garden, Bronx, NY, United States
- 3Smithsonian Tropical Research Institute, Balboa, Panama
- 4Department of Biology, Faculty of Natural Sciences, Universidad del Rosario, Bogotá, Colombia
- 5Department of Biological Sciences, Marquette University, Milwaukee, WI, United States
Lianas are a quintessential tropical plant growth-form; they are speciose and abundant in tropical forests worldwide. Lianas compete intensely with trees, reducing nearly all aspects of tree performance. However, the negative effects of lianas on trees have never been combined and quantified for multiple tropical forests. Here, we present the first comprehensive standardized quantification of the effect of lianas on trees across tropical forests worldwide. We used data from 50 liana removal experiments and quantified the effect size of lianas on tree growth, biomass accretion, reproduction, mortality, leaf water potential, sap flow velocity, and leaf area index (LAI) across different forest types. Using a three-level mixed-effect meta-analysis, we found unequivocal evidence that lianas significantly reduce tree growth and biomass accretion in ecological, logging, and silvicultural studies. Lianas also significantly reduce tree reproduction, recruitment, and physiological performance. The relative detrimental effect of lianas on trees does not increase in drier forests, where lianas tend to be more abundant. Our results highlight the substantial liana-induced reduction in tree performance and biomass accumulation, and they provide quantitative data on the effects of lianas on trees that are essential for large-scale plant demographic and ecosystem models that predict forest change and carbon dynamics.
Introduction
Lianas (woody vines) are a diverse polyphyletic guild of woody climbing plants that add substantially to forest structure, complexity, diversity, and dynamics. Lianas can contribute up to 35% of the woody species and up to 25% of all woody stems in lowland tropical forests, respectively (Schnitzer et al., 2012). Lianas use trees (apically-dominant, self-supporting woody plants) for structural support to climb to the top of the forest canopy (Stevenson, 1927; Trimble and Tryon, 1974), where they deploy their foliage above that of their tree hosts (Kira and Ogawa, 1971; Putz, 1984; Medina-Vega et al., 2020), thus reducing the amount of light that would have been otherwise available for trees (Avalos et al., 1999; Clark et al., 2008). Indeed, liana-removal studies have shown that lianas reduce forest leaf area index (LAI) by up to 20% (Schnitzer and Carson, 2010; Rodríguez-Ronderos et al., 2016; Estrada-Villegas et al., 2020). Lianas also compete with trees for belowground resources, such as soil water and nutrients (Dillenburg et al., 1995). By reducing light and belowground resources, lianas significantly reduce tree survival (Ingwell et al., 2010), fecundity (García León et al., 2018), recruitment (Schnitzer and Carson, 2010), and growth (Pérez-Salicrup, 2001; Van Der Heijden and Phillips, 2009; Álvarez-Cansino et al., 2015).
The detrimental effect of lianas goes beyond individual trees; lianas reduce forest-level biomass sequestration and storage, and can reduce tree species diversity and alter tree species composition (Schnitzer, 2018). Lianas significantly reduce forest biomass sequestration, from 52% (César et al., 2016) up to 180% (Schnitzer et al., 2014). Lianas also modify where biomass is allocated; forest plots where lianas were removed allocated 44% of their biomass to woody tissues and 33% in leaves. By contrast, control plots where lianas were present allocated 29% of their biomass to woody tissues and an impressive 53% to leaves (Van Der Heijden et al., 2015). Therefore, lianas both reduce biomass accumulation and influence where biomass is stored, shifting biomass turnover toward leaves, which increases carbon and nutrient cycling (Van Der Heijden et al., 2015). Lianas also influence forest composition by reducing tree species richness in gaps (Schnitzer and Carson, 2010), and by decreasing population growth rates of some tree species more than others (Visser et al., 2018).
The detrimental effects of lianas on individual trees and forest communities may be stronger at sites where lianas are more abundant. Thus, in areas such as seasonal forests, where mean annual precipitation is low and month rainfall variability is high, liana communities are abundant and diverse (Schnitzer, 2005; Dewalt et al., 2015; Parolari et al., 2020) and can severely decrease tree growth and performance. In other words, lianas likely have a stronger detrimental effect on trees in areas where they are more abundant, assuming that their effect on trees scales with their abundance. Given that there are now 16 studies indicating that liana density, productivity, and biomass are all increasing relative to trees in tropical forests (Pandian and Parthasarathy, 2016; Ceballos and Malizia, 2017; Hogan et al., 2017; Venegas-González et al., 2020; Schnitzer et al., 2021), it is paramount to determine the magnitude at which lianas reduce tree performance and forest biomass, and how these effects change along important environmental gradients.
A recent review of 64 published liana removal experiments by Estrada-Villegas and Schnitzer (2018) provided strong support for the capacity of lianas to reduce tree growth, survival, recruitment, fecundity, and physiological performance. These experiments were conducted at a variety of temporal and spatial scales; from removals around targeted tree individuals from a single species and spanning a few days, to liana removals with thousands of trees comprising many species and spanning nearly three decades (Okali and Ola-Adams, 1987; Tobin et al., 2012; Kainer et al., 2014; César et al., 2016). The main conclusion, in accordance with that of other reviews (Isnard and Silk, 2009; Paul and Yavitt, 2011; Durán and Sánchez-Azofeifa, 2015), is that lianas reduce nearly all aspects of tree performance.
Despite the numerous reviews, no study up to date has quantified the direction and magnitude of the effect of lianas on trees for multiple liana removal experiments. While reviews from published literature have shown clear general trends on how lianas affect tree performance and forest ecosystem processes, there has not been an accurate quantification of such effects in a standardized fashion using multiple studies. In other words, there has been no previous attempt to combine data and use a meta-analytic approach to quantify the strength and direction of liana removal after accounting for the variability within and across experiments. Such quantification is critical to understand the role of lianas in tropical forests, as well as provide the essential parameters for global vegetation models (e.g., Di Porcia E Brugnera et al., 2019). Furthermore, lianas can have large negative effects on valuable tropical timber trees, but there has been no large-scale, standardized quantification of the effects of lianas in conjunction with other forms of forest manipulation common in forestry and silvicultural practices.
In this study, we standardized and combined data from 50 published ecological, forestry, and silvicultural liana-removal experiments from 16 countries in the African, American, and Asian tropics and subtropics to quantify the magnitude by which lianas reduce tree growth, biomass accumulation, and reproduction, as well as alter tree water potential, sap flow velocity, and LAI. By calculating the effect size for each experiment and combining these data, we provide the most accurate mean quantification of the direction and strength of the effect of lianas on tropical tree performance, as well as extrapolate these findings to tropical forest ecosystem functioning. Specifically, we addressed the following three main questions: (1) What is the combined standardized effect of lianas on tree performance (growth, biomass accumulation, reproduction, mortality, leaf water potential, sap flow velocity, and LAI) for ecological studies? (2) How strong is the negative effect of lianas on trees in logging and silvicultural practices? (3) Does the effect of lianas vary across forest with different levels of rainfall or among ecosystem types (i.e., life zone)?
Methods
Literature Selection
To conduct the meta-analysis, we used an extensive list of published literature on liana removal experiments assembled by Estrada-Villegas and Schnitzer (2018), and added six more experiments that were either missed by, or published after, the 2018 review. We assembled this list of papers using Google Scholar, the LianaEcologyProject.com, and Web of Science (for more details on the search methods see Estrada-Villegas and Schnitzer, 2018). We included only tropical and subtropical in situ experiments, and excluded greenhouse experiments and observational studies.
We categorized the studies according to their goal (ecology or forestry), and whether the liana manipulation was paired with another manipulation, such as tree removal and canopy thinning. We divided the literature in three categories of liana removal: (1) ecological; (2) logging; and (3) silvicultural (following Estrada-Villegas and Schnitzer, 2018). Ecological studies were those with a clear distinction between the liana removal and control (no liana removal) treatments across a range of forest types (from dry to seasonal to wet forests, and both old-growth and secondary forests). Logging studies were those where liana removal had been done in conjunction with logging treatments, such as tree girdling or poisoning; thus, the effect of liana removal was compounded with that of tree removal. Silvicultural studies included those where a liana removal manipulation was imposed either before or after logging operations. Silvicultural studies, for example, often include liana cutting as part of experimental treatments either before or after logging, and sought to determine whether logging damage or future logging yield was affected by liana removal. By contrast, the goal of ecological studies was typically to quantify the effect of liana removal on the performance of trees and on emergent ecosystem processes, while not explicitly accounting for previous land use (Estrada-Villegas and Schnitzer, 2018).
We further classified the selected studies according to response variables at the tree-level (tree biomass accretion, growth, leaf water potential, mortality, reproduction, sap flow) and plot-level (tree seedling and sapling recruitment, forest canopy openness, and LAI) (Rodríguez-Ronderos et al., 2016). For each study, we noted the ecosystem type (i.e., life zone) where the experiments were conducted (Holdridge, 1964) (Tropical Dry Forest, Tropical Moist Forest, Tropical Wet Forests, Subtropical Moist Forest, Subtropical Marsh), the mean annual precipitation, and the length of the study period when available. We excluded studies that omitted a measure of the variability around a measure of central tendency for the response variables (with a few exceptions, see “Data collection” below). We also omitted studies that did not specify data from a control treatment and studies that used unique response variables that precluded comparisons with other experiments.
Data Collection
We found a total of 50 studies that met our criteria. From each study and response variable, we extracted data on the sample size, the mean value from the liana removal experiment (with or without co-occurring forestry treatment), and mean value of the control treatment (no liana removal and no co-occurring forestry treatment). From each study, we also extracted the standard deviations of the response variables from both the liana removal and the control treatments. We used WebPlotDigitizer Version 4.3 (Rohatgi, 2020) to extract the necessary data from published figures. Standard errors were transformed into standard deviations, and when medians and quantiles were reported, we used the methods from Wan et al. (2014) to calculate means and standard deviations.
We extracted data that were aggregated across size classes and species. However, if the data were not aggregated in the original study, we extracted the values per species or size class. In a few cases, when variances were not reported, we obtained means and standard deviations by averaging the means of several size classes (e.g., Pinard and Putz, 1996), or averaging means among replicates of the same logging treatment (e.g., Dekker and De Graaf, 2003). Given that many studies had multiple censuses over time, we included all observations each time a mean and a deviation were reported (i.e., multiple observations). Noting multiple observations per study allowed us to incorporate the dynamic responses of trees and forests to liana removal through time within studies without losing important data by averaging responses across time (Cheung, 2019). We accounted for these non-independent repeated measurements in our data analyses. Data stored as Supplementary Material 1 in a digital repository (https://doi.org/10.6084/m9.figshare.14770095.v1).
Data Analysis
To determine the effect size of each observation, we calculated standardized mean differences using Hedges' g (Hedges and Olkin, 1985). Standardized mean differences express the magnitude of the response of the liana removal manipulation minus the unmanipulated controls, divided by the pooled standard deviation of both treatments. Standardized mean differences corrects for small sample sizes, and are suitable when there are negative values, such as leaf water potentials (Gurevitch et al., 2001; Koricheva et al., 2013). Positive standardized mean differences indicate that trees were benefited by liana removal.
We calculated the standardized mean difference and the variance for every observation (i.e., time interval) of every study with the function “escalc” from the R package metafor (Viechtbauer, 2010). We used these values in a series of three-level mixed effect meta-analytic models (Konstantopoulos, 2011; Midolo and Wellstein, 2020) employing the function “rma.mv.” Many studies reported multiple measurements through time; therefore, we used time nested within study as a random effect. This random structure accounts for the non-independence of multiple measurements across time within a study (Konstantopoulos, 2011; Cheung, 2019). These models allowed us to calculate a standardized mean difference and 95% confidence intervals for each response variable within each category.
To investigate what is the combined standardized effect of lianas on tree performance and how strong is the effect of lianas on trees, we ran separate models for each response variable within each study category to capture the direction and magnitude of the liana effect. To determine whether lianas have a stronger effect in more seasonal sites, where they tend to be more abundant (Dewalt et al., 2015), we used mean annual precipitation and life zone separately as moderators in the meta-analytic models. Forty-six studies reported mean annual precipitation, so we used WorldClim data (Fick and Hijmans, 2017) to obtain mean annual precipitation for the remaining four studies. We used an omnibus test to evaluate the effect of the moderators but only for those response variables that were significantly affected by liana removal (Viechtbauer, 2010).
We evaluated whether there is evidence of potential publication bias in the liana removal literature where studies have selectively and systematically reported significant results in the same direction and magnitude as the available literature (Koricheva et al., 2013). Assessing publication bias gave us the capacity to incorporate the influence of that bias in the inference made from the meta-analysis. To assess publication bias, we first created a funnel plot for every meta-analytic model, and tested its symmetry with an Egger's regression test. We ran the tests with the “rma.mv” function, and used the inverse of the sampling variance as a moderator (Nakagawa and Santos, 2012). A significant effect of the moderator indicated a potential publication bias.
Results
We identified 282 observations from the 50 studies published from 1968 to 2021 (Supplementary Material 1). Seventeen of the 50 studies reported responses to liana removal over time, and 37 reported a single response variable regardless of category. We found more ecological studies, followed by logging and silvicultural experiments (Figure 1), with more observations in silvicultural than logging studies (Figure 1). The most commonly assessed response variables across categories were tree growth, mortality and biomass accretion (Table 1). Across all categories, 12 studies were conducted in Panama, 10 in Brazil, 8 in Bolivia, 4 in Indonesia, 2 studies in each of the following countries: Argentina, Cameroon, Costa Rica, and Malaysia, and 1 study in: Côte d'Ivoire, Mexico, Nigeria, Suriname, Tanzania, Uganda, USA, and Venezuela (Supplementary Material 1).
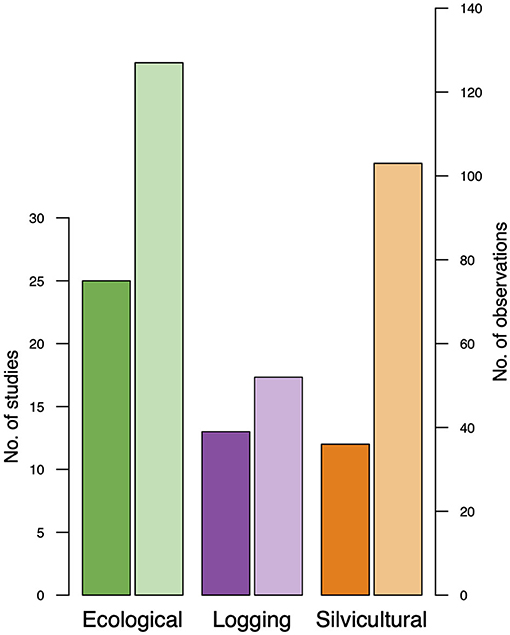
Figure 1. Number of liana removal studies and observations within studies used to conduct three-level meta-analyzes for ecological, logging, and silvicultural experiments. Number of studies in darker colors, number of observations in lighter shades.
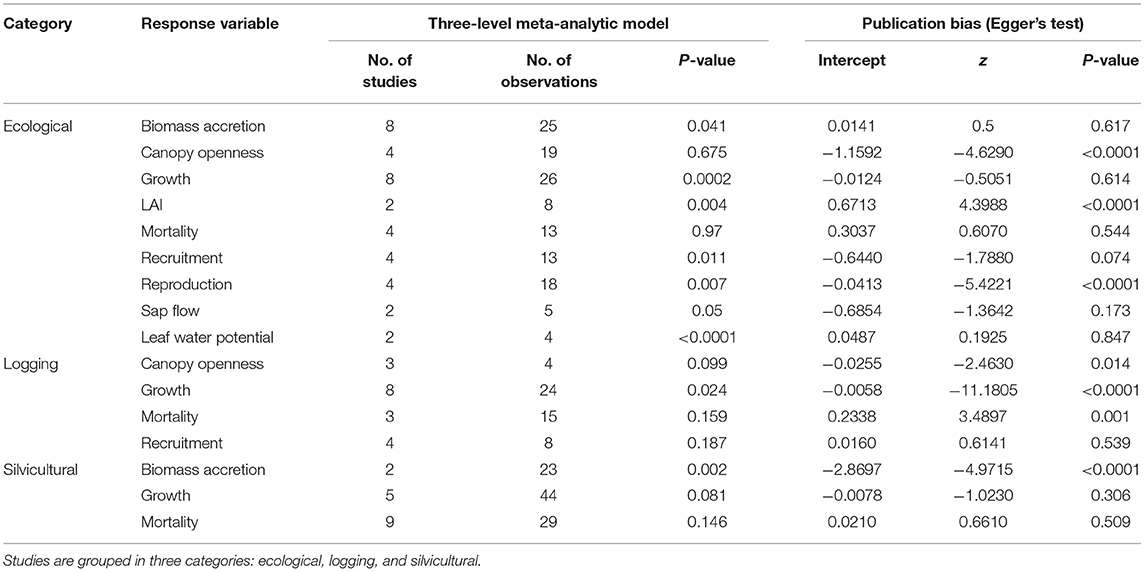
Table 1. The number of studies, observations per study, and results from a meta-analysis and assessment of publication bias of 50 liana removal experiments in tropical and subtropical forests worldwide.
For ecological studies, we found that liana removal had strong and significant positive effects on tree growth, biomass accretion, leaf water potential, recruitment of seedlings or saplings, and tree reproduction (Figure 2; Table 1). Liana removal had a marginally significant positive effect on tree sap flow (Figure 2; Table 1). However, there was a significant decrease in forest LAI, indicating that lianas contribute an important proportion of the foliage in the canopy. By contrast, liana removal did not influence tree mortality nor canopy openness (Figure 2; Table 1). For logging and silvicultural studies, lianas significantly decreased tree growth and biomass accretion (Figure 2; Table 1). Silvicultural liana removal had a marginally positive effect on tree growth. Mortality was not affected by liana removal for logging or silvicultural studies (Figure 2; Table 1). Across all three study categories, lianas significantly reduced tree biomass accretion, growth, reproduction, and decreased physiological performance in terms of water status.
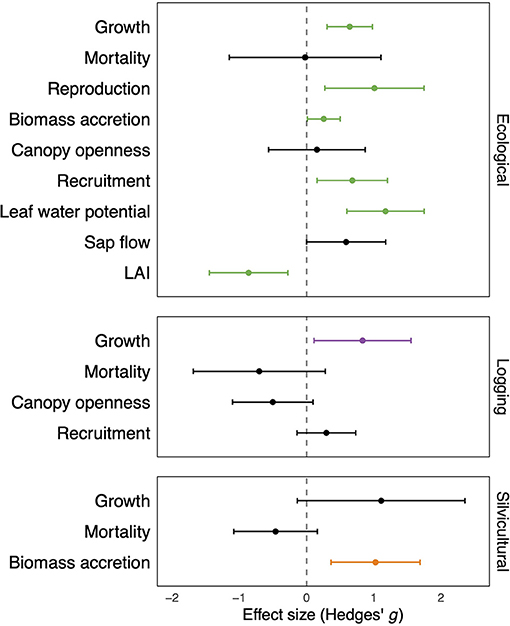
Figure 2. Tree responses to liana removal experiments conducted in tropical and subtropical forests in published ecological, logging, and silvicultural studies. Values are standardized effect sizes (Hedges' g) and their 95% confidence intervals estimated by three-level meta-analytic models. Values greater than zero indicate positive effects of liana removal. Green, purple, and orange values represent significant differences from zero; black values represent non-significant differences from zero.
The strength of liana removal effects did not increase in dryer ecosystems, and none of the response variables increased in magnitude as mean annual precipitation declined (Table 2). Egger's regression tests indicate a probable publication bias for studies that assessed LAI, canopy openness and reproduction, in the ecological literature, and for studies that assessed growth and biomass accretion in the logging and silvicultural literature, respectively (Table 1).
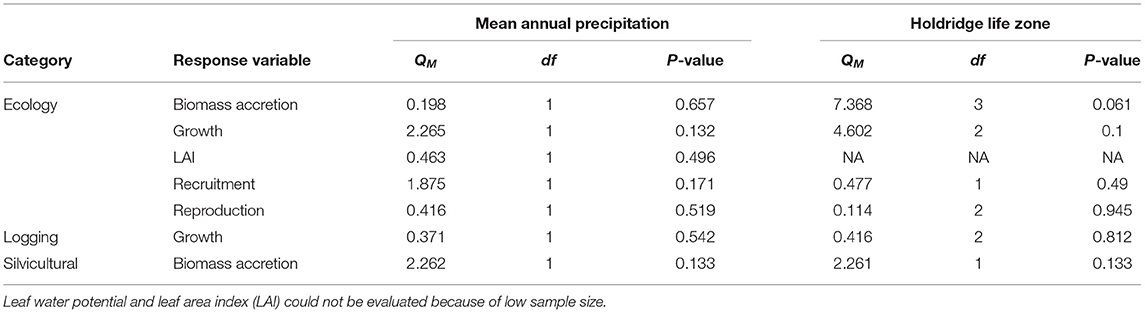
Table 2. Results from omnibus tests to evaluate the effect of mean annual precipitation and Holdridge life zone on response variables that showed a significant effect of liana removal.
Discussion
This study is the first standardized comparative quantification of the effect of lianas on tropical ecosystems worldwide. Our findings unambiguously show that lianas have a strong negative effect on tree growth, biomass accretion, water status, and reproductive output. Previous reviews reported results based on trends across data (e.g., Toledo-Aceves, 2015; Marshall et al., 2017; Estrada-Villegas and Schnitzer, 2018), but they did not use rigorous quantitative methods to compare between experimental treatments. The meta-analytic approach allowed us to incorporate the effect of sample sizes and variances within and across studies to quantitatively evaluate the effect of lianas on trees and on tropical and subtropical forest growth and performance. Our findings provide an unequivocal result: lianas have a strong negative effect on tree performance and reproduction, regardless of forest type or the goals of the study (i.e., ecological, logging, or silvicultural).
Effects of Lianas on Tree Growth and Biomass Accretion
Studies in all three categories (ecological, logging, and silvicultural) consistently showed that lianas had a negative effect on tree growth and biomass accumulation that scaled up to the whole-forest level. In fact, their effects on tree growth and biomass were more than one standard deviation greater in some of the comparisons, attesting to the strength by which lianas negatively affect trees. Trees grow less in the presence of lianas, and forests that have more lianas allocate more biomass to leaves than to stems (Van Der Heijden et al., 2015), indicating that lianas can have serious detrimental consequences for biomass accumulation and carbon cycling in tropical forests. In fact, recent removal experiments have shown that lianas can reduce up to 1.462 Mg of carbon per hectare per year, or 51.7% with respect to controls, and the effect is significant up to 6 years after experimental removal (Van Der Heijden et al., 2019).
Our results have important implications for global models of carbon sequestration. Current vegetation models usually incorporate differential tree growth and mortality among functional groups (Aleixo et al., 2019). These models, however, may fail to account the strength by which lianas reduce tree growth and biomass accretion, and probably overestimate how much biomass tropical forests will accumulate in the future. A recent vegetation model showed that forest net productivity was 2.6% lower when lianas were incorporated in the calculations compared to models that excluded them (Di Porcia E Brugnera et al., 2019). The reduction in productivity found in this model was mostly driven by the capacity of lianas to strongly compete for water and light (Meunier et al., 2021). Given that climate change may be accelerating tree mortality (Mcdowell et al., 2018), and that lianas increase in density and basal area in disturbed areas created by tree falls (Ledo et al., 2016; Schnitzer et al., 2021), it is necessary to include the detrimental effects that lianas exert on forest growth and biomass accretion we quantified in this meta-analysis in future vegetation models.
Effects of Lianas on Tree Reproduction
Lianas significantly reduce tree reproduction, which may have substantial effects on tree species demography. Trees bearing lianas accrue less resources, which may limit the amount of carbohydrates that can be allotted to flowers or fruits compared to trees without lianas in their canopies (Staudhammer et al., 2013; Kainer et al., 2014). Therefore, lianas can have the capacity to modify tree population dynamics and community turnover due to a reduction in tree reproduction (Schnitzer, 2018). Nevertheless, the number of studies assessing the effect of lianas on tree reproduction is low; there are only four studies that have reported species-specific cases and community-wide results that document the magnitude by which lianas affect tree reproduction. For example, studies have shown that flower and fruit production increases following liana removal for only three species: Bursera sminaruba, Chrysophyllum lucentifolium, and Bertholletia excelsa (Stevens, 1987; Fonseca et al., 2009). One set of experiments examined the 10-year effect of lianas on individuals of the Brazil Nut tree (Bertholletia excelsa), demonstrating that they significantly decrease fruit yield compared to trees without lianas (Staudhammer et al., 2013; Kainer et al., 2014). Studies that pool flowering and fruiting data across the tree community have also shown significant changes in tree reproduction after removal. For example, in a lowland moist forest in Panama, the number of tree species with fruits increased by 169% in liana removal plots compared to unmanipulated plots. Moreover, the number of fruiting trees was 173% higher in removal plots compared to unmanipulated plots (García León et al., 2018). While the limited data indicate that lianas strongly reduce tree reproduction, more species-specific experiments and community-wide manipulations are necessary to determine, for example, if a reduction in flowering or fruiting is coupled with a decrease in tree seed size and germination success when lianas are present in the forest canopy.
Effects of Lianas on Tree Recruitment and Water Status
Lianas often climb small trees as they begin their ascent to the forest canopy (Putz, 1984) and, by doing so, lianas can smother small trees, thus decreasing their recruitment. The negative effects of lianas on tree recruitment is especially prevalent in forest gaps, where lianas recruit rapidly and in high density (Schnitzer and Carson, 2010). Nevertheless, there are nuances on how lianas affect tree recruitment. In an experiment to compare the competitive effect of lianas and trees, Wright et al. (2015) removed similar amounts of tree and liana biomass in a moist forest in Panama. They found that seedling recruitment of the shade-tolerant species Dipteryx oleifera was greater in the liana removal treatment compared to the tree biomass treatment, but only during the dry season. In a separate study, the removal of tree saplings during the wet season had a stronger effect on recruitment compared to the removal of an equal mass of lianas (Venegas-González et al., 2020). Therefore, the liana effect on tree seedling recruitment may vary seasonally and across tree species.
The limited number of removal experiments that have assessed tree physiological performance have shown that lianas significantly reduce water availability for trees (Barker and Pérez-Salicrup, 2000; Pérez-Salicrup and Barker, 2000; Tobin et al., 2012; Álvarez-Cansino et al., 2015). Specifically, lianas reduce tree leaf water content and sap flow. Water drawdown by lianas, which is necessary to fuel their “fast and furious” physiological strategy (Schnitzer, 2018; Smith-Martin et al., 2019), may diminish water availability for trees (Reid et al., 2015). The reduction in water availability results in lower sap flow and, ultimately, in decreased tree growth (Álvarez-Cansino et al., 2015). Additional experiments are required to elucidate the extent and the mechanisms by which lianas reduce tree physiological performance and the cascading effects on tree growth and reproduction.
Liana-removal experiments have also shown that lianas contribute substantially to canopy foliage despite their relatively low basal area (Kira and Ogawa, 1971). Two manipulations from tropical moist forest in Panama reported that lianas contributed approximately 20% of the foliage in the canopy (Rodríguez-Ronderos et al., 2016; Estrada-Villegas et al., 2020). The 20% contribution is remarkable because lianas contribute <5% of the total forest basal area (Clark et al., 2008). Moreover, liana leaves displace tree leaves on a 1-to-1 mass ratio (Kira and Ogawa, 1971), which explains the increase in tree growth and biomass accretion following liana-removal, as tree foliage rapidly seizes the space vacated by lianas (Rodríguez-Ronderos et al., 2016; Van Der Heijden et al., 2019; Estrada-Villegas et al., 2020). In sum, lianas significantly reduce tree reproduction, recruitment, and water status. Moreover, the reduction of liana foliage matched an increase in tree foliage, which followed a marked increase in tree growth and biomass accretion.
Effect of Lianas Across Forest Types
The effect of lianas on tree growth and biomass accretion was expected to be greater in areas with lower mean annual precipitation and high rainfall variability, areas where lianas are usually more abundant and speciose (Schnitzer, 2005, 2018; Parolari et al., 2020). However, this was not the case. Lianas seem to have a similarly strong negative effect on trees in both wet and dry seasons, or sometimes not evidenced aboveground. For example, in a 5-year study of seasonal liana and tree growth in central Panama, lianas grew mostly during the dry season and less during the wet season, whereas trees grew poorly in the dry season and mostly in the wet season (Schnitzer and Van Der Heijden, 2019). Due to these differences in the optimal periods for liana and tree growth, the negative effects of lianas on trees were similar throughout the year. Indeed, a liana removal experiment in central Panama showed that over a 5-year period, lianas reduced biomass accretion similarly (by 46.9 and 48.5%, respectively) in the dry and wet seasons (Van Der Heijden et al., 2019). Moreover, a recent removal experiment in a Panamanian dry forest showed that lianas did not reduce above ground biomass accumulation (Estrada-Villegas et al., 2021), suggesting that the competitive effect of lianas is lower where water stress is greater despite high liana richness or abundance. Thus, the combined standardized data suggest that the relative strength of liana competition among forests that differ in rainfall or ecosystem type may not scale with liana density per se because as liana abundance increases with decreasing precipitation, drought lessens the per-capita competitive effect of lianas on trees.
Publication Bias
For ecological studies that showed a significant detrimental effect of lianas on trees, we found a probable publication bias for experiments assessing LAI and reproduction. This bias may be attributable to low sample size within response variables across study categories, heterogeneity across studies due to differences not accounted by the random effects of the statistical models, or high variability across observations within studies (Nakagawa and Santos, 2012; Koricheva et al., 2013). These potential causes for bias are not mutually exclusive. Perhaps there have been too few published experiments to elucidate the true magnitude and variability of the effect of lianas on these response variables.
The publication bias for experiments assessing growth and biomass accretion in logging and silvicultural studies may be due to high variability among observations within studies or high heterogeneity across studies. To evaluate whether variability among observations for biomass accretion in silvicultural studies is a potential cause of bias, we conducted a post hoc test and calculated the median response across observations within studies, and recalculated effect sizes and confidence intervals. This analysis corroborated the finding that lianas significantly decreased biomass accretion in silvicultural studies (Hedge's g = 1.208; 95%CI = 0.568–1.847; p-value = 0.0002), but Egger's regression test for plot asymmetry remained significant (Intercept = −2.679, z score = −2.65, p-value = 0.008). This finding indicates that factors other than variability across observations may be driving plot asymmetry (i.e., publication bias). Logging and silvicultural experiments have high heterogeneity across studies in terms of forest types, forest ages, and intensity or timing of liana removal that were not accounted in our analyzes (Estrada-Villegas and Schnitzer, 2018). Future manipulations in forestry-oriented studies will help elucidate whether the bias is an artifact of sample size, heterogeneity across studies, or variability within studies over time.
In conclusion, this study represents the first comprehensive meta-analysis of the experimental effects of lianas on tree growth and performance. The combined standardized effect sizes used in this study demonstrate unequivocally that lianas reduce tree growth, biomass accretion, reproduction, recruitment, and leaf water potential. At the forest level, lianas reduce biomass accumulation and shift carbon pools from stems to leaves. The standardized quantitative results we provide here can be used to parameterize global vegetation models so as to improve our understanding of the global carbon cycle and tropical forests dynamics.
Data Availability Statement
The original contributions presented in the study are included in the article/Supplementary Material, further inquiries can be directed to the corresponding author/s.
Author Contributions
SE-V and SS conceived the study. SE-V and SP collected the data. SE-V analyzed the data. SE-V, SP, AS, and SS interpreted the data and wrote the manuscript. All authors approved the final version of the manuscript.
Funding
This work was supported by a fellowship to SE-V from the Departamento Administrativo de Ciencia, Tecnología e Innovación COLCIENCIAS, and by the Cullman Fellowship from the School of the Environment at Yale University and the New York Botanical Garden. SS was funded by the US National Science Foundation (NSF IOS-1558093, DEB-1822473, and DEB-2001799).
Conflict of Interest
The authors declare that the research was conducted in the absence of any commercial or financial relationships that could be construed as a potential conflict of interest.
Publisher's Note
All claims expressed in this article are solely those of the authors and do not necessarily represent those of their affiliated organizations, or those of the publisher, the editors and the reviewers. Any product that may be evaluated in this article, or claim that may be made by its manufacturer, is not guaranteed or endorsed by the publisher.
Acknowledgments
We thank MaryRose Weatherton, who helped us compile a first version of the data set. We thank two reviewers for their comments on the manuscript.
Supplementary Material
The Supplementary Material for this article can be found online at: https://www.frontiersin.org/articles/10.3389/ffgc.2021.812066/full#supplementary-material
Supplementary Material 1. Data to conduct three-level mixed effect meta-analytic models to determine the direction and magnitud of the effect of lianas on tree growth, growth, biomass accumulation, reproduction, mortality, leaf water potential, sap flow velocity in ecological, silvicultural and logging studies. The information for the column headings are here: https://doi.org/10.6084/m9.figshare.14770095.v1.
References
Aleixo, I., Norris, D., Hemerik, L., Barbosa, A., Prata, E., Costa, F., et al. (2019). Amazonian rainforest tree mortality driven by climate and functional traits. Nat. Clim. Chang. 9, 384–388. doi: 10.1038/s41558-019-0458-0
Álvarez-Cansino, L., Schnitzer, S. A., Reid, J. P., and Powers, J. S. (2015). Liana competition with tropical trees varies seasonally but not with tree species identity. Ecology 96, 39–45. doi: 10.1890/14-1002.1
Avalos, G., Mulkey, S. S., and Kitajima, K. (1999). Leaf optical properties of trees and lianas in the outer canopy of a tropical dry forest. Biotropica 31, 517–520. doi: 10.1111/j.1744-7429.1999.tb00395.x
Barker, M. G., and Pérez-Salicrup, D. (2000). Comparative water relations of mature mahogany (Swietenia macrophylla) trees with and without lianas in a subhumid, seasonally dry forest in Bolivia. Tree Physiol. 20, 1167–1174. doi: 10.1093/treephys/20.17.1167
Ceballos, S. J., and Malizia, A. (2017). Liana density declined and basal area increased over 12 y in a subtropical montane forest in Argentina. J. Trop. Ecol. 33, 241–248. doi: 10.1017/S0266467417000153
César, R. G., Holl, K. D., Girão, V. J., Mello, F. N. A., Vidal, E., Alves, M. C., et al. (2016). Evaluating climber cutting as a strategy to restore degraded tropical forests. Biol. Conserv. 201, 309–313. doi: 10.1016/j.biocon.2016.07.031
Cheung, M. W. L. (2019). A guide to conducting a meta-analysis with non-independent effect sizes. Neuropsychol. Rev. 29, 387–396. doi: 10.1007/s11065-019-09415-6
Clark, D. B., Olivas, P. C., Oberbauer, S. F., Clark, D. A., and Ryan, M. G. (2008). First direct landscape-scale measurement of tropical rain forest Leaf Area Index, a key driver of global primary productivity. Ecol. Lett. 11, 163–172. doi: 10.1111/j.1461-0248.2007.01134.x
Dekker, M., and De Graaf, N. R. (2003). Pioneer and climax tree regeneration following selective logging with silviculture in Suriname. For. Ecol. Manage. 172, 183–190. doi: 10.1016/S0378-1127(01)00801-5
Dewalt, S. J., Schnitzer, S. A., Alves, L. F., Bongers, F., Burnham, R. J., Cai, Z., et al. (2015). “Biogeographical patterns of liana abundance and diversity,” in Ecology of Lianas, eds S. A. Schnitzer, F. Bongers, R. J. Burnham, and F. E. Putz (Oxford: John Wiley and Sons), 131–146.
Di Porcia E Brugnera, M., Meunier, F., Longo, M., Krishna Moorthy, S. M., De Deurwaerder, H., Schnitzer, S. A., et al. (2019). Modeling the impact of liana infestation on the demography and carbon cycle of tropical forests. Glob. Change Biol. 25, 3767–3780. doi: 10.1111/gcb.14769
Dillenburg, L. R., Teramura, A. H., Forseth, I. N., and Whigham, D. F. (1995). Photosynthetic and biomass allocation responses of Liquidambar styraciflua (Hamamelidaceae) to vine competition. Am. J. Bot. 82, 454–461. doi: 10.2307/2445691
Durán, S. M., and Sánchez-Azofeifa, G. A. (2015). “Liana effects on carbon storage and uptake in mature and secondary tropical forests,” in Biodiversity of Lianas, ed N. Parthasarathy (Cham: Springer International Publishing), 43–55.
Estrada-Villegas, S., Hall, J. S., Van Breugel, M., and Schnitzer, S. A. (2020). Lianas reduce biomass accumulation in early successional tropical forests. Ecology 101, e02989. doi: 10.1002/ecy.2989
Estrada-Villegas, S., Hall, J. S., Van Breugel, M., and Schnitzer, S. A. (2021). Lianas do not reduce tree biomass accumulation in young successional tropical dry forests. Oecologia. 195, 1019–1029. doi: 10.1007/s00442-021-04877-z
Estrada-Villegas, S., and Schnitzer, S. A. (2018). A comprehensive synthesis of liana removal experiments in tropical forests. Biotropica 50, 729–739. doi: 10.1111/btp.12571
Fick, S. E., and Hijmans, R. J. (2017). WorldClim 2: new 1-km spatial resolution climate surfaces for global land areas. Int. J. Climatol. 37, 4302–4315. doi: 10.1002/joc.5086
Fonseca, M. G., Vidal, E., and Maës Dos Santos, F. A. (2009). Intraspecific variation in the fruiting of an amazonian timber tree: implications for management. Biotropica 41, 179–185. doi: 10.1111/j.1744-7429.2008.00480.x
García León, M. M., Martínez Izquierdo, L., Mello, F. N. A., Powers, J. S., and Schnitzer, S. A. (2018). Lianas reduce community-level canopy tree reproduction in a Panamanian forest. J. Ecol. 106, 737–745. doi: 10.1111/1365-2745.12807
Gurevitch, J., Curtis, P. S., and Jones, M. H. (2001). Meta-analysis in ecology. Adv. Ecol. Res. 32, 199–247. doi: 10.1016/S0065-2504(01)32013-5
Hedges, L. V., and Olkin, I. (1985). Statistical Methods for Meta-Analysis. San Diego, CA: Academic Press.
Hogan, J. A., Mayorquín, S., Rice, K., Thompson, J., Zimmerman, J. K., and Brokaw, N. (2017). Liana dynamics reflect land-use history and hurricane response in a Puerto Rican forest. J. Trop. Ecol. 33, 155–164. doi: 10.1017/S0266467417000049
Ingwell, L. L., Joseph Wright, S., Becklund, K. K., Hubbell, S. P., and Schnitzer, S. A. (2010). The impact of lianas on 10 years of tree growth and mortality on Barro Colorado Island, Panama. J. Ecol. 98, 879–887. doi: 10.1111/j.1365-2745.2010.01676.x
Isnard, S., and Silk, W. K. (2009). Moving with climbing plants from Charles Darwin's time into the 21st century. Am. J. Bot. 96, 1205–1221. doi: 10.3732/ajb.0900045
Kainer, K. A., Wadt, L. H. O., and Staudhammer, C. L. (2014). Testing a silvicultural recommendation: Brazil nut responses 10 years after liana cutting. J. Appl. Ecol. 51, 655–663. doi: 10.1111/1365-2664.12231
Kira, T., and Ogawa, H. (1971). “Productivity of forest ecosystems assessment of primary prodution in tropical and equatorial forests,” in Production of Forest Ecosystems: Proc. Brussels Symp. Organized by UNESCO and IBP (27–31 Oct. 1969), ed P. Duvigneaud (Czechoslovakia: UNESCO), 309–321.
Konstantopoulos, S. (2011). Fixed effects and variance components estimation in three-level meta-analysis. Res. Synth. Methods 2, 61–76. doi: 10.1002/jrsm.35
Koricheva, J., Gurevitch, J., and Mengersen, K. (2013). Handbook of Meta-Analysis in Ecology and Evolution. Princeton, NJ: Princeton University Press.
Ledo, A., Illian, J. B., Schnitzer, S. A., Wright, S. J., Dalling, J. W., and Burslem, D. F. R. P. (2016). Lianas and soil nutrients predict fine-scale distribution of above-ground biomass in a tropical moist forest. J. Ecol. 104, 1819–1828. doi: 10.1111/1365-2745.12635
Marshall, A. R., Coates, M. A., Archer, J., Kivambe, E., Mnendendo, H., Mtoka, S., et al. (2017). Liana cutting for restoring tropical forests: a rare palaeotropical trial. Afr. J. Ecol. 55, 282–297. doi: 10.1111/aje.12349
Mcdowell, N., Allen, C. D., Anderson-Teixeira, K., Brando, P., Brienen, R., Chambers, J., et al. (2018). Drivers and mechanisms of tree mortality in moist tropical forests. New Phytol. 219, 851–869. doi: 10.1111/nph.15027
Medina-Vega, J. A., Bongers, F., Schnitzer, S. A., and Sterck, F. J. (2020). Lianas explore the forest canopy more effectively than trees under drier conditions. Funct. Ecol. 35, 318–329. doi: 10.1111/1365-2435.13717
Meunier, F., Verbeeck, H., Cowdery, B., Schnitzer, S. A., Smith-Martin, C. M., Powers, J. S., et al. (2021). Unraveling the relative role of light and water competition between lianas and trees in tropical forests: a vegetation model analysis. J. Ecol. 109, 519–540. doi: 10.1111/1365-2745.13540
Midolo, G., and Wellstein, C. (2020). Plant performance and survival across transplant experiments depend upon temperature and precipitation change along elevation. J. Ecol. 108, 2107–2120. doi: 10.1111/1365-2745.13387
Nakagawa, S., and Santos, E. S. A. (2012). Methodological issues and advances in biological meta-analysis. Evol. Ecol. 26, 1253–1274. doi: 10.1007/s10682-012-9555-5
Okali, D. U. U., and Ola-Adams, B. A. (1987). Tree population changes in treated rain forest at Omo Forest Reserve, south-western Nigeria. J. Trop. Ecol. 3, 291–313. doi: 10.1017/S0266467400002297
Pandian, E., and Parthasarathy, N. (2016). Decadal (2003–2013) changes in liana diversity, abundance and aboveground biomass in four inland tropical dry evergreen forest sites of peninsular India. J. For. Res. 27, 133–146. doi: 10.1007/s11676-015-0146-5
Parolari, A. J., Paul, K., Griffing, A., Condit, R., Perez, R., Aguilar, S., et al. (2020). Liana abundance and diversity increase with rainfall seasonality along a precipitation gradient in Panama. Ecography 43, 25–33. doi: 10.1111/ecog.04678
Paul, G. S., and Yavitt, J. B. (2011). Tropical vine growth and the effects on forest succession: a review of the ecology and management of tropical climbing plants. Bot. Rev. 77, 11–30. doi: 10.1007/s12229-010-9059-3
Pérez-Salicrup, D. R. (2001). Effect of liana cutting on tree regeneration in a liana forest in Amazonian Bolivia. Ecology 82, 389–396. doi: 10.2307/2679867
Pérez-Salicrup, D. R., and Barker, M. G. (2000). Effect of liana cutting on water potential and growth of adult Senna multijuga (Caesalpinioideae) trees in a Bolivian tropical forest. Oecologia 124, 469–475. doi: 10.1007/pl00008872
Pinard, M. A., and Putz, F. E. (1996). Retaining forest biomass by reducing logging damage. Biotropica 28, 278–295. doi: 10.2307/2389193
Putz, F. E. (1984). The natural history of lianas on Barro Colorado Island, Panama. Ecology 65, 1713–1724. doi: 10.2307/1937767
Reid, J. P., Schnitzer, S. A., and Powers, J. S. (2015). Short and long-term soil moisture effects of liana removal in a seasonally moist tropical forest. PLoS ONE 10, e0141891. doi: 10.1371/journal.pone.0141891
Rodríguez-Ronderos, M. E., Bohrer, G., Sanchez-Azofeifa, A., Powers, J. S., and Schnitzer, S. A. (2016). Contribution of lianas to plant area index and canopy structure in a Panamanian forest. Ecology 97, 3271–3277. doi: 10.1002/ecy.1597
Schnitzer, S. A. (2005). A mechanistic explanation for global patterns of liana abundance and distribution. Am. Nat. 166, 262–276. doi: 10.1086/431250
Schnitzer, S. A. (2018). Testing ecological theory with lianas. New Phytol. 220, 366–380. doi: 10.1111/nph.15431
Schnitzer, S. A., and Carson, W. P. (2010). Lianas suppress tree regeneration and diversity in treefall gaps. Ecol. Lett. 13, 849–857. doi: 10.1111/j.1461-0248.2010.01480.x
Schnitzer, S. A., Defilippis, D. M., Visser, M., Estrada-Villegas, S., Rivera-Camaña, R., Bernal, B., et al. (2021). Local canopy disturbance as an explanation for long-term increases in liana abundance. Ecol. Lett. 24, 2635–2647. doi: 10.1111/ele.13881
Schnitzer, S. A., Mangan, S. A., Dalling, J. W., Baldeck, C. A., Hubbell, S. P., Ledo, A., et al. (2012). Liana abundance, diversity, and distribution on Barro Colorado Island, Panama. PLoS ONE 7, e52114. doi: 10.1371/journal.pone.0052114
Schnitzer, S. A., and Van Der Heijden, G. M. F. (2019). Lianas have a seasonal growth advantage over co-occurring trees. Ecology 100, e02655. doi: 10.1002/ecy.2655
Schnitzer, S. A., Van Der Heijden, G. M. F., Mascaro, J., and Carson, W. P. (2014). Lianas in gaps reduce carbon accumulation in a tropical forest. Ecology 95, 3008–3017. doi: 10.1890/13-1718.1
Smith-Martin, C. M., Bastos, C. L., Lopez, O. R., Powers, J. S., and Schnitzer, S. A. (2019). Effects of dry-season irrigation on leaf physiology and biomass allocation in tropical lianas and trees. Ecology 100, e02827. doi: 10.1002/ecy.2827
Staudhammer, C., Wadt, L. H. O., and Kainer, K. (2013). Tradeoffs in basal area growth and reproduction shift over the lifetime of a long-lived tropical species. Oecologia 173, 45–57. doi: 10.1007/s00442-013-2603-1
Stevens, G. C. (1987). Lianas as structural parasites: the Bursera simaruba example. Ecology 68, 77–81. doi: 10.2307/1938806
Stevenson, N. S. (1927). Silvicultural treatment of mahogany forests in British Honduras. Emp. Forest. J. 6, 219–227. doi: 10.2307/42591669
Tobin, M. F., Wright, A. J., Mangan, S. A., and Schnitzer, S. A. (2012). Lianas have a greater competitive effect than trees of similar biomass on tropical canopy trees. Ecosphere 3, 1–11. doi: 10.1890/ES11-00322.1
Toledo-Aceves, T. (2015). “Above- and belowground competition between lianas and trees,” in Ecology of Lianas, eds S. A. Schnitzer, F. Bongers, R. J. Burnham, and F. E. Putz (Oxford: John Wiley and Sons), 147–163.
Trimble, G. R. J., and Tryon, E. H. (1974). Grapevines a serious obstacle to timber production on good hardwood sites in Appalachia. Northern Logger 23, 22–44.
Van Der Heijden, G. M. F., and Phillips, O. L. (2009). Liana infestation impacts tree growth in a lowland tropical moist forest. Biogeosciences 6, 2217–2226. doi: 10.5194/bg-6-2217-2009
Van Der Heijden, G. M. F., Powers, J. S., and Schnitzer, S. A. (2015). Lianas reduce carbon accumulation and storage in tropical forests. Proc. Natl. Acad. Sci. U.S.A. 112, 13267–13271. doi: 10.1073/pnas.1504869112
Van Der Heijden, G. M. F., Powers, J. S., and Schnitzer, S. A. (2019). Effect of lianas on forest-level tree carbon accumulation does not differ between seasons: results from a liana removal experiment in Panama. J. Ecol. 107, 1890–1900. doi: 10.1111/1365-2745.13155
Venegas-González, A., Mello, F. N. A., Schnitzer, S. A., César, R. G., and Tomazello-Filho, M. (2020). The negative effect of lianas on tree growth varies with tree species and season. Biotropica 52, 836–844. doi: 10.1111/btp.12796
Viechtbauer, W. (2010). Conducting meta-analyses in R with the metafor package. J. Stat. Softw. 36, 1–48. doi: 10.18637/jss.v036.i03
Visser, M. D., Schnitzer, S. A., Muller-Landau, H. C., Jongejans, E., Kroon, H., Comita, L. S., et al. (2018). Tree species vary widely in their tolerance for liana infestation: a case study of differential host response to generalist parasites. J. Ecol. 106, 781–794. doi: 10.1111/1365-2745.12815
Wan, X., Wang, W., Liu, J., and Tong, T. (2014). Estimating the sample mean and standard deviation from the sample size, median, range and/or interquartile range. BMC Med. Res. Methodol. 14, 135. doi: 10.1186/1471-2288-14-135
Keywords: liana-tree interactions, competition, tropical forests, removal experiments, experimental ecology, forestry, biomass reduction
Citation: Estrada-Villegas S, Pedraza Narvaez SS, Sanchez A and Schnitzer SA (2022) Lianas Significantly Reduce Tree Performance and Biomass Accumulation Across Tropical Forests: A Global Meta-Analysis. Front. For. Glob. Change 4:812066. doi: 10.3389/ffgc.2021.812066
Received: 09 November 2021; Accepted: 29 December 2021;
Published: 11 February 2022.
Edited by:
Nobuo Imai, Tokyo University of Agriculture, JapanReviewed by:
Yajun Chen, Xishuangbanna Tropical Botanical Garden (CAS), ChinaPatrick Addo-Fordjour, Kwame Nkrumah University of Science and Technology, Ghana
Copyright © 2022 Estrada-Villegas, Pedraza Narvaez, Sanchez and Schnitzer. This is an open-access article distributed under the terms of the Creative Commons Attribution License (CC BY). The use, distribution or reproduction in other forums is permitted, provided the original author(s) and the copyright owner(s) are credited and that the original publication in this journal is cited, in accordance with accepted academic practice. No use, distribution or reproduction is permitted which does not comply with these terms.
*Correspondence: Sergio Estrada-Villegas, estradavillegassergio@gmail.com