- 1Faculdade de Ciências Agrárias, Universidade do Oeste Paulista, Presidente Prudente-SP, Brazil
- 2Laboratório de Ecologia Evolutiva, Departamento de Biologia Geral, Universidade Estadual de Montes Claros (Unimontes), Montes Claros, Brazil
- 3Colegiado de Ecologia, Universidade Federal do Vale do São Francisco-UNIVASF, Senhor do Bonfim, Brazil
- 4Earth and Atmospheric Sciences Department, University of Alberta, Edmonton, AB, Canada
Leaf traits are good indicators of ecosystem functioning and can affect herbivory and leaf reflectance patterns, allowing a better understanding of changes in environmental conditions, such those observed during forest natural regeneration. The aim of this study was to evaluate the intraspecific variation in leaf traits and their influence on the pattern of herbivory and leaf reflectance in three species distributed along a successional gradient (early, intermediate and late stages) in a tropical dry forest (TDF) in northern Minas Gerais, Brazil. We sampled individuals of the following abundant tree species that occurred in multiple successional stages: Cenostigma pluviosum, Handroanthus ochraceus, and Tabebuia reticulata. We collected 10 leaves from each tree to determine the contents of chlorophyll a, b, and total, carotenoids and water, as well as the percentage of leaf area removed by herbivores and leaf specific mass (LSM). We also measured five spectral reflectance indices (Normalized Difference Vegetation Index-NDVI, Simple Ratio-SR, modified Normalized Difference-nND, modified SR-mSR and Water Index-WI) using a portable spectrometer. Our results showed intraspecific differences in most leaf traits along the successional gradient, suggesting that local adaptation may play an important role in plant community assembly. However, herbivory only differed for H. ochraceus in early and intermediate stages, but it was not affected by the leaf traits considered here. Spectral reflectance indices also differed among successional stage for all species together and for each species separately, except for T. reticulata in intermediate and late stages. Thus, leaf spectral signatures may be an important tool to the remote detection of different successional stages in TDFs, with implications for forest management.
Introduction
Leaf traits are often considered proxies of the relationships among efficiency in plant resource-use, investment in biomass and ecosystem functioning (Poorter et al., 2004; Lebrija-Trejos et al., 2010; Silva et al., 2015, 2021), because they can be easily quantified and are closely related with plant physiology (Cornelissen et al., 2003; Chaturvedi et al., 2011a,2021; Lohbeck et al., 2015). The occurrence of natural and anthropogenic disturbances has profound impacts on biodiversity loss and ecosystem self-regulation, which can thus be detected through variations in plant functional traits (Quesada et al., 2009; Alvarez-Añorve et al., 2012). Recovery from disturbance can occur naturally through ecological succession, a process marked by changes in plant community attributes over time (Chazdon, 2008; Faccion et al., 2021). Alterations in habitat conditions during succession result in a range of selective pressures, creating different environmental filters (Cornwell and Ackerly, 2009) that drive community assembly (Ronce et al., 2005; Lohbeck et al., 2015).
Indeed, environmental filtering is one of the processes that affect the composition and diversity of species at different successional stages (Baraloto et al., 2012; Becknell and Powers, 2014; Faccion et al., 2021). In this case, the regional species pool is represented at the local communities by a sub-group of species that converge in functional traits needed to colonization of habitats that change in conditions as the succession progresses (Cornwell and Ackerly, 2009; Lebrija-Trejos et al., 2010). However, variation in physiological traits can be found at local scale, and may be related to differences in the availability of light and nutrients in the soil, affecting plant growth rate and palatability to herbivores (Becknell and Powers, 2014; Silva et al., 2021). Plant species occurring in different successional stages may respond to long-term contrasting selective pressures and physiologically and morphologically adapt to local environmental conditions (i.e., ecotypic differentiation; Ramírez-Valiente et al., 2010). Such adaptation can also occur through phenotypic plasticity (Messier et al., 2010; Falcão et al., 2015), i.e., the range of phenotypes that a genotype can express as a function of changes in the environmental conditions (Nicotra et al., 2010). Thus, intraspecific differences in plant traits are expected along successional gradients (Alvarez-Añorve et al., 2008, 2012; Falcão et al., 2015).
Differential plant adaptation along secondary succession can be reflected in the leaf structure and production of pigments (Faccion et al., 2021). The morphophysiological plants traits associated to light availability (e.g., acquisitive or conservative traits) confer them specific spectral characteristics (Lebrija-Trejos et al., 2010; Alvarez-Añorve et al., 2012; Lohbeck et al., 2015). Leaves are the main photosynthetic organs and have great importance in the interaction of radiant energy with vegetation (Morisette et al., 2006). The use of technologies that detect spectral changes related to functional plant traits can allow the development of diagnostic tools to infer the successional and functional status of different plant communities (Alvarez-Añorve et al., 2012), permitting a better understanding of forest dynamics at the regional level (Sánchez-Azofeifa et al., 2005; Kalácska et al., 2007; Gu et al., 2018).
In general, tropical dry forests (TDFs) are still poorly studied in relation to humid tropical environments (Sánchez-Azofeifa et al., 2005; Quesada et al., 2009). Also, little is known about the optical properties of tropical plant species or about the potential for their identification using remote sensing (Castro-Esau et al., 2006; Gu et al., 2018). The spectral reflectance pattern of leaves is usually the dominant contribution to canopy reflectance (Castro-Esau et al., 2006; Kalácska et al., 2007; Gu et al., 2018). Leaf spectral reflectance is affected (1) by the biochemical properties of the leaf (water content, photosynthetic pigments and structural carbohydrates), which create specific absorption peaks of certain wavelengths; and (2) by leaf morphology (cell wall thickness, air spaces, epicuticular waxes), which affects photon dispersion (Grant, 1987; Asner, 1998; Roberts et al., 2004). Whereas pigment content plays an important role in visible light reflectance, leaf thickness and internal morphology apparently control reflectance closer to infrared (Castro-Esau et al., 2004, 2006). In this sense, leaf traits provide important indication of the differences in floristic composition and/or plant functionality at different successional stages, through spectral “signatures”—the relative intensity with which each tree reflects or emits electromagnetic radiation at different wavelengths (Castro-Esau et al., 2006).
Most studies on leaf reflectance patterns used healthy leaves (i.e., without damage by pathogens or herbivores) to measure leaf characteristics (Castro-Esau et al., 2004, 2006; Alvarez-Añorve et al., 2008, 2012). However, leaf traits are affected by damage caused by herbivores and vice versa (Carter and Knapp, 2001; Sanson et al., 2001; Peeters, 2002; Poorter et al., 2004). In a review using published and original data for different climate zones, Kozlov et al. (2015a) recorded that, in average, 7.55% of the area of woody plant foliage was eaten away or otherwise affected (mined, galled) by herbivores. In the case of TDFs, about 14% of plant biomass is removed annually by herbivory (Coley and Barone, 1996; Silva et al., 2021) and a much larger proportion of leaves, up to 75%, have some type of leaf damage (Dirzo and Domínguez, 1995). Although a leaf area loss of 14% per year does not seem to be of ecological importance, it is sufficient to reduce plant fitness, causing flowering delay and decreasing seed production and viability (Coley and Barone, 1996). Leaf herbivory rates, in turn, are strongly related to changes in morphophysiological traits along successional gradients (Poorter et al., 2004; Fonseca et al., 2018; Silva et al., 2021).
This study aimed to evaluate the intraspecific variation in leaf traits and its influence on herbivory and reflectance patterns in three species distributed along a successional gradient in a Brazilian TDF. Specifically, we addressed at following questions: i) Do leaf morphophysiological traits, herbivory and spectral reflectance differ at the intraspecific level for species that occur at different successional stages? ii) Do leaf traits affect leaf reflectance indices and leaf herbivory?
Materials and Methods
Study Area
The study was carried out in the Mata Seca State Park (hereafter MSSP), created in 2000 from the expropriation of four farms, in which the main land uses were extensive cattle raising and plantations of bean, corn and tomato. Approximately 1,525 ha of the MSSP are covered by abandoned pastures in earlier regeneration stage, whereas the remaining area is a mosaic of secondary and old-growth TDFs (intermediate and late successional stages), in addition to riparian forests (Instituto Estadual de Florestas [IEF], 2000).
The MSSP has an area of 15,466.44 ha and is located near to São Francisco River, in the municipality of Manga, Minas Gerais state, Brazil, between the coordinates 14°48′36″—14°56′59″ S and 43°55′12″—44°04′12″ W (Figure 1). The predominant original vegetation in the MSSP is the TDFs, occurring in flat and fertile soils (Instituto Estadual de Florestas [IEF], 2000). These formations are typically deciduous, with 90–95% of leaf loss during the dry season (May to October) (Pezzini et al., 2014). The climate of the region is Aw according to Köppen’s classification (updated by Peel et al., 2007), characterized by the existence of a severe dry season during the winter. The average temperature in the region is 24°C (Antunes, 1994) and the average annual rainfall is 818 ± 242 mm (data from the Manga meteorological station, approximately 10 km from the MSSP), which is concentrated from November to March.
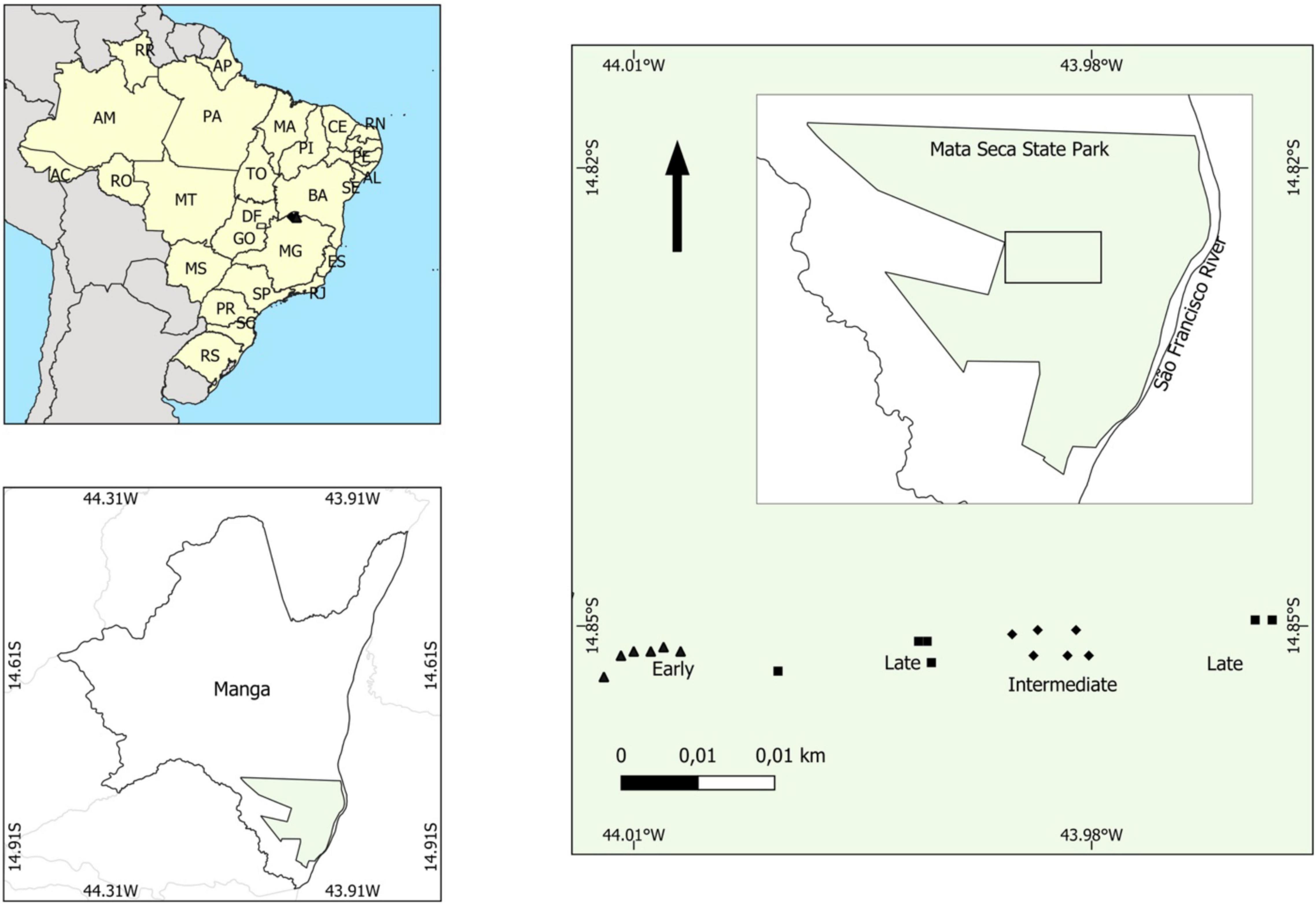
Figure 1. Location of the study site, in the Mata Seca State Park, north of Minas Gerais state in Brazil. The spatial distribution of the 18 plots in early, intermediate and late successional stages is also shown.
We studied TDFs in three different successional stages, determined according to the vertical (number of strata) and horizontal (density of trees and area basal) structural characteristics of the forest, as well as the time since the last anthropogenic disturbance and the type of previous land use (Madeira et al., 2009; Nunes et al., 2014). The early successional stage is mainly composed of sparse patches of woody vegetation, shrubs and herbaceous plants, with a single vertical stratum formed by a discontinuous canopy approximately four meters tall. This area was used as pasture for at least 20 years and abandoned in 2000, with the creation of the MSSP. The intermediate successional stage has two vertical strata. The first is composed of fast-growing trees, 10–12 m tall, forming a closed canopy with some emerging trees up to 15 m. The second stratum is composed of a dense understory, with many lianas and juvenile trees. This area was used as pasture for an indefinite period and abandoned in the late 1960s. The late successional stage also has two strata: the first is composed by tall trees, forming a closed canopy 18–20 m high, and the second is formed by a sparse understory with reduced light penetration and low density of juvenile trees and lianas. There are no records of clear-cut in this area for at least 50 years. Until the park creation in 2000, the intermediate and late stages suffered eventual, low-intensity impact from free-ranging cattle and selective logging. For more details on differences in species composition and forest structure between stages (see Madeira et al., 2009; Nunes et al., 2014).
Sampling Design
Sampling was carried out in 18 plots (50 × 20 m) delimited in 2006 and distributed equally in the three successional stages, for which phytosociological data were sampled annually until 2017 (Calvo-Rodriguez et al., 2021). Clusters of plots from different successional stages were located at 0.8–7.0 km apart from each other. In each cluster, plots from the same successional stage were positioned 0.2–1.0 km from each other (Figure 1; see Madeira et al., 2009). We recognize that this spatial distribution configures a “crumpled segregation” (sensu Hurlbert, 1984), but previous analyses by Madeira et al. (2009) indicated that similarities in species composition did not vary with plot distance within the same successional stage but decreased with distance for plots at different successional stages. Thus, we considered that plot distribution did not affect the variation on the parameters evaluated in the present study. Based on the data from 2009, three tree species (diameter at breast height ≥ 5 cm) with high IVI (especially with high abundance) and occurring in at least two successional stages (see Supplementary Table 1) were selected. Accessibility to sun-exposed leaves at the canopy was also considered for species selection. Thus, Cenostigma pluviosum (DC.) Gagnon & G.P. Lewis (Fabaceae Caesalpinoideae) was sampled in the three stages, Handroanthus ochraceus (Cham.) Mattos (Bignoniaceae) was sampled in the early and intermediate stages, and Tabebuia reticulata A.H. Gentry (Bignoniaceae) was sampled in the intermediate and late stages. For these species, nine individuals were sampled per stage and morphological and physiological traits that affect spectral reflectance and leaf herbivory were evaluated. All measurements were carried out during 9 days at the middle of the rainy season, in January 2012, when the plants had fully expanded leaves. A 5-year phenological study at the community level conducted in the same plots did not record differences in leaf flushing among successional stages (Santos, 2016), thus we considered all sampled leaves as belonging to same cohort.
To quantify the morphological and physiological characteristics, 10 fully expanded and sun-exposed leaves were randomly collected from each of the nine individuals previously marked at each stage (630 leaves in total). Cenostigma pluviosum has bipinnate leaves, with 2–11 pairs of pinna and 9–31 leaflets per pinna (Flora do Brasil, 2020). The leaves of H. ochraceus are 5-foliolate and leaflets are chartaceous, obovate to elliptic, with size ranging 4.2–10.1 × 3.1–7.3 cm and margins entire, rarely serrated (Costa et al., 2019). The leaves of T. reticulata are simple, obovate to obovate-oblong, with size ranging 2.6–5.6 × 1.9–3.1 cm and margins entire (Espírito-Santo et al., 2013). For C. pluviosa, all measurements considered the whole leaf (including all leaflets). For H. ochraceus, leaflets are large and each leaflet was considered as a separate leaf. All sampled leaves were wrapped in foil with a moistened paper to prevent leaf water loss. These samples were stored in a cooler with ice until processing. From 10 leaves collected per individual, five were used to leaf traits analyses (water content, thickness and sclerophylly), herbivory and reflectance, and the other five were used to pigment analyses (carotenoid and chlorophyll content).
Leaf Traits, Herbivory, and Reflectance
The five leaves used in the leaf analysis were numbered, photographed and readily weighted on a digital balance (accuracy of 0.0001) in the field to determine fresh weight, and leaf thickness and reflectance were immediately measured (up to 3 h after sampling). Thickness was obtained with three measurements on the leaf blade, avoiding leaf vein, with the aid of a caliper (mm). At each point in which leaf thickness was measured, the leaf reflectance was also measured. Reflectance measurements were taken with a portable spectrometer (Unispec, PP Systems, Haverhill, MA) which provides a spectral range from 350 to 1,100 nm. The reflectance indices chosen for correlation with pigment content were the Normalized Difference Vegetation Index (NDVI), Simple Ratio (SR) (Gitelson and Merzlyak, 1994), modified Normalized Difference (nND) and modified SR (mSR) (Sims and Gamon, 2002), the latter two based on the wavelength of 705 nm. We also used the Water Index (WI) of Peñuelas et al. (1997), which is a direct indicator of the amount of water in the leaf.
All 10 leaves sampled from each individual were taken to the laboratory, and five leaves were dried at 70° for 48 h (Poorter et al., 2004) and weighted to obtain the dry weight, the water content (%) and to calculate the specific leaf mass (SLM, g/m2). Leaf photographs were processed individually by binary conversion (black and white) using the software ImageJ (Rasband, 2006) to obtain total leaf area and area removed by herbivores, and the percentage of leaf area loss was calculated [(lost area/total area) * 100]. For the compound leaves of H. ochraceus, we calculated these parameters for each leaflet. For the bipinnate leaves of C. pluviosum, all leaflets of the entire leaf were considered for calculations.
Pigment Quantification
Five leaves were frozen at −18°C to pigment quantification in laboratory. We used the method adapted from Hiscox and Israelstam (1979), in which 0.1 g of powdered leaf tissue was placed in a tube with dimethylsulfoxide (DMSO, 99.9%), and submitted to a water bath at 65°C. The incubation time required for pigment extraction was determined for each species in preliminary tests, which was considered complete when leaf samples became visually transparent. Afterward, the absorbance from extracts was measured between 645 and 663 nm for chlorophylls b and a, respectively, and at 470 nm for the carotenoid content. Absorbance values were obtained using a spectrophotometer Femto 700 Plμs. We used equations proposed by Arnon (1949) to quantify the contents of chlorophylls a and b (and total). The quantification of total carotenoid content was obtained using the equation of Lichtenthaler and Wellburn (1983). The contents of chlorophyll and carotenoids were expressed in milligrams (mg) per gram (g) of leaf tissue.
Statistical Analyses
All variables obtained at the leaf level were averaged per tree for statistical analyses. To test the effect of the successional stage on leaf traits, herbivory and spectral reflectance indices at intraspecific level for the three species studied, we used generalized linear models (GLMs). The leaf thickness, water content, SLM, herbivory, spectral reflectance indices (mND705, mSR705, and WI), contents of a, b, and total chlorophyll and carotenoids were used as response variables, and the successional stage was used as explanatory variable. Post hoc tests and detection of the non-significant categorical groups (amalgamation) were performed by contrast analyses (Crawley, 2007) using “coms” function from “RT4Bio” package of R (Reis et al., 2015). The models were subjected to residual analysis to assess the adequacy of the error distribution model (Crawley, 2007) using the “rdiagnostic” function from the “RT4Bio” R package (Reis et al., 2015).
We used multiple linear regressions through generalized linear mixed-effect models (GLMMs) to verify if the spectral reflectance indices were influenced by leaf traits (pigments and water), without differentiation of species and stages. In each model, the species identity was used as a random effect variable in order to control for possible interspecific differences that may affect the spectral reflectance indices. We built the complete GLMM models with Gaussian error distribution in which the indices were used as response variables and the contents of chlorophyll a, b, and total, and carotenoids and water were used as explanatory variables. The variance inflation factor (VIF) was calculated as a measure of multicollinearity. We detected collinearity between the predictor variables chlorophyll a, b, and total (VIF values > 5 indicates strong collinearity; Logan, 2010), then only total chlorophyll was maintained in the models, which were then submitted to a residual analysis for the adequacy of the error distribution (Crawley, 2007). These analyses were performed using “lmer” function from the “lme4” R package (Bates et al., 2015).
To verify if leaf herbivory was influenced by SLM, chlorophyll a, b and total, and water content, we also performed GLMMs as previously described. Herbivory was used as the response variable and aforementioned leaf traits were used as explanatory variables. We verified that there was no collinearity between the predictor variables. The models were submitted to residual analyses for the adequacy of the error distribution (Crawley, 2007). The final models obtained were compared with a null model in order to test their significance. All analyses were performed using the R software (R Development Core Team, 2015).
Results
All leaf traits and herbivory differed among successional stages for at least one of the species considered (Table 1 and Supplementary Table 2). The mean reflectance values were higher for all the species in the early stage (Figures 2A–D). This variation was more evident for H. ochraceus, which presented higher reflectance values in the early compared to the intermediate stage for both the visible and for the infrared and near infrared regions (Figure 2C).
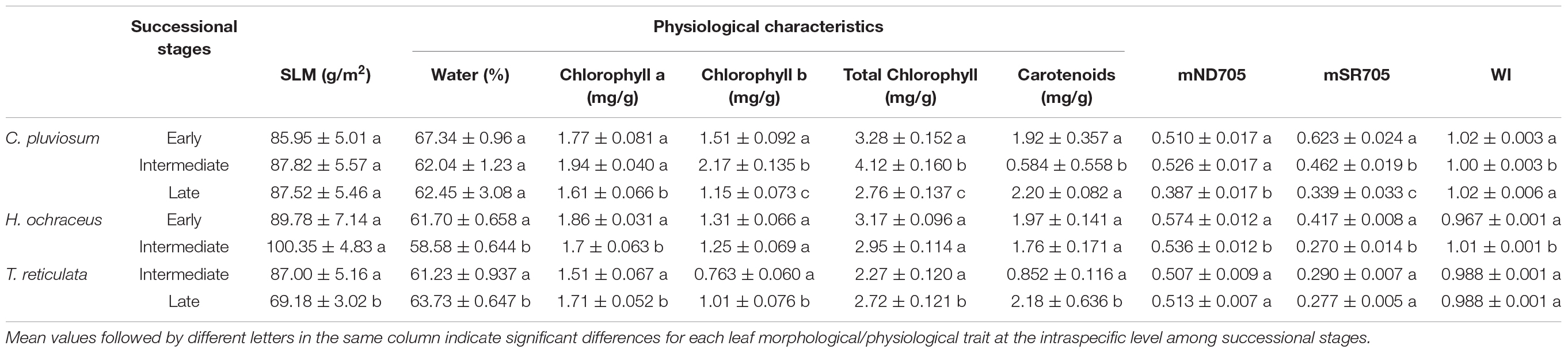
Table 1. Mean values (± standard deviation) for morphological (specific leaf mass-SLM) and physiological traits (water content, chlorophyll a, b, and total, and carotenoids), and spectral reflectance indices for C. pluviosum, H. ochraceus, and T. reticulata in different successional stages.
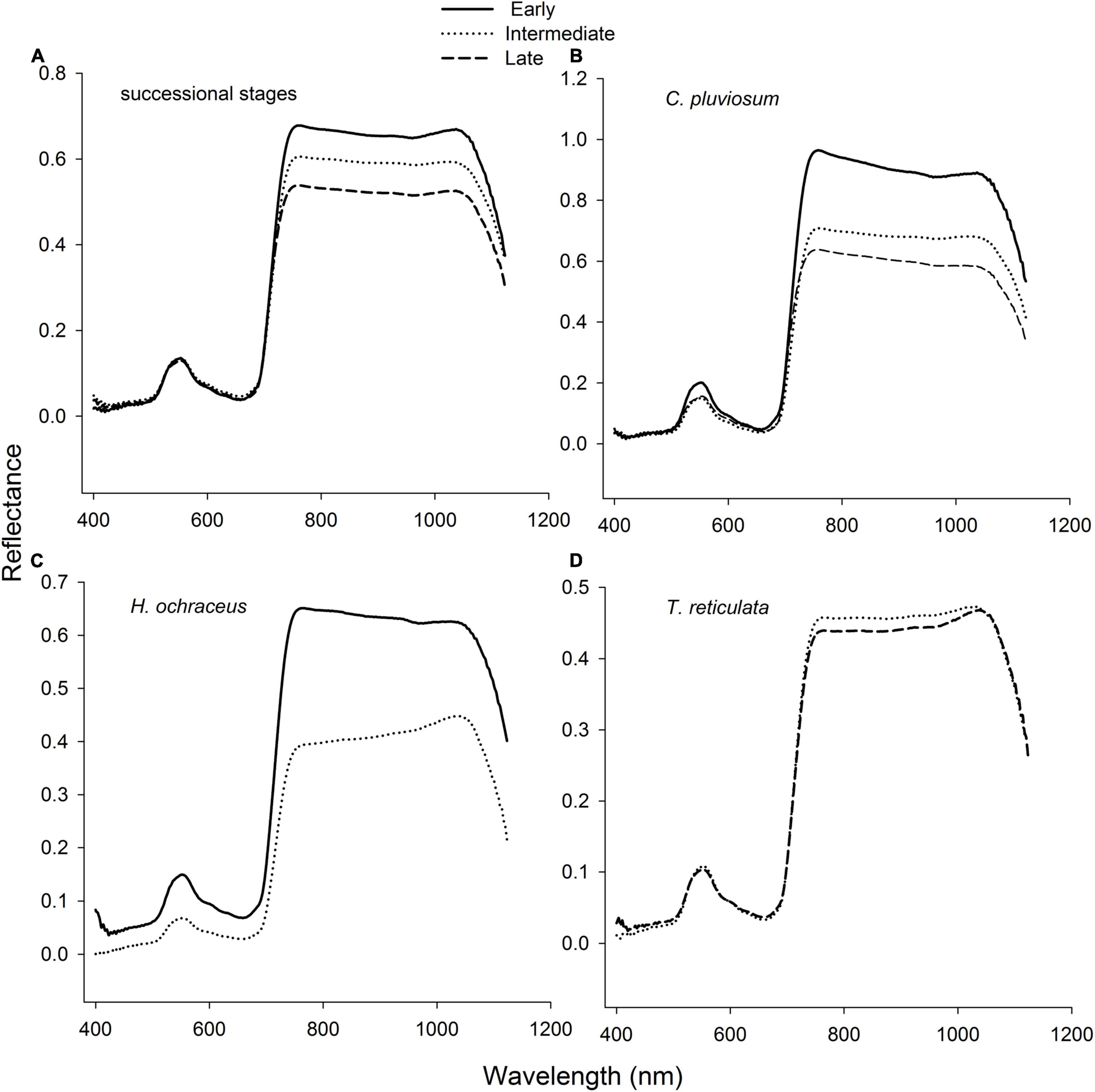
Figure 2. (A) Mean leaf reflectance spectra for (A) all three species together, and for each species separately (B–D) in different successional stages in a tropical dry forest.
For C. pluviosum, the percentage of leaf herbivory, LSM and water content did not differ between successional stages (Table 1 and Figure 3). On the other hand, chlorophyll a, b, total and carotenoid contents in this species varied among successional stages. The chlorophyll a, b, and total contents had lowest concentration in the early stage but carotenoid content was significantly lower in the intermediate stage (Table 1). For C. pluviosum, mND705 and mSR705 were lower in the late stage and the WI was lower in the intermediate stage.
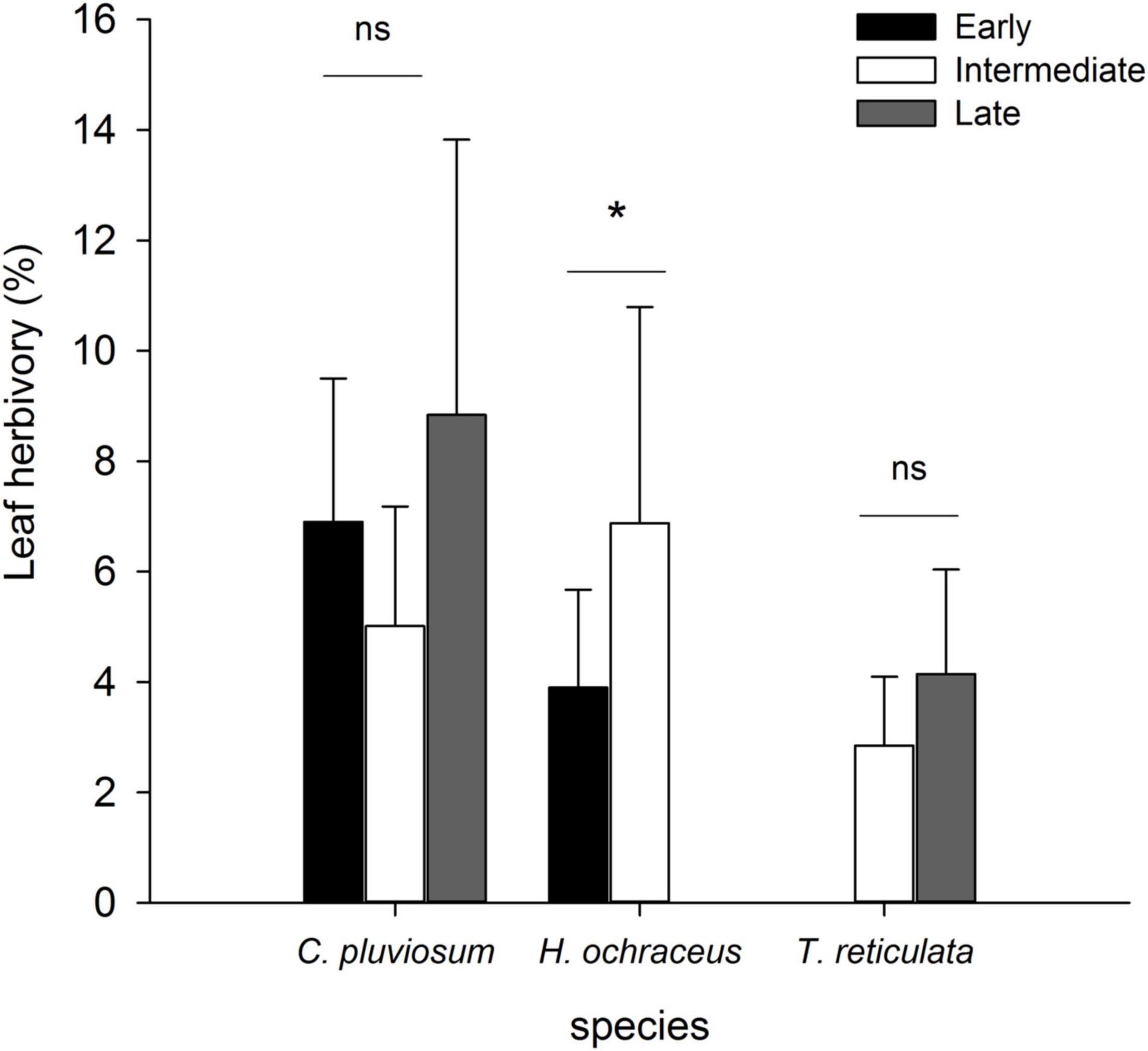
Figure 3. Mean (± standard deviation) percentage of leaf damage for three plant species at different successional stages in a tropical dry forest. The asterisk indicates differences on mean herbivory at the intraspecific level among successional stages (P < 0.05). ns = P > 0.05.
For H. ochraceus, SLM, chlorophyll b and total contents and carotenoids did not differ between successional stages. However, the water and chlorophyll a contents were higher (5.3 and 9.4%, respectively) for the early compared to the intermediate stage (Table 1), and the percentage of leaf herbivory was higher in the intermediate (76.9%) than in the early stage (Figure 3). For this species, the mND705 and mSR705 indices were significantly higher in the early stage compared to the intermediate stage (7 and 54.4%, respectively), and the WI was higher in the intermediate stage (4.4%).
For T. reticulata, all variables varied among successional stages except for herbivory (Figure 3). SLM was greater in the intermediate stage (25.7%) and the contents of chlorophyll a (13%), b (33.2%), total (19.8%), carotenoids (156%), and water (4%) were higher in the late stage. None of the spectral indices exhibited significant differences among stages for this species (Table 1 and Supplementary Table 2).
The spectral indices correlated differently with the physiological traits (pigment and water contents) considered here (Table 2). Only the NDVI and mND705 indices were positively influenced by the total chlorophyll content (Supplementary Figure 1 and Table 2). Carotenoid content was not related to any spectral index (Table 2). The water content also did not correlate the WI (Table 2). The herbivory levels (percentage of leaf area removed) were not influenced by any of the leaf characteristics evaluated in the present study (P ≥ 0.05 for all; see Table 3).
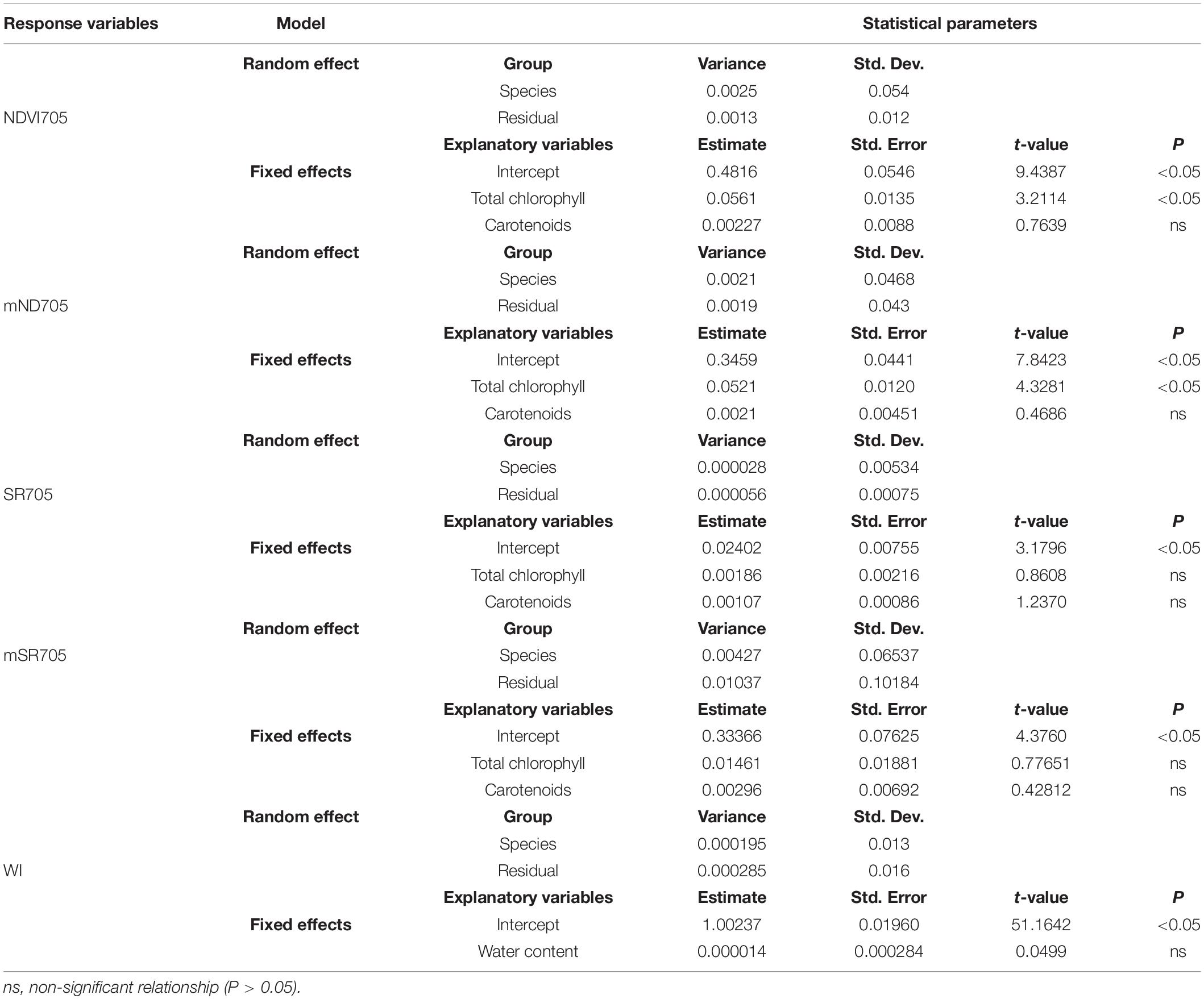
Table 2. Results of the generalized mixed effect linear models (GLMMs) evaluating the effects of total chlorophyll and carotenoids on spectral reflectance indices (NDVI705, mND705, SR705, and mSR705), and the effects of water content effect on Water Index (WI).
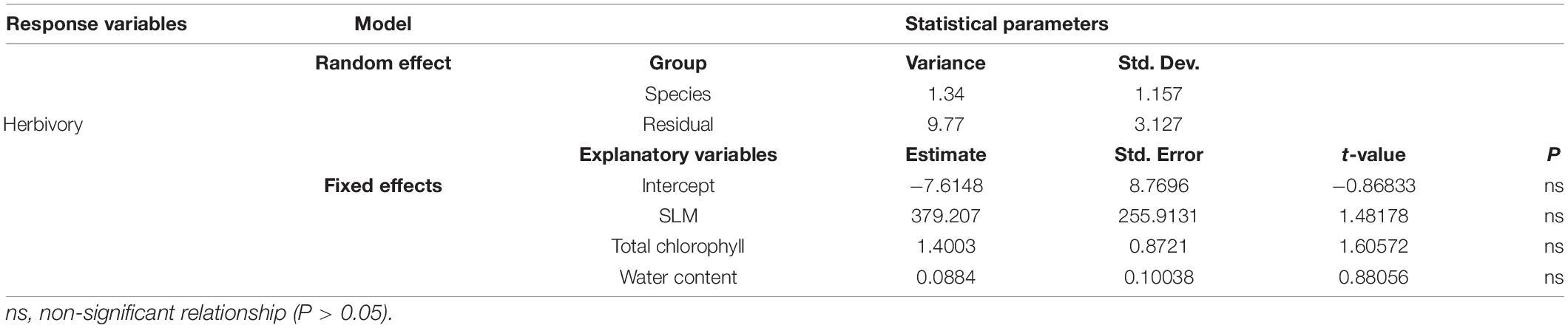
Table 3. Results of the generalized mixed effect linear models (GLMMs) evaluating the effects of specific leaf mass (SLM), total chlorophyll and water content on herbivory (percentage of leaf area removed).
Discussion
Leaf Traits
The leaf traits analyzed here showed intraspecific variation along the successional gradient for the three studied species, with different intensities and patterns for each of them. In the case of T. reticulata, all six morphophysiological variables (excluding the percentage of leaf area removed and spectral indices) varied significantly between stages. In H. ochraceus, this variation was detected only for two of these variables. For C. pluviosum, differences were observed for four of the morphophysiological variables, as well as for the spectral indices. Intraspecific variation in plant traits at contrasting successional habitats has been observed in other studies, and may be a consequence of local adaptation, phenotypic plasticity or genetic differences among individuals (Kawecki and Ebert, 2004; Ramírez-Valiente et al., 2010; Garzón et al., 2019). However, such idiosyncratic responses were not expected if environmental filtering in acting during community assembly in the studied TDF. This lack of consistent pattern may be related to the fact that each species is considered to belong to a different functional group (Nunes et al., 2014): H. ochraceus is a light-demanding climax species, whereas T. reticulata is shade-tolerant climax species and C. pluviosum is a pioneer species. It is likely that adaptation to environmental gradients, such as the observed for naturally regenerating forests, is mediated by the strength of the trade-offs that operate among traits, and no single trait values are optimal solutions for a particular habitat conditions (Umaña and Swenson, 2019).
SLM did not vary among successional stages for C. pluviosum and H. ochraceus, suggesting that this trait has a low intraspecific variation in response to different habitat conditions in the studied TDF chronosequence. Usually, plants from humid and temperate tropical forests have low SLM as a peculiar characteristic of pioneer species (Coley et al., 1985). This is because these species would not invest in chemical defenses, such as fibers, lignins, and phenolic compounds in short-lived leaves with a low initial construction cost (Coley et al., 1985; Reich et al., 1992; Poorter et al., 2004). The inverse pattern would be observed for late species. Since almost all species in TDFs have short-lived leaves as a result of a well-defined dry season (Chaturvedi et al., 2011b), it is likely that this classic successional pattern, typical of tropical rainforests, does not apply to TDFs (Lohbeck et al., 2015; Faccion et al., 2021). However, for T. reticulata SLM was greater in the intermediate stage compared to the late stage, which may be related to the lower amount of nutrients in this environment (Espírito-Santo et al., 2014; Silva et al., 2021). In habitats with nutrient-poor soils but high light availability, plants have low growth rates and accumulate the excess carbon from photosynthesis, causing cell wall thickening and, consequently, more sclerophyllous leaves with high SLM (Gonçalves-Alvim et al., 2006).
The water content did not vary along the successional stages for C. pluviosum. For individuals of H. ochraceus and T. reticulata, the water content was lower in the intermediate stage, where soil moisture is also lower than in the early and late stages (Rankine et al., 2017). It is known that the amount of water stored in leaves is strongly influenced by the availability of water in the soil and by plant’s water retention capacity (Rennó and Soares, 2007). Several factors can affect the soil’s water holding capacity, such as particle size and relief characteristics (Santos et al., 2013). In fact, the early and late stage plots used in the present study are located in areas of lower relief (closer to the water table) than the intermediate stage area (Coelho et al., 2013). Furthermore, rainfall interception by the forest canopy at the intermediate stage is higher (Calvo-Alvarado et al., 2018), and the amount of water reaching the ground at this stage is also lower. Thus, leaf water content seems to respond more directly to soil water conditions for H. ochraceus and T. reticulata in this TDF.
Chlorophyll levels showed inconsistent patterns among the studied species. The content of chlorophyll a, b and total in individuals of C. pluviosum was higher for the intermediate stage, while the opposite was observed for H. ochraceus and T. reticulata. Leaf chlorophyll content is indirectly related to soil nitrogen levels, as this nutrient participates in the constitution of amino acids, proteins, enzymes, and chlorophyll (Bojović and Marković, 2009; Nogueira et al., 2010). In fact, in our site soils from early and late stages have higher nitrogen concentration (2.0 and 2.2 g/kg, respectively) compared to the intermediate stage (1.7 g/kg, P < 0.01) (Espírito-Santo et al., 2014; Silva et al., 2021). Gruner et al. (2003) also found higher levels of leaf nitrogen in intermediate-stage forests using a chronosequence design in humid tropical forests. Thus, further studies are still needed to understand whether the differentiation in chlorophyll content between species and stages would be a characteristic physiological behavior of plant families or a phenotypic adaptation to nitrogen availability in TDF soils.
The content of carotenoids was lower in the intermediate stage for the three species studied, although there was no statistically significant difference for H. ochraceus. Carotenoids are accessory pigments that help to capture light and protect plants against reactive oxygen species (Demmig-Adams et al., 1990; Demmig-Adams and Adams, 1996). Gitelson and Merzlyak (1994) related the content of carotenoids, specifically its proportionality with chlorophyll, to leaf senescence, that is, plants with senescent leaves or under stress would tend to increase their proportion of carotenoids in relation to chlorophyll. However, this information should be interpreted with caution to TDFs, due to its high deciduousness in the dry season. Particularly for C. pluviosum, another study conducted in the same site by Faccion et al. (2021) recorded the lowest average leaf life span in the intermediate stage, followed by the late and early stages, which is consistent with the levels of carotenoids observed here.
Herbivory
The variation in herbivory levels along succession has showed inconsistent patterns in previous studies in TDFs (Neves et al., 2014; Silva et al., 2021). We observed that the percentage of leaf area removed did not differ between stages for C. pluviosum and T. reticulata. Previous studies in the same plots, conducted at the plant community level, have shown that herbivory varies significantly among stages over years, in addition to idiosyncratic variations at the plant species level (Neves et al., 2014; Silva et al., 2021). However, for H. ochraceus, greater leaf damage was observed in individuals of the intermediate stage, the same result found by Silva et al. (2012) in the same plots. It is possible that the increase in the canopy connectivity facilitates the dispersal of different species of generalist insects. A continuous and heterogeneous canopy would facilitate foraging, mainly by herbivores of the Crysomelidade family, which effectively respond to a greater availability of new leaves (Silva et al., 2012; Neves et al., 2014). This could explain the slightly higher herbivory levels in the intermediate stage, where the great abundance of lianas increases the canopy connectivity (Madeira et al., 2009; Sánchez-Azofeifa et al., 2009).
The percentage of leaf damage did not correlate with SLM, chlorophyll and water contents for any of the three species. In general, studies in TDFs at the same region showed that the plant traits affecting herbivory levels varied in a case-to-case basis. Silva et al. (2012, 2015) found that leaf nitrogen content and the concentration of phenolic compounds had a positive effect on leaf damage on H. ochraceus and six other deciduous and evergreen TDF species, which was also observed for phenolic compounds by Silva et al. (2020). For H. spongiosus, Oliveira et al. (2012) have reported no significant effects of total phenolic content and leaf toughness on herbivory levels. Fonseca et al. (2018), on the other hand, detected a negative effect of tannin concentration and SLM on leaf damage at the plant community level. Generally, leaves with higher chlorophyll content also have more nitrogen (Smeal and Zhang, 1994; Booij et al., 2000), a limiting nutrient in the diet of herbivorous insects, as well as water content (Filip et al., 1995; Silva et al., 2015). However, herbivory intensity is affected by several top-down and bottom-up forces acting in opposing directions (Scherber et al., 2010; Silva et al., 2012; Kozlov et al., 2015b; Vidal and Murphy, 2018). These complex interactions can be mediated by the focal plant’s neighbors (an associational effect; Castagneyrol et al., 2017) and are difficult to untangle without controlled experiments (Muehleisen et al., 2020).
Reflectance Indices
The observed correlations between spectral reflectance indices and leaf pigment content suggest that they can be useful for remote estimates of several ecological parameters (Kalácska et al., 2007; Gu et al., 2018). There was a positive relation between total chlorophyll content and the NDVI and mND indices. The adaptation of the normalized difference index (ND) was done by Sims and Gamon (2002) to remove the effect of the low absorption at 445 nm for chlorophylls and carotenoids. Leaves with low reflectance values present good correlations with SR705 and chlorophyll, whereas leaves with high reflectance values do not show a correlation between SR705 and chlorophyll (Sims and Gamon, 2002). The greater number of correlations between chlorophyll content and ND indices detected here corroborates other studies that used the same indices. Castro and Sanchez-Azofeifa (2008) found strong correlations between pigments and mND705 and mSR705 in both sun and shade leaves of two species of Populus. Values of mND 705 and pigment content were directly related for T. reticulata and H. ochraceus. Other studies have also observed a direct relation between modified indices and chlorophyll content, both under natural conditions and in laboratory experiments (Le Maire et al., 2004).
The WI (R900/R970), contrary to expected, was not influenced by the leaf water content. Peñuelas et al. (1997) found a strong relation between WI and plant water content in studies in species from Mediterranean climate with good water availability. In remote sensing, this index is used to identify water bodies but it is known that interactions of incident light energy with water are complex and depend on a series of factors such as specular reflection (surface roughness), type and concentration of compounds (chlorophyll and nutrients) and climatic variables that affect the spectral behavior (Roberts et al., 2004; Castro-Esau et al., 2006).
Conclusion
Our results contribute to the library of spectral signatures of the most representative species in the studied TDF (Kalácska et al., 2007; Gu et al., 2018), which can improve models to remote sensing monitoring of TDF regions that are highly threatened by anthropogenic and natural disturbances. The intraspecific variation in leaf traits along the successional stages suggest that different selective pressures may drive adaptation of the studied species to the local environmental conditions, either via ecotypic differentiation or phenotypic plasticity. Further studies should focus on other plant traits, and common garden and reciprocal transplant experiments with a larger number of species are needed to determine the causal effects of local adaptation and phenotypic plasticity on plant physiology and herbivory during community assembly in TDFs. Such knowledge is fundamental for a better understanding of successional patterns and for developing successful forest restoration programs.
Data Availability Statement
The raw data supporting the conclusions of this article will be made available by the authors, without undue reservation.
Author Contributions
AA, ME-S, and AS-A conceptualized the manuscript. AA, GF, JS, and KF organized the database. JS and ME-S analyzed the data. All authors wrote sections of the manuscript and contributed to data interpretation, manuscript revision, data acquisition, read, and approved the submitted version.
Funding
This study was supported by the Conselho Nacional de Desenvolvimento Científico e Tecnológico (Grant No. CNPq 563304/2010-3 and 308471/2017-2), Fundação de Amparo à Pesquisa (Grant Nos. FAPEMIG CRA-2288/07, CRA-APQ-04738-10, BPD-00252-14, and PPM-726-16), the Inter-American Institute for Global Change Research (Grant No. IAI-CRN II-021), and National Science and Engineering Research Council of Canada—Discovery Grant Program. The Coordenação de Aperfeiçoamento de Pessoal de Nível Superior provided scholarships to GF, AA, JS, and KF. The CNPq provided a research scholarship to ME-S (Grant No. 308471/2017-2).
Conflict of Interest
The authors declare that the research was conducted in the absence of any commercial or financial relationships that could be construed as a potential conflict of interest.
Publisher’s Note
All claims expressed in this article are solely those of the authors and do not necessarily represent those of their affiliated organizations, or those of the publisher, the editors and the reviewers. Any product that may be evaluated in this article, or claim that may be made by its manufacturer, is not guaranteed or endorsed by the publisher.
Supplementary Material
The Supplementary Material for this article can be found online at: https://www.frontiersin.org/articles/10.3389/ffgc.2021.780299/full#supplementary-material
References
Alvarez-Añorve, M. Y., Quesada, M., and Barrera, E. (2008). “Remote sensing and plant functional groups: physiology, ecology, and spectroscopy in tropical systems,” in Hyperspectral Remote Sensing of Tropical and Subtropical Forests, eds M. Kalácska and G. A. Sanchez-Azofeifa (Boca Raton, FL: CRC Press), 27–45.
Alvarez-Añorve, M. Y., Quesada, M., Sánchez-Azofeifa, G. A., Avila-Cabadilla, L. D., and Gamon, J. A. (2012). Functional regeneration and spectral reflectance of trees during succession in a highly diverse tropical dry forest ecosystem. Am. J. Bot. 99, 816–826. doi: 10.3732/ajb.1100200
Antunes, F. Z. (1994). Caracterização climática – caatinga do estado de minas gerais. Inf. Agropec. 17, 15–19.
Arnon, D. I. (1949). Copper enzymes in isolated chloroplasts. Polyphenoloxidase in Beta vulgaris. Plant Physiol. 24, 1–15. doi: 10.1104/pp.24.1.1
Asner, G. P. (1998). Biophysical and biochemical sources of variability in canopy reflectance. Remote Sens. Environ. 64, 234–253.
Baraloto, C., Hardy, O. J., Paine, C. E. T., Dexter, K. G., Cruaud, C., Dunning, L. T., et al. (2012). Using functional traits and phylogenetic trees to examine the assembly of tropical tree communities. J. Ecol. 100, 690–701. doi: 10.1111/j.1365-2745.2012.01966.x
Bates, D., Mächler, M., Bolker, B. M., and Walker, S. C. (2015). Fitting linear mixed-effects models using lme4. J. Stat. Softw. 67, 1–48. doi: 10.18637/jss.v067.i01
Becknell, J. M., and Powers, J. S. (2014). Stand age and soils as drivers of plant functional traits and aboveground biomass in secondary tropical dry forest. Can. J. For. Res. 44, 604–613. doi: 10.1139/cjfr-2013-0331
Bojović, B., and Marković, A. (2009). Correlation between nitrogen and chlorophyll content in wheat (Triticum aestivum L.). Kragujev. J. Sci. 31, 69–74.
Booij, R., Valenzuela, J. L., and Aguilera, C. (2000). “Determination of crop nitrogen status using non-invasive methods,” in Management of Nitrogen and Water in Potato Production, eds A. J. Haverkort and D. K. L. Mackerron (Wageningen: Wageningen Academic Publishers), 72–82.
Calvo-Alvarado, J. C., Jiménez-Rodríguez, C. D., Calvo-Obando, A. J., Espírito-Santo, M. M., and Gonçalves-Silva, T. (2018). Interception of rainfall in successional tropical dry forests in Brazil and Costa Rica. Geosciences 8:486. doi: 10.3390/geosciences8120486
Calvo-Rodriguez, S., Sánchez-Azofeifa, G. A., Durán, S. M., Espírito-Santo, M. M., and Nunes, Y. R. F. (2021). Dynamics of carbon accumulation in tropical dry forests under climate change extremes. Forests 12:106. doi: 10.3390/f12010106
Carter, G. A., and Knapp, A. K. (2001). Leaf optical properties in higher plants: linking spectral characteristics to stress and chlorophyll concentration. Am. J. Bot. 88, 677–684. doi: 10.2307/2657068
Castagneyrol, B., Bonal, D., Damien, M., Jactel, H., Meredieu, C., Muiruri, E. W., et al. (2017). Bottom-up and top-down effects of tree species diversity on leaf insect herbivory. Ecol. Evol. 7, 3520–3531. doi: 10.1002/ece3.2950
Castro, K. L., and Sanchez-Azofeifa, G. A. (2008). Changes in spectral properties, chlorophyll content and internal mesophyll structure of senescing Populus balsamifera and Populus tremuloides leaves. Sensors 8, 51–69. doi: 10.3390/s8010051
Castro-Esau, K. L., Sánchez-Azofeifa, G. A., and Caelli, T. (2004). Discrimination of lianas and trees with leaf-level hyperspectral data. Remote Sens. Environ. 90, 353–372. doi: 10.1016/j.rse.2004.01.013
Castro-Esau, K. L., Sánchez-Azofeifa, G. A., Rivard, B., Wright, S. J., and Quesada, M. (2006). Variability in leaf optical properties of Mesoamerican trees and the potential for species classification. Am. J. Bot. 93, 517–530. doi: 10.3732/ajb.93.4.517
Chaturvedi, R. K., Raghubanshi, A. S., and Singh, J. S. (2011a). Leaf attributes and tree growth in a tropical dry forest. J. Veg. Sci. 22, 917–931. doi: 10.1111/j.1654-1103.2011.01299.x
Chaturvedi, R. K., Raghubanshi, A. S., and Singh, J. S. (2011b). Plant functional traits with particular reference to tropical deciduous forests: a review. J. Biosci. 36, 963–981. doi: 10.1007/s12038-011-9159-1
Chaturvedi, R. K., Tripathi, A., Raghubanshi, A. S., and Singh, J. S. (2021). Functional traits indicate a continuum of tree drought strategies across a soil water availability gradient in a tropical dry forest. For. Ecol. Manage. 482:118740. doi: 10.1016/j.foreco.2020.118740
Chazdon, R. L. (2008). “Chance and determinism in tropical forest succession,” in Tropical Forest Community Ecology, eds W. P. Carson and S. A. Schnitzer (Oxford: Wiley-Blackwell), 384–408.
Coelho, M. R., Dart, R. D. O., Vasques, G. D. M., Teixeira, W. G., Oliveira, R. P., Brefin, M., et al. (2013). Levantamento Pedológico Semi-Detalhado (1: 30.000) do Parque Estadual da Mata Seca, Município de Manga-MG. Boletim de Pesquisa e Desenvolvimento 217. Rio de Janeiro: Embrapa Solos, 1–264.
Coley, P. D., and Barone, J. A. (1996). Herbivory and plant defenses in tropical forests. Annu. Rev. Ecol. Syst. 27, 305–335. doi: 10.1146/annurev.ecolsys.27.1.305
Coley, P. D., Bryant, J. P., and Chapin, F. S. (1985). Resource availability and plant antiherbivore defense. Science 230, 895–899. doi: 10.1126/science.230.4728.895
Cornelissen, J. H. C., Lavorel, S., Garnier, E., Díaz, S., Buchmann, N., Gurvich, D. E., et al. (2003). A handbook of protocols for standardised and easy measurement of plant functional traits worldwide. Aust. J. Bot. 51, 335–380. doi: 10.1071/BT02124
Cornwell, W. K., and Ackerly, D. D. (2009). Community assembly and shifts in plant trait distributions across an environmental gradient in coastal California. Ecol. Monogr. 79, 109–126. doi: 10.1890/07-1134.1
Costa, S., Lohmann, L. G., and Buril, M. T. (2019). Flora of Pernambuco, Brazil: Tabebuia alliance and tribe Jacarandeae (Bignoniaceae). Biota Neotrop. 19:e20190737. doi: 10.1590/1676-0611-BN-2019-0737
Crawley, M. J. (2007). Statistical Computing-An Introduction to Data Analysis Using S-Plus. London: John Wiley & Sons.
Demmig-Adams, B., and Adams, W. W. (1996). The role of xanthophyll cycle carotenoids in the protection of photosynthesis. Trends Plant Sci. 1, 21–26. doi: 10.1016/S1360-1385(96)80019-7
Demmig-Adams, B., Adams, W. W., Heber, U., Neimanis, S., Winter, K., Kruger, A., et al. (1990). Inhibition of zeaxanthin formation and of rapid changes in radiationless energy dissipation by dithiothreitol in spinach leaves and chloroplasts. Plant Physiol. 92, 293–301.
Dirzo, R., and Domínguez, C. A. (1995). “Plant-herbivore interactions in Mesoamerican tropical dry forests,” in Seasonally Dry Tropical Forests, eds S. H. Bullock, A. Mooney, and E. Medina (Cambridge: Cambridge University Press), 304–309.
Espírito-Santo, F. D. S., Silva-Castro, M. M., and Rapini, A. (2013). Flora da Bahia: Bignoniaceae 2–Aliança Tabebuia. Sitientibus Ciênc. Biol. 13, 1–38. doi: 10.13102/scb211
Espírito-Santo, M. M., Olívio-Leite, L., Neves, F. S., Nunes, Y. R. F., Borges, M. A. Z., Falcão, L. A. D., et al. (2014). “Tropical dry forests of Northern Minas Gerais, Brazil: diversity, conservation status and natural regeneration,” in Tropical Dry Forests in the Americas: Ecology, Conservation, and Management, eds G. A. Sánchez-Azofeifa, J. S. Powers, G. W. Fernandes, and M. Quesada (Boca Raton, FL: CRC), 69–82.
Faccion, G., Alves, A. M., Espírito-Santo, M. M., Silva, J. O., Sanchez-Azofeia, A., and Ferreira, K. F. (2021). Intra- and interspecific variations on plant functional traits along a successional gradient in a Brazilian tropical dry forest. Flora 279:151815. doi: 10.1016/j.flora.2021.151815
Falcão, H. M., Medeiros, C. D., Silva, B. L. R., Sampaio, E. V. S. B., Almeida-Cortez, J. S., and Santos, M. G. (2015). Phenotypic plasticity and ecophysiological strategies in a tropical dry forest chronosequence: a study case with Poincianella pyramidalis. For. Ecol. Manag. 340, 62–69. doi: 10.1016/j.foreco.2014.12.029
Filip, V., Dirzo, R., Maass, J. M., and Sarukhan, J. (1995). Within- and among-year variation in the levels of herbivory on the foliage of trees from a Mexican tropical deciduous forest. Biotropica 27, 78–86. doi: 10.2307/2388905
Flora do Brasil (2020). Jardim Botânico do Rio de Janeiro. Available online at: http://floradobrasil.jbrj.gov.br/ (accessed Nov 05, 2021).
Fonseca, M. B., Silva, J. O., Falcão, L. A. D., Dupin, M. G. V., Melo, G. A., and Espírito-Santo, M. M. (2018). Leaf damage and functional traits along a successional gradient in Brazilian tropical dry forests. Plant Ecol. 219, 403–415. doi: 10.1007/s11258-018-0804-8
Garzón, B. M., Robson, T. M., and Hampe, A. (2019). ΔTrait SDMs: species distribution models that account for local adaptation and phenotypic plasticity. New Phytol. 222, 1757–1765. doi: 10.1111/nph.15716
Gitelson, A. A., and Merzlyak, M. N. (1994). Spectral reflectance changes associate with autumn senescence of Aesculus hippocastanum L. and Acer platanoides L. leaves. Spectral features and relation to chlorophyll estimation. J. Plant Physiol. 143, 286–292. doi: 10.1016/S0176-1617(11)81633-0
Gonçalves-Alvim, S. J., Korndorf, G., and Fernandes, G. W. (2006). Sclerophylly in Qualea parviflora (Vochysiaceae): influence of herbivory, mineral nutrients, and water status. Plant Ecol. 187, 153–162. doi: 10.1007/s11258-004-3286-9
Grant, L. (1987). Diffuse and specular characteristics of leaf reflectance. Remote Sens. Environ. 22, 309–322. doi: 10.1016/0034-4257(87)90064-2
Gruner, D. S., Polhemus, D. A., Basset, Y., Novotny, V., Miller, S. E., and Kitching, R. L. (2003). “Arthropod assemblages across a long chronosequence in the Hawaiian Islands,” in Arthropods of Tropical Forests: Spatio-Temporal Dynamics and Resource Use in the Canopy, eds Y. Basset, V. Novotny, S. E. Miller, and R. L. Kitching (Cambridge: Cambridge University Press), 135–145.
Gu, Z., Cao, S., and Sanchez-Azofeifa, G. A. (2018). Using LiDAR waveform metrics to describe and identify successional stages of tropical dry forests. Int. J. Appl. Earth Obs. Geoinf. 73, 482–492. doi: 10.1016/j.jag.2018.07.010
Hiscox, J. D., and Israelstam, G. F. (1979). A method for the extraction of chlorophyll from leaf tissue without maceration. Can. J. For. Res. 148, 1332–1334. doi: 10.1139/b79-163
Hurlbert, S. H. (1984). Pseudoreplication and the design of ecological field experiments. Ecol. Monogr. 54, 187–211. doi: 10.2307/1942661
Instituto Estadual de Florestas [IEF] (2000). Parecer Técnico para a Criação do Parque Estadual da Mata Seca. Technical Report. Belo Horizonte: IEF.
Kalácska, M., Sanchez-Azofeifa, G. A., Rivard, B., Caelli, T., White, H. P., and Calvo-Alvarado, J. C. (2007). Ecological fingerprinting of ecosystem succession: estimating secondary tropical dry forest structure and diversity using imaging spectroscopy. Remote Sens. Environ. 108, 82–96. doi: 10.1016/j.rse.2006.11.007
Kawecki, T. J., and Ebert, D. (2004). Conceptual issues in local adaptation. Ecol. Lett. 7, 1225–1241. doi: 10.1111/j.1461-0248.2004.00684.x
Kozlov, M. V., Lanta, V., Zverev, V., and Zvereva, E. L. (2015a). Global patterns in background losses of woody plant foliage to insects. Glob. Ecol. Biogeogr. 24, 1126–1135. doi: 10.1111/geb.12347
Kozlov, M. V., Lanta, V., Zverev, V., and Zvereva, E. L. (2015b). Background losses of woody plant foliage to insects show variable relationships with plant functional traits across the globe. J. Ecol. 103, 1519–1528. doi: 10.1111/1365-2745.12471
Le Maire, G., François, C., and Dufrêne, E. (2004). Towards universal broad leaf chlorophyll indices using PROSPECT simulated database and hyperspectral reflectance measurements. Remote Sens. Environ. 89, 1–28. doi: 10.1016/j.rse.2003.09.004
Lebrija-Trejos, E., Pérez-García, E. A., Meave, J. A., and Bongers, F. P. L. (2010). Functional traits and environmental filtering drive community assembly in a species-rich tropical system. Ecology 91, 386–398. doi: 10.1016/j.ppees.2011.10.002
Lichtenthaler, H., and Wellburn, A. (1983). Determinations of total carotenoids and chlorophylls a and b of leaf extracts in different solvents. Biochem. Soc. Trans. 603, 591–592. doi: 10.1042/bst0110591
Logan, M. (2010). Biostatistical Design Analysis using R: A Practical Guide. Oxford: Wiley Blackwell.
Lohbeck, M., Lebrija-Trejos, E., Martínez-Ramos, M., Meave, J. A., Poorter, L., and Bongers, F. (2015). Functional trait strategies of trees in dry and wet tropical forests are similar but differ in their consequences for succession. PLoS One 10:e0123741. doi: 10.1371/journal.pone.0123741
Madeira, B. G., Espírito-Santo, M. M., Neto, S. D. A., Nunes, Y. R. F., Sánchez Azofeifa, G. A., Wilson Fernandes, G., et al. (2009). Changes in tree and liana communities along a successional gradient in a tropical dry forest in south-eastern Brazil. Plant Ecol. 201, 291–304. doi: 10.1007/s11258-009-9580-9
Messier, J., McGill, B. J., and Lechowicz, M. J. (2010). How do traits vary across ecological scales? A case for trait-based ecology. Ecol. Lett. 13, 838–848. doi: 10.1111/j.1461-0248.2010.01476.x
Morisette, J. T., Baret, F., Privette, J. L., Myneni, R. B., Nickeson, J. E., Garrigues, S., et al. (2006). Validation of global moderate-resolution LAI products: a framework proposed within the CEOS land product validation subgroup. IEEE Trans. Geosci. Remote Sens. 44, 1804–1817. doi: 10.1109/TGRS.2006.872529
Muehleisen, A. J., Engelbrecht, B. M., Jones, F. A., Manzané-Pinzón, E., and Comita, L. S. (2020). Local adaptation to herbivory within tropical tree species along a rainfall gradient. Ecology 101:e03151. doi: 10.1002/ecy.3151
Neves, F. S., Silva, J. O., Espírito-Santo, M. M., and Fernandes, G. W. (2014). Insect herbivores and leaf damage along successional and vertical gradients in a tropical dry forest. Biotropica 46, 14–24. doi: 10.1111/btp.12068
Nicotra, A. B., Atkin, O. K., Bonser, S. P., Davidson, A. M., Finnegan, E. J., Mathesius, U., et al. (2010). Plant phenotypic plasticity in a changing climate. Trends Plant Sci. 15, 684–692. doi: 10.1016/j.tplants.2010.09.008
Nogueira, P. D. M., Sena Júnior, D. G., and Ragagnin, V. A. (2010). Clorofila foliar e nodulação em soja adubada com nitrogênio em cobertura. Global Sci. Technol. 3, 117–124.
Nunes, Y. R. F., Luz, G. R., Souza, S. R., Silva, D. L., Veloso, M. D. M., Espírito-Santo, M. M., et al. (2014). “Floristic, structural, and functional group variations in tree assemblages in a Brazilian tropical dry forest: effects of successional stage and soil properties,” in Tropical Dry Forests in the Americas: Ecology, Conservation, and Management, eds G. A. Sánchez-Azofeifa, J. S. Powers, G. W. Fernandes, and M. Quesada (Boca Raton, FL: CRC), 325–349.
Oliveira, K. N., Espírito-Santo, M. M., Silva, J. O., and Melo, G. A. (2012). Ontogenetic and temporal variations in herbivory and defense of Handroanthus spongiosus (Bignoniaceae) in a Brazilian tropical dry forest. Environ. Entomol. 41, 541–550. doi: 10.1603/EN11259
Peel, M. C., Finlayson, B. L., and McMahon, T. A. (2007). Updated world map of the Köppen-Geiger climate classification. Hydrol. Earth Syst. Sci. 11, 1633–1644. doi: 10.5194/hess-11-1633-2007
Peeters, P. J. (2002). Correlations between leaf structural traits and the densities of herbivorous insect guilds. Biol. J. Linn. Soc. 77, 43–65. doi: 10.1046/j.1095-8312.2002.00091.x
Peñuelas, J., Pinol, J., Ogaya, R., and Filella, I. (1997). Estimation of plant water concentration by the reflectance Water Index WI (R900/R970). Int. J. Remote Sens. 18, 2869–2875. doi: 10.1080/014311697217396
Pezzini, F. F., Ranieri, B. D., Brandão, D. O., Fernandes, G. W., Quesada, M., Espírito-Santo, M. M., et al. (2014). Changes in tree phenology along natural regeneration in a seasonally dry tropical forest. Plant Biosyst. 148, 965–974. doi: 10.1080/11263504.2013.877530
Poorter, L., Van De Plassche, M., Willems, S., and Boot, R. G. A. (2004). Leaf traits and herbivory rates of tropical tree species differing in successional status. Plant Biol. 6, 746–754. doi: 10.1055/s-2004-821269
Quesada, M., Sanchez-Azofeifa, G. A., Alvarez-Añorve, M., Stoner, K. E., Avila-Cabadilla, L., Calvo-Alvarado, J., et al. (2009). Succession and management of tropical dry forests in the Americas: review and new perspectives. For. Ecol. Manage. 258, 1014–1024. doi: 10.1016/j.foreco.2009.06.023
R Development Core Team (2015). R: A Language and Environment for Statistical Computing. Vienna: R Foundation for Statistical Computing.
Ramírez-Valiente, J. A., Sánchez-Gómez, D., Aranda, I., and Valladares, F. (2010). Phenotypic plasticity and local adaptation in leaf ecophysiological traits of 13 contrasting cork oak populations under different water availabilities. Tree Physiol. 30, 618–627. doi: 10.1093/treephys/tpq013
Rankine, C., Sánchez-Azofeifa, G. A., Guzmán, J. A., Espirito-Santo, M. M., and Sharp, I. (2017). Comparing MODIS and near-surface vegetation indexes for monitoring tropical dry forest phenology along a successional gradient using optical phenology towers. Environ. Res. Lett. 12:105007. doi: 10.1088/1748-9326/aa838c
Reich, P. B., Walters, M. B., and Ellsworth, D. S. (1992). Leaf life-span in relation to leaf, plant and stand characteristics among diverse ecosystems. Ecol. Monogr. 62, 365–392. doi: 10.2307/2937116
Reis, R. Jr., Oliveira, M. L., and Borges, G. R. A. (2015). RT4Bio: R Tools for Biologists. Available online at: https://sourceforge.net/projects/rt4bio/ (accessed June 26, 2016).
Rennó, C. D., and Soares, J. V. (2007). “Conceitos básicos de modelagem hidrológica,” in Geomática: Modelos e Aplicações Ambientais, eds M. S. P. Meirelles, G. Câmara, and C. M. Almeida (Brasília: Embrapa Informação Tecnológica), 529–556.
Roberts, D. A., Ustin, S. L., Ogunjemiyo, S., Greenberg, J., Dobrowski, S. Z., Chen, J. Q., et al. (2004). Spectral and structural measures of northwest forest vegetation at leaf to landscape scales. Ecosystems 7, 545–562. doi: 10.1007/s10021-004-0144-5
Ronce, O., Brachet, S., Olivieri, I., Gouyon, P. H., and Clobert, J. (2005). Plastic changes in seed dispersal along ecological succession: theoretical predictions from an evolutionary model. J. Ecol. 93, 431–440. doi: 10.1111/j.1365-2745.2005.00972.x
Sánchez-Azofeifa, G. A., Kalácska, M., do Espírito-Santo, M. M., Fernandes, G. W., and Schnitzer, S. (2009). Tropical dry forest succession and the contribution of lianas to wood area index (WAI). For. Ecol. Manage. 258, 941–948. doi: 10.1016/j.foreco.2008.10.007
Sánchez-Azofeifa, G. A., Kalácska, M., Quesada, M., Calvo-Alvarado, J. C., Nassar, J. M., and Rodríguez, J. P. (2005). Need for integrated research for a sustainable future in tropical dry forests. Conserv. Biol. 19, 285–286. doi: 10.1111/j.1523-1739.2005.s01_1.x
Sanson, G., Read, J., Aranwela, N., Clissold, F., and Peeters, P. (2001). Measurement of leaf biomechanical properties in studies of herbivory: opportunities, problems and procedures. Aust. Ecol. 26, 535–546. doi: 10.1046/j.1442-9993.2001.01154.x
Santos, H. G., Jacomine, P. K. T., Anjos, L. H. C., Oliveira, V. A., Lumbreras, J. F., Coelho, M. R., et al. (2013). Sistema Brasileiro de Classificação de Solos. Brasília-DF: Embrapa Solos.
Santos, J. C. (2016). Comparing Ground-Based and Remote Sensing Methods to Assess Phenological Patterns Along a Successional Gradient in a Tropical Dry Forest. Master dissertation. Montes Claros: Universidade Estadual de Montes Claros.
Scherber, C., Eisenhauer, N., Weisser, W. W., Schmid, B., Voigt, W., Fischer, M., et al. (2010). Bottom-up effects of plant diversity on multitrophic interactions in a biodiversity experiment. Nature 468, 553–556. doi: 10.1038/nature09492
Silva, J. O., Espírito-Santo, M. M., and Melo, G. A. (2012). Herbivory on Handroanthus ochraceus (Bignoniaceae) along a successional gradient in a tropical dry forest. Arthropod Plant. Interact. 6, 45–57. doi: 10.1007/s11829-011-9160-5
Silva, J. O., Espírito-Santo, M. M., and Morais, H. C. (2015). Leaf traits and herbivory on deciduous and evergreen trees in a tropical dry forest. Basic Appl. Ecol. 16, 210–219. doi: 10.1016/j.baae.2015.02.005
Silva, J. O., Espírito-Santo, M. M., Santos, J. C., and Rodrigues, P. M. (2020). Does leaf flushing in the dry season affect leaf traits and herbivory in a tropical dry forest? Sci. Nat. 107:51. doi: 10.1007/s00114-020-01711-z
Silva, J. O., Souza-Silva, H., Rodrigues, P. M. S., Cuevas-Reyes, P., and Espírito-Santo, M. M. (2021). Soil resource availability, plant defense, and herbivory along a successional gradient in a tropical dry forest. Plant Ecol. 222, 625–637. doi: 10.1007/s11258-021-01131-7
Sims, D. A., and Gamon, J. A. (2002). Relationships between leaf pigment content and spectral reflectance across a wide range of species, leaf structures and developmental stages. Remote Sens. Environ. 81, 337–354. doi: 10.1016/S0034-4257(02)00010-X
Smeal, D. E., and Zhang, H. (1994). Chlorophyll meter evaluation for nitrogen management in corn. Commun. Soil Sci. Plant Anal. 25, 1495–1503. doi: 10.1080/00103629409369130
Umaña, M. N., and Swenson, N. G. (2019). Does trait variation within broadly distributed species mirror patterns across species? A case study in Puerto Rico. Ecology 100:e02745. doi: 10.1002/ecy.2745
Keywords: leaf reflectance, leaf pigments, ecological succession, herbivory, tropical dry forests, intraspecific variation
Citation: Alves AM, Espírito-Santo MMd, Silva JO, Faccion G, Sanchez-Azofeifa A and Ferreira KF (2021) Successional and Intraspecific Variations in Leaf Traits, Spectral Reflectance Indices and Herbivory in a Brazilian Tropical Dry Forest. Front. For. Glob. Change 4:780299. doi: 10.3389/ffgc.2021.780299
Received: 20 September 2021; Accepted: 07 December 2021;
Published: 24 December 2021.
Edited by:
Xoaquin Moreira, Misión Biológica de Galicia (MBG), Spanish National Research Council (CSIC), SpainReviewed by:
Ravi Kant Chaturvedi, Xishuangbanna Tropical Botanical Garden, Chinese Academy of Sciences (CAS), ChinaShunsuke Utsumi, Hokkaido University, Japan
Copyright © 2021 Alves, Espírito-Santo, Silva, Faccion, Sanchez-Azofeifa and Ferreira. This is an open-access article distributed under the terms of the Creative Commons Attribution License (CC BY). The use, distribution or reproduction in other forums is permitted, provided the original author(s) and the copyright owner(s) are credited and that the original publication in this journal is cited, in accordance with accepted academic practice. No use, distribution or reproduction is permitted which does not comply with these terms.
*Correspondence: Mário Marcos do Espírito-Santo, bWFyaW8ubWFyY29zQHVuaW1vbnRlcy5icg==