- 1Institute for Applied Plant Biology AG, Witterswil, Switzerland
- 2Meteotest, Berne, Switzerland
- 3Swiss Tropical and Public Health Institute, University of Basel, Basel, Switzerland
European beech is one of the most important deciduous tree species in natural forest ecosystems in Central Europe. Its dominance is now being questioned by the emerging drought damages due to the increased incidence of severe summer droughts. In Switzerland, Fagus sylvatica have been observed in the Intercantonal Forest Observation Program since 1984. The dataset presented here includes 179176 annual observations of beech trees on 102 plots during 37 years. The plots cover gradients in drought, nitrogen deposition, ozone, age, altitude, and soil chemistry. In dry regions of Switzerland, the dry and hot summer of 2018 caused a serious branch dieback, increased mortality in Fagus sylvatica and increased yellowing of leaves. Beech trees recovered less after 2018 than after the dry summer 2003 which had been similar in drought intensity except that the drought in 2018 started earlier in spring. Our data analyses suggest the importance of drought in subsequent years for crown transparency and mortality in beech. The drought in 2018 followed previous dry years of 2015 and 2017 which pre-weakened the trees. Our long-term data indicate that the drought from up to three previous years were significant predictors for both tree mortality and for the proportion of trees with serious (>60%) crown transparency. The delay in mortality after the weakening event suggests also the importance of weakness parasites. The staining of active vessels with safranine revealed that the cavitation caused by the low tree water potentials in 2018 persisted at least partially in 2019. Thus, the ability of the branches to conduct water was reduced and the branches dried out. Furthermore, photooxidation in light-exposed leaves has increased strongly since 2011. This phenomenon was related to low concentrations of foliar phosphorus (P) and hot temperatures before leaf harvest. The observed drought effects can be categorized as (i) hydraulic failure (branch dieback), (ii) energy starvation as a consequence of closed stomata and P deficiency (photooxidation) and (iii) infestation with weakness parasites (beech bark disease and root rots).
Introduction
Anthropogenic induced climate change has led to a global warming, which has occurred at a faster rate over land surfaces with an average increase of 1.5°C between 2006–2015 compared to 1850–1900 (Shukla et al., 2019). In Switzerland, the average temperature increase has been 2.5°C for the last decade (2011–2020) compared to 1871–1900 (MeteoSwiss, 2021). The projected heat waves are expected to occur more frequent and of longer duration (IPCC, 2014), leading to hotter droughts (Allen et al., 2010) as summer precipitation is expected to decrease in parts of Central Europe (IPCC, 2014). The European heatwave in 2003 has pointed out the urgency of understanding and predicting better the response of ecosystems to extreme drought events due to the large impacts on human health, crop yields and forest health (García-Herrera et al., 2010; Buras et al., 2020).
The drought event in 2018 was even more severe with unprecedented heat droughts in many parts of Central and Northern Europe (Buras et al., 2020). Also in Switzerland, the 2018 summer drought was one of the most severe and long-lasting droughts ever recorded and caused serious damages to forests in Switzerland (Schuldt et al., 2020). Two main processes are discussed as causes for drought damages of trees: (i) hydraulic failure (Choat et al., 2012; Engelbrecht, 2012) and/or (ii) carbon starvation (McDowell and Sevanto, 2010). Hydraulic failure means that the water filaments in the vessels rupture under high tension, when the soil is dry and the water demand of the atmosphere is high. Death occurs when a certain proportion of the vessels has lost their function due to embolism (Urli et al., 2013; Choat et al., 2018). Death by carbon starvation is a process usually occurring in understory trees because of the lack of light (McDowell and Sevanto, 2010) but it is supposed to occur in adult trees when stomata are closed for a long time. The knowledge on this process bases on indirect evidences (McDowell and Sevanto, 2010). Carbon starvation can be seen as a preparative process for the attack by weakness parasites (McDowell et al., 2011). Often drought is followed by insect outbreaks (Anderegg et al., 2015). Fungal outbreaks after drought are less frequent but also known. Weakness parasites usually lead to death with delay. An increase of beech bark disease after drought has been shown for European beech (Schindler, 1951; Jarcuska et al., 2013). Armillaria sp. is also a well known weakness parasite attacking drought stressed trees (Kubiak et al., 2017).
This study aimed at assessing and comparing the observed severe drought stress signs at sites of the long-term Intercantonal Forest Observation Program in Switzerland. The large gradients in precipitation, altitude, soil chemistry, age, nitrogen deposition, and ozone allow to disentangle the contribution of various factors to the observed tree health parameters such as crown transparency, discoloration, photobleaching, and mortality rates of Fagus sylvatica. The long-term data of 37 years of forest observation were further analysed to examine the extent of the above mentioned three hypotheses for drought damages in European beech forest stands: (i) hydraulic failure, (ii) energy starvation, (iii) weakness parasites.
Materials and Methods
Monitoring Plots and Assessments of Mortality, Crown Defoliation and Discoloration
The dataset is based on a 37 years time series including 179176 annual observations for 9826 individual beech trees in 102 plots across Switzerland. The plots cover gradients in drought, nitrogen deposition, ozone, age, altitude, and soil chemistry (Braun et al., 2017b). Trees have been assessed annually for crown transparency and discoloration according to ICP Forests (2016). Trees with a crown transparency of >60% are hereafter called “strongly damaged” trees. The number of dead trees is related to the population of the previous year, respectively.
Foliar Nutrient Analysis and Photobleaching in Leaves
Shoots for nutrient analysis have been collected in the years 1984, 1987, 1991 and thereafter every 4 years by helicopter from the upper sun canopy (nine harvest events in total). Each harvest consisted of eight trees per plot. Leaves were dried and analysed for N, P, and K as described in Braun et al. (2020). Photobleaching was assessed by selecting randomly 25 leaves from each harvested branch. Leaves showing signs of photobleaching, e.g., not uniformly green, were counted. For regression analysis, the number of unbleached and the number of bleached leaves per tree was used.
Measurements of Actual Cavitation in Tree Branches
During the harvest 2019 which followed the dry summer 2018 an analysis for actual cavitation was performed in 145 branches sampled in the sun canopy in 10 plots between July 22 and August 3 in 2019. The samples were wrapped into black plastic bags immediately after sampling and brought into the lab within the same day. Branch pieces of 15 cm were recut under water. Reversible embolies were removed by rinsing them under a pressure of 0.1 Pa (Hacke and Jansen, 2009) with a freshly membrane filtered (0.45 μm) solution of 0.1% safranine (Harvey and van den Driessche, 1997) in water during 30 min. The stained branches were kept frozen until image analysis of the stained areas with ImageJ1. The stained area was related to the total possible cross sectional area without the core.
Drought Indicators
Meteorological data were interpolated for each plot from the nearest eight monitoring stations of the Federal Office of Meteorology and Climatology (MeteoSwiss) as described by Braun et al. (2017b). The resulting daily averages were used in the regression analysis or as input to the hydrological model Wasim-ETH (Schulla, 2019). With this model the following drought indicators were calculated (i) ratio between actual and potential evapotranspiration (ETa/ETp), (ii) site water balance (SWB) which is the cumulated difference between precipitation and potential evapotranspiration at a daily basis, with added water storage capacity of the soil (available water capacity, AWC). The cumulation was limited to +AWC. The cumulation started on January 1st. The lowest value reached during summer was used in the data analysis. Temperature, precipitation, and drought indicators for the harvest year were averaged over the time between start of the growing season and the harvest date. Start and end of the season were taken from phenological data of beech budbreak and discoloration observed by MeteoSwiss and adjusted for altitude as described in Braun et al. (2017b). The season length thus differs between plots.
Statistics
All statistical analyses were performed using R 4.0.3 (RCore Team, 2020). Foliar concentrations were log transformed when the transformation improved the model. Staining results and discoloration were analysed using linear, mortality, numbers of strongly damaged trees per plot and photobleaching using binomial mixed regression models (Bates et al., 2015), with plots and years and – in the case of photobleaching also trees – as random factors, following the data analysis strategy by Braun et al. (2017a). In brief, backward selection of predictor variables using the Akaike Information Criterion (AIC) was applied. Estimated explained variance (Pseudo-R2) of the fixed effects only and of fixed and random effects were calculated according to Nakagawa and Schielzeth (2013). Natural cubic splines (one or two knots) were used to model non-linear functional relationships. All models including R scripts and diagnostic plots are provided in the Appendix. Residuals were checked for normality, homoscedasticity and outliers. Effect plots with estimated marginal means and the corresponding 95% confidence intervals taking into account all fixed and random effects were calculated according to Lüdecke (2018).
Results
Field Observations
In 2019 and 2020, the proportion of trees with a crown transparency of >60% increased by a factor of 6.1 and 6.7 compared to the long term average before 2019 (Figure 1). This increase was mainly due to an increase in dead branches. The mortality was also increased but less strongly: by a factor of 5.0 (2019) and 3.9 (2020), respectively (Figure 2). In the years 2018, 2019, and 2020 death occurred more suddenly as shown by the crown transparency one year before dieback (Figure 3).
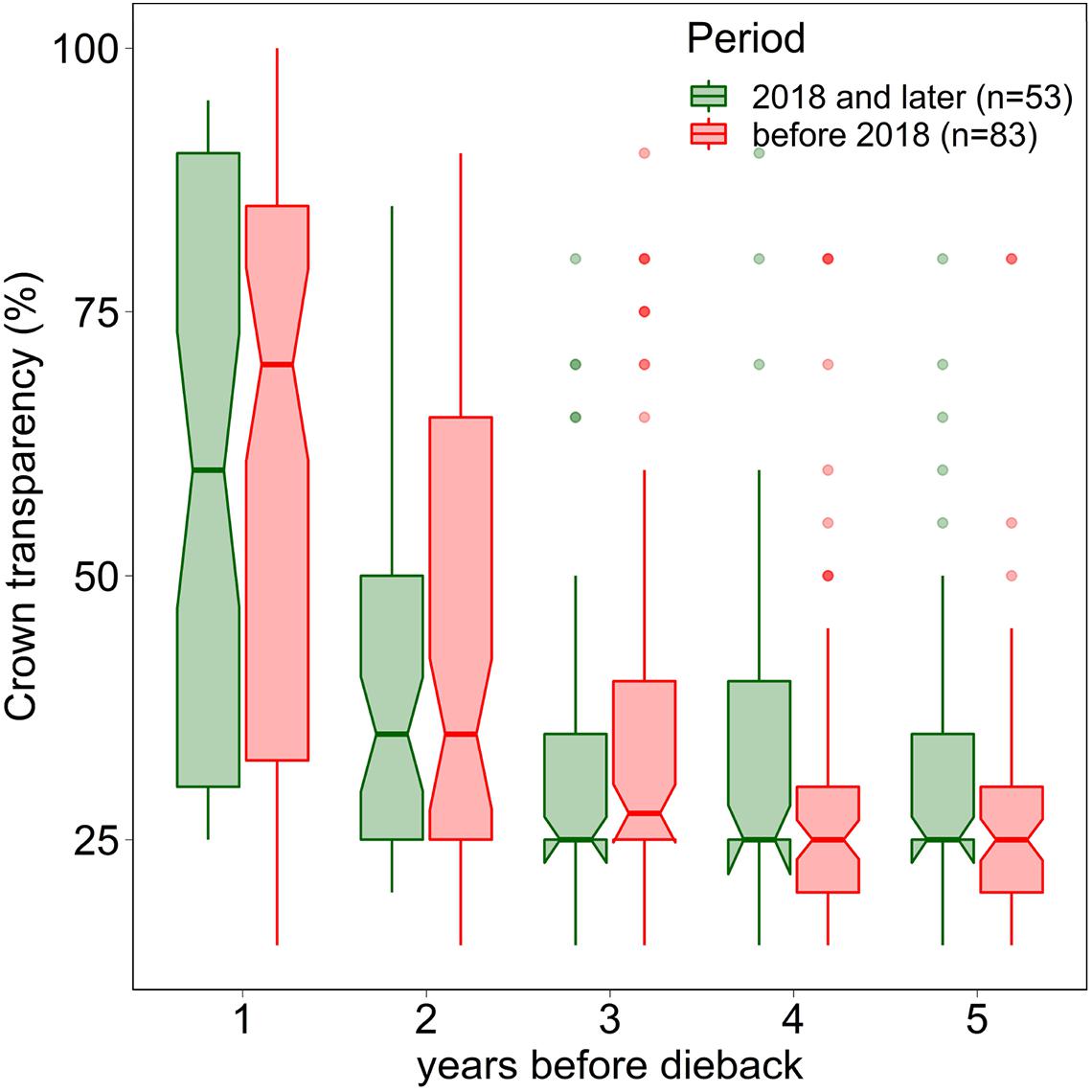
Figure 3. Crown transparency of beech in the years before dieback before 2018 (1984–2017) and in the years 2018, 2019, and 2020. Notches indicate a 95% confidence interval.
Predictors for Beech Mortality and Strong Crown Transparency
Both mortality and the proportion of strongly damaged trees were increased when foliar concentrations of P and K were low (Table 1 and Figures 4, 5). The relation of mortality with P was non-linear. For both parameters, drought was also an important predictor. The best drought indicator explaining mortality and strong crown transparency was the minimum site water balance of the previous year, two and three years before, for mortality also the precipitation in spring.
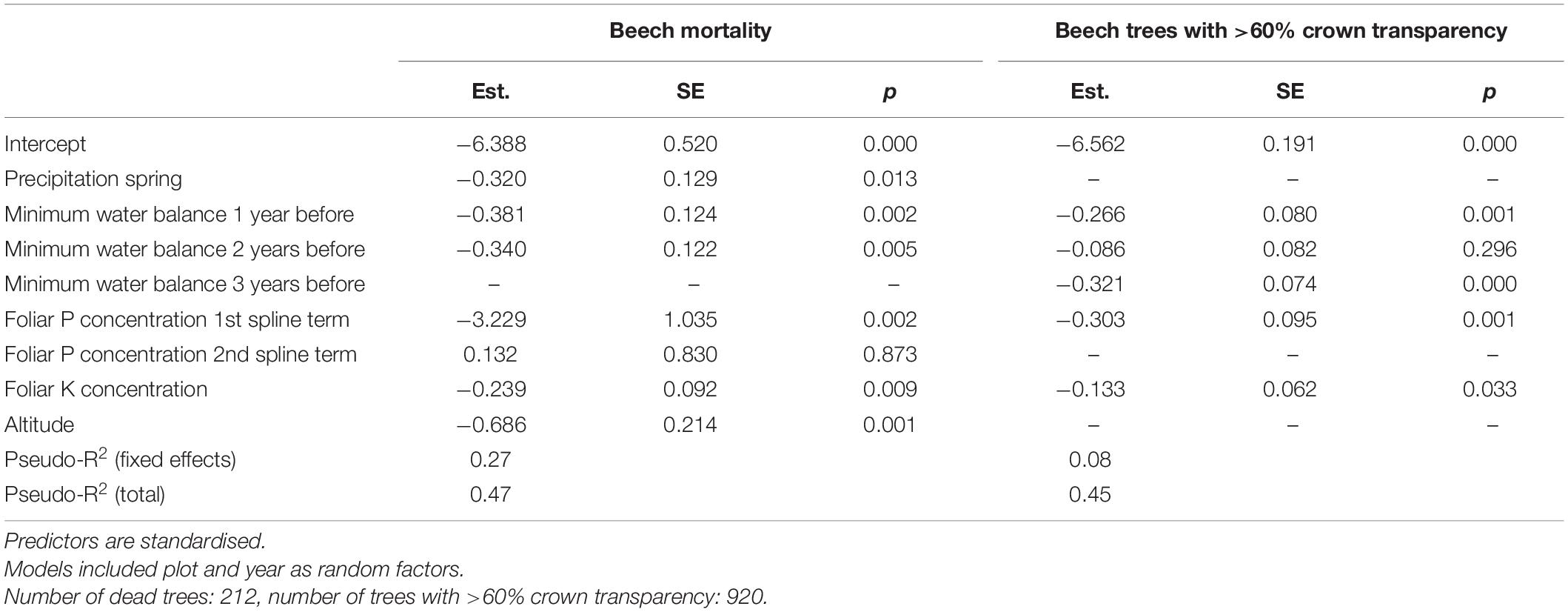
Table 1. Results of mixed binomial regression models of beech mortality (left) and trees with 60% crown transparency (right).
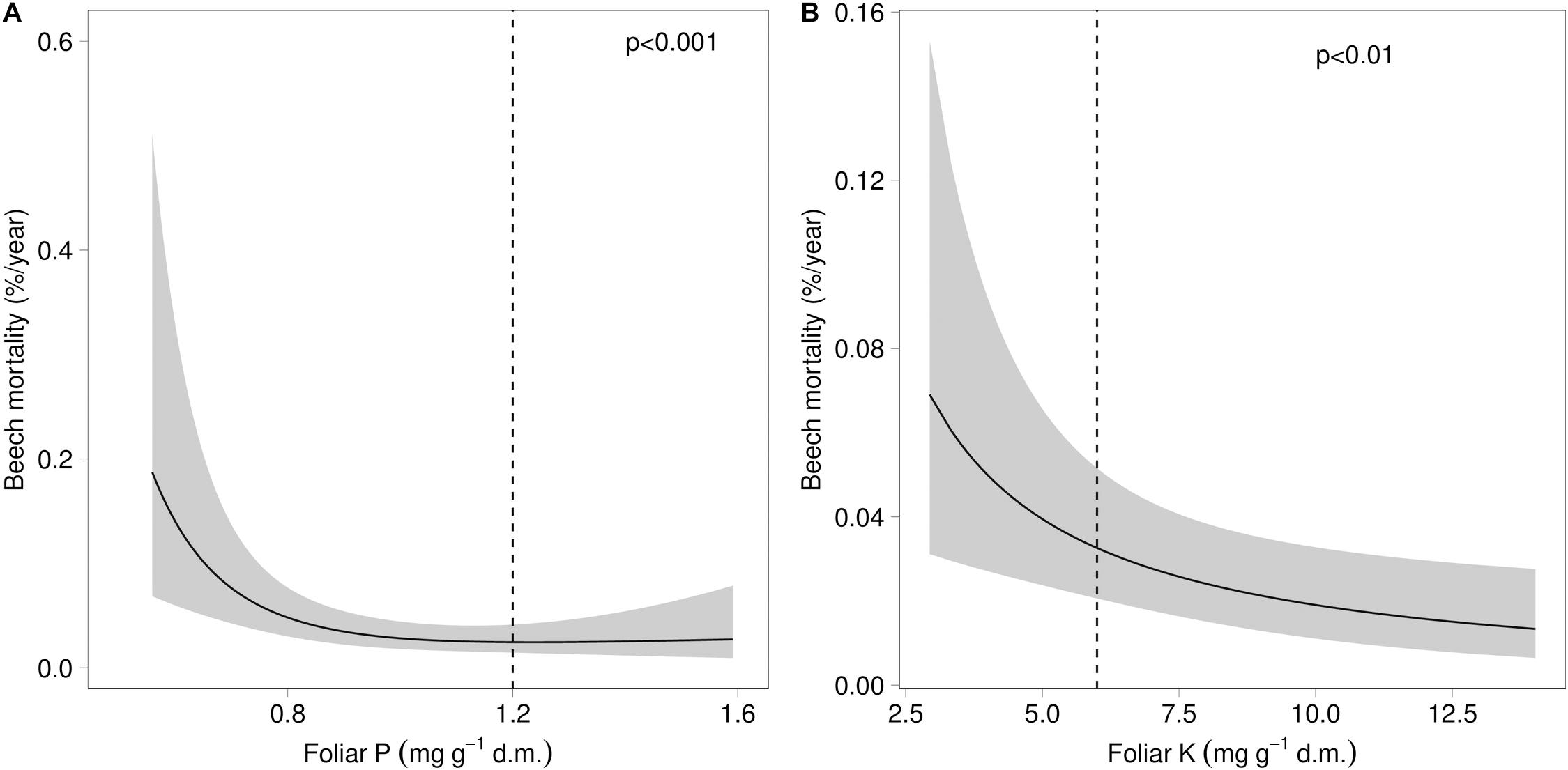
Figure 4. Relation between beech mortality and the concentration of phosphorus (A) and the concentration of potassium (B) in beech leaves. The dashed lines indicate the lower threshold value for normal nutrition according to Göttlein (2016).
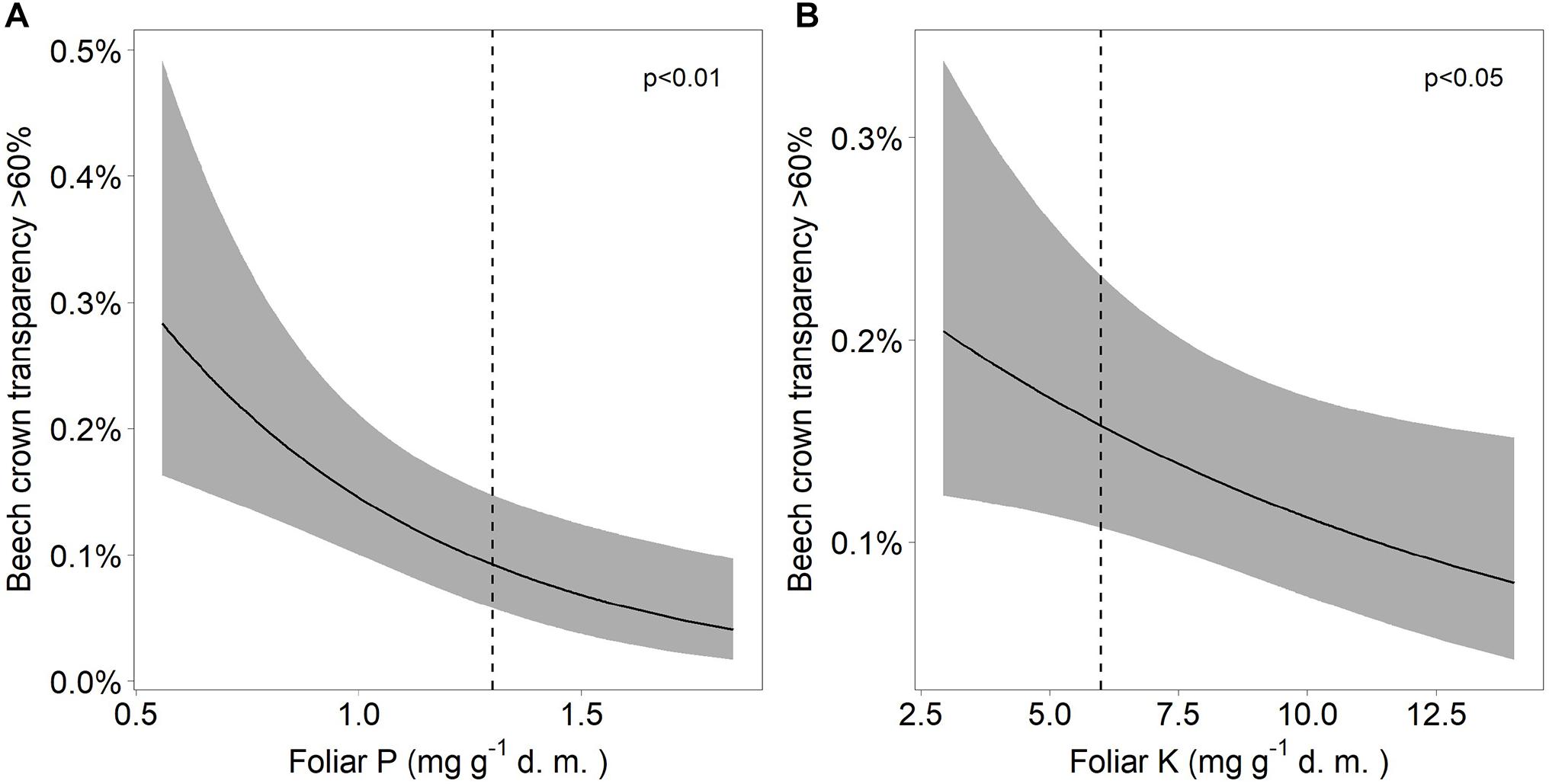
Figure 5. Relation between severe beech crown transparency and (A) the concentration of phosphorus (A) as well as the concentration of potassium (B). The dashed lines indicate the lower threshold values for normal nutrition according to Göttlein (2016).
Discoloration
Discoloration was another phenomenon which clearly increased in 2019 and 2020 (Figure 6). In addition, the phenomenon rated as photobleaching at the harvested shoots was greatly increased in 2015 and 2019 (Figure 7). For both parameters foliar P concentrations played a role. The relation with P suggested that photobleaching may be regarded as P deficiency symptom. It was analysed in a separate spline regression for showing the non-linear relations (Figure 8 left). The other predictors differed for photobleaching and discoloration. For discoloration, observed in the field together with crown transparency, the two climatic variables drought and irradiation and the fructification from the previous year improved the model (Table 2). For photobleaching the maximum July temperature and the irradiation were relevant predictors (Table 3). There was also a highly significant interaction between P concentration and maximum July temperature which is illustrated in Figure 8 right: when foliar P concentrations were low (e.g., 0.6 mg g–1), the effect of the maximum July temperature on photobleaching was stronger than when P concentrations were sufficient (e.g., 1.2 mg g–1).
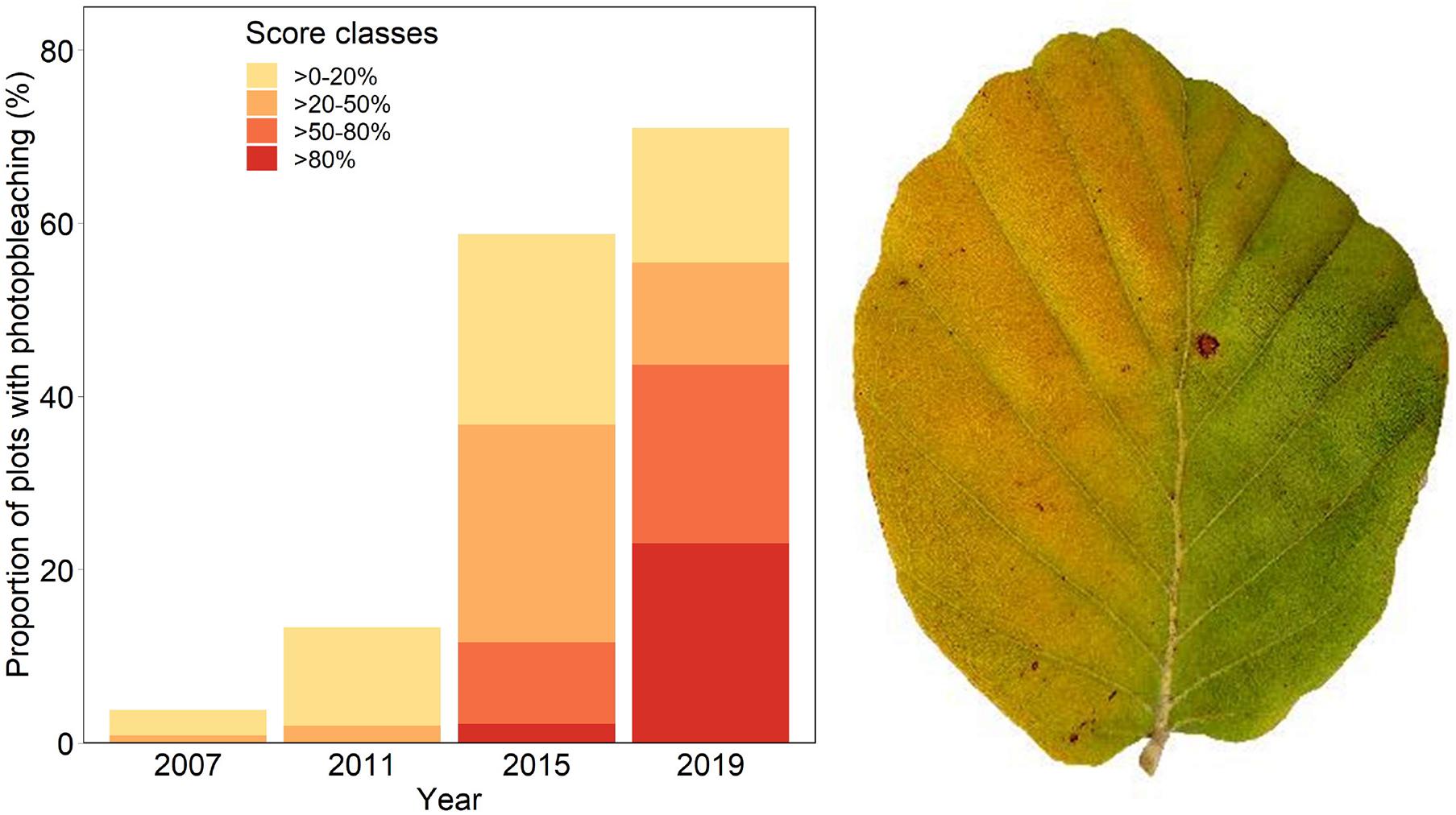
Figure 7. Frequency of occurrence of photobleaching (left) and leaf with sign of strong photobleaching (right): the right half of the leaf was covered by another leaf.
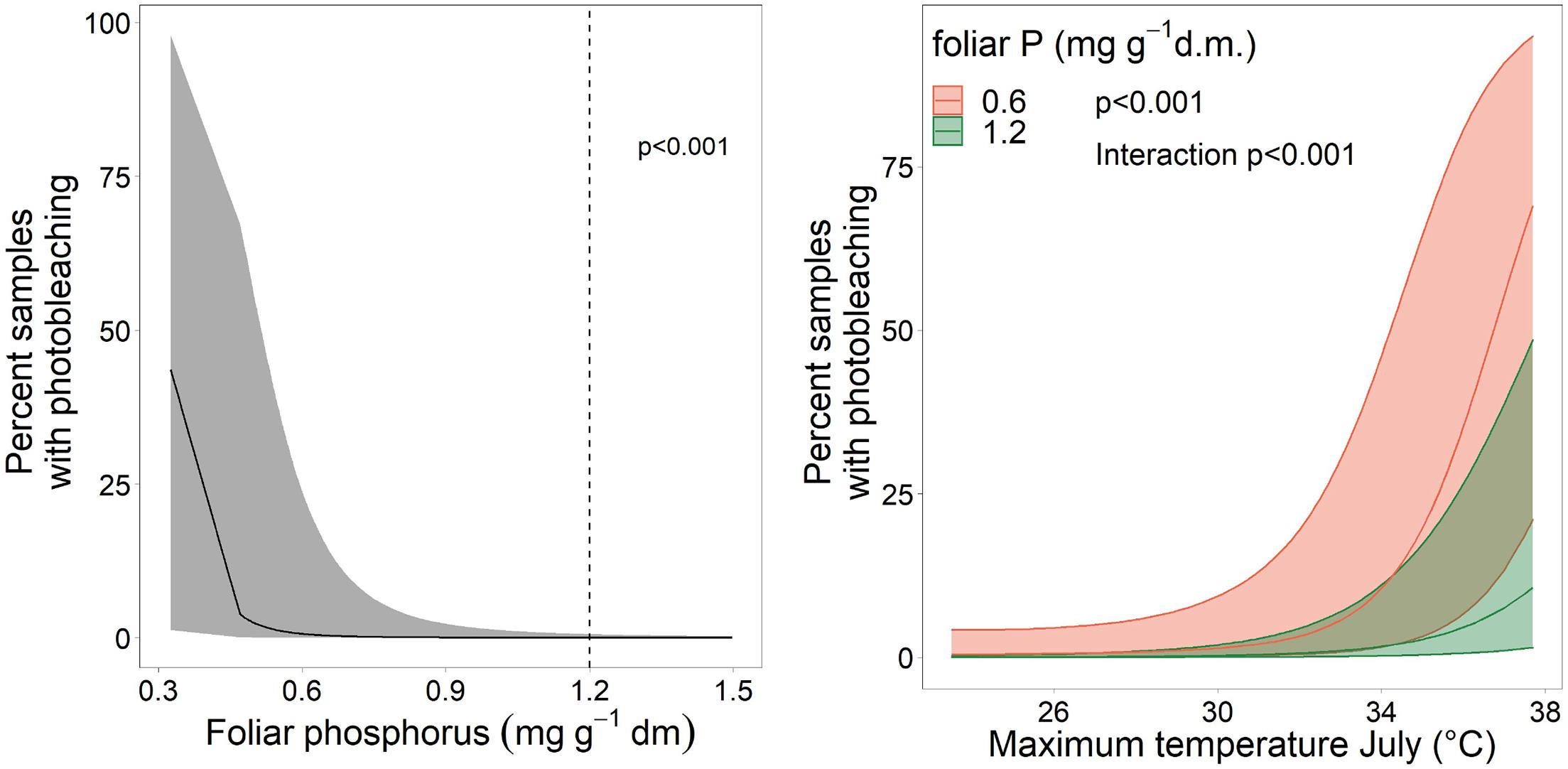
Figure 8. Left: Relation between the proportion of samples with photobleaching and the foliar concentration of P as spline function with two knots. The dotted line gives the lower threshold for normal nutrition according to Göttlein (2016). Right: Illustration of the interaction between maximum July temperature and foliar P concentration on photobleaching.
Actual Cavitation
Actual cavitation quantified as vessels stained with safranine (Figure 9) was related with drought in the previous year and with foliar N:K ratio in the current year (Figure 10). Neither drought of the actual year nor other foliar nutrients were related to current year cavitation.
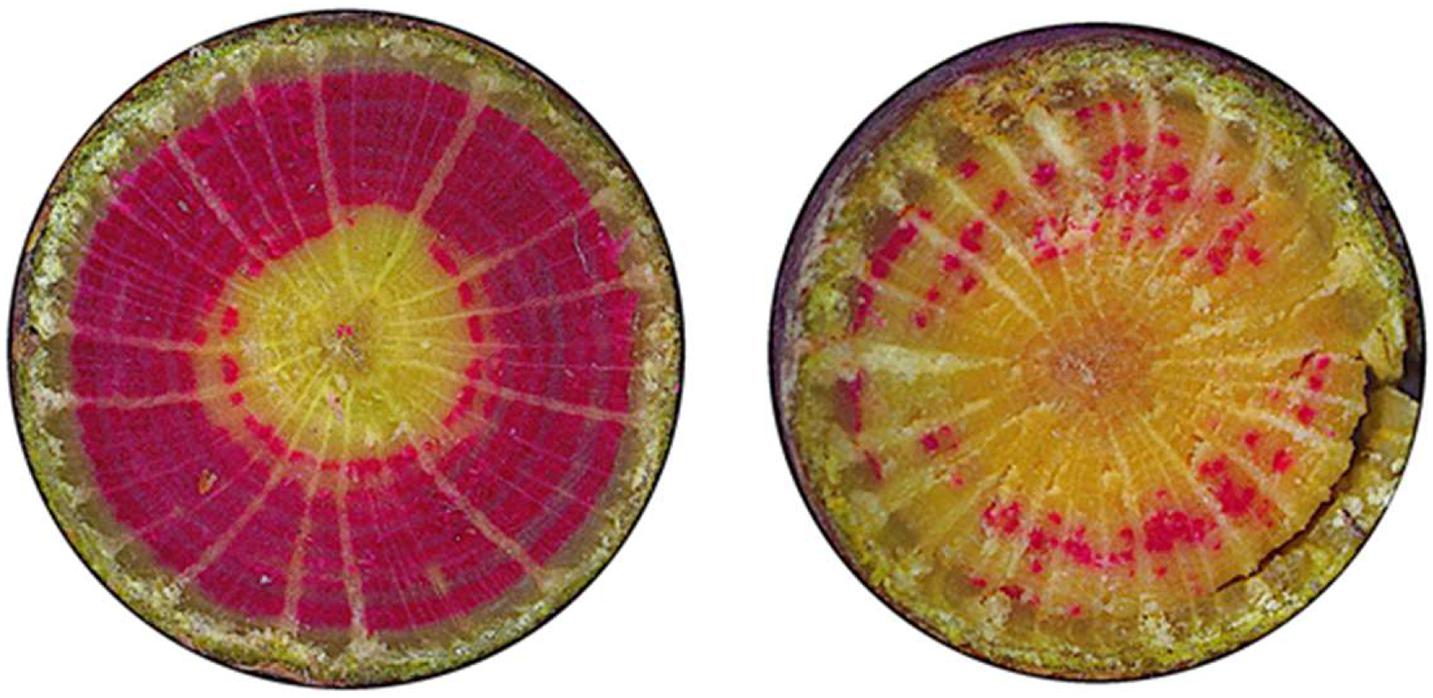
Figure 9. Staining of active vessels of beech: examples of a beech with crown transparency of 20% (left) and 70% (right).
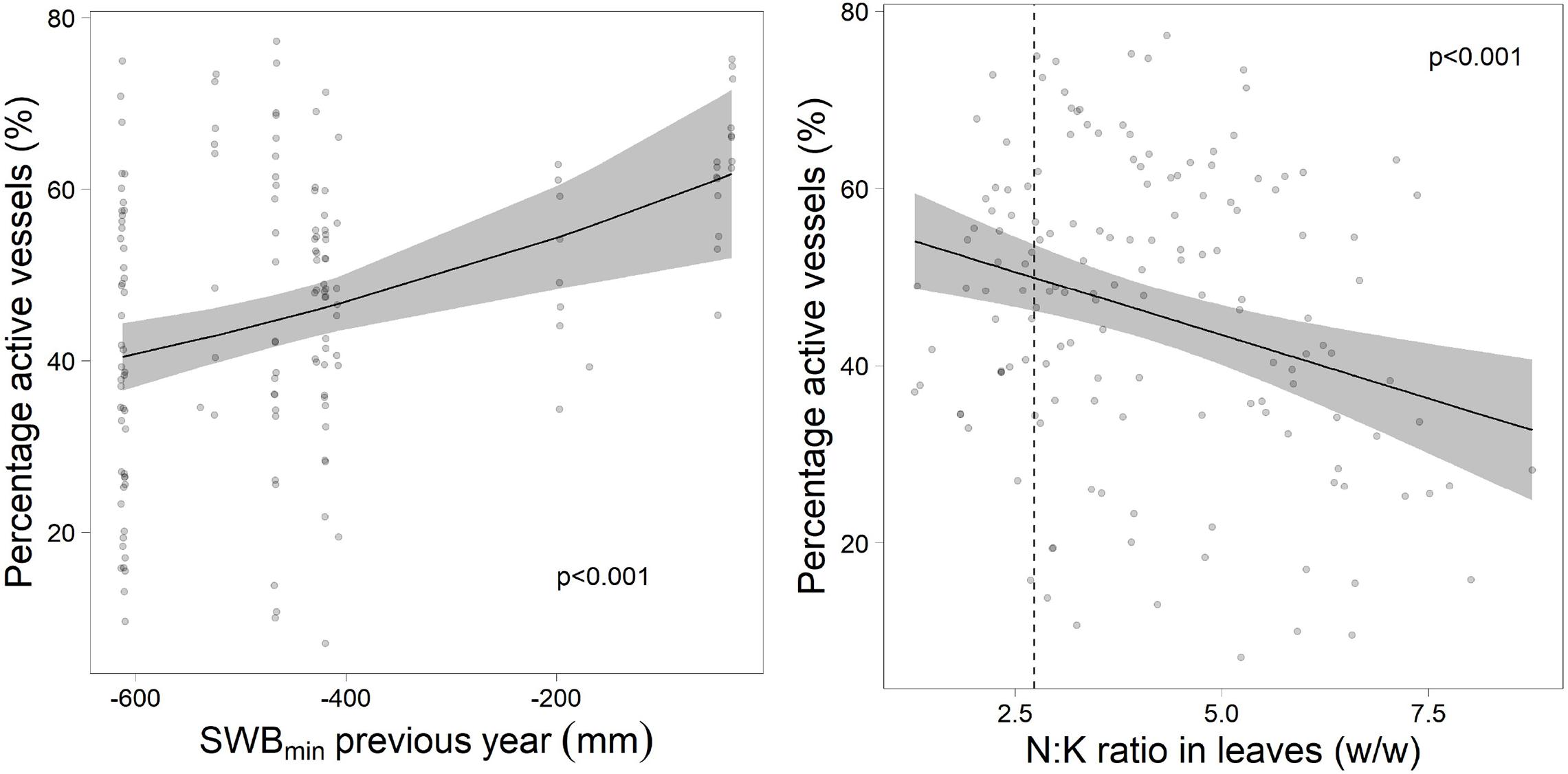
Figure 10. Proportion of active vessels in relation to the drought in the previous year (left) and the foliar ratio of N:K (right). The dotted line gives the upper threshold for harmonic N:K ratio according to Göttlein (2016).
Parasites
The observations on parasites naturally remain episodic. But there is a number of observations on known weakness parasites. Neonectria coccinea was observed frequently on dying beech trees, both the typical dark dots as well as fruit bodies. In a heavily drought affected forest district a number of uprooted beech trees were observed. Detailed examination revealed a number of primary root rot fungi which seem to be promoted by the drought: Armillaria sp., Pholiota aurivella, Meripilus giganteus.
Discussion
Effect of Drought on Crown Defoliation and the Hydraulic System of Beech
The extent of the drought induced crown dieback observed in the long-term Intercantonal Forest Observation Program in Switzerland after the severe summer drought in 2018 was out of previous experience. There have been records on drought damages of beech after the years 1947 and 1951 (Schindler, 1951; Wagenhoff and Wagenhoff, 1975). Both studies describe, however, an increased incidence of beech bark disease, a phenomenon, which has been common also in later years. In the study sites this has been associated with increased beech mortality in the 1980s. It seems that the extensive crown dieback due to hydraulic failure is a symptom which has not been known up to now in Central Europe. The drought damages observed after 2018 were much more severe than after the summer 2003 with a similar heat wave. The main differences between 2003 and 2018 were a dry spring in 2018 and a series of dry years preceding 2018 (2015 and 2017). 2003 followed a series of rather wet years, and the drought only started in June.
The increased numbers of beech trees with heavy crown defoliation and tree mortality in 2019 and 2020 provide evidence of a breakdown of the hydraulic system for parts or the entire tree crown. Beech is not known as species where catastrophic hydraulic failure is common (Leuschner, 2020). However, the actual cavitation measurements confirm the hypothesis (i) of hydraulic failure as one of the explaining factors for the observed drought damages in beech trees. The observations are in agreement with the results of Schuldt et al. (2020) who made similar staining experiments in the drought experimental plot in Hölstein, Switzerland. Branch cavitation seems to be the main reason for the serious branch dieback. Similar results were obtained by Walthert et al. (2021) who measured actual percent loss of conductivity in branch samples. The partial hydraulic failure of the branches may later lead to complete hydraulic failure of the whole tree.
Are Drought Related Damages to Vessels of Beech Reversible?
The refilling of cavitated vessels in spring is based on two processes (Cochard et al., 2001): production of new vessels, which is common in all plants with secondary cambium and active refilling, which is more species specific. This latter process has been shown to occur in Fagus sylvatica during the time of leaf expansion and sap flow (Cochard et al., 2001). Our data show that this refilling process was disturbed following the severe summer drought in 2018. This was indicated by the correlation of the proportion of active vessels with the drought of the previous year. Furthermore, the proportion of active vessels was also correlated with K nutrition. The importance of N and K nutrition for cavitation vulnerability has been shown already by Harvey and van den Driessche (1999) and more recently by Oddo et al. (2014). In the present study, low K concentrations were related to higher proportions of dead branches and higher tree mortality which may have a similar physiological background with increased cavitation.
Link Between Drought and Observed Photobleaching of Beech Leaves
The increased observed occurrence of photobleaching can be interpreted as a result of energy shortage, leading to strong discoloration in the presence of phosphorus deficiency and at high temperature levels. Photooxidation of chlorophyll by radiation is a normal concomitant process of photosynthesis requiring energy for reduction. When this energy is lacking the chlorophyll is oxidized (Powles, 1984). Therefore, photobleaching may be interpreted as a sign of “starvation”. However, as Körner (2015) suggested, plant growth is not carbon limited and in the current case the carbon starvation hypothesis should be replaced by a phosphorus limitation hypothesis which is also supported by the mortality increase at low foliar P concentrations. The strong increase in time is the result of high temperatures in 2015 and 2019, but also an effect of the interaction between P deficiency and high temperatures in July. The foliar P concentrations have decreased over the last decades, probably due to high N deposition (Braun et al., 2020). While in arable crops acute P deficiency symptoms are well known as yellowing, for tree species there are only a few descriptions of visible symptoms only for the seedling stage (Bergmann, 1992). We suggest that the observed photobleaching is regarded as a P deficiency symptom, as it starts to increase when foliar P concentrations fall below 40% of the threshold for normal nutrition.
Drought Response of Beech Due to Parasites
It is well known that weakened trees are less resistant against parasites. Several field observations from a number of plots from the long-term Intercantonal Forest Observation Program of strongly damaged trees support this hypothesis (iii). As these events are based on a small number of trees, no modeling could be done. However, the time delay observed between drought and mortality suggests that weakness parasites play an important role in the drought response of beech.
Before the severe drought in 2018, the higher crown transparency before dieback suggests that mortality due to weakness parasites played a more important role in Swiss beech. With the persisting cavitation damages since 2018 it appears to be difficult to separate between hydraulic failure effects and parasite infestation in living beech trees. Therefore, we argue that an overall assessment of the 2018 drought response of European Beech needs to take into account the drought damages due to hydraulic failure [hypothesis (i)], but also effects such as the lack of energy [hypothesis (ii)] as well as increased occurrence of weakness parasites [hypothesis (iii)].
Conclusion
With the results of 37 years of forest observation data we can conclude, that the acute reactions of beech due the summer drought in 2018 can be identified as hydraulic failure, thus confirming hypothesis (i). In a global meta-analysis, Walker et al. (2014) found that foliar P was related with carboxylation rates when leaf N was high. Carboxylation has been shown to be a key process in photobleaching (Powles, 1984): when carboxylation is limiting photosynthesis, high investment in Jmax relative to Vcmax would lead to electron transport not used in photosynthesis requiring dissipation of that energy to avoid photoinhibition. Thus, we suggest that the evidence of strong foliar P deficiency and photobleaching as well as the observed discoloration can be interpreted as a lack of energy. We are postulating to transform the “carbon starvation hypothesis” to an “energy starvation hypothesis”, since this seems to be the decisive part of the “starvation”; this is still supporting the hypothesis (ii). In particular, the increase in mortality risk at low foliar P concentrations supports a kind of starvation hypothesis (ii) as P is involved in all energy processes. However, at the moment it is still difficult to quantify the effect of weakness parasites following the severe drought, although single events have been recorded yet. In conclusion, we suggest that for the assessment of drought damage to beech trees, all aspects need to be critically evaluated in order to quantify the impact of drought events, that are expected to occur more frequently.
The damages to beech presented in this paper are clearly caused by drought. It has, however, to be kept in mind that low P and low K concentrations are a consequence of high N deposition (Braun et al., 2020). High N deposition is decreasing the mycorrhiza (de Witte et al., 2017; van der Linde et al., 2018) and thus water uptake. At high N deposition, the drought effect on stem increment of beech was stronger in the presented monitoring plots (Braun et al., 2017b).
Data Availability Statement
The original contributions presented in the study are included in the article/Supplementary Material, further inquiries can be directed to the corresponding author.
Author Contributions
SB wrote the manuscript and performed data analysis. ST made data analysis. S-EH performed forest monitoring. JR provided interpolated meteorological data. CS advised the statistical evaluation. All authors contributed to the article and approved the submitted version.
Funding
The monitoring was funded by the cantons of AG, BE, BL, BS, SO, GR, TG, and ZH and the cantons of Central Switzerland.
Conflict of Interest
The authors declare that the research was conducted in the absence of any commercial or financial relationships that could be construed as a potential conflict of interest.
Publisher’s Note
All claims expressed in this article are solely those of the authors and do not necessarily represent those of their affiliated organizations, or those of the publisher, the editors and the reviewers. Any product that may be evaluated in this article, or claim that may be made by its manufacturer, is not guaranteed or endorsed by the publisher.
Acknowledgments
We thank the local foresters and forest owners for their patience in keeping long-term monitoring. We also thank Valentine Kamm for performing the safranine analysis.
Supplementary Material
The Supplementary Material for this article can be found online at: https://www.frontiersin.org/articles/10.3389/ffgc.2021.765782/full#supplementary-material
Footnotes
- ^ https://imagej.net/Fiji/Downloads, downloaded 13.08.2019.
References
Allen, C. D., Macalady, A. K., Chenchouni, H., Bachelet, D., McDowell, N., Vennetier, M., et al. (2010). A global overview of drought and heat-induced tree mortality reveals emerging climate change risks for forests. For. Ecol. Manage. 259, 660–684. doi: 10.1016/j.foreco.2009.09.001
Anderegg, W. R. L., Hicke, J. A., Fisher, R. A., Allen, C. D., Aukema, J., Bentz, B., et al. (2015). Tree mortality from drought, insects, and their interactions in a changing climate. New Phytol. 208, 674–683. doi: 10.1111/nph.13477
Bates, D., Maechler, M., Bolker, B., and Walker, S. (2015). Fitting linear mixed-effects models using lme4. J. Stat. Softw. 67, 1–48.
Braun, S., Schindler, C., and Rihm, B. (2017b). Growth trends of beech and Norway spruce in Switzerland: the role of nitrogen deposition, ozone, mineral nutrition and climate. Sci. Total Environ. 599–600, 637–646. doi: 10.1016/j.scitotenv.2017.04.230
Braun, S., Achermann, B., De Marco, A., Pleijel, H., Karlsson, P. E., Rihm, B., et al. (2017a). Epidemiological analysis of ozone and nitrogen impacts on vegetation – critical evaluation and recommendations. Sci. Total Environ. 603–604, 785–792. doi: 10.1016/j.scitotenv.2017.02.225
Braun, S., Schindler, C., and Rihm, B. (2020). Foliar nutrient concentrations of european beech in switzerland: relations with nitrogen deposition, ozone, climate and soil chemistry. Front. For. Glob. Chang. 3:33. doi: 10.3389/ffgc.2020.00033
Buras, A., Rammig, A., and Zang, C. S. (2020). Quantifying impacts of the 2018 drought on European ecosystems in comparison to 2003. Biogeosciences 17, 1655–1672. doi: 10.5194/bg-17-1655-2020
Choat, B., Brodribb, T. J., Brodersen, C. R., Duursma, R. A., López, R., and Medlyn, B. E. (2018). Triggers of tree mortality under drought. Nature 558, 531–539. doi: 10.1038/s41586-018-0240-x
Choat, B., Jansen, S., Brodribb, T. J., Cochard, H., Delzon, S., Bhaskar, R., et al. (2012). Global convergence in the vulnerability of forests to drought. Nature 491, 752–755. doi: 10.1038/nature11688
Cochard, H., Lemoine, D., Améglio, T., and Granier, A. (2001). Mechanisms of xylem recovery from winter embolism in Fagus sylvatica. Tree Physiol. 21, 27–33.
de Witte, L. C., Rosenstock, N. P., van der Linde, S., and Braun, S. (2017). Nitrogen deposition changes ectomycorrhizal communities in Swiss beech forests. Sci. Total Environ. 605–606, 1083–1096. doi: 10.1016/j.scitotenv.2017.06.142
Engelbrecht, B. M. J. (2012). Plant ecology: forests on the brink. Nature 491, 675–676. doi: 10.1038/nature11756
García-Herrera, R., Díaz, J., Trigo, R. M., Luterbacher, J., and Fischer, E. M. (2010). A review of the european summer heat wave of 2003. Crit. Rev. Environ. Sci. Technol. 40, 267–306. doi: 10.1080/10643380802238137
Göttlein, A. (2016). Ableitung von nährelelementrelationen für die mitteleuropäischen hauptbaumarten aus dem wertebereich normaler ernährung im vergleich zu verfügbaren literaturdaten. Allg. For. Jagdzeitung 187, 237–246.
Hacke, U. G., and Jansen, S. (2009). Embolism resistance of three boreas conifer speices varies with pit structure. New Phytol. 182, 675–686. doi: 10.1111/j.1469-8137.2009.02783.x
Harvey, H. P., and van den Driessche, R. (1997). Nutrition, xylem cavitation and drought resistance in hybrid poplar. Tree Physiol. 17, 647–654.
Harvey, H. P., and van den Driessche, R. (1999). Nitrogen and potassium effect on xylem cavitation and water use efficiency in poplars. Tree Physiol. 19, 943–950.
ICP Forests (2016). Manual on Methods and Criteria for Hamonized Sampling, Assessment, Monitoring and Analysis of the Effects of Air Pollution on Forests. Hamburg: Johann Heinrich von Thünen Institute.
IPCC (2014). Climate Change 2013 – The Physical Science Basis. Cambridge: Cambridge University Press. doi: 10.1017/CBO9781107415324
Jarcuska, B., Mihal, I., Cicak, A., and Tsakov, H. (2013). Beech bark necrosis: partitioning the environmental and spatial variation of the damage severity in Central and South-Eastern Europe. Ann. For. Res. 56, 317–338.
Körner, C. (2015). Paradigm shift in plant growth control. Curr. Opin. Plant Biol. 25, 107–114. doi: 10.1016/j.pbi.2015.05.003
Kubiak, K., Zolciak, A., Damszel, M., Lech, P., and Sierota, Z. (2017). Armillaria pathogenesis under climate changes. Forests 8:100.
Leuschner, C. (2020). Drought response of European beech (Fagus sylvatica L.)—a review. Perspect. Plant Ecol. Evol. Syst. 47:125576. doi: 10.1016/j.ppees.2020.125576
Lüdecke, D. (2018). ggeffects: tidy data frames of marginal effects from regression models. J. Open Source Softw. 3:772. doi: 10.21105/joss.00772
McDowell, N. G., Beerling, D. J., Breshears, D. D., Fisher, R. A., Raffa, K. F., and Stitt, M. (2011). The interdependence of mechanisms underlying climate-driven vegetation mortality. Trends Ecol. Evol. 26, 523–532.
McDowell, N. G., and Sevanto, S. (2010). The mechanisms of carbon starvation: how, when, or does it even occur at all? New Phytol. 186, 264–266. doi: 10.1111/j.1469-8137.2010.03232.x
MeteoSwiss (2021). Wärmstes Jahrzehnt Seit Messbeginn [WWW Document]. Available online at: https://www.meteoschweiz.admin.ch/home/aktuell/meteoschweiz-blog.subpage.html/de/data/blogs/2021/1/waermstes-jahrzehnt-sein-messbeginn.html (accessed June 20, 2021).
Nakagawa, S., and Schielzeth, H. (2013). A general and simple method for obtaining R 2 from generalized linear mixed-effects models. Methods Ecol. Evol. 4, 133–142. doi: 10.1111/j.2041-210x.2012.00261.x
Oddo, E., Inzerillo, S., Grisafi, F., Sajeva, M., Salleo, S., and Nardini, A. (2014). Does short-term potassium fertilization improve recovery from drought stress in laurel? Tree Physiol. 34, 906–913. doi: 10.1093/treephys/tpt120
Powles, S. B. (1984). Photoinhibition of photosynthesis induced by visible light. Annu. Rev. Plant Physiol. 35, 15–44.
RCore Team (2020). R: A Language and Environment for Statistical Computing. Vienna: R Foundation for Statistical Computing. Available online at: https://www.R-project.org/
Schindler, U. (1951). Das Buchensterben; bisherige Veröffentlichungen und Auswertungen der staatlichen Unterlagen. Forstarchiv 22, 109–119.
Schuldt, B., Buras, A., Arend, M., Vitasse, Y., Beierkuhnlein, C., Damm, A., et al. (2020). A first assessment of the impact of the extreme 2018 summer drought on Central European forests. Basic Appl. Ecol. 45, 86–103. doi: 10.1016/j.baae.2020.04.003
Shukla, P. R., Skea, J., Slade, R., van Diemen, R., Haughey, E., Malley, J. M., et al. (2019). “IPCC technical summary 2019,” in Climate Change and Land: An IPCC Special Report on Climate Change, Desertification, Land Degradation, Sustainable Land Management, Food Security, and Greenhouse Gas Fluxes in Terrestrial Ecosystems, eds P. R. Shukla, J. Skea, E. Calvo Buendia, V. Masson-Delmotte, H.-O. Pörtner, D. C. Roberts, et al. (Geneva: IPCC).
Urli, M., Porté, A. J., Cochard, H., Guengant, Y., Burlett, R., and Delzon, S. (2013). Xylem embolism threshold for catastrophic hydraulic failure in angiosperm trees. Tree Physiol. 33, 672–683. doi: 10.1093/treephys/tpt030
van der Linde, S., Suz, L. M., Orme, C. D. L., Cox, F., Andreae, H., Asi, E., et al. (2018). Environment and host as large-scale controls of ectomycorrhizal fungi. Nature 558, 243–248. doi: 10.1038/s41586-018-0189-9
Wagenhoff, A., and Wagenhoff, E. (1975). Course and consequences of the dieback of beech [Fagus] bark at the Bovenden forest district headquarters from 1959 to 1965. Aus dem Walde, Mitt Niedersaechs Landesforstverwalt 24, 111–168.
Walker, A. P., Beckerman, A. P., Gu, L., Kattge, J., Cernusak, L. A., Domingues, T. F., et al. (2014). The relationship of leaf photosynthetic traits – V cmax and J max – to leaf nitrogen, leaf phosphorus, and specific leaf area: a meta-analysis and modeling study. Ecol. Evol. 4, 3218–3235. doi: 10.1002/ece3.1173
Walthert, L., Ganthaler, A., Mayr, S., Saurer, M., Waldner, P., Walser, M., et al. (2021). From the comfort zone to crown dieback: sequence of physiological stress thresholds in mature European beech trees across progressive drought. Sci. Total Environ. 753:141792. doi: 10.1016/j.scitotenv.2020.141792
Keywords: phosphorus nutrition, P deficiency, tree mortality, photobleaching, discoloration, hydraulic failure, energy starvation, weakness parasites
Citation: Braun S, Hopf S-E, Tresch S, Remund J and Schindler C (2021) 37 Years of Forest Monitoring in Switzerland: Drought Effects on Fagus sylvatica. Front. For. Glob. Change 4:765782. doi: 10.3389/ffgc.2021.765782
Received: 27 August 2021; Accepted: 04 October 2021;
Published: 28 October 2021.
Edited by:
Arthur Gessler, Swiss Federal Institute for Forest, Snow and Landscape Research (WSL), SwitzerlandReviewed by:
Christoph Leuschner, University of Göttingen, GermanyYasutomo Hoshika, Institute for Sustainable Plant Protection, National Research Council (CNR), Italy
Copyright © 2021 Braun, Hopf, Tresch, Remund and Schindler. This is an open-access article distributed under the terms of the Creative Commons Attribution License (CC BY). The use, distribution or reproduction in other forums is permitted, provided the original author(s) and the copyright owner(s) are credited and that the original publication in this journal is cited, in accordance with accepted academic practice. No use, distribution or reproduction is permitted which does not comply with these terms.
*Correspondence: Sabine Braun, sabine.braun@iap.ch