- School of Environmental Sciences, Jawaharlal Nehru University, New Delhi, India
This study quantifies the effect of low- to moderate-intensity anthropogenic activities and natural activities affecting less than 40% of the canopy cover on major plant functional traits and associated soil microbial diversity in western Himalayan temperate forests. Plots of 0.1 ha were placed in the temperate forests along disturbance gradient and were categorized based on lopping intensity, deadwood counts, grazing, and litter removal. Plots were classified into three classes, i.e., low disturbance intensity (LDI) and moderate disturbance intensities (MDI1 and MDI2) based on lopping activities and canopy cover. The study was conducted on functional traits related to growth and survival strategies of a species in a complex forest ecosystem. Furthermore, DNA was extracted and metagenome of soil samples was performed using Illumina MiSeq platform from three disturbance classes to study the effect of disturbance and plant traits on microbial diversity. Tree basal area was found to be most significantly affected by disturbance intensity. Total density was found to be greater for the LDI site. Specific leaf area (SLA) and crown cover (CC) were the most affected traits in the moderately disturbed sites, whereas maximum plant height (HT) and seed mass (SM) were least affected by disturbance. Soil microbial diversity was found to be negatively associated with disturbance index. Microbial biomass carbon (MBC) and microbial biomass nitrogen (MBN) were found to be significantly higher in LDI sites. Disturbance level elicited variation in microbial community composition. Proteobacteria was the most abundant phylum and Phenylobacterium, DA101, and Candidatus solibacter were mainly abundant at the genus level. A decreasing level of disturbance due to the absence of human residences in the LDI site led to the dominance of Phenylobacterium (27%) which reduced to 18% in MDI1 site. Shannon alpha bacterial diversity and plant species diversity (H′) were found to be greatest for MDI2 site. In forests with varying levels of management, treefall gaps due to low levels of logging intensity might have a similar effect to those of reduced lopping intensities and deadwood count (due to natural disturbances) in the study sites. The study concludes that moderate disturbance is important for promoting species diversity and species richness (SR), but species having conservative ecological strategies would be more prone to continued disturbance intensity. Therefore, low- to moderate-level disturbance in such forests can be used as a model for natural treefall gaps, and moderate-level disturbance intensity plays a powerful role in buffering ecosystem processes.
Introduction
Natural and anthropogenic forms of disturbances are known to alter forest structure and functions, but variation in disturbance intensity and frequency causes variable outcomes (Atkins et al., 2020). The anthropogenic forms of disturbances have abruptly increased with increase in population and urbanization and increased demand for land and forest resources causing adverse effects on climate changes. Anthropogenic disturbances such as overgrazing, logging, and litter removal directly or indirectly affect the biodiversity and ecosystem processes (Heywood and Watson, 1995). Most of the human populations reside in the range of 1,000–2,000 masl in the Indian Himalayan region (Singh, 1998). The forest ecosystem in these regions is severely impacted by human activities such as deforestation, lopping for fuel and fodder, litter removal, and frequent forest fire. While there have been numerous studies on the impact of long-term chronic disturbances on forest ecosystem in the Indian Himalayan region (Thakur et al., 2020; Kaushal et al., 2021), there has been limited evaluation of moderate to low severity disturbances (e.g., lopping that is known to alter canopy cover and litter removal that causes denudation of the ground) in the region. Low to moderate levels of disturbances could lead to a wide range of compositional and structural variation throughout the region (Fahey et al., 2015), therefore have different consequences for ecosystem functioning and services (Nave et al., 2011).
Functional traits could help in better understanding the variation of species responses to external factors (McGill et al., 2006). Specific leaf area (SLA) is known to define resource allocation strategies for acquisition and storage, thus reflecting important trade-offs between construction cost and survival under varied conditions (Poorter et al., 2008, Poorter et al., 2010; Iida et al., 2014). Also, leaves with low SLA exhibit high thickness and toughness that suggests increased resource allocation into defense than into growth (Wright and Cannon, 2001). This leads to greater leaf life span (Sterck et al., 2006), thus affecting overall leaf economic spectrum. Such species also tend to produce small seeds having less generation time (Salguero-Gómez et al., 2016). Plant functional traits are also known to be an important predictor to assess the interaction between aboveground and belowground ecosystem processes (Grigulis et al., 2013; Cantarel et al., 2015; Moreau et al., 2015). Plant diversity along with functional traits such as plant height (HT), basal area, and SLA is known to affect soil bacterial communities (Pei et al., 2016), which directly or indirectly affects belowground ecosystem processes (Cao et al., 2017) specifically in the mountainous soils (Singh and Gupta, 2018). Carbon and nitrogen intake by variation in plant litter and fine root composition are known to further influence the soil microbial biomass. The community composition altered by disturbances can further affect soil abiotic properties by inducing changes in microbial community characteristics (Bagchi et al., 2014; Delgado-Baquerizo et al., 2018) and vice versa (Demenois et al., 2018).
Disturbance can cause variation in plant functional trait composition but not community composition or vice versa (Mayfield et al., 2010). Studies in the Indian Himalayan region have focused on the effect of disturbance on species composition (Kumar and Ram, 2005; Rawal et al., 2012), but variations in functional traits have limited information. Also, despite such insights and awareness, studies linking varied levels of disturbances and plant community composition and soil microbial communities are limited in the Indian Himalayan regions (Rawat et al., 2020). The studies majorly focus on species composition and diversity; hence, the role of plant traits and community composition on soil microbial community composition remains unclear. Thus, inclusion of several plant traits and plant diversity might play an important role in governing soil bacterial diversity and composition.
This study explains the role of low- to moderate-intensity disturbance activities on plant functional traits and associated soil microbial diversity in the temperate forests of western Himalaya. Driven by the aforementioned facts and circumstances, a systematic study in reserved temperate forests of western Himalaya was conducted considering the following broad hypotheses: (i) variation in disturbance intensity would significantly impact community characteristics, plant traits, and associated microbial diversity in the studied region; and (ii) species richness (SR) and diversity will be greater following intermediate disturbance hypothesis. The present study is based upon the following objectives: (i) to observe the variation in different community characteristics across varying intensity of disturbances, (ii) to study the patterns of change in the plant functional traits and soil microbial diversity with disturbance intensity, and (iii) to investigate consistent patterns in association of soil microbial diversity and plant factors with variation in disturbance intensity.
Materials and Methods
Study Site
The study site was located in Sawana Reserved Forest which lies in Rajgarh range of Sirmour District, Himachal Pradesh (Figure 1). Its geographical range lies within 30°86′N–31°01′N and 77°38′E and 77°49′E. It covers an area of 1,395.38 ha with an altitudinal variation of 1,537–3,164 masl. Climate varies from moderate and cold to very cold at higher elevations. Temperature range from 10 to 30°C and rainfall is about 125 cm annually (District Survey Report, 2016). The forest is classified under group 12–Himalayan moist–temperate forest as per the forest classification of Champion and Seth (1968). These forests occur in pure as well as mixed forms. The soil type is clayey loam type. Granite, gneiss, micaceous, schist, and quartzite are the main rock types. Moderate to steep slope occurs throughout the region. Lopping and grazing remain to be the most important primary disturbance factors that affect the plant species diversity in the study region.
Plot Selection
The reserved forest was classified into three different categories of disturbance based on varied anthropogenic intensity and human settlements in the region, i.e., low disturbance intensity site (LDI) and moderately disturbed intensity sites (MD1 and MD2) (Table 1). Eighteen plots of 0.1 ha (31 m × 32 m) were established along the forest to sample live trees having diameter at breast height (DBH) ≥ 10 cm. Six plots were established in each disturbed site to account for better representation within each category. Plant traits were sampled from each site to study the effect of low- to moderate-level disturbances on plant growth and associated soil microbial diversity in the region. Plant functional traits were selected given that they have an impact when grown in disturbed sites. Ocular estimation based on field survey suggested deadwood counts in the region to be associated with natural disturbances, whereas anthropogenic disturbances were mainly due to human disturbances and livestock population. However, livestock populations are known to severely impact canopy species in the area. This was beyond the scope of this study because the major focus was only for tree layer (mature individuals). Lopping, defined as cutting off branches from the main stem of the tree, could be seen as the most common anthropogenic activity in the region. Lopped branches are generally used for fuel and fodder purposes. Lopping intensity was calculated in each plot by counting the lopped tree individuals against total tree individuals. The sites were also classified based on canopy cover. Canopy cover in the forest was measured using a densiometer. The categorization of canopy cover was done following the Forest Survey of India (2015). Grazing intensities were estimated based on the number of livestock dungs found in each plot during the survey, and litter removal was calculated as the percentage of bare land to the total ground cover in each plot. The information regarding different disturbance activities was collected following Rawal et al. (2012). Deadwood counts (as a source of natural disturbance) were categorized as veteran tree, standing dead tree, wind-thrown tree, fallen deadwood tree, and stump in each plot against the total number of individuals (Humphrey et al., 2002).
Floristic Survey and Trait Measurements
Tree species in the study sites were identified with the help of the glossary of forest flora provided by the Rajgarh Forest Department, Sirmour. CBH (circumference at breast height) was measured during the field survey which was later converted into DBH for determination of tree basal area. The data were quantitatively analyzed to calculate stem density, frequency, and abundance following Curtis and McIntosh (1950) for the three disturbance categories. Similarly, the Shannon–Wiener diversity index (H′) (Shannon and Weaver, 1963), species evenness (Pielou, 1966), and SR (Margalef, 1958) were also calculated for each site. All species that contributed to 90% of plot basal area (in decreasing order of species basal area) were identified for sampling. In each plot, 10 individuals per species were sampled to study all the FTs chosen for the study. Sampling was done from April to June 2019 on 287 mature tree individuals. Selection of traits has been done following Westoby (1998) and Grime (1977) who suggest summarizing plant strategy through three major axes: resource exploitation, competition ability, and response to disturbance. Leaf traits represent resource use, and plant height and crown cover (CC) for competitive ability and seed mass embody the response to perturbations (Westoby, 1998). Table 2 represents the rationale behind the selection of plant traits and associated variables. Plant height was measured using a clinometer. CC for each selected tree individual was calculated using the line intercept method (USDA, 1996). Samples were collected aiming for leaves fully expanded with little or no damage by herbivores or pathogens. Fresh weight was taken by a portable weighing machine after drying through gently blotting with tissue paper to remove any surface water. After sampling, the leaves were air dried and transported in sealed plastic bags to the laboratory. SLA and SM were calculated following Cornelissen et al. (2003).
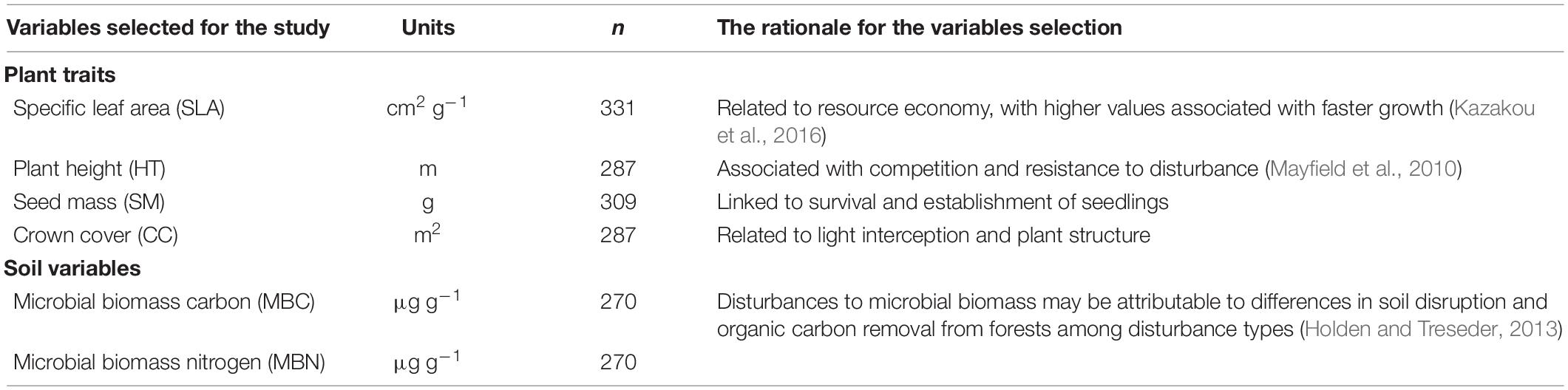
Table 2. Information regarding the selection of plant traits and soil variables and the rationale for their selection (n = number of samples collected throughout the study sites).
Soil Sampling
Five randomly located soil core samples at depths of 0–10, 10–20, and 20–30 cm were collected from each plot using a soil corer of 5 cm diameter. Soil microbial carbon and soil microbial nitrogen were estimated following the chloroform fumigation-extraction method (Brookes et al., 1984; Vance et al., 1987). Soil samples from each disturbed class were collected to make composite samples (from varying depths) that were sent for DNA extraction and purification in the laboratory.
DNA Extraction and Purification
Metagenomics sequencing was performed on the collected soil sample (0.25 g) to evaluate the relationship between microbial diversity and plant functional traits. DNA extraction was done using commercially available kits from Qiagen (Hilden, Germany), Zymo Research (Irvine, CA, United States), and Thermo Fisher (Waltham, MA, United States) followed by PCR amplification of the V3–V4 region. Forty nanograms of extracted DNA were used for amplification along with 10 pM of each primer. Twenty-five cycles each of initial denaturation were done at 95°C for 15 s, annealing at 60°C for 15 s, elongation at 72°C for 2 min, final extension at 72°C for 10 min, and hold at 4°C. The amplicons from each sample were purified with Ampure beads to remove unused primers and eight additional cycles of PCR were performed using Illumina barcoded adapters to prepare the sequencing libraries. Libraries were purified using Ampure beads and quantitated using Qubit dsDNA High Sensitivity assay kit. Sequencing was performed using Illumina MiSeq with 2 × 300 PE v3 sequencing kit. Raw data QC was done using FASTQC and MULTIQC, followed by trimming of adapters and low-quality reads by TRIMGALORE. The trimmed reads are further taken for processing which includes merging of paired end reads, chimera removal, and operational taxonomic unit (OTU) abundance calculation and estimation correction—this is achieved by QIIME/MOTHUR/KRAKEN/BRACKEN workflows (Supplementary Table 1). This workflow enables highly accurate investigations at the genus level. Each read was classified based on percent coverage and identity. Extracted DNA from the samples was subjected to NanoDrop and GEL Check before being taken for PCR amplification: the NanoDrop readings of 260/280 at an approximate value of 1.8–2 were used to determine the quality of DNA. The amplified 16S PCR product was purified and subjected to GEL Check and NanoDrop QC. The Nano Drop readings of 260/280 at an approximate value of 1.8–2 were used to determine the quality of DNA.
Statistical Analysis
Data normality was checked with Shapiro–Wilk’s test and homogeneity of variance of data with Levene’s test. Kernel density plots were drawn for each plant trait and soil variables to understand the variability within each disturbed site. One-way ANOVA followed by Tukey post hoc test was conducted to evaluate the effect of disturbance on plant functional traits, community composition, and associated soil microbial diversity, and the differences were reported significant at p < 0.001. Principal component analysis (PCA) was performed including the plant traits for 287 individuals in the study sites and soil properties from each plot. The importance value index of plant species was calculated following Curtis (1959), and its association with different plant traits and soil parameters was determined using canonical correspondence analysis (CCA). CCA was also used to explore changes in traits and diversity with forest disturbances. Pearson correlations were performed to examine the relationships between soil microbial communities, PFTs, and plant community composition. All statistical analyses were conducted using R version 3.5.0 (R Core Team, 2018) using the packages ggplot, vegan, ggbiplot, imputeTs, and corrplot respectively.
Results
Changes in Plant Community Composition and Functional Traits With Varying Disturbance Intensity
After phytosociological survey, 22 tree species having 1,220 individuals were found throughout the study site. Total basal area and tree density were found to be maximum for the LDI site (71.45 m2 ha–1 and 834.16 individuals ha–1) and least for the MDI1 site (22.86 m2 ha–1 and 546.12 individuals ha–1), respectively. Total basal area of mature individuals was found to be significantly varying among the study sites (Table 3). However, the differences were not significant for tree density. Plant SR varied from 0.88 to 2.09 being maximum for the MDI2 site and least for the LDI site (Supplementary Table 2). Species diversity (H′) did not vary much throughout the study sites. However, values for H′ were also greater for moderately disturbed sites. Values for plant traits (CC and SLA) varied significantly among the study sites, whereas there was no significant difference found for HT and SM (Table 3). HT as a function of growth and competitive vigor was found to be maximum for the MDI1 site and least for the LDI site. CC was found to be varying throughout the study site due to the effect of lopping. SM showed greater variation within the study sites reflecting fundamental divergence in the phylogenetic distance between deciduous and evergreen species (Figure 2). Maximum variation accounted for SLA suggesting that with an increase in disturbance intensity, the values shifted toward species having higher trait values for acquisitive traits such as SLA, indicating higher competitive ability and faster resource acquisition. MDI2 was found to have the greatest SLA followed by MDI1 and LDI.
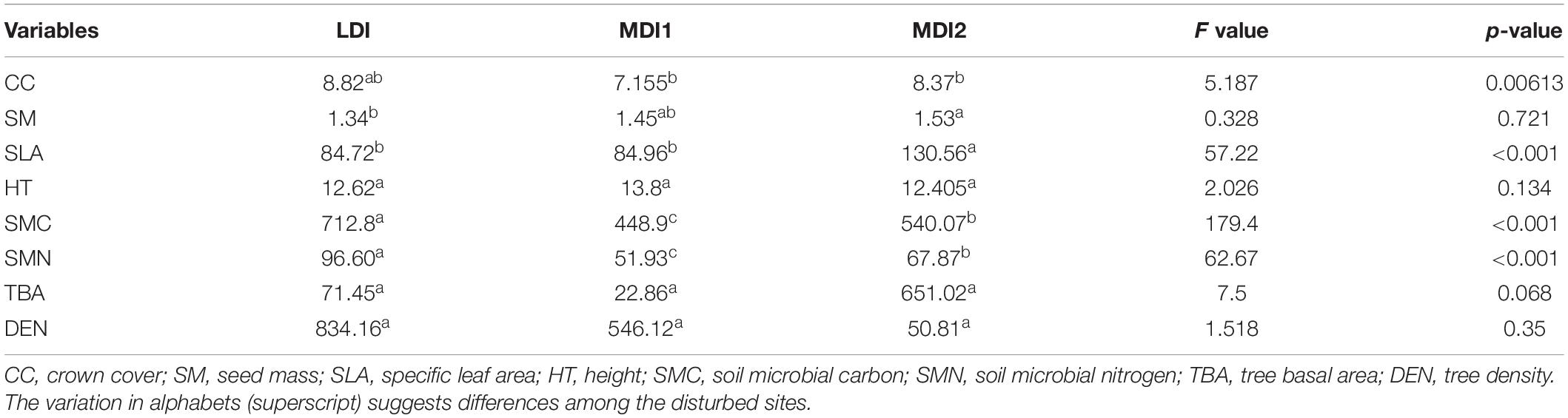
Table 3. One-way ANOVA table suggesting the effects of disturbance on plant traits, community composition, and soil properties followed by Tukey post hoc test.
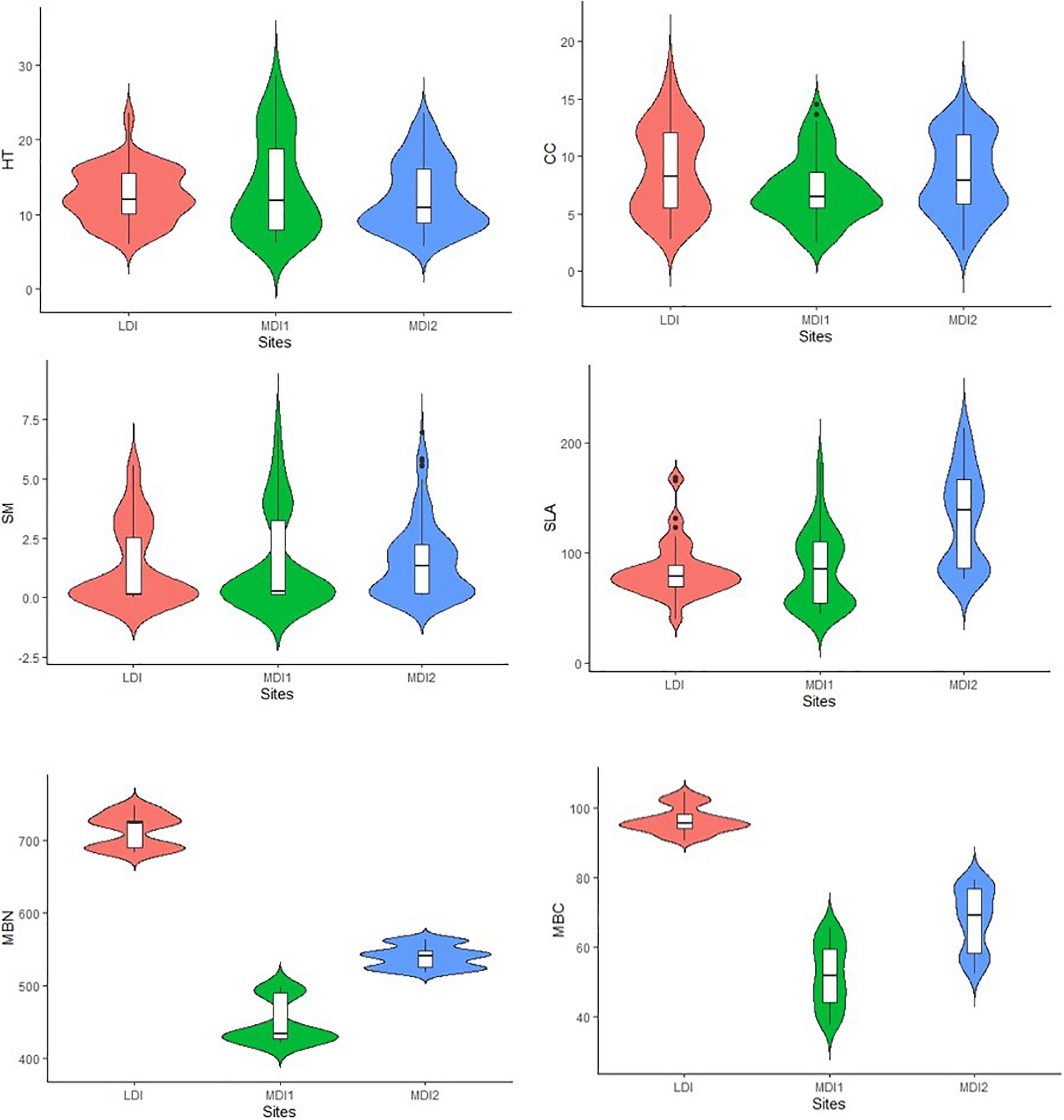
Figure 2. Violin plots representing variation in plant traits and soil variables for each disturbed class.
Changes in Soil Microbial Community Composition and Microbial Biomass C and N With Varying Disturbance Intensity
After quality trimming, a total of 123,138 sequences in moderately disturbed sites and 141,170 sequences in LDI sites were found. Analysis of soil bacterial community composition revealed sequences belonging to 19 phyla, 44 classes, 70 orders, 127 families, and 221 genera in soil samples across all the stands. At the phylum level, the soil bacterial communities were found to be dominated by Proteobacteria, Acidobacteria, Actinobacteria, Firmicutes, Planctomycetes, Verrucomicrobia, Bacteroidetes, and Cyanobacteria together accounting for more than 80% of the bacterial community composition (Figure 3). Furthermore, Nitrospirae, Gemmatimonadetes, Spirochetes, Chlamydiae, Nitrospirae, Chlorofelxi, Planctomycetes, and Fusobacteria were also present in the soil samples with varying proportions across the study sites. Proteobacteria was the most abundant phylum and Phenylobacterium, DA101, and Candidatus solibacter were mainly abundant at the genus level. Decreasing the level of disturbance with increasing elevation due to the absence of human residences in higher elevation led to the dominance of Phenylobacterium (27%) that reduced to 18% in moderately disturbed sites. The alpha diversity indices (Shannon and Simpson) revealed similar patterns to plant species diversity and richness, i.e., the values were found to be maximum for the MDI2 site and lowest for the LDI site (Supplementary Table 2). Phylotypes within Actinobacteria and Acidobacteria were found to be greater for moderately disturbed sites, whereas phylotypes within Proteobacteria, Planctomycetes, Verrucomicrobia, and Bacteroidetes were greater for LDI sites. Soil microbial biomass carbon and nitrogen were found to increase around twofold with values being significantly greater for the LDI site and least for the MDI1 site (Figure 2). One-way ANOVA test revealed that soil microbial biomass carbon and nitrogen were the most affected variables with increase in disturbance intensity (Table 3).
Relationship Between Plant Community Characteristics and Soil Microbial Diversity
Both plant species diversity and bacterial species diversity followed the same pattern being maximum for the MDI2 site and least for the LDI site. Pearson correlation coefficients calculated for plant traits, community characteristics, and soil microbial diversity along the disturbance gradient revealed that total basal area (TBA) and tree density (DEN) were found to have a positive correlation among the study sites (Figure 4). They were also found to have a strong positive correlation with soil MBC and MBN and bacterial phyla Proteobacteria, Planctomycetes, and Verrucomicrobia and negatively correlated with phyla Actinobacteria, Firmicutes, and Acidobacteria. Phyla Proteobacteria, Planctomycetes, and Verrucomicrobia were found to have a strong positive correlation with soil MBC and MBN, whereas phyla Actinobacteria, Firmicutes, and Acidobacteria were found to have a negative correlation with soil MBC and MBN. Similarly, CC was found to have negative correlation with bacterial phyla Actinobacteria, Firmicutes, and Acidobacteria and positively correlated with soil MBC and MBN and bacterial phyla Proteobacteria, Planctomycetes, Bacteroidetes, and Verrucomicrobia. Principal component analysis suggested that the first and second principal components explained 36.93 and 30.74% of data variability, respectively, while the third component accounted for 15.42% of data variability (Figure 5). The first PCA axis was associated with SLA and SM and opposed by CC, whereas the second axis was associated with MBC and MBN. HT was found to be negatively correlated with SM. CCA explained the relationship of plant community characteristics with soil variables in all the disturbed sites (Figure 6). MBN, CC, and SLA were found to be the major contributing factors in the LDI and MDI2 sites, respectively. Eigenvalues of CCA axis 1 and CCA axis 2 were 0.80 and 0.46, respectively. Variances explained by CCA axis 1 and CCA axis 2 were was 42 and 24%, respectively. Correlation between CCA axis 1 and CC was found to be positive with CC (0.66) and MBN (0.92) and negative with SLA (−0.21), SM (−0.12), and HT (−0.08).
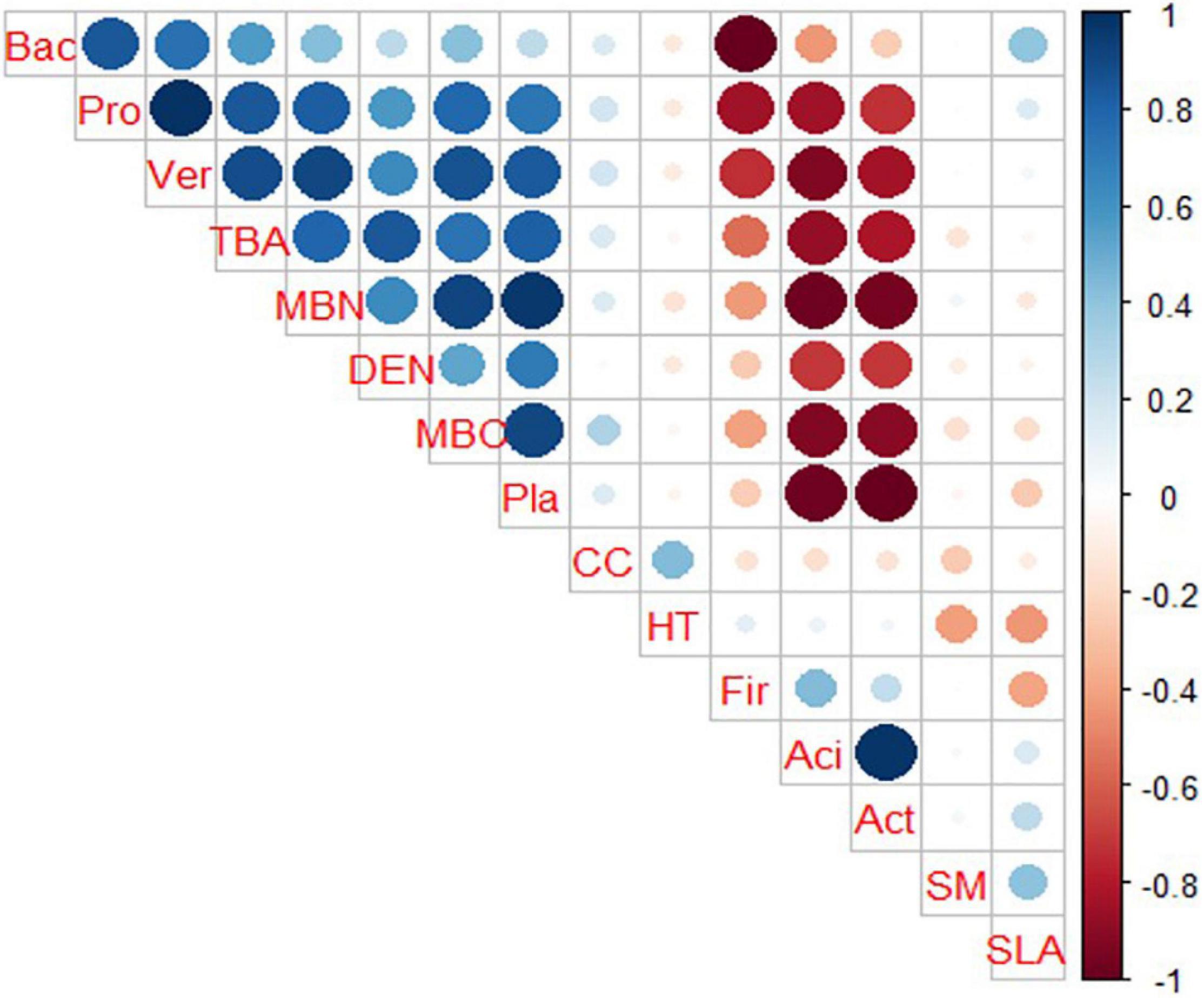
Figure 4. Correlation among plant community characteristics, functional traits and soil microbial community characteristics (CC, crown cover; Fi, Firmicutes; Aci, Acidobacteria; Act, Actinobacteria; SLA, specific leaf area; SM, seed mass; Bac, Bacteroidetes; Pro, Proteobacteria; Ver, Verrucomicrobia; SMC, soil microbial carbon; SMN, soil microbial nitrogen; DEN, tree density; Pl, Planctomycetes; TBA, total basal area; HT, plant height).
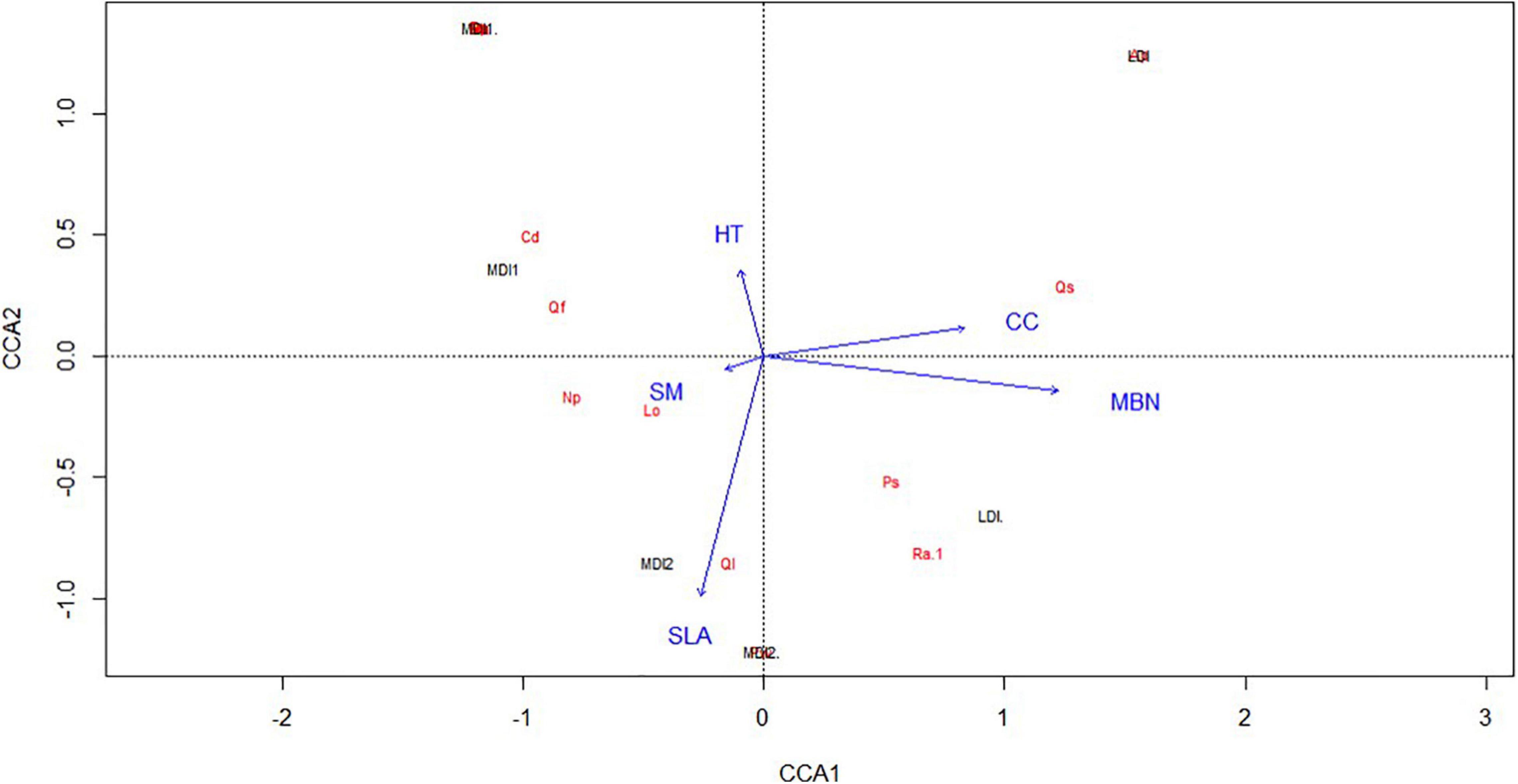
Figure 6. CCA plot diagram to analyze the major gradients among the combination of explanatory variables. The length of vectors in the ordination diagram represents the strength of the correlation, while the angle between two vectors shows the degree of correlation among variables with each axis.
Discussion
In the present study, the effect of low- to moderate-level disturbances on plant community characteristics and functional traits along with soil microbial diversity in temperate reserved forests in the western Himalayan region was explored. Tree basal area was found to be significantly varying throughout the study sites (p < 0.01). CC (p < 0.01) and SLA (p < 0.001) along with MBC (p < 0.001) and MBN (p < 0.001) were found to be significantly varying among the study sites. However, there was no effect on HT and SM based on disturbance intensity. The findings indicate that low to moderate level of disturbance does not affect the community composition, but with increasing and continued disturbance intensity, species with conservative growth strategies promoting resistance of species to environmental stresses will be replaced with species having higher trait values for acquisitive traits such as SLA, indicating higher competitive ability and faster resource acquisition. Here, the ecological significance of the present findings and their proper implications for sustainable forest management are discussed.
Changes in Plant Community Composition and Functional Traits With Varying Disturbance Intensity
Neither species diversity (H′) nor any of the plant functional traits were significantly affected by low to moderate levels of disturbance intensity. This holds for the hypothesis suggesting that SR and diversity will not be affected because of limited disturbance activities throughout the study sites. This lack of effect was probably due to regular management implications that do not allow the local people to barely denude the tree individuals which affects their growth and survival. However, SR was found to be increased by more than twofold in MDI2 sites that could be attributed to differences in management practices (Toledo et al., 2012) as well as recruitment of new species in moderately disturbed sites due to scattered canopy (Kumar, 2000). Studies have proven that mild disturbances provide greater opportunity for species turnover, establishment, and colonization, thus leading to higher SR (Whittaker and Likens, 1975; Connell, 1978; Mishra et al., 2004). Additionally, other factors might contribute to the differences among studies, such as topographical heterogeneity, environmental conditions, and forest types in the study site. Local disturbance causes tree gaps resulting in scattered canopy that creates patches of greater light availability and availability of optimum resources and nutrients for lower subcanopy and regenerating species (Raghubanshi and Tripathi, 2009). The findings tend to approach closer to the intermediate disturbance hypothesis theory which suggests an increase in species diversity with a low to moderate amount of disturbance (Connell, 1978). The hypothesis that the increasing level of lopping intensity impacts forest community characteristics holds for tree basal area that tends to decrease with increase in disturbance intensities but remains insignificant for tree density and other community characteristics. Similarly, low to moderate levels of disturbances caused no effect on species composition and diversity in Bornean rainforest (Verburg and van Eijk-Bos, 2003), tropical rainforest (Carreño-Rocabado et al., 2012), and semiarid forest (Mayfield et al., 2010).
Varying levels of disturbance intensity had a significant impact on SLA and CC, whereas there was no effect on HT and SM. With an increase in disturbance intensity, SLA was found to be maximum at MDI2 sites suggesting shifting to acquisitive traits rather than conservative traits (Kitajima and Poorter, 2010; Onoda et al., 2011), whereas for HT and SM, low to moderate disturbance promoted species with conservative traits rather than acquisitive (Loehle and Namkoong, 1987). Similar results were found in a study conducted by Hall et al. (2020) in European vineyards indicating higher photosynthetic capacity and growth rates in bare soil vineyards. This suggests a faster turnover of plants as a response to disturbance (Kazakou et al., 2016). LDI sites were found to have the lowest value for SLA, and such individuals tend to have longer leaf life spans causing lower growth rates (Louault et al., 2005). SM was not found to be significantly affected by varying disturbance intensity, but within site, variation was mainly due to phylogenetic distances between the species. Conifers in the study site are being characterized by a higher number of seeds with lower seed mass as compared with broadleaved trees that are known to have increased seed mass. CC is considered to be another trait that is highly affected by disturbance activities, especially lopping. Variation in CC further affects light intensity variation in the study site as well loss of structural components, i.e., branches and photosynthetic organs, i.e., leaves (Sterck et al., 2001). CCs are also known to be affected by topographic and edaphic conditions (Lang, 2010). HT did not vary much with disturbance but was found to be lowest for the LDI site being less light demanding hence tends to be shorter, whereas for species at the MDI1 site being higher light demanding, successional species tends to be taller (Falster and Westoby, 2005). This suggests that additional factors such as topography (slope aspect and elevation), forest types, and environmental conditions (Castro-Luna et al., 2011) might be important in governing plant traits and processes in the region. Therefore, the inclusion of the combined effect of disturbances, topographical heterogeneity, forest types, and environmental conditions might play an important role in the regulation of plant species diversity and functional traits in the study site.
Effect of Varied Disturbance Intensity on Soil Microbial Diversity
The study reported that soil bacterial communities were mostly composed of Proteobacteria, Acidobacteria, Actinobacteria, Planctomycetes, Verrucomicrobia, and Bacteroidetes across the study sites. Various previous studies confirm the present findings conducted in temperate forest ecosystems (Shen et al., 2013), subtropical forest ecosystems (Delgado-Baquerizo et al., 2018), and temperate grassland ecosystems (Zhang et al., 2016). It was found that plant species diversity and bacterial species diversity showed a similar pattern of increment with increment in disturbance intensity. Thus, soil bacterial diversity was found to be positively correlated with plant species diversity. This might be due to an increase in aboveground plant communities and belowground microbial communities. Plant communities are also known to modify the resource availability and microclimatic conditions for belowground soil level which affects microbial growth responses (Zumsteg et al., 2012). The study was also in confirmation with other studies conducted in temperate grassland ecosystems (Chai et al., 2016) and tropical forest ecosystems (Barberán et al., 2015). Proteobacteria remained to be the most abundant bacterial phylum through the study site. These are represented by fast-growing copiotrophs that are acclimatized to increased carbon content and nutrient availability (Cong et al., 2015). This was in support of this study that showed increased density and basal area at LDI sites leading to higher carbon content and nutrient accumulation than other sites. This supports the suggested hypothesis that soil bacterial diversity would be largely determined by soil attributes supporting a strong correlation between soil microbial biomass and bacterial phyla. Soil microbial biomass carbon and nitrogen remained to be the only variables that varied significantly among study sites. The values were found to be maximum for the LDI site followed by the MDI2 and MDI1 sites. Values for soil MBC and soil MBN showed a similar pattern of increment with bacterial phyla such as Proteobacteria, Verrucomicrobia, and Planctomycetes, suggesting a strong link between soil microbial biomass and community composition. Thus, the present study confirms that the role of moderate disturbances is in one way or another helpful in the regulation of aboveground and belowground ecosystem processes for sustainable growth and diversity in the region. The increased basal area and tree density at LDI sites might be the most important reason for increased MBC and MBN. This could be attributed to an increase in litter inputs which governs soil organic matter and nutrients (Tiwari et al., 2019). The values for MBC and MBN decreased with increasing depth throughout the study site.
Relationship Between Plant Community Characteristics and Soil Microbial Diversity
Many studies have been reported confirming the important link between aboveground community characteristics and belowground microbial composition at regional and landscape levels (de Vries et al., 2012; Legay et al., 2014; Sterkenburg et al., 2015). Plant community characteristics and soil microbial biomass were important predictors of soil bacterial community composition and diversity in the sampling site. The Pearson correlation coefficient diagram (Figure 5) suggested a strong positive correlation between plant community composition and soil MBC and MBN. This suggests an increased amount of litterfall due to increased basal area at LDI sites and optimum decomposition rates might lead to increased soil MBC and MBN. This was found to be consistent with other studies that suggested that soil MBC and MBN are highly influenced by carbon content in pine forests (Arunachalam et al., 1996). TBA and density were also found to have a negative correlation with bacterial phyla Actinobacteria and Acidobacteria. Actinobacteria maximally dominated in dryland regions thriving under scarce-nutrient conditions and relatively drier environments (Maestre et al., 2015). Hence, MDI1 and MDI2 sites being lower in tree basal area and density have higher counts of Actinobacteria. Similarly, CC was found to have a negative correlation with bacterial phylum Actinobacteria suggesting reduced canopy cover leads to increased solar radiation intensity and, thus, suitable conditions for phylum growth. The effect of plant traits in governing belowground microbial communities was not found in this study. This was in confirmation with other studies that found no link between plant traits and microbial communities (Barberán et al., 2015; Leff et al., 2018). A strong positive link between SMN and bacterial phyla suggests that soil nitrogen plays an important role in governing microbial composition since nitrogen is considered to be the most important limiting nutrient in the forest ecosystem (Chai et al., 2016). The first PCA axis was associated with SLA and SM and opposed by CC, whereas the second axis was associated with MBC and MBN. This suggests that species with higher SLA tend to have lower CC due to the effect of disturbance. PCA revealed that the three sites having variation in disturbance intensity (low and moderate) differed in trait values; however, a partial overlap between the MDI1 and MDI2 sites was still observed. This shows that the trait values for the LDI site are different from the MDI1 and MDI2 sites (Figure 6). The analyses confirmed that the plant traits (CC and SLA) along with MBN explained around one-third of the variation in disturbance intensities among species in all the sites. CCA explained that MBN, CC, and SLA were found to be major contributing factors in the LDI and MDI2 sites, respectively. This suggests overdependence of species present in the MDI2 site. For instance, Quercus leucotrichophora, one of the most dominant species in the region and utilized for local livelihood, is known to be heavily lopped for fuel and fodder (Kumar and Ram, 2005). Since the species remains to be restricted in certain environmental conditions, the same species could not be found in another environmental condition to account for the effect of disturbance within species.
Implications and Conclusions
Varying levels of disturbance intensity are known to affect ecosystem functioning and productivity in forest ecosystems. Positive effects of disturbance could be accounted as increased resource availability for recruitment of new species; thus, increased diversity and SR in MDI2 sites and negative effects could be considered through variation in plant functional traits throughout the sites. The study concludes that moderate disturbance is important for promoting species diversity and SR by recruiting new species to the community leading to mixing of pioneer and climax-community species in the region. Plant traits related to competitive ability (height) and resource exploitation (SLA) were suggested to be good predictors of disturbance effect in the study. Our study suggests that species with more conservative ecological strategies would be more prone to continued disturbance intensity. The Himalayan forests being highly fragile should be protected following periodic forest management with initiation at the site level, to maintain sustainable ecosystem services and biodiversity in the region.
Data Availability Statement
The datasets presented in this study can be found in online repositories. The names of the repository/repositories and accession number(s) can be found below: NCBI (accession: PRJNA691764, PRJNA692097, and PRJNA692098).
Author Contributions
The author confirms being the sole contributor of this work and has approved it for publication.
Funding
The research was funded by University Grants Commission, India, Ref. No.: 3787(NET-DEC 2018).
Conflict of Interest
The author declares that the research was conducted in the absence of any commercial or financial relationships that could be construed as a potential conflict of interest.
Publisher’s Note
All claims expressed in this article are solely those of the authors and do not necessarily represent those of their affiliated organizations, or those of the publisher, the editors and the reviewers. Any product that may be evaluated in this article, or claim that may be made by its manufacturer, is not guaranteed or endorsed by the publisher.
Acknowledgments
The author is grateful to Late Shri Ambadutt Sharma (Forest Guard) for his continuous support during the sampling period. The author is also thankful to Abhishek K. Verma (Forest Ecology and Climate Change Division, FRI, Dehradun) for his help in statistical analysis.
Supplementary Material
The Supplementary Material for this article can be found online at: https://www.frontiersin.org/articles/10.3389/ffgc.2021.710658/full#supplementary-material
References
Arunachalam, A., Maithani, K., Pandey, H. N., and Tripathi, R. S. (1996). The impact of disturbance on detrital dynamics and soil microbial biomass of a Pinus kesiya forest in north-east India. For. Ecol. Manage 88, 273–282. doi: 10.1016/s0378-1127(96)03801-7
Atkins, J. W., Bond-Lamberty, B., Fahey, R. T., Haber, L. T., Stuart-Haëntjens, E., Hardiman, B. S., et al. (2020). Application of multidimensional structural characterization to detect and describe moderate forest disturbance. Ecosphere 11:e03156. doi: 10.1002/ecs2.3156
Bagchi, R., Gallery, R. E., Gripenberg, S., Gurr, S. J., Narayan, L., Addis, C. E., et al. (2014). Pathogens and insect herbivores drive rainforest plant diversity and composition. Nature 506, 85–88. doi: 10.1038/nature12911
Barberán, A., McGuire, K. L., Wolf, J. A., Jones, F. A., Wright, S. J., Turner, B. L., et al. (2015). Relating below ground microbial composition to the taxonomic, phylogenetic, and functional trait distributions of trees in a tropical forest. Ecol. Lett. 18, 1397–1405. doi: 10.1111/ele.12536
Brookes, P. C., Powlson, D. S., and Jenkinson, D. S. (1984). Phosphorus in the soil microbial biomass. Soil boil. Biochem. 16, 169–175. doi: 10.1016/0038-0717(84)90108-1
Cantarel, A. A., Pommier, T., Desclos-Theveniau, M., Diquélou, S., Dumont, M., Grassein, F., et al. (2015). Using plant traits to explain plant–microbe relationships involved in nitrogen acquisition. Ecology 96, 788–799. doi: 10.1890/13-2107.1
Cao, C., Zhang, Y., Qian, W., Liang, C., Wang, C., and Tao, S. (2017). Land-use changes influence soil bacterial communities in a meadow grassland in Northeast China. Solid Earth 8, 1119–1129. doi: 10.5194/se-8-1119-2017
Carreño-Rocabado, G., Peña-Claros, M., Bongers, F., Alarcón, A., Licona, J. C., and Poorter, L. (2012). Effects of disturbance intensity on species and functional diversity in a tropical forest. J. Ecol. 100, 1453–1463. doi: 10.1111/j.1365-2745.2012.02015.x
Castro-Luna, A. A., Castillo-Campos, G., and Sosa, V. J. (2011). Effects of selective logging and shifting cultivation on the structure and diversity of a tropical evergreen forest in south-eastern Mexico. J. Trop. For. Sci. 1, 17–34.
Chai, Y., Yue, M., Wang, M., Xu, J., Liu, X., Zhang, R., et al. (2016). Plant functional traits suggest a change in novel ecological strategies for dominant species in the stages of forest succession. Oecologia 180, 771–783. doi: 10.1007/s00442-015-3483-3
Champion, H. G., and Seth, S. K. (1968). A Revised Survey of the Forest Types of India. Delhi: Manager of publications.
Cong, J., Yang, Y., Liu, X., Lu, H., Liu, X., Zhou, J., et al. (2015). Analyses of soil microbial community compositions and functional genes reveal potential consequences of natural forest succession. Sci. Rep. 5:10007.
Connell, J. H. (1978). Diversity in tropical rain forests and coral reefs. Science 199, 1302–1310. doi: 10.1126/science.199.4335.1302
Cornelissen, J. H. C., Lavorel, S., Garnier, E., Diaz, S., Buchmann, N., Gurvich, D. E., et al. (2003). A handbook of protocols for standardised and easy measurement of plant functional traits worldwide. Aust. J. Bot. 51, 335–380. doi: 10.1071/bt02124
Curtis, J. T. (1959). The Vegetation of Wisconsin: An Ordination of Plant Communities. Madison, WI: University of Wisconsin Pres.
Curtis, J. T., and McIntosh, R. P. (1950). The interrelations of certain analytic and synthetic phytosociological characters. Ecology 31, 434–455. doi: 10.2307/1931497
de Vries, F. T., Manning, P., Tallowin, J. R., Mortimer, S. R., Pilgrim, E. S., Harrison, K. A., et al. (2012). Abiotic drivers and plant traits explain landscape-scale patterns in soil microbial communities. Ecol. Lett. 15, 1230–1239. doi: 10.1111/j.1461-0248.2012.01844.x
Delgado-Baquerizo, M., Oliverio, A. M., Brewer, T. E., Benavent-González, A., Eldridge, D. J., Bardgett, R. D., et al. (2018). A global atlas of the dominant bacteria found in soil. Science 359, 320–325. doi: 10.1126/science.aap9516
Demenois, J., Rey, F., Ibanez, T., Stokes, A., and Carriconde, F. (2018). Linkages between root traits, soil fungi and aggregate stability in tropical plant communities along a successional vegetation gradient. Plant Soil 424, 319–334. doi: 10.1007/s11104-017-3529-x
District Survey Report (2016). District Survey Report of District Sirmour. Himachal Pradesh: District Survey Report.
Fahey, R. T., Fotis, A. T., and Woods, K. D. (2015). Quantifying canopy complexity and effects on productivity and resilience in late-successional hemlock– hardwood forests. Ecol. App. 25, 834–847. doi: 10.1890/14-1012.1
Falster, D. S., and Westoby, M. (2005). Alternative height strategies among 45 dicot rain forest species from tropical Queensland. Aust. J. Ecol. 93, 521–535. doi: 10.1111/j.0022-0477.2005.00992.x
Grigulis, K., Lavorel, S., Krainer, U., Legay, N., Baxendale, C., Dumont, M., et al. (2013). Relative contributions of plant traits and soil microbial properties to mountain grassland ecosystem services. J. Ecol. 101, 47–57. doi: 10.1111/1365-2745.12014
Grime, J. P. (1977). Evidence for the existence of three primary strategies in plants and its relevance to ecological and evolutionary theory. Am. Nat. 111, 1169–1194. doi: 10.1086/283244
Hall, R. M., Penke, N., Kriechbaum, M., Kratschmer, S., Jung, V., Chollet, S., et al. (2020). Vegetation management intensity and landscape diversity alter plant species richness, functional traits and community composition across European vineyards. Agric. Syst. 177:102706. doi: 10.1016/j.agsy.2019.102706
Heywood, V. H., and Watson, R. T. (1995). Global Biodiversity Assessment. Cambridge: Cambridge university press.
Holden, S. R., and Treseder, K. K. (2013). A meta-analysis of soil microbial biomass responses to forest disturbances. Front. Microbiol. 4:163. doi: 10.3389/fmicb.2013.00163
Humphrey, J., Stephenson, A., Whitfield, P., and Swailes, J. (2002). Life in the Deadwood: a Guide to Managing Deadwood in Forestry Commission Forests. Edinburgh: Forest Enterprise.
Iida, Y., Kohyama, T. S., Swenson, N. G., Su, S. H., Chen, C. T., Chiang, J. M., et al. (2014). Linking functional traits and demographic rates in a subtropical tree community: the importance of size dependency. J. Ecol. 102, 641–650. doi: 10.1111/1365-2745.12221
Kaushal, S., Siwach, A., and Baishya, R. (2021). Diversity, regeneration, and anthropogenic disturbance in major Indian Central Himalayan forest types: implications for conservation. Biodivers. Conserv. 1, 1–30.
Kazakou, E., Fried, G., Richarte, J., Gimenez, O., Violle, C., and Metay, A. (2016). A plant trait based response-and-effect framework to assess vineyard inter-row soil management. Bot. Lett. 163, 373–388. doi: 10.1080/23818107.2016.1232205
Kitajima, K., and Poorter, L. (2010). Tissue-level leaf toughness, but not lamina thickness, predicts sapling leaf lifespan and shade tolerance of tropical tree species. New phytol. 186, 708–721. doi: 10.1111/j.1469-8137.2010.03212.x
Kumar, A. (2000). Plant Biodiversity in Forests of Middle Central Himalaya in Relation to Various Disturbances. Doctoral dissertation, Ph.D. thesis, Nainital: Kumaun University.
Kumar, A., and Ram, J. (2005). Anthropogenic disturbances and plant biodiversity in forests of Uttaranchal, central Himalaya. Biodivers. Conserv. 14, 309–331. doi: 10.1007/s10531-004-5047-4
Leff, J. W., Bardgett, R. D., Wilkinson, A., Jackson, B. G., Pritchard, W. J., Jonathan, R., et al. (2018). Predicting the structure of soil communities from plant community taxonomy, phylogeny, and traits. ISME J. 12, 1794–1805. doi: 10.1038/s41396-018-0089-x
Legay, N., Baxendale, C., Grigulis, K., Krainer, U., Kastl, E., Schloter, M., et al. (2014). Contribution of above-and below-ground plant traits to the structure and function of grassland soil microbial communities. Ann. Bot. 114, 1011–1021. doi: 10.1093/aob/mcu169
Loehle, C., and Namkoong, G. (1987). Constraints on tree breeding: growth tradeoffs, growth strategies, and defensive investments. For. Sci. 33, 1089–1097.
Louault, F., Pillar, V. D., Aufrere, J., Garnier, E., and Soussana, J. F. (2005). Plant traits and functional types in response to reduced disturbance in a semi-natural grassland. J. Veg. Sci. 16, 151–160.
Maestre, F. T., Delgado-Baquerizo, M., Jeffries, T. C., Eldridge, D. J., Ochoa, V., Gozalo, B., et al. (2015). Increasing aridity reduces soil microbial diversity and abundance in global drylands. Proc. Natl. Acad. Sci. U S A. 112, 15684–15689. doi: 10.1073/pnas.1516684112
Mayfield, M. M., Bonser, S. P., Morgan, J. W., Aubin, I., McNamara, S., and Vesk, P. A. (2010). What does species richness tell us about functional trait diversity? predictions and evidence for responses of species and functional trait diversity to land-use change. Glob. Ecol. Biogeogr. 19, 423–431.
McGill, B. J., Enquist, B. J., Weiher, E., and Westoby, M. (2006). Rebuilding community ecology from functional traits. Trends Ecol. Evol. 21, 178–185. doi: 10.1016/j.tree.2006.02.002
Mishra, B. P., Tripathi, O. P., Tripathi, R. S., and Pandey, H. N. (2004). Effects of anthropogenic disturbance on plant diversity and community structure of a sacred grove in Meghalaya, northeast India. Biodivers. Conserv. 13, 421–436. doi: 10.1023/b:bioc.0000006509.31571.a0
Moreau, D., Pivato, B., Bru, D., Busset, H., Deau, F., Faivre, C., et al. (2015). Plant traits related to nitrogen uptake influence plant-microbe competition. Ecology 96, 2300–2310. doi: 10.1890/14-1761.1
Nave, L. E., Gough, C. M., Maurer, K. D., Bohrer, G., Hardiman, B. S., Le Moine, J., et al. (2011). Disturbance and the resilience of coupled carbon and nitrogen cycling in a north temperate forest. J. Geophys. Res. Biogeosci. 116, 1–14.
Onoda, Y., Westoby, M., Adler, P. B., Choong, A. M., Clissold, F. J., Cornelissen, J. H., et al. (2011). Global patterns of leaf mechanical properties. Ecol. Lett. 14, 301–312. doi: 10.1111/j.1461-0248.2010.01582.x
Pei, Z., Eichenberg, D., Bruelheide, H., Kröber, W., Kühn, P., Li, Y., et al. (2016). Soil and tree species traits both shape soil microbial communities during early growth of Chinese subtropical forests. Soil Biol. Biochem. 96, 180–190. doi: 10.1016/j.soilbio.2016.02.004
Pielou, E. C. (1966). The measurement of diversity in different types of biological collections. J. Theor. Biol. 13, 131–144. doi: 10.1016/0022-5193(66)90013-0
Poorter, H., Niinemets, Ü, Walter, A., Fiorani, F., and Schurr, U. (2010). A method to construct dose-response curves for a wide range of environmental factors and plant traits by means of a meta-analysis of phenotypic data. J. Exp. Bot. 61, 2043–2055. doi: 10.1093/jxb/erp358
Poorter, L., Wright, S. J., Paz, H., Ackerly, D. D., Condit, R., Ibarra-Manriquez, G., et al. (2008). Are functional traits good precictors of demographic rates? evidence from five neotropical forests. Ecology 89, 1908–1920. doi: 10.1890/07-0207.1
R Core Team (2018). R: A Language and Environment for Statistical Computing. Vienna: R Foundation for Statistical Computing.
Raghubanshi, A. S., and Tripathi, A. (2009). Effect of disturbance, habitat fragmentation and alien invasive plants on floral diversity in dry tropical forests of Vindhyan highland: a review. Trop. Ecol. 50:57.
Rawal, R. S., Gairola, S., and Dhar, U. (2012). Effects of disturbance intensities on vegetation patterns in oak forests of Kumaun, west Himalaya. J. Mount. Sci. 9, 157–165.
Rawat, M., Arunachalam, K., Arunachalam, A., Alatalo, J. M., Kumar, U., Simon, B., et al. (2020). Relative contribution of plant traits and soil properties to the functioning of a temperate forest ecosystem in the Indian Himalayas. Catena 194:104671. doi: 10.1016/j.catena.2020.104671
Salguero-Gómez, R., Jones, O. R., Jongejans, E., Blomberg, S. P., Hodgson, D. J., Mbeau-Ache, C., et al. (2016). Fast–slow continuum and reproductive strategies structure plant life-history variation worldwide. Proc. Natl. Acad. Sci. U.S.A. 113, 230–235. doi: 10.1073/pnas.1506215112
Shannon, C. E., and Weaver, W. (1963). The Mathematical Theory of Information. Urbana, ILL: University of Illinois Press.
Shen, C., Xiong, J., Zhang, H., Feng, Y., Lin, X., Li, X., et al. (2013). Soil pH drives the spatial distribution of bacterial communities along elevation on Changbai Mountain. Soil Biol. Biochem. 57, 204–211. doi: 10.1016/j.soilbio.2012.07.013
Singh, J. S., and Gupta, V. K. (2018). Soil microbial biomass: a key soil driver in management of ecosystem functioning. Sci. Total Environ. 634, 497–500. doi: 10.1016/j.scitotenv.2018.03.373
Singh, S. P. (1998). Chronic disturbance, a principal cause of environmental degradation in developing countries. Environ. Conserve 25, 1–2. doi: 10.1017/s0376892998000010
Sterck, F. J., Bongers, F., and Newbery, D. M. (2001). Tree architecture in a Bornean lowland rain forest: intraspecific and interspecific patterns. Plant Ecol. 153, 279–292. doi: 10.1007/978-94-017-3606-0_22
Sterck, F. J., Poorter, L., and Schieving, F. (2006). Leaf traits determine the growth-survival trade-off across rain forest tree species. Am. Nat. 167, 758–765. doi: 10.2307/3844782
Sterkenburg, E., Bahr, A., Brandström Durling, M., Clemmensen, K. E., and Lindahl, B. D. (2015). Changes in fungal communities along a boreal forest soil fertility gradient. New Phytol. 207, 1145–1158. doi: 10.1111/nph.13426
Thakur, S., Negi, V. S., Pathak, R., Dhyani, R., Durgapal, K., and Rawal, R. S. (2020). Indicator based integrated vulnerability assessment of community forests in Indian west Himalaya. For. Ecol. Manag 457:117674. doi: 10.1016/j.foreco.2019.117674
Tiwari, S., Singh, C., Boudh, S., Rai, P. K., Gupta, V. K., and Singh, J. S. (2019). Land use change: a key ecological disturbance declines soil microbial biomass in dry tropical uplands. J. Environ. Manage 242, 1–10. doi: 10.1016/j.jenvman.2019.04.052
Toledo, M., Poorter, L., Peña-Claros, M., Alarcon, A., Balcazar, J., Leano, C., et al. (2012). Driving factors of forest growth: a reply to Ferry et al. (2012). J. Ecol. 100, 1069–1073. doi: 10.1111/j.1365-2745.2012.01990.x
Vance, E. D., Brookes, P. C., and Jenkinson, D. S. (1987). An extraction method for measuring soil microbial biomass C. Soil Biol. Biochem. 19, 703–707. doi: 10.1016/0038-0717(87)90052-6
Verburg, R., and van Eijk-Bos, C. (2003). Effects of selective logging on tree diversity, composition and plant functional type patterns in a Bornean rain forest. J. Veg. Sci. 14, 99–110. doi: 10.1111/j.1654-1103.2003.tb02132.x
Westoby, M. (1998). A leaf-height-seed (LHS) plant ecology strategy scheme. Plant Soil 199, 213–227.
Whittaker, R. H., and Likens, G. E. (1975). “The biosphere and man,” in Primary Productivity of the Biosphere, eds H. Lieth and R. H. Whittaker (Berlin: Springer), 305–328. doi: 10.1007/978-3-642-80913-2_15
Wright, I. J., and Cannon, K. (2001). Relationships between leaf lifespan and structural defences in a low-nutrient, sclerophyll flora. Func. Ecol. 15, 351–359. doi: 10.1046/j.1365-2435.2001.00522.x
Zhang, C., Liu, G., Xue, S., and Wang, G. (2016). Soil bacterial community dynamics reflect changes in plant community and soil properties during the secondary succession of abandoned farmland in the Loess Plateau. Soil Biol. Biochem. 97, 40–49. doi: 10.1016/j.soilbio.2016.02.013
Keywords: functional traits, lopping intensity, microbial diversity, species richness, western Himalaya
Citation: Singh S (2021) Low- to Moderate-Level Forest Disturbance Effects on Plant Functional Traits and Associated Soil Microbial Diversity in Western Himalaya. Front. For. Glob. Change 4:710658. doi: 10.3389/ffgc.2021.710658
Received: 17 May 2021; Accepted: 06 July 2021;
Published: 02 August 2021.
Edited by:
Rishikesh Singh, Panjab University, IndiaReviewed by:
Abhishek Kumar, Panjab University, IndiaPrasant Kumar Singh, Government Vijay Bhushan Singh Deo Girls College, India
Copyright © 2021 Singh. This is an open-access article distributed under the terms of the Creative Commons Attribution License (CC BY). The use, distribution or reproduction in other forums is permitted, provided the original author(s) and the copyright owner(s) are credited and that the original publication in this journal is cited, in accordance with accepted academic practice. No use, distribution or reproduction is permitted which does not comply with these terms.
*Correspondence: Shipra Singh, c2hpcHJhOTFzaW5naEBnbWFpbC5jb20=