- Department of Forestry, Mississippi State University, Mississippi State, MS, United States
The southeastern United States has wide-scale potential to achieve high productivity from elite eastern cottonwood and hybrid poplar varietals to produce renewable bioenergy and bioproducts. In order to determine how environmental drivers impact water use and growth so that individuals can maintain growth during drought periods, varietals that use water efficiently, and/or tolerate water stress conditions, are needed to make planting recommendations across a variety of sites. Additionally, inoculation with nitrogen-fixing endophytic bacteria may improve water stress tolerance. The goals of this research were (1) to determine water use strategies using measurements of diurnal sapflow and differences in leaf retention for three eastern cottonwood (Populus deltoides, ST66, S7C8, and 110412) and three hybrid poplar (two P. deltoides × Populus maximowiczii, 6329 and 8019, and one Populus trichocarpa × P. deltoides, 5077) varietals on contrasting field sites, (2) determine the physiological impact of endophyte inoculation, and (3) determine which physiological parameters were most highly correlated with aboveground biomass. We found that whole-tree water use efficiency (WUE) was similar across varietals at 5.2 g biomass per kg water used and that water use scaled with tree size. We found that water use strategies in terms of scaled stomatal sensitivity to vapor pressure deficit converged across varietals under stressful soil water conditions at both sites, but that varietals 8019 and 110412 tended to exhibit the highest plasticity in stomatal sensitivity exhibiting the largest range in scaled stomatal sensitivity under different soil moisture conditions. Endophyte inoculation increased growth and stomatal sensitivity at the nitrogen-limited site. Leaf area, whole-tree WUE, and plasticity in stomatal sensitivity were correlated with aboveground biomass production across sites and varietals. Overall, these data can be used to model hydrologic impacts of large-scale Populus biofuel production as well as recommend varietals with efficient water use and stomatal sensitivity under a range of soil and atmospheric moisture stress factors.
Introduction
Short rotation woody crops (SRWCs) including Populus species gained popularity in the past as a prime feedstock for quality printing paper, however, Populus is now considered important as a renewable source of bioenergy and a wide array of bioproducts. The southeastern United States has the potential to provide substantial biofuel products to the United States and global customers due to its existing forestry infrastructure, land base and favorable climate. Both elite eastern cottonwood (Populus deltoides Bartr. ex Marsh.) as well as hybrid poplar varietals have been developed for superior growth, survival, disease resistance and wood characteristics (Ceulemans et al., 1992; Bandaru et al., 2015). Other traits including water use have been less commonly explored in these varietals, but may be important if water use is high and widespread planting occurs (Bloemen et al., 2017) as these fast-growing woody crops have the potential to deplete groundwater resources and alter hydrology (Rijtema and De Vries, 1994; Sevigne et al., 2011). However, water use impacts are likely site and varietal specific with some studies finding that water use in hybrid poplars was similar to natural forests (Perry et al., 2001), and others finding that water use of hybrid poplar plantations exceeded annual precipitation (Jassal et al., 2013; Jones et al., 2017). On the other hand, high water use in varietals may be beneficial if SRWCs are planted to provide ecosystem services for phytoremediation or excessive nutrient removal for water quality improvement (Zalesny et al., 2006; Dimitriou et al., 2009).
In order for SRWC production to be financially viable, sites should be capable of high productivity, located near processing refineries, or an economical source of transportation (Stoof et al., 2015) and should not compete with existing food production (Dipesh et al., 2017). These factors may restrict planting locations to sites with more limited water availability than prime agricultural land (Zalesny et al., 2016) and irrigation may not be feasible depending on costs and profit margins (Coyle et al., 2013). Across the genus, poplars have been shown to exhibit either a conservative (isohydric) strategy in which they restrict water use and, in turn, productivity under water stress conditions [low soil moisture and/or high vapor pressure deficit (VPD)] or a more risky (anisohydric) strategy in which they continue to function under increasing water stress (Fichot et al., 2009; Silim et al., 2009; Attia et al., 2015; Navarro et al., 2020). Under a conservative water use strategy, trees restrict the loss of water by closing stomata under high water stress conditions which protects their water transport pathway, but limits photosynthesis by restricting CO2 entry into leaves. In trees with an anisohydric strategy, stomata remain open during increasing water stress, risking hydraulic failure but maintaining photosynthetic rates (Tardieu and Simonneau, 1998; Klein, 2014). By analyzing the response of stomata to increasing VPD, stomatal sensitivity can be discerned and the water use strategy of Populus varietals can be placed on an isohydricity continuum (Schmidt-Walter et al., 2014). In addition to leaf-level responses, whole-tree responses to water stress may also play a critical role including more allocation to root growth and decreases in total leaf area due to leaf senescence during the growing season. Finally, inoculation of trees with diazotrophic endophyte bacteria may improve water stress tolerance. In addition to improved disease resistance against Melampsora leaf rust and poplar stem canker (Raghavendra and Newcombe, 2013; Ren et al., 2013) and increased nutrient status through nitrogen fixation and improved soil acquisition (Kandel et al., 2017; Rho et al., 2018), endophytes may improve water stress tolerance (Rho et al., 2018). In Populus deltoides × Populus nigra, inoculation with endophytes increased total biomass by 28% and root biomass by 42%, and reduced damage by reactive oxygen species to provide potential drought tolerance (Rogers et al., 2012; Khan et al., 2016). Both choosing Populus varietals that exhibit the best water stress strategies for site conditions and potentially improving water stress tolerance with endophyte inoculation can increase the sites available for planting Populus SRWCs.
The water use strategies and total water use of Populus varietals over the growing season remain important for its influence on site hydrology, however the amount of water used per unit aboveground biomass produced, or whole-tree water use efficiency (WUE), is also important when comparing varietals as well as providing perspective for their water use. Attia et al. (2015) found a 10-fold difference in WUE for various Populus genotypes. The possibility of selecting genotypes with high productivity and high WUE would allow for short rotation Populus to be grown with lower impacts on surrounding hydrology, groundwater recharge and streamflow (Petzold et al., 2011). However, results are mixed in terms of the relationship between WUE and productivity with some studies finding that the most productive genotypes were also the most water use efficient (Zhang et al., 2004; Monclus et al., 2009; King et al., 2013), and other studies finding no relationship between productivity and WUE (Rae et al., 2004; Marron et al., 2005; Monclus et al., 2005, 2006; Bonhomme et al., 2008; Dillen et al., 2011). The lack of agreement in correlations between productivity and WUE may be due to limited variability in site and climate conditions (Prasolova et al., 2003; Rae et al., 2004) and/or the parameters used to estimate a tree’s WUE. Water use efficiency can be estimated at differing scales including at the leaf-level as the ratio of photosynthesis and transpiration or from carbon isotope ratios and discrimination of various tissues which provide a proxy for temporally and spatially integrated WUE (Rae et al., 2004; Monclus et al., 2005, 2009; Bonhomme et al., 2008; Dillen et al., 2011; Broeckx et al., 2014) based on theory and equations from Farquhar et al. (1989). Although relatively easy to measure, carbon isotope discrimination needs to be interpreted with caution when inferring WUE (Broeckx et al., 2014) because other physiological parameters may be a larger driver of carbon isotopic differences than water use (Gilbert et al., 2011). These discrepancies may be resolved by presenting WUE, not at the leaf level or with carbon isotopes, but instead at the tree level where values of whole-tree biomass per unit water use across the growing season will provide more meaningful data and also capture tree-level responses to water stress including leaf area reductions (Monclus et al., 2006).
This research sought to answer the following research objectives: (1) Determine water use strategies for six Populus varietals (three eastern cottonwood and three hybrid poplars) planted on research sites on the Upper Gulf Coastal Plain and the Lower Mississippi Alluvial Valley (LMAV) and determine if strategies differ depending on planting site, (2) determine if Populus varietals differ in their leaf retention in response to summer water stress and leaf pathogens, (3) determine if inoculation with diazotrophic endophyte bacteria impacts growth and water use strategies in these tested varietals and if site conditions impact the endophyte effect, and (4) determine 2-year aboveground biomass production for all varietals at both sites and identify physiological parameters that are most correlated with growth. Tested water use parameters include seasonal water use, and whole-tree WUE as well as canopy stomatal conductance and its correlation with environmental parameters including soil moisture, VPD and photosynthetic photon flux density (PPFD). Overall, this research provides information to better model the water use and productivity potential of the tested Populus varietals in the southeastern United States (Headlee et al., 2013a; Stanturf et al., 2019) to better predict ecosystem impacts of SRWCs and their productivity potential in the region. This research also provides additional information to match planted varietals to sites where they will perform optimally.
Materials and Methods
Study Sites, Varietals and Experimental Design
Two field sites were planted in 2018, one on the Gulf Coastal Plain in northeastern Mississippi (“Coastal Plain”; 33°51′ N, 88°17′ W) and one in the “Delta” portion of Mississippi in the LMAV (“LMAV”, 33°24′ N, 90°11′ W). The Coastal Plain site consists of Prentiss fine silty loam soils that are moderately well drained (Natural Resources Conservation Service, 2021). Soils to a depth of 66 cm were reported to have an oven dry bulk density of 1.59 g cm–3, an available water capacity of 0.14 cm cm–1, a clay content of about 13%, a sand content of about 54% and a silt content of about 33% (Natural Resources Conservation Service, 2021). This site did not receive intensive agricultural production prior to this study but was previously planted in peanuts. The LMAV site consists of somewhat poorly drained Tensas silty clay loam soils (Natural Resources Conservation Service, 2021) that tend to be saturated throughout the winter and early spring. Soils to a depth of 100 cm were reported to have an oven dry bulk density of about 1.62 g cm–3, an available water capacity of 0.17 cm cm–1, a clay content of about 41%, a sand content of about 20%, and a silt content of about 39% (Natural Resources Conservation Service, 2021). This site has been in row crop agriculture for decades and had previously been planted in soybeans. Before planting, sites were tilled and subsoiled to a depth of about 35.5 cm. Six Populus varietals were planted at each site. Tested varietals consisted of three eastern cottonwood varietals (P. deltoides × P. deltoides, “D × D”) including 110412 (D × D), S7C8 (D × D), ST66 (D × D), and three hybrid poplar varietals, two of which were P. deltoides × Populus maximowiczii A. Henry crosses [“D × M”; 6329 (D × M); identification #: 284-93-6335 and 8019 (D × M); identification #: 605-97-19163] and one Populus trichocarpa (Torr. & Gray ex Hook.) × P. deltoides cross [“T × D”; 5077 (T × D); identification #: 123-91-1903]. These hybrid poplar varietals were selected for study because they showed some resistance to Septoria musiva stem canker, a fungal pathogen prevalent in the southern United States, in previous studies. Eastern cottonwood varietals exhibit natural resistance to S. musiva (Newcombe and Ostry, 2001). Eastern cottonwood cuttings, approximately 40.6 cm long, were collected during winter, 2018 from the Mississippi State University, Department of Forestry Cottonwood Cutting Orchard, soaked in water and insecticide (Admire®, Pro, Bayer Corp., Whippany, NJ, United States) for approximately 18 h and kept in plastic bags in a walk-in freezer at −2°C until planting. Dormant, unrooted hybrid poplar cuttings, approximately 30.5 cm long, were obtained from Greenwood Resources Inc. (Portland, OR, United States) and placed into the walk-in freezer upon receipt. Before planting, hybrid cuttings were soaked in a water/insecticide solution for approximately 3 to 4 days prior to planting.
Cuttings were planted in the subsoiled rows that were approximately 2.74 m apart with cuttings spaced every 1.83 m within rows. The Coastal Plain site was planted on April 18, 2018 and the LMAV site was planted on June 14, 2018 due to extended soil saturation that impeded site preparation. This late planting date likely impeded establishment survival and growth with survival at the LMAV site ranging from 10 to 50% across varietals and year one heights ranging from 1.6 to 2.4 m. At the Coastal Plain site survival ranged from 89 to 100% and year one heights ranged from 4 to 5.6 m. Each varietal was planted in 15-tree varietal plots (3 trees × 5 trees) replicated across five (at the LMAV site) or six (at the Coastal Plain site) replicate blocks depending of site size limitations. Twelve varietal plots were contained within each replicate block and half received an inoculation of diazotrophic endophyte bacteria shortly after planting while the other half remained uninoculated. Endophytic bacteria isolated from Populus and Salix spp. were obtained from Intrinsyx Bio (Moffett Federal Airfield, Mountain View, CA, United States). Endophytes were encapsulated inside calcium alginate “beads” and were placed in approximately 25 cm deep holes next to the planted cuttings. Immediately after planting, the test site was broadcast treated with a preemergent combination of Goal 2XL (Dow AgroSciences LLC, Indianapolis, IN, United States) and Pendulum 3.3 EC (BASF, Florham Park, NJ, United States) to control both broadleaf and grass competition. During the first growing season, sites were maintained in “free to grow” conditions by controlling herbaceous competition through tilling, hand-weeding around trees and chemical herbicide applications. Sites were examined in the second growing season to determine if additional weed control was necessary and no herbaceous weed control was performed as trees had grown above herbaceous competition. Cottonwood leaf beetle (Chrysomela scripta Fabricius) larvae were found at the Coastal Plain site during the first and second growing seasons and were treated with a ground application of insecticide (Admire®, Pro) in August, 2018 and aerial applications in July and September, 2019. A small amount of cottonwood leaf beetle feeding was observed at the LMAV site, however, damage was not extensive enough to warrant an insecticide application.
At both sites, environmental monitoring sensors were installed in the beginning of the first growing season. Atmospheric conditions including air temperature and relative humidity were monitored with a sensor (HMP60 Temperature and Relative Humidity Probe; Vaisala, Helsinki, Finland) located about 1.8 m aboveground and these data were used to calculate VPD (Goff and Gratch, 1946). PPFD (LI190SB Quantum Sensor; LI-COR Biosciences Inc., Lincoln, NE, United States) and rainfall (TE525MM Rain Gauge; Texas Electronics, Dallas, TX, United States) were measured near plots in an open location. Thirty-cm-long time domain reflectometry probes (CS 616 Time Domain Reflectometry Probe; Campbell Scientific Inc., Logan, UT, United States) were installed vertically to continuously measure volumetric soil moisture at four locations across each field site. At the LMAV site, soil moisture sensors were continually damaged by animals, therefore only one sensor located beneath the battery box was maintained continuously at that site. All environmental sensors were connected to a CR1000 datalogger (Campbell Scientific Inc., Logan, UT, United States) at each site that measured data every 30 s and recorded data averages every 30 min. The datalogger and sensors were powered by a solar panel and deep cycle batteries located at each site.
Water Use and Leaf Area Index Measurements
In order to continuously measure tree-level water use, laboratory-made, Granier-style, heat dissipation sapflow sensors (Granier, 1987) were installed in trees at the beginning of the second growing season (May, 2019). If trees were large enough in diameter, 2-cm-long sensors were installed and 1-cm-long sensors were installed in smaller diameter trees. Heat dissipation sensors consist of a pair of sensors installed radially into the sapwood about 10 cm vertically from one another and each containing an inner thermocouple. The upper sensor received constant heat and the lower sensor remained unheated. Water flow through the sapwood cools the heated sensor such that temperature differences between the sensor pair can estimate sap flow rates through an empirical equation (Granier, 1987). Sensor pairs were covered with reflective insulation to prevent sunflecks from affecting temperature measurements. At the Coastal Plain site, sensors were installed in one of the inner, middle trees of each 15-tree varietal plot being monitored for water use. Three trees per varietal per endophyte treatment (two for varietal 6329, endophyte inoculated) were monitored in plots located closest to the datalogger for a total of 35 trees monitored (due to space limitations in the datalogger). At the LMAV site, survival was low (see above), therefore two of the largest trees in each varietal/endophyte treatment group were selected for study for a total of 24 trees monitored at that site. The central CR1000 datalogger and AM16/32B multiplexer (Campbell Scientific Inc.) at each site measured sensor temperature differences every 30 s and averaged data every 30 min and sensors were powered by a solar panel and deep cycle batteries.
Half-hourly sensor temperature differences were converted to sapflow rates (JS; kg m–2 sapwood area s–1) using the software program BaseLiner version 4 (Oishi et al., 2016). Missing half-hourly data were gapfilled from corresponding sensors in other trees of the same varietal/endophyte treatment group for each site. Half-hourly data were integrated into daily and monthly water use and determined on a tree-level basis by multiplying sapflow rates by stem sapwood areas. Stem diameters were measured after sensor installation and again at the end of the second growing season during stem harvest (see below). During stem harvest, the proportion of radius occupied by bark or sapwood was determined and a relationship between whole stem diameter and sapwood diameter (Diamsapwood = Diamstem × 0.9 − 0.107; r2 = 0.89) across both sites was used to calculate sapwood areas from earlier stem diameter measurements. Linear growth in sapwood areas was assumed throughout the second growing season and between measurement periods and all sapwood area was assumed to be functional with no radial adjustments in sapflow rate applied to the data (Samuelson et al., 2007). Leaf-specific transpiration rates (EL; kg m–2 leaf area s–1) were then calculated by dividing these sapwood-area-scaled sapflow rates by tree leaf area. Leaf areas were determined during biomass harvest (see below) and varietal-specific linear relationships between tree diameter2 × height (D2H) and leaf area (r2 = 0.49 to 0.96) were developed and used to calculate leaf areas at the beginning of the growing season. For hybrid varietals that experience leaf drop during the growing season due to leaf diseases and senescence, relationships between D2H and leaf area for eastern cottonwood from biomass harvests were used to estimate leaf areas at the beginning of the growing season before significant leaf drop. A linear relationship between leaf area during the beginning and end of the growing season was assumed. Canopy-scaled stomatal conductance (canopy GS; m s–1) was calculated as follows:
where KG is a coefficient calculated as 115.8 + 0.4226 × air temperature (Phillips and Oren, 1998). Canopy stomatal conductance was further scaled to units of mol m–2 s–1 by dividing values by the density of water vapor scaled based on air temperature [0.0224 × air temp(K)/27].
At the peak of the second growing season in June, 2019 during a sunny, cloud-free day, leaf area index (LAI) was estimated at three locations within each 15-tree plot at the Coastal Plain site using a LAI 2000 canopy analysis system (LI-COR Biosciences Inc., Lincoln, NE, United States). Due to low plot-level survival, LAI was not measured at the LMAV site. Measurements were made in the first tree-row of each plot between trees 1 and 2 and trees 4 and 5 of that row with the sensor facing plot center. A 90-degree view cap was placed on the sensor to block the view of surrounding plots and the outermost rings were excluded from analysis. Open sky conditions were measured repeatedly after six plots were measured and open sky data were interpolated between measurements. LAI for each 15-tree plot was calculated using FV2200 software version 2.1.1 from LI-COR Biosciences Inc.
Biomass Harvest and Whole Tree Water Use Efficiency
At the end of the second growing season but before autumn leaf drop (October 2019), trees measured for sapflow at each site were harvested to measure their biomass. Groundline and diameter at breast height were measured and trees were cut near their base. Sapwood diameter was measured in two perpendicular directions on the cut stump to compare with groundline diameter and determine the bark component. Once cut, the stem lengths were measured to estimate tree height. All stems containing leaves were removed in the field and placed in plastic bags for transport back to the lab. An approximately 10 cm long sample was cut from the stem and its green weight was determined before transport back to the lab. The remaining stem weight was measured in the field with a hanging balance. Once back in the lab, the volume of the fresh stem sample was obtained using water volume displacement and it was placed in a drying oven at 105°C until all water was expelled. The dry weight of the sample was obtained and specific gravity was calculated as sample dry weight divided by fresh volume divided by the density of water. All leaves were removed from remaining stems in the lab and the weight of these remaining stems was added to the weight of stems measured in the field. This total stem weight was converted to a dry wood biomass (kg) using the stem sample fresh weight/dry weight ratio for each tree. The fresh weight of all leaves was measured, then a random subsample of leaves was obtained from each tree. The fresh weight of the leaf subsample was measured as well as its cross-sectional area using a LI3100C area meter (LI-COR Biosciences Inc.). The area to weight ratio of the leaf subsample was multiplied by the total fresh leaf weight of each tree to determine total tree leaf area.
To estimate whole-tree WUE, an equation was developed to predict dry woody biomass from stem basal area (BA) × tree height [Biomass = (BA × H) × 12.45 + 935.592; r2 = 0.87] measured during the tree harvest. This equation was used to calculate dry woody biomass of each tree during sapflow sensor installation from height and diameter measurements taken during that time. Biomass during sensor installation was subtracted from biomass measured during the tree harvest to calculate aboveground biomass added during the 2019 growing season. This aboveground biomass growth was divided by the water used during that time period and estimated by the sapflow data to calculate whole-tree WUE (whole-tree WUE; g biomass/kg water used).
Data Analysis
For all reported parameters except LAI, individual trees measured for sapflow and harvested for biomass represent the experimental unit. LAI measurements were averaged at the plot level for the Coastal Plain site. Overall analysis of variance to test for significant differences in measured parameters based on site, varietal, endophyte inoculation as fixed effects and their interaction was performed in R version (R Core Team, 2014) using linear mixed effect models [nlme package; Pinheiro et al. (2014)] with replicate block included as a random effect in models. In addition, to compare varietals with one another at each testing site, multiple comparison tests were accomplished for each site separately using Tukey contrasts in the multcomp package (Hothorn et al., 2008) in R.
Linear and non-linear relationships between canopy stomatal conductance (GS) and environmental parameters were fitted with Sigmaplot version 13 (Systat Software Inc., San Jose, CA, United States). Canopy GS was compared with the natural log of VPD (lnVPD) to produce a linear correlation between these parameters. Likewise, the natural log of canopy GS was calculated to generate a linear relationship with soil moisture at the LMAV site. Relationships between canopy GS and lnVPD or PPFD were estimated at both low soil moisture (low θ) and average to high soil moistures (high θ) by first splitting the datasets into low soil moisture conditions (θ < 0.2 at the Coastal Plain site and θ < 0.55 at the LMAV site), then averaging GS by VPD or PPFD categories. These soil moisture thresholds were determined by identifying inflection points in the canopy GS vs. soil moisture data. In order to determine if slope terms differed between varietals in linear regression relationships, linear mixed effect models were fitted using the lme package in R and multiple comparison tests of slope terms were analyzed using the phia package (De Rosario-Martinez, 2015) in R. To estimate stomatal sensitivity to VPD (Oren et al., 1999), parameters were estimated from the linear regression equations fitted to the canopy GS vs. lnVPD relationships. The y-intercept of the equation represents the reference canopy GS at VPD = 1 kPa (GS,ref), the slope of the relationship (m) represents the rate of change between canopy GS and lnVPD (GS sensitivity), and the ratio of the slope and y-intercept represents the rate of change between canopy GS and lnVPD per unit reference canopy GS (scaled GS sensitivity). Scaled GS sensitivity at low vs. high soil moisture were compared for each varietal using paired t-tests (with measurements within each soil category paired within individuals) using R.
Results
Biomass and Leaf Area Across Varietals and Sites
Two-year dry biomass was significantly greater at the Coastal Plain site compared with the LMAV site (p = 0.008; Table 1) averaging 6.7 ± 0.5 and 2.4 ± 0.4 kg per tree respectively across varietals. Varietals also differed significantly at each site with varietal 8019 (D × M) and the eastern cottonwoods having the largest biomass at the Coastal Plain site. Varietal 8019 (D × M) had significantly greater biomass than the other hybrid poplar varietals (Table 2). At the LMAV site, eastern cottonwoods again tended to have the greatest biomass with S7C8 (D × D) having the largest biomass and 6329 (D × M) having the smallest biomass. In contrast, specific gravity was significantly greater at the LMAV site (p < 0.001; Table 1) compared with the Coastal Plain site across varietals (0.44 ± 0.006 vs. 0.33 ± 0.006 g cm–3, respectively). At the Coastal Plain site, S7C8 (D × D) and 6329 (D × M) had the highest specific gravity and 110412 (D × D) exhibited the lowest specific gravity (Table 2). At the LMAV site, S7C8 (D × D) again had the largest specific gravity and ST66 (D × D) had the lowest specific gravity. At the Coastal Plain site, for plot-level LAIs measured in July, 2019, D × M hybrids tended to exhibit the highest leaf areas with statistically larger LAI than 5077 (T × D) and eastern cottonwoods had intermediate LAI values (Figure 1A). In October at the Coastal Plain site, eastern cottonwoods S7C8 (D × D) and ST66 (D × D) tended to have the greatest leaf areas estimated from tree harvest with significantly more leaf area than hybrid poplars 8019 (D × M) and 5077 (T × D; Figure 1B).
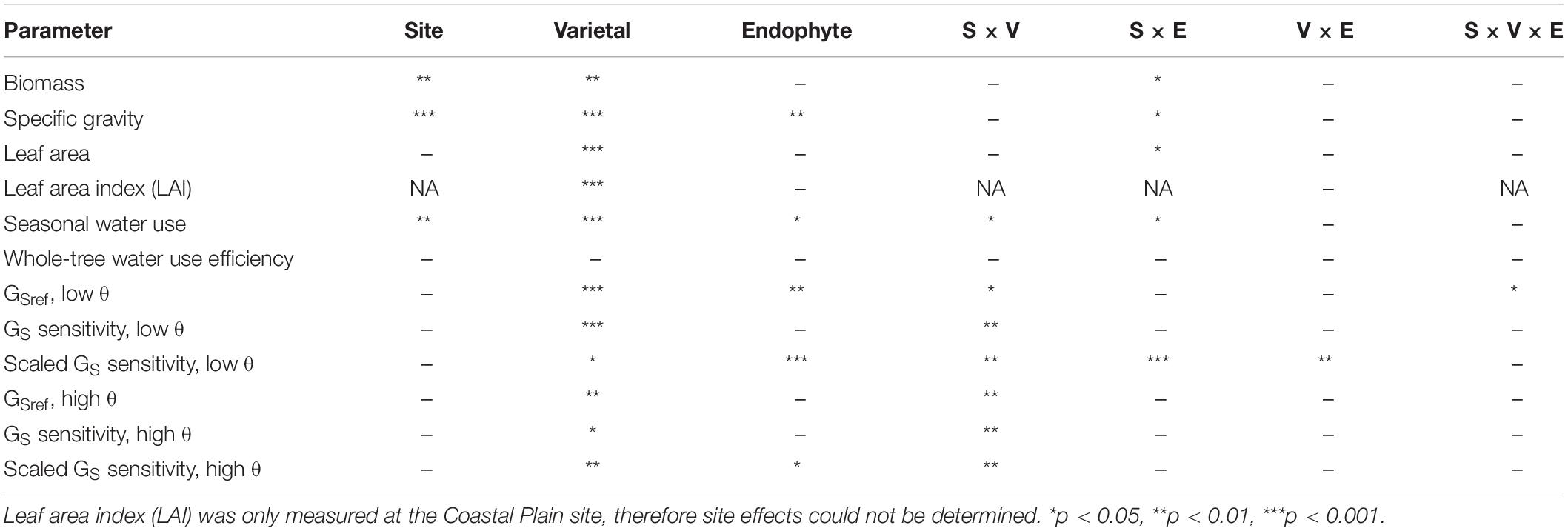
Table 1. Analysis of variance results presenting significant effects of site (S), varietal (V) and endophyte inoculation (E), as well as their interaction (×) on productivity, water use, and canopy stomatal conductance (GS) parameters under low and average to high soil moisture (θ).
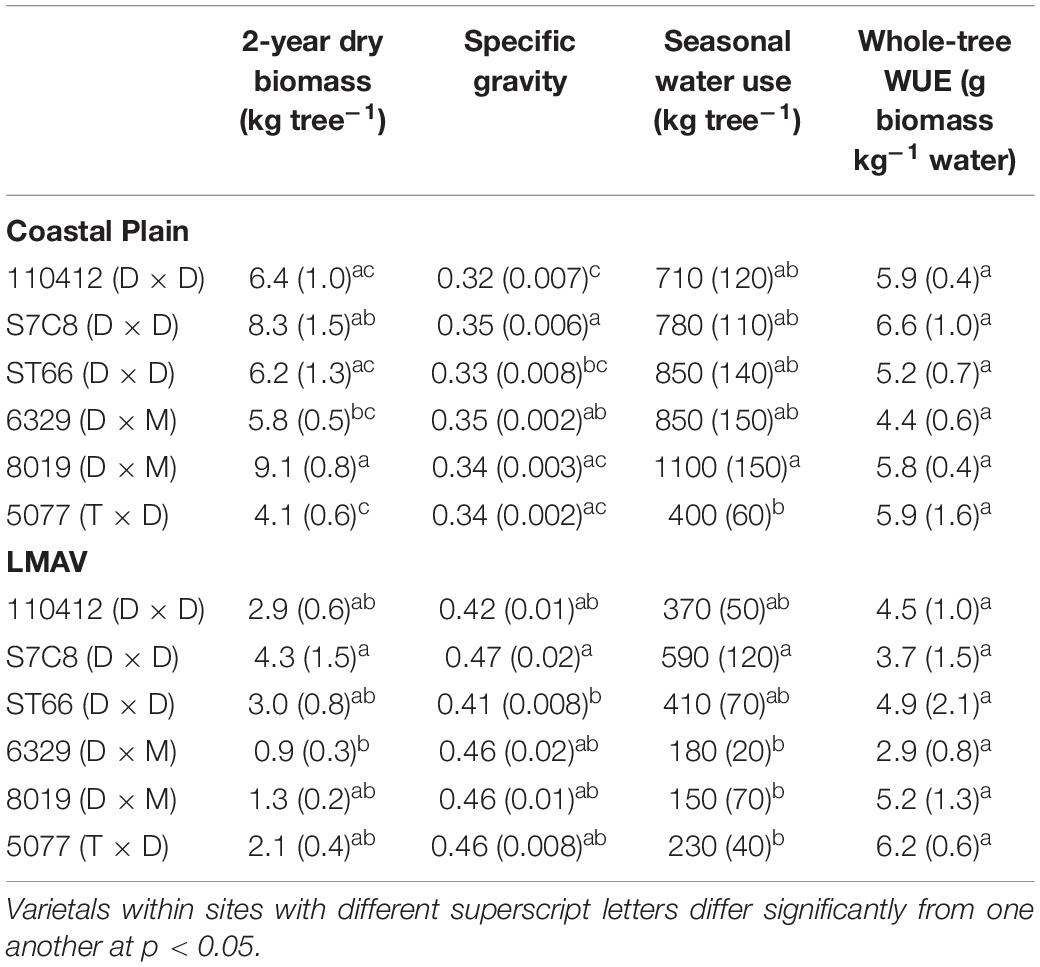
Table 2. Productivity, water use, and water use efficiency (WUE) parameters (mean and SE in parentheses) for varietals growing on the Coastal Plain and LMAV sites.
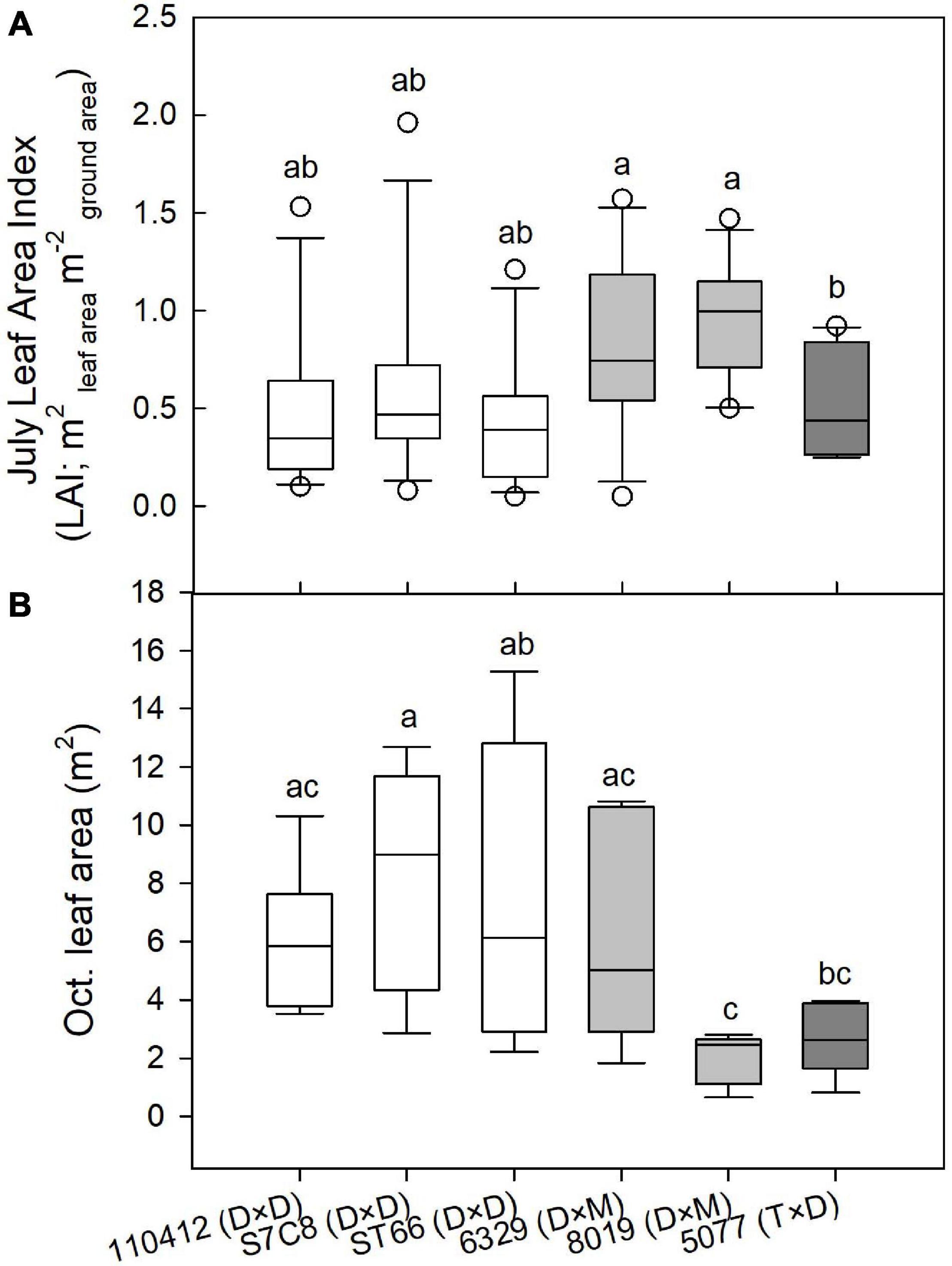
Figure 1. (A) Plot-level July leaf area index (LAI; m2 m–2) at the Coastal Plain site and (B) individual tree leaf area (m2) estimated from tree harvests in October at the Coastal Plain site for P. deltoides × P. deltoides taxa (D × D; white), P. deltoides × P. maximowiczii taxa (D × M; light gray), and P. trichocarpa × P. deltoides taxa (T × D; dark gray). Varietals with different letters in each panel differ significantly at p < 0.05.
Water Use and Canopy GS vs. Soil Moisture and PPFD
Water use over the second growing season was significantly greater at the Coastal Plain site compared with the LMAV site (p = 0.007; Table 1) corresponding to the size differences of the trees at each site. Within the Coastal Plain site, 8019 (D × M) used the most water and differed significantly from 5077 (T × D) which used the least water (Table 2). Throughout the growing season, 8019 (D × M) used more water during the early portion of the growing season with water use declining after June (Supplementary Figure 1). Other varietals, including S7C8 (D × D), ST66 (D × D), and 6329 (D × M) increased water use during the growing season using the most water in August or used similar amounts of water for most months of the growing season (Supplementary Figure 1). At the LMAV site, S7C8 (D × D) used the most water differing significantly from all hybrid poplars which exhibited low water use (Table 1). In terms of monthly water use at the LMAV site, S7C8 (D × D) and 5077 (T × D) used the most water in the June and July, while other varietals either maintained or increased water use throughout the growing season (Supplementary Figure 1). Whole-tree WUE did not differ significantly between sites (p = 0.34) or varietals (p = 0.51; Table 1) and averaged 5.2 ± 0.3 g biomass per kg water used.
Canopy-scaled stomatal conductance (canopy GS) exhibited differing relationships with soil moisture depending on site (Figure 2A). At the Coastal Plain site, cubic functions best fit the data, with canopy GS increasing to the greatest extent with increasing soil moisture under the drier soil moisture range (0.14–0.2 cm3 cm–3). Under drier soil moistures, 8019 (D × M) exhibited the greatest increase in canopy GS, while 6329 (D × M) exhibited the lowest increase. When soil moistures were above 0.2 cm3 cm–3, canopy GS remained relatively consistent, with slight decreases seen in all varietals around 0.24 cm3 cm–3 (Figure 2A). At the LMAV site, canopy GS increased exponentially with increasing soil moistures (Figure 2B). The natural log of canopy GS resulted in a linear relationship, and the slope of the relationship between canopy GS and soil moisture was significantly larger for all hybrid poplars compared with all eastern cottonwoods (Figure 2B).
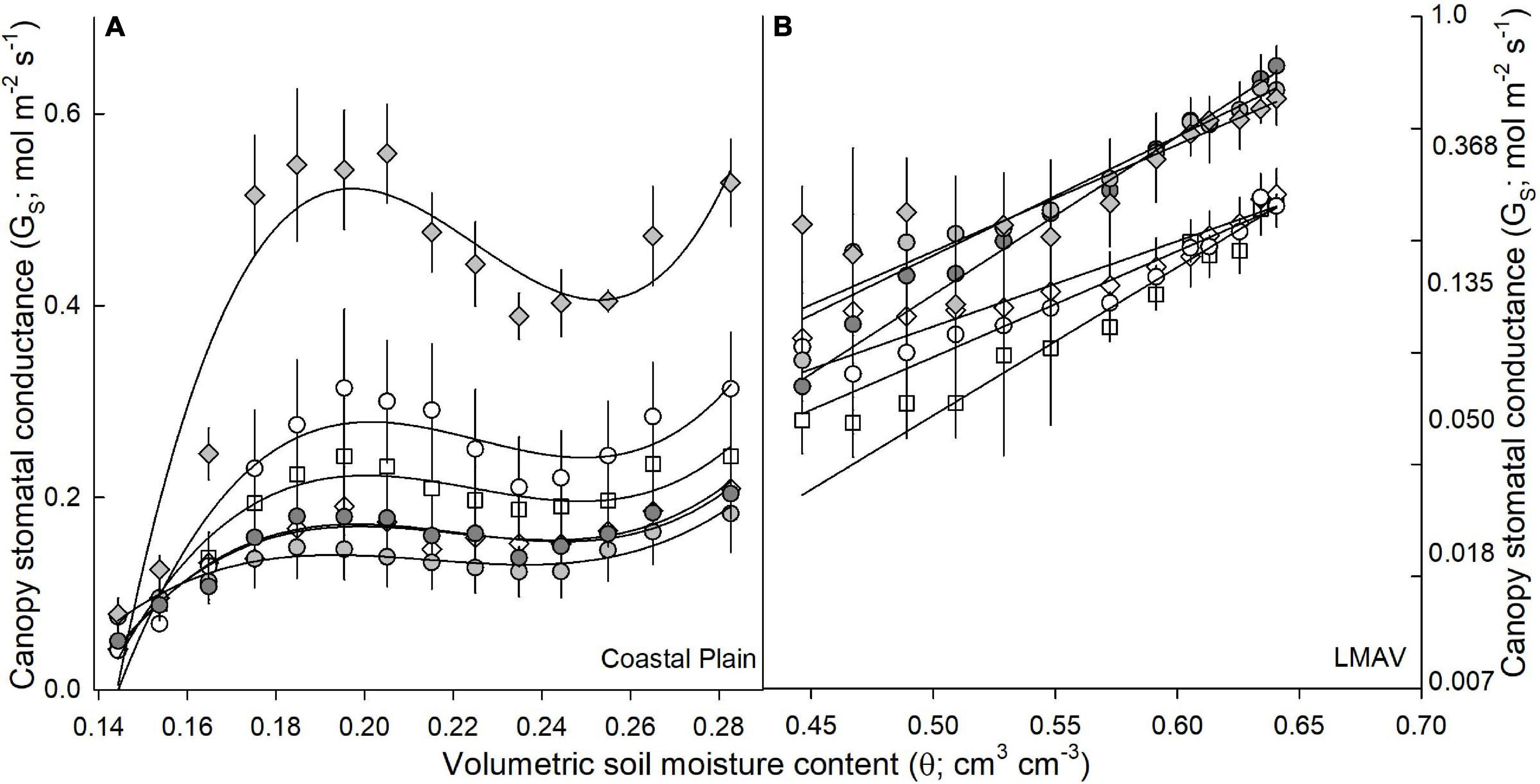
Figure 2. Canopy stomatal conductance (GS; mol m–2 s–1; mean and SE) vs. volumetric soil moisture content (θ; cm3 cm–3) for eastern cottonwoods (D × D) including varietals 110412 (white circles), S7C8 (white diamonds), and ST66 (white squares) and hybrid poplars in the P. deltoides × P. maximowiczii taxa (D × M) including 6329 (light gray circles) and 8019 (light gray diamonds) and the P. trichocarpa × P. deltoides taxa (T × D) including varietal 5077 (dark gray circles) at the (A) Coastal Plain and (B) LMAV sites.
At both sites, canopy GS exhibited nonlinear relationships with PPFD under low and high soil moisture conditions, reaching a maximum at low to mid PPFD values then decreasing again at the highest light levels (Supplementary Figure 2). At the Coastal Plain site under low soil moistures, all varietals reached maximum canopy GS at PPFD around 825 μmol m–2 s–1. Under high soil moisture, canopy GS reached a maximum at a lower light level of around 550 μmol m–2 s–1 in all varietals (Supplementary Figure 2). At the LMAV site, canopy GS peaked at lower PPFD values under low soil moisture compared with higher soil moisture values (Supplementary Figure 2). Under low soil moisture, canopy GS in 8019 (D × M) and S7C8 (D × D) peaked around 400 μmol m–2 s–1 while all other varietals peaked around 550 μmol m–2 s–1. At high soil moistures, canopy GS of all varietals reached a maximum at PPFD values around 750 μmol m–2 s–1 (Supplementary Figure 2).
Stomatal Sensitivity to Vapor Pressure Deficit
In relationships between canopy GS and lnVPD at low and average to high soil moistures, sites exhibited similar GS,ref, GS sensitivity and scaled GS sensitivity, but varietals, as well as their ranking within sites (S × V), differed significantly (Table 1). At the Coastal Plain site (Figures 3A,B), both GS,ref (y intercept of lines) and GS sensitivity (slope of lines) exhibited the same ranking among varietals at low (Figure 3A) and high (Figure 3B) soil moistures (Table 3). Varietal 8019 (D × M) exhibited the largest GS,ref and GS sensitivity, 110412 (D × D) and ST66 (D × D) were intermediate and S7C8 (D × D), 6329 (D × M), and 5077 (T × D) had the lowest GS,ref and GS sensitivity regardless of soil moisture (Table 3). However, scaled GS sensitivity (slope/y intercept) differed based on soil moisture regime with all varietals being statistically similar at low soil moisture and scaled GS sensitivity averaging 0.71 ± 0.008 (Table 3). At average to high soil moistures, S7C8 (D × D) and 6329 (D × M) exhibited the highest scaled GS sensitivity and differed significantly from 110412 (D × D) and 8019 (D × M) which exhibited lower scaled GS sensitivity. Across soil moisture categories, all varietals at the Coastal Plain site exhibited significantly lower scaled GS sensitivity at high soil moistures compared with low soil moisture conditions except varietal 6329 (D × M; Table 3).
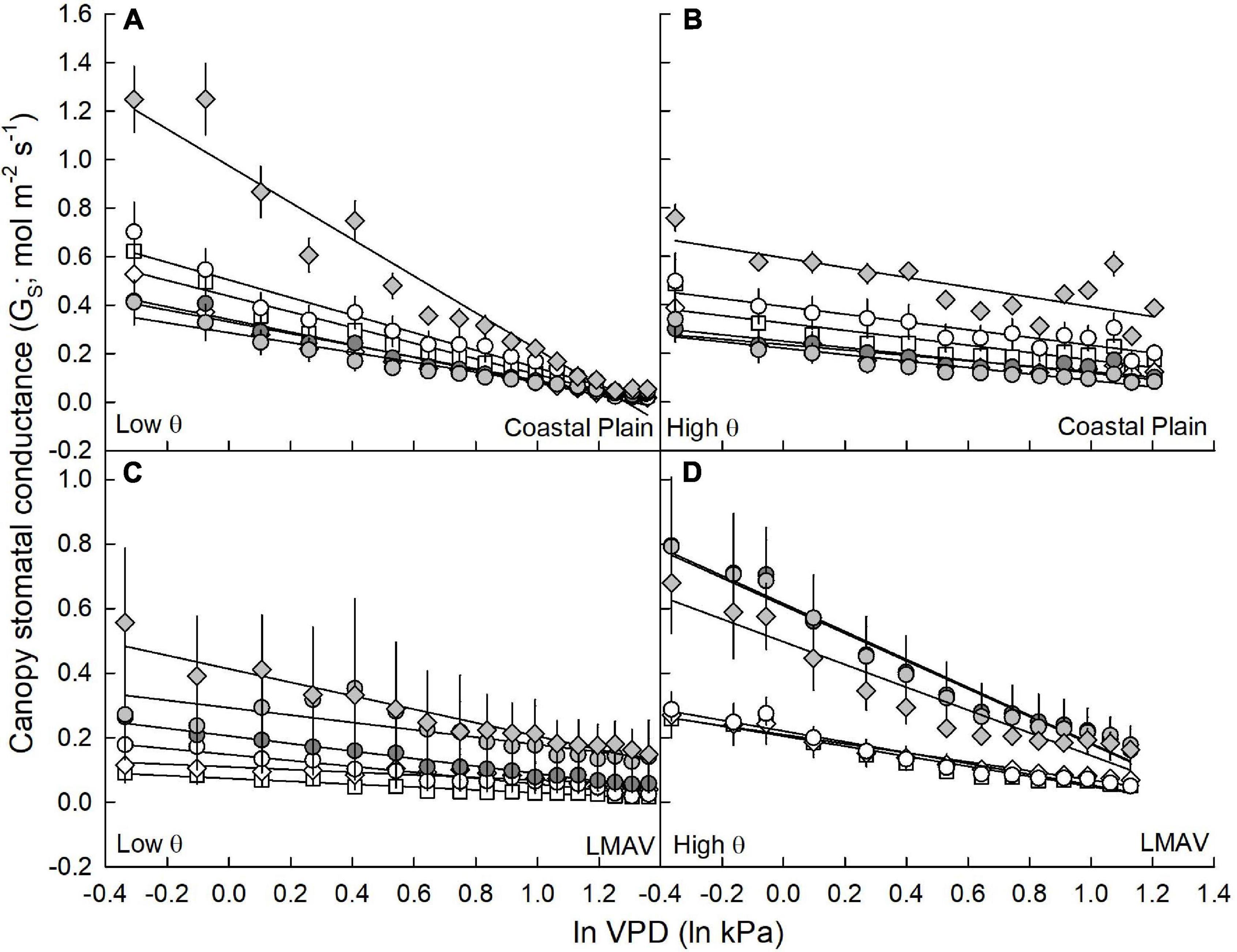
Figure 3. Canopy stomatal conductance (GS; mol m–2 s–1; mean and SE) vs. the natural log of vapor pressure deficit (ln VPD; ln kPa) under (A,C) low soil moisture (θ; cm3 cm–3) conditions and (B,D) average to high soil moisture conditions. Eastern cottonwoods (D × D) including varietals 110412 (white circles), S7C8 (white diamonds) and ST66 (white squares) and hybrid poplars in the P. deltoides × P. maximowiczii taxa (D × M) including 6329 (light gray circles) and 8019 (light gray diamonds) and the P. trichocarpa × P. deltoides taxa (T × D) including varietal 5077 (dark gray circles) for trees at the (A,B) Coastal Plain and (C,D) LMAV sites are shown.
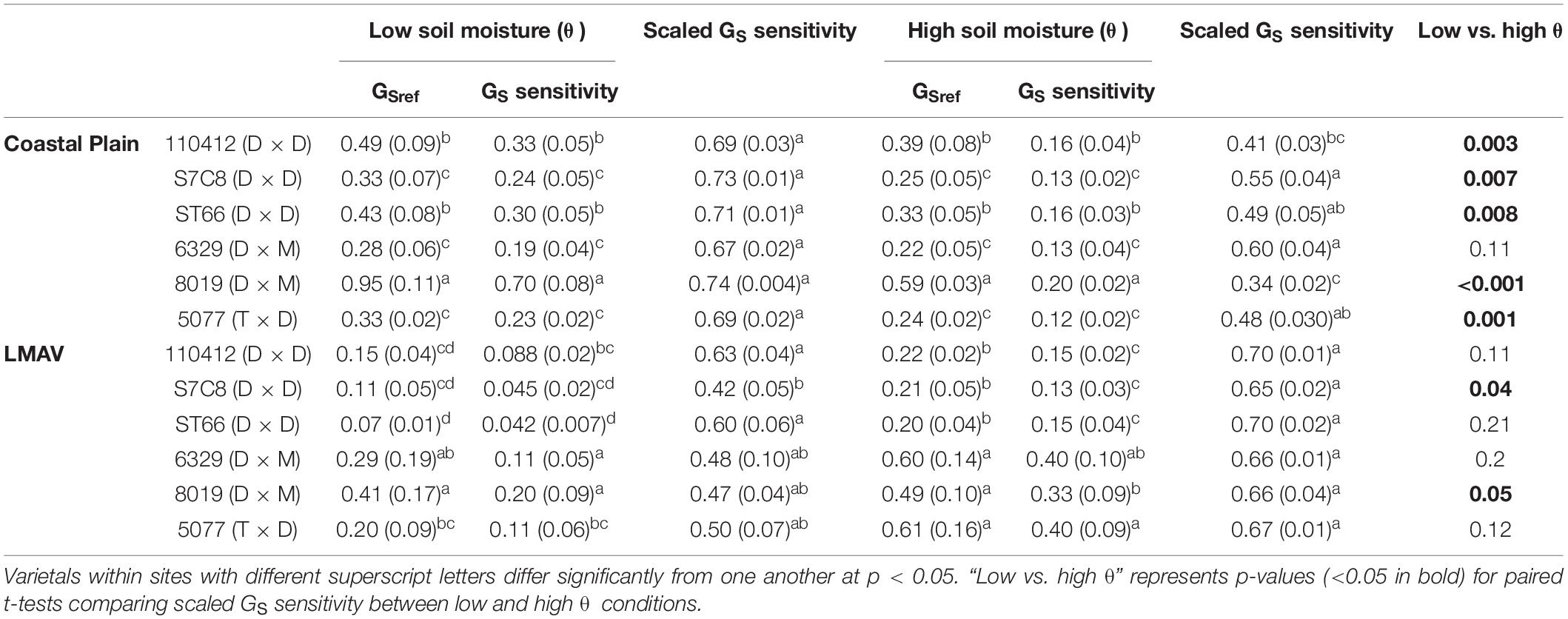
Table 3. Parameters for varietals growing in the Coastal Plain and LMAV sites estimated from linear relationships between canopy stomatal conductance (GS) and the natural log of vapor pressure deficit (lnVPD; Figure 3) estimated under low vs. average to high soil moisture (θ; cm3 cm–3) conditions including GSref (mol m–2 s–1; y-intercept; or GS when VPD = 1 kPa), GS sensitivity (mol m–2 s–1 ln kPa–1; slope of line) and scaled GS sensitivity (slope/y-intercept).
At the LMAV site (Figures 3C,D), varietals differed in their rankings of GS,ref and GS sensitivity and these rankings differed depending on if parameters were estimated at low (Figure 3C) or average to high (Figure 3D) soil moisture (Table 3). At low soil moistures (Figure 3C), D × M varietals had the highest GS,ref, 5077 (T × D) was intermediate and the eastern cottonwoods tended to have the lowest GS,ref (Table 3). The D × M varietals also exhibited the highest GS sensitivity, 5077 (T × D) and 110412 (D × D) were intermediate and S7C8 (D × D) and ST66 (D × D) had the lowest GS sensitivity at low soil moistures. At low soil moistures, scaled GS sensitivity was similar among varietals, although S7C8 (D × D) had significantly lower scaled GS sensitivity compared with the other eastern cottonwood varietals. Under average to high soil moistures at the LMAV site (Figure 3D), all hybrid poplars had significantly greater GS,ref and GS sensitivity than eastern cottonwoods with varietal 5077 (T × D) having significantly greater GS sensitivity than varietal 8019 (D × M; Table 3). However, all varietals exhibited statistically similar scaled GS sensitivity at high soil moistures at the LMAV site. Likewise, most varietals exhibited statistically similar scaled GS sensitivity at low and high soil moistures at the LMAV site with only S7C8 (D × D) and 8019 (D × M) exhibiting significantly higher sensitivity at high soil moistures compared with low soil moisture conditions (Table 3).
Endophyte Effects
While year two biomass at the Coastal Plain site was statistically similar (p = 0.19) between trees receiving the endophyte treatment and control trees, at the LMAV site, endophyte-treated trees differed significantly (p = 0.04) from control trees having about 70% more biomass across all varietals (Figure 4A). Endophyte inoculated trees at the LMAV site also used significantly more water than non-inoculated trees given their larger size and biomass, however, whole-tree WUE was marginally, significantly greater (p = 0.057) for trees receiving the endophyte treatment compared with control trees at the LMAV site. Under low soil moisture conditions, scaled GS sensitivity was marginally, significantly greater in endophyte-treated vs. control trees at the Coastal Plain site (p = 0.056) and significantly greater at the LMAV site (p < 0.001; Figure 4B). At the Coastal Plain site, endophyte inoculated trees had about 4% higher scaled GS sensitivity compared with control trees, whereas at the LMAV site, the endophyte treatment resulted in about 35% higher scaled stomatal sensitivity compared with control trees.
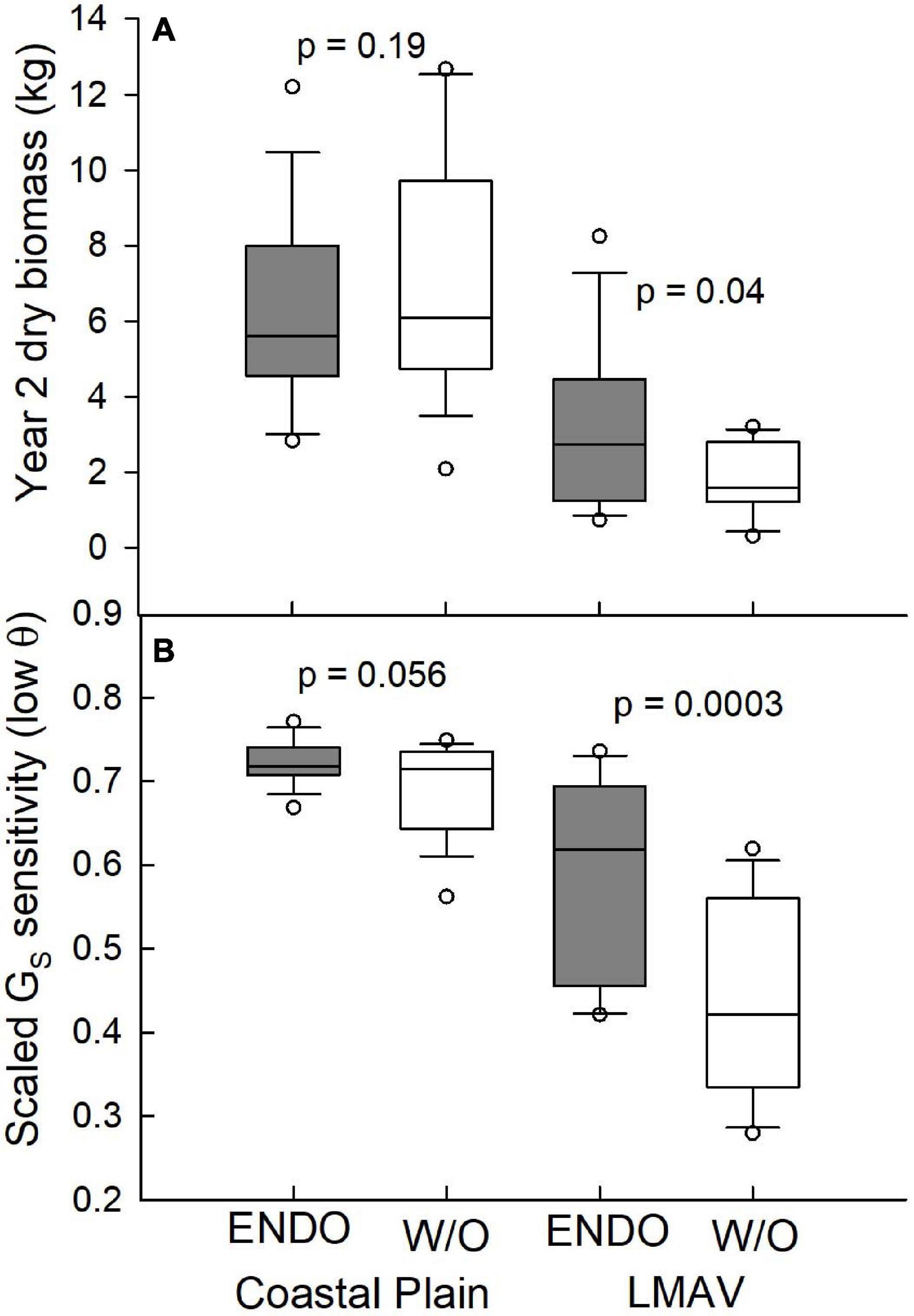
Figure 4. (A) Year two dry biomass (kg), and (B) scaled canopy stomatal conductance (GS) sensitivity at low soil moisture (θ; cm3 cm–3; bottom panel) for the Coastal Plain (left) and LMAV (right) field sites across all varietals inoculated with endophytes (ENDO) and those without the endophyte inoculation (W/O). p-Values for endophyte ANOVA comparisons are shown above graphs.
Physiological Parameters Predicting Growth
Across varietals and sites, tree leaf area was significantly (r2 = 0.29; p < 0.0001), positively correlated with aboveground woody biomass (Figure 5A). Whole-tree WUE also exhibited a statistically significant, positive correlation (r2 = 0.23; p = 0.0002) with biomass across all sites and varietals (Figure 5B). For relationships between biomass and scaled GS sensitivity, results differed depending on soil moisture conditions. Under low soil moisture conditions, trees that exhibited greater scaled GS sensitivity had greater biomass (r2 = 0.49; p < 0.0001 for the relationship between the log of biomass and scaled GS sensitivity) with this relationship driven largely by trees at the LMAV site (Figure 5C). Under average to high soil moisture conditions, trees with lower scaled GS sensitivity had higher biomass (r2 = 0.33; p < 0.0001 for the relationship between the log of biomass and scaled GS sensitivity) and this relationship was largely driven by trees at the Coastal Plain site (Figure 5D).
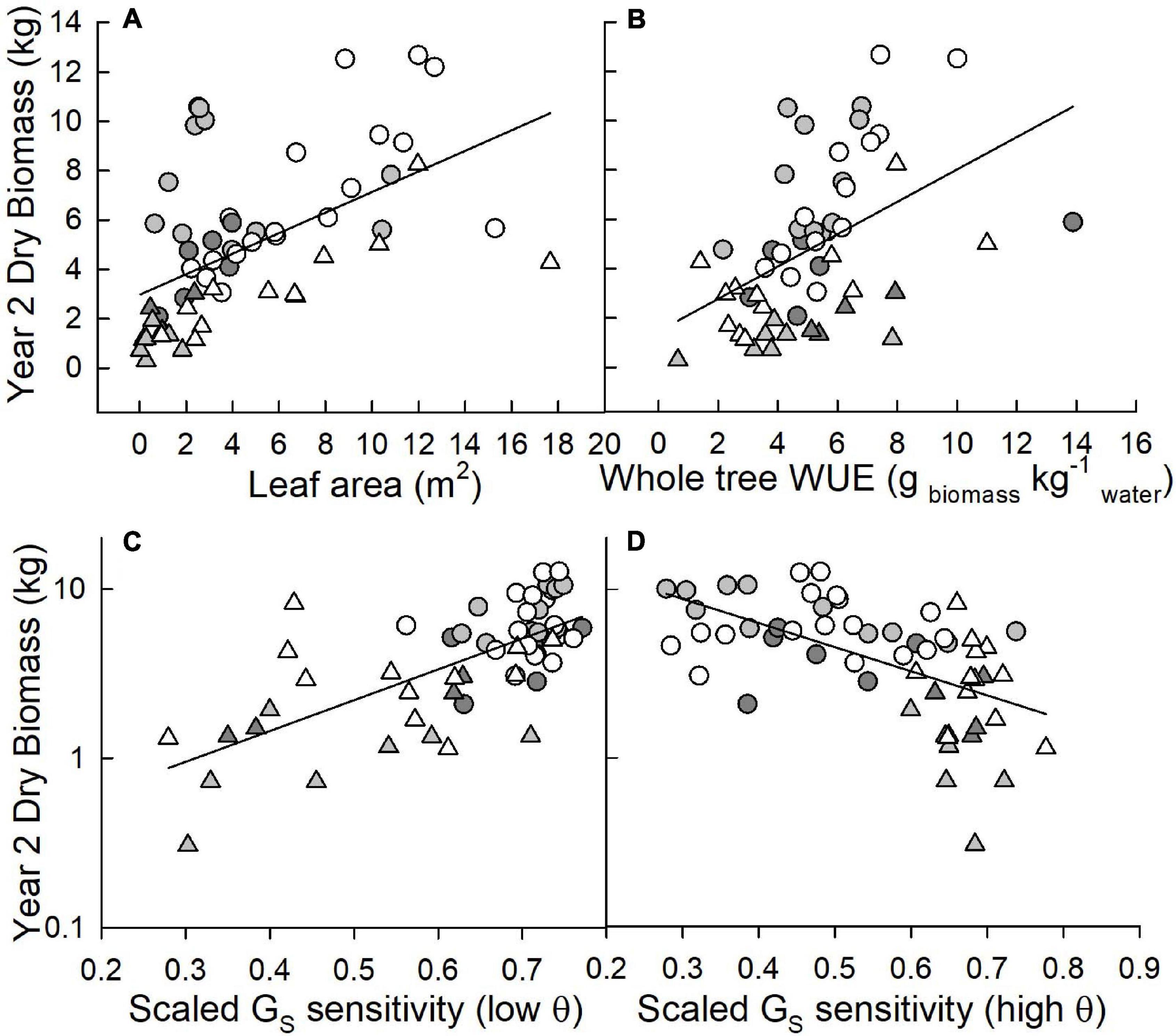
Figure 5. Correlations between dry biomass measured after two growing seasons (kg; note the log scale on the lower graphs) and (A) leaf area measured at harvest (m2), (B) whole-tree water use efficiency (g biomass kg–1 water) and scaled canopy stomatal conductance (GS) sensitivity at (C) low and (D) average to high soil moistures (θ; cm3 cm–3). The Coastal Plain site is presented as circles, the LMAV site as triangles. Eastern cottonwoods (D × D) are white symbols, D × M taxa are light gray, T × D taxa are dark gray.
Discussion
Water Use Strategies – Whole Tree Water Use and Efficiency
For the measured Populus varietals at two sites, we found that water use mirrored tree sizes in terms of biomass with larger varietals [8019 (D × M)] at the better quality, Coastal Plain site using the most water at around 1200 kg water per tree during the measurement period (May–September) or about 450 mm of water over the growing season (April–October) based on this tree spacing. Smaller trees at the more marginal LMAV site ranged in water use from 170 to 730 kg water used per tree from May to September with eastern cottonwoods using more water than hybrids. The lowest water user and smallest trees at the Coastal Plain site, varietal 5077 (T × D) used about 400 kg water per tree during the measurement period or about 150 mm of water over the growing season. This differs from findings of Bassman and Zwier (1991) who found that T × D hybrids had higher transpiration rates than P. deltoides varietals. However, Zalesny et al. (2019) found that varietals from an F1 backcross population [(T × D) × P. deltoides] tended to use less water than eastern cottonwood varietals. Eastern cottonwoods at the Coastal Plain site were intermediate and used between 600 and 800 kg water per tree during the measurement period or 320–360 mm of water over the growing season. During the same time period (April–October), the Coastal Plain site received about 980 mm of rainfall in 2019 and generally receives about 740 mm of rainfall based on a 30-year average (Arguez et al., 2010). Therefore, if single varietals were planted on a hectare scale at the spacing of the present study, transpiration from these plantations would account for 20% [for 5077 (T × D)] to 61% [for 8019 (D × M)] of rainfall during the growing season based on the 30-year average rainfall amount. This differs from other studies which found that Populus evapotranspiration exceeded precipitation for the same time period in a hybrid poplar plantation in Canada (Jassal et al., 2013), a D × N plantation (Clone I-214) in northern Italy (Migliavacca et al., 2009), and D × N varietals growing near Beijing, China (Xu et al., 2018). Of course, these studies estimated total evapotranspiration which includes interception losses as well as soil evaporation in addition to transpiration. Song et al. (2021) found that transpiration in Populus simonii × P. nigra varietals growing in China was about 45% of total precipitation over the same time period which is similar to our transpiration/rainfall proportion. In addition, water use may increase as trees get larger, are planted on a closer spacing or after coppice, therefore these data represent water use for young, pre-coppice stands.
Daily water use at the Coastal Plain site averaged about 0.9 (5077 T × D) to 2.3 (8019 D × M) mm day–1 across varietals throughout the growing season which is similar to reported average daily water use of 1.5 mm day–1 for P. simonii × P. nigra growing in China (Song et al., 2021), 1.8 mm day–1 for varietal I214 (D × N) in southwestern France (Muller and Lambs, 2009), 2.2 mm day–1 for P. maximowiczii × P. nigra varietals in Germany (Petzold et al., 2011), and 2.3–3.1 mm day–1 for hybrid poplars growing in Germany (Schmidt-Walter et al., 2014). However, this water use is lower than the 3.6 mm day–1 for a T × D hybrid poplar growing in Washington State (Hinckley et al., 1994) and 3.4 mm day–1 for an irrigated T × D varietal growing on the east side of the Cascade Mountains in Washington (Kim et al., 2008). Overall, these higher daily water usage values compared to our study may be due to the drier atmospheric growing conditions (i.e., higher VPDs) at these sites during the growing season coupled with the availability of soil moisture or irrigation conditions which allow trees to use higher amounts of water.
Overall, both sites and all varietals in our study exhibited a statistically similar whole-tree WUE of around 5.2 g biomass per kg water used during the second growing season. This suggests that these varietals exhibited similar strategies in terms of whole tree WUE and further highlights the positive relationship between WUE and biomass. Likewise, since these tested varietals were chosen for their high productivity in previous studies, this suggests that our WUE represent the upper range for high performing Populus grown in natural environmental conditions. For example, our calculated whole tree WUE is higher than found by Kim et al. (2008) who calculated WUE of about 2.3 g C per kg water used (or about 4.6 g biomass per kg water assuming 50% C content) in 3-year-old irrigated T × D trees, as well as Souch and Stephens (1998) for 1 to 2-year old T × D and D × N hybrids grown in a greenhouse which exhibited WUE of 4.4 g biomass kg–1 and 3.5 g biomass kg–1, respectively. The lower reported WUE of trees in these studies is likely the result of supplemental watering in either greenhouse or irrigated conditions. Additionally, our measured WUE is about double what was found by Maier et al. (2019) for eastern cottonwood and hybrid poplars across a range of growth performance in North Carolina, United States. Because Maier et al. (2019) included varietals across a range of growth rates, their reported WUE is likely lower than in the varietals tested in our study which were selected in part due to their higher growth rates.
Water Use Strategies – Stomatal Sensitivity
Although whole tree WUE was statistically similar across varietals, scaled stomatal sensitivity differed between sites, across varietals and between soil moisture levels (low soil moisture vs. average to high soil moisture) suggesting that differing strategies under different environmental conditions and in different varietals can lead to similarly high WUE. At the more well drained, Coastal Plain site, varietals converged at a high scaled GS sensitivity of around 0.7 under low soil moistures suggesting similar moisture stress among varietals during these low soil moisture conditions as well as a similar ability to increase GS sensitivity at low soil moistures. At average to high soil moistures, scaled GS sensitivity decreased in every varietal except 6329 (D × M) with 8019 (D × M) and 110412 (D × D) exhibiting the lowest scaled GS sensitivity around 0.38 at high soil moistures signifying a high degree of plasticity in GS sensitivity in these varietals depending on soil moisture conditions. Therefore, varietals 8019 (D × M) and 110412 (D × D) which exhibited the greatest plasticity in scaled GS sensitivity may be the most adaptable to a range of growing conditions and able to best match their stomatal functioning to soil moisture conditions. Song et al. (2021) measured a similar plasticity in GS sensitivity among years in a P. simonii × P. nigra varietal in Northeast China which decreased stomatal regulation from 1 year to another. Schmidt-Walter et al. (2014) found that scaled GS sensitivity changed by month during the growing season, generally becoming more sensitive in the later months of the growing season. In this study, varietals S7C8 (D × D), ST66 (D × D), and 5077 (T × D) exhibited intermediate GS sensitivity of around 0.51 at high soil moistures suggesting some degree of stomatal adjustment based on soil moisture conditions. Varietal 6329 (D × M) exhibited the highest sensitivity at ample soil moisture conditions, but also the lowest (although not statistically different) stomatal sensitivity at low soil moisture conditions suggesting relatively isohydric water use and potentially the most conservative water use strategy.
At the LMAV site with more poorly drained, clay soils, scaled GS sensitivity converged across varietals under high soil moistures at around 0.67. This relatively high GS sensitivity suggests stress under high soil moisture conditions potentially due to anaerobic conditions in the soil at high moisture levels. Under low soil moistures, scaled GS sensitivity tended to be slightly lower in all varietals, but only differed significantly in S7C8 (D × D) and 8019 (D × M). Migliavacca et al. (2009) shared similar results for a D × N varietal in northern Italy where trees exhibited less GS sensitivity under low soil moisture conditions. S7C8 (D × D) had higher biomass at the LMAV site suggesting that its lower stomatal regulation at lower soil moistures allowed it to take in more CO2 and exhibit greater productivity. On the other hand, 8019 (D × M) had relatively low productivity at the LMAV but also exhibited high plasticity at the Coastal Plain site suggesting high stomatal plasticity as a trait for varietal 8019 across sites. Overall, scaled GS sensitivity averaged 0.59 across all study individuals which is very close to the estimated sensitivity of 0.6 across a variety of species and sites (Oren et al., 1999) and similar to findings of Kim et al. (2008) who reported a GS sensitivity of 0.64 for an irrigated T × D plantation in eastern Washington, Maier et al. (2019) who reported stomatal sensitivities of around 0.63 for T × D, D × D, and D × M varietals in North Carolina, United States, but higher than Xu et al. (2018) who reported GS sensitivities of 0.36–0.48 for D × N hybrids growing near Beijing, China.
At the two field sites, we found that canopy GS responded differently to increases in soil moisture with canopy GS mainly increasing at low soil moistures (below 20%) at the Coastal Plain site, but increasing exponentially with increasing soil moisture at the LMAV site. This is likely related to the differing soil types and soil moisture release curves at each site with the Coastal Plain site occurring on silty loam soils and the LMAV site occurring on silty clay loam soils (Roy et al., 2018). These relationships differ from those identified by Song et al. (2021) who found linear relationships between canopy GS and soil moisture for poplars growing on sandy soils although soil moisture explained a relatively small proportion of canopy GS compared with VPD and PPFD in that study. However, Maier et al. (2019) found similar relationships between canopy GS and relative extractable soil water as our Coastal Plain site for their site located on sandy loam soils with canopy GS increasing until relative extractable water reached about 20% then remaining relatively constant at higher extractable water contents. We also found that soil moisture affected the relationships between canopy GS and PPFD or VPD. Especially at the Coastal Plain site, relationships between canopy GS and PPFD had higher r2 values at high soil moistures while relationships between canopy GS and VPD had higher r2 values at lower soil moisture suggesting that VPD is a stronger driver of canopy GS at low soil moisture while PPFD is a stronger driver at high soil moistures. We also found that canopy GS peaked at lower PPFD levels at high soil moistures compared with lower soil moistures at the Coastal Plain site whereas the opposite was true at the LMAV site. We expected that, at lower soil moistures, canopy GS would peak at lower PPFD values, however, the opposite findings at the Coastal Plain site could suggest other interacting factors between environmental parameters are causing lower peak PPFD values at high soil moistures (Xu et al., 2017). Overall, these findings can aid in modeling of water use in these Populus varietals under the interacting environmental drivers of soil moisture, VPD and incoming radiation.
Interactions Between Water Use and Leaf Retention
We found that varietals also differed in their water use patterns throughout the growing season, which corresponds to leaf area differences measured in the peak of the growing season (July) vs. the late growing season (October). Varietal 8019 (D × M) at the Coastal Plain site exhibited peak water use in June with water use declining every month thereafter. This varietal had among the highest leaf areas in July but the lowest leaf areas in October, suggesting its declining water use was due to successive leaf losses throughout the growing season, likely due to Septoria leaf spot. In contrast, at the Coastal Plain site, eastern cottonwoods ST66 and S7C8 increased water use throughout the growing season exhibiting peak water use in August. All eastern cottonwood varietals also tended to have lower crown leaf areas based on LAI estimates than the hybrid poplars in July, but among the highest crown leaf areas (based on tree harvest) in October suggesting increased water use was due to increasing leaf areas throughout the growing season. It should be noted that, because eastern cottonwoods tend to hold their leaves more vertically while hybrid poplars hold their leaves more horizontally, LAI measurements based on understory light levels (i.e., from the LAI 2000) may have underestimated leaf areas for eastern cottonwoods compared with hybrid poplar. However, overall, similar results were found by Ceulemans et al. (1992) who found that hybrid poplars had the most leaves early in the growing season, while eastern cottonwoods had more leaves in the middle of the growing season. Leaf losses from hybrid poplars may relate to both premature leaf drop as a result of water stress (Souch and Stephens, 1998; Giovannelli et al., 2007) and effects of leaf pathogens which may cause leaf drop (Pellis et al., 2004; Coyle et al., 2006; Elena et al., 2014). In terms of resistance to leaf pathogens, tested eastern cottonwoods in this study are native to the region (110412 and ST66) or from Texas (S7C8) meaning they may exhibit relatively more resistance to native pathogens (Land and Jeffreys, 2006) particularly Septoria and Marsonnina leaf spot compared with hybrid poplars. However, eastern cottonwoods have still been shown to be vulnerable to Melampsora medusae foliar rust. Hybrid poplar 6329 (D × M) exhibited the lowest relative differences in crown leaf area among varietals between July and October suggesting higher leaf-level resistance to leaf pathogens than other hybrid poplar taxa.
Endophyte Inoculation Impacts
We found that the endophyte inoculation increased biomass across varietals at the LMAV site but not at the Coastal Plain site. Other results demonstrate that nitrogen availability limited growth at the LMAV site, while water use limited growth at the Coastal Plain site (Stewart, 2020). We also found greater scaled GS sensitivity at low soil moistures leading to marginally higher WUE in addition to the greater biomass of inoculated trees at the LMAV site. Khan et al. (2016) found that stomatal conductance was lower during drought conditions in endophyte inoculated D × N individuals compared with control plants, but inoculated plants maintained higher growth signifying higher WUE. Endophyte inoculated Arizona fescue (Festuca arizonica Vasey) also exhibited significantly less negative leaf water potentials, lower stomatal conductances and transpiration rates than non-inoculated plants which conferred increased drought tolerance of inoculated individuals (Morse et al., 2002). Khan et al. (2016) also found that endophytes produced several plant hormones including indole-3-acetic acid, jasmonic acid, gibberellin-3-acid and abscisic acid which have been shown to be involved in plant stress tolerance as well as growth, specifically for roots, which may confer additional tolerance to drought stress conditions. Overall, these findings suggest that endophytes can alter the drought tolerance of Populus varietals by improving the GS sensitivity of trees under soil moisture stress conditions.
Biomass and Physiological Parameters That Predict Growth
Two-year biomass was almost three times higher at the Coastal Plain site compared with the LMAV site. Other studies have reported good growth of eastern cottonwood in the LMAV (Stanturf et al., 2019), however, our site may have been more marginal in terms of soil inundation and potential nutrient limitations. Likewise, the biomass average across all varietals in the LMAV site is decreased by the low biomass of the hybrid poplars at the LMAV site. Hybrid poplars are generally not planted in the LMAV due to susceptibility to Septoria stem canker (Robison et al., 2006), however, the planted varietals displayed some Septoria resistance. The Coastal Plain site exhibited high biomass growth for the eastern cottonwoods and hybrid poplar 8019 (D × M) but lower growth for 5077 (T × D). In contrast, Zalesny et al. (2019) found that eastern cottonwood produced greater biomass than T × D and D × M varietals on a Coastal Plain site, but hybrids, particularly T × D varietals, performed better at a mountain site. At a better quality site similar to our Coastal Plain site, Ghezehei et al. (2019) found that eastern cottonwood varietals exhibited better growth than hybrids (T × D and D × M). Similar to our study Ghezehei et al. (2019) also found that site affected specific gravity likely due to differences in growth rate. We found some overarching trends, specifically with S7C8 (D × D) which tended to have higher specific gravity than other eastern cottonwoods at both sites. Overall, our specific gravity measurements ranged from 0.32 to 0.47 which is similar to ranges reported in Headlee et al. (2013b) and Ghezehei et al. (2019). Assuming high initial survival (which is generally seen in hybrid poplar) and similar growth as this study, 8019 (D × M) in the first 2 years of growth produced about 18 ± 1.6 Mg ha–1 of dry woody biomass (assuming the spacing of the current study) or about 9 Mg ha–1 year–1. This early productivity compares favorably with other studies being larger than P. deltoides varietals (ST66 and S7C15) growing with irrigation and fertilization in South Carolina [3.1–6.3 Mg ha–1 year–1; (Coyle and Coleman, 2005; Coyle et al., 2013)], P. deltoides growing in the Great Lakes region[2.5 Mg ha–1 year–1 (Netzer et al., 2002)], and in the Mississippi River Valley [6–7.7 Mg ha–1 year–1 (Switzer et al., 1976; Francis and Baker, 1981)]. However, the biomass seen in the first 2 years of growth in 8019 (D × M) was slightly lower than T × D hybrids growing in the Pacific Northwest [9.7 Mg ha–1 year–1 (Heilman and Zie, 1993)] and lower than estimates for hybrid poplar productivity (9.5–11.9 Mg ha–1 year–1) reported in Zalesny et al. (2016) and about half the productivity of the most superior selections (20 Mg ha–1 year–1) reported in Zalesny et al. (2009) although these growth rates may be for older, more established plantations.
We found that, across varietals and sites, leaf area and whole-tree WUE were positively correlated with biomass. Coyle et al. (2016) found that net primary productivity was driven by both leaf and fine root production in eastern cottonwoods ST66 and S7C15. Similarly, Maier et al. (2019) also found that WUE was positively correlated with growth and suggested that this relationship was controlled to a greater extent by larger photosynthetic rates as opposed to lower stomatal conductances. Our positive correlation between biomass and tree leaf area also supports the suggestion that increased photosynthesis is playing a larger role in achieving WUE as opposed to a minimization of water use. However, Jones et al. (2017) found a significant correlation between annual gross primary productivity and stand level evapotranspiration rates estimated from eddy covariance in hybrid poplars growing in Canada suggesting higher water use was associated with greater growth. In addition, we found contrasting effects of scaled GS sensitivity under differing soil moisture conditions where trees with greater stomatal sensitivity under low soil moisture but less stomatal sensitivity under high soil moisture conditions exhibited greater biomass. Although studies differ on whether the most productive Populus varietals are relatively drought tolerant (Giovannelli et al., 2007) or drought sensitive (Monclus et al., 2006), we found that individuals exhibiting plasticity in GS sensitivity depending on site and soil moisture conditions were the most productive.
Conclusion
In this study, we found that overall water use was related to tree size with larger trees (across varietals and sites) using more water than smaller trees and daily water use in this study being similar to studies in other non-irrigated Populus systems. In addition, across sites and varietals whole-tree WUE was about 5.2 g biomass per kg water which was slightly higher than WUE for Populus reported in the literature suggesting that high whole tree WUE is necessary for the high productivity. Although varietals displayed statistically similar whole-tree WUE, they used water differently throughout the growing season and exhibited different water use strategies in terms of canopy GS and its sensitivity to VPD. Varietal 8019 (D × M) used more water early in the growing season while eastern cottonwoods ST66 and S7C8 increased water use throughout the growing season likely reflecting differences in leaf phenology for these varietals. Varietal 8019 (D × M) along with 110412 (D × D) also exhibited the lowest scaled GS sensitivity under average to high soil moistures at the Coastal Plain site which differed significantly from GS sensitivity at low soil moisture suggesting a high amount of plasticity in their water use strategies corresponding to soil conditions. This plasticity in stomatal sensitivity may allow varietals 8019 (D × M) and 110412 (D × D) to exhibit higher adaptability to a range of growing sites. Across varietals, scaled GS sensitivity converged under low soil moistures at the Coastal Plain site and under high soil moistures at the LMAV site suggesting water use strategies of varietals converge during stressful conditions. We found that inoculation with endophytes caused significant impacts at the more N limited, LMAV site leading to increased biomass production and scaled GS sensitivity under low soil moistures. Across sites and varietals, whole-tree WUE was positively correlated with woody biomass, while plasticity in scaled GS sensitivity was important with lower sensitivity under high soil moisture as well as higher sensitivity under low soil moisture being positively correlated with biomass. Overall, these data shed light on the water use strategies of eastern cottonwood and hybrid poplar varietals to aid in planting recommendations and the modeling of their environmental impacts.
Data Availability Statement
The raw data supporting the conclusions of this article will be made available by the authors, without undue reservation.
Author Contributions
HR and RR conceived of, planned and received funding for the research, and revised the manuscript. HR, LS, and RR carried out the experiments and analyzed the data. HR led the writing of the manuscript. All authors contributed to the article and approved the submitted version.
Funding
This research was supported by grants from the Southeastern Sun Grant Center and the National Institute of Food and Agriculture, United States Department of Agriculture, McIntire-Stennis project (MISZ-399080).
Conflict of Interest
The authors declare that the research was conducted in the absence of any commercial or financial relationships that could be construed as a potential conflict of interest.
Acknowledgments
We would like to thank C. James, M. Murphy, T. Bowling, J. Yow, H. Stapleton, S. Avery, W. Booth, H. Borsarge, R. Calvert, C. Hinton, T. Y. Kyaw, L. Noe, J. Pitts, N. Schroeder, and K. Torres for fieldwork assistance and laboratory analysis. This publication is a contribution of the Forest and Wildlife Research Center, Mississippi State University.
Supplementary Material
The Supplementary Material for this article can be found online at: https://www.frontiersin.org/articles/10.3389/ffgc.2021.704799/full#supplementary-material
References
Arguez, A., Durre, I., Applequist, S., Squires, M., Vose, R., Yin, X., et al. (2010). NOAA’s U.S. Climate Normals (1981-2010). Asheville, NC: NOAA National Centers for Environmental Information, doi: 10.7289/V7285PN7293JP
Attia, Z., Domec, J. C., Oren, R., Way, D. A., and Moshelion, M. (2015). Growth and physiological responses of isohydric and anisohydric poplars to drought. J. Exp. Bot. 66, 4373–4381. doi: 10.1093/jxb/erv1195
Bandaru, V., Parker, N. C., Hart, Q., Jenner, M., Yeo, B. L., Crawford, J. T., et al. (2015). Economic sustainability modeling provides decision support for assessing hybrid poplar-based biofuel development in California. Calif. Agric. 69, 171–176. doi: 10.3733/ca.v069n03p171
Bassman, J. H., and Zwier, J. C. (1991). Gas exchange characteristics of Populus trichocarpa, Populus deltoides and Populus trichocarpa x P. deltoides clones. Tree Physiol. 8, 145–159. doi: 10.1093/treephys/8.2.145
Bloemen, J., Fichot, R., Horemans, J. A., Broeckx, L. S., Verlinden, M. S., Zenone, T., et al. (2017). Water use of a multigenotype poplar short-rotation coppice from tree to stand scale. Glob. Change Biol. Bioenergy 9, 370–384. doi: 10.1111/gcbb.12345
Bonhomme, L., Barbaroux, C., Monclus, R., Morabito, D., Berthelot, A., Villar, M., et al. (2008). Genetic variation in productivity, leaf traits and carbon isotope discrimination in hybrid poplars cultivated on contrasting sites. Ann. For. Sci. 65:503. doi: 10.1051/forest:2008024
Broeckx, L., Fichot, R., Verlinden, M., and Ceulemans, R. (2014). Seasonal variations in photosynthesis, intrinsic water-use efficiency and stable isotope composition of poplar leaves in a short-rotation plantation. Tree Physiol. 34, 701–715. doi: 10.1093/treephys/tpu057
Ceulemans, R., Scarascia-Mugnozza, G., Wiard, B. M., Braatne, J. H., Hinckley, T. M., Stettler, R. F., et al. (1992). Production physiology and morphology of Populus species and their hybrids grown under short rotation. I. Clonal comparisons of 4-year growth and phenology. Can. J. For. Res. 22, 1937–1948. doi: 10.1139/x92-253
Coyle, D. R., Aubrey, D. P., and Coleman, M. D. (2016). Growth responses of narrow or broad site adapted tree species to a range of resource availability treatments after a full harvest rotation. For. Ecol. Manage. 326, 107–119. doi: 10.1016/j.foreco.2015.11.047
Coyle, D. R., Aubrey, D. P., Siry, J. P., Volfovicz-Leon, R. R., and Coleman, M. D. (2013). Optimal nitrogen application rates for three intensively-managed hardwood tree species in the southeastern USA. For. Ecol. Manage. 303, 131–142. doi: 10.1016/j.foreco.2013.04.016
Coyle, D. R., and Coleman, M. D. (2005). Forest production responses to irrigation and fertilization are not explained by shifts in allocation. For. Ecol. Manage. 208, 137–152. doi: 10.1016/j.foreco.2004.11.022
Coyle, D. R., Coleman, M. D., Durant, J. A., and Newman, L. A. (2006). Multiple factors affect pest and pathogen damage on 31 Populus clones in South Carolina. Biomass Bioenergy 30, 759–768. doi: 10.1016/j.biombioe.2005.07.019
De Rosario-Martinez, H. (2015). phia: Post-Hoc Interaction Analysis. R package version 0.2-1. Available online at: https://CRAN.R-project.org/package=phia (accessed July 25, 2015).
Dillen, S. Y., Monclus, R., Barbaroux, C., Bastien, C., Ceulemans, R., Dreyer, E., et al. (2011). Is the ranking of poplar genotypes for leaf carbon isotope discrimination stable across sites and years in two different full-sib families? Ann. For. Sci. 68, 1265–1275. doi: 10.1007/s13595-011-0092-0
Dimitriou, I., Busch, G., Jacobs, S., Schmidt-Walter, P., and Lamersdorf, N. (2009). A review of the impacts of short rotation coppice cultivation on water issues. Agric. For. Res. 3, 197–206.
Dipesh, K. C., Blazier, M. A., Pelkki, M. H., and Liechty, H. O. (2017). Genotype influences survival and growth of eastern cottonwood (Populus deltoides L.) managed as a bioenergy feedstock on retired agricultural sites of the Lower Mississippi Alluvial Valley. New For. 48, 95–114. doi: 10.1007/s11056-016-9558-0
Elena, G., Fernàndez-Martínez, J., Zacchini, M., Moret, A., and Fleck, I. (2014). Susceptibility to Melampsora leaf rust of poplar clones from diverse genetic backgrounds: Effects on photochemistry and water relations. J. Plant Stud. 3:1. doi: 10.5539/jps.v5533n5532p5531
Farquhar, G. D., Ehleringer, J. R., and Hubick, K. T. (1989). Carbon isotope discrimination and photosynthesis. Annu. Rev. Plant Physiol. Plant Mol. Biol. 40, 503–537.
Fichot, R., Laurans, F., Monclus, R., Moreau, A., Pilate, G., and Brignolas, F. (2009). Xylem anatomy correlates with gas exchange, water-use efficiency and growth performance under contrasting water regimes: evidence from Populus deltoides x Populus nigra hybrids. Tree Physiol. 29, 1537–1549. doi: 10.1093/treephys/tpp087
Francis, J. K., and Baker, J. B. (1981). Biomass and nutrient accumulation in a cottonwood plantation - the first four years, Research Note SO-278. Southern Research Station New Orleans, LA: USDA Forest Service.
Ghezehei, S. B., Nichols, E. G., Maier, C. A., and Hazel, D. W. (2019). Adaptability of Populus to physiography and growing conditions in the southeastern USA. Forests 10:118. doi: 10.3390/f10020118
Gilbert, M. E., Zwieniecki, M. A., and Holbrook, N. M. (2011). Independent variation in photosynthetic capacity and stomatal conductance leads to differences in intrinsic water use efficiency in 11 soybean genotypes before and during mild drought. J. Exp. Bot. 62, 2875–2887. doi: 10.1093/jxb/erq461
Giovannelli, A., Deslauriers, A., Fragnelli, G., Scaletti, L., Castro, G., Rossi, S., et al. (2007). Evaluation of drought response of two poplar clones (Populus x canadensis Mönch ‘I-214’ and P. deltoides Marsh. ‘Dvina’) through high resolution analysis of stem growth. J. Exp. Bot. 58, 2673–2683. doi: 10.1093/jxb/erm117
Goff, J. A., and Gratch, S. (1946). “Low-pressure properties of water from -160 to 212 oF,” in Transactions of the American Society of Heating and Ventilating Engineers, (New York, NY: The Society), 95–122.
Granier, A. (1987). Evaluation of transpiration in a Douglas-fir stand by means of sap flow measurements. Tree Physiol. 3, 309–320. doi: 10.1093/treephys/3.4.309
Headlee, W. L., Zalesny, R. S. J., Donner, D. M., and Hall, R. B. (2013a). Using a process-based model (3-PG) to predict and map hybrid poplar biomass productivity in Minnesota and Wisconsin. USA. Bioenergy Res. 6, 196–210. doi: 10.1007/s12155-012-9251-x
Headlee, W. L., Zalesny, R. S. J., Hall, R. B., Bauer, E. O., Bender, B., Birr, B. A., et al. (2013b). Specific gravity of hybrid poplars in the North-Central Region, USA: within-tree variability and site x genotype effects. Forests 4, 251–269. doi: 10.3390/f4020251
Heilman, P. E., and Zie, F. (1993). Influence of nitrogen on growth and productivity of short-rotation Populus trichocarpa x Populus deltoides hybrids. Can. J. For. Res. 23, 1863–1869.
Hinckley, T. M., Brooks, J. R., Čermák, J., Ceulemans, R., Kučera, J., Meinzer, F. C., et al. (1994). Water flux in a hybrid poplar stand. Tree Physiol. 14, 1005–1018. doi: 10.1093/treephys/14.7-8-9.1005
Hothorn, T., Bretz, F., and Westfall, P. (2008). Simultaneous inference in general parametric models. Biometr. J. 50, 346–363. doi: 10.1002/bimj.200810425
Jassal, R. S., Black, T. A., Arevalo, C., Jones, H. G., Bhatti, J. S., and Sidders, D. (2013). Carbon sequestration and water use of a young hybrid poplar plantation in north-central Alberta. Biomass Bioenergy 56, 323–333. doi: 10.1016/j.biombioe.2013.05.023
Jones, H. G., Black, T. A., Jassal, R. S., Nesic, Z., Grant, N., Bhatti, J. S., et al. (2017). Water balance, surface conductance and water use efficiency of two young hybrid-poplar plantations in Canada’s aspen parkland. Agric. For. Meteorol. 246, 256–271. doi: 10.1016/j.agrformet.2017.04.007
Kandel, S. L., Firrincieli, A., Joubert, P. M., Okubara, P. A., Leston, N. D., Mcgeorge, K. M., et al. (2017). An in vitro study of bio-control and plant growth promotion potential of Salicaceae endophytes. Front. Microbiol. 8:386. doi: 10.3389/fmicb.2017.00386
Khan, Z., Rho, H., Firrincieli, A., Hung, S. H., Luna, V., Masciarelli, O., et al. (2016). Growth enhancement and drought tolerance of hybrid poplar upon inoculation with endophyte consortia. Curr. Plant Biol. 6, 38–47. doi: 10.1016/j.cpb.2016.08.001
Kim, H. S., Oren, R., and Hinckley, T. M. (2008). Actual and potential transpiration and carbon assimilation in an irrigated poplar plantation. Tree Physiol. 28, 559–577. doi: 10.1093/treephys/28.4.559
King, J., Ceulemans, R., Albaugh, J., Dillen, S. Y., Domec, J. C., Fichot, R., et al. (2013). The challenge of lignocellulosic bioenergy in a water-limited world. Bioscience 63, 102–117. doi: 10.1525/bio.2013.63.2.6
Klein, T. (2014). The variability of stomatal sensitivity to leaf water potential across tree species indicates a continuum between isohydric and anisohydric behaviours. Funct. Ecol. 28, 1313–1320. doi: 10.1111/1365-2435.12289
Land, S. B. J., and Jeffreys, J. P. (2006). “Geographic origin of cottonwood from the Southeast affects Melampsora infection in 3-year-old clonal trials,” in Proceedings of the 13th Biennial Southern Silvicultural Research Conference, ed. K. F. Connor (Asheville, NC: Department of Agriculture Forest Service, Southern Research Station), 431–437.
Maier, C. A., Burley, J., Cook, R., Ghezehei, S. B., Hazel, D. W., and Nichols, E. G. (2019). Tree water use, water use efficiency, and carbon isotope discrimination in relation to growth potential in Populus deltoides and hybrids under field conditions. Forests 10:993. doi: 10.3390/f10110993
Marron, N., Villar, M., Dreyer, E., Delay, D., Boudouresque, E., Petit, J. M., et al. (2005). Diversity of leaf traits related to productivity in 31 Populus deltoides × Populus nigra clones. Tree Physiol. 25, 425–435. doi: 10.1093/treephys/25.4.425
Migliavacca, M., Meroni, M., Manca, G., Matteucci, G., Montagnani, L., Grassi, G., et al. (2009). Seasonal and interannual patterns of carbon and water fluxes of a poplar plantation under peculiar eco-climatic conditions. Agric. For. Meteorol. 149, 1460–1476. doi: 10.1016/j.agrformet.2009.04.003
Monclus, R., Dreyer, E., Delmotte, F. M., Villar, M., Delay, D., Boudouresque, E., et al. (2005). Productivity, leaf traits and carbon isotope discrimination in 29 Populus deltoides × P. nigra clones. New Phytol. 167, 53–62. doi: 10.1111/j.1469-8137.2005.01407.x
Monclus, R., Dreyer, E., Villar, M., Delmotte, F., Delay, D., Petit, J., et al. (2006). Impact of drought on productivity and water use efficiency in 29 genotypes of Populus deltoides x Populus nigra. New Phytol. 169, 765–777. doi: 10.1111/j.1469-8137.2005.01630.x
Monclus, R., Villar, M., Barbaroux, C., Bastien, C., Fichot, R., Delmotte, F. M., et al. (2009). Productivity, water-use efficiency and tolerance to moderate water deficit correlate in 33 poplar genotypes from a Populus deltoides x Populus trichocarpa F1 progeny. Tree Physiol. 29, 1329–1339. doi: 10.1093/treephys/tpp075
Morse, L., Day, T., and Faeth, S. (2002). Effect of neotyphodium endophyte infection on growth and leaf gas exchange of Arizona fescue under contrasting water availability regimes. Environ. Exp. Bot. 48, 257–268. doi: 10.1016/s0098-8472(02)00042-4
Muller, E., and Lambs, L. (2009). Daily variations of water use with vapor pressure deficit in a plantation of I214 poplars. Water 1, 32–42. doi: 10.3390/w1010032
Natural Resources Conservation Service (2021). United States Department of Agriculture. Web Soil Survey [Online]. Available online at: https://websoilsurvey.sc.egov.usda.gov/ (accessed May, 2021).
Navarro, A., Portillo-Estrada, M., and Ceulemans, R. (2020). Identifying the best plant water status indicators for bioenergy poplar genotypes. Glob. Change Biol. Bioenergy 12, 426–444. doi: 10.1111/gcbb.12687
Netzer, D. A., Tolsted, D. N., Ostry, M. E., Isebrands, J. G., Riemenschneider, D. E., and Ward, K. T. (2002). Growth, yield, and disease resistance of 7- to 12-year old poplar clones in the North Central United States,” General Technical Report NC-229. St. Paul, MN: U.S. Department of Agriculture Forest Service, 31.
Newcombe, G., and Ostry, M. (2001). Recessive resistance to septoria stem canker of hybrid poplar. Phytopathology 91, 1081–1084. doi: 10.1094/phyto.2001.91.11.1081
Oishi, A. C., Hawthorne, D. A., and Oren, R. (2016). Baseliner: an open-source, interactive tool for processing sap flux data from thermal dissipation probes. SoftwareX 5, 139–143. doi: 10.1016/j.softx.2016.07.003
Oren, R., Sperry, J. S., Katul, G. G., Pataki, D. E., Ewers, B. E., Phillips, N., et al. (1999). Survey and synthesis of intra- and interspecific variation in stomatal sensitivity to vapour pressure deficit. Plant Cell Environ. 22, 1515–1526. doi: 10.1046/j.1365-3040.1999.00513.x
Pellis, A., Laureysens, I., and Ceulemans, R. (2004). Genetic variation of the bud and leaf phenology of seventeen poplar clones in a short rotation coppice culture. Plant Biol. 6, 38–46. doi: 10.1055/s-2003-44746
Perry, C. H., Miller, R. C., and Brooks, K. N. (2001). Impacts of short-rotation hybrid poplar plantations on regional water yield. For. Ecol. Manage. 143, 143–151. doi: 10.1016/s0378-1127(00)00513-2
Petzold, R., Schwärzel, K., and Feger, K. H. (2011). Transpiration of a hybrid poplar plantation in Saxony (Germany) in response to climate and soil conditions. Eur. J. For. Res. 130, 695–706. doi: 10.1007/s10342-010-0459-z
Phillips, N., and Oren, R. (1998). A comparison of daily representations of canopy conductance based on two conditional time-averaging methods and the dependance of daily conductance on environmental factors. Ann. For. Sci. 55, 217–235. doi: 10.1051/forest:19980113
Pinheiro, J., Bates, D., Debroy, S., and Sarkar, D. (2014). nlme: Linear and nonlinear mixed effects models”. (R package version 3.1-118). Available online at: http://CRAN.R-project.org/package=nlme (accessed July 25, 2015).
Prasolova, N. V., Xu, Z. H., Lundkvist, K., Farquhar, G. D., Dieters, M. J., Walker, S., et al. (2003). Genetic variation in foliar carbon isotope composition in relation to tree growth and foliar nitrogen concentration in clones of the F1 hybrid between slash pine and Caribbean pine. For. Ecol. Manage. 172, 145–160. doi: 10.1016/s0378-1127(01)00807-6
R Core Team (2014). R: A Language and Environment for Statistical Computing. Vienna: R Foundation for Statistical Computing.
Rae, A. M., Robinson, K. M., Street, N. R., and Taylor, G. (2004). Morphological and physiological traits influencing biomass productivity in short-rotation coppice poplar. Can. J. For. Res. 34, 1488–1498. doi: 10.1139/x04-033
Raghavendra, A. K. H., and Newcombe, G. (2013). The contribution of foliar endophytes to quantitative resistance to Melampsora rust. New Phytol. 197, 909–918. doi: 10.1111/nph.12066
Ren, J. H., Li, H., Wang, Y. F., Ye, J. R., Yan, A. Q., and Wu, X. Q. (2013). Biocontrol potential of an endophytic Bacillus pumilus JK-SX001 against poplar canker. Biol. Control 67, 421–430. doi: 10.1016/j.biocontrol.2013.09.012
Rho, H., Hsieh, M., Kandel, S. L., Cantillo, J., Doty, S. L., and Kim, S. H. (2018). Do endophytes promote growth of host plants under stress? A meta-analysis on plant stress mitigation by endophytes. Microb. Ecol. 75, 407–418. doi: 10.1007/s00248-017-1054-3
Rijtema, P. E., and De Vries, W. (1994). Differences in precipitation excess and nitrogen leaching from agricultural lands and forest plantations. Biomass Bioenergy 6, 103–113. doi: 10.1016/0961-9534(94)90089-2
Robison, T. L., Rousseau, R. J., and Zhang, J. (2006). Biomass productivity improvement for eastern cottonwood. Biomass Bioenergy 30, 735–739. doi: 10.1016/j.biombioe.2006.01.012
Rogers, A., Mcdonald, K., Muehlbauer, M. F., Hoffman, A., Koenig, K., Newman, L., et al. (2012). Inoculation of hybrid poplar with the endophytic bacterium Enterobacter sp. 638 increases biomass but does not impact leaf level physiology. GCB Bioenergy 4, 364–370. doi: 10.1111/j.1757-1707.2011.01119.x
Roy, D., Jia, X., Steele, D. D., and Lin, D. (2018). Development and comparison of soil water release curves for three soils in the red river valley. Soil Sci. Soc. Am. J. 82, 568–577. doi: 10.2136/sssaj2017.09.0324
Samuelson, L. J., Stokes, T. A., and Coleman, M. D. (2007). Influence of irrigation and fertilization on transpiration and hydraulic properties of Populus deltoides. Tree Physiol. 27, 765–774. doi: 10.1093/treephys/27.5.765
Schmidt-Walter, P., Richter, F., Herbst, M., Schuldt, B., and Lamersdorf, N. P. (2014). Transpiration and water use strategies of a young and a full-grown short rotation coppice differing in canopy cover and leaf area. Agric. For. Meteorol. 195-196, 165–178. doi: 10.1016/j.agrformet.2014.05.006
Sevigne, E., Gasol, C. M., Brun, F., Rovira, L., Pagés, J. M., Camps, F., et al. (2011). Water and energy consumption of Populus spp. bioenergy systems: a case study in Southern Europe. Renew. Sustain. Energy Rev. 15, 1133–1140. doi: 10.1016/j.rser.2010.11.034
Silim, S., Nash, R., Reynard, D., White, B., and Schroeder, W. (2009). Leaf gas exchange and water potential responses to drought in nine poplar (Populus spp.) clones with contrasting drought tolerance. Trees 23, 959–969. doi: 10.1007/s00468-009-0338-8
Song, L., Zhu, J., Zhang, T., Wang, K., Wang, G., and Liu, J. (2021). Higher canopy transpiration rates induced dieback in poplar (Populus x xiaozhuanica) in a semiarid sandy region of Northeast China. Agric. Water Manage. 243:106414. doi: 10.1016/j.agwat.2020.106414
Souch, C. A., and Stephens, W. (1998). Growth, productivity and water use in three hybrid poplar clones. Tree Physiol. 18, 829–835. doi: 10.1093/treephys/18.12.829
Stanturf, J. A., Perdue, J. H., Young, T. M., Huang, X., Guo, Z., Dougherty, D., et al. (2019). A spatially explicit approach to modeling biological productivity and economic attractiveness of short rotation woody crops in the eastern USA. Energy Sustain. Soc. 9:28. doi: 10.1186/s13705-13019-10211-13706
Stewart, L. F. (2020). Using Physiological Parameters to Refine Estimates of Short Rotation Poplar Performance and Productivity. Master’s thesis. Mississippi State, MS: Mississippi State University.
Stoof, C. R., Richards, B. K., Woodbury, P. B., Fabio, E. S., Brumbach, A. R., Cherney, J., et al. (2015). Untapped potential: opportunties and challenges for sustainable bioenergy production from marginal alnds in the Northeast USA. Bioenergy Res. 8, 482–501. doi: 10.1007/s12155-014-9515-8
Switzer, G. L., Nelson, L. E., and Baker, J. B. (1976). “Accumulation and distribution of dry matter and nutrients in Aigeiros poplar plantations,” in Proceedings of the Symposium on Eastern Cottonwood and Related Species, eds B. A. Thielges and S. B. J. Land (Baton Rouge, LA: Division of Continuing Education, Louisiana State University), 359–369.
Tardieu, F., and Simonneau, T. (1998). Variability among species of stomatal control under fluctuating soil water status and evaporative demand: modelling isohydric and anisohydric behaviours. J. Exp. Bot. 49, 419–432. doi: 10.1093/jexbot/49.suppl_1.419
Xu, H., Zhang, Z., Chen, J., Xiao, J., Zhu, M., Kang, M., et al. (2018). Regulations of cloudiness on energy partitioning and water use strategy in a riparian poplar plantation. Agric. For. Meteorol. 262, 135–146. doi: 10.1016/j.agrformet.2018.07.008
Xu, H., Zhang, Z., Chen, J., Zhu, M., and Kang, M. (2017). Cloudiness regulates gross primary productivity of a poplar plantation under different environmental conditions. Can. J. For. Res. 47, 648–658. doi: 10.1139/cjfr-2016-0413
Zalesny, R. S. J., Berndes, G., Dimitriou, I., Fritsche, U., Miller, C., Eisenbies, M., et al. (2019). Positive water linkages of producing short rotation poplars and willows for bioenergy and phytotechnologies. Wires Energy Environ. 8:e345. doi: 10.1002/wene.1345
Zalesny, R. S. J., Hall, R. B., Zalesny, J. A., Mcmahon, B. G., Berguson, W. E., and Stanosz, G. R. (2009). Biomass and genotype x environment interactions of Populus energy crops in the Midwestern United States. Bioenergy Res. 2, 106–122. doi: 10.1007/s12155-009-9039-9
Zalesny, R. S. J., Stanturf, J. A., Gardiner, E. S., Perdue, J. H., Young, T. M., Coyle, D. R., et al. (2016). Ecosystem services of woody crop production systems. Bioenergy Res. 9, 465–491. doi: 10.1007/s12155-016-9737-z
Zalesny, R. S. J., Wiese, A. H., Bauer, E. O., and Riemenschneider, D. E. (2006). Sapflow of hybrid poplar (Populus nigra L. x P. maximowiczii A. Henry ‘NM6’) during phytoremediation of landfill leachate. Biomass Bioenergy 30, 784–793. doi: 10.1016/j.biombioe.2005.08.006
Keywords: biofuels, canopy conductance, endophytes, Populus deltoides, sapflow, short rotation woody crop, SRWC
Citation: Renninger HJ, Stewart LF and Rousseau RJ (2021) Water Use, Efficiency, and Stomatal Sensitivity in Eastern Cottonwood and Hybrid Poplar Varietals on Contrasting Sites in the Southeastern United States. Front. For. Glob. Change 4:704799. doi: 10.3389/ffgc.2021.704799
Received: 03 May 2021; Accepted: 22 June 2021;
Published: 14 July 2021.
Edited by:
Thomas J. Dean, Louisiana State University, United StatesReviewed by:
Michael Tyree, Indiana University of Pennsylvania, United StatesRodney Will, Oklahoma State University, United States
Copyright © 2021 Renninger, Stewart and Rousseau. This is an open-access article distributed under the terms of the Creative Commons Attribution License (CC BY). The use, distribution or reproduction in other forums is permitted, provided the original author(s) and the copyright owner(s) are credited and that the original publication in this journal is cited, in accordance with accepted academic practice. No use, distribution or reproduction is permitted which does not comply with these terms.
*Correspondence: Heidi J. Renninger, hr427@msstate.edu