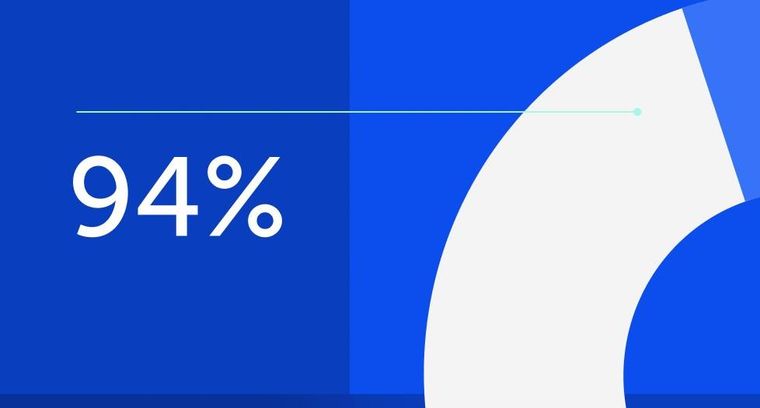
94% of researchers rate our articles as excellent or good
Learn more about the work of our research integrity team to safeguard the quality of each article we publish.
Find out more
ORIGINAL RESEARCH article
Front. For. Glob. Change, 12 July 2021
Sec. Forest Hydrology
Volume 4 - 2021 | https://doi.org/10.3389/ffgc.2021.704190
This article is part of the Research TopicBark-Water InteractionsView all 13 articles
Epiphytes, including bryophytes and lichens, can significantly change the water interception and storage capacities of forest canopies. However, despite some understanding of this role, empirical evaluations of canopy and bole community water storage capacity by epiphytes are still quite limited. Epiphyte communities are shaped by both microclimate and host plant identity, and so the canopy and bole community storage capacity might also be expected to vary across similar spatial scales. We estimated canopy and bole community cover and biomass of bryophytes and lichens from ground-based surveys across a temperate-boreal ecotone in continental North America (Minnesota). Multiple forest types were studied at each site, to separate stand level and latitudinal effects. Biomass was converted into potential canopy and bole community storage on the basis of water-holding capacity measurements of dominant taxa. Bole biomass and potential water storage was a much larger contributor than outer canopy. Biomass and water storage capacity varied greatly, ranging from 9 to >900kg ha–1 and 0.003 to 0.38 mm, respectively. These values are lower than most reported results for temperate forests, which have emphasized coastal and old-growth forests. Variation was greatest within sites and appeared to reflect the strong effects of host tree identity on epiphyte communities, with conifer-dominated plots hosting more lichen-dominated epiphyte communities with lower potential water storage capacity. These results point to the challenges of estimating and incorporating epiphyte contributions to canopy hydrology from stand metrics. Further work is also needed to improve estimates of canopy epiphytes, including crustose lichens.
The storage and evaporation of atmospheric waters by forest canopies cannot only directly impact precipitation inputs to the surface but also indirectly influence transpiration by lowering canopy VPD (Van Stan et al., 2020). Forest canopies are extensive and structurally complex spaces, made up not only of the surfaces of the tree itself (branches and leaves), but also sometimes highly diverse epiphytic communities (Zotz, 2016). Epiphytes have been found to significantly increase canopy water storage in a range of ecosystems (Van Stan and Pypker, 2015; Porada et al., 2018; Mendieta-Leiva et al., 2020).
However, despite their potential importance, studies of the hydrological role of epiphytes have been geographically patchy, focusing on ecosystems with very large amounts of vascular or non-vascular epiphytes such as old growth wet temperate (Pypker et al., 2006, 2017), tropical montane forests (Chang et al., 2002; Villegas et al., 2008; Ah-Peng et al., 2017) or other exceptionally epiphyte-rich ecosystems (Stanton et al., 2014). For example, while Porada et al. (2018) cite a number of studies of epiphyte biomass and water storage from boreal and temperate ecosystems, the majority of these were conducted in old-growth forests in humid coastal or mountainous areas: ideal conditions for the development of dense non-vascular epiphyte cover (Gehrig-Downie et al., 2011). While valuable for asserting the importance of non-vascular epiphytes, such studies may be difficult to extend to drier or younger forests at the same latitudes, on which the epiphyte communities are notably less developed. In such ecosystems, the dominant epiphytes may be small bryophytes (e.g., Frullania, Orthotrichum, and Pylaisia), microfoliose lichens (e.g., Physcia, Physconia, and associated genera), and crustose lichens. These taxa have drawn less attention from hydrologists due to their small size and therefore individually limited water-holding capacity, such that their impact is poorly quantified but a recent study of crustose lichens in dry forests found comparable biomasses to larger-bodied lichens (Miranda-González and McCune, 2020).
Here we focus on the potential water storage of non-vascular epiphytes across a range of continental temperate to boreal forests in central North America. Vascular epiphytes are essentially absent from such forests, and epiphyte communities are dominated by non-vascular epiphytes: lichens, mosses, and liverworts. Non-vascular epiphytes are notable for their dynamic water content (poikilohydry), which is often associated with considerable water storage capacity. The water-holding capacity of lichens and bryophytes can be quite substantial given their relatively small mass and/or size. For example, lichens can store up to 3,360% of their dry weight in water and some bryophytes even more (Cornelissen et al., 2007; Elumeeva et al., 2011; Gauslaa and Coxson, 2011; Klamerus-Iwan et al., 2020).
In evaluating the epiphyte communities and their potential hydrologic effects we consider several scales of organization. Firstly, location within canopy: the communities on the bole and bases of large branches are often quite different from those in the upper and outer regions of the canopy (Zotz, 2016). In continental temperate forests the former are more often dominated by bryophytes, crustose and foliose lichens while the latter can harbor more fruticose lichens. The likely hydrologic impacts also differ: while both may influence canopy interception and storage, bole epiphyte communities primarily intercept stemflow whereas the outer canopy community may have more impact on through-fall (Mendieta-Leiva et al., 2020). Furthermore, north- and south-facing sides of the bole differ in their microclimate and sometimes epiphyte communities (Ellis, 2012). These are important hydrological and ecological factors to consider as canopy interception remains largely under-studied (Van Stan et al., 2020; Zheng and Jia, 2020; Linhoss and Siegert, 2020).
Secondly, epiphyte communities can vary with host tree due to light-penetration, crown architecture, and bark texture and chemistry (Smith, 1982; Ellis, 2012; Zotz, 2016). Tree species may also shape stand-level microclimate, which can vary greatly between forest types. Lastly, macroclimate can also greatly shape epiphyte communities following changing temperature and precipitation across latitudinal and longitudinal (Smith et al., 2020) or altitudinal scales (Rodriguez-Quiel et al., 2019).
To systematically evaluate the potential hydrologic impacts of epiphytes in northern temperate forests we evaluated epiphytic communities of dominant forest types across a latitudinal gradient marking the transition from savanna and temperate forest to boreal forest in Minnesota, United States. The sites span the ecotone of three of the major biomes of continental North America (Prairie, Eastern temperate deciduous forest, Laurentian boreal forest) and each include a mosaic of upland and lowland forests with differing dominant trees. Because this range abuts the edge of extensive forests (forest-prairie ecotone), it covers an ecologically important if climatically narrow range of temperature and precipitation. We hypothesized that epiphyte biomass and water storage potential would increase with latitude, reflecting decreasing evaporative stresses that might favor the development of epiphyte communities.
Epiphyte communities were sampled on or near University of Minnesota field stations (Figure 1A), namely Cedar Creek Ecosystem Science Reserve (East Bethel, MN, United States), Cloquet Forestry Center (Cloquet, MN, United States), Itasca State Park (Park Rapids, MN, United States), and Hubachek Wilderness Research Center (Ely, MN, United States). Dominant forest communities as described by the Minnesota Department of Natural Resources (MDNR)’s Native Plant Community classification (Aaseng et al., 2011) were sampled at each site (Figure 1B), and site specific data are presented in Table 1. These 4 sites were chosen because they span the western limit of the Eastern Deciduous Forest biome and the ecotones with Laurentian Shield Boreal and Prairie biomes in North America, encompassing some of the largest North-American biomes in a small geographic space.
Figure 1. Sampling design at state (A), site (B), and plot (C) scales. A-Sites (1-Cedar Creek Ecosystem Reserve, 2-Cloquet Forestry Center, 3-Hubachek Wilderness Research Center, and 4-Itasca State Park) are located across the eastern temperate (light green) to boreal (dark green) forest transition. (B) Within each site plots were selected to represent in forest types (top-Aspen-Birch, middle-Tamarack-Black Spruce bog, and bottom- Black Ash swamp). (C) Each plot includes 2 m-radius microplots for litter collection and 20 cm × 50 cm quadrats at 4 locations on 12 microplot center trees for bole epiphyte sampling. Photo credit: A. Glauser and D. Stanton.
Plots were chosen by overlaying MDNR GIS maps1 of the selected dominant forest communities onto our sites, then marking a coordinate that was (a) well away from the community edges and (b) easily accessible to the field team. The field team then used the MDNR Native Plant Community classification system to confirm the forest community type upon arrival at the coordinate. If the forest community was correct and far enough away from community edges and disturbances, a plot was set up. If the coordinates were too close to an edge, not the best representation, or showed disturbance, the field team chose a different coordinate. Plot centers were in a few occasions moved 5–20 m to avoid edge effects or overlap with adjacent forest types.
Temperature and precipitation data for each site was obtained from the nearest weather station in the Climate Data Online repository2 with a 10 years record of daily means (January 01, 2010–December 31, 2020): Cambridge MN (for Cedar Creek), Cloquet, Itasca University of Minnesota and Ely 25 E (for HWRC). From these we calculated the median rainfall event size for each site.
Biomass estimation: Plots were established as circular plots of 36.6 m (120 ft) radius with care to avoid patch edges. Forest type was assigned following the Minnesota Native Plant Community Classification (Aaseng et al., 2011) and basal area measured using a wedge prism (Jim-Gem Rectangular prism, Forestry Suppliers). Within each plot, transects were traced in the 4 cardinal directions, and microplots (2 m radius circles) established at 6, 14, and 22 m from plot center (Figure 1C). Upper canopy communities were estimated by harvesting all recently fallen litter (epiphytes or twigs/branches with epiphytes attached) as an indirect measure (modified from McCune, 1993). The living tree nearest to the microplot center was used for bole community surveys. Trees for bole community estimates (12/plot) were identified to species and diameter at breast height (DBH) recorded. Quadrats (20 cm × 50 cm) were affixed to the bole on the north and south sides of the trunk at ground level and 1.5 m height (4 total per tree). Within each quadrat the relative cover of each epiphyte functional group was recorded. Taxa were assigned to the following functional groups based on growth form: Crustose Lichen (e.g., Lecanora), Cyanolichen (e.g., Peltigera), Small Foliose Lichen (e.g., Physcia and Physconia), Large Foliose Lichen (e.g., Parmelia and Platismatia), Tufted Fruticose Lichen (e.g., Ramalina and Usnea), Pendulous Fruticose Lichen (e.g., Bryoria), Jelly Lichen (e.g., Leptogium), Small Prostrate Bryophyte (e.g., Frullania), Large Prostrate Bryophyte (e.g., Anomodon), Feathermoss Bryophyte (e.g., Pleurozium), Small Bryophyte Cushion (e.g., Orthotrichum), Large Bryophyte Cushion (e.g., Dicranum) and Pendent Bryophyte Mat (e.g., Neckera and Porella). Results of species surveys and ground sampling will be presented in a forthcoming publication (Route et al., in prep).
Water-holding capacity (WHC) and Specific Mass (SM) were measured for representative lichen and bryophyte taxa following established methods (Esseen et al., 2017; Ure and Stanton, 2019). Briefly, individual lichen thalli and bryophyte clumps were detached from their substrate and cleaned of debris in the lab before hydration. To hydrate, samples were placed over mesh and sprayed three times with distilled water at 2 min intervals for at least 15 min (20 min for large samples), flipping the sample between sprays to ensure even hydration. Following hydration the lichen thalli were placed between two mesh pieces and gently shaken three times on each side. After which filter paper is used to absorb the external water still remaining, thus leaving the internal water. Between each of these processes the wet mass was recorded. Once fully hydrated wet mass was recorded with a Mettler BB2400 digital scale (Mettler Toledo, Columbus, OH, United States) following gentle blotting (internal WHC). After these measurements the thalli were placed between two glass plates and a ruler was added for scale. A photograph of the hydrated sample projected area was taken using a light-table (to emphasize contrast) and a vertically mounted Canon EOS 80D DSLR camera (Canon U.S.A., Inc., Melville, NY, United States). The areas were measured from photographs using ImageJ 2.0 (Rueden et al., 2017). Samples were air dried for at least 48 h to obtain a dry mass.
All calculations and analyses were carried out R 4.0.5 (R Core Team, 2021). Specific Mass was calculated as: SM = oven dry mass (DM)/blotted wet area (Awet). Water-holding capacity as calculated as (WHC) = (wet mass (WM) − DM)/Awet.
Data from multiple species was used to calculate a mean SM and WHC for each functional group, which are presented in Table 2. Although these properties may also vary within a functional group depending on site and species characteristics, this will be explored in a forthcoming study.
The functional group mean values of Specific Mass and WHC were then multiplied by cover to calculate the quadrat-scale SM and WHC attributable to each functional group:
These were then summed to calculate four quadrat-level Biomass and WHC values for each bole (North and South aspects at 0 and 1.5 m, respectively).
To convert quadrat-level values to tree-level we approximated each tree bole as a 10 m cylinder of constant diameter. Mean Biomass and WHC from the North and South ground level quadrats was used to calculate Biomass and WHC of the base of the tree 0–0.5 m; mean SM and WHC from the North and South 1.5 m quadrats was used to calculate Biomass and WHC of the rest (0.5–10 m):
Stand-scale estimates were made by dividing the total basal area of trees by the mean bole cross-sectional area to approximate the number of trees:
All data analysis was conducted in R 4.0.5 (R Core Team, 2021). Linear regressions were used to evaluate the relationships between epiphyte community properties (biomass and potential water storage capacity) and broad geographic (Latitude), macroclimatic (Mean Annual Precipitation) and forest structure (% conifers, physiographic position).
A total of 23 plots were studied across 4 sites, encompassing 7 major forest types and 11 different native plant communities according to the MN DNR Classification. Biomass and potential water storage capacity varied across 2 orders of magnitude, ranging from ∼9 to >900 kg ha–1 and 0.003–0.38 mm, respectively (Table 3). The nearly full spectrum of variation was present within sites and even sometimes within native plant communities, indicating stand-level variation in epiphyte biomass and potential water storage. Nearly all of the estimated biomass was due to bole epiphytes, with canopy epiphyte biomass 100–300 times smaller.
Table 3. Biomass and potential water-holding capacity (as both L/ha and mm depth equivalent) of bole, canopy and total epiphyte communities at each study site.
Bole biomass showed no significant change with latitude (t = 1.934, F1,21 = 3.739, p = 0.0668, adj-R2 = 0.11, Figure 2A) or precipitation (t = −0.497, F1,21 = 0.2472, p = 0.624, adj-R2 = −0.035, Figure 2B). There was no correlation between bole and canopy biomass (t = 0.878, F1,21 = 0.7713, p = 0.0.39, adj-R2 = −0.01, Figure 2C) or water storage capacity. Canopy biomass and thus water holding capacity was much lower than on the boles, but showed stronger responses to latitude and climate. Canopy biomass increased with latitude (t = 2.303, F1,21 = 5.305, p = 0.0319, adj-R2 = 0.16), and a slight but significant decrease with precipitation (t = −2.152, F1,21 = 4.631, p = 0.0432, adj-R2 = 0.14).
Figure 2. Relationships of bole epiphyte community biomass (A–C) and potential water storage capacity (D–F) to plot and site characteristics, including site latitude (A,C), mean annual precipitation (B,E) and stand composition (F). Results of linear regressions are reported in each sub-panel. n.s., not significant at p < 0.05.
These translate into equivalent patterns with bole community water storage capacity: no change with latitude (t = 0.046, F1,21 = 0.002, p = 0.9637, adj-R2 = −0.05, Figure 2D) or precipitation (t = −1.910, F1,21 = 3.648, p = 0.0699, adj-R2 = 0.11, Figure 2E). Water storage capacity on the bole community was significantly related to forest composition, with lower capacity in conifer-dominated forests (t = −2.814, F1,21 = 7.92, p = 0.0104, adj-R2 = 0.24, Figure 2F). As with biomass, patterns were more marked in canopy water storage capacity: increase with latitude (t = 2.208, F1,21 = 4.855, p = 0.0388, adj-R2 = 0.15) and decrease with precipitation (t = 2.131, F1,21 = 4.539, p = 0.0451, adj-R2 = 0.14).
Water-holding capacity was much greater in the bryophytes than the lichens (Figure 3A). As a result, total water storage was strongly inversely correlated with the proportion of lichens in the epiphyte community (t = −3.787, F1,21 = 14.34, p = 0.001, adj-R2 = 0.38, Figure 3B). The proportion of lichens varied considerably within sites, ranging from 10 to >95%, but with no significant relationship with latitude. Due to the greater water capacity of bryophytes in our study sites, this translated into a usually <50% contribution of lichens to bole community water capacity but no significant latitudinal pattern (t = 0.949, F1,21 = 0.9004, p = 0.3535, adj-R2 = −0.004, Figure 3C). Proportion of lichens was significantly greater in conifer-dominated stands (t = 4.557, F1,21 = 20.76, p = 0.0002, adj-R2 = 0.47, Figure 3D).
Figure 3. Contrasting impacts of bryophytes and lichens on potential canopy water storage. Bryophytes tended to have greater internal water holding capacity across all functional groups (A), leading to a negative relationship between lichen dominance and potential water storage (B). Lichen dominance of epiphyte communities was not explained by latitude (C) but increased significantly in conifer dominated stands (D). n.s., not significant at p < 0.05.
Potential water storage by bole and canopy epiphytes amounted to 5–15% of the median 24 h rainfall at the study sites (Figure 4), with a significantly greater potential impact at the driest site (Itasca State Park; F3 = 8.568, p < 0.0008). This pattern was driven more by differences in rain event size (Table 3) than epiphyte biomass.
Figure 4. Potential canopy water storage by epiphytes as a proportion of median rainfall event size (median 24 h precipitation 2010–2020) at study sites. Letters indicate significantly different groups (p < 0.05) according to Tukey HSD post-hoc tests.
Estimated biomass and water storage potential of epiphytes on boles was significant, but varied greatly between and even within forest types. Contrary to expectation, bole biomass did not show a clear latitudinal or climatic pattern across the ranges considered, which cover the transition between continental temperate and boreal forests in North America. Although estimated potential water storage by epiphytes was lower than reported for more epiphyte rich coastal and montane temperate environments, it reached hydrologically important levels in some stands.
We hypothesized a latitudinal increase in epiphyte biomass and storage capacity, based on decreasing potential evapotranspiration typically favoring greater epiphyte cover. Contrary to expectations, bole epiphyte biomass showed no response to latitude, and a negative correlation with annual precipitation. In contrast, canopy biomass, which represented only a minor fraction of the estimated biomass, increased with latitude and decreased with precipitation. A likely explanation for this result is the extensive cover of crustose lichens and small bryophytes at our lower latitude sites. These groups are less showy than the larger bodied macrolichens, and have often been overlooked in studies of ecosystem roles of epiphytes due to their small size. However, some studies of crustose lichen communities have found that they can represent comparable biomass to macrolichens (Miranda-González and McCune, 2020). Although the total estimated biomass of crustose lichens at our sites was far less than reported for tropical dry forests by Miranda-González and McCune (2020), it is nonetheless indicative of an under-valued component of canopy communities.
In the absence of macroclimatic predictors of epiphyte biomass and potential water storage, forest type appeared to be a strong driver of epiphyte community properties. Non-vascular epiphytes communities are known to be strongly influenced by host tree species, reflecting effects of bark texture, chemistry, and light availability (Ellis, 2012; Mitchell et al., 2021). Canopy structure also influences the partitioning of rainfall between throughfall and stemflow (Levia and Frost, 2003; Levia et al., 2019; Van Stan et al., 2020), which can further influence bole epiphyte communities. This effect of tree species can be seen in the strong correlations between potential water storage capacity and hardwood dominance: conifer-dominated plots had more lichen-dominated epiphyte communities and lower potential water storage. However, this broad pattern is insufficient to account for the full variation in potential water storage, especially in hardwood-dominated plots, which may be attributable in part to variation in specific host tree species. This impact of host tree identity suggests that future attempts to incorporate epiphytes into temperate-boreal ecohydrology need to account for the interactions between epiphytes, trees and climate. Preferably, species-specific allometries of canopy structure might also be applied to obtain more precise estimates of epiphyte biomass than the coarse and conservative scaling estimates presented here.
Potential storage capacity is not necessarily the same as realized capacity in natural rain events. Because of the focus on the bole, the epiphyte communities documented here primarily influence stemflow, and may have limited impacts on throughfall. Some additional factors also influence the realized storage capacity. Water storage in many bryophytes and lichens can be external as well as internal, with external water holding capacity sometimes equally or exceeding internal (e.g., Elumeeva et al., 2011; Esseen et al., 2017). Because external water-holding capacity estimates were not available for all functional groups, and external water-holding capacity is less closely correlated to mass and area (Eriksson et al., 2018), we chose to only focus on internal water storage. This likely under-estimated potential water storage capacity.
Other factors may decrease realized storage capacity when compared to estimated potential. As with leaves and bark (Eller et al., 2013; Holder, 2013; Klamerus-Iwan and Błońska, 2018), the surface hydrophobicity of lichens and bryophytes can vary not just across species, but also with hydration status, with dry thalli often more hydrophobic than those “primed” by high humidity or wetting (Lakatos et al., 2006). Epiphytes may also still be retaining water from previous precipitation or fog (Hargis et al., 2019). As such, the humidity conditions preceding a rain event may impact the amount of water retained by epiphytes. Secondly, stemflow does not proceed equally down the bole, and so some regions of the bole may contribute more than others to canopy interception. Experimental validation of epiphyte interception is needed for future work. Experimental measurements of epiphyte impacts on stem flow have been conducted in other forests (Van Stan and Pypker, 2015; Chen et al., 2019; Van Stan et al., 2020), and these methods might be adapted to temperate non-vascular epiphytes.
The estimations of epiphyte biomass applied here were ground-based, which is far more efficient than climbing or crane-based approaches but rely on assumptions of relative vertical homogeneity of epiphyte properties. Epiphyte communities can vary with vertical position in the canopy (Ellis, 2012), such that ground-based observations only capture a portion of the cover and diversity. Many non-vascular epiphytes are slow-growing, and epiphytes communities vary with branch age as well as position (Woods, 2017).However, we sought to minimize these impacts through conservative estimates of bole surface area, ignoring large branches and any portions of the main stem >10 m. Our ground-based estimate of canopy epiphyte biomass is also intended to be a conservative estimate, emphasizing the larger-bodied epiphytes on terminal branches that are most likely to be collected in litter-fall (McCune, 1993; Dettki and Esseen, 2003).
Forest age is also likely to be an important factor. In contrast to many prior studies in older forests (e.g., Liu et al., 2000; Köhler et al., 2007; Pypker et al., 2017), only one site in the present study was >100 years (Cloquet Forestry Center Pinus resinosa forest). This age bias reflects both natural disturbance from fire and windthrow and a history of logging in Minnesota, such that few centennial stands of forest persist (Friedman and Reich, 2005; Vogeler et al., 2020). The potential hydrological importance of epiphytes and other components of old-growth forests is a strong argument for their conservation, but may not represent regional patterns in areas where forest cover is more dynamic, such as Eastern and Central North America. Our estimated potential water storage capacities lie toward the lower end of the range estimated by Porada et al. (2018) in their global model of canopy interception in temperate forests.
While the results reported here suggest greater microclimatic than macroclimatic effects on epiphyte water storage potential, extension to regional patterns is limited by the low number of sites and uneven replication of forest types. Macroclimate patterns in Minnesota reflect the intersection of a South to North decreasing gradient in temperature and a South-East to North-West gradient of decreasing precipitation. These combine to create a longitudinal gradient in Potential Evapotranspiration, with PET increasing from East to West. Although our selection of sites captured the latitudinal range in Temperature, all but one of the sites (Itasca State Park) were at the same longitude. Furthermore the clustering of plots within macrosites (due to logistical constraints of accessibility) limits the interpretation of macroclimate as a linear variable. Further studies are currently ongoing to broaden the geographic, macroclimatic and vegetational range of data.
Although easily overlooked, non-vascular epiphytes have the potential to impact forest hydrology even in continental environments where their apparent biomass can seem small. The potential impact on forest hydrology found in our sites across the temperate-boreal ecotone was lower than that reported in more humid, coastal temperate and sub-boreal forests where most prior studies have been conducted. This potential canopy storage by epiphytes was site and forest-type specific, with considerable variation even in nearly adjacent plots. While approximate, we hope that the relatively rapid and easy techniques presented here might serve a wider survey of the potential roles of epiphytes in forest hydrology, one that does not just consider the large-bodied non-vascular and vascular components, but also the small but extensive cover of small non-vascular epiphytes as well.
The original contributions presented in the study are included in the article/Supplementary Material, further inquiries can be directed to the corresponding author/s.
DS and AG designed the study. AG and TR oversaw site selection, sample collection, and processing. AG, TR, AM, and KH collected data and processed samples. KH and DS analyzed the data. KH, AM, and DS wrote the manuscript, with contributions from TR. All authors contributed to the article and approved the submitted version.
Funding for this project was provided by the Minnesota Environmental and Natural Resources Trust Fund Project M.L. 2018, Chp. 214, Art. 4, Sec. 02, Subd. 03e (Assessing Natural Resource Benefits Provided by Lichens and Mosses) awarded to DS.
The authors declare that the research was conducted in the absence of any commercial or financial relationships that could be construed as a potential conflict of interest.
Work at each of the sites was made possible by the invaluable assistance of station staff, including K. Gill, B. Prange, S. Oberg, L. Domine, L. Knoll, M. Lauzon, T. Mielke, K. Worm, and others. Field and labwork collecting and processing samples for biomass estimation included the assistance of J. Ure, K. Stone, M. Valentin, D. Last, H. Bjorklund, L. Liulevicius, M. Syed, and S. Treuchel. O. Gockman, J. Thayer, T. McDonald, T. Clark, and members of the Stanton lab provided valuable feedback on project design and manuscript drafts.
The Supplementary Material for this article can be found online at: https://www.frontiersin.org/articles/10.3389/ffgc.2021.704190/full#supplementary-material
Aaseng, N. E., Almendinger, J. C., Dana, R. P., Hanson, D. S., Lee, M. D., Rowe, E. R., et al. (2011). Minnesota’s Native Plant Community Classification: A Statewide Classification of Terrestrial and Wetland Vegetation Based on Numerical Analysis of Plot Data. Biological Report No. 108. Minnesota County Biological Survey, Ecological Land Classification Program, and Natural Heritage and Nongame Research Program. St. Paul: Minnesota Department of Natural Resources.
Ah-Peng, C., Cardoso, A. W., Flores, O., West, A., Wilding, N., Strasberg, D., et al. (2017). The role of epiphytic bryophytes in interception, storage, and the regulated release of atmospheric moisture in a tropical montane cloud forest. J. Hydrol. 548, 665–673. doi: 10.1016/j.jhydrol.2017.03.043
Chang, S.-C., Lai, I.-L., and Wu, J.-T. (2002). Estimation of fog deposition on epiphytic bryophytes in a subtropical montane forest ecosystem in northeastern Taiwan. Atmospheric Res. 64, 159–167. doi: 10.1016/s0169-8095(02)00088-1
Chen, L.-C., Wang, L.-J., Martin, C. E., and Lin, T.-C. (2019). Mediation of stemflow water and nutrient availabilities by epiphytes growing above other epiphytes in a subtropical forest. Ecohydrology 12:e2140.
R Core Team (2021). R: A Language and Environment for Statistical Computing. Vienna: R Foundation for Statistical Computing.
Cornelissen, J. H. C., Lang, S. I., Soudzilovskaia, N. A., and During, H. J. (2007). Comparative cryptogam ecology: a review of bryophyte and lichen traits that drive biogeochemistry. Ann. Bot. 99, 987–1001. doi: 10.1093/aob/mcm030
Dettki, H., and Esseen, P.-A. (2003). Modelling long-term effects of forest management on epiphytic lichens in northern Sweden. Forest Ecol. Manag. 175, 223–238. doi: 10.1016/s0378-1127(02)00131-7
Eller, C. B., Lima, A. L., and Oliveira, R. S. (2013). Foliar uptake of fog water and transport belowground alleviates drought effects in the cloud forest tree species, Drimys brasiliensis (Winteraceae). New Phytol. 199, 151–162. doi: 10.1111/nph.12248
Ellis, C. J. (2012). Lichen epiphyte diversity: A species, community and trait-based review. Perspect. Plant Ecol. Evol. Syst. 14, 131–152. doi: 10.1016/j.ppees.2011.10.001
Elumeeva, T. G., Soudzilovskaia, N. A., During, H. J., and Cornelissen, J. H. C. (2011). The importance of colony structure versus shoot morphology for the water balance of 22 subarctic bryophyte species: Factors affecting bryophyte water balance. J. Veget. Sci. 22, 152–164. doi: 10.1111/j.1654-1103.2010.01237.x
Eriksson, A., Gauslaa, Y., Palmqvist, K., Ekström, M., and Esseen, P.-A. (2018). Morphology drives water storage traits in the globally widespread lichen genus Usnea. Fungal Ecol. 35, 51–61. doi: 10.1016/j.funeco.2018.06.007
Esseen, P.-A., Rönnqvist, M., Gauslaa, Y., and Coxson, D. S. (2017). Externally held water – a key factor for hair lichens in boreal forest canopies. Fungal Ecol. 30, 29–38. doi: 10.1016/j.funeco.2017.08.003
Friedman, S. K., and Reich, P. B. (2005). Regional legacies of logging: departure from presettlement forest conditions in Northern Minnesota. Ecol. Appl. 15, 726–744. doi: 10.1890/04-0748
Gauslaa, Y., and Coxson, D. (2011). Interspecific and intraspecific variations in water storage in epiphytic old forest foliose lichens. Botany 89, 787–798. doi: 10.1139/b11-070
Gehrig-Downie, C., Obregón, A., Bendix, J., and Gradstein, S. R. (2011). Epiphyte biomass and canopy microclimate in the tropical lowland cloud forest of French Guiana: Epiphyte abundance in lowland cloud forest. Biotropica 43, 591–596. doi: 10.1111/j.1744-7429.2010.00745.x
Hargis, H., Gotsch, S. G., Porada, P., Moore, G. W., Ferguson, B., and Van Stan, J. T. (2019). Arboreal epiphytes in the soil-atmosphere interface: how often are the biggest “buckets” in the canopy empty? Geosciences 9:342. doi: 10.3390/geosciences9080342
Holder, C. D. (2013). Effects of leaf hydrophobicity and water droplet retention on canopy storage capacity. Ecohydrology 6, 483–490. doi: 10.1002/eco.1278
Klamerus-Iwan, A., and Błońska, E. (2018). Canopy storage capacity and wettability of leaves and needles: the effect of water temperature changes. J. Hydrol. 559, 534–540. doi: 10.1016/j.jhydrol.2018.02.032
Klamerus-Iwan, A., Kozłowski, R., Przybylska, J., Solarz, W., and Sikora, W. (2020). Variability of water storage capacity in three lichen species. Biologia 75, 899–906. doi: 10.2478/s11756-020-00437-7
Köhler, L., Tobón, C., Frumau, K. F. A., and Bruijnzeel, L. A. (2007). Biomass and water storage dynamics of epiphytes in old-growth and secondary montane cloud forest stands in Costa Rica. Plant Ecol. 193, 171–184. doi: 10.1007/s11258-006-9256-7
Lakatos, M., Rascher, U., and Büdel, B. (2006). Functional characteristics of corticolous lichens in the understory of a tropical lowland rain forest. New Phytol. 172, 679–695. doi: 10.1111/j.1469-8137.2006.01871.x
Levia, D. F., and Frost, E. E. (2003). A review and evaluation of stemflow literature in the hydrologic and biogeochemical cycles of forested and agricultural ecosystems. J. Hydrol. 274, 1–29. doi: 10.1016/s0022-1694(02)00399-2
Levia, D. F., Nanko, K., Amasaki, H., Giambelluca, T. W., Hotta, N., Iida, S., et al. (2019). Throughfall partitioning by trees. Hydrol. Process. 33, 1698–1708. doi: 10.1002/hyp.13432
Linhoss, A. C., and Siegert, C. M. (2020). Calibration reveals limitations in modeling rainfall interception at the storm scale. J. Hydrol. 584:124624. doi: 10.1016/j.jhydrol.2020.124624
Liu, C., Ilvesniemi, H., and Westman, C. J. (2000). Biomass of arboreal lichens and its vertical distribution in a boreal coniferous forest in central Finland. Lichenologist 32, 495–504. doi: 10.1006/lich.2000.0288
McCune, B. (1993). Gradients in epiphyte biomass in three Pseudotsuga-Tsuga forests of different ages in western Oregon and Washington. Bryologist 96, 405–411. doi: 10.2307/3243870
Mendieta-Leiva, G., Porada, P., and Bader, M. Y. (2020). “Interactions of epiphytes with precipitation partitioning,” in Precipitation Partitioning by Vegetation: A Global Synthesis, eds I. Van Stan John, T. E. Gutmann, and J. Friesen (Cham: Springer International Publishing), 133–146. doi: 10.1007/978-3-030-29702-2_9
Miranda-González, R., and McCune, B. (2020). The weight of the crust: biomass of crustose lichens in tropical dry forest represents more than half of foliar biomass. Biotropica 52, 1298–1308. doi: 10.1111/btp.12837
Mitchell, R. J., Hewison, R. L., Beaton, J., and Douglass, J. R. (2021). Identifying substitute host tree species for epiphytes: the relative importance of tree size and species, bark and site characteristics. Appl. Veget. Sci. 24:e12569.
Porada, P., Stan, J. T. V., and Kleidon, A. (2018). Significant contribution of non-vascular vegetation to global rainfall interception. Nat. Geosci. 11, 563–567. doi: 10.1038/s41561-018-0176-7
Pypker, T. G., Unsworth, M. H., and Bond, B. J. (2006). The role of epiphytes in rainfall interception by forests in the Pacific Northwest. II. Field measurements at the branch and canopy scale. Can. J. Forest Res. 36, 819–832. doi: 10.1139/x05-286
Pypker, T. G., Unsworth, M. H., Van Stan, J. T., and Bond, B. J. (2017). The absorption and evaporation of water vapor by epiphytes in an old-growth Douglas-fir forest during the seasonal summer dry season: implications for the canopy energy budget: Impact of epiphytes on the canopy hydrology of Douglas-fir forests. Ecohydrology 10:e1801. doi: 10.1002/eco.1801
Rodriguez-Quiel, E. E., Mendieta-Leiva, G., and Bader, M. Y. (2019). Elevational patterns of bryophyte and lichen biomass differ among substrates in the tropical montane forest of Barú Volcano, Panama. J. Bryol. 41, 95–106. doi: 10.1080/03736687.2019.1584433
Rueden, C. T., Schindelin, J., Hiner, M. C., DeZonia, B. E., Walter, A. E., Arena, E. T., et al. (2017). ImageJ2: IMAGEJ for the next generation of scientific image data. BMC Bioinform. 18:529. doi: 10.1186/s12859-017-1934-z
Smith, R. J., Jovan, S., Stanton, D., and Will-Wolf, S. (2020). Epiphytic macrolichen communities indicate climate and air quality in the U.S. Midwest. Bryo 123, 517–533.
Stanton, D. E., Huallpa Chávez, J., Villegas, L., Villasante, F., Armesto, J., Hedin, L. O., et al. (2014). Epiphytes improve host plant water use by microenvironment modification. Funct. Ecol. 28, 1274–1283. doi: 10.1111/1365-2435.12249
Ure, J. D., and Stanton, D. E. (2019). Co-dominant anatomically disparate lichens converge in hydrological functional traits. Bryo 122, 463–470. doi: 10.1639/0007-2745-122.3.463
Van Stan, J. T., Gutmann, E., and Friesen, J. (2020). Precipitation Partitioning by Vegetation: A Global Synthesis. Salmon Tower Building, NY: Springer International Publishing.
Van Stan, J. T., and Pypker, T. G. (2015). A review and evaluation of forest canopy epiphyte roles in the partitioning and chemical alteration of precipitation. Sci. Total Environ. 536, 813–824. doi: 10.1016/j.scitotenv.2015.07.134
Villegas, J. C., Tobón, C., and Breshears, D. D. (2008). Fog interception by non−vascular epiphytes in tropical montane cloud forests: dependencies on gauge type and meteorological conditions. Hydrol. Process. 22, 2484–2492. doi: 10.1002/hyp.6844
Vogeler, J. C., Slesak, R. A., Fekety, P. A., and Falkowski, M. J. (2020). Characterizing over four decades of forest disturbance in Minnesota. USA. Forests 11:362. doi: 10.3390/f11030362
Woods, C. L. (2017). Primary ecological succession in vascular epiphytes: the species accumulation model. Biotropica 49, 452–460. doi: 10.1111/btp.12443
Zheng, C., and Jia, L. (2020). Global canopy rainfall interception loss derived from satellite earth observations. Ecohydrology 13:e2186.
Keywords: interception, stemflow, water-holding capacity (WHC), bryophytes, lichen, canopy hydrology
Citation: Hembre K, Meyer A, Route T, Glauser A and Stanton DE (2021) Stand-Level Variation Drives Canopy Water Storage by Non-vascular Epiphytes Across a Temperate-Boreal Ecotone. Front. For. Glob. Change 4:704190. doi: 10.3389/ffgc.2021.704190
Received: 01 May 2021; Accepted: 11 June 2021;
Published: 12 July 2021.
Edited by:
Anna Klamerus-Iwan, University of Agriculture in Krakow, PolandReviewed by:
Paolo Giordani, University of Genoa, ItalyCopyright © 2021 Hembre, Meyer, Route, Glauser and Stanton. This is an open-access article distributed under the terms of the Creative Commons Attribution License (CC BY). The use, distribution or reproduction in other forums is permitted, provided the original author(s) and the copyright owner(s) are credited and that the original publication in this journal is cited, in accordance with accepted academic practice. No use, distribution or reproduction is permitted which does not comply with these terms.
*Correspondence: Daniel E. Stanton, c3RhbkB1bW4uZWR1; c3RhbjA0NzdAdW1uLmVkdQ==
Disclaimer: All claims expressed in this article are solely those of the authors and do not necessarily represent those of their affiliated organizations, or those of the publisher, the editors and the reviewers. Any product that may be evaluated in this article or claim that may be made by its manufacturer is not guaranteed or endorsed by the publisher.
Research integrity at Frontiers
Learn more about the work of our research integrity team to safeguard the quality of each article we publish.