- 1Oak Ridge National Laboratory, Environmental Sciences Division and Climate Change Science Institute, Oak Ridge, TN, United States
- 2Ecology and Evolutionary Biology, University of Tennessee, Knoxville, Knoxville, TN, United States
- 3Viticulture and Enology, University of California, Davis, Davis, CA, United States
Tree species that are successful in tropical lowlands have different acquisition strategies to overcome soil phosphorus (P) limitations. Some of these strategies belowground include adjustments in fine-root traits, such as morphology, architecture, association with arbuscular mycorrhizal fungi, and phosphatase activity. Trade-offs among P-acquisition strategies are expected because of their respective carbon cost. However, empirical evidence remains scarce which hinders our understanding of soil P-acquisition processes in tropical forests. Here, we measured seven fine-root functional traits related to P acquisition of five common tree species in three sites of the Luquillo Experimental Forest in Puerto Rico. We then described species-specific P-acquisition strategies and explored the changes in fine-root trait expression from 6 months before to 6 months after two consecutive hurricanes, Irma and María, passed over the island. We found that variations in root trait expression were driven mainly by the large interspecific differences across the three selected sites. In addition, we revealed a trade-off between highly colonized fine roots with high phosphatase activity and fine roots that have a high degree of branching. Furthermore, the former strategy was adopted by pioneer species (Spathodea campanulata and Cecropia schreberiana), whereas the latter was adopted by non-pioneer species (mostly Dacryodes excelsa and Prestoea montana). Additionally, we found that root trait expression did not change comparing 6 months before and after the hurricanes, with the exception of root phosphatase activity. Altogether, our results suggest a combination of structural and physiological root traits for soil P acquisition in P-poor tropical soils by common tropical tree species, and show stability on most of the root trait expression after hurricane disturbances.
Introduction
The availability of soil inorganic phosphorus (orthophosphate; hereafter, available P) strongly limits plant growth in lowland tropical forests where climate conditions lead to high soil weathering rates (Walker and Syers, 1976; Vitousek and Sanford, 1986; Reed et al., 2011; Lugli et al., 2021). Tree species that are successful in tropical lowlands have multiple acquisition strategies to overcome P limitation (Zalamea et al., 2016; Lugli et al., 2021). Aboveground, plants may increase P-use efficiency, which in turn leads to higher retention time of P in the canopy and higher resorption efficiency of P as tissues senesce (Paoli et al., 2005; Dalling et al., 2016). Belowground, plants optimize P acquisition by displaying multiple strategies commonly described by the expression of the traits of the narrowest, most absorptive roots (i.e., fine roots) and their mycorrhizal symbionts (Bardgett et al., 2014; Kramer-Walter et al., 2016; Weemstra et al., 2016; McCormack and Iversen, 2019; Bergmann et al., 2020; Lugli et al., 2021). Fine-root and mycorrhizal fungal trait expression is closely related to root function, such as resource foraging and uptake. For example, specific root length (SRL) and root branching are linked to soil space occupancy while root phosphatase activity and mycorrhizal colonization are critical determinants of P uptake (Lee, 1988; McCormack et al., 2017; Freschet et al., 2021).
Belowground, adjustments in morphology, architecture, association with arbuscular mycorrhizal fungi, and phosphatase activity expression may be all viable P-acquisition strategies. First, plants may alter the morphology of their fine roots in such a way as to increase their total absorptive length per unit of biomass (i.e., high SRL) and to decrease their thickness (Treseder and Vitousek, 2001; Santiago, 2015). These morphological adjustments enable plants to maximize resource acquisition while minimizing the cost of root tissue construction and maintenance (Valverde-Barrantes et al., 2015; Weemstra et al., 2016; McCormack and Iversen, 2019). Within the conceptual framework of the root economic space, this strategy is named the “do-it-yourself” strategy (Bergmann et al., 2020), which associates a competitive advantage with exploration of a larger soil volume and faster P assimilation over species that build shorter, thicker-diameter roots (Hodge, 2004; Kong et al., 2014; Liu et al., 2015). Second, plants that construct short, thick, fine roots may still acquire P effectively by outsourcing to mycorrhizal fungi—also called the “outsourcing” strategy (Comas et al., 2014; Kong et al., 2014; Eissenstat et al., 2015; Liu et al., 2015; Kramer-Walter et al., 2016; Ma et al., 2018; Bergmann et al., 2020). Arbuscular mycorrhizal (AM) fungi associate with most neotropical trees and efficiently forage for P in spaces away from P-depleted rhizospheres via extraradical hyphae. In turn, P is translocated to the plant host within arbuscules and intracellular hyphal coils in exchange for photosynthetically fixed carbon (Smith and Smith, 2011). Third, plants may adjust fine-root physiological traits to overcome soil P-limitation by regulating the exudation of phosphatase (Hinsinger, 2001; Treseder and Vitousek, 2001; Vance et al., 2003; Olander and Vitousek, 2004; Lambers et al., 2006). The mineralization of organic P by both root and microbial phosphatase, such as phosphomonoesterase (PME) is especially important in soils of tropical forests where organic P can comprise 30–60% of soil P (Smith and Read, 2008; Kong et al., 2014; Liu et al., 2015; Cabugao et al., 2017; Lugli et al., 2019).
Research on belowground P-acquisition strategies suggests that plants simultaneously adjust the expression of multiple fine-root traits related to P-acquisition to overcome soil P limitation (Lugli et al., 2019, 2021). Therefore, root morpho-architectural adjustments, association with AM fungi, and phosphatase activity may be complementary strategies as opposed to alternatives (Smith and Read, 2008; Turner, 2008; Nasto et al., 2019), but empirical evidence remains scarce in tropical ecosystems. For example, Lugli et al. (2019) found no relationship between mycorrhizal colonization and fine-root morphological traits at the ecosystem level, but SRL and phosphatase activity were shown to be related (Lugli et al., 2019; Cabugao et al., 2021). However, at the species level, thicker roots exhibited higher AM fungal colonization and more P-mobilizing exudates (Zangaro et al., 2005; Wen et al., 2019). Furthermore, the relationship between fine-root architectural traits (i.e., root branching) and mycorrhizal colonization has received little attention at the species level in the tropics (Kong et al., 2014; Liu et al., 2015). Altogether, the relationships among fine-root morphology, architecture, root phosphatase activity, and microbial associations remain understudied in tropical plant species, and the place of these relationships within the root economic space remains indeterminate (Treseder and Vitousek, 2001; Lugli et al., 2019; Nasto et al., 2019). This partly stems from the paucity of studies that measure “hard” traits, such as root enzymatic activity and mycorrhizal colonization. However, “hard” traits generally strongly relate to root function compared to the commonly measured “soft” traits, such as SRL and root nitrogen concentration (Freschet et al., 2015, 2021). To date, knowledge on the relationships among plant fine-root traits related to P acquisition in lowland tropical forests mostly comes from community or ecosystem level studies (Lugli et al., 2019, 2021; Addo-Danso et al., 2020; Cabugao et al., 2021). Consequently, individual relationships between fine-root morphology, phosphatase activity, and mycorrhizal colonization at the species level are underexplored. Yet, plant species can have a strong influence on root and microbial phosphatase activity (Cabugao et al., 2017; Guilbeault-Mayers et al., 2020) and they are likely to differ widely in their nutrient requirement (Townsend et al., 2007). Furthermore, plant species of different successional status are likely to differ in their fine-root trait expression, which may inform their resource acquisition strategies (Coll et al., 2008; Xiang et al., 2013; Caplan et al., 2019).
Here, we describe belowground P-acquisition strategies of five common tree species that have different successional status (two pioneers and three non-pioneers) growing in the lowland tropical wet forests of Puerto Rico, where residence time of soil labile P is short (Liptzin et al., 2015). For each species, we explored relationships among fine-root traits related to P acquisition (Freschet et al., 2021), such as (i) morphological, architectural, and chemical traits (SRL, diameter, P concentration, branching intensity, and branching ratio) and (ii) physiological and microbial traits (root phosphatase activity and mycorrhizal colonization intensity). Based on results from tropical lowland studies (Lugli et al., 2019) and the conceptual root economic space framework (Bergmann et al., 2020), we hypothesized that: (H1) root phosphatase activity and architectural traits would covary with morphological traits, such that high root phosphatase activity would relate to high root branching ratio or intensity and high SRL, representing complementary P-acquisition strategies (combined strategy); and (H2) mycorrhizal colonization would negatively covary with phosphatase activity, branching ratio or intensity, and SRL, representing a trade-off in P-acquisition strategies. Furthermore, (H3) both strategies would covary with root P concentration assuming that root P is an indicator of plant P status and likely reflects P acquisition (based on our analysis of data in Holdaway et al., 2011; Schreeg et al., 2014; Supplementary Figure 1). Altogether, we expected that the existence of a trade-off between these two strategies would lead tree species to adopt one or the other. Most of the analyses we made in this study focused on fine roots collected 6 months after two consecutive hurricanes passed through Puerto Rico in 2017. We used this unique opportunity to also explore differences in some of the aforementioned root traits before and after the hurricanes for each tree species.
Materials and Methods
Study Site and Sampling
In March 2017, we selected three sites in the Luquillo Experimental Forest (LEF; Figure 1 and Table 1) in Puerto Rico. Two sites were close to El Verde Field Station (EVS and EV1; ca. 550 m a.s.l.), and one site was near the USDA Forest Service Sabana Field Research Station (SB2; 150 m a.s.l.). The EVS site is a mature forest with the greatest concentration of soil organic P of the three sites, EV1 is a secondary forest, and SB2 is a young secondary forest with the greatest concentration of soil available P (Brown et al., 1983; Scatena, 1989; Uriarte and Zimmerman, 2017; Table 1). We sampled fine roots of the most common tree species at these sites including (1) Cecropia schreberiana Miq. (Urticaceae) (native pioneer), (2) Dacryodes excelsa Vahl (Burceraceae), Prestoea montana (Graham) G. Nicholson (Arecaceae) and Calophyllum calaba L. (Calophyllaceae) (native non-pioneers) and (3) Spathodea campanulata P. Beauv. (Bignoniaceae) (non-native pioneer). Only Prestoea occurred at the three sites, Cecropia, and Calophyllum occurred at both EV1 and SB2, while Spathodea only occurred at SB2, and Dacryodes was collected only from EVS. All tree species form symbioses with AM fungi.
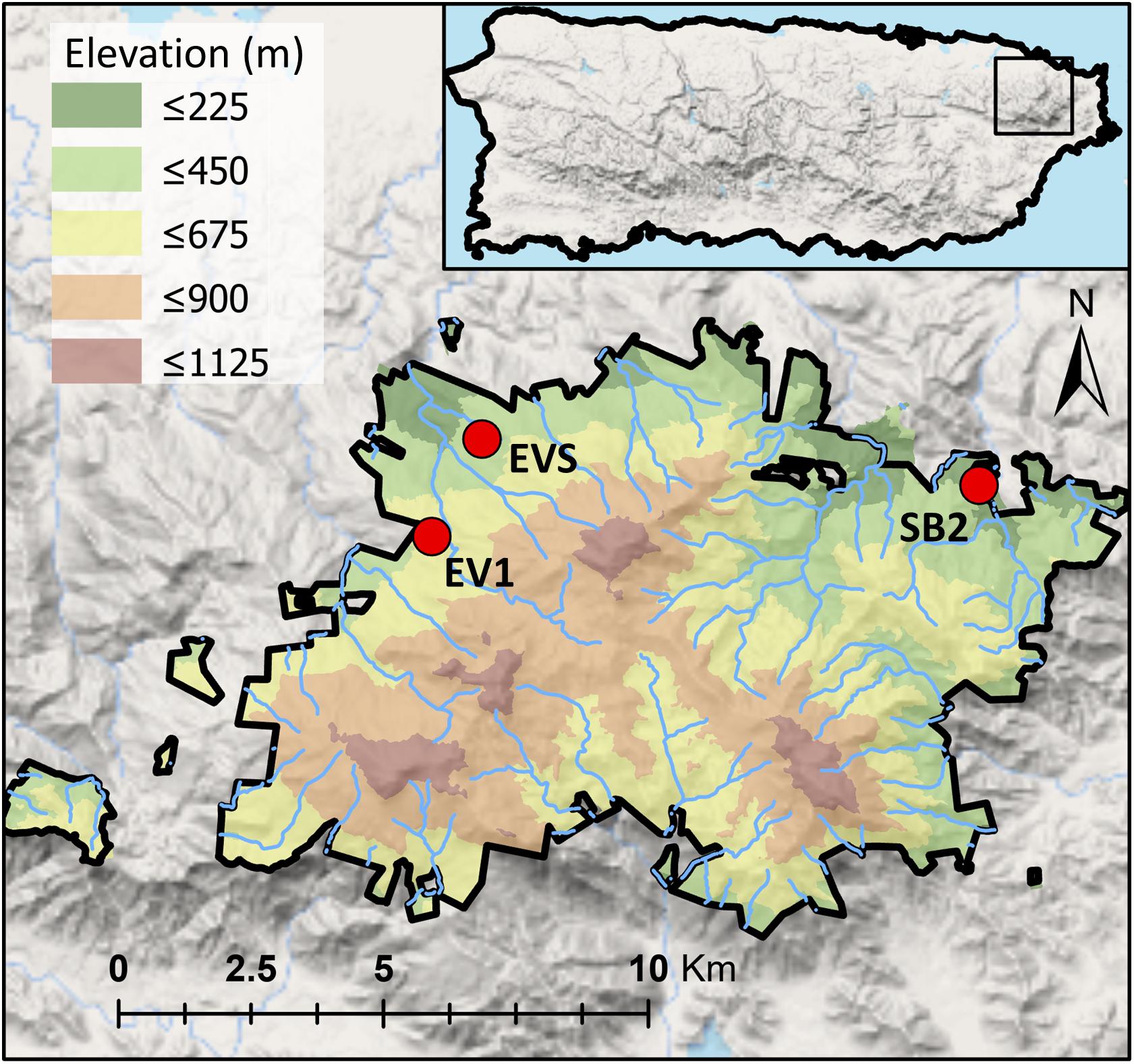
Figure 1. Map of the Luquillo Experimental Forest (LEF)—Puerto Rico, with the location of the studied sited (red circles). Two sites (EV1 and EVS) were located near El Verde Field Station, and one site (SB2) was located near the USDA Forest Service Sabana Field Research Station.
We selected three mature individuals (>10 cm of DBH) from each species that were at least 10 m away from each other and collected at least two root samples from each tree (composed of multiple fine-root branches) by tracing out two coarse roots of each tree from the tree bole until reaching the fine-root system (∼ 2 m away from the tree boles). We combined the two samples per tree, and then we used three subsamples for subsequent root analyses: (i) we assessed mycorrhizal colonization at the Center of Applied Tropical Ecology and Conservation of the University of Puerto Rico (samples were kept in 75% ethanol), (ii) we measured phosphatase activity at Oak Ridge National Laboratory (ORNL; Cabugao et al., 2021), and (iii) we conducted morphological and architectural analyses at the USDA Sabana Field Station Puerto Rico; once dried, we used those samples to measure root P concentration at ORNL. Due to sampling restrictions, we did not measure root phosphatase activity for Spathodea, and we did not assess mycorrhizal colonization before the hurricanes; we made all other measurements both before and after the hurricanes (March 2017 and 2018, respectively).
Fine-Root Trait Measurements
We measured seven fine-root traits from which five were directly related to P acquisition according to Freschet et al. (2021): root phosphatase activity, mycorrhizal colonization, SRL, root branching ratio, root branching intensity. We included root diameter as often this trait is related to mycorrhizal colonization (Bergmann et al., 2020), and root P concentration assuming this trait is an indicator of plant P status and reflects P acquisition (based on our analysis of data in Holdaway et al., 2011 and Schreeg et al., 2014; Supplementary Figure 1).
We used the subsample for morphological and architectural trait measurements (ca. five branches in total) from each tree species and separated them by root order following the morphometric classification approach (Pregitzer et al., 2002). We scanned the first two root orders separately using WinRHIZO (version 12, 1,400 dpi, Regent Instruments Inc., Quebec City, Canada) to obtain total root length (cm) and average diameter (mm). We calculated root branching intensity as the number of first-order roots per length of second-order roots and root branching ratio as the number of first-order roots per number of second-order roots. We dried each group of root orders separately at 65°C until samples reached a stable weight, and we calculated SRL by dividing total root length by root dry weight (cm mg–1). We then shipped the dried root samples to ORNL for P concentration measurements (first- and second-order roots were combined). At ORNL, we ground the samples in 50 ml falcon tubes using a Geno/Grinder 2010 (Spex Sampler Prep, Metuchen, NJ, United States) and measured root P concentration (%) using a Lachat BD40 block digestor for the high temperature digestion and a Lachat QuikChem 8000 series for the flow injection analysis (Hach Company, Loveland CO, United States) following methods described by Cabugao et al. (2021).
Mycorrhizal Colonization and Phosphatase Activity
We cleared, stained and de-stained first- and second-order roots previously preserved in ethanol following Kormanik and Mcgraw (1982) to measure AM fungal colonization. Specifically, we cleared fine roots in potassium hydroxide in a water bath (40°C), stained them with acid fuchsin and de-stained them in glycerol. We then recorded the presence of mycorrhizal structures (100 intersections per sample) disregarding the nature of the structures (e.g., hyphae, arbuscules, coils, vesicles) under a compound microscope (200× magnification) following McGonigle et al. (1990).
We measured root phosphatase activity as the phosphomonoesterase (PME) activity from the first and second-order roots using a modified version of the colorimetric para-nitrophenyl (pNP) phosphate assay (Tabatabai and Bremner, 1969; Cabugao et al., 2017; Png et al., 2017). This assay consists of measuring the activity of PME bound to the root surface in the presence of an organic P substrate (para-nitrophenyl phosphate). We washed the root samples (∼1 g) with milliQ water multiple times, mixed them with 9 ml of 50 mM sodium acetate-acetic acid (for 1 L: 2.88 g sodium acetate; pH = 5.0 using acetic acid) and 1 ml of 50 mM pNP (1.856 g pNP in 100 ml sodium acetate buffer), and incubated in a shaker at 27°C for 1 h. We then took 0.5 ml of this solution, added 4.5 ml of 0.11 M NaOH to terminate the reaction and measured the concentration of the product (para-nitrophenol) by spectrophotometry (405 nm, spectrophotometer 110, Cole Parmer, East Banker Court Vernon Hills, Illinois). Standard curves were made using 0, 100, 200, 400, 1,000 μM of pNP. PME activity is expressed as μmol pNP groot–1 hr–1.
Hurricane Events
Most of the analyses we made in this study focused on fine roots collected 6 months after two consecutive hurricanes passed through Puerto Rico. However, we had also collected roots samples 6 months before the hurricanes Irma on September 6th, 2017 (category 5) and María 2 weeks after, on September 20th 2017 (category 4). Sustained winds reached 250 km hr–1 (among the strongest on record for the island; Lugo, 2020) and precipitation over 500 mm in just 24 h from María (Pasch et al., 2019). The hurricanes had a strong effect on the forest structure, which was still evident when we performed sample collection 6 months following the hurricanes. Tree mortality as a consequence of the disturbance was high (∼15%; Uriarte et al., 2019), and it included two trees we previously measured, which we replaced by a nearby individual of the same species and similar DBH. There was a large reduction in canopy cover (from ∼90 to 30%), and soil available P concentration decreased at EV1 and SB2, while organic P concentration decreased only at SB2 (Table 1).
Data Analysis
We conducted all statistical analyses in R version 3.3.4 (R Core Team, 2019) and considered statistically significant results at P ≤ 0.05. To test for outliers in the data, we used the check_outliers function from the “performance” package in R (Lüdecke et al., 2020), and dropped two datapoints from all the analysis that were statistical outliers and ecologically improbable based on the literature (FRED 3.0; Iversen et al., 2018). To test for changes in root trait expression among species and sites separately we performed ANOVAs followed by post-hoc HSD Tukey’s tests (Chambers et al., 2017). For multiple testing, we corrected P-values with the Bonferroni correction (McDonald, 2014). All mycorrhizal colonization data were transformed to percentages. To normalize the distribution of the mycorrhizal colonization percentage, we used a logit transformation (gtools package, Fox and Weisberg, 2019). To visualize dimensions in root trait variation among species and sites, we used Non-metric Multi-Dimensional Scaling (Kruskal, 1964). To explore bivariate relationships among root traits (for example, the effect of mycorrhizal colonization on root phosphatase activity) we used linear mixed effects models with the lmer function (lme4 package, Bates et al., 2015) considering site, and species within sites as nested random intercepts as two separate models (see Supplementary Table 2), and reported the one with only site in the results. To test for models’ assumptions, we used the check_model function from the “performance” package in R, based on these results we log transformed the data from root phosphatase activity. Confidence intervals for the model parameters were estimated using the confint function (stats package; Ponciano et al., 2009; R Core Team, 2019). To test for differences in root trait expression and soil P concentration before and after the hurricanes we also fitted a linear mixed effect model where tree within species within sites was the nested random intercept. We did not consider mycorrhizal colonization in this last analysis due to sample restrictions before the hurricanes. We used the estimated marginal means using the emmeans function from the emmeans package to compare traits expression before and after the hurricane (Lenth et al., 2020).
Results
Inter- and Intraspecific Root Trait Variation
Most fine-root traits we measured had greater variation among species within sites (interspecific mean variation, CV = 62.4%) than within species among sites (intraspecific mean variation, CV = 40.5%; Supplementary Table 1). However, root phosphatase activity and branching ratio varied in only one species among sites, while root P concentration did not differ among species (Supplementary Table 1). Overall, root trait expression did not vary among sites (Figure 2A), whereas root branching ratio, phosphatase activity and mycorrhizal colonization explained most of the variation (>50%) among species (Figure 2B).
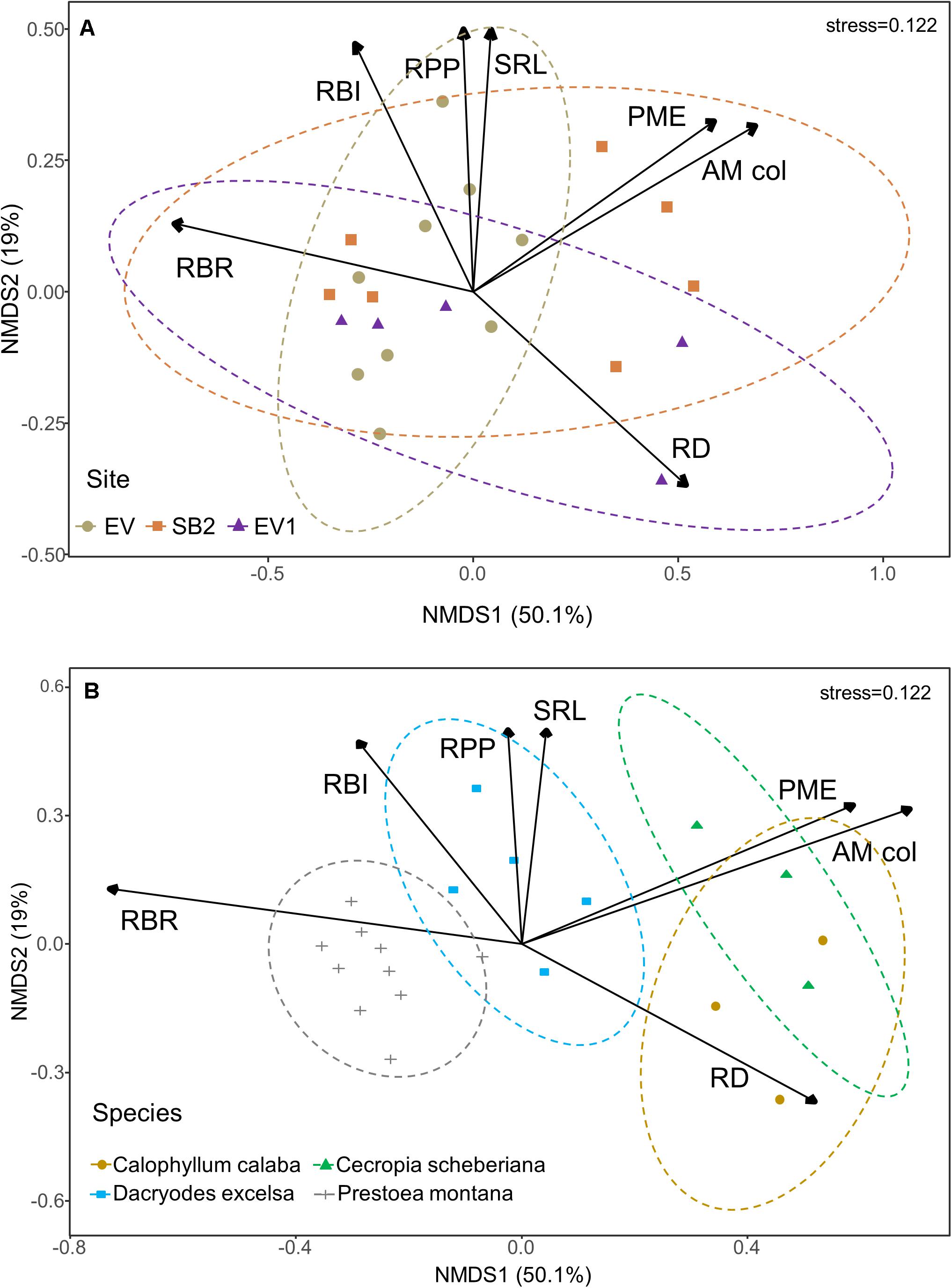
Figure 2. Functional root traits of the first two fine-root orders of five common tree species at the Luquillo Experimental Forest in Puerto Rico. Non-Metric Multi-Dimensional Scaling (NMDS) of the fine-root traits: root branching ratio (RBR), root branching intensity (RBI), specific root length (SRL), root phosphatase activity (phosphomonoesterase; PME), root diameter (RD), root phosphorus concentration (RPP), and arbuscular mycorrhizal colonization (AM col). The NMDS plots are grouped by (A) Sites and (B) Species. Data are shown after the hurricanes that passed over the island in 2017. Due to sampling constraints, root PME activity was not measured for Spathodea campanulata.
Calophyllum displayed the thickest first-order root diameter (0.46 mm) that had the lowest values of SRL (0.79 cm mg–1; Figures 3A,B) while the pioneers Cecropia and Spathodea had the greatest SRL values (10.3 and 10.6 cm mg–1, respectively) and the highest percentage mycorrhizal colonization (70 and 75%, respectively; Figure 3E). In addition, fine roots of Cecropia had the highest phosphatase activity (272.9 μmol pNP groot–1hr–1; Figure 3F), while those of Spathodea had the highest P concentration values (0.1%; Figure 3G), although differences among species for root P concentration were not statistically significant. Lastly, Prestoea built highly branched fine roots (branching ratio of 5.9; Figure 3C) that had the smallest diameter (first-order root measured 0.2 mm), the lowest activity of phosphatase (16.4 μmol pNP groot–1hr–1) and lowest mycorrhizal colonization (12%). Dacryodes had the highest root branching intensity (Figure 3D).
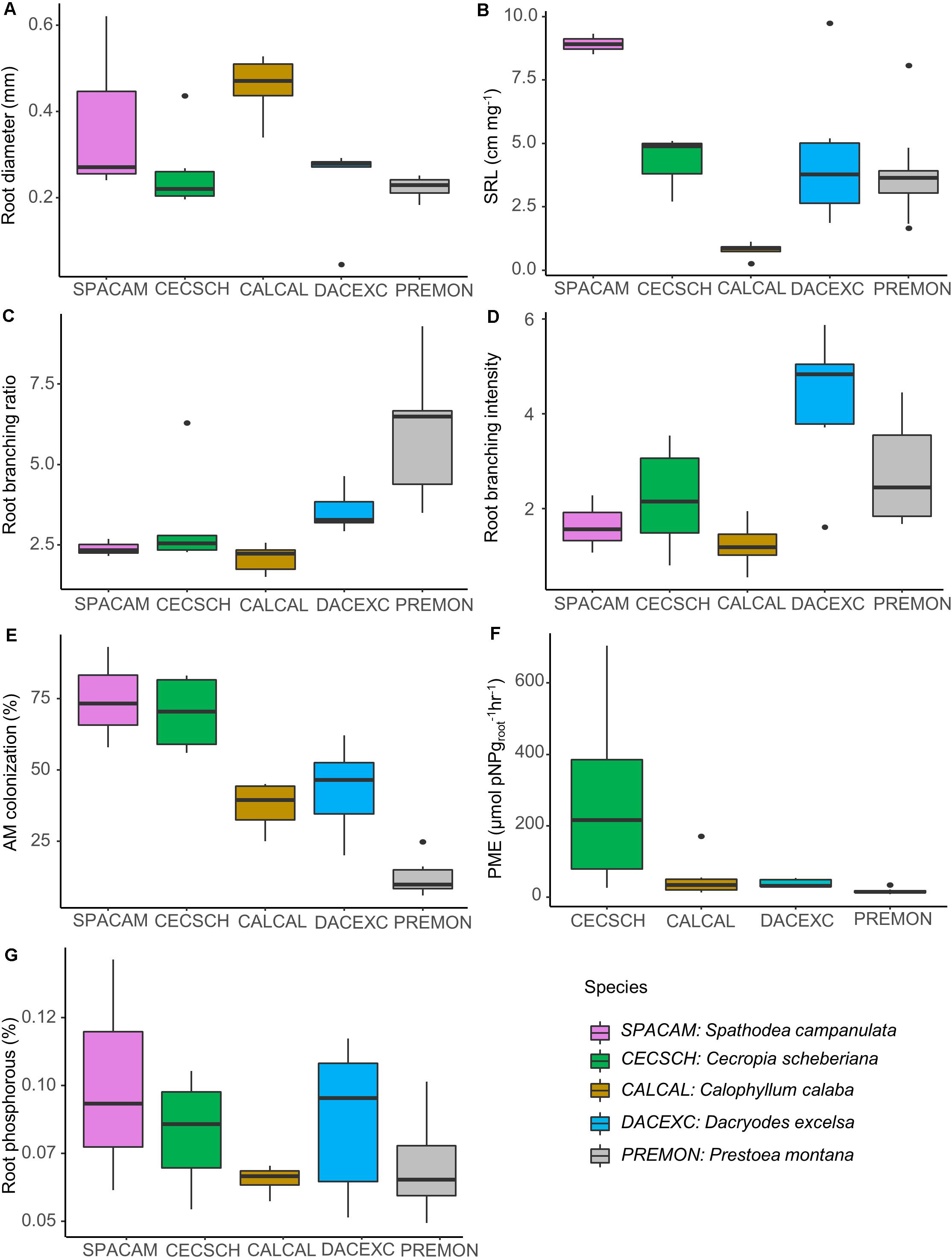
Figure 3. Fine-root trait values for five common trees species at the Luquillo Experimental Forest in Puerto Rico. (A) Root diameter and (B) specific root length (SRL) were measured from 1st root order. (C) Root branching ratio, (D) root branching intensity, (E) arbuscular mycorrhizal (AM) colonization, (F) root phosphatase activity (phosphomonoesterase; PME), and (G) root phosphorus concentration were measured from 1st and 2nd orders combined. Data are shown after the hurricanes that passed over the island in 2017. Due to sampling constraints, root PME activity was not measured for Spathodea campanulata.
Bivariate Relationships Among Fine-Root Traits
Bivariate relationships between fine-root traits were driven by interspecific variations (Supplementary Table 2 and Figure 4). Mycorrhizal colonization covaried with root phosphatase activity such that root phosphatase activity increased linearly by 3.06 μmol pNP groot–1hr–1 for every percent increase in mycorrhizal colonization (p < 0.01; R2m = 0.43; Supplementary Table 2 and Figure 4A). Conversely, root phosphatase activity decreased by 21.2 μmol pNP groot–1hr–1 for every number of first-order root per number of second-order root increase in branching ratio (p = 0.02; R2m = 0.17; Supplementary Table 2 and Figure 4B). Mycorrhizal colonization was also negatively related to root branching ratio, decreasing by 6.5% for every number of first-order root per number of second-order root increase in branching ratio (p = 0.04; R2m = 0.25; Supplementary Table 2 and Figure 4C), and positively related to SRL, increasing by 3.4% for every 1 cm mg–1 increase (p = 0.02; R2m = 0.19; Supplementary Table 2 and Figure 4D). Furthermore, root diameter, SRL, root P concentration, and root branching intensity were not related to root phosphatase activity (Supplementary Figures 2A–D and Supplementary Table 2), nor were root branching intensity and root diameter related to mycorrhizal colonization (Supplementary Figures 2E,F and Supplementary Table 2). Root P concentration was positively related to mycorrhizal colonization (p = 0.05; R2m = 0.14; Supplementary Table 2 and Figure 4E), root branching intensity (p = 0.02; R2m = 0.12; Supplementary Table 2 and Figure 4F), and soil available P (Supplementary Table 2). No other root measurement was related to soil available P (not shown).
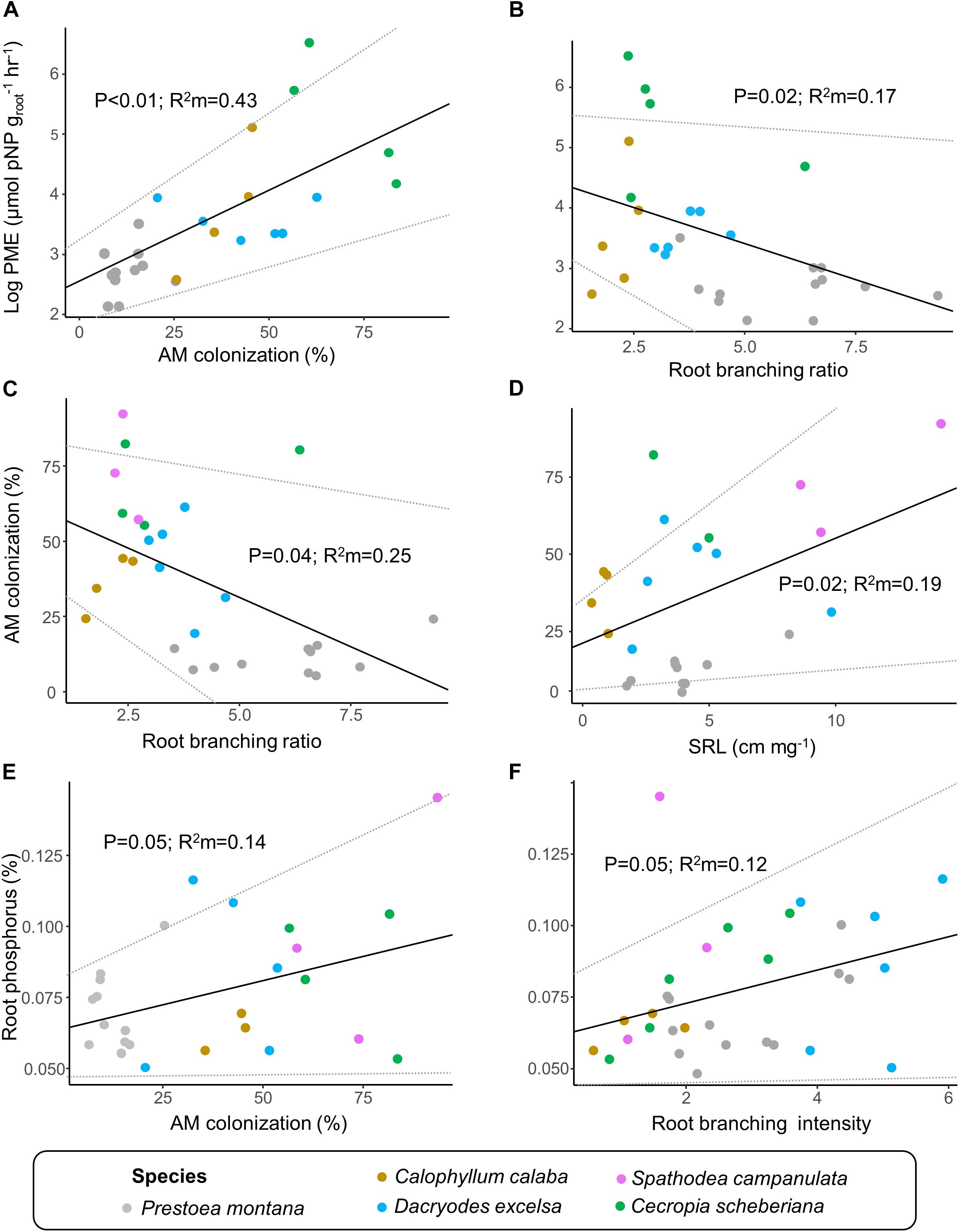
Figure 4. Bivariate significant relationships among the seven fine-root traits measured in this study. Linear mixed-effects models were fitted for each root trait combination to account for the hierarchical nature of our dataset (see Supplementary Table 2). We report the marginal R2 of each model which represents the variance explained by the fixed effect. The dotted gray lines represent the confidence interval for a given model parameter. The relationships include root phosphatase activity (phosphomonoesterase; PME) with (A) arbuscular mycorrhizal (AM) colonization and with (B) root branching ratio; AM colonization with (C) root branching ratio and with (D) specific root length (SRL); and root phosphorus concentration with (E) AM colonization and with (F) root branching intensity. Data are shown after the hurricanes that passed over the island in 2017. Due to sampling constraints, root PME activity was not measured for Spathodea campanulata.
Fine-Root Measurement Before vs. After the Hurricanes
After two consecutive hurricanes in September 2017, most of the tree species did not display changes in morphological, architectural, or chemical root trait expression (Figures 5A–D,F). For instance, the estimated marginal mean of SRL was 4.72 cm mg–1 pre-hurricanes and 4.78 cm mg–1 post-hurricanes. We found a strong decrease in the activity of root phosphatase overall (Figure 5E) (when we fitted a model with trees nested within species within sites; Supplementary Table 3). Estimated marginal means for root phosphatase activity were 220.0 μmol pNPgroot–1hr–1 pre-hurricanes and 76.9 μmol pNPgroot–1hr–1 post-hurricanes.
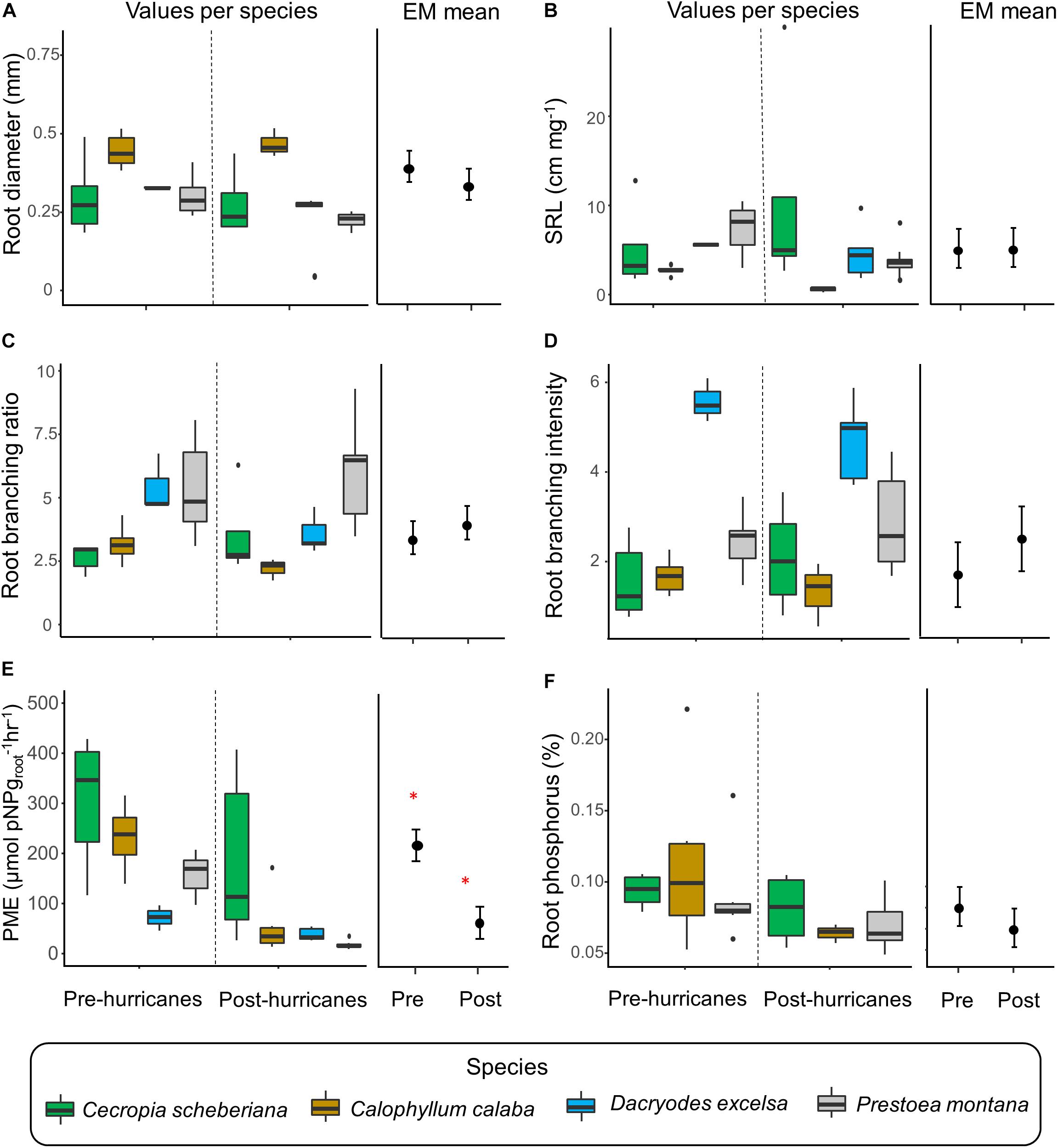
Figure 5. Fine-root trait values for four common trees species at the Luquillo Experimental Forest in Puerto Rico before and after two consecutive hurricanes (boxplots on the left of each panel). We fitted linear mixed-effects models with tree within species within sites as a nested random effect and report the estimated marginal means (EM means; right plot of each panel) and standard error of the means for each root trait. (A) Root diameter and (B) specific root length (SRL) were measured from 1st root order. (C) Root branching ratio, (D) root branching intensity, (E) root phosphatase activity (phosphomonoesterase; PME), and (F) root phosphorus concentration were measured from 1st and 2nd orders combined. Significant differences in (mean) root trait values before and after the hurricanes are marked with a red asterisk. Due to sampling restriction, root phosphatase activity (phosphomonoesterase; PME) was not measured for Spathodea campanulata and root phosphorus concentration was not estimated for Dacryodes excelsa.
Discussion
Tropical forests are highly productive, comprising one fourth of the planet’s terrestrial carbon stored in aboveground vegetation biomass (Bonan, 2008). This high productivity might be modulated by feedbacks between plant functional traits and the P cycle (Quesada et al., 2010; Cernusak et al., 2013). However, the role of belowground plant functional traits in soil P acquisition remains unclear, in part because knowledge on the relationships among fine-root traits and potential trait trade-offs is limited (Freschet et al., 2021). Here, we explored relationships among a suite of fine-root traits related to P acquisition in the P-poor soils of a lowland tropical forest of Puerto Rico. Variations in root trait expression of the five tree species we studied were mainly driven by the large interspecific differences across the three selected sites. Our results contradict our hypotheses of a trade-off in phosphorus acquisition strategies between (H1) fine roots with high phosphatase activity, high SRL, and high root branching, and (H2) fine roots with greater mycorrhizal colonization. In contrast, we reveal a trade-off between highly colonized fine roots with high phosphatase activity and fine roots that have a high degree of branching. Furthermore, the former strategy was adopted by the pioneer trees (Spathodea and Cecropia) whereas the latter was adopted by the non-pioneer trees (mostly Dacryodes and Prestoea). Our results confirmed that both high root branching and high mycorrhizal colonization are two contrasting strategies that covaried with root P concentration (H3). Additionally, we found that none of the root traits except phosphatase activity changed when comparing 6 months before and after the hurricanes. Our study, although encompassing only five tree species, highlights the need to combine measurements of fine-root morphology, architecture, physiology, and mycorrhizal colonization to better define belowground plant P-acquisition strategies in the tropics.
Large Interspecific Fine-Root Trait Variation
Interspecific root trait variation was high among the five tree species selected and for most of the traits. Interspecific fine-root trait variation has been widely explored at the global and biome scales, and some traits are phylogenetically constrained, such as SRL and root diameter (Ryser and Eek, 2000; Kong et al., 2014; Ma et al., 2018; Wang R. et al., 2018; Liu et al., 2019; Valverde-Barrantes et al., 2020). Nonetheless, the expression of other functional traits, such as mycorrhizal colonization and root branching ratio could vary greatly with environmental conditions (Kong et al., 2014; Weemstra et al., 2016). Accordingly, we found that root branching ratio and phosphatase activity varied between sites and within species (Supplementary Table 1), which confirms our previous findings in Cabugao et al. (2017). However, the percent of mycorrhizal colonization was similar within species present in at least two of our three sites (Supplementary Table 1). Similarly, site differences in environmental conditions did not appear to drive variation in AM hyphal length in tropical forests of Costa Rica, Panama, Peru, and Brazil (Powers et al., 2005). In addition, some studies suggest that AM fungi may colonize root cortical tissue independently of soil P availability, due to a lack of efficient plant mechanisms to control mycorrhizal colonization (Johnson, 2010; Valverde-Barrantes et al., 2016).
We found that the pioneers Cecropia and Spathodea had greater mycorrhizal colonization than non-pioneers. This has also been shown in some studies from Brazil where AM colonization decreased as species composition changed during forest succession (Zangaro et al., 2008, 2012). However, other studies from Puerto Rico showed that pioneer plant species were more independent of their fungal mycorrhizal partners than non-pioneers (Myster et al., 2013; Bachelot and Lee, 2018). These apparent contradictions represent a genuine challenge in the rhizosphere ecology. Although broader studies are needed in tropical ecosystems, we can attribute some of the differences between our study and others to the selection of tree species, sample size, and tree age, as most other studies estimated mycorrhizal colonization on seedlings, whereas we sampled mature trees.
No Trade-Off Between Fine-Root Physiology and Mycorrhizal Colonization
We hypothesized that high root phosphatase activity and adjustments in architectural and morphological traits would represent a combined strategy for P acquisition (H1), which would be in contrast with a strategy of high mycorrhizal colonization (H2). In theory, both strategies represent a significant cost to the plants, which can either invest in P-mineralizing enzymes, high length per unit biomass, and high number of first-order roots or in mycorrhizal symbionts (Treseder and Vitousek, 2001; Liu et al., 2015; Nasto et al., 2017, 2019; Cabugao et al., 2021). In addition, root phosphatase activity has been shown to relate positively to SRL at the community level within the LEF (Cabugao et al., 2021), which implies that root phosphatase activity would be part of the “do-it-yourself” end of the collaboration gradient, opposite from mycorrhizal colonization (Bergmann et al., 2020). However, we partially rejected both hypotheses as no relationships were detected between root phosphatase activity and branching intensity or SRL (Supplementary Figure 2) except for the negative relationship between phosphatase activity and branching ratio, and as mycorrhizal colonization and root phosphatase activity were positively related (when considering site variation only). This suggests that enzyme exudation and mycorrhizal colonization are not contrasting strategies for the five species considered at the LEF. This could partly be attributed to the large interspecific variations in root trait expression because the relationship between mycorrhizal colonization and phosphatase activity was not statistically significant when considering species within sites as a random effect in the models (Supplementary Table 2). In addition, our results suggest that in our five tree species, both fine roots and AM fungi may have contributed to phosphatase activity given that 43% of the root phosphatase activity variation was explained by the mycorrhizal colonization in our model (Figure 4A). Mycorrhizal fungi, such as AM fungi are capable of exuding phosphatase, yet their ability to hydrolyze organic P is still controversial (Koide and Kabir, 2000; Smith and Read, 2008). This points out the additional work needed to understand the contribution of AM fungi to root phosphatase activity.
The percent of root colonization by AM fungi was weakly positively related to SRL of first-order roots (19% of variation explained; Figure 4D), contradicting the root economic space framework in which mycorrhizal colonization is expected to co-vary positively with root diameter and thus, negatively with SRL (Brundrett, 2002; Kong et al., 2014; Bergmann et al., 2020). However, another study in a tropical forest of Brazil found a positive relationship between mycorrhizal colonization and SRL (Zangaro et al., 2008). Furthermore, SRL observations across a world-wide range of species have also shown that plants can construct roots with many combinations of morphological traits (Kramer-Walter et al., 2016; Weemstra et al., 2016; Bergmann et al., 2020) and that mycorrhizal colonization is highly dependent on tree developmental stage and the anatomy of the fine root (i.e., cortex cell properties; Brundrett, 2002; Bachelot et al., 2017). Thus, the relationship among these traits is complex and depends on different species-specific factors. When fitting models with species within sites as a random effect, SRL and mycorrhizal colonization were no longer positively correlated; in contrast mycorrhizal colonization appeared to be positively related to root diameter, as predicted by the root economic space framework (Supplementary Table 2). This highlights that root trait trade-offs at the species level inform species-specific resource acquisition strategies that may differ from patterns at the global scale (Freschet et al., 2021).
Trade-Offs Between Mycorrhizae, Root Branching, and Phosphatase Activity
Fine-root branching ratio and colonization by AM fungi were negatively related, such that species exhibiting high levels of colonization had a lower number of first-order roots per number of second-order roots (less branching). This partially supports our hypotheses (H1 and H2) as root branching and mycorrhizal colonization represent different strategies for soil P acquisition. Both AM fungi and root branching greatly contribute to soil P foraging (Treseder, 2013; Liu et al., 2018; Wang Y. et al., 2018), yet plants must balance their investment in these P strategies due to their significant costs (Eissenstat et al., 2015). Moreover, as hypothesized (H3), both traits representing contrasting strategies were weakly positively related to root P concentration, which is a good indicator of plant P status (and this correlation holds even when including site and species within sites as random effects in the models). Although we have no direct measure of P acquisition (e.g., instantaneous P uptake rate by roots or annual whole-plant P increment), root P concentration potentially reflects P acquisition due to its strong correlation to both leaf P concentration (Holdaway et al., 2011) and soil inorganic and total P (Holdaway et al., 2011; Schreeg et al., 2014; Freschet et al., 2021; Supplementary Figure 1A) which we also found in this study (Supplementary Figure 1B). Finally root branching was weakly negatively related to phosphatase activity (17% of variation explained; Figure 4B), probably due to the possible combined phosphatase activity from roots and their fungal partners.
P-Acquisition Strategies Vary Across Species
We considered two pioneer species Spathodea campanulata and Cecropia schreberiana that generally display acquisitive leaf traits (e.g., high leaf nitrogen concentration; Lugo and Abelleira Martínez, 2018), and three non-pioneer species Calophyllum calaba, Dacryodes excelsa, and Prestoea montana that display conservative leaf traits (e.g., high specific leaf area; Harris and Medina, 2013; Figure 6). These differences in plant leaf traits for resource acquisition and resource conservation typically divide fast-growing pioneer species from slow-growing non-pioneer species (Grime, 1977; Wright et al., 2004; Weemstra et al., 2016). Similarly, some studies also found important root trait differences across different plant successional status (Coll et al., 2008; Zangaro et al., 2012; Xiang et al., 2013; Caplan et al., 2019). Accordingly, we found that fine-root architectural traits, phosphatase activity, and mycorrhizal colonization are all important in separating pioneers from non-pioneer tree species, but there was no equivalent pattern for morphological traits (Figure 6).
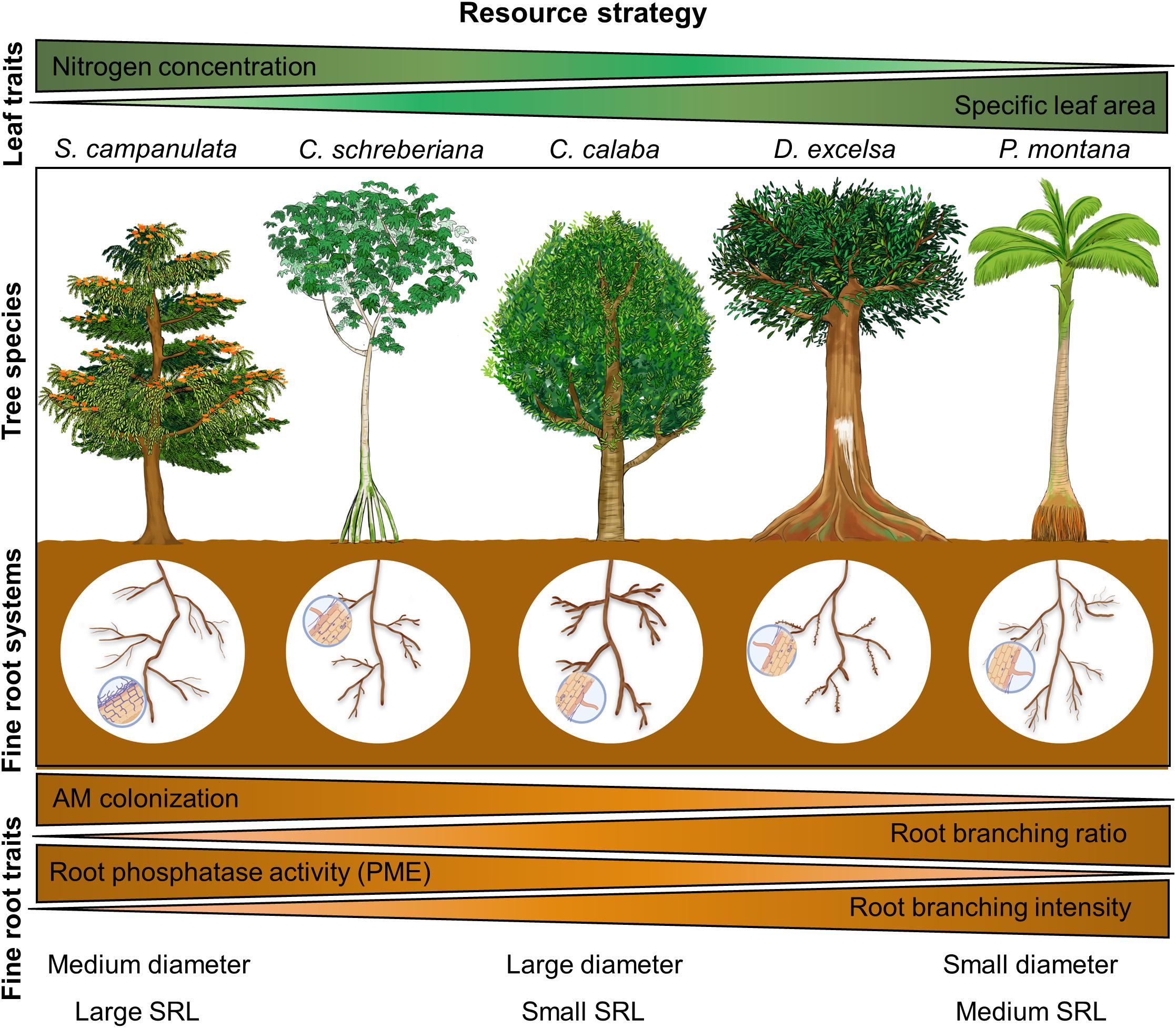
Figure 6. Conceptual diagram of belowground phosphorus-acquisition strategies of five tropical tree species from Puerto Rico. Our results highlight a trade-off between highly colonized fine roots with high phosphatase activity and fine roots that have a high degree of branching. Furthermore, the former strategy is adopted by pioneer species (Spathodea campanulata and Cecropia schreberiana), whereas the latter is adopted by non-pioneer species (mostly Dacryodes excelsa and Prestoea montana). Root phosphorus concentration was not included here because no clear pattern was found across species.
Both fast-growing pioneers, Spathodea and Cecropia, had greater mycorrhizal colonization, and Cecropia had the highest phosphatase activity compared to the other species studied. Investing carbon in fungal partners to outsource nutrient acquisition could benefit plants with higher P nutrient demand, and therefore enable faster growth (Wright et al., 2004; Freschet et al., 2021), which appears to support both the collaboration and the conservation gradient framework (Bergmann et al., 2020). The combined above- and belowground P-acquisition strategies from these pioneer species might partially explain their large dominant presence in Puerto Rico (Brandeis et al., 2003), and may help them thrive in gaps formed from natural and anthropogenic disturbances (i.e., hurricanes; for Cecropia) and in degraded soils (for Spathodea; Aide et al., 2000; Francis, 2000; Lugo et al., 2011). In contrast, the slow-growing non-pioneers, Dacryodes and Prestoea, showed greater root branching ratio and intensity compared to the other species, which represents a greater carbon investment in root construction for nutrient exploration (Hodge, 2004; Liese et al., 2017; Figure 6). Because root branching is considered a leading root trait due to its plasticity and relationship with mycorrhizal association type and resource exploitation (Kong et al., 2014; Liese et al., 2017; Freschet et al., 2021), the high values of branching ratio and intensity might play an important role in the large dominant presence of Dacryodes and Prestoea in the mature wet forests of the LEF (Aide et al., 2000; Francis, 2000). Further, although Calophyllum is a non-pioneer species, it had low values of root branching, compared to the other non-pioneer species, and low values of phosphatase activity, and mycorrhizal colonization compared to the pioneer species (Figure 6). This species’ distribution is mostly attributed to its use for reforestation on degraded soils (Francis, 2000), and its soil P-acquisition strategy might be related to other root trait syndromes that we have not considered in this study.
Stability of Root Trait Expression Before vs. After the Hurricanes
We showed no apparent changes in most root trait expression 6 months after hurricanes Irma and María impacted the forest compared to their pre-hurricane values. Root phosphatase activity, however, was significantly different after the hurricanes. This difference may have reflected normal seasonal environmental changes (Sardans et al., 2007), but it is likely that there also were effects of the numerous changes in forest structure, soil biogeochemistry, and environmental conditions created by the hurricanes. These influences could have included changes in soil available P (Dakora and Phillips, 2002; Burns et al., 2013; Dalling et al., 2016; Margalef et al., 2017). However, although we expected that the decrease in root phosphatase activity could have been triggered by an increase in soil available P, we in fact measured a decrease in soil available P in our sites (Table 1). Reed et al. (2020) also reported an initial decrease in soil available P (first 3 months after Hurricane María), but soon after, they measured a sharp increase. Differences in methods assessing soil available P can account for important differences in P-values (Neyroud and Lischer, 2003), which represents a challenge and limitation in tropical forest ecology. Nevertheless, other potential factors influencing changes in root phosphatase activity could have been related to changes in soil moisture (SB2 was flooded for several months), a decrease of plant demand for P because of extensive defoliation, or changes in competition from understory vegetation abundance (Kennard et al., 2020; Reed et al., 2020). We cannot separate these different possible influences but note that the responsiveness of root phosphatase to environmental change may be an important consideration in evaluating phosphorus nutrition of tropical forests.
Conclusion
To overcome soil P limitation in lowland tropical forests, plants employ multiple strategies belowground, such as increasing SRL, root branching, root phosphatase activity and their reliance on AM fungi. However, relationships and potential trade-offs among these strategies are poorly understood. This limits our ability to predict potential feedbacks between plant traits and the P cycle in these ecosystems. Here, we reveal a trade-off between highly colonized fine roots with high root phosphatase activity and fine roots that have a high degree of branching, using five tree species growing in Puerto Rico. Tree successional status was a good predictor of belowground P-acquisition strategies as pioneers invested resources in mycorrhizal fungi and root phosphatase activity, whereas non-pioneers increased fine-root branching. In addition, most root traits before and after two consecutive hurricanes did not show significant changes in the species we studied. Root phosphatase activity, however, did vary when comparing pre- and post-hurricanes, and the causation of this variation might be relevant to future studies. Finally, expanding studies on belowground traits in the tropics to include “hard” functional traits, such as phosphatase activity should help to predict plant responses to natural disturbances and aid in the simulation of trait diversity and coexistence under resource limitation in tropical forests.
Data Availability Statement
The data used in this study are publicly available at the NGEE Tropics repository under the doi: 10.15486/ngt/1778242.
Author Contributions
DY developed the concept for the project, took the field and laboratory measurements, analyzed the data, and wrote the manuscript. CD provided essential insight to the concept of the project and to writing the manuscript. KGC helped to developed the concept for the project, took field and laboratory measurements, and reviewed the manuscript. SNK assisted in data analysis, reviewed, and edited the manuscript. JC and NC participated in the field and laboratory measurements and reviewed the manuscript. RJN developed the concept for the project, helped with the field and laboratory measurements, helped in data analysis, and reviewed and edited the manuscript. All authors contributed to the article and approved the submitted version.
Funding
This research was supported by the Next Generation Ecosystem Experiments-Tropics, funded by the United States Department of Energy, Office of Science, Office of Biological and Environmental Research. Oak Ridge National Laboratory is managed by UT-Battelle, LLC, for the United States Department of Energy under contract DE-AC05–00OR22725.
Conflict of Interest
The authors declare that the research was conducted in the absence of any commercial or financial relationships that could be construed as a potential conflict of interest.
Acknowledgments
We acknowledge Joshua Price, Benjamin Branoff, and Lalasia Bialic-Murphy for the statistical and graphical guidance in the analysis; Ariel Lugo, Colleen Iversen, Elvira Cuevas, Jean Lodge, and Tana Wood for their essential insights and equipment; Deanne Brice, Nathan Stenson, and Holly Vander Stel for the help in the field and in the laboratory; and Gabriela Moncada for the tree designs.
Supplementary Material
The Supplementary Material for this article can be found online at: https://www.frontiersin.org/articles/10.3389/ffgc.2021.698191/full#supplementary-material
References
Addo-Danso, S. D., Defrenne, C. E., McCormack, M. L., Ostonen, I., Addo-Danso, A., Foli, E. G., et al. (2020). Fine-root morphological trait variation in tropical forest ecosystems: an evidence synthesis. Plant Ecol. 221, 1–13. doi: 10.1007/s11258-019-00986-1
Aide, M. T., Zimmerman, J. K., Pascarella, J. B., Rivera, L., and Marcano-Vega, H. (2000). Forest regeneration in a chronosequence of tropical abandoned pastures: implications for restoration ecology. Restor. Ecol. 8, 328–338. doi: 10.1046/j.1526-100X.2000.80048.x
Bachelot, B., and Lee, C. T. (2018). Dynamic preferential allocation to arbuscular mycorrhizal fungi explains fungal succession and coexistence. Ecology 99, 372–384. doi: 10.1002/ecy.2080
Bachelot, B., Uriarte, M., McGuire, K. L., Thompson, J., and Zimmerman, J. (2017). Arbuscular mycorrhizal fungal diversity and natural enemies promote coexistence of tropical tree species. Ecology 98, 712–720. doi: 10.1002/ecy.1683
Bardgett, R. D., Mommer, L., and De Vries, F. T. (2014). Going underground: Root traits as drivers of ecosystem processes. Trends Ecol. Evol. 29, 692–699. doi: 10.1016/j.tree.2014.10.006
Bates, D., Mächler, M., Bolker, B. M., and Walker, S. C. (2015). Fitting linear mixed-effects models using lme4. J. Stat. Softw. 67, 1–48. doi: 10.18637/jss.v067.i01
Bergmann, J., Weigelt, A., Van Der Plas, F., Laughlin, D. C., Kuyper, T. W., Guerrero-Ramirez, N., et al. (2020). The fungal collaboration gradient dominates the root economics space in plants. Sci. Adv. 6:27. doi: 10.1126/sciadv.aba3756
Bonan, G. B. (2008). Forests and climate change: forcings, feedbacks, and the climate benefits of forests. Science 320, 1444–1449. doi: 10.1126/science.1155121
Brandeis, T. J., Helmer, E. H., and Oswalt, S. N. (2003). The Status of Puerto Rico’s Forests, 2003. Resource Bulletin SRS-119. Asheville, NC: U.S. Department of Agriculture Forest Service.
Brown, S., Lugo, A. E., Silander, S., and Liegel, L. (1983). Research History and Opportunities in the Luquillo Experimental Forest. General Technical Report SO-44. New Orleans, LA: U.S. Dept of Agriculture, Forest Service, doi: 10.2737/SO-GTR-44
Brundrett, M. C. (2002). Coevolution of roots and mycorrhizas of land plants. New Phytol. 154, 275–304. doi: 10.1046/j.1469-8137.2002.00397.x
Burns, R. G., DeForest, J. L., Marxsen, J., Sinsabaugh, R. L., Stromberger, M. E., Wallenstein, M. D., et al. (2013). Soil enzymes in a changing environment: current knowledge and future directions. Soil Biol. Biochem. 58, 216–234. doi: 10.1016/j.soilbio.2012.11.009
Cabugao, K. G., Timm, C. M., Carrell, A. A., Childs, J., Lu, T. Y. S., Pelletier, D. A., et al. (2017). Root and rhizosphere bacterial phosphatase activity varies with tree species and soil phosphorus availability in puerto rico tropical forest. Front. Plant Sci. 8:1834. doi: 10.3389/fpls.2017.01834
Cabugao, K. G., Yaffar, D., Stenson, N., Childs, J., Phillips, J., Mayes, M. A., et al. (2021). Bringing function to structure: root–soil interactions shaping phosphatase activity throughout a soil profile in Puerto Rico. Ecol. Evol. 11, 1150–1164. doi: 10.1002/ece3.7036
Caplan, J. S., Meiners, S. J., Flores-Moreno, H., and McCormack, M. L. (2019). Fine-root traits are linked to species dynamics in a successional plant community. Ecology 100:e02588. doi: 10.1002/ecy.2588
Cernusak, L. A., Winter, K., Dalling, J. W., Holtum, J. A. M., Jaramillo, C., Körner, C., et al. (2013). Tropical forest responses to increasing atmospheric CO2: current knowledge and opportunities for future research. Funct. Plant Biol. 40, 531–551. doi: 10.1071/FP12309
Chambers, J. M., Freeny, A. E., and Heiberger, R. M. (2017). “Analysis of variance; designed experiments,” in Statistical Models in S, eds J. M. Chambers and T. J. Hastie (Boca Raton, FL: Routledge), doi: 10.1201/9780203738535
Coll, L., Potvin, C., Messier, C., and Delagrange, S. (2008). Root architecture and allocation patterns of eight native tropical species with different successional status used in open-grown mixed plantations in Panama. Trees Struct. Funct. 22:585. doi: 10.1007/s00468-008-0219-6
Comas, L. H., Callahan, H. S., and Midford, P. E. (2014). Patterns in root traits of woody species hosting arbuscular and ectomycorrhizas: implications for the evolution of belowground strategies. Ecol. Evol. 4, 2979–2990. doi: 10.1002/ece3.1147
Dakora, F. D., and Phillips, D. A. (2002). “Root exudates as mediators of mineral acquisition in low-nutrient environments,” in Food Security in Nutrient-Stressed Environments: Exploiting Plants’ Genetic Capabilities, ed. J. J. Adu-Gyamfi (Dordrecht: Springer), 201–213. doi: 10.1023/A:1020809400075
Dalling, J. W., Heineman, K., Lopez, O. R., Wright, S. J., and Turner, B. L. (2016). “Nutrient availability in tropical rain forests: the paradigm of phosphorus limitation,” in Tropical tree physiology, eds G. Guillermo and L. S. Santiago (Cham: Springer International Publishing), 261–273. doi: 10.1007/978-3-319-27422-5_12
Eissenstat, D. M., Kucharski, J. M., Zadworny, M., Adams, T. S., and Koide, R. T. (2015). Linking root traits to nutrient foraging in arbuscular mycorrhizal trees in a temperate forest. New Phytol. 208, 114–124. doi: 10.1111/nph.13451
Fox, J., and Weisberg, S. (2019). CAR – An R Companion to Applied Regression. Thousand Oaks, CA: Sage.
Francis, J. K. (2000). Bioecología de Arboles Nativos y Exóticos de Puerto Rico y las Indias Occidentales. General Technical Report IITF-15. Río Piedras, PR: USDA.
Freschet, G. T., Kichenin, E., and Wardle, D. A. (2015). Explaining within-community variation in plant biomass allocation: A balance between organ biomass and morphology above vs below ground? J. Veg. Sci. 26, 431–440. doi: 10.1111/jvs.12259
Freschet, G. T., Roumet, C., Comas, L. H., Weemstra, M., Bengough, A. G., Rewald, B., et al. (2021). Root traits as drivers of plant and ecosystem functioning: current understanding, pitfalls and future research needs. New Phytol. doi: 10.1111/nph.17072
Grime, J. P. (1977). Evidence for the existence of three primary strategies in plants and its relevance to ecological and evolutionary theory. Am. Nat. 111, 1169–1194. doi: 10.1086/283244
Guilbeault-Mayers, X., Turner, B. L., and Laliberté, E. (2020). Greater root phosphatase activity of tropical trees at low phosphorus despite strong variation among species. Ecology 101:e03090. doi: 10.1002/ecy.3090
Harris, N. L., and Medina, E. (2013). Changes in leaf properties across an elevation gradient in the luquillo mountains. Puerto Rico. Ecol. Bull. 54, 169–180.
Hinsinger, P. (2001). Bioavailability of soil inorganic P in the rhizosphere as affected by root-induced chemical changes: a review. Plant Soil 237, 173–195. doi: 10.1023/A:1013351617532
Hodge, A. (2004). The plastic plant: root responses to heterogeneous supplies of nutrients. New Phytol. 162, 9–24. doi: 10.1111/j.1469-8137.2004.01015.x
Holdaway, R. J., Richardson, S. J., Dickie, I. A., Peltzer, D. A., and Coomes, D. A. (2011). Species- and community-level patterns in fine root traits along a 120000-year soil chronosequence in temperate rain forest. J. Ecol. 99, 954–963. doi: 10.1111/j.1365-2745.2011.01821.x
Iversen, C. M., Powell, A. S., McCormack, M. L., Blackwood, C. B., Freschet, G. T., Kattge, J., et al. (2018). Fine-Root Ecology Database (FRED): A Global Collection of Root Trait Data with Coincident Site, Vegetation, Edaphic, and Climatic Data, Version 2. Oak Ridge National Lab. TES SFA. Oak Ridge, TN: U.S. Department Energy.
Johnson, N. C. (2010). Resource stoichiometry elucidates the structure and function of arbuscular mycorrhizas across scales. New Phytol. 185, 631–647. doi: 10.1111/j.1469-8137.2009.03110.x
Kennard, D. K., Matlaga, D., Sharpe, J., Wood, T. E., Alonso-Rodríguez, A. M., Reed, S. C., et al. (2020). Tropical understory herbaceous community responds more strongly to hurricane disturbance than to experimental warming. Ecol. Evol. 10, 8906–8915. doi: 10.1002/ece3.6589
Koide, R. T., and Kabir, Z. (2000). Extraradical hyphae of the mycorrhizal fungus Glomus intraradices can hydrolyse organic phosphate. New Phytol. 148, 511–517. doi: 10.1046/j.1469-8137.2000.00776.x
Kong, D., Ma, C., Zhang, Q., Li, L., Chen, X., Zeng, H., et al. (2014). Leading dimensions in absorptive root trait variation across 96 subtropical forest species. New Phytol. 203, 863–872. doi: 10.1111/nph.12842
Kormanik, P. P., and Mcgraw, A. C. (1982). “Quantification of vesicular-arbuscular mycorrhizas in plant roots,” in Methods and Principles of Mycorrhizal Research, ed. N. C. Schenck (St Paul, MI: The American Phytopathological Society), 37–45.
Kramer-Walter, K. R., Bellingham, P. J., Millar, T. R., Smissen, R. D., Richardson, S. J., and Laughlin, D. C. (2016). Root traits are multidimensional: specific root length is independent from root tissue density and the plant economic spectrum. J. Ecol. 104, 1299–1310. doi: 10.1111/1365-2745.12562
Kruskal, J. B. (1964). Multidimensional scaling by optimizing goodness of fit to a nonmetric hypothesis. Psychometrika 29, 1–27. doi: 10.1007/BF02289565
Lambers, H., Shane, M. W., Cramer, M. D., Pearse, S. J., and Veneklaas, E. J. (2006). Root structure and functioning for efficient acquisition of phosphorus: matching morphological and physiological traits. Ann. Bot. 98, 693–713. doi: 10.1093/aob/mcl114
Lee, R. B. (1988). Phosphate influx and extracellular phosphatase activity in barley roots and rose cells. New Phytol. 109, 141–148. doi: 10.1111/j.1469-8137.1988.tb03701.x
Lenth, R., Singmann, H., Love, J., Buerkner, P., and Herve, M. (2020). Estimated Marginal Means, Aka Least-Squares Means. R Package version 1.5.0. Available online at: https://CRAN.R-project.orgpackage=emmeans (accessed April 23, 2021).
Liese, R., Alings, K., and Meier, I. C. (2017). Root branching is a leading root trait of the plant economics spectrum in temperate trees. Front. Plant Sci. 8:315. doi: 10.3389/fpls.2017.00315
Liptzin, D., Silver, W. L., and Pan, Y. (2015). Spatial patterns in oxygen and redox sensitive biogeochemistry in tropical forest soils. Ecosphere 6, 1–14. doi: 10.1890/ES14-00309.1
Liu, B., Li, H., Zhu, B., Koide, R. T., Eissenstat, D. M., and Guo, D. (2015). Complementarity in nutrient foraging strategies of absorptive fine roots and arbuscular mycorrhizal fungi across 14 coexisting subtropical tree species. New Phytol. 208, 125–136. doi: 10.1111/nph.13434
Liu, C., Ravnskov, S., Liu, F., Rubæk, G. H., and Andersen, M. N. (2018). Arbuscular mycorrhizal fungi alleviate abiotic stresses in potato plants caused by low phosphorus and deficit irrigation/partial root-zone drying. J. Agric. Sci. 156, 46–58. doi: 10.1017/S0021859618000023
Liu, C., Xiang, W., Zou, L., Lei, P., Zeng, Y., Ouyang, S., et al. (2019). Variation in the functional traits of fine roots is linked to phylogenetics in the common tree species of Chinese subtropical forests. Plant Soil 436, 347–364. doi: 10.1007/s11104-019-03934-0
Lüdecke, D., Makowski, D., Waggoner, P., and Patil, I. (2020). Performance: Assessment of Regression Models Performance. Available online at: https://cran.r-project.org/package=performance (accessed April 23, 2021).
Lugli, L. F., Andersen, K. M., Aragão, L. E. O. C., Cordeiro, A. L., Cunha, H. F. V., Fuchslueger, L., et al. (2019). Multiple phosphorus acquisition strategies adopted by fine roots in low-fertility soils in Central Amazonia. Plant Soil 450, 49–63. doi: 10.1007/s11104-019-03963-9
Lugli, L. F., Rosa, J. S., Andersen, K. M., Di Ponzio, R., Almeida, R. V., Pires, M., et al. (2021). Rapid responses of root traits and productivity to phosphorus and cation additions in a tropical lowland forest in Amazonia. New Phytol. 230, 116–128. doi: 10.1111/nph.17154
Lugo, A. E. (2020). Effects of extreme disturbance events: from ecesis to social–ecological–technological systems. Ecosystems 23, 1726–1747. doi: 10.1007/s10021-020-00491-x
Lugo, A. E., Abelleira, O. J., Collado, A., Viera, C. A., Santiago, C., Vélez, D. O., et al. (2011). Allometry, biomass, and chemical content of Novel African Tulip Tree (Spathodea campanulata) forests in puerto rico. New For. 42, 267–283. doi: 10.1007/s11056-011-9258-8
Lugo, A. E., and Abelleira Martínez, O. L. (2018). Stoichiometry of decomposing Spathodea campanulata leaves in novel puertorrican forests. For. Ecol. Manage. 430, 176–187. doi: 10.1016/j.foreco.2018.07.059
Ma, Z., Guo, D., Xu, X., Lu, M., Bardgett, R. D., Eissenstat, D. M., et al. (2018). Evolutionary history resolves global organization of root functional traits. Nature 555, 94–97. doi: 10.1038/nature25783
Margalef, O., Sardans, J., Fernández-Martínez, M., Molowny-Horas, R., Janssens, I. A., Ciais, P., et al. (2017). Global patterns of phosphatase activity in natural soils. Sci. Rep. 7: 1337. doi: 10.1038/s41598-017-01418-8
McCormack, M. L., Guo, D., Iversen, C. M., Chen, W., Eissenstat, D. M., Fernandez, C. W., et al. (2017). Building a better foundation: improving root-trait measurements to understand and model plant and ecosystem processes. New Phytol. 215, 27–37. doi: 10.1111/nph.14459
McCormack, M. L., and Iversen, C. M. (2019). Physical and functional constraints on viable belowground acquisition strategies. Front. Plant Sci. 10:1215. doi: 10.3389/fpls.2019.01215
McDonald, J. H. (2014). Multiple comparisons – Handbook of Biological Statistics. Baltimore, MD: Sparky House Publishing.
McGonigle, T. P., Miller, M. H., Evans, D. G., Fairchild, G. L., and Swan, J. A. (1990). A new method which gives an objective measure of colonization of roots by vesicular—arbuscular mycorrhizal fungi. New Phytol. 115, 495–501. doi: 10.1111/j.1469-8137.1990.tb00476.x
Myster, R. W., Lebron, L., Loayza, A. B. P., and Zimmerman, J. K. (2013). Mycotrophic strategy of 13 common neotropical trees and shrubs. J. Trop. For. Sci. 25, 34–41.
Nasto, M. K., Osborne, B. B., Lekberg, Y., Asner, G. P., Balzotti, C. S., Porder, S., et al. (2017). Nutrient acquisition, soil phosphorus partitioning and competition among trees in a lowland tropical rain forest. New Phytol. 214, 1506–1517. doi: 10.1111/nph.14494
Nasto, M. K., Winter, K., Turner, B. L., and Cleveland, C. C. (2019). Nutrient acquisition strategies augment growth in tropical N2-fixing trees in nutrient-poor soil and under elevated CO2. Ecology 100:e02646. doi: 10.1002/ecy.2646
Neyroud, J. A., and Lischer, P. (2003). Do different methods used to estimate soil phosphorus availability across Europe give comparable results? J. Plant Nutr. Soil Sci. 166, 422–431. doi: 10.1002/jpln.200321152
Olander, L. P., and Vitousek, P. M. (2004). Biological and geochemical sinks for phosphorus in soil from a wet tropical forest. Ecosystems 7, 404–419. doi: 10.1007/s10021-004-0264-y
Paoli, G. D., Curran, L. M., and Zak, D. R. (2005). Phosphorus efficiency of Bornean rain forest productivity: evidence against the unimodal efficiency hypothesis. Ecology 86, 1548–1561. doi: 10.1890/04-1126
Pasch, R. J., Penny, A. B., and Berg, R. (2019). National Hurricane Center Tropical Cyclone Report: Hurricane Maria (AL152017). Silver Spring, MD: National Weather Service.
Png, G. K., Turner, B. L., Albornoz, F. E., Hayes, P. E., Lambers, H., and Laliberté, E. (2017). Greater root phosphatase activity in nitrogen-fixing rhizobial but not actinorhizal plants with declining phosphorus availability. J. Ecol. 105, 1246–1255. doi: 10.1111/1365-2745.12758
Ponciano, J. M., Taper, M. L., Dennis, B., and Lele, S. R. (2009). Hierarchical models in ecology: confidence intervals, hypothesis testing, and model selection using data cloning. Ecology 90, 356–362. doi: 10.1890/08-0967.1
Powers, J. S., Treseder, K. K., and Lerdau, M. T. (2005). Fine roots, arbuscular mycorrhizal hyphae and soil nutrients in four neotropical rain forests: patterns across large geographic distances. New Phytol. 165, 913–921. doi: 10.1111/j.1469-8137.2004.01279.x
Pregitzer, K. S., DeForest, J. L., Burton, A. J., Allen, M. F., Ruess, R. W., and Hendrick, R. L. (2002). Fine root architecture of nine north american trees. Ecol. Monogr. 72, 293–309. doi: 10.2307/3100029
Quesada, C. A., Lloyd, J., Schwarz, M., Patiño, S., Baker, T. R., Czimczik, C., et al. (2010). Variations in chemical and physical properties of Amazon forest soils in relation to their genesis. Biogeosciences 7, 1515–1541. doi: 10.5194/bg-7-1515-2010
R Core Team (2019). R: A Language and Environment for Statistical Computing. Vienna: R Foundation Statistics Computing.
Reed, S. C., Reibold, R., Cavaleri, M. A., Alonso-Rodríguez, A. M., Berberich, M. E., and Wood, T. E. (2020). Soil biogeochemical responses of a tropical forest to warming and hurricane disturbance. Adv. Ecol. Res. 62, 226–248. doi: 10.1016/bs.aecr.2020.01.007
Reed, S. C., Townsend, A. R., Taylor, P. G., and Cleveland, C. C. (2011). “Phosphorus cycling in tropical forests growing on highly weathered soils,” in Phosphorus in Action. Soil Biology, Vol. 26, eds E. Bünemann, A. Oberson, and E. Frossard (Berlin: Springer), doi: 10.1007/978-3-642-15271-9_14
Ryser, P., and Eek, L. (2000). Consequences of phenotypic plasticity vs. interspecific differences in leaf and root traits for acquisition of aboveground and belowground resources. Am. J. Bot. 87, 402–411. doi: 10.2307/2656636
Santiago, L. S. (2015). Nutrient limitation of eco-physiological processes in tropical trees. Trees Struct. Funct. 29, 1291–1300. doi: 10.1007/s00468-015-1260-x
Sardans, J., Peñuelas, J., and Estiarte, M. (2007). Seasonal patterns of root-surface phosphatase activities in a Mediterranean shrubland. Responses to experimental warming and drought. Biol. Fertil. Soils 43, 779–786. doi: 10.1007/s00374-007-0166-1
Scatena, F. N. (1989). An Introduction to the Physiography and History of the Bisley Experimental Watersheds in the Luquillo Mountains of Puerto Rico. General Technical Report SO-72. New Orleans, LA: Forest Service Southern Forest Experiment Station, 22.
Schreeg, L. A., Santiago, L. S., Wright, S. J., and Turner, B. L. (2014). Stem, root, and older leaf N: P ratios are more responsive indicators of soil nutrient availability than new foliage. Ecology 95, 2062–2068. doi: 10.1890/13-1671.1
Smith, S., and Read, D. (2008). Mycorrhizal Symbiosis, 3rd Edn. London: Academic Press, doi: 10.1016/B978-0-12-370526-6.X5001-6
Smith, S. E., and Smith, F. A. (2011). Roles of arbuscular mycorrhizas in plant nutrition and growth: new paradigms from cellular to ecosystem scales. Annu. Rev. Plant Biol. 62, 227–250. doi: 10.1146/annurev-arplant-042110-103846
Stone, M. M., DeForest, J. L., and Plante, A. F. (2014). Changes in extracellular enzyme activity and microbial community structure with soil depth at the Luquillo Critical Zone Observatory. Soil Biol. Biochem. 75, 237–247. doi: 10.1016/j.soilbio.2014.04.017
Tabatabai, M. A., and Bremner, J. M. (1969). Use of p-nitrophenyl phosphate for assay of soil phosphatase activity. Soil Biol. Biochem. 1, 301–307. doi: 10.1016/0038-0717(69)90012-1
Townsend, A. R., Cleveland, C. C., Asner, G. P., and Bustamante, M. M. C. (2007). Controls over foliar N: P ratios in tropical rain forests. Ecology 88, 107–118. doi: 10.1890/0012-9658(2007)88[107:cofnri]2.0.co;2
Treseder, K. K. (2013). The extent of mycorrhizal colonization of roots and its influence on plant growth and phosphorus content. Plant Soil. 371, 1–13. doi: 10.1007/s11104-013-1681-5
Treseder, K. K., and Vitousek, P. M. (2001). Effects of soil nutrient availability on investment in acquisition of N and P in Hawaiian rain forests. Ecology 82, 946–954. doi: 10.1890/0012-9658(2001)082[0946:eosnao]2.0.co;2
Turner, B. L. (2008). Resource partitioning for soil phosphorus: a hypothesis. J. Ecol. 96, 698–702. doi: 10.1111/j.1365-2745.2008.01384.x
Uriarte, M., and Zimmerman, J. (2017). El Yunque Chronosequence Tree Census Data ver 17. Environmental Data Initiative. Available online at: https://doi.org/10.6073/pasta/7a8315e46e841e20413968144c9e3076 (accessed June 27, 2021).
Uriarte, M., Thompson, J., and Zimmerman, J. K. (2019). Hurricane María tripled stem breaks and doubled tree mortality relative to other major storms. Nat. Commun. 10, 1–7. doi: 10.1038/s41467-019-09319-2
Valverde-Barrantes, O. J., Horning, A. L., Smemo, K. A., and Blackwood, C. B. (2016). Phylogenetically structured traits in root systems influence arbuscular mycorrhizal colonization in woody angiosperms. Plant Soil 404, 1–12. doi: 10.1007/s11104-016-2820-6
Valverde-Barrantes, O. J., Maherali, H., Baraloto, C., and Blackwood, C. B. (2020). Independent evolutionary changes in fine-root traits among main clades during the diversification of seed plants. New Phytol. 228, 541–553. doi: 10.1111/nph.16729
Valverde-Barrantes, O. J., Smemo, K. A., and Blackwood, C. B. (2015). Fine root morphology is phylogenetically structured, but nitrogen is related to the plant economics spectrum in temperate trees. Funct. Ecol. 29, 796–807. doi: 10.1111/1365-2435.12384
Vance, C. P., Uhde-Stone, C., and Allan, D. L. (2003). Phosphorus acquisition and use: Critical adaptations by plants for securing a nonrenewable resource. New Phytol. 157, 423–447. doi: 10.1046/j.1469-8137.2003.00695.x
Vitousek, P. M., and Sanford, R. L. (1986). Nutrient cycling in moist tropical forest. Annu. Rev. Ecol. Syst. 17, 137–167. doi: 10.1146/annurev.es.17.110186.001033
Walker, T. W., and Syers, J. K. (1976). The fate of phosphorus during pedogenesis. Geoderma 15, 1–19. doi: 10.1016/0016-7061(76)90066-5
Wang, R., Wang, Q., Zhao, N., Xu, Z., Zhu, X., Jiao, C., et al. (2018). Different phylogenetic and environmental controls of first-order root morphological and nutrient traits: evidence of multidimensional root traits. Funct. Ecol. 32, 29–39. doi: 10.1111/1365-2435.12983
Wang, Y., Wang, M., Li, Y., Wu, A., and Huang, J. (2018). Effects of arbuscular mycorrhizal fungi on growth and nitrogen uptake of Chrysanthemum morifolium under salt stress. PLoS One 13:e0196408. doi: 10.1371/journal.pone.0196408
Weemstra, M., Mommer, L., Visser, E. J. W., van Ruijven, J., Kuyper, T. W., Mohren, G. M. J., et al. (2016). Towards a multidimensional root trait framework: a tree root review. New Phytol. 211, 1159–1169. doi: 10.1111/nph.14003
Wen, Z., Li, H., Shen, Q., Tang, X., Xiong, C., Li, H., et al. (2019). Tradeoffs among root morphology, exudation and mycorrhizal symbioses for phosphorus-acquisition strategies of 16 crop species. New Phytol. 223, 882–895. doi: 10.1111/nph.15833
Wright, I. J., Reich, P. B., Westoby, M., Ackerly, D. D., Baruch, Z., Bongers, F., et al. (2004). The worldwide leaf economics spectrum. Nature 428, 821–827. doi: 10.1038/nature02403
Xiang, W., Wu, W., Tong, J., Deng, X., Tian, D., Zhang, L., et al. (2013). Differences in fine root traits between early and late-successional tree species in a Chinese subtropical forest. Forestry 86, 343–351. doi: 10.1093/forestry/cpt003
Zalamea, P. C., Turner, B. L., Winter, K., Jones, F. A., Sarmiento, C., and Dalling, J. W. (2016). Seedling growth responses to phosphorus reflect adult distribution patterns of tropical trees. New Phytol. 212, 400–408. doi: 10.1111/nph.14045
Zangaro, W., Alves, R. A., Lescano, L. E., Ansanelo, A. P., and Nogueira, M. A. (2012). Investment in fine roots and arbuscular mycorrhizal fungi decrease during succession in three brazilian ecosystems. Biotropica 44, 141–150. doi: 10.1111/j.1744-7429.2011.00781.x
Zangaro, W., De Assis, R. L., Rostirola, L. V., De Souza, P. B., Gonçalves, M. C., Andrade, G., et al. (2008). Changes in arbuscular mycorrhizal associations and fine root traits in sites under different plant successional phases in southern Brazil. Mycorrhiza 19, 37–45. doi: 10.1007/s00572-008-0202-5
Keywords: root architecture, specific root length, root diameter, phosphorus concentration, Luquillo Experimental Forest, phosphatase activity, root traits
Citation: Yaffar D, Defrenne CE, Cabugao KG, Kivlin SN, Childs J, Carvajal N and Norby RJ (2021) Trade-Offs in Phosphorus Acquisition Strategies of Five Common Tree Species in a Tropical Forest of Puerto Rico. Front. For. Glob. Change 4:698191. doi: 10.3389/ffgc.2021.698191
Received: 20 April 2021; Accepted: 11 June 2021;
Published: 13 July 2021.
Edited by:
Erik Verbruggen, University of Antwerp, BelgiumReviewed by:
Kerstin Pierick, University of Göttingen, GermanyThomas Kuyper, Wageningen University and Research, Netherlands
Copyright © 2021 Yaffar, Defrenne, Cabugao, Kivlin, Childs, Carvajal and Norby. This is an open-access article distributed under the terms of the Creative Commons Attribution License (CC BY). The use, distribution or reproduction in other forums is permitted, provided the original author(s) and the copyright owner(s) are credited and that the original publication in this journal is cited, in accordance with accepted academic practice. No use, distribution or reproduction is permitted which does not comply with these terms.
*Correspondence: Daniela Yaffar, ZGFuaWVsYXlhZmZhckBnbWFpbC5jb20=; ZHlhZmZhckB2b2xzLnV0ay5lZHU=