- 1Sustainable Forestry, Forest Resources and Management, WSL Birmensdorf, Birmensdorf, Switzerland
- 2Boreal Ecosystems Research Group, Department of Biological and Environmental Science, University of Jyväskylä, Jyväskylä, Finland
- 3Resource Analysis, Forest Resources and Management, WSL Birmensdorf, Birmensdorf, Switzerland
Sustainable forest management plays a key role for forest biodiversity and the provisioning of ecosystem services (BES), including the important service of carbon sequestration for climate change mitigation. Forest managers, however, find themselves in the increasingly complex planning situation to balance the often conflicting demands in BES. To cope with this situation, a prototype of a decision support system (DSS) for strategic (long-term) planning at the forest enterprise level was developed in the present project. The DSS was applied at three case study enterprises (CSEs) in Northern Switzerland, two lowland and one higher-elevation enterprise, for a 50-year time horizon (2010 to 2060) under present climate and three climate change scenarios (‘wet’, ‘medium’, ‘dry’). BES provisioning (for biodiversity, timber production, recreation, protection against gravitational hazards and carbon sequestration) was evaluated for four management scenarios (no management, current (BAU), lower and higher management intensity) using a utility-based multi-criteria decision analysis. Additionally, four alternative preference scenarios for BES provisioning were investigated to evaluate the robustness of the results to shifting BES preferences. At all CSEs, synergies between carbon sequestration, biodiversity and protection function as well as trade-offs between carbon sequestration and timber production occurred. The BAU management resulted in the highest overall utility in 2060 for different climate and BES preference scenarios, with the exception of one lowland CSE under current BES preference, where a lower intensity management performed best. Although climate change had a relatively small effect on overall utility, individual BES indicators showed a negative climate change impact for the lowland CSEs and a positive effect for the higher elevation CSE. The patterns of overall utility were relatively stable to shifts in BES preferences, with exception of a shift toward a preference for carbon sequestration. Overall, the study demonstrates the potential of the DSS to investigate the development of multiple BES as well as their synergies and trade-offs for a set of lowland and mountainous forest enterprises. The new system incorporates a wide set of BES indicators, a strong empirical foundation and a flexible multi-criteria decision analysis, enabling stakeholders to take scientifically well-founded decisions under changing climatic conditions and political goals.
Introduction
Forest ecosystems play a key role for biodiversity and ecosystem services (BES) provisioning (UNCCC 2015; United Nation CBD 2020). Over the past decades, the portfolio of demands for BES has increased considerably, most recently by the rising awareness for the role of forests in climate change (CC) mitigation due to carbon sequestration (Luyssaert et al., 2010). Forest managers therefore find themselves in the difficult position to balance the political demands in biodiversity promotion and ecosystem service provisioning with other socio-economic demands (Borges et al., 2014). Besides the importance of forests for carbon sequestration, timber production and biodiversity, progressive urbanization leads to an increasing demand in recreation value of forests (e.g., Hegetschweiler et al., 2020). Furthermore, many forests offer protection functions, e.g., in mountainous areas where forests play a key role in protecting settlements and infrastructure against gravitational hazards, such as rockfall, avalanches and landslides (Frehner et al., 2005). Since these diverse demands in BES are often at conflict with each other (e.g., Mina et al., 2017), forest managers have to cope with significant trade-offs in planning (e.g., Langner et al., 2017; Blattert et al., 2018; Bont et al., 2019). Further complexity is added to the planning situation by the impacts of climate change on forest ecosystems, which will likely induce profound shifts in forest BES provisioning (Mina et al., 2017; Seidl et al., 2019). In this increasingly complex and diverse planning situation, science-based decision support is thus key for planning the sustainable management of multifunctional forests (Kangas et al., 2015).
For this purpose, various decision support systems (DSS) have been developed in forestry across the globe (Vacik and Lexer, 2014; Nordström et al., 2019) and are increasingly used to explore synergies and trade-offs in BES (e.g., Biber et al., 2020). Although a DSS can in principal be any system that aids decision makers, the term typically refers to model-based software systems which provide a user interface, a ‘knowledge system’ (database, models, etc.) and a ‘problem processing system’ (e.g., for calculating decision analyses) (Borges et al., 2014). Over time, DSS have been developed from systems for a single purpose (e.g., evaluation of sustainable timber production) to systems including multiple criteria (e.g., a wide variety of BES) and a modular construction (i.e., providing an integrative and flexible software framework) (Eriksson and Borges, 2014). A particular challenge is to keep the system easy to handle and to provide results in a condensed and transparent way for the decision maker (Vacik and Lexer, 2014). DSS in forestry are therefore mostly developed for a specific region and particular environmental, social, economic situation at a specific spatial and temporal scale of interest (Eriksson and Borges, 2014). Despite the various DSS existing worldwide, such systems thus need to be tailored toward the specific needs of local forest management and planning tasks (Nordström et al., 2019).
In Switzerland, forests are characterized by a wide variety of forest types, reflecting the large elevational and environmental gradients from lowland to alpine conditions (Rigling and Schaffer, 2015). As in several European countries, forests in Switzerland are managed according to the principle of ‘close-to-nature’ forestry (Hanewinkel and Kammerhofer, 2015), which aims at reaching multiple ecological, economic and social goals in a sustainable way by applying management interventions which follow natural processes in forest ecosystems (Messier et al., 2013). The large variety of forest conditions is also reflected in a number of DSS and tools that have already been developed to aid forest practitioners (Heinimann et al., 2014), such as WIS2 for short- to mid-term silvicultural planning (Rosset et al., 2014). In recent years, a DSS has been introduced by Blattert et al. (2018) that allows combining forest ecosystem simulation and multi-criteria decision analysis (MCDA, see also Wolfslehner and Seidl (2010)) and that focuses on long-term planning (i.e., several decades) at the forest enterprise level. The MCDA approach allows to account for multiple, often conflicting criteria, to integrate explicitly stated stakeholder preferences, and to explore the performance of alternative assumptions, which leads to a rationale and structured decision process that can be communicated in a justified and transparent way (Wolfslehner and Seidl, 2010; Uhde et al., 2015; Schweier et al., 2019). Moreover, the MCDA approach allows to integrate a wide range of BES indicators as well as Swiss-wide relationships between simulated BES supply and the demands of the society (via so-called value functions) (Blattert et al., 2017). The DSS framework of Blattert et al. (2018) furthermore goes beyond previous assessments of BES in Switzerland (e.g., Bircher, 2015) by incorporating also social services (i.e., recreation function) and offering a more holistic perspective on carbon sequestration by assessing not only sequestration within forests (‘in-situ’) but also outside the forest system boundary (‘ex-situ’). Particularly assessments of ‘ex-situ’ carbon sequestrations that are accounting for harvested wood products and substitution effects are highly relevant for future forestry (Nabuurs et al., 2017), but are still rarely included in DSS (Seidl et al., 2007; Blattert et al., 2020). This DSS framework is hence well-suited to explore not only shifts in the long-term BES provisioning, but also to conduct a more detailed analysis of potential shifts in management strategies on carbon sequestration and associated synergies and trade-offs with other BES. However, the DSS of Blattert et al. (2018) was based on a forest growth model developed for Northern Germany and was restricted to applications under present climatic conditions and hence not suited to explore developments under climate change.
In recent years, climate change has increasingly impacted forests in Switzerland (e.g., Brun et al., 2020) and is gaining rising importance in the strategic (long-term) planning of forest management (Streit et al., 2017). While climate change impacts on Swiss forests are predominantly negative at lower elevations (e.g., reduced growth, increased mortality due to drought and heat), the trend toward a prolonged growing seasons has positive effects on forest growth at higher elevations (Bugmann et al., 2014). These large-scale patterns of climate change impacts can however be significantly modified by smaller-scale environmental heterogeneity, e.g., due to the effect of aspect, slope or orographic rainfall (Whiteman, 2000; Zou et al., 2007). Climate change impacts at the forest enterprise level can consequently be complex and site-specific, particularly in mountainous areas (Mina et al., 2017; Thrippleton et al., 2020).
It is therefore important to develop a DSS for Swiss conditions, which: (1) is built on a strong empirical basis reflecting the large gradient of climatic and environmental conditions, (2) is suitable for strategic (long-term) planning at the forest enterprise level under both lowland and mountainous conditions, (3) covers a large variety of BES relevant for Swiss forestry, including the service of carbon sequestration by accounting for ‘in-situ’ as well as ‘ex-situ’ sequestration, and which (4) is able to investigate different climate change trajectories to provide scientific decision support in complex planning situations.
Here, we present a prototype for a new DSS for strategic planning at the forest enterprise level that addresses these aspects. The system is based on the revised MCDA framework of Blattert et al. (2018) and a new climate-sensitive forest growth model developed for Switzerland (SwissStandSim, Zell et al., 2020). The DSS was applied to three representative case study enterprises (CSEs) in Northern Switzerland with different priorities in BES provisioning (two lowland and one mountainous enterprise) for a 50-year time-period (2010 to 2060). Particularly, our research objectives were to: (1) identify and quantify synergies and trade-offs between BES for different management strategies accounting also for the effect of increasing carbon sequestration on other BES, (2) identify the management strategy that best provides multiple BES, and (3) analyze shifts in BES provisioning under climate change (‘dry’, ‘wet’, ‘moderate’ scenarios). Since the implications of shifting demands (i.e., weighting preferences) in BES provisioning is highly relevant for decision making (Langner et al., 2017), we also analyzed (4) four alternative BES demand preferences.
Materials and Methods
Case Study Enterprises
Three case study enterprises (CSEs) in Northern Switzerland were selected for the DSS application, with different demands in BES provisioning (Figure 1). Enterprise 1 (‘Wagenrain’, abbreviated as WAG) is located in the Swiss lowland plateau and focuses mainly on timber production. Enterprise 2 (‘Bülach’, abbreviated BUE) is also located in the lowlands of the Swiss plateau, and focuses more on the recreation service due to its close proximity to urban areas. Enterprise 3 (‘Gottschalkenberg’, abbreviated GOT), in contrast, is located in the Northern Pre-Alps at higher elevations and has a specific focus on biodiversity and protection against gravitational hazards (mostly erosion and landslides). The specific environmental conditions (geology, soil, climate, vegetation) of each CSE are summarized in Table 1, an overview of stand structure and tree species composition is provided in the Supplementary Appendix 1.1.
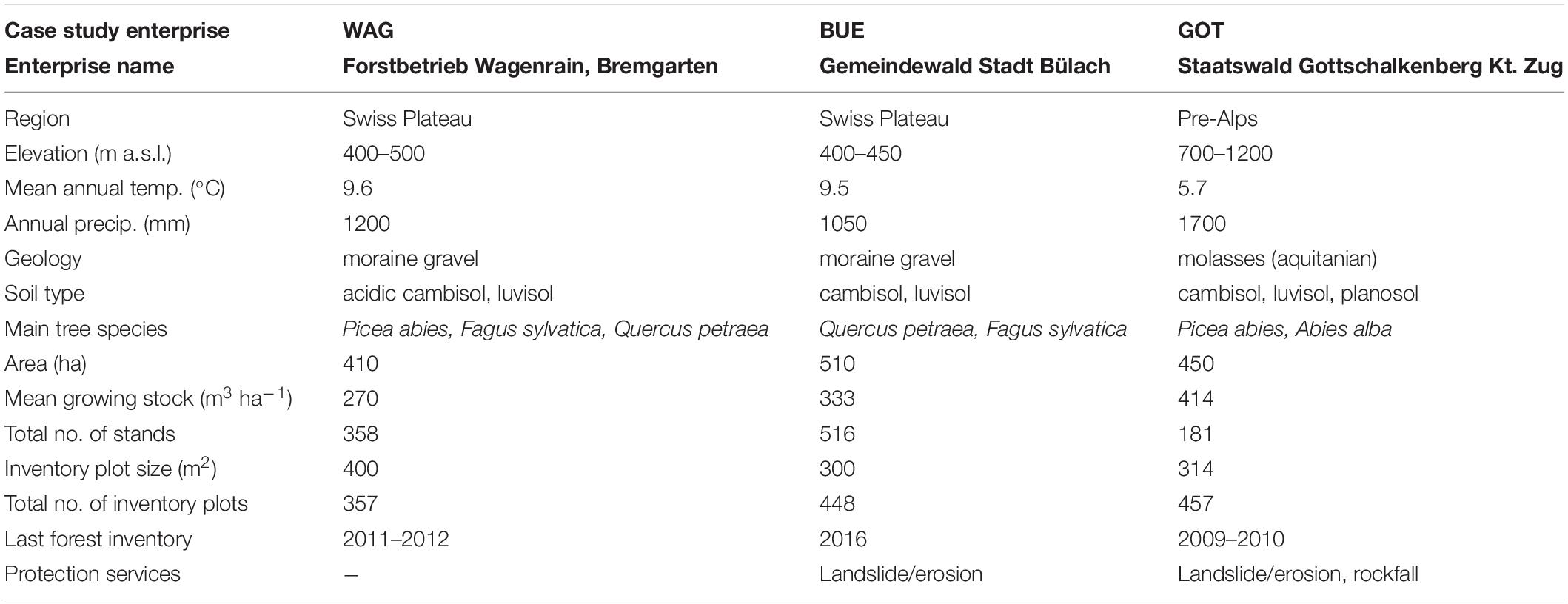
Table 1. Environmental conditions, forest composition, and inventory plot information for each case study enterprise.
Decision Support System
The DSS aims at providing forest managers with information about BES development in Swiss forest enterprises under changing management and climate conditions, thereby highlighting particularly synergies and trade-offs among BES. At the spatial scale, it is designed for applications at the enterprise level (typically several 100 ha) with the representation of individual stands. At the temporal scale, it provides information at 5- or 10-year intervals and can be used for future projections of several decades. In the present study, a time horizon of 50 years (2010 to 2060) was considered.
The system consists of three core components, which are further described in more detail below: (1) a database (data on climate, soils, stands and management settings), (2) a forest growth model (SwissStandSim, Zell, 2018; Zell et al., 2020) and (3) a multicriteria decision analysis (MCDA) system (Blattert et al., 2018), which evaluates the BES indicators calculated from the simulation results (see Figure 2 for a conceptual figure of the DSS structure). In its current prototype stage (v1.0), the DSS is fully functional, but does not provide an interactive graphical user interface (GUI) yet.
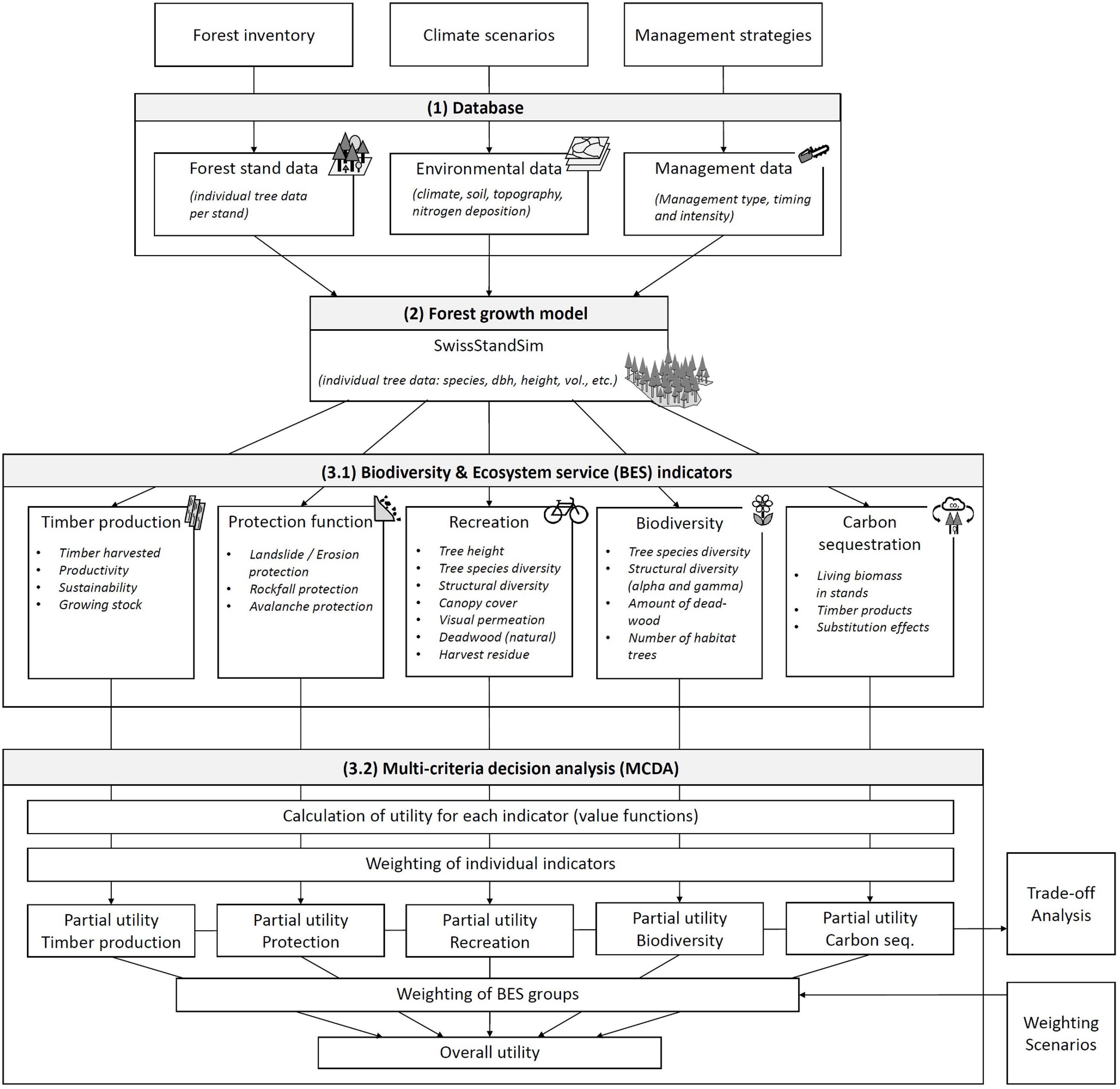
Figure 2. Structure of the decision support system (DSS), consisting of three main components, a database (1), a forest growth model (2) and a problem-processing system, comprising of a set of biodiversity and ecosystem service (BES) indicators calculated from the simulated forest characteristics (3.1) and a multi-criteria decision analysis (MCDA, 3.2).
Database
The datasets required for the application of the forest model comprise (1) environmental data (soil conditions, elevation, slope, aspect, climate, nitrogen deposition), (2) stand-level data of forest structures and composition (diameter, height and species of individual trees) and (3) management settings (defining time, intensity and type of management interventions), see also Zell (2018) and Zell et al. (2020).
For topographic information, a digital terrain model for Switzerland (200 m resolution, Swisstopo, 2010) was used to derive mean elevation, slope and aspects for each stand. Soil conditions (soil depth, water holding capacity, water permeability, nutrient availability) at each stand were derived from the Swiss soil suitability map (FSO, 2012), following the approach of Zell et al. (2020). For the climate data, the Swiss wide climate data of Brunner et al. (2019) were used for the respective location of the forest enterprises, see section ‘climate scenarios’ below for further information. Nitrogen deposition required by the forest growth model was estimated from the Swiss Nitrogen deposition maps (FOEN, 2015b) for each CSE and assumed to remain constant for the considered timeframe.
For the simulation of the forest growth with SwissStandSim, individual tree data (i.e., species, diameter, height) is required at the stand level (Zell et al., 2020). Since individual tree data is typically not available at the level of the entire enterprise, this information was derived from local-scale forest enterprise inventories for WAG (Bont et al., 2020), BUE (‘Kt. Zürich, ALN, Abt. Wald, forest inventory 2016’) and GOT (‘Kt. Zug, Amt für Wald und Wild, forest inventory 2009’). Forest enterprise inventories were conducted using the method of Schmid-Haas et al. (1993), comprising data of individual tree records (e.g., species, diameter) measured within circular plots, which are located along a dense regular grid (see Table 1 for further details). Due to the 5-year resolution of the DSS, it was assumed that the last inventories of the CSEs (which were measured between 2008 and 2016) provide representative datasets for generating stand structure and composition of the year 2010 (i.e., the starting point of the simulation).
For each forest enterprise, the local-scale enterprise level inventory data and information from the Swiss national forest inventory (NFI) were used to predict locally adapted complete stand descriptions using the approach of Mey et al. (2021). The approach consists of four steps: (1) forest enterprise inventory data was used to calculate stand-level summary statistics, (2 and 3) the summary statistics were adjusted to account for young trees (based on NFI-data, since enterprise-level inventories did not measure trees smaller than 12 cm diameter) and were subsequently used to predict stand-level diameter distributions data, and (4) tree species composition was assigned based on the forest enterprise inventory data. A detailed description and an evaluation of the stand initialization approach is provided in Supplementary Appendix 1.1.
Forest Growth Model
The forest model SwissStandSim is an empirical, climate-sensitive forest growth model, which was developed for single- and mixed-species forests in Swiss lowland and mountain forests (Zell, 2018; Zell et al., 2020). The calibration dataset of SwissStandSim covers 374 stands located throughout Switzerland with observation timespans of 15 to 112 years from the experimental forest management network (Forrester et al., 2019). In terms of forest management, the user can select different forest management types (e.g., thinning from below, thinning from above, crop tree selective thinning) and define beginning, end and time intervals as well as intensity of interventions (Zell, 2018).
SwissStandSim represents processes of individual tree demography explicitly via species-specific statistical models for regeneration (ingrowth), growth and mortality. These demographic models consider climatic factors as explanatory variables, i.e., mean annual temperature, precipitation and a moisture index, which feature non-linear effects and contain interactions (Zell, 2016). Changes in climatic conditions thus have species-specific impacts on growth, ingrowth and mortality, which are described in further detail in Zell (2018) and Zell et al. (2020). The forest model considers 11 species and species groups, covering the main tree species in Switzerland, i.e., Fagus sylvatica, Acer sp., Quercus sp., Picea abies, Abies alba, Pinus sylvestris and Larix decidua. Furthermore, douglas fir (Pseudotsuga menziesii) as well as species groups of ‘long-lived broadleaved’, ‘short-lived broadleaved’ and ‘other conifers’ were represented (see Zell, 2016; Zell et al., 2020).
Simulations can be carried out for timespans of several decades with a temporal resolution of 5 years. For the present study, all simulations were carried out for the timeframe of 2010 to 2060, i.e., a time span of 50 years, corresponding to a typical timeframe of several decades for long-term strategic forest management planning (Segura et al., 2014).
Management Strategies
Four management strategies were defined at all CSEs: (1) ‘no management’ (NO), which serves as a baseline scenario, (2) a ‘business as usual’ (BAU, current management strategy), which aims at a multifunctional use of the forest, (3) a ‘higher intensity’ (HIGH) management strategy, aiming at an increased timber use as an essential component for future bio-economy, as well as (4) a ‘lower intensity’ (LOW) management, which can be interpreted as a focus on a more biodiversity conservation-oriented strategy. In respect to carbon sequestration, the management strategies can also be regarded as a gradient from more ‘in-situ carbon storage’ in the forest ecosystem (‘NO’ management strategy), to an increased focus on ‘ex-situ carbon storage’ in wood products and substitution effects for the ‘HIGH’ intensity management strategy.
The BAU strategy (i.e., management type, intervention times and intensity) was defined in collaboration with the respective forest managers of each enterprise (Table 2, see also Supplementary Appendix 1.2). The corresponding ‘LOW’ and ‘HIGH’ management strategies were defined using the same management type as in BAU, but adapted, so that the amount of basal area removed equals −50% (‘LOW’ intensity) and +50% (‘HIGH’ intensity), relative to the BAU strategy (see Table 2). Further details about the management strategies are provided in Supplementary Appendix 1.2.
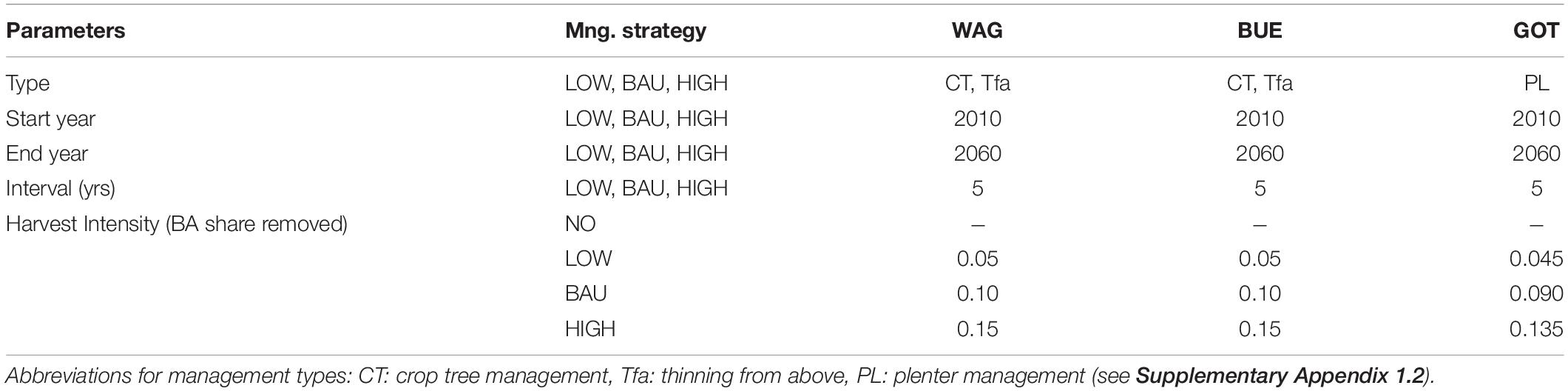
Table 2. Settings for the four alternative management strategies applied at the three case study enterprises WAG, BUE and GOT (NO: no management, LOW: low intensity, HIGH: high intensity, BAU: business as usual, representing current management).
Climate Scenarios
For the simulation of present and future climate conditions, downscaled climate datasets by Brunner et al. (2019) were used for each CSE and aggregated to 5 year averages (annual mean temperature, precipitation sum and moisture index), as described in Zell (2018). The climate data by Brunner et al. (2019) were based on representative concentration pathways (RCP) and downscaled using a regional down-scaling approach based on quantile mapping (Gudmundsson et al., 2012). For simulations under present (historic) climate conditions, climate data (dataset CC22, see Table 3) from the reference period 1981 to 2010 (CH2018, 2018) was used and expanded to a 50-year climate time series by randomly resampling climate data. When compared with data from nearby climate station for each CSE, the downscaled historic climate datasets showed a good agreement with measured climate data of the same reference period. For the climate change scenarios, three scenarios recommended by Brunner et al. (2019) as representing a typical ‘dry’ (CC1), ‘medium’ (CC22) and ‘wet’ (CC7) future climate were used for the time 2010 to 2060 (see Table 3). An overview over the climate change scenarios is provided in Table 3, for technical details about the datasets, cf. Brunner et al. (2019).
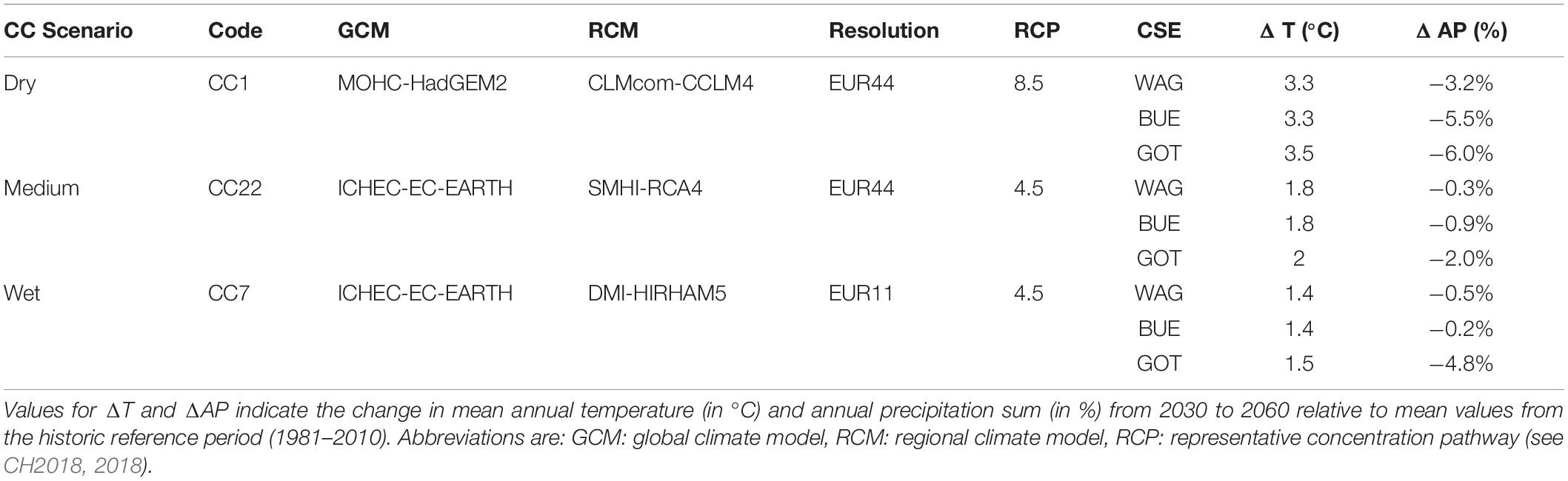
Table 3. Selected climate change (CC) scenarios from Brunner et al. (2019) at the different case study enterprises (CSEs).
BES Indicators
The effect of different management and climate scenarios were analyzed for five selected groups of BES indicators: timber production, biodiversity, recreation value (visual attractiveness), protection against gravitational hazards and carbon sequestration, using the indicator-based analysis framework of Blattert et al. (2017). The framework comprises 21 individual BES indicators applicable for entire Switzerland, which were calculated based on the simulated forest stand structures for each timestep. Below, a short summary of all BES indicators is given, a detailed description can be found in the Supplementary Appendix 1.3.
Timber production indicators included the amount of timber volume harvested (m3 ha–1 year–1), the productivity of the stand (annual net volume increment), the sustainability of timber use (expressed by the ratio between harvest and productivity) and the growing stock of the stands.
Biodiversity indicators comprised species diversity (expressed by the Shannon index, Shannon and Weaver, 1949) and structural diversity (PostHoc index, Staudhammer and LeMay, 2001) at the level of alpha diversity (representing diversity within each stand) and a gamma diversity (representing diversity at the enterprise level, i.e., the landscape scale), see also Jost (2007) and Sebald et al. (2021). Furthermore, the amount of deadwood as well as the number of habitat trees (defined as number trees per ha with a diameter of > 70 cm) were considered, which are particularly important structural attributes for biodiversity conservation (e.g., Bütler et al., 2020; Haeler et al., 2021).
Recreation value was considered in terms of forest visual attractiveness, which can be linked to stand structural attributes (Edwards et al., 2012). Based on the framework of Edwards et al. (2012), the considered indicators were: size of largest trees (m), variation in tree size (PostHoc index), extent of canopy cover (i.e., percentage of ground covered by canopy), visual permeation through stand (expressed via the stand density index, Daniel and Sterba, 1980), variation in tree species (Shannon index), residues from harvest and thinning as well as deadwood from natural mortality (deadwood volume in m3 ha–1).
For carbon sequestration, the amount of carbon in living aboveground and belowground tree biomass was calculated using species-, region- and elevation-specific allometric equations from the Swiss National Forest Inventory (Didion et al., 2019; Herold et al., 2019), as well as deadwood originating from natural mortality and harvest residues. Using an adapted approach of Blattert et al. (2018), furthermore carbon stored in three harvested wood product pools (sawnwood, wood-based panels, paper and paperboard, classification by UNFCCC, see IPCC, 2014a) as well as substitution effects were considered, with energy wood substituting fossil fuel emissions and construction wood substituting emissions by fossil-fuel intensive construction materials (e.g., concrete, steel) (Taverna et al., 2007). Since the developed DSS is intended to be a tool for forest enterprises aiming at the comparison of potential impacts of different forest management strategies on the provision of BES, we define the system boundary at the level of the respective forest enterprises. A detailed description of the carbon sequestration is provided in Supplementary Appendix 1.4.
MCDA Approach
To evaluate the effect of changing management strategies and climatic scenarios, a multi-criteria decision analysis (MCDA) approach was employed in the DSS. It is based on multi-attribute value theory (MAVT), which is particularly suitable for a small number of criteria (in our case five BES) and well-defined decision makers (in our case one decision maker per enterprise, i.e., the forest manager). MAVT aims at quantifying a partial utility value for each individual criterion and helps to identify the best performing management strategy that maximizes multiple BES simultaneously (overall utility) (Kangas et al., 2015; Uhde et al., 2015). Therefore, the relationship between the simulated BES indicators and its provided societal demands were quantified via value functions, which represent human judgements of supply and benefit of BES (Ananda and Herath, 2009). In the present study, the value functions of Blattert et al. (2017) were used to convert the result of the BES indicators into utility scores between 0 and 1 (with 0 indicating the lowest and 1 indicating the best provision of an indicator). MAVT is a compensatory MCDA approach (i.e., a utility decrease of one indicator can be compensated by an increase of another indicator), making it particularly suitable to analyzing trade-off situations (Blagojević et al., 2019).
To obtain the partial utilities for each BES group and overall utility at the enterprise level for each scenario (climate and management), an additive function was applied. In this approach, decision makers can express their preferences by assigning weights (λ) to the different indicators and the BES groups (Kangas et al., 2015).
Where Va,i (Ya,i) is the normalized utility for each individual indicator (i) per BES group (a) based on the value function, λa are weights of each BES indicator group, λa,i are weights of each individual indicator per BES group, na the number of individual indicators per BES group and m the number of BES groups.
The compensatory nature of the MCDA framework therefore applies to the level of individual indicators as well as to the level of BES groups. Notably, the framework is flexible and allows integrating additional indicators and BES groups, which can become important in further DSS applications.
All weights (λa, λa,i) were defined by the forest managers of each CSE using the simple multi-attribute rating technique (Kangas et al., 2015) and are shown in Table 4 (BES group weights) and Supplementary Appendix 1.5 (individual indicator weights). Further details about the stakeholder preference elicitation approach is provided in Supplementary Appendix 1.6.
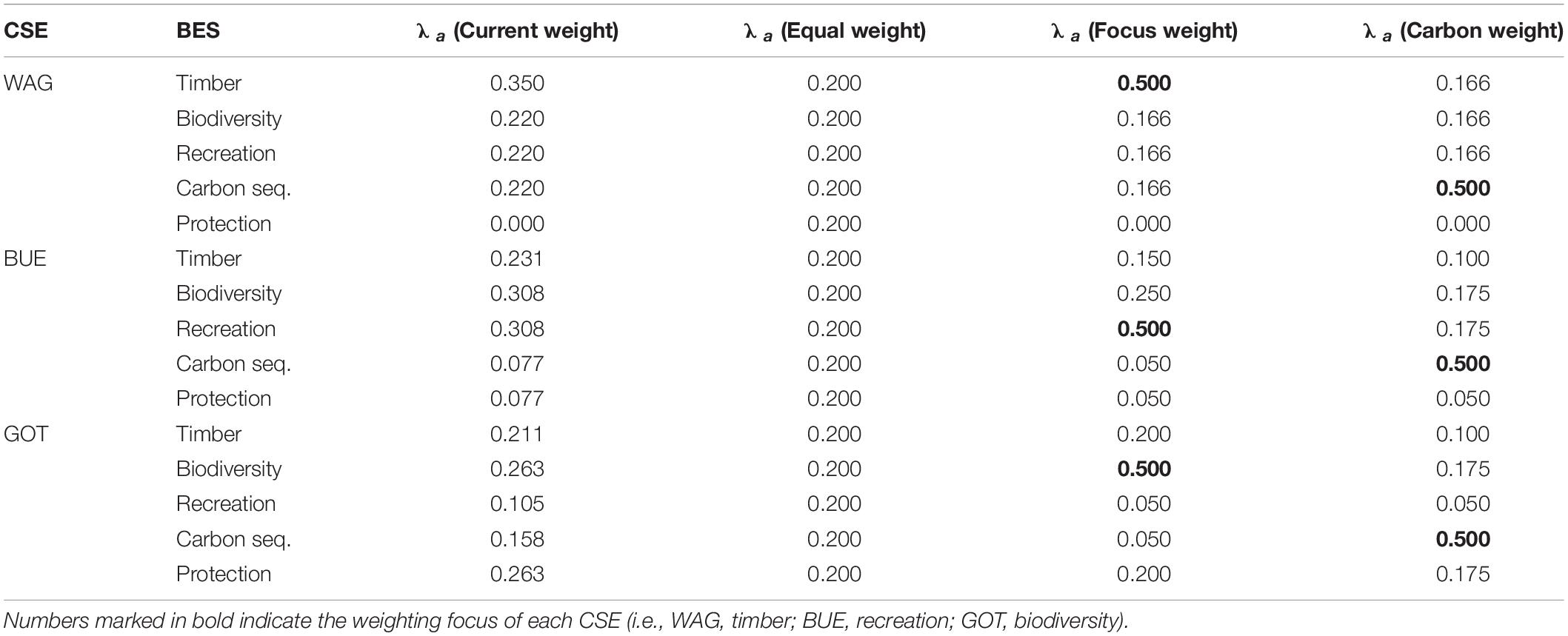
Table 4. Weights for biodiversity and ecosystem service (BES) group indicators (λa) for four alternative weighing scenarios at three case study enterprises (CSEs).
Robustness of MCDA to Shifts in Weighting Preferences
Four alternative weighting scenarios were applied for the BES groups (λa) to explore the robustness of the MCDA results to shifting demands in BES provisioning (see Table 4). The weighting scenarios were (1) ‘Current weight’, with stakeholder-defined BES weights representing the current management priorities in the CSE, (2) ‘Equal weight’, a default scenario for comparability, where each BES receives the same weight, (3) ‘Focus weight’, an increase in weight of the priority BES to 0.5 at each CSE (with priority at GOT focusing at biodiversity, see Table 4), (4) ‘Carbon weight’, an increase in the weight of carbon sequestration to 0.5. For ‘Focus weight’ and ‘Carbon weight’, all non-focus BES weights were adjusted to maintain their relative importance as defined in ‘Current weight’ by the stakeholders. All individual indicator weights within the BES groups (λa,i) were maintained as defined by the stakeholders (see Supplementary Appendix 1.5). The detailed BES weights for each scenario are provided in Table 4.
Analysis of BES Development and Trade-Offs
For further analyzing the development of the BES as well as their synergies and trade-offs, partial utilities of each BES group (i.e., timber, biodiversity, recreation, carbon sequestration, protection) were compared for each CSE, management strategy and climate change scenario. To quantify the direction and the strength of trade-offs and synergies between different BES, Spearman’s rank correlation was used, due to it is robustness against outliers.
The trade-off analysis was based on the partial utilities of the respective BES (see Figure 2), including results of all management strategies under present climate conditions (results from future climate scenarios yielded the same patterns and were therefore not shown).
The DSS framework, as well as all analyses and visualizations were conducted with R version 4.0.0 (R Core Team, 2020).
Results
Synergies and Trade-Offs Between BES Under Present Climate Conditions
Under present climate conditions, changing management intensities affected the development of all BES from 2010 to 2060, with strongest effects occurring for the BES carbon sequestration and timber production (Figure 3). An increasing management intensity led to a generally higher timber utility, but decreased the utility of carbon sequestration. Utilities of biodiversity, recreation and protection were less affected by altered management intensities and remained relatively stable over the simulation timespan. Only a minor negative effect of increasing management intensity was evident for biodiversity, as well as on recreation and protection function.
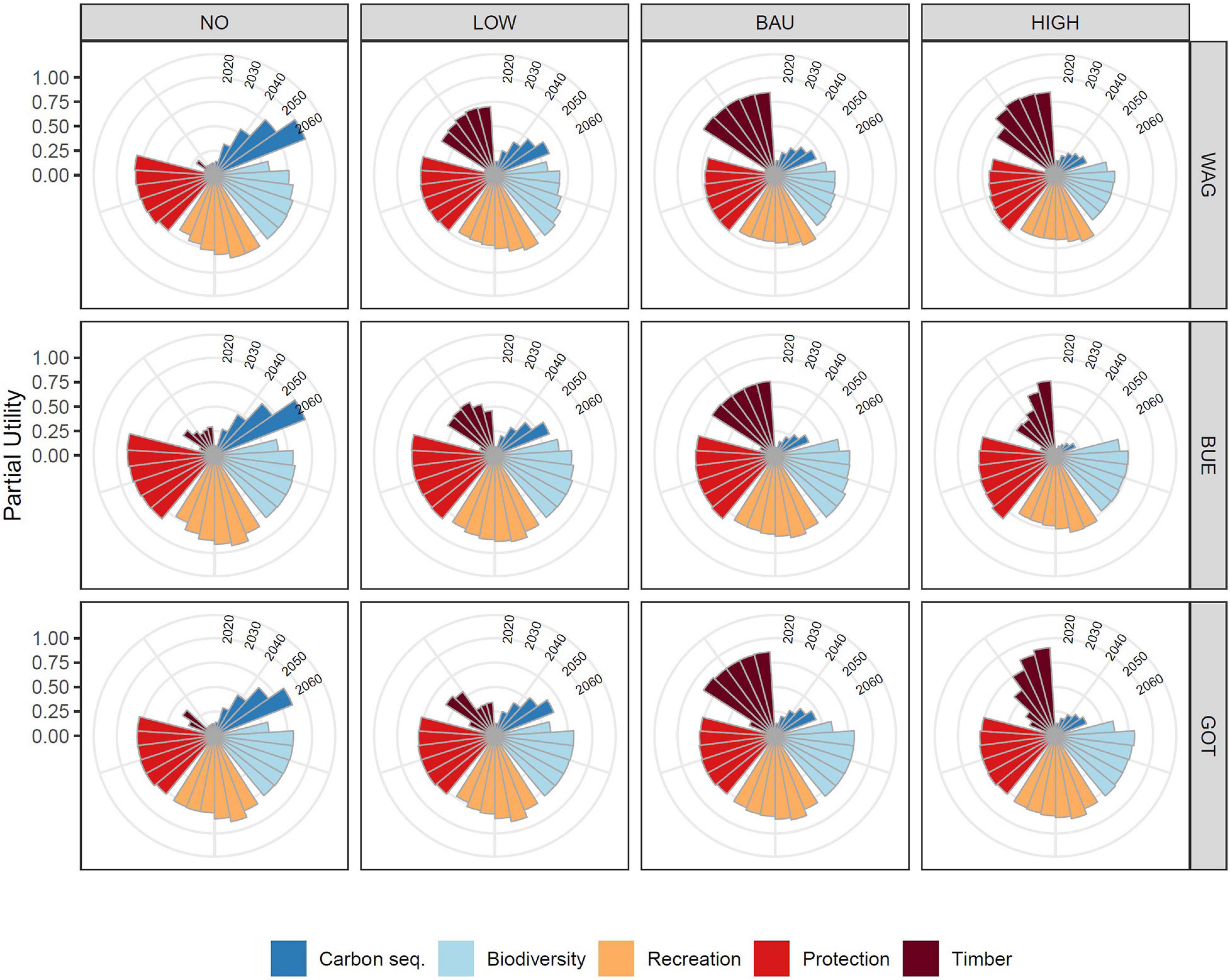
Figure 3. Partial utility of biodiversity and ecosystem service (BES) indicators for four alternative management strategies (No management: ‘NO’; Low intensity: ‘LOW’; business as usual: ‘BAU’; High intensity: ‘HIGH’) at the three case study enterprises (WAG, BUE, GOT) under present climate conditions. Bars display temporal development and colors indicate different BES. Partial utility is scaled between 0 (lowest utility) to 1 (highest utility) for comparability. Note, that the absence of bars indicates a very low partial utility.
When comparing partial utilities of BES with each other, a consistent positive relationship between biodiversity and protection, biodiversity and recreation as well as between recreation and protection was found across all CSEs, indicating a synergy among these ecosystem services (Figure 4 and Supplementary Appendix Figure 2.1). Furthermore, a positive relationship between carbon sequestration and biodiversity occurred (Figure 4). In contrast, negative relationships between carbon sequestration and timber, as well as between timber and protection function (mainly against landslides) were found. Besides, biodiversity and timber utility were negatively correlated, but the degree varied among the CSEs (Supplementary Appendix Figure 2.1). Other relationships between BES were either weak or inconsistent.
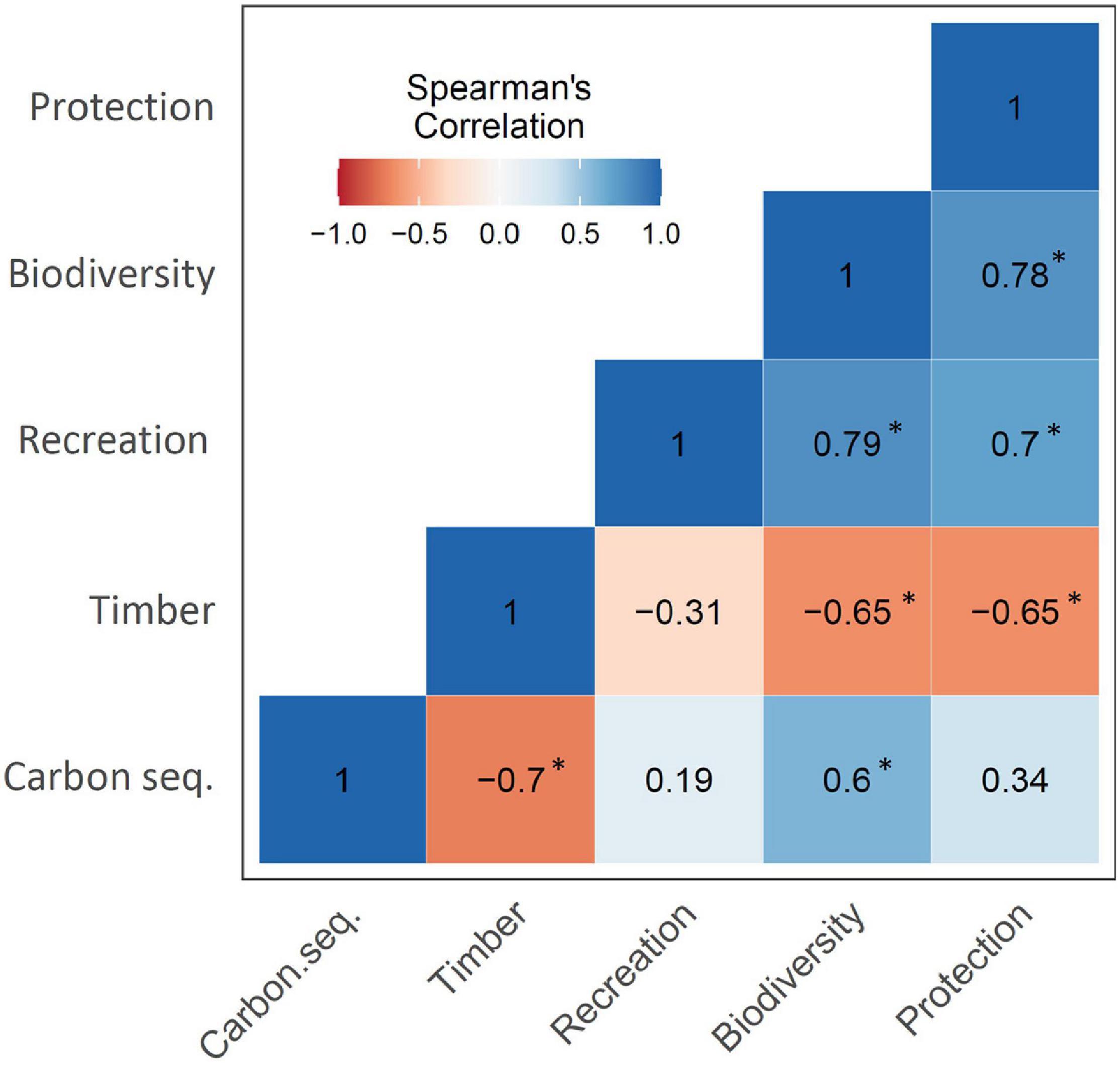
Figure 4. Spearman rank-correlation matrix between biodiversity and ecosystem service (BES) indicators for all case study enterprises (mean of partial utilities over simulation timespan for all management strategies under present climate conditions. Results from future climate scenarios were virtually identical and therefore not shown). Negative values (red) indicate a trade-off (negative correlation) and positive values (blue) a synergy (positive relationship) between BES. The strength and direction of the correlation is expressed by Spearman’s rank correlation coefficient (n = 12, with asterisk indicating significance, p < 0.05). Site-specific results are provided in Supplementary Appendix Figure 2.1.
Utility of Management Strategies
Under present climate conditions (‘PC’), the highest overall utility for all BES (based on ‘Current weights’, Table 4) at the year 2060 was found for the ‘BAU’ management strategy for the sites BUE and GOT (Figure 5). For site WAG, the ‘LOW’ strategy resulted in the highest overall utility. The lowest overall utility occurred under the ‘NO’ management strategy for all CSEs.
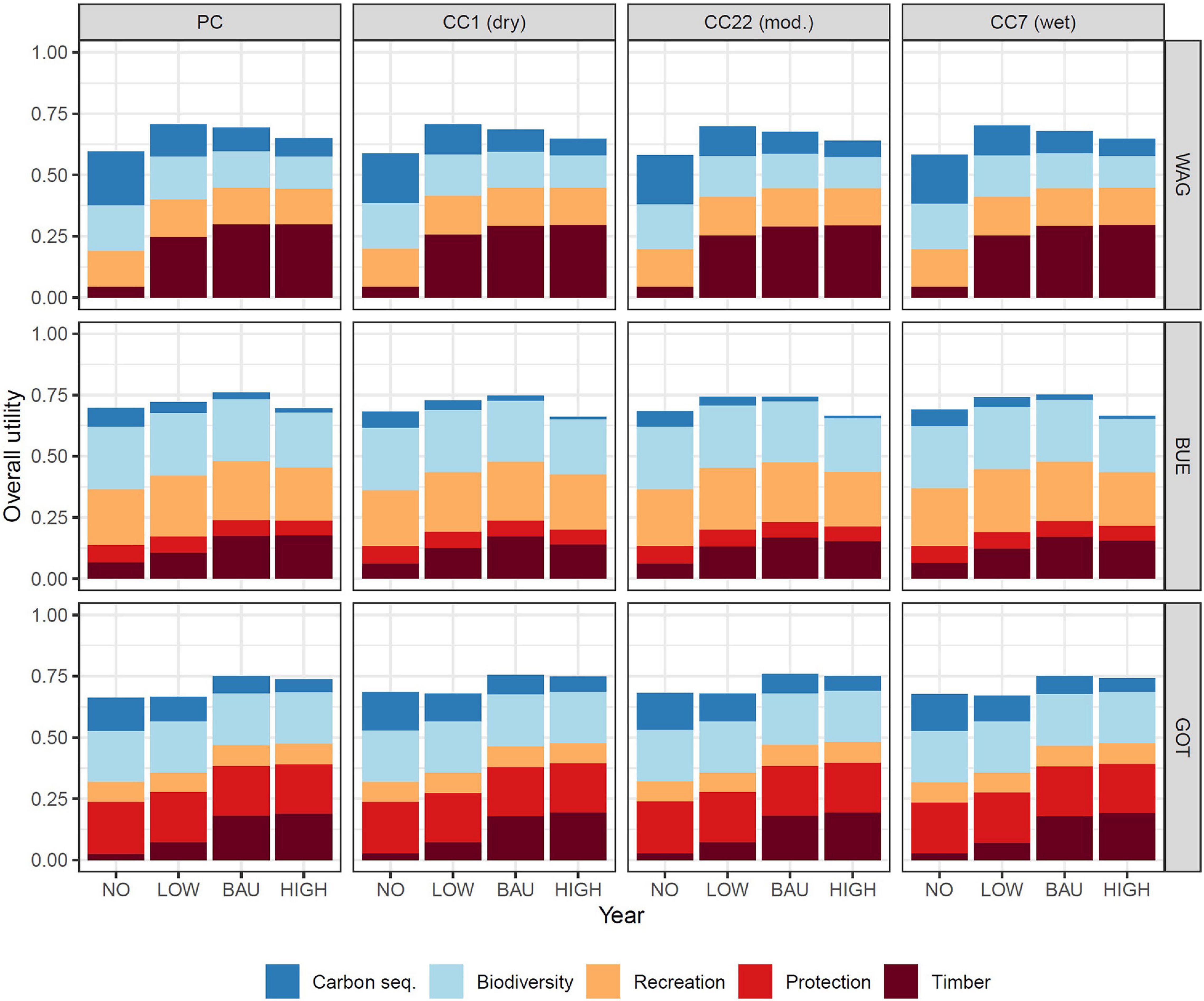
Figure 5. Overall utility, shown as the sum of weighted partial utilities (based on ‘Current weight’, see Table 4) for all biodiversity and ecosystem service (BES) indicators for four management strategies at the three case study enterprises (WAG, BUE, GOT) under present climate (PC) as well as three future climate change scenarios (CC1: dry, CC7: wet, CC22: moderate) for the year 2060.
Effects of Climate Change on BES Provisioning
Under future climate change scenarios, relatively few changes occurred until 2060 at the level of overall utility (Figure 5, based on ‘Current weight’ of BES, see Table 4). Hence, no marked changes in overall and partial utilities were found for the three CSEs with increasing climate change intensity (i.e., between the ‘wet’ CC7 and the ‘dry’ CC1 scenario). Altogether, highest overall utilities were achieved by the same management strategies for the three climate change scenarios as under present climate conditions. Notably, these patterns remained relatively stable over time, with exception of the CSE WAG, where the BAU strategy performed best for a shorter time horizon (i.e., 2030, see Supplementary Appendix Figure 2.2).
At the level of individual indicators, the effect of climate change was more apparent, leading particularly to changes of basal area (up to −6% for WAG, −10% for BUE and +6% for GOT, Figure 2.4) and productivity (up to −7% for WAG, −19% for BUE and +15% for GOT, Supplementary Appendix Figure 2.4). Furthermore, slight changes occurred for deadwood and visual permeation under the ‘dry’ CC scenario (BUE: more mortality, GOT: less mortality under the ‘dry’ CC scenario, Supplementary Appendix Figure 2.3.3).
Robustness of MCDA to Shifting Weights
Shifting the BES priorities in the MCDA (i.e., BES group weights, see weighting scenarios in Table 4) resulted in changes in overall utility until 2060 (Figure 6). Nevertheless, for the ‘Equal weight’ and ‘Focus weight’ scenarios, the best-performing management strategy remained the same as under the ‘Current weight’, i.e., ‘BAU’ for BUE and GOT as well as ‘LOW’ for WAG (Figure 6), with exception of ‘Focus weight’ for WAG, where ‘BAU’ performed best. For the ‘Carbon weight’ scenario, substantial changes occurred for all CSEs, showing an increasing overall utility with decreasing management intensity.
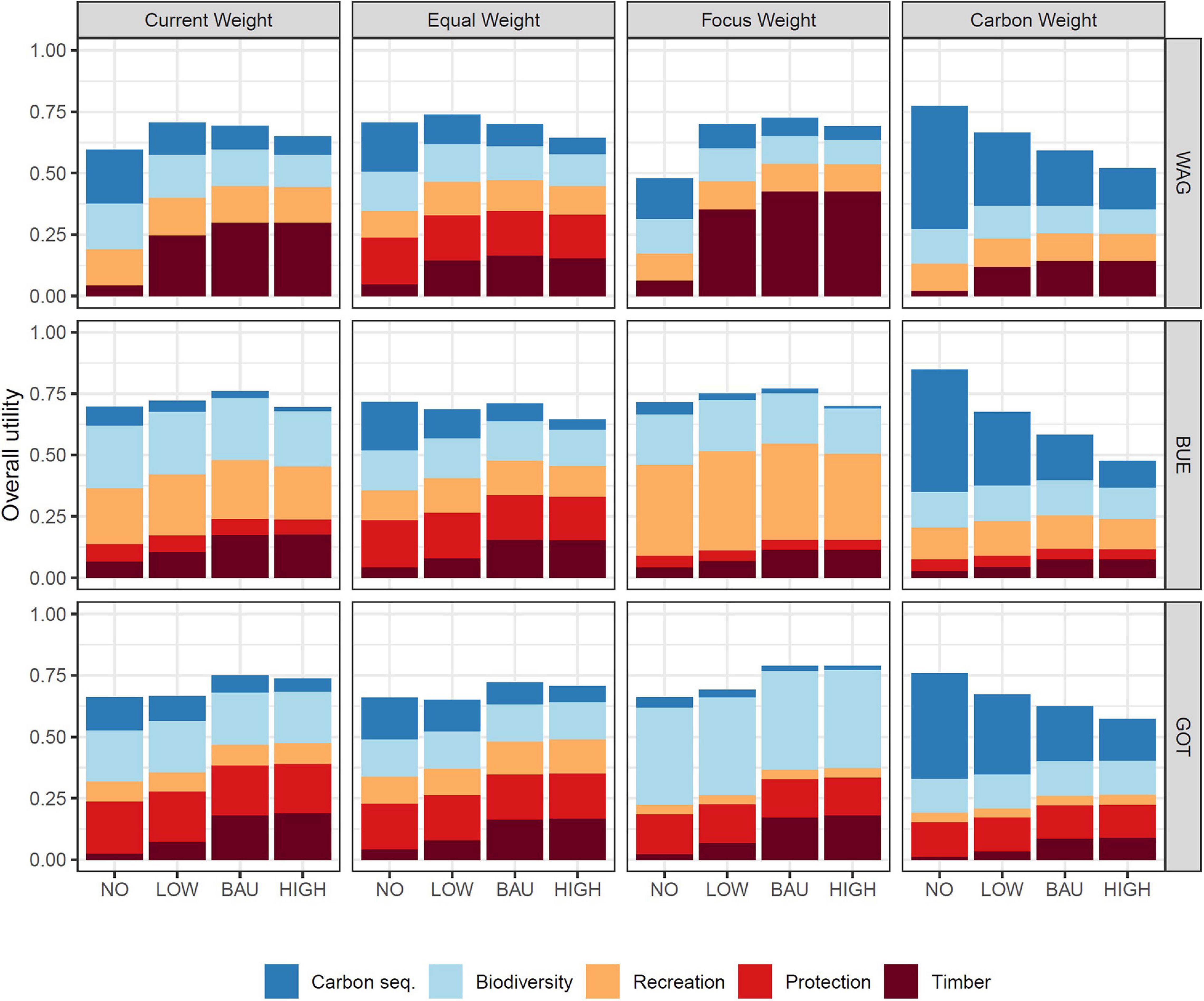
Figure 6. Overall utility for four alternative biodiversity and ecosystem service (BES) weighting preferences (Table 4), for four management strategies at three case study enterprises (WAG, BUE, GOT) under present climate (year 2060). For underlying weighting scheme, see Table 4.
Discussion
Our study demonstrated the capacity of the new DSS prototype to provide enterprise-specific scenario estimates under changing climate and alternative management strategies, enabling stakeholders to take scientifically well-founded decisions for sustainable forest management. In particular, the new DSS offers: (1) a stronger link to inventory data and forest growth conditions in Switzerland by incorporating the new forest model SwissStandSim (Zell et al., 2020), the stand initialization approach by Mey et al. (2021) and the allometric functions for tree biomass and carbon content based on the latest national forest inventory (Didion et al., 2019; Herold et al., 2019), (2) a climate sensitive framework, which is of increasing importance for strategic planning (Mina et al., 2017), (3) a widened portfolio of BES indicators, integrating also the socially important recreation function (e.g., Hegetschweiler et al., 2020), as well as an updated framework for harvested wood products and substitution effects for carbon sequestration (FOEN, 2020), and (4) a revised MCDA framework allowing stakeholders to perform trade-off analyses under different management strategies and weighting preferences. Altogether, the new DSS provides a flexible and dynamic tool for strategic (i.e., long-term) planning of sustainable forest management under changing climatic conditions and political/strategic goals. In the following sections, we discuss the DSS results in view of our research objectives and conclude with an overall discussion of limitations and potentials of the new DSS prototype.
Synergies and Trade-Offs Between BES Under Present Climate Conditions
We found on the one side synergies among BES for carbon sequestration and biodiversity as well as between biodiversity, recreation and protection function, and on the other side trade-offs of timber production with carbon sequestration, biodiversity and protection function for the considered time horizon until 2060. Previous studies found similar synergies and trade-offs in European mountain forests, for instance Mina et al. (2017) and Irauschek et al. (2017), reporting a positive relationship between increasing carbon sequestration and biodiversity indicators (e.g., for the number of habitat trees and deadwood amount), as well as a negative relationship of carbon sequestration with timber use. Similarly, a larger-scale study by Gutsch et al. (2018) found a synergy between carbon sequestration and biodiversity, and a trade-off between timber use and biodiversity conservation in forests of Central-Europe. We furthermore found a synergy between biodiversity and recreation, which varied between the CSEs. These case-study specific effects are related to different tree species settings: while the lower elevation forests enterprises (WAG, BUE) have a relatively high species diversity (mixed-broadleaved stands), the higher elevation enterprise GOT is characterized by a lower species diversity (dominated by Picea abies). Furthermore, the weighting for biodiversity at GOT focused more on the number of habitat trees and deadwood availability, which was overall high and thus led to a high biodiversity utility. These aspects explained the relatively little variation for biodiversity utility at GOT, and hence the absence of a synergy between biodiversity and recreation at this CSE. At both other CSEs, the positive relationship between those services was due to the beneficial effect of a higher natural deadwood amount as well as a high species and structural diversity for both, biodiversity (e.g., Müller and Bütler, 2010; Haeler et al., 2021) and recreation (Edwards et al., 2012). It has to be noted, however, that in case of biodiversity, e.g., including a wider range of indicators with a focus on light-demanding species with habitats in more open forests (e.g., herbaceous and insect species, Hilmers et al., 2018) could significantly change the relationship or even revert a trade-off into a synergy. The reported trade-offs should therefore be considered with care, keeping the case-study specific nature of the results in mind.
A noticeable trade-off between utility of timber use and protection function occurred in our CSEs, although to a relatively small degree. A similar negative effect was found by Mina et al. (2017) in a case study in the western Alps, where protection against rockfall and avalanches are of primary importance. In contrast to their study, protection function in our lowland and pre-Alpine CSEs mainly focused on protection from landslides and erosion. Since the landslide indicator is directly linked to canopy cover (Blattert et al., 2017; Irauschek et al., 2017), an increasing timber use led to a slight decrease in average canopy cover, which explained the negative relationship. Studies focusing on the longer-term relationship between forest development and protection against rockfall or avalanches however emphasize the importance of natural regeneration fostered by close-to-nature management in Swiss mountain forests (Frehner et al., 2005; Thrippleton et al., 2020).
Altogether, our DSS results are in good accordance with other studies investigating BES synergies and trade-offs in Central European and Alpine regions. The case-study specific results and utility-based framework of the DSS can help the forest managers to evaluate particularly the strength of trade-offs occurring in their enterprise under their specifically defined BES weightings.
Carbon Sequestration
In recent years, the role of forests in carbon sequestration and their contribution to comply with the Kyoto Protocol targets have received considerable political interest (Rogiers et al., 2015; Nabuurs et al., 2017). The Swiss forest strategy 2020 defined the goal to mobilize the sustainable harvest potentials and promoted the use of wood for construction purposes or substituting non-wood products and fossil energy carriers and account for its greenhouse gas mitigation potentials (Taverna et al., 2007; FOEN, 2013).
Over the considered time horizon of 50 years, our results implied an increasing utility of carbon sequestration by reducing management intensity, i.e., by storing the carbon ‘in-situ’ in above and belowground biomass of the forest ecosystem. Previous studies considering only ‘in-situ’ carbon sequestration (e.g., Mina et al., 2017; Gutsch et al., 2018) or ‘in-situ’ as well as ‘ex-situ’ carbon sequestration (Seidl et al., 2007) reported similar results in other regions of Central Europe. However, larger-scale assessments (with time spans ≥ 100 years) found that storing carbon in wood products and substitution effects may in fact be the preferable strategy in the long term (e.g., Werner et al., 2010; Weiss et al., 2020). A lower management intensity may lead to a large buildup of carbon in the standing stock in the short to mid-term (e.g., a few decades), which becomes however progressively instable and prone of turning into a large carbon source when stands disintegrate (e.g., due to disturbance impacts, Seidl et al., 2017). Furthermore, the substitution effect accumulates and becomes increasingly important over longer time horizons (Werner et al., 2010). However, it is also important for wood products to consider efficient resource utilization and long life-spans to improve their greenhouse gas footprint (Weiss et al., 2020). In view of the increasing disturbance frequency and intensity under future climate conditions (Seidl et al., 2017) as well as the rising importance of wood as a sustainable resource for bio-economy (Nabuurs et al., 2017), a sustainable use of the harvest potential accounting for trade-offs with other BES appears to be a prudent long-term strategy.
Our study demonstrated how the DSS can be used to evaluate alternative management strategies for carbon sequestration from the perspective of a forest enterprise. Further extensions are however necessary for a more comprehensive evaluation of the soil carbon pool, which is currently not considered, and which stores on average an equal amount of carbon as the living biomass in Switzerland (Thürig and Kaufmann, 2010). Although forest management effects on changes in the soil carbon pools are typically rather low compared to aboveground biomass (Jandl et al., 2007), some studies emphasize the importance of considering soil carbon pools in response to management (e.g., Pukkala, 2014). The DSS could be extended in this respect by coupling it to a dynamic soil carbon model, such as Yasso07 (e.g., Didion et al., 2014) or other soil biochemical models, which could also allow to address the important topic of nutrient removal by forest management (Wilpert et al., 2018).
To upscale the contribution of single forest enterprises to Switzerland and to evaluate the Swiss potential of forest carbon sequestration utility, system boundaries have to be adapted. A more sophisticated approach for the initialization of harvested wood product pool would for example be required (see e.g., Thürig and Kaufmann, 2010; Blattert et al., 2020). For the single forest enterprise, however, the current approach represents an important step toward considering the entire value chain from the forest ecosystem to wood products, energy and substitution effects (Nabuurs et al., 2017). Moreover, our DSS provides a helpful tool to reach the goals of the Swiss Forest Strategy (FOEN, 2013), i.e., assessing the sustainability of different management alternatives and accounting for their carbon sequestration potentials.
Utility of Management Strategies and Robustness to Shifting BES Priorities
The results of our study showed that the BAU management strategy performed best for the lowland site BUE and for the Pre-Alpine site GOT, while the strategy ‘LOW’ was best for WAG. Notably, these results were relatively robust to shifts in the BES weighting priorities, with exception of the ‘Carbon focus’ preference. Studies using a similar MCDA approach to evaluate management strategies in other regions of Europe found partly larger differences in overall utilities between alternative management strategies (e.g., Fürstenau et al., 2007) and in response to shifting weights (e.g., Langner et al., 2017). In comparison to most other European countries, forest conditions and silviculture in Switzerland are special due to the large proportion of mountainous forests and their protection function (FOEN, 2015a) as well as the long history of sustainable forestry and focus on multifunctionality (Forest law of 1874, 1902 and 1965) via a close-to-nature management (Ott et al., 1997). This focus of multifunctional management is also reflected in the close similarity of the ‘Current weight’ and the ‘Equal weight’ scenarios (giving all BES equal importance) in our analysis (see Table 4).
Another aspect emphasized by our results is the importance of management for BES-provisioning. Although less evident at the level of overall utility, this was important for the social service of recreation, which we assessed in terms of visual attractiveness (Edwards et al., 2012). While a high degree of naturalness in forests (high species and size diversity, natural deadwood) is generally perceived as positive for visual attractiveness, a good visual permeation into the forest is frequently noted as very important as well (e.g., Gundersen and Frivold, 2008). In our study, an intermediate management intensity (‘BAU’) was found most suitable to prevent stands from becoming too dense (negative for visual permeation), which caused a decrease of recreation value, e.g., for the ‘NO’ management at WAG over time. On the other hand, a too strong increase in management intensity can impact recreation function negatively again, due to large amounts of harvest residuals and unnatural canopy openings (e.g., Kangas and Niemelainen, 1996). Our study therefore underlines the importance of a continuous management of forest in a sustainable, close-to-nature way, which best promoted multifunctionality in our case study enterprises.
Climate Change Effects on BES Provisioning
We found generally small effects of climate change scenarios on the simulated partial and overall BES utilities. This is at first glance surprising, given that the scenarios included a significantly drier ‘high impact’ (RCP 8.5) scenario (CC1, Brunner et al., 2019), which could be expected to cause substantial changes in forest structure and composition (Bugmann et al., 2014; IPCC, 2014b). However, it is important to differentiate between the level of individual indicators and the more aggregated level of partial and overall utility when considering climate change impacts.
At the level of individual BES indicators, climate change caused an increase in mortality at the low-elevation enterprise BUE for the ‘dry’ (CC1) scenario, which increased deadwood availability and decreased the number of habitat trees. In contrast, the higher-elevation enterprise GOT responded with higher growth rates and less mortality to the CC1 scenario. These elevation-specific results are in line with other studies on climate change impacts on forests in Switzerland (Rigling et al., 2013; Etzold et al., 2019; Huber et al., 2021) and in other European countries (e.g., Mina et al., 2017). Notably, the elevational patterns of climate change in this study are also related to the prevalent tree species, which show a distinctively different response to changes in climate (Zell, 2016). In the longer-term (i.e., time scales exceeding the focus of the present study), it is furthermore possible, that enterprises like the higher-elevation, currently spruce-dominated GOT experience a profound compositional shift from coniferous-dominated to broadleaved-dominated stands, with considerable impacts on forest structure and ecosystem service provisioning (see also Albrich et al., 2020). The aspect of species shifts therefore represents an additional uncertainty which warrants further investigation in long-term applications of the DSS under climate change.
At the level of overall utility, the number of individual indicators and the hierarchical structure of the MCDA approach can however have a buffering effect on the overall results (see also Fürstenau et al., 2007). Our study included a wide set of indicators, many of which were less climate-sensitive at the considered timescale (e.g., tree species diversity), leading to a diminished climate change impact signal. Further, the MCDA approach is compensatory, which means that indicators with opposite developmental trends can cancel each other out (see e.g., Blagojević et al., 2019). This was for instance the case for the CC1 scenario on biodiversity in BUE, where climate change induced mortality led to a decrease of alive habitat trees (e.g., negative for species inhabiting the crown area, Bütler et al., 2020) and an increase in natural deadwood (e.g., positive for saproxylic species, Haeler et al., 2021). Further reasons for the relatively small climate change impacts were: (1) a relatively short time horizon (50 years), whereas most severe climate change impacts are expected by the end of the 21st century for Switzerland (CH2018, 2018); (2) the climate data used in the forest modeling, which was based on 5 year averages (Zell et al., 2020) and does not represent climatic extreme events; and (3) the problem of a lack of observation data regarding warmer and drier climatic conditions in the calibration range of the forest model, which can lead to underestimated climate change impacts under extreme scenarios (Adams et al., 2013). A similarly small effect of climate change was found in a recent DSS study by Lundholm et al. (2020), which was also based on an empirical growth and yield model, while studies using process-based dynamic models typically report more severe climate change impacts on BES provisioning (e.g., Fürstenau et al., 2007; Irauschek et al., 2017; Mina et al., 2017). Nevertheless, the simulated basal area changes found in our study are in good agreement with a recent study on climate change impacts on Swiss forests by Huber et al. (2021) using a process-based model and reporting basal area changes of a similar magnitude for 2070. Furthermore, it has to be noted, that despite recent progress, large uncertainties still surround the modeling of tree mortality under climate change (Adams et al., 2013; Hartmann et al., 2018). This is especially the case, when different disturbance impacts are considered, e.g., by windthrows, wildfires and bark beetles (e.g., Temperli et al., 2013; Thom et al., 2017). The aspect of vulnerability of stands to disturbances is therefore another key point for further developments of the DSS, and approaches like the windthrow and bark beetle vulnerability index by Temperli et al. (2020) could be integrated into the MCDA framework.
Importance, Limitations and Future Potentials of the New DSS
DSS are becoming increasingly essential tools for forest managers to cope with the complexity of the planning situation (Vacik and Lexer, 2014). In recent years, a large number of DSS for forest management worldwide have been reviewed (e.g., Borges et al., 2014). According to a review by Segura et al. (2014), key issues to improve the practical relevance of DSS are to strengthen the link to empirical data underlying the system and to increase multiple criteria for a comprehensive evaluation of forest BES. Our DSS fulfills these claims by providing a strong link to data from forest inventories at the national and enterprise level (Brändli et al., 2020; Mey et al., 2021) and forest growth in Switzerland (Zell et al., 2020), as well as by increasing a wide portfolio of BES indicators and value functions for Switzerland (Blattert et al., 2017), thereby providing a tool for a holistic evaluation of sustainable forest management. With its focus on strategic planning at the enterprise level, it complements other DSS in Switzerland (Heinimann et al., 2014) focusing more on operational planning (e.g., WIS2, Rosset et al., 2014) and model applications at the local to national scale (e.g., Huber et al., 2021). By integrating a utility-based MCDA framework, our DSS provided condensed results to the forest manager, thereby balancing the problem of increasing complexity and the need for simplicity in communication (Vacik and Lexer, 2014).
A limitation of the current prototype version of the DSS is the software implementation, which does not feature a simple user-friendly design yet, and can require adaptations for applications to different regions of Switzerland. Main barriers for a simple applicability of the system are in particular: (1) the differences in input data which partly exist between the cantons, especially in respect to local forest inventory datasets, (2) the rapidly increasing computational time with increasing enterprise size and increasing scenario assessments, which currently prevents a real-time application, (3) the incorporation of additional BES indicators and value functions, which requires programming experience. A further development of the DSS prototype is therefore recommended. Improvements comprise an automatic handling of input data (e.g., inventory datasets), a more efficient software framework for faster computation, and an extension of the indicator framework. Additional indicators could for instance address further aspects of timber production (e.g., sustainability indicators for forest operations, Schweier et al., 2019), forest infrastructure (Bont et al., 2019), biodiversity (e.g., species associated with different successional stages, Hilmers et al., 2018), recreation (e.g., Hegetschweiler et al., 2020) as well as soil- and water related indicators (e.g., groundwater recharge, Schwaiger et al., 2018). Further aspects of key importance for forest managers are information about the uncertainty of the DSS results (Knoke et al., 2016), as well as the monetarization of BES (Gret-Regamey et al., 2017). Fast progress in other scientific fields also offer ample opportunities for DSS (Vacik and Lexer, 2014), such as remote sensing which provides high-resolution data at the level of forest enterprises (e.g., Bont et al., 2020).
The procedure outlined in this study is well suited for evaluating different silvicultural strategies for an entire enterprise. If different management strategies are to be used at the same time, the question arises at which exact locations the respective strategies are to be implemented best. To solve such challenging combinatorial problems, optimization models can be used, as described for example by Knoke et al. (2016) for robust optimization or Pohjanmies et al. (2019) to reconcile economic and conservation objectives. For communication with stakeholders, a furthermore highly promising development are visualization systems at the stand and enterprise scale (e.g., Pretzsch et al., 2008). Due to the increasing level of immersivity of visualization systems, results of DSS can become more intuitive for forest managers (Fabrika et al., 2019), thus helping to shape their vision for strategic planning.
Conclusion
In this study, we presented a new multi-criteria DSS prototype for strategic (long-term) planning at the forest enterprise level for Switzerland, which offers various possibilities to explore the effect of alternative management strategies, climate change scenarios and shifts in political focus on multiple BES provisioning. The DSS provides a MCDA evaluation framework, which enables forest managers to assess the consequences of different management strategies and to evaluate synergies and trade-offs among management objectives. The DSS furthermore provides the scientific foundation for a transparent decision making and communication, which is of increasing relevance for forest stakeholder interactions. Due to its strong empirical foundation and flexible architecture, the DSS can be applied in lowland as well as mountain forest enterprises. Consequently, next steps will be the application of the DSS to further enterprises in Switzerland as well as extending the indicator and MCDA framework and improve its user-friendliness for forest planners in Switzerland.
Data Availability Statement
The original contributions presented in the study are included in the article/Supplementary Material, further inquiries can be directed to the corresponding author/s.
Author Contributions
TT, JS, and ET: conceptualization of the study. TT, RM, JZ, and LB: data and DSS preparation for the CSEs. TT: simulation and data analysis and writing of the first manuscript draft. TT, CB, and LB: MCDA analysis. ET: funding acquisition. JS and ET: project administration. All authors contributed substantially to the writing of the article and approved the submitted version.
Funding
This study was conducted in the framework of the NRP 73 ‘Sustainable Economy’ project SessFor funded by the Swiss National Science Foundation (SNF), 407340_172372.
Conflict of Interest
The authors declare that the research was conducted in the absence of any commercial or financial relationships that could be construed as a potential conflict of interest.
Publisher’s Note
All claims expressed in this article are solely those of the authors and do not necessarily represent those of their affiliated organizations, or those of the publisher, the editors and the reviewers. Any product that may be evaluated in this article, or claim that may be made by its manufacturer, is not guaranteed or endorsed by the publisher.
Acknowledgments
We thank Laura Ramstein, Julian Muhmenthaler, and Anton Bürgi from the WSL group Sustainable forestry, as well as Golo Stadelmann, Christian Temperli and Markus Didion from the WSL group resource analysis for valuable discussions about the development of the DSS. Furthermore, Raphaela Tinner and Sabrina Maurer (Canton Zug, forest management GOT) as well as Denise Lüthy, Anja Bader (Canton Zürich), Thomas Kuhn (forest manager BUE), and Anton Bürgi (forest manager WAG) are gratefully acknowledged for providing the forest inventory data, defining the management strategies and BES weights for the simulations and providing helpful feedback to the results. We would like to thank Marjo Kunnala and Nele Rogiers (FOEN) as well as Frank Werner for their help with the harmonization of the carbon sequestration approach with the framework applied by the Swiss Federal Office for the Environment. We also thank two reviewers for their helpful comments on an earlier version of the manuscript.
Supplementary Material
The Supplementary Material for this article can be found online at: https://www.frontiersin.org/articles/10.3389/ffgc.2021.693020/full#supplementary-material
References
Adams, H. D., Williams, A. P., Xu, C. G., Rauscher, S. A., Jiang, X. Y., and McDowell, N. G. (2013). Empirical and process-based approaches to climate-induced forest mortality models. Front. Plant Sci. 4:438.
Albrich, K., Rammer, W., and Seidl, R. (2020). Climate change causes critical transitions and irreversible alterations of mountain forests. Glob. Change Biol. 26, 4013–4027. doi: 10.1111/gcb.15118
Ananda, J., and Herath, G. (2009). A critical review of multi-criteria decision making methods with special reference to forest management and planning. Ecol. Econ. 68, 2535–2548. doi: 10.1016/j.ecolecon.2009.05.010
Biber, P., Felton, A., Nieuwenhuis, M., Lindbladh, M., Black, K., Bahýl, J., et al. (2020). Forest biodiversity, carbon sequestration, and wood production: modeling synergies and trade-offs for ten forest landscapes across Europe. Front. Ecol. Evol. 8:e547696.
Bircher, N. (2015). To Die Or Not To Die – Forest Dynamics In Switzerland Under Climate Change. Ph.D. thesis No 22775. Zurich: ETH Zurich.
Blagojević, B., Jonsson, R., Björheden, R., Nordström, E. M., and Lindroos, O. (2019). Multi-criteria decision analysis (MCDA) in forest operations – an introductional review. Croatian J. For. Eng. 40, 191–205.
Blattert, C., Lemm, R., Thees, O., Hansen, J., Lexer, M. J., and Hanewinkel, M. (2018). Segregated versus integrated biodiversity conservation: value-based ecosystem service assessment under varying forest management strategies in a Swiss case study. Ecol. Indic. 95, 751–764. doi: 10.1016/j.ecolind.2018.08.016
Blattert, C., Lemm, R., Thees, O., Lexer, M. J., and Hanewinkel, M. (2017). Management of ecosystem services in mountain forests: review of indicators and value functions for model based multi-criteria decision analysis. Ecol. Indic. 79, 391–409. doi: 10.1016/j.ecolind.2017.04.025
Blattert, C., Lemm, R., Thürig, E., Stadelmann, G., Brändli, U. B., and Temperli, C. (2020). Long-term impacts of increased timber harvests on ecosystem services and biodiversity: a scenario study based on national forest inventory data. Ecosyst. Serv. 45, 1–13.
Bont, L. G., Hill, A., Waser, L. T., Burgi, A., Ginzler, C., and Blattert, C. (2020). Airborne-laser-scanning-derived auxiliary information discriminating between broadleaf and conifer trees improves the accuracy of models for predicting timber volume in mixed and heterogeneously structured forests. For. Ecol. Manag. 459, 1–18.
Bont, L. G., Maurer, S., and Breschan, J. R. (2019). Automated cable road layout and harvesting planning for multiple objectives in steep terrain. Forests 10, 1–26.
Borges, J. G., Nordström, E. M., Garcia-Gonzalo, J., Hujala, T., and Trasobares, A. (2014). Computer-Based Tools For Supporting Forest Management. Report Of Cost Action Fp 0804 Forest Management Decision Support Systems (FORSYS). Umeå: Swedish University of Agricultural Sciences, Dept of Forest Resource Management, 507.
Brändli, U.-B., Abegg, M., and Allgaier Leuch, B. (2020). Schweizerisches Landesforstinventar. Ergebnisse der Vierten Erhebung 2009–2017. Birmensdorf: Eidgenössische Forschungsanstalt für Wald, Schnee und Landschaft WSL. Bundesamt für Umwelt, 341.
Brun, P., Psomas, A., Ginzler, C., Thuiller, W., Zappa, M., and Zimmermann, N. E. (2020). Large-scale early-wilting response of Central European forests to the 2018 extreme drought. Glob. Change Biol. 26, 7021–7035. doi: 10.1111/gcb.15360
Brunner, M. I., Gurung, A. B., Zappa, M., Zekollari, H., Farinotti, D., and Stahli, M. (2019). Present and future water scarcity in Switzerland: potential for alleviation through reservoirs and lakes. Sci. Total Environ. 666, 1033–1047. doi: 10.1016/j.scitotenv.2019.02.169
Bugmann, H., Brang, P., Elkin, C. M., and Henne, P. D. (2014). “Climate change impacts on tree species, forest properties, and ecosystem services,” in CH2014-Impacts, Toward Quantitative Scenarios of Climate Change Impacts in Switzerland, eds C. C. Raible and K. M. Strassmann (Bern: OCCR, FOEN, MeteoSwiss, C2SM, Agroscope, and ProClim), 79–90.
Bütler, R., Lachat, T., Krumm, F., Kraus, D., and Larrieu, L. (2020). Habitatbäume kennen, schützen und fördern. Merkblatt für die Praxis 64, 1–12. doi: 10.1007/bf00445730
CH2018 (2018). CH2018 – Climate Scenarios for Switzerland, Technical Report. Zurich: National Centre for Climate Services, 271.
Didion, M., Frey, B., Rogiers, N., and Thürig, E. (2014). Validating tree litter decomposition in the Yasso07 carbon model. Ecol. Modelling 291, 58–68. doi: 10.1016/j.ecolmodel.2014.07.028
Didion, M., Herold, A., and Thürig, E. (2019). “Whole tree biomass and carbon stock,” in Swiss National Forest Inventory – Methods And Models Of The Fourth Assessment, eds C. Fischer and B. Traub (Cham: Springer), 243–248. doi: 10.1007/978-3-030-19293-8_14
Edwards, D., Jay, M., Jensen, F. S., Lucas, B., Marzano, M., Montagne, C., et al. (2012). Public preferences for structural attributes of forests: towards a pan-European perspective. For. Policy Econ. 19, 12–19. doi: 10.1016/j.forpol.2011.07.006
Eriksson, L. O., and Borges, J. G. (2014). “Computerized decision support tools to address forest management planning problems: history and approach for assessing the state of art world-wide. Report of Cost Action FP 0804 Forest Management Decision Support Systems,” in computer-based tools for supporting forest management. The Experience And The Expertise World-Wide, eds J. G. Borges, E. M. Nordström, J. Garcia-Gonzalo, T. Hujala, and A. Trasobares (Umeå: Swedish University of Agricultural Sciences), 3–15.
Etzold, S., Zieminska, K., Rohner, B., Bottero, A., Bose, A. K., Ruehr, N. K., et al. (2019). One century of forest monitoring data in switzerland reveals species- and site-specific trends of climate-induced tree mortality. Front. Plant Sci. 10:307.
Fabrika, M., Valent, P., and Merganičova, K. (2019). Forest modelling and visualisation – state of the art and perspectives. Cent. Eur. For. J. 65, 147–165. doi: 10.2478/forj-2019-0018
FOEN (2013). Forest Policy 2020. Visions, Objectives And Measures For The Sustainable Management Of Forests In Switzerland. Fede. Bern: Federal Office for the Environment, 66.
FOEN (2015a). Forest Report 2015 – Condition And Use Of Swiss Forests, eds A. Rigling and H. P. Schaffer (Bern: Federal Office for the Environment FOEN).
FOEN (2015b). Stickstoffdeposition - Modellierte Gesamtdeposition Von Stickstoff Für Das Bezugsjahr 2015. Bern: Federal Office for the Environment.
FOEN (2020). Harvested Wood Products (HWP) in The Swiss National Inventory Report 2020 (GHG inventory 1990-2018). Bern: Forest Division, Federal Office for the Environment FOEN.
Forrester, D. I., Nitzsche, J., and Schmid, H. (2019). The Experimental Forest Management Project: An Overview And Methodology Of The Longterm Growth And Yield Plot Network. Birmensdorf: Swiss Federal Institute of Forest, Snow and Landscape Research WSL, 73.
Frehner, M., Wasser, B., and Schwitter, R. (2005). Nachhaltigkeit im Schutzwald (Projekt NaiS). Bern: Bundesamt für Umwelt BAFU.
Fürstenau, C., Badeck, F. W., Lasch, P., Lexer, M. J., Lindner, M., Mohr, P., et al. (2007). Multiple-use forest management in consideration of climate change and the interests of stakeholder groups. Eur. J. For. Res. 126, 225–239. doi: 10.1007/s10342-006-0114-x
Gret-Regamey, A., Siren, E., Brunner, S. H., and Weibel, B. (2017). Review of decision support tools to operationalize the ecosystem services concept. Ecosyst. Serv. 26, 306–315. doi: 10.1016/j.ecoser.2016.10.012
Gudmundsson, L., Bremnes, J. B., Haugen, J. E., and Engen-Skaugen, T. (2012). Technical Note: downscaling RCM precipitation to the station scale using statistical transformations – a comparison of methods. Hydrol. Earth Syst. Sci. 16, 3383–3390. doi: 10.5194/hess-16-3383-2012
Gundersen, V. S., and Frivold, L. H. (2008). Public preferences for forest structures: a review of quantitative surveys from Finland, Norway and Sweden. Urban For. Urban Green. 7, 241–258. doi: 10.1016/j.ufug.2008.05.001
Gutsch, M., Lasch-Born, P., Kollas, C., Suckow, F., and Reyer, C. P. O. (2018). Balancing trade-offs between ecosystem services in Germany’s forests under climate change. Environ. Res. Lett. 13, 1–12.
Haeler, E., Bergamini, A., Blaser, S., Ginzler, C., Hindenlang, K., Keller, C., et al. (2021). Saproxylic species are linked to the amount and isolation of dead wood across spatial scales in a beech forest. Landsc. Ecol. 36, 89–104. doi: 10.1007/s10980-020-01115-4
Hanewinkel, M., and Kammerhofer, A. W. (2015). “Forest report 2015 – chapter 3: use,” in Forest Report 2015. Condition and Use of Swiss Forests, eds A. S. Rigling and H. P. Schaffer (Bern: Federal Office of the Environment (FOEN), Swiss Federal Institute WSL), 144.
Hartmann, H., Moura, C. F., Anderegg, W. R. L., Ruehr, N. K., Salmon, Y., Allen, C. D., et al. (2018). Research frontiers for improving our understanding of drought-induced tree and forest mortality. New Phytol. 218, 15–28. doi: 10.1111/nph.15048
Hegetschweiler, K. T., Fischer, C., Moretti, M., and Hunziker, M. (2020). Integrating data from National Forest Inventories into socio-cultural forest monitoring – a new approach. Scand. J. For. Res. 35, 274–285. doi: 10.1080/02827581.2020.1799066
Heinimann, H. R., Roset, C., Brang, P., and Trasobares, A. (2014). “The design and use of forest decision support systems in Switzerland. Computer-based tools for supporting forest management,” in Report of Cost Action FP 0804 Forest Management Decision Support Systems (FORSYS), eds J. G. Borges, E. M. Nordström, J. Garcia-Gonzalo, T. Hujala, and A. Trasobares (Umeå: Swedish University of Agricultural Sciences, Dept of Forest Resource Management), 420–440.
Herold, A., Zell, J., Rohner, B., Didion, M., Thürig, E., and Rösler, E. (2019). “State and change of forest resources,” in Swiss National Forest Inventory – Methods And Models Of The Fourth Assessment, eds C. Fischer and B. Traub (Cham: Springer), 205–230.
Hilmers, T., Friess, N., Bassler, C., Heurich, M., Brandl, R., Pretzsch, H., et al. (2018). Biodiversity along temperate forest succession. J. Appl. Ecol. 55, 2756–2766. doi: 10.1111/1365-2664.13238
Huber, N., Bugmann, H., Cailleret, M., Bircher, N., and Lafond, V. (2021). Stand-scale climate change impacts on forests over large areas: transient responses and projection uncertainties. Ecol. Appl. 31, 1–19. doi: 10.1002/eap.2313
IPCC (2014a). 2013 Revised Supplementary Methods and Good Practice Guidance Arising from the Kyoto Protocol, eds T. Hiraishi, T. Krug, K. Tanabe, N. Srivastava, J. Baasansuren, M. Fukuda, et al. (Geneva: Intergovernmental Panel on Climate Change IPCC), 268.
IPCC (2014b). Climate Change 2014: Impacts, Adaptation, and Vulnerability, Pt A: Global and Sectoral Aspects. Geneva: IPCC, 1–1131.
Irauschek, F., Rammer, W., and Lexer, M. J. (2017). Can current management maintain forest landscape multifunctionality in the Eastern Alps in Austria under climate change? Regional Environ. Change 17, 33–48. doi: 10.1007/s10113-015-0908-9
Jandl, R., Lindner, M., Vesterdal, L., Bauwens, B., Baritz, R., Hagedorn, F., et al. (2007). How strongly can forest management influence soil carbon sequestration? Geoderma 137, 253–268. doi: 10.1016/j.geoderma.2006.09.003
Jost, L. (2007). Partitioning diversity into independent alpha and beta components. Ecology 88, 2427–2439. doi: 10.1890/06-1736.1
Kangas, A., Kurttila, M., Hujala, T., Eyvindson, K., and Kangas, J. (2015). Decision Support for Forest Management, 2nd Edn. Berlin: Springer Science + Business Media B.V.
Kangas, J., and Niemelainen, P. (1996). Opinion of forest owners and the public on forests and their use in Finland. Scand. J. For. Res. 11, 269–280. doi: 10.1080/02827589609382936
Knoke, T., Paul, C., Hildebrandt, P., Calvas, B., Castro, L. M., Hartl, F., et al. (2016). Compositional diversity of rehabilitated tropical lands supports multiple ecosystem services and buffers uncertainties. Nat. Commun. 7, 1–12.
Langner, A., Irauschek, F., Perez, S., Pardos, M., Zlatanov, T., Ohman, K., et al. (2017). Value-based ecosystem service trade-offs in multi-objective management in European mountain forests. Ecosyst. Serv. 26, 245–257. doi: 10.1016/j.ecoser.2017.03.001
Lundholm, A., Black, K., Corrigan, E., and Nieuwenhuis, M. (2020). Evaluating the impact of future global climate change and bioeconomy scenarios on ecosystem services using a strategic forest management decision support system. Front. Ecol. Evol. 8:200.
Luyssaert, S., Ciais, P., Piao, S. L., Schulze, E. D., Jung, M., Zaehle, S., et al. (2010). The European carbon balance. Part 3: forests. Glob. Change Biol. 16, 1429– 1450.
Messier, C., Puettmann, K. J., and Coates, D. K. (2013). Managing Forests as Complex Adaptive Systems: Building Resilience to the Challenge of Global Change. London: Routledge.
Mey, R., Stadelmann, G., Thürig, E., Bugmann, H., and Zell, J. (2021). From small forest samples to generalised uni-and bimodal stand descriptions. Methods Ecol. Evol. 12, 634–645. doi: 10.1111/2041-210x.13566
Mina, M., Bugmann, H., Cordonnier, T., Irauschek, F., Klopcic, M., Pardos, M., et al. (2017). Future ecosystem services from European mountain forests under climate change. J. Appl. Ecol. 54, 389–401. doi: 10.1111/1365-2664.12772
Müller, J., and Bütler, R. (2010). A review of habitat thresholds for dead wood: a baseline for management recommendations in European forests. Eur. J. For. Res. 129, 981–992. doi: 10.1007/s10342-010-0400-5
Nabuurs, G. J., Delacote, P., Ellison, D., Hanewinkel, M., Hetemaki, L., and Lindner, M. (2017). By 2050 the mitigation effects of EU forests could nearly double through climate smart forestry. Forests 8, 1–14. doi: 10.1007/978-3-642-13253-7_1
Nordström, E. M., Nieuwenhuis, M., Başkent, E. Z., Biber, P., Black, K., Borges, J. G., et al. (2019). Forest decision support systems for the analysis of ecosystem services provisioning at the landscape scale under global climate and market change scenarios. Eur. J. For. Res. 138, 561–581. doi: 10.1007/s10342-019-01189-z
Ott, E., Frehner, M., Frey, H. U., and Lüscher, P. (1997). Gebirgsnadelwälder: Ein Praxisorientierter Leitfaden Für Eine Standortgerechte Waldbehandlung. Bern: P. Haupt Verlag.
Pohjanmies, T., Eyvindson, K., and Mönkkönen, M. (2019). Forest management optimization across spatial scales to reconcile economic and conservation objectives. PLoS One 14:e0218213. doi: 10.1371/journal.pone.0218213
Pretzsch, H., Grote, R., Reineking, B., Roetzer, T., and Seifert, S. (2008). Models for forest ecosystem management: a European perspective. Ann. Bot. 101, 1065–1087. doi: 10.1093/aob/mcm246
Pukkala, T. (2014). Does biofuel harvesting and continuous cover management increase carbon sequestration? For. Policy Econ. 43, 41–50. doi: 10.1016/j.forpol.2014.03.004
R Core Team (2020). R: A Language And Environment For Statistical Computing. Vienna: R Foundation for Statistical Computing.
Rigling, A., Bigler, C., Eilmann, B., Feldmeyer-Christe, E., Gimmi, U., Ginzler, C., et al. (2013). Driving factors of a vegetation shift from Scots pine to pubescent oak in dry Alpine forests. Glob. Change Biol. 19, 229–240. doi: 10.1111/gcb.12038
Rigling, A., and Schaffer, H. P. (2015). Forest Report 2015. Condition and Use of Swiss Forests. Bern: Federal Office for the Environment, Swiss Federal Institute WSL.
Rogiers, N., Hagedorn, F., and Thürig, E. (2015). Carbon stock. Forest Report 2015 – Conditions and Use of Swiss Forest, eds A. Rigling and H. P. Schaffer (Bern: Federal Office of the Environment FOEN, Swiss Federal Institute for Forest, Snow and Landscape Research WSL), 38–41.
Rosset, C., Schütz, J.-P., Güntner, M., and Gollut, C. (2014). WIS.2 – a sustainable forest management decision support system. Math. Comput. For. Nat. Resour. Sci. 6, 89–100.
Schmid-Haas, P., Baumann, E., and Werner, J. (1993). Kontrollstichproben: Aufnahmeinstruktion. Birmensdorf: Swiss Federal Institute of Forest, Snow and Landscape Research WSL.
Schwaiger, F., Poschenrieder, W., Rötzer, T., Biber, P., and Pretzsch, H. (2018). Groundwater recharge algorithm for forest management models. Ecol. Modelling 385, 154–164. doi: 10.1016/j.ecolmodel.2018.07.006
Schweier, J., Magagnotti, N., Labelle, E. R., and Athanassiadis, D. (2019). Sustainability impact assessment of forest operations: a review. Curr. For. Rep. 5, 101–113. doi: 10.1007/s40725-019-00091-6
Sebald, J., Thrippleton, T., Rammer, W., Bugmann, H., and Seidl, R. (2021). Mixing tree species at different spatial scales: The effect of alpha, beta and gamma diversity on disturbance impacts under climate change. J. Appl. Ecol. 1–15. doi: 10.1111/1365-2664.13912
Segura, M., Ray, D., and Maroto, C. (2014). Decision support systems for forest management: a comparative analysis and assessment. Comput. Electron. Agric. 101, 55–67. doi: 10.1016/j.compag.2013.12.005
Seidl, R., Albrich, K., Erb, K., Formayer, H., Leidinger, D., Leitinger, G., et al. (2019). What drives the future supply of regulating ecosystem services in a mountain forest landscape? For. Ecol. Manag. 445, 37–47. doi: 10.1016/j.foreco.2019.03.047
Seidl, R., Rammer, W., Jäger, D., Currie, W. S., and Lexer, M. J. (2007). Assessing trade-offs between carbon sequestration and timber production within a framework of multi-purpose forestry in Austria. For. Ecol. Manag. 248, 64–79. doi: 10.1016/j.foreco.2007.02.035
Seidl, R., Thom, D., Kautz, M., Martin-Benito, D., Peltoniemi, M., Vacchiano, G., et al. (2017). Forest disturbances under climate change. Nat. Clim. Change 7, 395–402. doi: 10.1038/nclimate3303
Shannon, C. E., and Weaver, W. (1949). The Mathematical Theory of Communication. Urbana, IL: University of Illinois Press.
Staudhammer, C. L., and LeMay, V. M. (2001). Introduction and evaluation of possible indices of stand structural diversity. Can. J. For. Res. 31, 1105–1115. doi: 10.1139/x01-033
Streit, K., Leuch, B. A., and Brang, P. (2017). Der richtige Eingriff zur richtigen Zeit. Wald Holz 98, 32–35.
Swisstopo (2010). The Digital Height Model of Switzerland with a 200 m Grid, DHM25/200. Wabern: Federal Office of Topography.
Taverna, R., Hofer, P., Werner, F., Kaufmann, E., and Thürig, E. (2007). CO2-Effekte der Schweizer Wald- und Holzwirtschaft. Szenarien zukünftiger Beiträge zum Klimaschutz. Umwelt-Wissen Nr. 0739. Bern: Bundesamt für Umwelt, 102.
Temperli, C., Blattert, C., Stadelmann, G., Brandli, U. B., and Thurig, E. (2020). Trade-offs between ecosystem service provision and the predisposition to disturbances: a NFI-based scenario analysis. For. Ecosyst. 7, 1–17.
Temperli, C., Bugmann, H., and Elkin, C. (2013). Cross-scale interactions among bark beetles, climate change, and wind disturbances: a landscape modeling approach. Ecol. Monogr. 83, 383–402. doi: 10.1890/12-1503.1
Thom, D., Rammer, W., and Seidl, R. (2017). The impact of future forest dynamics on climate: interactive effects of changing vegetation and disturbance regimes. Ecol. Monogr. 87, 665–684. doi: 10.1002/ecm.1272
Thrippleton, T., Lüscher, F., and Bugmann, H. (2020). Climate change impacts across a large forest enterprise in the Northern Pre-Alps: dynamic forest modelling as a tool for decision support. Eur. J. For. Res. 139, 483–498. doi: 10.1007/s10342-020-01263-x
Thürig, E., and Kaufmann, E. (2010). Increasing carbon sinks through forest management: a model-based comparison for Switzerland with its Eastern Plateau and Eastern Alps. Eur. J. For. Res. 129, 563–572. doi: 10.1007/s10342-010-0354-7
Uhde, B., Hahn, W. A., Griess, V. C., and Knoke, T. (2015). Hybrid MCDA methods to integrate multiple ecosystem services in forest management planning: a critical review. Environ. Manag. 56, 373–388. doi: 10.1007/s00267-015-0503-3
Vacik, H., and Lexer, M. J. (2014). Past, current and future drivers for the development of decision support systems in forest management. Scand. J. For. Res. 29, 2–19. doi: 10.1080/02827581.2013.830768
Weiss, P., Braun, M., Fritz, D., Gschwantner, T., Hesser, F., Jandl, R., et al. (2020). Zusammenschau der treibhausgasergebnisse das waldbasierten sektors für verschiedene careforparis-senarien. BFW Praxisinformation 51, 20–24.
Werner, F., Taverna, R., Hofer, P., Thürig, E., and Kaufmann, E. (2010). National and global greenhouse gas dynamics of different forest management and wood use scenarios: a model-based assessment. Environ. Sci. Policy 13, 72–85. doi: 10.1016/j.envsci.2009.10.004
Whiteman, D. C. (2000). Mountain Meteorology : Fundamentals And Applications. New York, NY: Oxford University Press.
Wilpert, K. V., Ahrends, B., Weis, W., Vonderach, C., Puhlmann, H., Köhler, D., et al. (2018). Standortangepasste Nutzungsintensitäten und forstliche Handlungsoptionen. Holznutzung und Nährstoffnachhaltigkeit. Abschlussbericht zum Projekt Energieholzernte und stoffliche Nachhaltigkeit in Deutschland (EnNa). Freiburg im Breisgau: Fakultät für Umwelt und Natürliche Ressourcen der Albert-Ludwigs-Universität Freiburg;, 325–373.
Wolfslehner, B., and Seidl, R. (2010). Harnessing ecosystem models and multi-criteria decision analysis for the support of forest management. Environ. Manag. 46, 850–861. doi: 10.1007/s00267-009-9414-5
Zell, J. (2016). SwissStandSim: A Climate Sensitive Single Tree Stand Simulator For Switzerland. Birmensdorf: Swiss Federal Institute of Forest, Snow and Landscape Research WSL, 107.
Zell, J. (2018). Climate sensitive tree growth functions and the role of transformations. Forests 9, 1–20.
Zell, J., Nitzsche, J., Stadelmann, G., and Thürig, E. (2020). SwissStandSim: ein klimasensitives, einzelbaumbasiertes waldwachstumsmodell. Schweiz. Z. For. 171, 116–123.
Keywords: decision support system, forest biodiversity, ecosystem services, forest modeling, sustainable forest management, climate change, carbon sequestration, synergies and trade-offs
Citation: Thrippleton T, Blattert C, Bont LG, Mey R, Zell J, Thürig E and Schweier J (2021) A Multi-Criteria Decision Support System for Strategic Planning at the Swiss Forest Enterprise Level: Coping With Climate Change and Shifting Demands in Ecosystem Service Provisioning. Front. For. Glob. Change 4:693020. doi: 10.3389/ffgc.2021.693020
Received: 09 April 2021; Accepted: 15 July 2021;
Published: 04 August 2021.
Edited by:
Marco Mina, Eurac Research, ItalyReviewed by:
Matija Klopčič, University of Ljubljana, SloveniaAlejandra Morán-Ordóñez, Ecological and Forestry Applications Research Centre (CREAF), Spain
Copyright © 2021 Thrippleton, Blattert, Bont, Mey, Zell, Thürig and Schweier. This is an open-access article distributed under the terms of the Creative Commons Attribution License (CC BY). The use, distribution or reproduction in other forums is permitted, provided the original author(s) and the copyright owner(s) are credited and that the original publication in this journal is cited, in accordance with accepted academic practice. No use, distribution or reproduction is permitted which does not comply with these terms.
*Correspondence: Timothy Thrippleton, timothy.thrippleton@wsl.ch; orcid.org/0000-0002-1017-7083