- 1Austrian Forest Research Center BFW, Institute of Forest Ecology, Vienna, Austria
- 2Austrian Forest Research Center BFW, Institute of Silviculture, Vienna, Austria
- 3Environmental Agency Austria, Vienna, Austria
Question: We compared the soil organic carbon stock of the forests of an entire country. The objective of our research was establishing the differences between coniferous or deciduous forests with respect to soil carbon stocks. The question is relevant because coniferous forests are increasingly damaged by abiotic and biotic disturbances that are related to climate change. Deciduous forests are considered to be less vulnerable. Their soils are expected to be more persistent and reliable sinks for carbon dioxide.
Methods: Soil data are available from the Austrian Forest Soil Survey. Soils have been sampled on sites of the Austrian Forest Inventory. The data were stratified according to geology (calcareous vs. silicatic bedrock), orientation of the slopes, and forest type (coniferous vs. mixed-deciduous forest). These data were used to establish ground truth of soil organic carbon stocks. Further, we had simulation results of a coupled forest growth/soil carbon model. The scenarios built on the results of the Forest Inventory 2007/09 and reflect a business-as-usual forest management vs. a climate-change adaptation scenario where forest managers replace coniferous with deciduous forests if site conditions permit it. The simulations were performed with the forest growth simulator CÂLDIS and the soil carbon model Yasso07.
Results: Based on the Austrian Forest Soil Survey carbon stocks of coniferous forests were consistently higher than in mixed-deciduous forests. This result applies both for the organic litter layer and the mineral soil to a depth of 50 cm. The depth gradients of carbon were similar in both forest types. The simulation under a strong warming scenario showed an increase in the carbon stocks of soils when conifers are replaced by deciduous tree species. In the 150-year simulation the majority of forest sites will become suitable for deciduous forests. The build-up of a large soil organic carbon stock is driven by the stronger harvesting pressure on the remaining coniferous forests. Deciduous forests were in lesser demand and developed under a light forest intervention regime. However, toward the end of the century, when the temperature level is far above present levels, the soil organic carbon stocks declined.
1. Introduction
Organic carbon storage in forest soils is a potential contributor to climate change mitigation. Temperate cool forest soils store on average 120 t C/ha and are an important carbon sink (Post et al., 1982; Pan et al., 2011). Changing preferences for tree species as part of the adaptation of forest management to climate change can affect the size of the soil organic carbon stocks. Historically, many deciduous forests in Central Europe have been replaced with Norway spruce (Picea abies) stands in order to optimize productivity. The so-called “secondary Norway spruce stands” presently proof to be particularly vulnerable to biotic and abiotic disturbances and options of eventually replacing them with less vulnerable deciduous forests are investigated (Seidl et al., 2017; Hlásny et al., 2019; Jandl, 2020; Lindner et al., 2020; Mayer et al., 2020). Moreover, climate change is leading to shifts in the habitat of forest types and deciduous tree species are expected to move into higher elevation where forests are presently dominated by Norway spruce (Hanewinkel et al., 2012; Bircher et al., 2015; Lexer et al., 2015).
Soil organic carbon stocks are determined by several site factors and also by the amount of above- and belowground litterfall (forest productivity), rooting depth (allocation of organic matter), and decomposition rate of organic material (chemical quality). The overall effect of these three factors is complex (Liski et al., 2005; Andivia et al., 2016). Field observations give evidence for different soil organic carbon stocks of coniferous vs. deciduous forests. Under pine (Pinus sp.) and Norway spruce forests an organic layer of litter material builds up because needles are more recalcitrant toward decomposition than leaves. Under deciduous forests a shallower organic layer with a higher turnover rate forms (Achilles et al., 2020). Yet, deciduous trees tend to develop deeper rooting systems, thereby supplying the subsoil with organic matter (deB Richter jr. and Markewitz, 2001; Berger et al., 2002, 2006; Schmid and Kazda, 2002; Rehschuh et al., 2021). The effect of storing organic carbon in the litter layer of coniferous forests could be partially compensated by the deeper distribution of organic carbon in soils of deciduous forests. Evidence for the dominant effect of root-derived carbon for the formation of stable soil C has been provided (Rasse et al., 2005). The relevance of subsoil organic carbon has been often stressed and it has been pointed out that considerable quantities of organic carbon are encountered at depths that are often not captured by soil surveys that are confined to the organic litter layer and the uppermost part of the mineral soil (Harrison et al., 2011; deB. Richter and Billings, 2015).
Much research has been conducted on the organic carbon stocks in European forest soils. Differences between soil types have been identified and the relative importance of tree species was high in the litter layer, and of lesser relevance for the organic carbon stock in the mineral soil (De Vos et al., 2015). A detailed analysis of German forest soils showed significantly higher carbon stocks in the litter layer of spruce forests, as compared to beech (Fagus sylvatica). In the mineral soil the difference was statistically not significant (Grüneberg et al., 2014, 2019). The findings are supported by the comprehensive analysis of Swiss soil data that have been presented for the litter layer and the mineral soil to a depth of 1.2 m (Gosheva et al., 2017). Large-scale studies of tree species effects on soil carbon stocks in Central Europe are scarce. Comparisons on a national scale are hampered by the fact that forest management strategies are overriding the effect of natural site factors. Whether a site is presently stocked with coniferous or deciduous forests only partially reflects site conditions and eventually limiting ecological factors, but rather reflects the choice of forest managers favoring a particular forest type.
Some information on the impact of tree species on soil carbon stocks is available from common garden experiments. A comparison conducted in Southern Sweden showed significantly more carbon under Norway spruce than under beech in the organic layer and the upper 20 cm of the mineral soil (Oostra et al., 2006). Six common gardens in Denmark supported the findings for the litter layer. In the upper 30 cm of the mineral soil no differences were found (Vesterdal et al., 2008, 2013).
Stand conversion experiments provide further evidence for smaller organic carbon stocks in the organic layer under deciduous forests as compared to coniferous forests. Converting pine forests to beech reduces the thickness of the organic layer and the signature of Norway spruce on the chemical quality of the organic layer is evident (Fischer et al., 2002; Prietzel and Bachmann, 2012). Establishing coniferous forests on abandoned agricultural land leads to a buildup of the organic layer (Hager, 1988; Strohschneider, 1991; Markewitz et al., 2002; Smal et al., 2019).
There is a clear indication that soils of deciduous forests have consistently lower soil organic carbon stocks than soils of coniferous forests (Osei et al., 2021). Deciduous forests are favored over coniferous forests mostly for the sake of stand stability and resilience toward expected climate change effects. Changing the tree species composition has ecological and economic consequences for the forestry sector. The price to be paid for presumingly more stable forests is high. The technology of the forest product chain needs to be adapted and the timber production of deciduous forests and mixed-species forests is substantially lower than the productivity of Norway spruce forests. Based on existing knowledge it is not clear yet, whether forest managers are better off when accepting a higher risk of climate-change related damages of coniferous forests or when they are opting for less productive yet more stable deciduous forests (Braun et al., 2016; Paul et al., 2019; Knoke et al., 2020).
In our analysis we investigate the evidence for differences in soil organic carbon stocks under coniferous vs. mixed-deciduous forests of Austria. We investigate whether the differences that are reported from case studies are evident in our large-scale dataset from a national forest soil survey that presumingly covers a wider range of site conditions than silvicultural experiments. Secondly, we evaluate three scenarios of a forest simulation experiment. Other than a case study or a common garden experiment, the simulation reflects the response of soil organic carbon to a combined effect of climate change, forest management, and the response of the market to an altered supply of timber. In our simulation we changed the tree species composition according to arising opportunities after final harvests. In order to easier detect the consequences of changes in tree species a simulation period of 150 years was chosen.
2. Methods
2.1. Characteristics of Austrian Forests
According to the Austrian Forest Inventory 2016/18 the country has a forest cover of 4.02 Mio ha or almost 50% of the surface, an average standing stock of stemwood of 351 m3, and an average productivity of 8.9 m3 ha−1. Presently, coniferous tree species comprise 61.4% of the forest area [Norway spruce 49.2%, pine 5%, European larch (Larix decidua) 4.4%, Silver fir (Abies alba) 2.5%, and others], and deciduous trees 24.5% [European beech 10.2%, oak (Quercus sp.) 2.1%, and others]. The remaining 14.4% of the surface are legally forest land that is currently not forested. Typically, these are open meadows, forest roads, or timber manipulation areas (BFW, 2021). The economically most relevant trees species is Norway spruce. It is managed with high efficiency and the forest value chain is adapted to a continuous supply of spruce timber. The forest-based sector of the economy contributes 1.9% to the gross domestic product. This value is twice the European average (Schwarzbauer, 2018). Yet, the figure is declining due to a continuous shift toward the tertiary sector of the economy (Braun et al., 2020).
2.2. Austrian Forest Soil Survey
The Austrian Forest Soil Survey is placed on a regular grid with 8 km side length and comprises 526 plots. The soil sampling plots are a subset of the assessment grid of the Austrian Forest Inventory. Therefore, the available soil information is supported with detailed information on forest stands such as tree species composition, stand age, and standing stock. We also used the available site information on geological bedrock, altitude, and growth district. The advantage of the sampling design is the representativity for the entire country, the disadvantage is that rare soil or forest types are not necessarily well-captured.
Soils have been sampled and chemically analyzed according to ICP Forest standards (ICP, 2020). The soil organic carbon stock in the organic surface layer and the mineral soil to a depth of 50 cm was calculated based on the measured carbon concentration, the estimated bulk density from a nationally derived function, and the fine earth fraction (Foldal et al., 2021). The rock content has been visually estimated in the field. Some plots were removed from the dataset due to incomplete records. The available sample size for our analysis was finally 511 plots. Ancillary variables to soil carbon concentration and soil organic carbon stocks were altitude, geology (calcareous and silicatic bedrock, respectively), slope exposition, and forest type. Slope exposition was stratified into “East/West” (161 sites) for slopes facing to E, W, SE, SW, “South/even” (163 sites) for south-facing slopes and sites on even terrain, and “North” (190 sites) for N-, NW-, NE-facing slopes. With hindsight to the dominance of Norway spruce forests we defined the forest type as “coniferous” when conifers contributed more than 60% to the standing stock of stemwood, and otherwise “mixed-deciduous” (Figure 1). This split gave 136 mixed-deciduous forests and 375 coniferous forests. A further stratification was considered impractical due to small populations in some strata.
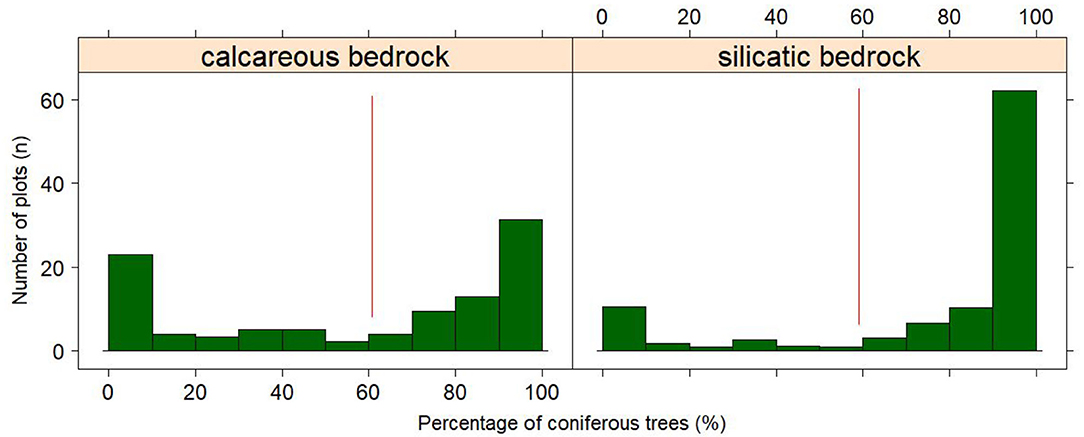
Figure 1. Distribution of tree species combinations in Austria on calcareous vs. silicatic bedrock. The vertical red reference lines indicate the pragmatically defined threshold between mixed-deciduous and coniferous forests.
2.3. Simulation Experiment
In a simulation experiment we investigated the development of Austrian forests. We used two scenarios of climate change in order to capture a range of possible futures. In the period 1980–2010 the mean air temperature in Austria was 6.3 °C and the annual precipitation was 1,084 mm. The regionally downscaled climate change indicated a warming trend between 2 °C and 3.5 °C, depending whether a path of RCP 4.5 or RCP 8.5 will be followed (Jacob et al., 2014; Chimani et al., 2016). The RCP 4.5 scenario is an ambitious pathway that assumes the successful implementation of climate change mitigation measures, even though the Paris target of 1.5 °C is exceeded (Rogelj et al., 2018). RCP 8.5 is described as pessimistic scenario reflecting little success on curbing the annual emissions of greenhouse gases (Riahi et al., 2011). Both scenarios show only a slight change of the annual precipitation; a seasonal change in precipitation patterns is possible.
The climate data were used to run the forest growth model CÂLDIS. The model is based on the growth simulator PrognAUS that has been initially developed from data of the Austrian forest inventory period 1981/85 and was refined with new modules based on later inventory periods until 2007/09 (Monserud and Sterba, 1996). The single-tree model yields information on stem volume, stem diameter and height for every tree at annual time steps. In order to derive the input of organic matter to the soil we used the output of CÂLDIS. The simulation period started at year 2010 and was based on data of the Austrian Forest Inventory 2007/09 that represents the most recent complete dataset and reflects the current forest management (Ledermann et al., 2017). Forest practitioners have several options to cope with climate change. In the RCP 4.5 scenario we assumed that no adaptation is required. The RCP 8.5 scenario was used on one hand without changes of forest management and on the other hand with the gradual change of the tree species composition in order to replace increasingly vulnerable coniferous with deciduous forests. The scenarios are summarized below.
• RCP 4.5 Business-as-usual: The forests are experiencing a moderate warming. The 2 °C target of the Paris Agreement is not fully reached. Forest management follows the presently encountered path.
• RCP 8.5 Business-as-usual: The forests are experiencing an unprecedented warming trend. The mean annual temperatures will rise until the end of the century by ~3.5 °C as compared to 2010 and will continue rising thereafter. No modification of the forest management plan is envisioned.
• RCP 8.5 Change of tree species from conifer-dominated to deciduous-dominated forests: The forests are experiencing an unprecedented warming trend. The forestry sector responds by changing the tree species composition.
The forest management scenario was based on the demand of the timber market that is reflected in the data of the Austrian Forest Inventory 2007/09. The future demand for timber was defined by an assumed economic development until the year 2150 that has been described previously (Braun et al., 2016). The required timber resources were supplied according to the demand of a business-as-usual scenario for the entire simulation period. We avoided assumptions on technological developments in timber processing in order to keep the simulation outputs overall sufficiently constrained.
In the model the option of changing of tree species was explored whenever a forest stand was harvested. The replacement of conifers with deciduous tree species followed a defined rule based on the expected mean annual temperature 50 years after the reforestation as provided by the respective climate scenario (Table 1). We opted for a simulation period beyond 2100 in order to ensure that most managed forests were harvested at least once.
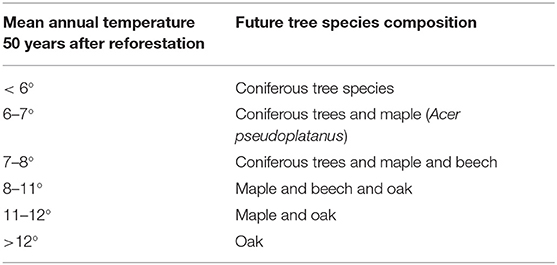
Table 1. Replacement of coniferous tree species based on the expected mean annual temperature in 50 years after reforestation.
In order to keep the number of influencing factors low, a constant forest area was assumed. This condition does not fully reflect reality, because the forest area is annually increasing by 3,000 ha, mostly due to abandonment of high elevation pastures and marginal agricultural land (Gschwantner, 2019).
The soil organic carbon stock was simulated with Yasso07 (Liski et al., 2009). The crucial parameter for Yasso07 is the influx of organic carbon from trees into the soil. No measured flux data were available. Therefore, the influx was estimated in a two-step procedure. Firstly, the standing stock of the above- and belowground biomass, i.e., stem mass, branch mass, mass of needles or leaves, and the mass of fine (∅ <2 mm) and coarse roots (∅ > 2 mm) was calculated with a set of biomass equations. Stem volume was converted to stem mass with tree-species specific wood densities. The tree-species-specific equations for the mass of branches, needles and leaves, and total roots have been derived from Austrian experiments and are completely referenced in a previous paper (Jandl et al., 2018). The biomass equations use stem diameter, stem height, and canopy height of individual trees as input parameters. The mass of fine roots was pragmatically chosen to be 2% of the total root biomass. Secondly, the annual flux of organic carbon from the respective biomass compartments to the soil was estimated by assigning each biomass compartment a certain turnover rate. The assumptions on the longevity of different tree compartments were based on observations from intensive monitoring plots and from literature information, as also previously described (Jandl et al., 2018). The forest inventory data do not provide information on the biomass of herbaceous plants and bushes. Their eventual contribution to the flux of organic carbon from plants to the soil is therefore not reflected in our simulations. Later, the individual biomass compartments are assigned chemical properties and sizes that are affecting the decomposition rate of soil organic matter (Didion et al., 2016; Hernández et al., 2017).
The next step was the stratification of trees into the classes “Standing stock,” “Mortality,” and “Harvest.” Each stratum was treated differently with respect to the release of organic material from the biomass into the soil. The class “Standing Stock” releases organic matter in the form of aboveground and belowground litterfall. Deciduous trees and larch are shedding all photosynthetic active tissue each year and have therefore a turnover of 1 year. For pine and Norway spruce we had observations on the longevity of needles, ranging from 2 years of pine (Pinus silvestris) to 5 years for Norway spruce. Therefore, the annual input from needles and leaves to the soil was quantified. The annual flux of branch biomass to the soil is difficult to estimate. It widely depends on local conditions (exposure to storms) and the number and severity of storm events. Missing site-specific information we based our estimate on a pragmatically chosen fixed value (Jandl et al., 2018). The turnover of coarse roots was the same as the turnover of branch biomass. The turnover of fine roots is a relevant, yet most elusive flux of carbon (Brunner et al., 2013). Fine roots are short-lived, highly decomposable and supply a significant amount of organic matter to the soil (Trumbore and Gaudinski, 2003). Based on many controversial discussions with root experts we defined the turnover time of fine roots to be 1 year. The class “Harvest” assumed the common strategy in Austria: Trees are cut and de-limbed. Branches, needles and leaves, and roots remain on site. A small portion of the stem, the canopy, is also remaining on site. The merchantable stem is exported. All remaining compartments enter the soil and are decomposing according to the quality- and size-depending rules of Yasso07. The class “Mortality” followed a similar logic as “Harvest.” The difference is that stems (logs) remained on site. Salvaging of previously dying trees was reported in the data of the National Forest Inventory. Therefore, it could be defined at which point in time the dead trees were either removed from the site or entered the soil as slowly decaying logs.
Yasso07 does not distinguish between carbon in the litter layer and carbon in the mineral soil, neither does it distinguish between soil horizons. The output of Yasso07 gives some resemblance to the terminology of soil science. Yet, the match between strata in Yasso07 and soils is not fully defined and is left to the imagination of the model user. The unconstrained soil depth of the Yasso07 model informs, how much carbon is expected to reside in the entire soil in order to meet steady-state conditions. The model does not define at which soil position exactly the carbon is sitting.
2.4. Data Analysis
The data of the Austrian Soil Survey were statistically analyzed. An Analysis of Covariance was used to identify differences in soil carbon stocks between coniferous and mixed-deciduous forests at different geological substrates (two categories) and elevation (continuous variable), and exposition of slopes (four categories). Further, the difference in different strata was analyzed with a t-test. Soil data were analyzed with the AQP package (Beaudette et al., 2013). All data analyses were done with R version 4.0.3 (codename: “Bunny-Wunnies Freak Out”; R Core Team, 2017).
3. Results
3.1. Austrian Forest Soil Survey
Owed to the geological structure of Austria two thirds of the forests are on silicatic bedrock. Overall, coniferous forests are dominating (73% of the investigated sites) over mixed-deciduous forests (27%). On silicatic bedrock, 82% of the forests are coniferous, and only 18% are mixed-deciduous. On calcareous bedrock the ratio is rather even with 42% deciduous and 58% coniferous forests. The soil organic carbon stocks of the Austrian Forest Soil Survey are shown in Table 2. The mean total organic carbon stock is 104 t C/ha with 17% residing in the organic surface layer and 83% in the upper 50 cm of the mineral soil. Mineral soils derived from calcareous rocks have significantly higher organic carbon stocks than silicatic soils. The difference in organic carbon stocks in the litter layer is statistically not significant due to the high variability within the dataset. The stratification by bedrock material shows consistently higher soil organic carbon stocks in the litter layer of coniferous forests whereas the stocks in the mineral soils of both forest types are more even. The highest soil organic carbon stocks are found in coniferous forests on calcareous bedrock.
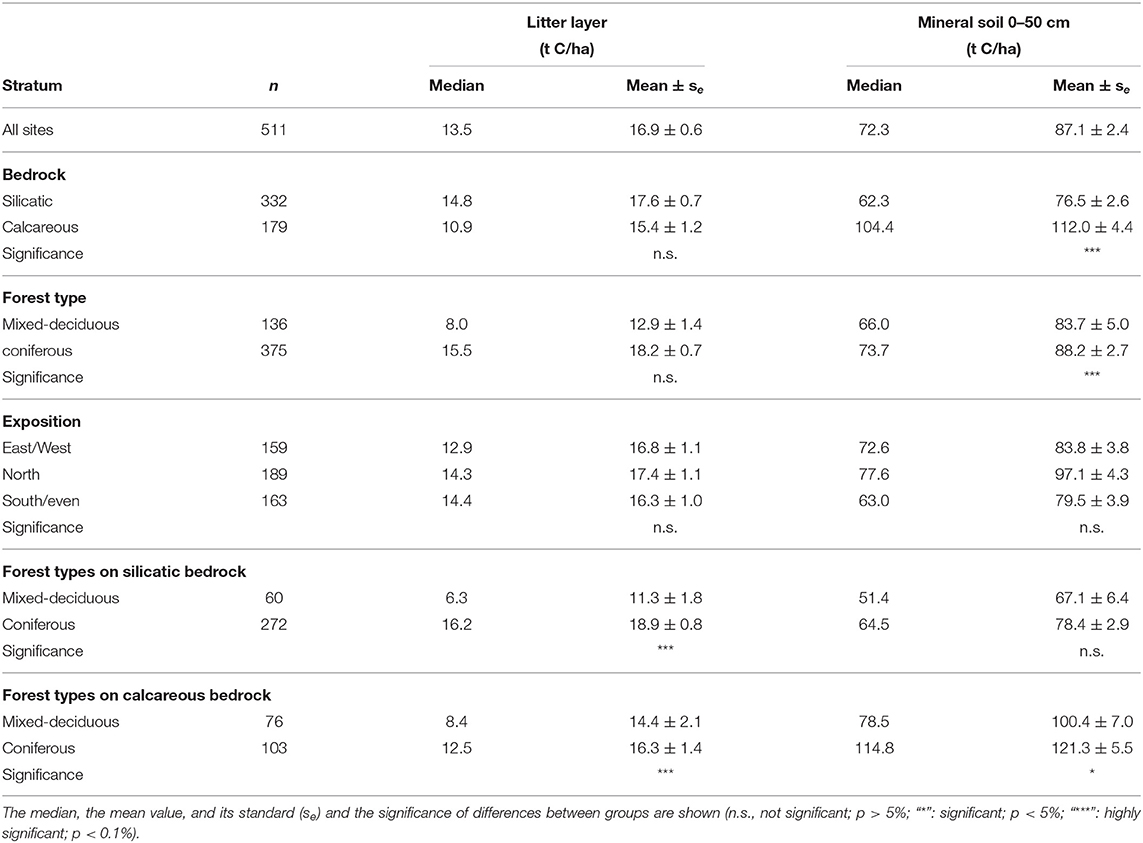
Table 2. Soil organic carbon stocks (t C/ha) in Austrian forest soils stratified according to geological bedrock (silicatic vs. calcareous material), forest type (coniferous vs. mixed-deciduous), and slope exposition.
Figure 2 and the difference between the median and the mean value in Table 2 show left-skewed distributions of the soil organic carbon stocks for both forest types. The highest frequency of soil organic carbon stocks in mixed-deciduous forests is around 75 t C/ha and somewhat lower in coniferous forests. Low soil organic carbon stocks are mainly found on shallow soils, which are commonly found independently of the bedrock material. Very high soil organic carbon stocks are reported for peatlands, which are rare in Austria, yet more commonly encountered on silicatic bedrock because the population of silicate sites is larger and therefore has a higher chance to include rare soil types.
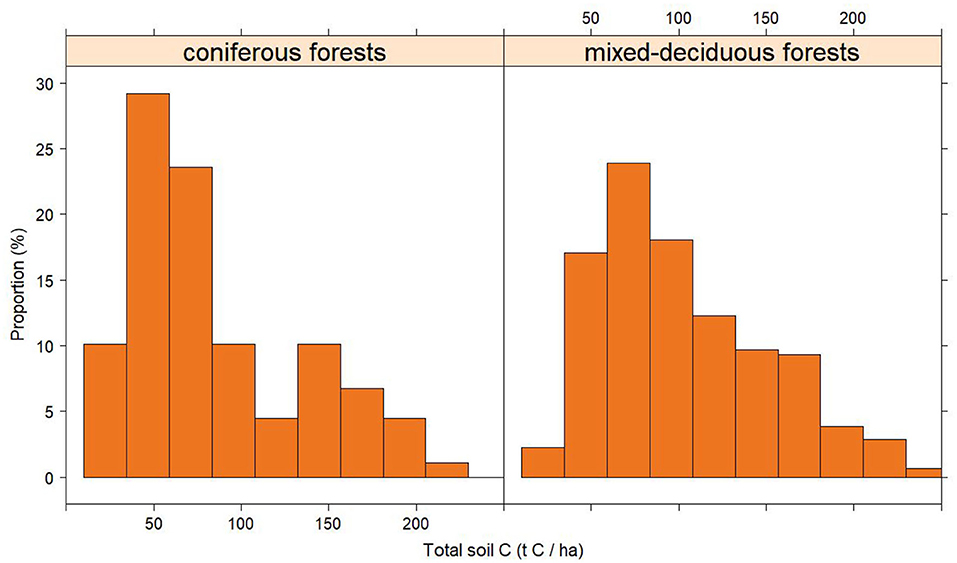
Figure 2. The total soil organic carbon stock (t C/ha) of coniferous and mixed-deciduous forests in Austria based on data of the Austrian Forest Soil Survey.
The elevational gradient of the total soil organic carbon stock is shown in Figure 3. On calcareous bedrock there is a gradual elevational increase of soil organic carbon in mixed-deciduous forests, and no increasing gradient in coniferous forests. On silicatic bedrock a strong elevational trend is found for both forest types. Yet, there is a wide scatter around the regression lines. The results of the analysis of covariance are presented in Table 3. The organic carbon pool in the forest floor material is always higher in coniferous as compared to mixed-deciduous forests, as shown by the numerical difference in the “intercept.” There are statistically significant slopes (“Coefficient for Altitude”) for all strata.
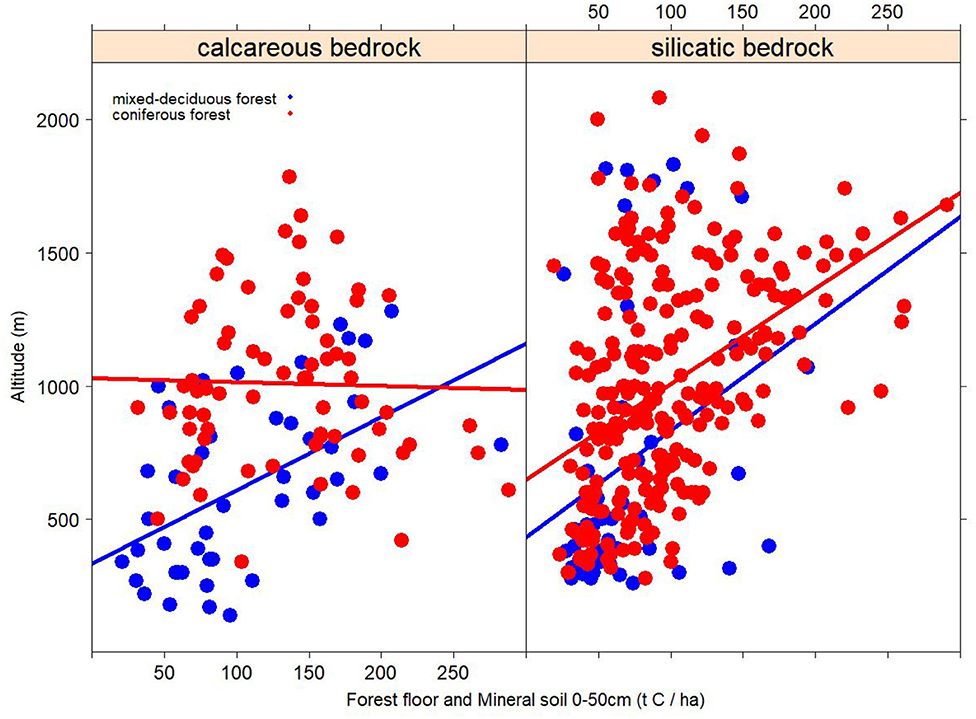
Figure 3. The total soil organic carbon stock differentiated between sites on calcareous bedrock and silicatic bedrock. The elevational gradient is indicated by a linear trend line. Different forest types (coniferous vs. mixed-deciduous forests) are shown in different colors.
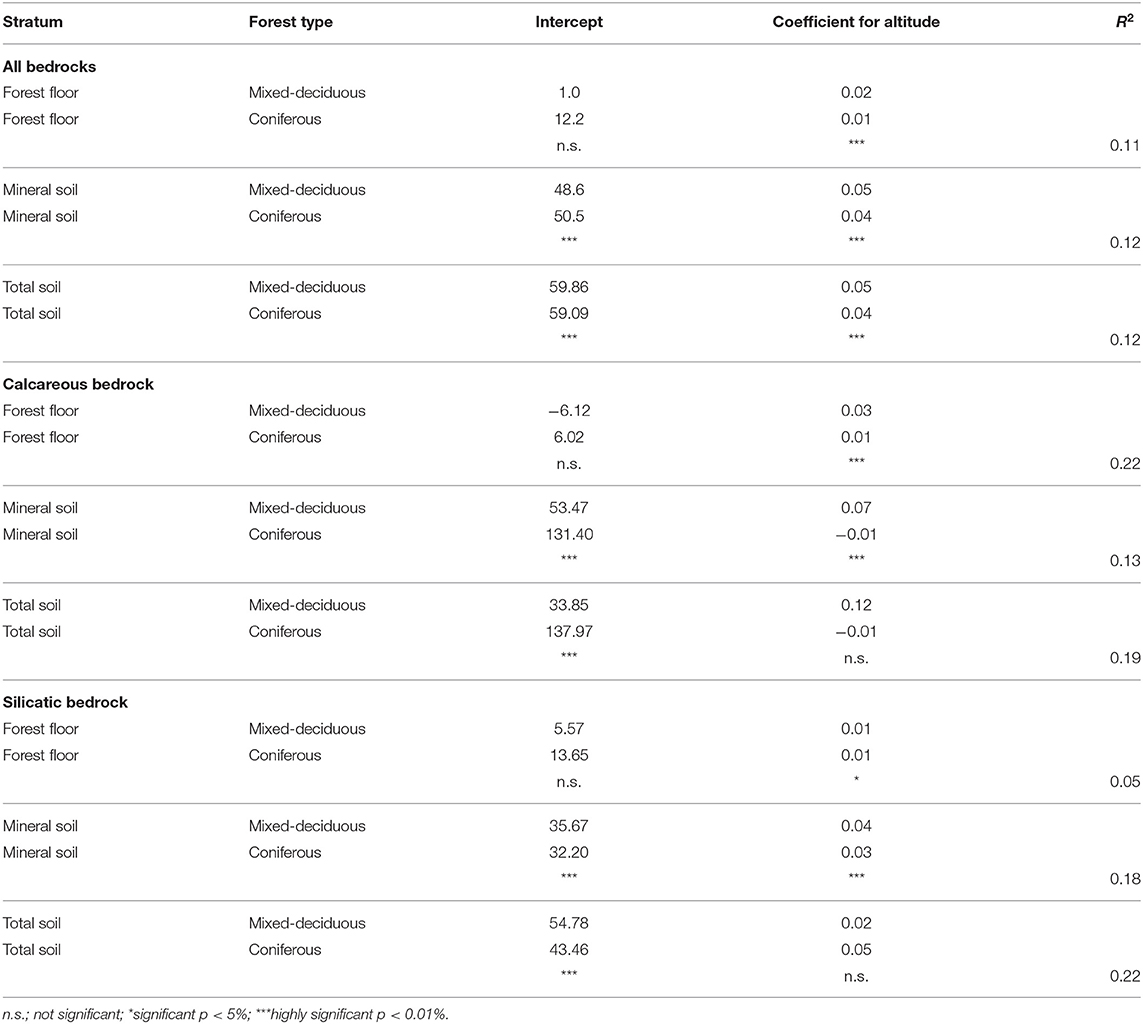
Table 3. Linear regression functions for the estimation of the soil carbon stock in different strata, as obtained by a covariance analysis with elevation as continuous and bedrock type as categorical variable.
The effect of slope exposition is shown in Figure 4. Obviously, slope exposition is among the key stratification parameters. Yet, in our dataset we found some evidence for the influence of exposition on the soil organic carbon stocks. Yet, other dominant factors such as bedrock, forest type, and elevational zone are over-shadowing its effect (Table 2).
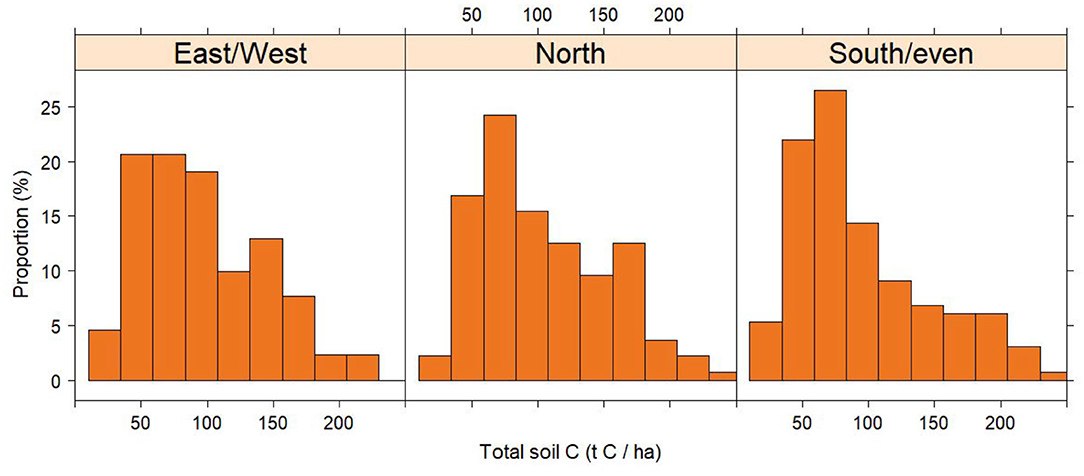
Figure 4. The total soil organic carbon stock (t C/ha) of coniferous and mixed-deciduous forests in Austria based on data of the Austrian Forest Soil Survey. The data are stratified according to slope orientation.
The depth gradients of organic carbon in the mineral soil is shown in Figure 5. Coniferous forests have higher carbon stocks in the upper part of the mineral soil. Deeper in the soil mixed-deciduous forests hold slightly more organic carbon. Yet, the difference is statistically not significant.
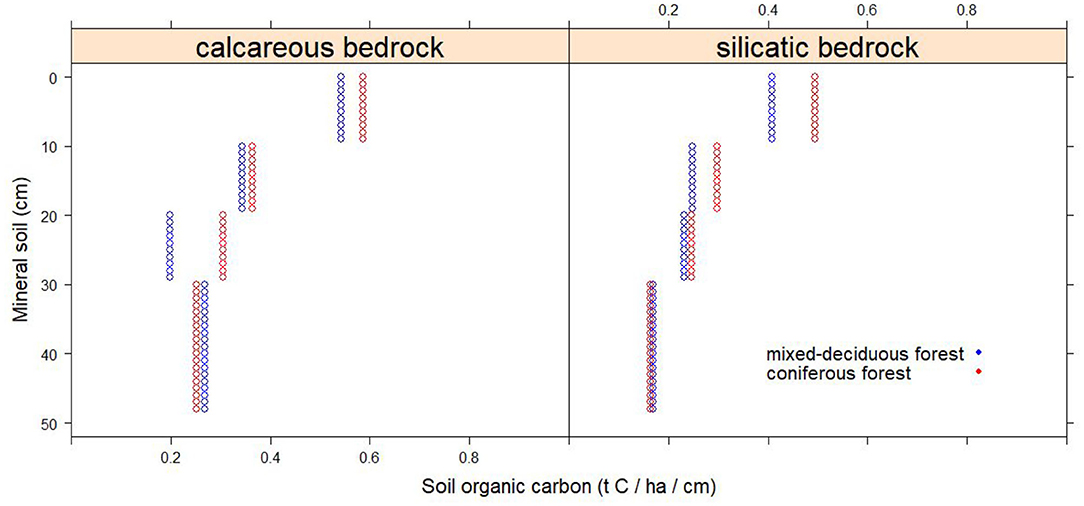
Figure 5. Depth gradients of soil organic carbon in the mineral soil of coniferous and mixed-deciduous forest stands differentiated between sites on calcareous vs. silicatic bedrock.
3.2. Modeling
The replacement of coniferous with deciduous tree species in order to adapt forests to a warmer world on a national scale is a long process. A replacement can be implemented either after the final harvest or after major disturbances of the stand structure. In Figure 6, we show the gradual increase in deciduous trees in time slices until the year 2150 when we apply the rules provided in Table 1. The present dominance of coniferous forests includes sites where conifers are dominating for natural reasons and where Norway spruce stands have been established for economic reasons. Until the year 2050 the sites in low elevation are already dominated by deciduous forests. By the end of the century coniferous forests merely prevail in the Inner Alps at high elevation sites. A further 50 years later the map is similar to the situation in 2100. This reflects the remaining sites in Inner Alpine areas and high elevation where site conditions are not suitable for deciduous forests in the foreseeable future.
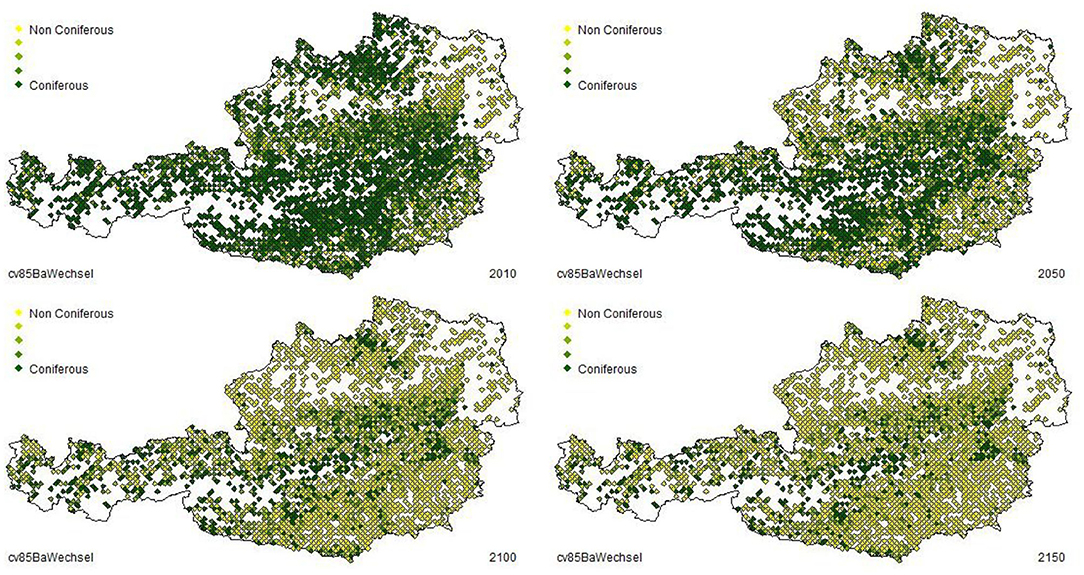
Figure 6. Forest adaptation to climate change via replacement of coniferous with deciduous tree species, depending on expected climatic conditions. The used climate scenario (RCP 8.5) reflects a strong warming trend. The initial conditions are presented for the year 2010 (upper panel, left), the distribution of tree species in 2050 (upper panel, right), 2100 (lower panel, left), and 2150 (lower panel, right) is shown. The color gradient describes the proportion of deciduous forests from 0 (yellow), 20, 40, 60, 80, 100% (increasing darkness of greens).
The simulated total soil organic carbon stock is constantly rising under the scenario of moderate warming (RCP 4.5, forest management following business-as-usual). In the next 70 years the soil organic carbon stock increases by almost 20%. Stronger warming (RCP 8.5) leads to increasing soil organic carbon stocks during several decades. However, after a peak toward the end of the century the soil organic carbon stocks decline and eventually drop below the presently encountered levels (Figure 7).
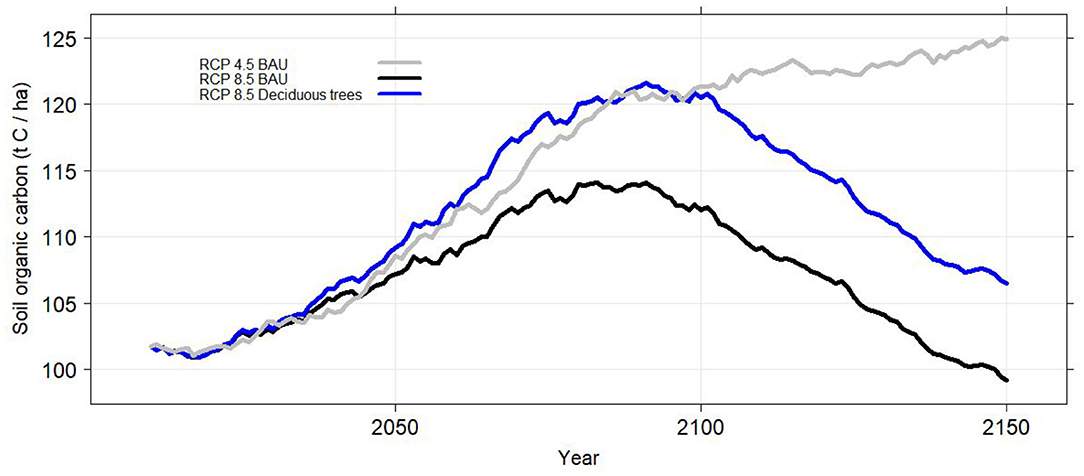
Figure 7. Temporal trend of the soil organic carbon stock of Austrian soils in a simulation experiment. The scenarios are a moderate temperature increase (RCP 4.5 Business-as-usual forest management), and a strong warming trend (RCP 8.5 Business-as-usual forest management, and RCP 8.5 replacement of coniferous forests with deciduous forests). The y-axis ranges from 100 to 125 t C/ha.
4. Discussion
Forest sciences have a rich body of information on the effect of tree species on soil properties. Differences on the soil organic carbon stocks are driven by the species-specific growth rates and the allocation of photosynthates to above- or belowground tissue. Common garden experiments and case studies are taking place under site conditions that support an experiment but are not necessarily representative for regions (Binkley, 2020). Moreover, in a common setting in Central Europe the distribution of tree species is not primarily driven by the expected formation of a stock of soil organic carbon but rather by management decisions of forest practitioners. Niche models are a powerful tool for the prediction of future tree species compositions that are based on site conditions and to some extent on the competitive advantages of different tree species (Zimmermann et al., 2009; Chauvier et al., 2021). Yet, forest management decisions are not reflected.
The data of the Austrian Forest Soil Survey do not allow a deep stratification of the data set to the identification of the effects of single tree species except for Norway spruce because strata sizes are forbiddingly low. Our analysis is therefore hampered by pooling all deciduous tree species into one group, even though differences in the decomposition rate among deciduous trees are known (Gustafson, 1943). Further, deciduous forests in our study are not strictly composed of deciduous trees, but hold a fair share of coniferous tree species. Hence, we are referring to “mixed-deciduous forests.” Strictly deciduous forests are only found in some regions in the east of the country. In other regions, the dogma of “as much coniferous tree species as possible, as much deciduous tree species as required” has been widely followed. The dogma is based on the demand of the Austrian timber market, where timber from conifers, in particular Norway spruce, is in higher esteem than timber from deciduous trees. Lumping all deciduous trees into one group does not have a decisive impact on our topic. The influence of external effects on leaf decomposition rates is larger than the difference between the litter of different deciduous tree species (Cornelissen et al., 2007).
Ground truth based on the Austrian Forest Soil Survey reinforces existing knowledge that coniferous forests have higher soil organic carbon stocks than mixed-deciduous forests. This applies both for the organic surface layer and the upper 50 cm of the mineral soil and independent of the geological bedrock material (Table 2). The depth gradient of carbon in the mineral soil shows higher organic carbon stocks in the upper soil profile of coniferous forests, because there the rooting density is higher and some carbon leaches out of the organic surface layer. Our data do not confirm the theory, that deciduous tree species lead to higher carbon stocks in deeper parts of the mineral soil due to their tendency to root deeper (Figure 5). Most soils in Austrian forests are rather shallow. Deeply weathered soils in low-elevation areas are predominantly under agricultural use where slope inclinations allow for it. The majority of soil organic carbon resides in the organic litter layer and the upper 30 cm of the mineral soil. The expectations of deeper distribution of organic carbon due to the decomposition of organic matter due to deep-rooting deciduous trees as compared to the shallow rooting Norway spruce is not supported by the analysis of national data due to insufficient information on subsoil horizons. The relevant information may be more efficiently derived from case studies where the experimental setup is specifically addressing deep soil horizons.
However, the encountered differences between forest types (“coniferous forests” vs. “mixed-deciduous forests”) are not as clear-cut as the results from common-garden experiments and case studies would suggest. We emphasize that our observation of differences in total soil organic carbon stocks are partially an effect of tree species, partially owed to site conditions, and mostly driven by forest management decisions. Firstly, the distribution of total soil organic carbon stocks for different forest types is rather similar. The difference is caused by a higher frequency of coniferous forests on sites with above-average soil carbon stocks such as peatlands (Figure 2). Secondly, even more important, is the geographical location of different forest types. Deciduous forests are mostly confined to low-elevation sites because many deciduous tree species cannot tolerate site conditions in higher elevation. Coniferous forests are encountered in low elevation where they are often replacing naturally dominating deciduous tree species, and in high elevation, where they are dominating for ecological reasons. In addition, the Inner Alps, that are characterized by a continental cool climate, are naturally dominated by coniferous tree species. At low-elevation sites generally a quicker turnover of soil organic carbon is expected. At high elevation under cooler conditions the productivity of forests and the soil microbial activity is low. Our data corroborate that site conditions in high elevation are supporting the accumulation of soil organic carbon. Finally, the spatial distribution of tree species is mostly reflecting the decisions of the forest manager at work in the particular region who is choosing his tree species under consideration of economic effects of his forest-ecological system understanding.
Modeling results allow analyzing the long-term consequences of climate warming under realistic conditions where the ecological dynamics of forest ecosystems are embedded in management decisions of the timber producing sector. The difference to case studies and common-garden experiments is that on top of tree species characteristics forest management decisions are reflected in the results. The gradual increase in soil organic carbon stocks in a moderate warming scenario (RCP 4.5) reflects that currently the productivity of many mountain forests is constrained by the brevity of the growing season. Increasing temperature as the current growth-limiting factor will increase forest productivity in mountain forests (Lexer et al., 2015; Pretzsch et al., 2020). Under conditions of increasing biomass production an increase in the total soil carbon stock is expected (Figure 7). Even under increased warming rates (RCP 8.5; business-as-usual) this effect prevails for several decades. However, eventually climate conditions become problematic for Norway spruce and the growth rate declines. As a consequence of decreasing litter input to the soil and higher microbial activity the soil organic carbon stock declines.
The “deciduous tree species scenario” was embedded in the extreme climate change scenario RCP 8.5. With hindsight to the strong warming trend of the RCP 8.5 and envisioned accumulating disturbances of forests we assumed that all forest managers are changing their management strategy. The strategy was replacing Norway spruce with oak, beech, or maple, depending on the extent of climate change (Table 1). We pragmatically omitted the option of admitting non-native tree species because we lack the required species-specific model parameters. Thereby, we adhere to the claim of nature conservation of not-introducing additional tree species, even though we entertain this future option based on a more solid knowledge basis that is currently emerging. However, we followed the scheme of assisted migration because we simply introduced the deciduous tree species instead of enabling their slow natural expansion into a new habitat (Chakraborty et al., 2019).
In Figure 7, it is shown that in “RCP 8.5—deciduous tree species” soil organic carbon stocks are increasing as compared to “RCP 8.5—business-as-usual” in the second half of the century. This trend rather reflects several processes that cannot be dis-entangled with the available data. Firstly, the most productive forest sites are currently populated by coniferous forests. Deciduous tree species may be able to establish comparably high soil organic carbon stocks. An unintended effect is the lack of demand for timber from deciduous forests. A known challenge of the conversion of conifer to deciduous forests is the lack of demand for timber from deciduous trees, because the wood-technology options are still limited and hardwood timber still cannot replace timber of Norway spruce. Therefore, deciduous forests are under-used until the remaining conifer forests can supply the demand for timber and can develop with less disturbance. However, we cannot rule out that the simulation is overestimating the increase of soil organic carbon stocks under deciduous trees. It is possible that our biomass functions for the foliage of deciduous trees are delivering values on the high end of the natural spectrum. Hence, a high estimate of leaf biomass translates into high annual influx of organic material via aboveground litterfall. A second potential source for bias is the uncertainty of the belowground litterfall via root turnover that is pertinent in soil carbon models (Brunner et al., 2013). Thirdly, the results are quantitatively emphasized because the scenario holds that all forest managers adopt the same adaptation strategy in order to cope with climate change.
Irrespective of tree species selection the forest soil organic carbon stock declines in a strong warming scenario due to the establishment of a new equilibrium level of lower productivity of forests and increasing decomposition rates of soil organic matter. This finding reflects that carbon sequestration in the biomass and the soils of forest ecosystems is only a temporary solution. Concepts such as increasing the forest area on a global scale can buy additional time but in the long term are not capable to resolve the challenge of climate change (Bastin et al., 2020).
Figure 6 shows the current dominance of coniferous forests in Austria. Only in the Eastern and South-Eastern part of the country as well as along major valleys deciduous trees are currently more abundant than coniferous tree species. In a strong climate warming scenario within only 50 years, i.e., half a lifetime of trees, deciduous trees are gradually replacing conifers, because sites at medium altitudes in mountains are becoming increasingly suitable for their growth. This scenario is indicative, but more a reflection of a gedanken experiment than a real future situation. The scenario assumes that all forest owners follow the mindset of abandoning coniferous tree species when prognosticated climate conditions advise against them. In a real world situation we rather expect that a group of forest managers follows the ecological advise, whereas others adhere to traditional beliefs of forestry, and will continue favoring coniferous tree species at sites that are no longer suitable for them. Such a position is perfectly understandable, given that no convincing model has been presented showing that timber from deciduous trees can replace the Norway-spruce dominated economic model. In reality, the climate-change induced shift from coniferous forests to mixed-deciduous and deciduous forests will be a slower process. In the simulation experiment, after the year 2100 the tree species composition is not changing further. There are only some few forests sites in high elevation that are not suitable for deciduous trees. Except for that, forests will be dominated by deciduous tree species. Such a scenario has many implications, such as the aesthetics of the entire landscape, the provision of protective services by forests in mountain communities, and the regulatory effects of forests to air and water quality. The implications are not fully discussed here. Yet, the role of forests for society will need to be re-evaluated.
We emphasize that we are showing an extreme scenario that shows a potential for the future distribution of forests dominated by deciduous trees. It can be overruled by alternative forest management decisions. Main reasons are the driven by the demand side of the timber market. Due to the technological properties of timber from Norway spruce, forest managers will seek options to grow Norway spruce and may accept an increasing production risk. Current wood technology does not have the means to replace coniferous wood with timber from deciduous trees. In addition, the implemented rule for switching from coniferous to deciduous tree species may be instrumental, yet overly simple. In high-elevation forests the decision to implement a change of tree species may be more complex. An example is the exposure of trees to eventual extreme events such as early and late frost that are not reflected in the described rule. We therefore insist, that our scenarios depict a possible/potential future. Regional or local forest management decisions will rely on more refined assumptions on the appropriate choice of tree species.
Data Availability Statement
The datasets can be requested from the corresponding author. Data access is provided upon reasonable requests and in compliance with the data policy of the Austrian Forest Research Center.
Author Contributions
RJ wrote the manuscript. He participated in the discussions and provided input with respect to soil carbon modeling. He further analyzed and interpreted the data of the Austrian Forest Soil Survey. TL simulated forest growth and provided input to the writing. GK simulated the Yasso07 runs and gave critical input to the manuscript. He programmed the visualization of the effect of tree species change. PW coordinated the project that provided the forest and forest soil simulations. He also established the first benchmark for organic carbon stocks in Austrian forests and forest soils. All authors contributed to the article and approved the submitted version.
Funding
The research has been funded by funds of the Austrian Climate Research Programme ACRP (CareForParis and CASAS) and base funding of the Austrian Forest Research Center (BFW) that was provided by the Federal Austrian Ministry of Regions, Tourism, and Sustainability for the implementation of the Austrian Forest Soil Survey.
Conflict of Interest
The authors declare that the research was conducted in the absence of any commercial or financial relationships that could be construed as a potential conflict of interest.
Acknowledgments
We acknowledge the considerable contribution of our colleagues Franz Mutsch and Robert Hacker who have initially analyzed the data set of the Austrian forest soil survey in great detail and have left us much too early. Moreover, we acknowledge the efforts of Walter Kilian and Michael Englisch who have initiated and implemented the Austrian Forest Soil Survey, and the consortium members of the ACRP project CareForParis.
References
Achilles, F., Tischer, A., Bernhardt-Römermann, M., Heinze, M., Reinhardt, F., Makeschin, F., et al. (2020). European beech leads to more bioactive humus forms but stronger mineral soil acidification as Norway spruce and Scots pine-Results of a repeated site assessment after 63 and 82 years of forest conversion in Central Germany. For. Ecol. Manage. 2020:118769. doi: 10.1016/j.foreco.2020.118769
Andivia, E., Rolo, V., Jonard, M., Formánek, P., and Ponette, Q. (2016). Tree species identity mediates mechanisms of top soil carbon sequestration in a Norway spruce and European beech mixed forest. Ann. For. Sci. 73, 437–447. doi: 10.1007/s13595-015-0536-z
Bastin, J.-F., de Haulleville, T., Maniatis, D., Marchi, G., Massacessi, E., Mollicone, D., et al. (2020). The Restoration Potential in the European Union. Technical Report 070202/2020/825508/ETU/ENV.D.I, EU Commission.
Beaudette, D., Roudier, P., and O'Geen, A. (2013). Algorithms for quantitative pedology: a toolkit for soil scientists. Comput. Geosci. 52(Suppl. C), 258–268. doi: 10.1016/j.cageo.2012.10.020
Berger, T. W., Neubauer, C., and Glatzel, G. (2002). Factors controlling soil carbon and nitrogen stores in pure stands of Norway spruce (Picea abies) and mixed species stands in Austria. For. Ecol. Manage. 159, 3–14. doi: 10.1016/S0378-1127(01)00705-8
Berger, T. W., Swoboda, S., Prohaska, T., and Glatzel, G. (2006). The role of calcium uptake from deep soils for spruce (Picea abies) and beech (Fagus sylvatica). For. Ecol. Manage. 229, 234–246. doi: 10.1016/j.foreco.2006.04.004
Binkley, D. (2020). Ecology and Management of Forest Soils, 5th Edn. New York, NY: Wiley. doi: 10.1002/9781119455745
Bircher, N., Cailleret, M., Huber, M., and Bugmann, H. (2015). Empfindlichkeit typischer schweizer waldbestände auf den klimawandel. Schweizer. Zeitsch. Forstw. 166, 408–419. doi: 10.3188/szf.2015.0408
Braun, M., Fritz, D., Braschel, N., Büchsenmeister, R., Freudenschuss, A., Gschwantner, T., et al. (2016). A holistic assessment of green house gas dynamics from forests to the effects of wood products use in Austria. Carbon Manage. 7, 271–283. doi: 10.1080/17583004.2016.1230990
Braun, M., Schwarzbauer, P., and Hesser, F. (2020). Wirtschaftliche entwicklung des forst- und holzsektors - eine analyse der wettbewerbsfähigkeit. BFW Praxisinform. 51, 25–28.
Brunner, I., Bakke, M. R., Björk, R. G., Hirano, Y., Lukac, M., Aranda, X., et al. (2013). Fine-root turnover rates of European forests revisited: an analysis of data from sequential coring and ingrowth cores. Plant Soil 362, 357–372. doi: 10.1007/s11104-012-1313-5
Chakraborty, D., Gaviria, J., Bednárová, D., Bolte, A., Bouissou, C., Buchacher, R., et al. (2019). Implementing assisted migration. Output of the INTERREG CENTRAL EUROPE Programme 2014-2020. D. SUSTREE Policy Brief 2. doi: 10.3220/DATA20191016132031
Chauvier, Y., Thuiller, W., Brun, P., Lavergne, S., Descombes, P., Karger, D. N., et al. (2021). Influence of climate, soil, and land cover on plant species distribution in the European Alps. Ecol. Monogr. 2021:e01433. doi: 10.1002/ecm.1433
Chimani, B., Heinrich, G., Hofstätter, M., Kerschbaumer, M., Kienberger, S., Leuprecht, A., et al. (2016). Endbericht ÖKS15 - Klimaszenarien für Österreich. CCCA Data Centre. Available online at: https://hdl.handle.net/20.500.11756/06edd0c9 (accessed February 5, 2018).
Cornelissen, J. H., Van Bodegom, P. M., Aerts, R., Callaghan, T. V., Van Logtestijn, R. S., Alatalo, J., et al. (2007). Global negative vegetation feedback to climate warming responses of leaf litter decomposition rates in cold biomes. Ecol. Lett. 10, 619–627. doi: 10.1111/j.1461-0248.2007.01051.x
De Vos, B., Cools, N., Ilvesniemi, H., Vesterdal, L., Vanguelova, E., and Carnicelli, S. (2015). Benchmark values for forest soil carbon stocks in Europe: results from a large scale forest soil survey. Geoderma 251–252, 33–46. doi: 10.1016/j.geoderma.2015.03.008
deB Richter, D. JR., and Markewitz, D. (2001). Understanding Soil Change - Soil Sustainability Over Millenia, Centuries, and Decades. Cambridge: Cambridge University Press.
deB. Richter, D., and Billings, S. A. (2015). Tansley review – “one physical system”: Tansley's ecosystem as earth's critical zone. New Phytol. 206, 900–912. doi: 10.1111/nph.13338
Didion, M., Blujdea, V., Grassi, G., Hernández, L., Jandl, R., Kriiska, K., et al. (2016). Models for reporting forest litter and soil c pools in national greenhouse gas inventories: methodological considerations and requirements. Carbon Manage. 7, 79–92. doi: 10.1080/17583004.2016.1166457
Fischer, H., Bens, O., and Hüttl, R. (2002). Veränderung von Humusform, -vorrat und -verteilung im Zuge von Waldumbau-Massnahmen im nordostdeutschen Tiefland. Forstwissensch. Centralblatt 121, 322–334. doi: 10.1046/j.1439-0337.2002.02037.x
Foldal, C., Bohner, A., Jandl, R., and Berger, A. (2021). Deriving regional pedotransfer functions to estimate soil bulk density in Austria. J. Land Manage. Food Environ. In Press.
Gosheva, S., Walthert, L., Niklaus, P. A., Zimmermann, S., Gimmi, U., and Hagedorn, F. (2017). Reconstruction of historic forest cover changes indicates minor effects on carbon stocks in Swiss forest soils. Ecosystems 20, 1512–1528. doi: 10.1007/s10021-017-0129-9
Grüneberg, E., Schöning, I., Riek, W., Ziche, D., and Evers, J. (2019). “Carbon stocks and carbon stock changes in German forest soils,” in Status and Dynamics of Forests in Germany : Results of the National Forest Monitoring, eds N. Wellbrock, and A. Bolte (Cham: Springer International Publishing), 167–198. doi: 10.1007/978-3-030-15734-0_6
Grüneberg, E., Ziche, D., and Wellbrock, N. (2014). Organic carbon stocks and sequestration rates of forest soils in Germany. Glob. Change Biol. 20, 2644–2662. doi: 10.1111/gcb.12558
Gustafson, F. G. (1943). Decomposition of the leaves of some forest tree species under field conditions. Tree Physiol. 18, 704–707. doi: 10.1104/pp.18.4.704
Hager, H. (1988). Stammzahlreduktion - die auswirkungen auf wasser-, energie- und nährstoffhaushalt von fichtenjungwüchsen. Forstl. Schriftenr. Univ. Bodenk. 1, 1–189.
Hanewinkel, M., Cullmann, D. A., Schelhaas, M.-J., Nabuurs, G.-J., and Zimmermann, N. E. (2012). Climate change may cause severe loss in the economic value of European forest land. Nat. Clim. Change 3, 203–207. doi: 10.1038/nclimate1687
Harrison, R. B., Footen, P. W., and Strahm, B. D. (2011). Deep soil horizons: contribution and importance to soil carbon pools and in assessing whole-ecosystem response to management and global change. For. Sci. 57, 67–76. doi: 10.1093/forestscience/57.1.67
Hernández, L., Jandl, R., Blujdea, V. N., Lehtonen, A., Kriiska, K., Alberdi, I., et al. (2017). Towards complete and harmonized assessment of soil carbon stocks and balance in forests: the ability of the Yasso07 model across a wide gradient of climatic and forest conditions in Europe. Sci. Tot. Environ. 599–600, 1171–1180. doi: 10.1016/j.scitotenv.2017.03.298
Hlásny, T., Krokene, P., Liebhold, A., Montagné-Huck, C., Müller, J., Qin, H., et al. (2019). Living With Bark Beetles: Impacts, Outlook and Management Options, Vol. 8 of From Science to Policy. European Forest Institute.
Jacob, D., Petersen, J., Eggert, B., Alias, A., Christensen, O. B., Bouwer, L. M., et al. (2014). Euro-cordex: new high-resolution climate change projections for European impact research. Region. Environ. Change 14, 563–578. doi: 10.1007/s10113-013-0499-2
Jandl, R. (2020). Climate-induced challenges of Norway spruce in Northern Austria. Trees For. People 1:100008. doi: 10.1016/j.tfp.2020.100008
Jandl, R., Ledermann, T., Kindermann, G., Freudenschuss, A., Gschwantner, T., and Weiss, P. (2018). Strategies for climate-smart forest management in Austria. Forests 9. doi: 10.3390/f9100592
Knoke, T., Kindu, M., Jarisch, I., Gosling, E., Friedrich, S., Bödeker, K., and Paul, C. (2020). How considering multiple criteria, uncertainty scenarios and biological interactions may influence the optimal silvicultural strategy for a mixed forest. For. Pol. Econ. 118:102239. doi: 10.1016/j.forpol.2020.102239
Ledermann, T., Kindermann, G., and Gschwantner, T. (2017). “Chapter 6: National woody biomass projection systems based on forest inventory in Austria,” in Forest Inventory-Based Projection Systems for Wood and Biomass Availability eds Barreiro, S., Schelhaas, M.-J., McRoberts, R.E., Kändler, G. (New York, NY: Springer International), 79–95. doi: 10.1007/978-3-319-56201-8_6
Lexer, M. J., Jandl, R., Nabernegg, S., and Bednar-Friedl, B. (2015). “Forestry,” in Economic Evaluation of Climate Change Impacts- Development of a Cross-Sectoral Framework and Results for Austria, eds K. W. Steininger, M. König, B. Bednar-Friedl, L. Kranzl, W. Loibl, and F. Prettenthaler (New York, NY: Springer), 145–165. doi: 10.1007/978-3-319-12457-5_9
Lindner, M., Schwarz, M., Spathelf, P., de Koning, J. H., Jandl, R., Viszlai, I., et al. (2020). Adaptation to Climate Change in Sustainable Forest Management in Europe. Bratislava: Liaison Unit.
Liski, J., Palosuo, T., Peltoniemi, M., and Sievänen, R. (2005). Carbon and decomposition model Yasso for forest soils. Ecol. Model. 189, 168–182. doi: 10.1016/j.ecolmodel.2005.03.005
Liski, J., Tuomi, M., and Rasinmäki, J. (2009). Yasso07 User-Interface Manual. Technical report, Finnish Environment Institute.
Markewitz, D., Sartori, F., and Craft, C. (2002). Soil change and carbon storage in longleaf pine stands planted on marginal agricultural lands. Ecol. Appl. 12, 1276–1285. doi: 10.1890/1051-0761(2002)012[1276:SCACSI]2.0.CO;2
Mayer, M., Prescott, C. E., Abaker, W. E., Augusto, L., Cécillon, L., Ferreira, G. W., et al. (2020). Influence of forest management activities on soil organic carbon stocks: a knowledge synthesis. For. Ecol. Manage. 466:118127. doi: 10.1016/j.foreco.2020.118127
Monserud, R. A., and Sterba, H. (1996). A basal area increment model for individual trees growing in even- and uneven-aged forest stands in Austria. For. Ecol. Manage. 80, 57–80. doi: 10.1016/0378-1127(95)03638-5
Oostra, S., Majdi, H., and Olsson, M. (2006). Impact of tree species on soil carbon stocks and soil acidity in southern Sweden. Scand. J. For. Res. 21, 364–371. doi: 10.1080/02827580600950172
Osei, R., Titeux, H., Bielak, K., Bravo, F., Collet, C., Cools, C., et al. (2021). Tree species identity drives soil organic carbon storage more than species mixing in major two-species mixtures (pine, oak, beech) in Europe. For. Ecol. Manage. 481:118752. doi: 10.1016/j.foreco.2020.118752
Pan, Y., Birdsey, R. A., Fang, J., Houghton, R., Kauppi, P. E., Kurz, W. A., et al. (2011). A large and persistent carbon sink in the world's forests. Science 333, 988–993. doi: 10.1126/science.1201609
Paul, C., Brandl, S., Friedrich, S., Falk, W., Härtl, F., and Knoke, T. (2019). Climate change and mixed forests: how do altered survival probabilities impact economically desirable species proportions of Norway spruce and European beech? Ann. For. Sci. 76:14. doi: 10.1007/s13595-018-0793-8
Post, W. M., Emanuel, W. R., Zinke, P. J., and Stangenberger, A. G. (1982). Soil carbon pools and world life zones. Nature 298, 156–159. doi: 10.1038/298156a0
Pretzsch, H., Hilmers, T., Biber, P., Avdagić, A., Binder, F., Bončina, A., et al. (2020). Evidence of elevation-specific growth changes of spruce, fir, and beech in European mixed mountain forests during the last three centuries. Can. J. For. Res. 50, 689–703. doi: 10.1139/cjfr-2019-0368
Prietzel, J., and Bachmann, S. (2012). Changes in soil organic C and N stocks after forest transformation from Norway spruce and Scots pine into Douglas fir, Douglas fir/spruce, or European beech stands at different sites in Southern Germany. For. Ecol. Manage. 269, 134–148. doi: 10.1016/j.foreco.2011.12.034
R Core Team (2017). R: A Language and Environment for Statistical Computing. Vienna: R Foundation for Statistical Computing.
Rasse, D. P., Rumpel, C., and Dignac, M.-F. (2005). Is soil carbon mostly root carbon? Mechanisms for a specific stabilisation. Plant Soil 269, 341–356. doi: 10.1007/s11104-004-0907-y
Rehschuh, S., Jonard, M., Wiesmeier, M., Rennenberg, H., and Dannenmann, M. (2021). Impact of European beech forest diversification on soil organic carbon and total nitrogen stocks–a meta-analysis. Front. For. Glob. Change 4:2. doi: 10.3389/ffgc.2021.606669
Riahi, K., Rao, S., Krey, V., Cho, C., Chirkov, V., Fischer, G., et al. (2011). RCP 8.5 -a scenario of comparatively high greenhouse gas emissions. Climat. Change 109:33. doi: 10.1007/s10584-011-0149-y
Rogelj, J., Popp, A., Calvin, K. V., Luderer, G., Emmerling, J., Gernaat, D., et al. (2018). Scenarios towards limiting global mean temperature increase below 1.5 °C. Nat. Clim. Change 8, 325–332. doi: 10.1038/s41558-018-0091-3
Schmid, I., and Kazda, M. (2002). Root distribution of Norway spruce in monospecific and mixed stands on different soils. For. Ecol. Manage. 159, 37–47. doi: 10.1016/S0378-1127(01)00708-3
Schwarzbauer, P. (2018). Bedeutung des Wald-Basierten Sektors in der osterreichischen Gesamtwirtschaft-Anteile des Sektors an Gesamtwirtschaftlichen Bezugsgrössen. BOKU.
Seidl, R., Thom, D., Kautz, M., Martin-Benito, D., Peltoniemi, M., Vacchiano, G., et al. (2017). Forest disturbances under climate change. Nat. Clim. Change 7, 395–402. doi: 10.1038/nclimate3303
Smal, H., Ligȩza, S., Pranagal, J., Urban, D., and Pietruczyk-Popławska, D. (2019). Changes in the stocks of soil organic carbon, total nitrogen and phosphorus following afforestation of post-arable soils: a chronosequence study. For. Ecol. Manage. 451:117536. doi: 10.1016/j.foreco.2019.117536
Strohschneider, I. (1991). Mittelfristige Veränderungen des Bodenzustandes auf Exaktdüngungsflächen. Mitteil. Forstlich. Bundesversuch. 167, 1–200.
Trumbore, S. E., and Gaudinski, J. B. (2003). The secret live of roots. Science 302, 1344–1345. doi: 10.1126/science.1091841
Vesterdal, L., Clarke, N., Sigurdsson, B., and Gundersen, P. (2013). Do tree species influence soil carbon stocks in temperate and boreal forests? For. Ecol. Manage. 309, 4–18. doi: 10.1016/j.foreco.2013.01.017
Vesterdal, L., Schmidt, I. K., Callesen, I., Nilsson, L. O., and Gundersen, P. (2008). Carbon and nitrogen in forest floor and mineral soil under six common European tree species. For. Ecol. Manage. 255, 35–48. doi: 10.1016/j.foreco.2007.08.015
Keywords: Austrian forest soil survey, coniferous forest, mixed-deciduous forest, soil organic carbon stock, climate change mitigation, climate change adaptation
Citation: Jandl R, Ledermann T, Kindermann G and Weiss P (2021) Soil Organic Carbon Stocks in Mixed-Deciduous and Coniferous Forests in Austria. Front. For. Glob. Change 4:688851. doi: 10.3389/ffgc.2021.688851
Received: 31 March 2021; Accepted: 18 May 2021;
Published: 16 June 2021.
Edited by:
Jeff Allen Hatten, Oregon State University, United StatesReviewed by:
Nicole Wellbrock, Thuenen Institute of Forest Ecology, GermanyJörg Luster, Swiss Federal Institute for Forest, Snow and Landscape Research (WSL), Switzerland
Copyright © 2021 Jandl, Ledermann, Kindermann and Weiss. This is an open-access article distributed under the terms of the Creative Commons Attribution License (CC BY). The use, distribution or reproduction in other forums is permitted, provided the original author(s) and the copyright owner(s) are credited and that the original publication in this journal is cited, in accordance with accepted academic practice. No use, distribution or reproduction is permitted which does not comply with these terms.
*Correspondence: Robert Jandl, cm9iZXJ0LmphbmRsJiN4MDAwNDA7YmZ3Lmd2LmF0