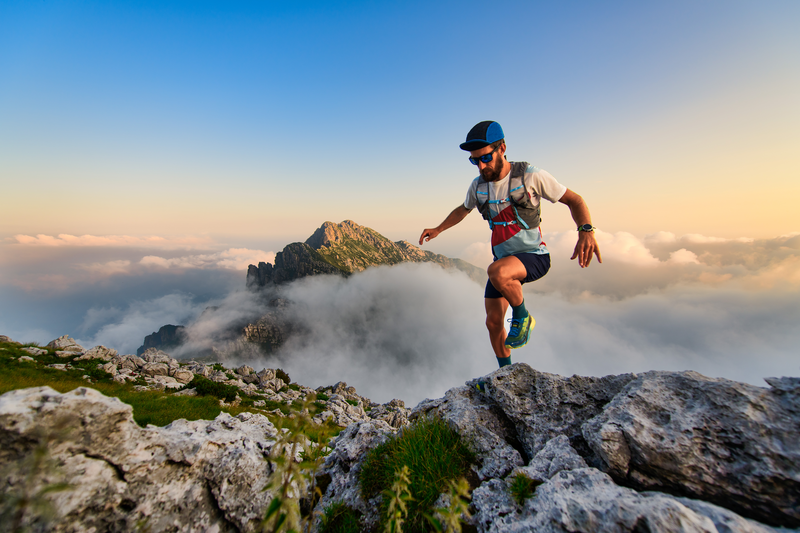
95% of researchers rate our articles as excellent or good
Learn more about the work of our research integrity team to safeguard the quality of each article we publish.
Find out more
ORIGINAL RESEARCH article
Front. For. Glob. Change , 14 July 2021
Sec. Forest Management
Volume 4 - 2021 | https://doi.org/10.3389/ffgc.2021.684087
This article is part of the Research Topic Proceedings of the 21st Biennial Southern Silvicultural Research Conference View all 8 articles
Emulating natural disturbance has become an increasingly important restoration strategy. In the fire-maintained woodlands of the southeastern United States, contemporary restoration efforts have focused on approximating the historical fire regime by burning at short intervals. Due to concerns over escape and damage to mature trees, most prescribed burning has occurred in the dormant season, which is inconsistent with the historical prevalence of lightning-initiated fire in the region. This discordance between contemporary prescribed burning and what is thought to be the historical fire regime has led some to question whether dormant season burning should remain the most common management practice; however, little is known about the long-term effects of repeated growing season burning on the health and productivity of desirable tree species. To address this question, we report on a long-term experiment comparing the effects of seasonal biennial burning (winter, spring, and summer) and no burning on the final survival status, height, diameter, and volume growth of 892 mature longleaf pine (Pinus palustris) over 23 years in three mature even-aged stands in southern Alabama, United States. Overall, longleaf pine survival across all treatments averaged 81 ± 2% [s.e]. Among seasonal burn treatments, survival was highest in the spring burns (82 ± 4%) but did not vary significantly from any other treatment (summer – 79 ± 4%, winter – 81 ± 4%, unburned – 84 ± 4%). However, survival was statistically influenced by initial diameter at breast height, as survival of trees in the largest size class (30 cm) was 40% higher than trees in the smallest size class (5 cm). Productivity of longleaf pine was not significantly different among treatment averages in terms of volume (38.9–44.1 ± 6.0 m3 ha–1), diameter (6.0–6.7 ± 0.3 cm), and height (2.5–3.4 ± 0.4 m) growth. Collectively, our results demonstrate that burning outside the dormant season will have little impact on mature longleaf pine survival and growth. This finding has important implications for the maintenance of restored southeastern woodlands, as interest in burning outside the dormant season continues to grow.
Restoring degraded woodlands has become an international conservation priority (Dey et al., 2017; Buisson et al., 2019; Gordon et al., 2020). Prescribed fire often plays a key role in restoration prescriptions. Efforts to reestablish historical burning regimes have appropriately focused on fire frequency, which has been shown to have prominent effects on ecosystem response (Mitchell et al., 2006). For example, frequent, low intensity surface fire (2–4 years) is generally recommended for maintaining forest structure and promoting understory diversity in the longleaf pine ecosystem of the southeastern United States (Van Lear et al., 2005). However, a lack of consideration for other factors, such as fire season, may result in undesirable restoration outcomes. In fact, there is growing concern that contemporary burning operations are oversimplified and may be adversely affecting wildlife habitat and diversity (Lashley et al., 2015, 2017; Darracq et al., 2016; Hiers et al., 2016).
Prior to European settlement, fires in the southeastern United States were often created by lightning strikes in the growing season (Robbins and Myers, 1992; Outcalt, 2008; Ryan et al., 2013). Although application of prescribed fire in the growing season has increased over the past three decades (Ferguson, 1998; Cronan et al., 2015), dormant season burning remains the most common practice to mitigate risk (Stanturf et al., 2002; Knapp et al., 2009). This discordance in seasonal burning could have implications for fire behavior and subsequent fire effects. For example, while the risk of crown scorch increases with ambient temperature, growing season burns typically occur with lower available fuel loads, higher relative humidity, higher fine fuel moisture contributed by green herbaceous plants, and result in lower fire intensity compared to dormant season burns (Sparks et al., 2002; Govender et al., 2006). Moreover, growing season burns are considered beneficial for controlling hardwood encroachment and promoting wiregrass (Aristida stricta) reproduction, which are often objectives in woodland restoration (Outcalt, 1994; Glitzenstein et al., 1995; Haywood et al., 2001; Robertson and Hmielowski, 2014; Addington et al., 2015).
While burning outside the dormant season corresponds more closely with historical burning patterns and may be beneficial for certain aspects of ecological restoration, concerns remain about the long-term impacts of repeated growing season fire on overstory health and productivity. Limiting root damage is an important consideration for protecting desirable tree species during prescribed burning (Hood et al., 2018). Longleaf pine (Pinus palustris), a foundational tree species in southeastern woodlands, is well-adapted to survive surface fire but is not impervious to fire effects (Boyer, 1990). Previous research has indicated that growing season burns can inhibit root growth leading to speculation that dormant season burns are preferable for maintaining longleaf pine productivity (Sayer and Haywood, 2006). Growing season burns may also negatively affect non-structural carbohydrate stocks, which could further constrain recovery and productivity (Guo et al., 2004; Sayer et al., 2020). Such negative effects may be magnified in locations where fire has long-been excluded, as burning in stands with accumulated understory vegetation and duff can produce higher fire intensity (Menges and Deyrup, 2001), reduce non-structural carbohydrate reserves (Varner et al., 2009), and enhance post-fire physiological stress (O’Brien et al., 2010).
Recent evidence documenting the effects of fire seasonality on longleaf pine productivity has been inconclusive. After 10 years of dormant and growing-season biennial burning in young stands, Boyer (1987) reported an overall loss of productivity from burning but that burn season was of little consequence. Similarly, longleaf pine growth has been shown to recover rapidly after growing season burns regardless of fire frequency (Ford et al., 2010). However, after 37 years of growing-season biennial burning, Haywood et al. (2001) reported that summer burned longleaf pine were shorter and contained less volume than those burned in late spring. While our understanding of seasonal burning on longleaf pine productivity has improved, to our knowledge, no studies have examined the effects of repeated seasonal biennial burning at the latter stages of a timber rotation. Moreover, while it is generally accepted that the risk of mortality decreases with increasing size (Hare, 1965; Jackson et al., 1999; Hood et al., 2018), little is known if this relationship varies with fire season.
To answer these questions, we build upon a long-term experiment (Boyer, 1987) to assess survival and growth of longleaf pine after 23-years of seasonal biennial burning (winter, spring, and summer) and an unburned treatment in three 37-year-old even-aged stands in southern Alabama, United States. Specifically, we asked whether: (1) biennial burning outside the dormant season will negatively affect longleaf pine survival and productivity and, (2) fire resistance increases with tree size in all burn seasons. We hypothesized that: (1) burning outside the dormant season will not affect longleaf pine survival and productivity and, (2) fire resistance will increase with tree size in all burn seasons. The results of this study will contribute to our functional understanding of seasonal burning in the longleaf pine ecosystem and will address a fundamental debate surrounding the application of prescribed fire in the southeastern United States.
The study was conducted at the Escambia Experimental Forest near Brewton, Alabama, United States (31°01′N, 87°04′W). The climate is classified as humid subtropical with the hottest average temperatures occurring in August (22.7–32.8°C) and the coldest in January (4.4–16.7°C). Average growing season length is 250 days. Precipitation occurs almost exclusively in the form of rain and is abundant throughout the year (1,680 mm annual average). Soils at the site are generally classified as belonging to the Troup Series (loamy, kaolinitic, and thermic Grossarenic Kandiudults), which consist of sandy and loamy sediments that are somewhat excessively drained. Naturally regenerated longleaf pine is the dominant tree species at the site in stands receiving frequent prescribed fire (3-year return interval) (approximately 95% of the experimental forest). In stands where fire is less frequent or is excluded (approximately 5% of the experimental forest) longleaf pine occurs in a mixture of water oak (Quercus nigra), dogwood (Cornus florida), and sweetgum (Liquidambar styraciflua). The understory is dominated by a mix of shrub and herbaceous species primarily consisting of gallberry (Ilex spp.), bracken fern (Pteridium aquilinum), shiny blueberry (Vaccinium myrsinites), narrowleaf silkgrass (Pityopsis graminifolia), gopher apple (Geobalanus oblongifolius), and greenbriers (Smilax spp.). Site index for longleaf pine is estimated to be 25 m at age 50.
The study was originally established by Boyer (1987). Three even-aged longleaf pine stands (hereafter referred to as blocks) were used in the experiment. Each block naturally regenerated from the 1957–1958 seed crop with a seed tree harvest. At the initiation of the study in 1973 (stand age 15), the blocks were thinned to an average density of 1,236 trees ha–1. Twelve plots (0.16 ha) were established in each block and randomly assigned a treatment featuring fully crossed combinations of four seasonal biennial burning and three non-fire hardwood control methods in a randomized complete block design. The plots were square and located adjacent to each other within the blocks. Season of biennial burning (hereafter referred to as seasonal burning treatments) included winter (November–March), spring (April–May), summer (July–August), and an unburned treatment. Biennial burning began in 1974 and continued through 2018 (22 total burns). Burning was primarily applied as a strip head fire with flame lengths averaging <1 m and a rate of spread <2 m min–1. Non-fire hardwood control methods considered were 2, 4-D (a phenoxy herbicide, all woody stems in 1973), mechanical (hand clearing all woody stems > 1.3 m in height every 5 years), and no hardwood control. In 1990 (stand age 32), each plot was thinned to approximately 16–17 m2 ha–1 to reduce intraspecific competition.
In this report, the effect of non-fire hardwood control treatments was not considered, as they did not initially influence any growth metric (Boyer, 1987) and were not found to be statistically significant in preliminary analyses. Therefore, all non-fire hardwood control treatments were pooled within seasonal burning treatments in a split-plot design (three seasonal burn replicates per block). Beginning in 1995 (stand age 37), we remeasured total height (m) with a hypsometer, diameter (cm) at breast height (1.37 m) with a diameter tape, and visually assessed survival status of marked longleaf pine trees in an internal measurement plot (0.1 ha) (Figure 1). Additional measurements of each metric were also taken in 1998, 2003, 2009, 2014, and 2018. Survival was determined by the presence of a living crown. Changes in growth and survival were calculated over the entire 23-year increment (1995–2018). At the start of this study, average longleaf pine diameter and height across treatments ranged from 19.3–22.1 cm and 20.0–22.2 m, respectively. For further detail on the experimental design see Boyer (1987) or Kush et al. (1999).
We used a mixed-effects analysis of variance (ANOVA) to compare the height, diameter, volume growth, and final survival percentage of longleaf pine established in different seasonal burning treatments over 23 years (1995–2018). Prior to analysis, all metrics of growth and survival were averaged at the plot level. Additionally, all longleaf pine that died over the duration of the experiment were removed from the height, diameter, and volume analyses. In each model, seasonal burning treatment was considered a fixed effect, while the interaction between block and seasonal burning treatment was considered a random effect. Significant treatment effects were further investigated with Tukey’s adjusted multiple comparison tests. Differences among seasonal treatments were considered significant at α = 0.05. These analyses were performed with the MIXED procedure in SAS 9.4 software (SAS Institute, Cary, NC, United States).
A generalized linear mixed-effects logistic regression was used to explore the effect of initial size on final survival status (1995–2018). The model assumed a binomial distribution with a logit link and estimated the probability of survival of individual longleaf pine (n = 892) with an odds ratio statistic. Parameter estimation was accomplished through Laplace approximation. Fixed effects in the model included seasonal burning treatment, initial diameter (tree age 37), and the interaction between seasonal burning treatment and initial diameter. The interaction between block and seasonal burning treatment was considered a random effect in the model. Factors were considered statistically significant at α = 0.05. This analysis was performed with the GLIMMIX procedure in SAS 9.4 software (SAS Institute, Cary, NC, United States).
Over 23 years, longleaf pine diameter growth averaged across all treatments was 6.1 ± 0.3 cm (30% increase). Diameter growth of longleaf pine ranged from 6.0–6.7 ± 0.3 cm and did not differ statistically among treatments (F = 0.51, P = 0.6889, Hedges’ g = 0.39) (Table 1 and Figure 2). Overall, longleaf pine height growth averaged 3.0 ± 0.2 m across all treatments (14% increase). Among treatments, height growth ranged from 2.5–3.4 ± 0.4 m and was not statistically significant (F = 0.95, P = 0.4630, Hedges’ g = 0.53) (Table 1 and Figure 2). Longleaf pine volume growth across all treatments averaged 41.0 ± 3.0 m3 ha–1 (62% increase). Volume growth ranged from 38.9–44.1 ± 6.0 m3 ha–1 and did not differ significantly among treatments (F = 0.14, P = 0.9357, Hedges’ g = 0.20) (Table 1 and Figure 2).
Table 1. Results of mixed-effects analyses of variance (ANOVA) for the effect of seasonal biennial fire (winter, spring, summer) and the unburned treatment on longleaf pine diameter growth, height growth, volume growth, and survival.
Figure 2. The estimated survival, diameter growth, height growth, and volume growth ± 1 SE of longleaf pine after 23 years of winter, spring, summer biennial burning and an unburned treatment.
Overall, longleaf pine survival averaged across all treatments was 81 ± 2%. Longleaf pine survival ranged from 79–84 ± 4% and did not differ statistically among treatments (F = 0.17, P = 0.9138, Hedges’ g = 0.22) (Table 1 and Figure 2). Initial diameter had a statistically significant positive association with survival across all treatments (F = 19.43, P < 0.0001) (Table 2 and Figure 3). The positive effect of initial diameter on survival was more evident in the winter and growing season burns compared to spring burns and the unburned treatment (data not shown); however, the interaction between initial diameter and seasonal treatment was not statistically significant (F = 1.35, P = 0.2558) (Table 2).
Table 2. Results of a generalized linear mixed-effects analysis of variance (ANOVA) for the effects of seasonal biennial burning (winter, spring, summer) and the unburned treatment, diameter, the interaction between seasonal biennial burning and diameter on the odds of survival for individual longleaf pine.
Figure 3. The estimated effect of initial diameter (37 years old) on longleaf pine survival averaged across all seasonal biennial burn treatments and the unburned treatment.
Dormant season burning has been a conventional management practice in the southeastern United States for decades. The rationale behind this paradigm is partially based on an ingrained cultural belief that growing season burning presents a greater risk to productivity than dormant season burning (Cary, 1932; Garren, 1943). Yet, studies examining the effects of seasonal burning on longleaf pine have produced conflicting evidence supporting (Haywood et al., 2001) and refuting this notion (Boyer, 1987; Ford et al., 2010). Our results indicate that biennially burned longleaf pine diameter, height, and volume growth were not affected by burn season, and thus provide further evidence that fire seasonality has little influence on longleaf pine productivity in a biennial burning regime. Finally, at least under the conditions of fire application and behavior studied, fire seasonality had no discernable impact on longleaf pine survival indicating that burning outside the dormant season does not inherently increase the risk of mortality in mature longleaf pine. However, it should be recognized that prescribed burning in this study was applied under favorable climatic conditions with conservative ignition techniques to minimize crown scorch. Burning under less-optimal conditions or with different ignition patterns may have resulted in a different outcome.
Two factors may have strongly contributed to our results. First, the trees evaluated in this experiment were 37 years-old at the start of the sampling period, and therefore likely possessed sufficient bark thickness and crown height to confer resistance to surface fire at the start of this study (Jackson et al., 1999). Evidence from a previous study reported an overall decline in productivity in all seasonal burning treatments at age 14–24 relative to the unburned treatment, which indicates that longleaf pine is more susceptible to negative fire effects at a younger age (Boyer, 1987). In similar studies featuring younger longleaf pine, Grelen (1975) and Haywood et al. (2001) found that summer burning negatively affected longleaf pine height and volume production compared to late spring burning. Outside the longleaf ecosystem, studies examining the influence of repeated prescribed fire have also reported minimal impacts on burning on larger, older trees (Peterson et al., 1994; Hutchinson et al., 2012; Bottero et al., 2017). Based on these collective findings, we hypothesize that biennial growing season burns present minimal risk to productivity once longleaf pine reaches 25 cm dbh in even-aged stands.
A second explanation for the minimal effect of fire seasonality may be related to the burning regime. Studies reporting high post-fire mortality in mature longleaf pine have generally occurred in stands where duff has accumulated after decades of fire exclusion (Kush et al., 2004; Varner et al., 2005, 2009; O’Brien et al., 2010). Such conditions promote smoldering fire behavior, which can damage trees through extended transfer of heat to the roots and cambium (Varner et al., 2009). In this study, plots were subjected to biennial burning for over two decades, which resulted in the exposure of mineral soil and little duff accumulation. While we can only speculate on the potential mechanisms influencing fire effects in our burn treatments, observational evidence of limited surface organic matter, scant midstory development, and relatively low flame lengths suggest that biennial burning maintained conditions that prevented smoldering and reduced crown scorch and consumption. Limiting damaging fire behavior may be particularly important for maintaining long term productivity, as prolonged soil heating has been shown to have negative short-term effects on radial growth and adverse long-term effects on growth when combined with drought (Varner et al., 2009; Slack et al., 2016). Moreover, crown scorch is considered the primary factor influencing mortality for some conifer species (Stephens and Finney, 2002; Hull-Sieg et al., 2006). Thus, it is possible that the fuel conditions created by repeated biennial burning may have overwhelmed any potential effects associated with seasonal burning.
Although mortality was generally low and did not differ across seasonal treatments, our results clearly demonstrate that risk of mortality diminished with increasing initial diameter. Similar results have been reported from other frequently burned ecosystems and is an expected result with longleaf pine given its rapid ability to accumulate bark thickness (Peterson and Reich, 2001; Ordóñez et al., 2005; Schafer et al., 2015). However, our results suggest that intraspecific competition rather than biennial burning was likely responsible for the death of smaller-diameter longleaf pine, as survival in the unburned treatment did not differ significantly from any seasonal biennial burning treatment. In support of this interpretation, calculations of stand density index revealed that all biennially burned plots had nearly reached or exceeded the self-thinning range of intraspecific competition by 2018 (Stand Density Index > 250) (Shaw and Long, 2007). Competition in the unburned treatments was undoubtedly higher due to the presence of established hardwood stems. Given that the smaller-diameter trees were almost always found in suppressed growing positions, and the relatively high light demands of longleaf pine (Boyer, 1990), it is not surprising that smaller-diameter trees were at greater risk of mortality.
The importance of incorporating regular prescribed fire in southeastern woodland restoration is well established (Van Lear et al., 2005). However, what remains relatively unknown is how other features of the burning regime, such as burn season, impact key ecosystem properties. Due to its importance for understory flammability, maintaining a productive longleaf pine overstory is a restoration objective (Mitchell et al., 2006). Thus, understanding the response of mature longleaf pine to fire seasonality is an important consideration in restoration prescriptions.
Under the conditions of fire application and behavior studied, our findings suggest a continuance of seasonal biennial burning has minimal impact on the survival and productivity of mature (37–60-year-old) longleaf pine. Similar findings have also been reported for longleaf pine in the 14–23 age range (Boyer, 1987). Collectively, these findings have important implications for woodland restoration at the regional scale, as many of the stands established in previous decades have reached the age where accumulated fire resistance minimizes the impact of fire seasonality in a biennial burn regime. Incorporating spring and summer burning into stands featuring low duff accumulation and longleaf pine at least 25 cm in diameter, would alleviate strain on local burning capacity, which often struggles to meet demand for dormant season burning. Moreover, burning outside the dormant season could benefit other restoration objectives such as promoting wire grass expansion and reducing hardwood encroachment (Outcalt, 1994; Addington et al., 2015). While climate change is expected to reduce the availability of summer burning days (Kupfer et al., 2020), we expect growing season burning will become an increasingly important practice for maintaining restoration efforts in southeastern woodlands.
In addition, our results may have important implications for other fire-prone ecosystems where prescribed fire is used to reduce hazardous fuels (Boer et al., 2009; Prichard et al., 2017). Our results do not directly link fire frequency with wildfire resistance; however, they do provide further evidence that damaging fire behavior is minimal in areas receiving frequent fire (Fernandes and Rigolot, 2007; Fernandes et al., 2015). Consequently, implementing a frequent prescribed fire regime would likely aid management efforts focused on mitigating wildfire risk.
The raw data supporting the conclusions of this article will be made available by the authors, without undue reservation.
JW developed the research questions, wrote the first draft of the manuscript, and performed the statistical analyses. AS and JK collected data and revised drafts of the manuscript. All authors contributed to the article and approved the submitted version.
Partial funding from the USDA NIFA McIntire Stennis project #1014653. This research was supported in part by the USDA Forest Service. The findings and conclusions in this publication are those of the author(s) and should not be construed to represent an official USDA, Forest Service, or United States Government determination or policy.
The authors declare that the research was conducted in the absence of any commercial or financial relationships that could be construed as a potential conflict of interest.
We would like to thank Justin McKeithen, Daniel Brethauer, and Sara Hunt for helping with field work in 2018. We would also like to thank Ronnie Tucker, George Ward, Erwin Chambliss, Corey Tucker, and Edward Andrews for helping to maintain the experiment over multiple decades.
Addington, R. N., Greene, T. A., Harrison, W. C., Sorrell, G. G., Elmore, M. L., and Hermann, S. M. (2015). Restoring longleaf pine: effects of seasonal prescribed fire and overstory density on vegetation structure of a young longleaf pine plantation. For. Sci. 61, 135–143. doi: 10.5849/forsci.13-618
Boer, M. M., Sadler, R. J., Wittkuhn, R. S., McCaw, L., and Grierson, P. F. (2009). Long-term impacts of prescribed burning on regional extent and incidence of wildfires - evidence from 50 years of active fire management in SW Australian forests. For. Ecol. Manage. 259, 132–142. doi: 10.1016/j.foreco.2009.10.005
Bottero, A., D’Amato, A. W., Palik, B. J., Kern, C. C., Bradford, J. B., and Scherer, S. S. (2017). Influence of repeated prescribed fire on tree growth and mortality in Pinus resinosa forests, northern Minnesota. For. Sci. 63, 94–100. doi: 10.5849/forsci.16-035
Boyer, W. D. (1987). Volume growth loss: a hidden cost of periodic prescribed burning in longleaf pine? South. J. Appl. For. 11, 154–157. doi: 10.1093/sjaf/11.3.154
Boyer, W. D. (1990). “Pinus palustris mill. (longleaf pine),” in Silvics of North America, Vol. Vol. 1, eds R. M. Burns and B. H. Honkala (Washington, WA: USDA), 405–412.
Buisson, E., Le Stradic, S., Silveira, F. A., Durigan, G., Overbeck, G. E., Fidelis, A., et al. (2019). Resilience and restoration of tropical and subtropical grasslands, savannas, and grassy woodlands. Biol. Rev. 94, 590–609. doi: 10.1111/brv.12470
Cronan, J. B., Wright, C. S., and Petrova, M. (2015). Effects of dormant and growing season burning on surface fuels and potential fire behavior in northern Florida longleaf pine (Pinus palustris) flatwoods. For. Ecol. Manage. 354, 318–333. doi: 10.1016/j.foreco.2015.05.018
Darracq, A. K., Boone, W. W., and McCleery, R. A. (2016). Burn regime matters: a review of the effects of prescribed fire on vertebrates in the longleaf pine ecosystem. For. Ecol. Manage. 378, 214–221. doi: 10.1016/j.foreco.2016.07.039
Dey, D. C., Kabrick, J. M., and Schweitzer, C. J. (2017). Silviculture to restore oak savannas and woodlands. J. For. 115, 202–211. doi: 10.5849/jof.15-152
Ferguson, J. P. (1998). “Prescribed fire on the Apalachicola Ranger District: the shift from dormant season to growing season and effects on wildfire suppression,” in Fire in Ecosystem Management: Shifting the Paradigm from Suppression to Prescription:Proceedings of the 20th Tall Timbers Fire Ecology Conference, eds T. L. Pruden and L. A. Brennan (Tallahassee, TLH: Tall Timbers Research Station), 120–126.
Fernandes, P. M., Fernandes, M. M., and Loureiro, C. (2015). Post-fire live residuals of maritime pine plantations in Portugal: structure, burn severity, and fire recurrence. For. Ecol. Manage. 347, 170–179. doi: 10.1016/j.foreco.2015.03.023
Fernandes, P. M., and Rigolot, E. (2007). The fire ecology and management of maritime pine (Pinus pinaster Ait.). For Ecol. Manage 241, 1–13. doi: 10.1016/j.foreco.2007.01.010
Ford, C. R., Minor, E. S., and Fox, G. A. (2010). Long-term effects of fire and fire-return interval on population structure and growth of longleaf pine (Pinus palustris). Can. J. For. Res. 40, 1410–1420. doi: 10.1139/x10-080
Garren, K. H. (1943). Effects of fire on vegetation of the southeastern United States. Bot. Rev. 9, 617–654. doi: 10.1007/bf02872506
Glitzenstein, J. S., Platt, W. J., and Streng, D. R. (1995). Effects of fire regime and habitat on tree dynamics in north Florida longleaf pine savannas. Ecol. Monogr. 65, 441–476. doi: 10.2307/2963498
Gordon, J. S., Willis, J. L., and Grala, R. K. (2020). Public and forest landowner attitudes towards longleaf pine ecosystem restoration using prescribed fire. Can. J. For. Res. 50, 917–924. doi: 10.1139/cjfr-2019-0415
Govender, N., Trollope, W. S., and Van Wilgen, B. W. (2006). The effect of fire season, fire frequency, rainfall and management on fire intensity in savanna vegetation in South Africa. J. Appl. Ecol. 43, 748–758. doi: 10.1111/j.1365-2664.2006.01184.x
Grelen, H. E. (1975). Vegetative Response to Twelve Years of Seasonal Burning on a Louisiana Longleaf Pine Site. Research Note SO-192. New Orleans, NO: USDA.
Guo, D. L., Mitchell, R. J., and Hendricks, J. J. (2004). Fine root branch orders respond differentially to carbon source-sink manipulations in a longleaf pine forest. Oecologia 140, 450–457. doi: 10.1007/s00442-004-1596-1
Hare, R. C. (1965). Contribution of bark to fire resistance of southern trees. J. Forest. 63, 248–251. doi: 10.1093/jof/63.4.248
Haywood, J. D., Harris, F. L., Grelen, H. E., and Pearson, H. A. (2001). Vegetative response to 37 years of seasonal burning on a Louisiana longleaf pine site. South. J. Appl. For. 25, 122–130. doi: 10.1093/sjaf/25.3.122
Hiers, J. K., Jackson, S. T., Hobbs, R. J., Bernhardt, E. S., and Valentine, L. E. (2016). The precision problem in conservation and restoration. Trends Ecol. Evol. 31, 820–830. doi: 10.1016/j.tree.2016.08.001
Hood, S. M., Varner, J. M., van Mantgem, P., and Cansler, C. A. (2018). Fire and tree death: understanding and improving modeling of fire-induced tree mortality. Environ. Res. Lett. 13:113004. doi: 10.1088/1748-9326/aae934
Hull-Sieg, C., McMillin, J. D., Fowler, J. F., Allen, K. K., Negron, J. F., Wadleigh, L. L., et al. (2006). Best predictors for postfire mortality of ponderosa pine trees in the Intermountain West. For. Sci. 52, 718–728. doi: 10.1093/forestscience/52.6.718
Hutchinson, T. F., Yaussy, D. A., Long, R. P., Rebbeck, J., and Sutherland, E. K. (2012). Long-term (13-year) effects of repeated prescribed fires on stand structure and tree regeneration in mixed-oak forests. For. Ecol. Manage. 286, 87–100. doi: 10.1016/j.foreco.2012.08.036
Jackson, J. F., Adams, D. C., and Jackson, U. B. (1999). Allometry of constitutive defense: a model and comparative test with tree bark and fire regime. Am. Nat. 153, 614–632. doi: 10.2307/2463619
Knapp, E. E., Estes, B. L., and Skinner, C. N. (2009). Ecological Effects of Prescribed Fire Season: A Literature Review and Synthesis for Managers. New York, NY: USDA.
Kupfer, J. A., Terando, A. J., Gao, P., Teske, C., and Hiers, J. K. (2020). Climate change projected to reduce prescribed burning opportunities in the south-eastern United States. Int. J. Wildland Fire. 29, 764–778. doi: 10.1071/wf19198
Kush, J. S., Meldahl, R. S., and Avery, C. (2004). A restoration success: longleaf pine seedlings established in a fire-suppressed, old-growth stand. Ecol. Res. 22, 6–10. doi: 10.3368/er.22.1.6
Kush, J. S., Meldahl, R. S., and Boyer, W. D. (1999). Understory plant community response after 23 years of hardwood control treatments in natural long leaf pine (Pinus palustris) forests. Can. J. Forest Res. 29, 1047–1054. doi: 10.1139/x99-063
Lashley, M. A., Chitwood, M. C., DePerno, C. S., and Moorman, C. E. (2017). Frequent fires eliminate fleshy fruit production. For. Ecol. Manage. 405, 9–12. doi: 10.1016/j.foreco.2017.09.034
Lashley, M. A., Chitwood, M. C., Harper, C. A., DePerno, C. S., and Moorman, C. E. (2015). Variability in fire prescriptions to promote wildlife foods in the longleaf pine ecosystem. Fire Ecol. 11, 62–79. doi: 10.4996/fireecology.1103062
Menges, E. S., and Deyrup, M. A. (2001). Postfire survival in south Florida slash pine: interacting effects of fire intensity, fire season, vegetation, burn size, and bark beetles. Int. J. Wildland Fire. 10, 53–63. doi: 10.1071/wf01009
Mitchell, R. J., Hiers, J. K., O’Brien, J. J., Jack, S. B., and Engstrom, R. T. (2006). Silviculture that sustains: the nexus between silviculture, frequent prescribed fire, and conservation of biodiversity in longleaf pine forests of the southeastern United States. Can. J. Forest Res. 36, 2724–2736. doi: 10.1139/x10-017
O’Brien, J. J., Hiers, J. K., Mitchell, R. J., Varner, J. M., and Mordecai, K. (2010). Acute physiological stress and mortality following fire in a long-unburned longleaf pine ecosystem. Fire Ecol. 6, 1–12. doi: 10.4996/fireecology.0602001
Ordóñez, J. L., Retana, J., and Espelta, J. M. (2005). Effects of tree size, crown damage, and tree location on post-fire survival and cone production of Pinus nigra trees. For. Ecol. Manage. 206, 109–117. doi: 10.1016/j.foreco.2004.10.067
Outcalt, K. W. (1994). Seed production of wiregrass in central Florida following growing-season prescribed burns. Int. J. Wildland Fire. 4, 123–125.
Outcalt, K. W. (2008). Lightning, fire and longleaf pine: using natural disturbance to guide management. For. Ecol. Manage. 255, 3351–3359. doi: 10.1016/j.foreco.2008.02.016
Peterson, D. L., Sackett, S. S., Robinson, L. J., and Haase, S. M. (1994). The effects of repeated prescribed burning on Pinus ponderosa growth. Int. J. Wildland Fire. 4, 239–247. doi: 10.1071/wf9940239
Peterson, D. W., and Reich, P. B. (2001). Prescribed fire in oak savanna: fire frequency effects on stand structure and dynamics. Ecol. Appl. 11, 914–927.
Prichard, S. J., Stevens-Rumann, C. S., and Hessburg, P. F. (2017). Tamm review: shifting global fire regimes: lessons from reburns and research needs. For. Ecol. Manage. 396, 217–233. doi: 10.1016/j.foreco.2017.03.035
Robbins, L. E., and Myers, R. L. (1992). Seasonal Effects of Prescribed Burning in Florida: A Review. Tallahassee, TLH: Timbers Research, Inc.
Robertson, K. M., and Hmielowski, T. L. (2014). Effects of fire frequency and season on resprouting of woody plants in southeastern US pine-grassland communities. Oecologia 174, 765–776. doi: 10.1007/s00442-013-2823-4
Ryan, K. C., Knapp, E. E., and Varner, J. M. (2013). Prescribed fire in North American forests and woodlands: history, current practice, and challenges. Front. Ecol. Evol. 11, e15–e24. doi: 10.1890/120329
Sayer, M. A. S., and Haywood, J. D. (2006). Fine root production and carbohydrate concentrations of mature longleaf pine (Pinus palustris Mill.) as affected by season of prescribed fire and drought. Trees 20:165. doi: 10.1007/s00468-005-0022-6
Sayer, M. A. S., Tyree, M. C., Kuehler, E. A., Jackson, J. K., and Dillaway, D. N. (2020). Physiological mechanisms of foliage recovery after spring or fall crown scorch in young longleaf pine (Pinus palustris Mill.). Forests 11:208. doi: 10.3390/f11020208
Schafer, J. L., Breslow, B. P., Hohmann, M. G., and Hoffmann, W. A. (2015). Relative bark thickness is correlated with tree species distributions along a fire frequency gradient. Fire Ecol. 11, 74–87. doi: 10.4996/fireecology.1101074
Shaw, J. D., and Long, J. N. (2007). A density management diagram for longleaf pine stands with application to red-cockaded woodpecker habitat. South. J. Appl. For. 31, 28–38. doi: 10.1093/sjaf/31.1.28
Slack, A. W., Zeibig-Kichas, N. E., Kane, J. M., and Varner, J. M. (2016). Contingent resistance in longleaf pine (Pinus palustris) growth and defense 10 years following smoldering fires. For. Ecol. Manage. 364, 130–138. doi: 10.1016/j.foreco.2016.01.014
Sparks, J. C., Masters, R. E., Engle, D. M., and Bukenhofer, G. A. (2002). Season of burn influences fire behavior and fuel consumption in restored shortleaf pine–grassland communities. Rest. Ecol. 10, 714–722. doi: 10.1046/j.1526-100x.2002.01052.x
Stanturf, J. A., Wade, D. D., Waldrop, T. A., Kennard, D. K., and Achtemeier, G. L. (2002). “Fire in southern forest landscapes,” in Southern Forest Resource Assessment. Gen. Tech. Rep. SRS-53, eds D. M. Wear and J. Greis (Asheville, AVL: USDA), 607–630.
Stephens, S. L., and Finney, M. A. (2002). Prescribed fire mortality of Sierra Nevada mixed conifer tree species: effects of crown damage and forest floor combustion. For. Ecol. Manage. 162, 261–271. doi: 10.1016/s0378-1127(01)00521-7
Van Lear, D. H., Carroll, W. D., Kapeluck, P. R., and Johnson, R. (2005). History and restoration of the longleaf pine-grassland ecosystem: implications for species at risk. For. Ecol. Manage. 211, 150–165. doi: 10.1016/j.foreco.2005.02.014
Varner, J. M., Gordon, D. R., Putz, F. E., and Hiers, J. K. (2005). Restoring fire to long−unburned Pinus palustris ecosystems: novel fire effects and consequences for long−unburned ecosystems. Rest. Ecol. 13, 536–544. doi: 10.1111/j.1526-100x.2005.00067.x
Keywords: Pinus palustris, woodland restoration pine, seasonal burning, prescribed fire, productivity
Citation: Willis JL, Sharma A and Kush JS (2021) Seasonality of Biennial Burning Has No Adverse Effects on Mature Longleaf Pine Survival or Productivity. Front. For. Glob. Change 4:684087. doi: 10.3389/ffgc.2021.684087
Received: 22 March 2021; Accepted: 16 June 2021;
Published: 14 July 2021.
Edited by:
Isabel Cañellas, Centro de Investigación Forestal (INIA), SpainReviewed by:
Daniel Moya, University of Castilla-La Mancha, SpainCopyright © 2021 Willis, Sharma and Kush. This is an open-access article distributed under the terms of the Creative Commons Attribution License (CC BY). The use, distribution or reproduction in other forums is permitted, provided the original author(s) and the copyright owner(s) are credited and that the original publication in this journal is cited, in accordance with accepted academic practice. No use, distribution or reproduction is permitted which does not comply with these terms.
*Correspondence: John L. Willis, am9obi53aWxsaXNAdXNkYS5nb3Y=
†These authors have contributed equally to this work and share last authorship
Disclaimer: All claims expressed in this article are solely those of the authors and do not necessarily represent those of their affiliated organizations, or those of the publisher, the editors and the reviewers. Any product that may be evaluated in this article or claim that may be made by its manufacturer is not guaranteed or endorsed by the publisher.
Research integrity at Frontiers
Learn more about the work of our research integrity team to safeguard the quality of each article we publish.