- Department of Environmental and Biological Sciences, University of Eastern Finland, Joensuu, Finland
Northern forest ecosystems are exposed to rapid climate change, i.e., climate warming, extended growing seasons, increasing greenhouse gases, and changes in precipitation and water availability, accompanied by increasing pressure of herbivores and pathogens. Silver birch (Betula pendula Roth) is an important deciduous trees species in the boreal zone, with extensive distribution across Eurasia. Silver birch is an excellent model system for the adaptation of northern trees to climate change due to recent advances in genomics, high genetic variation, and intensive studies with different abiotic and biotic stress factors. In this paper, the current understanding about the responses and acclimation mechanisms of birch to changing environment is presented, based on Fennoscandian studies. Several complementary experiments in laboratory, semi-field and natural field conditions have shown that warming climate and increasing CO2 is expected to increase the growth and biomass of birch, but the risk of herbivore damage will increase with negative impact on carbon sink strength. Deleterious impacts of high humidity, soil drought and increasing ozone has been clearly demonstrated. All these environmental changes have led to metabolic shifts or changes in carbon/nutrient balance which may have further ecological impacts. However, high plasticity and genotypic variation predict excellent acclimation capacity in rapidly changing environment and a rich genetic pool for sustainable forestry. Because the trees and forest ecosystems are exposed to multiple environmental factors simultaneously, it is necessary to continue research with multiple-stress interaction studies.
Introduction
White birches (Betula sp.) are among the key species in the northern ecosystems which are strongly affected by current climate warming, increased pests and pathogen damage, land-use changes and forest fragmentation (Gauthier et al., 2015; IPCC, 2019). These factors may reduce genetic diversity and make forests more fragile and sensitive to other threats. Betula pendula (silver birch) and B. pubescens (downy birch) are the most common broad-leaved tree species in Fennoscandia and found in most other parts of Europe (Kuuluvainen et al., 2017). B. pendula is economically important in plywood, fiber and furniture production particularly in Finland due to fast growth and good stem form (Verkasalo, 2002; Hynynen et al., 2010; Dubois et al., 2020). Besides the development of industrial potential in wider areas of Europe, the ecological and societal (aesthetic and recreational) value of B. pendula and B. pubescens has been recognized and evaluated (Dubois et al., 2020). Due to efficient seed propagation, birches are strong pioneer species in colonizing forest gaps in diverse climates and soils, enhancing biodiversity and soil functioning and improving the forest resilience e.g., against climate change, forest fires, and wind and herbivore damage (Mauer and Palatova, 2003; Kanerva and Smolander, 2007; Ascoli and Bovio, 2010; Rosenvald et al., 2011; Lehnert et al., 2013; Dubois et al., 2020). Besides many opportunities, high sensitivity to wood rot, crown competition and aero-allergenic properties of birches must not be ignored (Panula et al., 2009; Hynynen et al., 2010; Vanhellemont et al., 2016; Dubois et al., 2020).
Silver birch (B. pendula) is an excellent model system for the adaptation of northern trees to climate change due to recent advances in birch genomics (Salojärvi et al., 2017), intensive long-term studies e.g., for herbivory resistance, nitrogen economy, photosynthetic efficiency and phenology, and high genetic variation (Heimonen et al., 2016; Silfver et al., 2020; Tenkanen et al., 2020). In general, northern tree species of the boreal zone are adapted to cool climate with four seasons. Therefore, rapid climate warming is expected to strongly modify the function, phenology and development of these species. In addition to warming, changes in precipitation, drought and increasing greenhouse gases, and increasing herbivore and pathogen pressure are the most important factors affecting the boreal and subarctic forest trees and ecosystems (IPCC, 2019; Oksanen et al., 2019). The aim of this review is to present and discuss what we have learned about the impacts of these environmental factors on birch species growing in northern parts of Europe.
Responses to Warming
Heating Experiments
Artificial heating experiments with infra-red heaters have provided basic knowledge about the direct physiological and biochemical responses and mechanisms of plants to warming environment (Mäenpää et al., 2011; Silfver et al., 2020). Field experiments using infra-red heaters with silver birch saplings revealed that elevated temperature (ambient +1.2°C) increased whole-plant photosynthesis, leaf area, specific leaf area (SLA), biomass, stem height and delayed autumnal leaf abscission, while stomatal conductance was declined (Riikonen et al., 2009; Mäenpää et al., 2011). Gas exchange profiles along the vertical axis of the saplings showed that the leaves near the top of the plant were the most responsive to warming (Mäenpää et al., 2011). Improved defence capacity of birch due to warming was demonstrated by higher total antioxidant capacity and redox state of ascorbate (Riikonen et al., 2009). Elevation of night-time temperature in the growth chamber conditions increased the leaf level respiration and shifted the metabolic profiles, depending strongly on the genotypes (Mäenpää et al., 2013). The most clear responses were seen as increased concentrations of triperpenoids, hydrolysable tannins, certain phenolic compounds such as DHPPG (3,4′-dihydroxypropiophenone-3-ß-D-glucopyranoside) and fructose (Mäenpää et al., 2013). Metabolic shifts were also observed in VOC emissions, with the clearest increases in homoterpene DMNT and several sesquiterpenes (SQT) (Ibrahim et al., 2010). Higher emissions of certain volatile compounds were related to improved thermotolerance (Ibrahim et al., 2010).
Another open-air warming experiment using infra-red heaters in Subarctic area demonstrated the positive impacts of warming (2.3 and 1.2°C increases in air and soil temperature, respectively) on four native Betula species (silver birch, B. pendula; downy birch, B. pubescens, dwarf birch, B. nana; and mountain birch, B. pubescens subsp. Czerepanovii) (Silfver et al., 2020). Warming treatment increased the plant height growth, leaf chlorophyll concentration, CO2 uptake potential, and advanced the bud break. When the warming was combined with insecticide treatment reducing the pressure from insect feeding, the carbon sink strength of birches was even stronger, indicating the counteractive and negative impact of herbivores on carbon fluxes (Silfver et al., 2020). It is therefore expected that birch populations in the northern boreal and subarctic areas are vulnerable to climate warming and increasing herbivore pressure, as predicted earlier e.g., by Pöyry et al. (2011). Further, competition with the more southern genotypes can affect the genetic structure of the northern silver birch populations.
Translocation Experiments
A recent translocation experiment with 26 different silver birch genotypes simulating rapid climate warming across Finland has provided ecologically relevant information about the acclimation traits and capacity of birch as well as interactions with the other biotic and abiotic factors, such as herbivorous insect communities and nutrient availability (Heimonen et al., 2015a,b, 2016; Tenkanen et al., 2020, 2021). The results of herbivory studies show that climate warming can change the composition of herbivorous insect communities on birch, many herbivores can feed on novel host plant genotypes and the herbivory community composition is dependent on the physical size of plants and the phenological pattern of the genotypes (Heimonen et al., 2015a,b, 2016). Most severe herbivore damage was found in the small-size (height, leaf area) genotypes characterized by late bud burst and early growth cessation, and originating from high-latitude provenances (Heimonen et al., 2016).
Long-term field experiments have shown that Finnish silver birch populations can be divided into northern and southern groups according to several physiological traits, reflecting the evolutionary history of this species (Salojärvi et al., 2017; Tenkanen et al., 2020). Northern birch genotypes showed higher stomatal conductance (gs) rates but lower water-use efficiency (WUE) accompanied by more efficient N acquisition compared to southern genotypes (Ruhanen, 2018; Tenkanen et al., 2020). Clear differences in the chemical profiles of the leaves have been confirmed between the northern and southern groups (Deepak et al., 2018). Complementary laboratory studies in equal growth conditions revealed also different biomass accumulation strategies between the northern and southern birch origins (Tenkanen et al., 2021): while southern trees invest in canopy biomass and leaves, northern trees show more efficient photosynthetic capacity per leaf area (higher gas exchange, higher Fv/Fm) and relatively more investment in the below-ground fraction of the plant, but have a shorter total growth period. High root biomass is assumed to be an adaptation to better exploit and store the scarce nutrients and water resources in poor northern and arctic soils, safeguarding the leaf burst in the spring (Tenkanen et al., 2021). On the other hand, high soil N availability seems to increase the survival of silver birch plantlets, and growth and phenology may be adapted to soil N and day length rather than to temperature (Possen et al., 2014, 2021). Therefore, it is expected that the role of root compartment and root processes will be more important for birches in rapidly changing environment. Transfer of silver birch provenances in field experiments has, however, produced mixed results for fitness and survival and more research is needed for resistance traits. Viherä-Aarnio et al. (2013) indicated that long transfers (>2 latitudes) lead to a sharp decrease in survival and growth, while successful long-distance transfers of Finnish birches have been reported in North America and South Korea (Han et al., 1985; Rousi et al., 2012).
Responses to Water Stress
Soil Drought and Wetness
Impacts of soil drought on ozone-sensitive and ozone-tolerant silver birch genotypes have been studied in short-term and high-stress chamber experiment and long-term and mild-stress open-field experiment together with the ozone fumigation (Pääkkönen et al., 1998a,b). In both experiments, reduced leaf area, dry mass of leaves, stem and roots, gas exchange, transpiration rate, the number of stomata and starch was observed, accompanied by the higher contents or activity of Rubisco and chlorophyll, depending on the genotype (Pääkkönen et al., 1998a,b). Improved water-use-efficiency (WUE) developed in the open-field conditions (Pääkkönen et al., 1998a,b), while the induction of transcript levels for stress-protein PR-10 occurred in the chamber study (Pääkkönen et al., 1998c). Drought treatment mitigated the negative ozone effects in the acute chamber study, whereas negative interaction on the two stresses was detected in the open-field study (Pääkkönen et al., 1998a,b). Accordingly, high plasticity and a substantial variation in physiological and morphological responses, particularly in stomatal conductance, net photosynthesis, WUE, leaf carbon isotope composition, leaf osmotic potentials, leaf area indicators, root-to-shoot ratio and fine root growth have been detected among the silver birch clones exposed to drought in a common garden experiment by Aspelmeier and Leuschner (2004, 2006).
A semi-field experiment with three silver birch genotypes exposed to three soil moisture conditions (wet-control-dry) showed that drought stress reduced the growth, biomass, shoot-to-root ratio and gas exchange, while the wet treatment has opposite effects (Possen et al., 2011). Although there was a clear variation between the genotypes, the maintenance of the foliar processes as increasing number of leaves and high SLA during the stress treatments was observed for all genotypes (Possen et al., 2011). Silver birch seedlings have been also investigated for the impacts of waterlogging and soil freezing throughout the year, showing better tolerance against waterlogging during the winter dormancy compared to growing season (Repo et al., 2021). However, without the proper insulating snow cover, the downy birch seedlings suffered from the impaired growth and carbohydrate metabolism during the next following season (Domisch et al., 2019).
High Humidity
Responses of silver birch under long-term exposure to high humidity (low VPD) have been studied with the Free Air Humidity Manipulation (FAHM) facility in Estonia (Lihavainen et al., 2016b; Sellin et al., 2016; Oksanen et al., 2019). The results show that atmospheric humidity is an important climatic factor for birch trees affecting metabolism, physiology, water and nutrient cycling and balance, structure and development of leaf, roots and stem and growth properties (Sellin et al., 2016; Oksanen et al., 2019). At the whole-tree level, high humidity reduced the above-ground growth, total leaf area, leaf biomass, sap flux, slenderness, bud size, LAI (leaf area index) and soil respiration, delayed leaf fall and enhanced spring-term bud break, while increases in root biomass was detected concomitant with the higher number of root tips, increased fine root biomass, hydraulic conductance and hydrophilic ectomycorrhiza (Sellin et al., 2016; Oksanen et al., 2019). At the leaf level, high humidity increased starch, antioxidants (ascorbate metabolites, tocopherols), phenolic compounds and SLA, while glandular trichome density, N and P contents, leaf size, dark respiration, photosynthetic capacity and hydraulic conductance were declined (Lihavainen et al., 2016b; Sellin et al., 2016; Oksanen et al., 2019). In the stem, N and P contents and number of living parenchyma cells were increased, while wood density was reduced and wood chemistry altered (Sellin et al., 2016).
Short-term complementary laboratory experiments with high humidity (low VPD) provided more detailed information about metabolic responses and nutrient cycling of silver birch (Lihavainen et al., 2016a). In addition to starch, high humidity increased the foliar concentrations of quercetin glycosides, raffinose and glutamate, whereas the levels of amino acids alanine and valine, several citric acid cycle intermediates, glutamine-to-glutamate ratio, shikimic acid, ribonic acid, nutrient concentrations (N in particular) and glandular trichome density were reduced (Lihavainen et al., 2016a). This metabolic adjustment and limited nutrient availability shifted the balance towards carbohydrates and phenolics. Leaf surface wax composition shifted towards less hydrophobic waxes, with lower alkane and hydrophobic flavonoid contents, but the responses were mitigated by high N availability (Lihavainen et al., 2017). The two different experimental approaches revealed also some differences in plant responses between the field and laboratory studies (Lihavainen et al., 2016a). For instance, the transient increase in biomass, height, leaf number, whole-plant leaf area, stem diameter and root growth was observed in the laboratory, along with the higher stomatal conductance rates, while antioxidants were unaffected (Lihavainen et al., 2016a; Oksanen et al., 2019). Increasing leaf wettability was considered predisposing the leaves to pathogen and pest damage (Lihavainen et al., 2017).
Responses to Greenhouse Gases
Increasing CO2
Impacts of increasing CO2 level has been studied in young in silver birch trees growing in open-top-chambers and in paper birch (Betula papyrifera) growing in the Aspen FACE site (Riikonen et al., 2004, 2005; Kontunen-Soppela et al., 2010a,b). High CO2 (2 × ambient) increased biomass, growth and leaf area of the trees and lengthened the duration of the green leaves at the end of the season (Riikonen et al., 2004). Responses of net photosynthesis rates varied from considerable increase measured at high-CO2 environment to decline measured at the ambient-CO2 level, accompanied by reduced leaf N content, lower activity and amount of Rubisco and accumulation of starch (Riikonen et al., 2005). Genetic studies have supported these physiological and biochemical results and revealed the CO2 caused down-regulation of the expression of several genes relating to the light reactions of the photosynthesis (Oksanen et al., 2005; Kontunen-Soppela et al., 2010a,b). Shift in secondary compound profiles due to high CO2 was observed as increased phenolic acid and decreased flavonone aglycon and DHPPG contents (Peltonen et al., 2005). At the leaf structural level, increased size of starch grains and chloroplasts and higher number of mitochondria was observed at high CO2 treatment, while SLA was reduced (Oksanen et al., 2005). Further, leaf chemistry was altered, reflecting lower nutrient contents, and cell wall composition was affected, containing a higher proportion of hemicellulose but lower proportion of a-cellulose, uronic acids, acid-soluble lignin and acetone-soluble extractives (Oksanen et al., 2005). As a whole, high CO2 was considered strengthening the oxidative stress tolerance of silver birch trees through increased carbon pool for phenolic compounds, detoxification and cell wall structural modifications, but also exposing the trees to nutrient imbalance (Oksanen et al., 2005; Riikonen et al., 2005; Kontunen-Soppela et al., 2010a,b).
Increasing Ozone
Responses of silver birch to increasing tropospheric ozone, alone and in combination with other environmental factors such as CO2, warming, soil nitrogen and drought, have been extensively investigated (e.g., Oksanen et al., 2007 and references therein). Harmful impacts of ozone has been reported as impaired growth and photosynthesis, declined Rubisco and chlorophyll content, altered shoot-to-root ratio at the expense of roots, visible leaf injuries, H2O2 accumulation (indicating oxidative stress) and related ultrastructural injuries particularly in the chloroplasts, altered cell wall chemistry, and disturbed nutrient cycling and phenology through slower bud break and earlier leaf senescence (Oksanen et al., 2005, 2007; Oksanen and Kontunen-Soppela, 2021). These changes have been accompanied by shifted metabolic profiles towards phenolic compounds and increased antioxidative capacity through ascorbate metabolism (Yamaji et al., 2003; Kontunen-Soppela et al., 2007; Riikonen et al., 2009). Genetic variation in ozone responses is large, allowing the identification of ozone sensitive and ozone tolerant genotypes (Oksanen et al., 2007). Similar ozone responses have been reported also for paper birch growing in the Aspen FACE site in the temperate zone of the United States (Oksanen et al., 2003; Kontunen-Soppela et al., 2010b).
Discussion
The present understanding about the impacts of warming, high air humidity, soil drought and wetness, increasing CO2 and ozone on birch is shown in Figure 1, based on Fennoscandian studies. Warming climate is expected to increase the growth and biomass of birch, but the risk of herbivore damage will increase with negative impact on carbon sink strength (Mäenpää et al., 2011; Heimonen et al., 2015a,b, 2016; Silfver et al., 2020; Figure 1). Deleterious impacts of high humidity, soil drought and increasing ozone on birch has been clearly demonstrated (Pääkkönen et al., 1998a,b; Oksanen et al., 2007; Possen et al., 2011; Lihavainen et al., 2016b, 2017; Figure 1), as well as the positive impact of increasing CO2 on growth (Riikonen et al., 2004). All these environmental changes have led to metabolic shifts which have been regarded as stress protection mechanisms, or changes in carbon/nutrient balance which may have further ecological impacts (Peltonen et al., 2005; Kontunen-Soppela et al., 2007; Mäenpää et al., 2013; Lihavainen et al., 2016a). Practically all experiments described above have shown high plasticity and genotypic variation in silver birch, predicting excellent acclimation capacity in rapidly changing environment and a rich genetic pool for sustainable forestry (Possen et al., 2015, 2021; Deepak et al., 2018; Tenkanen et al., 2020, 2021). High net assimilation rate, investment in leaf mass and high shoot-to-root ratio could be related to the fast growth rate of young birch trees (Possen et al., 2015), while high soil fertility (N content in particular) promoted the survival of birch in rapidly changing conditions (Possen et al., 2021).
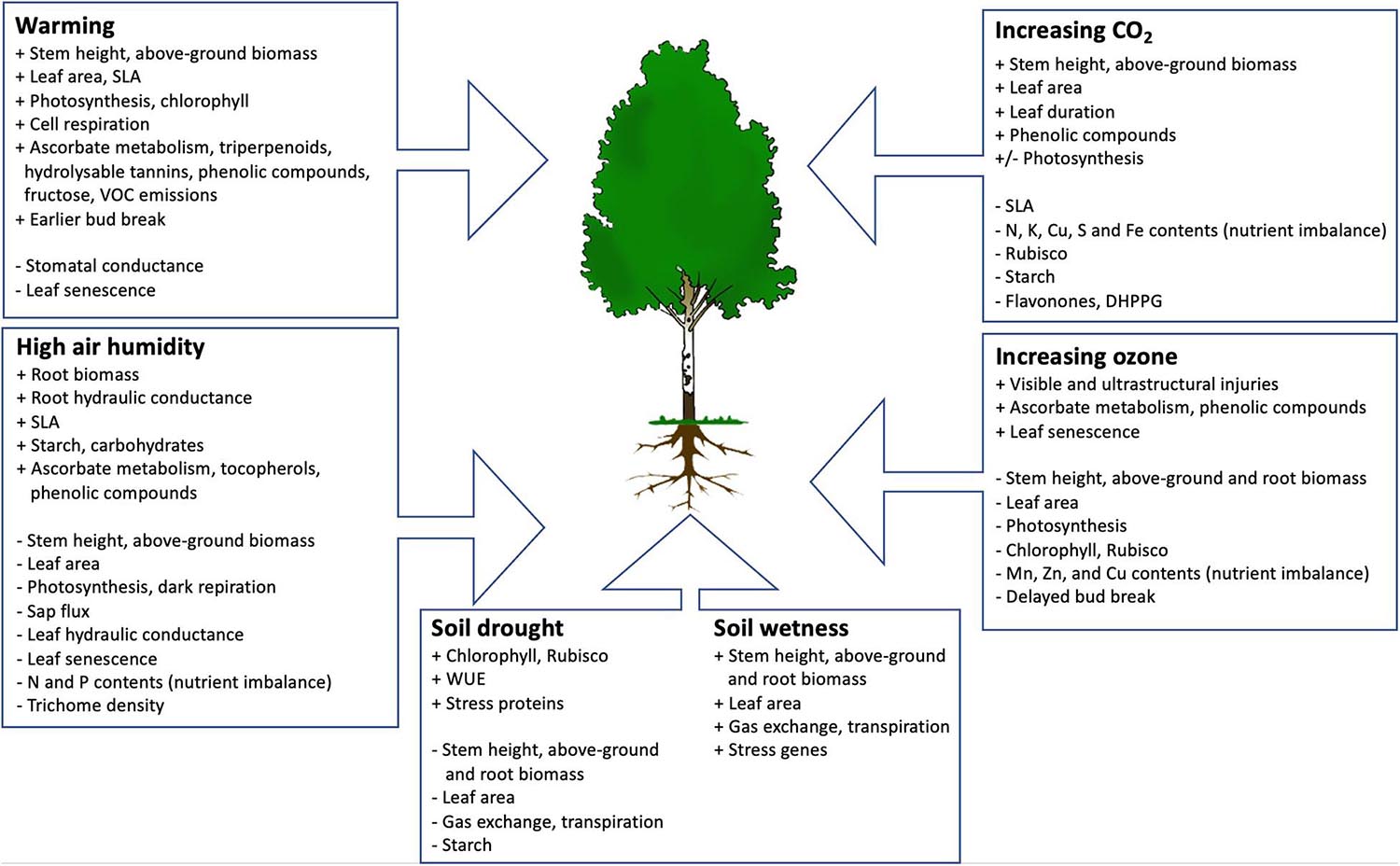
Figure 1. The impacts of warming, high air humidity, soil drought and wetness, increasing CO2 and ozone on birch. Positive responses are indicated with (+), negative responses with (−) and variable (both positive and negative) with (±). ‘Above-ground biomass’ includes stem and leaf biomass.
An increasing body of literature underlines the value of birch as a model system for understanding how northern tree respond to climate change. To date, most studies on European birch species explore tree responses to changes in a single environmental variable. Yet, in the face of climate change trees and forest ecosystems will be exposed to multiple environmental factors simultaneously. However, the effects of multiple, interacting environmental factors on tree traits cannot be reliably assessed based on single factor analyses (Niinemets, 2010). Therefore, it is important to increasingly explore the multiple interactions, as done e.g., by Pääkkönen et al. (1998a, 1998b), Oksanen et al. (2005); Riikonen et al. (2005), and Kontunen-Soppela et al. (2010a, b) finding that ozone injuries were mitigated when birch trees were jointly exposed to drought, elevated CO2, and increased nitrogen availability. More research is needed particularly for increasing CO2 × water stress (drought, wetness, humidity) interactions, and for below-ground processes.
Finally, this review implies that birch with its rapid growth rate combined with a relatively high herbivore resistance, its ability to adapt to climatic changes and its recolonization traits possesses large potential in future silviculture. This is particularly true for mixed tree stands and reforestation areas. Therefore, birch deserves more attention in European tree breeding programms and silvicultural strategies.
Data Availability Statement
The datasets presented in this study can be found in online repositories. The names of the repository/repositories and accession number(s) can be found in the article/supplementary material.
Author Contributions
EO wrote all parts of the manuscript and made the Figure.
Funding
The work was funded by the University of Eastern Finland and The Finnish Cultural Foundation (project 00210798).
Conflict of Interest
The author declares that the research was conducted in the absence of any commercial or financial relationships that could be construed as a potential conflict of interest.
References
Ascoli, D., and Bovio, G. (2010). Tree encroachment dynamics in heathlands of Northwest Italy: the fire regime hypothesis. iForest 3, 137–143. doi: 10.3832/ifor0548-003
Aspelmeier, S., and Leuschner, C. (2004). Genotypic variation in drought response of silver birch (Betula pendula): Leaf water status and carbon gain. Tree Physiol. 24, 517–528. doi: 10.1093/treephys/24.5.517
Aspelmeier, S., and Leuschner, C. (2006). Genotypic variation in drought response of silver birch (Betula pendula Roth): leaf and root morphology and carbon partitioning. Trees 20, 42–52. doi: 10.1007/s00468-005-0011-9
Deepak, M., Lihavainen, J., Keski-Saari, S., Kontunen-Soppela, S., Tenkanen, A., Oksanen, E., et al. (2018). Genotypic variation and provenance-related clinal patterns in leaf surface secondary compounds of silver birch. J. Can. For. Res. 48, 494–505. doi: 10.1139/cjfr-2017-0456
Domisch, T., Martz, F., Repo, T., and Rautio, P. (2019). Let it snow! winter conditions affect growth of birch seedlings during the following growing season. Tree Phys. 39, 544–555. doi: 10.1093/treephys/tpy128
Dubois, H., Verkasalo, E., and Claessens, H. (2020). Potential of birch (Betula pendula Roth and B. pubescens Ehrh.) for forestry and forest-based industry sector within the changing climatic and socio-economic context of Western Europe. Forests 11:336. doi: 10.3390/f11030336
Gauthier, S., Bernier, P., Kuuluvainen, T., Shvidenko, A. Z., and Schepaschenko, D. G. (2015). Boreal forest health and global change. Science 349, 819-822. doi: 10.1126/science.aaa9092
Han, Y., Lee, Y., Ryu, K., and Park, M. (1985). Growth of European white birch (Betula pendula Roth) introduced from Finland at age 11 in Korea (No. Research Report 21). Suwon: Institute of Forest Genetics.
Heimonen, K., Valtonen, A., Kontunen-Soppela, S., Keski-Saari, S., Rousi, M., Oksanen, E., et al. (2015a). Colonization of a host tree by herbivorous insects under a changing climate. Oikos 124, 1013–1022. doi: 10.1111/oik.01986
Heimonen, K., Valtonen, A., Kontunen-Soppela, S., Keski-Saari, S., Rousi, M., Oksanen, E., et al. (2015b). Insect herbivore damage on latitudinally translocated silver birch (Betula pendula) – predicting the effects of climate change. Clim. Change 131, 245–257. doi: 10.1007/s10584-015-1392-4
Heimonen, K., Valtonen, A., Kontunen-Soppela, S., Keski-Saari, S., Rousi, M., Oksanen, E., et al. (2016). Susceptibility of silver birch (Betula pendula) to herbivorous insects is associated with size and phenology of birch– implications for climate warming. Scan. J. For. Res. 32, 95–104. doi: 10.1080/02827581.2016.1195867
Hynynen, J., Niemistö, P., Viherä-Aarnio, A., Brunner, A., Hein, S., and Velling, P. (2010). Silviculture of birch (Betula pendula Roth and Betula pubescens Ehrh.) in northern Europe. Forestry 83, 103–119. doi: 10.1093/forestry/cpp035
Ibrahim, M. A., Mäenpää, M., Hassinen, V., Kontunen-Soppela, S., Malec, L., Rousi, M., et al. (2010). Elevation of night-time temperature increases terpenoid emissions from Betula pendula and Populus tremula. J. Exp. Bot. 61, 1583–1595. doi: 10.1093/jxb/erq034
IPCC (2019). Climate Change and Land, Report 2019. Available online at: https://www.ipcc.ch/site/assets/uploads/2019/08/2c.-Chapter-2_FINAL.pdf (accessed February 15, 2021).
Kanerva, S., and Smolander, A. (2007). Microbial activities in forest floor layers under silver birch, Norway spruce and Scots pine. Soil Biol. Biochem. 39, 1459–1467. doi: 10.1016/j.soilbio.2007.01.002
Kontunen-Soppela, S., Ossipov, V., Ossipova, S., and Oksanen, E. (2007). Shift in birch leaf metabolome and carbon allocation during long-term open-field ozone exposure. Glob. Change Biol. 13, 1053–1067. doi: 10.1111/j.1365-2486.2007.01332.x
Kontunen-Soppela, S., Riikonen, J., Ruhanen, H., Brosché, M., Peltonen, P., Kangasjärvi, J., et al. (2010a). Differential gene expression in senescing leaves of two silver birch genotypes in response to elevated CO2 and tropospheric ozone. Plant Cell Environ. 33, 1016–1028. doi: 10.1111/j.1365-3040.2010.02123.x
Kontunen-Soppela, S., Parviainen, J., Brosché, M., Keinänen, M., Kolehmainen, M., Kangasjärvi, J., et al. (2010b). Gene expression responses of paper birch (Betula papyrifera) to elevated CO2 and O3 during leaf maturation and senescence. Environ. Poll. 158, 959–968. doi: 10.1016/j.envpol.2009.10.008
Kuuluvainen, T., Hofgaard, A., Aakala, T., and Jonsson, B. G. (2017). North Fennoscandian mountain forests: history, composition, disturbance dynamics and the unpredictable future. For. Ecol. Manag. 385, 140–149. doi: 10.1016/j.foreco.2016.11.031
Lehnert, L. W., Bassler, C., Brandl, R., Burton, P. J., and Muller, J. (2013). Conservation value of forests attacked by bark beetles: Highest number of indicator species is found in early successional stages. J. Nat. Conserv. 21, 97–104. doi: 10.1016/j.jnc.2012.11.003
Lihavainen, J., Ahonen, V., Keski-Saari, S., Kontunen-Soppela, S., Sober, A., Oksanen, E., et al. (2016a). Low vapour pressure affects nitrogen nutrition and foliar metabolites of silver birch. J. Exp. Bot. 67, 4353–4365. doi: 10.1093/jxb/erw218
Lihavainen, J., Ahonen, V., Keski-Saari, S., Sober, A., Oksanen, E., and Keinänen, M. (2017). Low vapor pressure deficit reduces glandular trichome density and modifies the chemical composition of cuticular waxes in silver birch leaves. Tree Phys. 28, 1–16. doi: 10.1093/treephys/tpx045
Lihavainen, J., Keinänen, M., Keski-Saari, S., Kontunen-Soppela, S., Sober, A., and Oksanen, E. (2016b). Artificially decreased VPD in field conditions modifies foliar metabolite profiles of birch and aspen. J. Exp. Bot. 67, 4367–4378. doi: 10.1093/jxb/erw219
Mäenpää, M., Ossipov, V., Kontunen-Soppela, S., Keinänen, M., Rousi, M., and Oksanen, E. (2013). Biochemical and growth acclimation of birch to night-time temperatures: genotypic similarities and differences. Plant Biol. 15, 36–43. doi: 10.1111/j.1438-8677.2012.00609.x
Mäenpää, M., Riikonen, J., Kontunen-Soppela, S., Rousi, M., and Oksanen, E. (2011). Vertical profiles reveal impact of ozone and temperature on carbon assimilation of Betula pendula and Populus tremula. Tree Phys. 31, 819–830. doi: 10.1093/treephys/tpr075
Mauer, O., and Palatova, E. (2003). The role of root system in silver birch (Betula pendula Roth) dieback in the air-polluted area of Krušné hory Mts. J. For. Sci. 49, 191–199. doi: 10.17221/4693-JFS
Niinemets, Ü (2010). Responses of forest trees to single and multiple environmental stresses from seedlings to mature plants: Past stress history, stress interactions, tolerance and acclimation. For. Ecol. Manag. 260, 1623–1639. doi: 10.1016/j.foreco.2010.07.054
Oksanen, E., Häikiö, E., Sober, J., and Karnosky, D. (2003). Ozone-induced H2O2 accumulation in field-grown aspen and birch is linked to foliar ultrastructure and peroxisomal activity. New Phytol. 161, 791–800. doi: 10.1111/j.1469-8137.2003.00981.x
Oksanen, E., and Kontunen-Soppela, S. (2021). Plants have different strategies to defend against air pollutants. Curr. Opin. Env. Sci. Health 19:100222. doi: 10.1016/j.coesh.2020.10.010
Oksanen, E., Kontunen-Soppela, S., Riikonen, J., Uddling, J., and Vapaavuori, E. (2007). Northern environment predisposes birches to ozone damage. Plant Biol. 9, 191–196. doi: 10.1055/s-2006-924176
Oksanen, E., Lihavainen, J., Keinänen, M., Kontunen-Soppela, S., Keski-Saari, S., Sellin, A., et al. (2019). “Northern forest trees under increasing atmospheric humidity,” in Progress in Botany ISBN 978-3-030-10760-4, Vol. 80, eds U. Lüttge, F. M. Canovas, R. Matyssek, and H. Pretzsch (Cham: Springer Nature), 317–336. doi: 10.1007/124_2017_15
Oksanen, E., Riikonen, J., Kaakinen, S., Holopainen, T., and Vapaavuori, E. (2005). Structural characteristics and chemical composition of birch (Betula pendula) leaves are modified by increasing and CO2 and ozone. Glob. Change Biol. 11, 732–748. doi: 10.1111/j.1365-2486.2005.00938.x
Pääkkönen, E., Vahala, J., Holopainen, T., and Kärenlampi, L. (1998a). Physiological and ultrastructural responses of birch clones exposed to ozone and drought stress. Chemosphere 36, 679–684. doi: 10.1016/S0045-6535(97)10107-2
Pääkkönen, E., Vahala, J., Pohjola, M., Holopainen, T., and Kärenlampi, L. (1998b). Physiological, stomatal and ultrastructural ozone responses in birch (Betula pendula Roth) are modified by water stress. Plant Cell Env. 21, 671–684. doi: 10.1046/j.1365-3040.1998.00303.x
Pääkkönen, E., Seppänen, S., Holopainen, T., Kärenlampi, S., Kärenlampi, L., and Kangasjärvi, J. (1998c). Induction of genes for the stress proteins PR-10 and PAL in relation to growth, visible injuries and stomatal responses in ozone and drought exposed birch (Betula pendula) clones. New Phytol. 138, 295–305. doi: 10.1046/j.1469-8137.1998.00898.x
Panula, E. Y., Fekedulegn, D. B., Green, B. J., and Ranta, H. (2009). Analysis of airborne Betula pollen in Finland; a 31-year perspective. Int. J. Environ. Res. Public Health 6, 1706–1723. doi: 10.3390/ijerph6061706
Peltonen, P. A., Vapaavuori, E., and Julkunen-Tiitto, R. (2005). Accumulation of phenolic compounds in birch leaves is changed by elevated carbon dioxide and ozone. Glob. Change Biol. 11, 1305–1324. doi: 10.1111/j.1365-2486.2005.00979.x
Possen, B. J. H. M., Heinonen, J., Anttonen, M., Rousi, M., Kontunen-Soppela, S., Oksanen, E., et al. (2015). Trait syndromes underlying stand-level differences in growth and acclimation in 10 silver birch (Betula pendula Roth) genotypes. For. Ecol. Manag. 343, 123–135. doi: 10.1016/j.foreco.2015.02.004
Possen, B. J. H. M., Oksanen, E., Rousi, M., Ruhanen, H., Ahonen, V., Tervahauta, A., et al. (2011). Adaptability of birch (Betula pendula Roth) and aspen (Populus tremula L.) genotypes to different soil moisture conditions. For. Ecol. Manag. 262, 1387–1399. doi: 10.1016/j.foreco.2011.06.035
Possen, B. J. H. M., Rousi, M., Keski-Saari, S., Kontunen-Soppela, S., Oksanen, E., and Mikola, J. (2021). New evidence for the importance of soil nitrogen on the survival and adaptation of birch to climate warming. Ecosphere
Possen, B. J. H. M., Rousi, M., Silfver, T., Anttonen, M., Ruotsalainen, S., Oksanen, E., et al. (2014). Within-stand variation in silver birch (Betula pendula Roth) phenology. Trees 28, 1801–1812. doi: 10.1007/s00468-014-1087-x
Pöyry, J., Leinonen, R., Söderman, G., Nieminen, M., Heikkinen, R. K., and Carter, T. R. (2011). Climate-induced increase of moth multivoltinism in boreal regions. Glob. Ecol. Biogeogr. 20, 289–298. doi: 10.1111/j.1466-8238.2010.00597.x
Repo, T., Domisch, T., Roitto, M., Kilpeläinen, J., Wang, A.-F., Piirainen, S., et al. (2021). Dynamics of above- and belowground responses of silver birch saplings and soil gases to soil freezing and waterlogging during dormancy. Tree Phys. tab002. doi: 10.1093/treephys/tpab002
Riikonen, J., Holopainen, T., Oksanen, E., and Vapaavuori, E. (2005). Leaf photosynthetic characteristics of silver birch during three years of exposure to elevated CO2 and O3 in the field. Tree Phys. 25, 621–632. doi: 10.1093/treephys/25.5.621
Riikonen, J., Lindsberg, M.-M., Holopainen, T., Oksanen, E., Peltonen, P., Lappi, J., et al. (2004). Silver birch and climate change: Variable growth and carbon allocation responses to elevated concentrations of carbon dioxide and ozone. Tree Phys. 24, 1227–1237. doi: 10.1093/treephys/24.11.1227
Riikonen, J., Mäenpää, M., Alavillamo, M., Silfver, T., and Oksanen, E. (2009). Interactive effects of elevated temperature and O3 on antioxidant capacity and gas exchange in Betula pendula saplings. Planta 230, 419–427. doi: 10.1007/s00425-009-0957-8
Rosenvald, K., Ostonen, I., Truu, M., Truu, J., Uri, V., Vares, A., et al. (2011). Fine-root rhizosphere and morphological adaptations to site conditions in interaction with tree mineral nutrition in young silver birch (Betula pendula Roth.) stands. Eur. J. For. Res. 130, 1055–1066. doi: 10.1007/s10342-011-0492-6
Rousi, M., Possen, B., Hagqvist, R., and Thomas, B. (2012). From the Arctic circle to the Canadian prairies - a case study of silver birch acclimation capacity. Silva Fenn. 46, 355–364. doi: 10.14214/sf.46
Ruhanen, H. (2018). Effects of Soil, Birch Origin and Genotype on Growth and Physiology of Silver Birch and Downy birch. Ph.D. Thesis. Joensuu: University of Eastern Finland.
Salojärvi, J., Smolander, O.-P., Nieminen, K., Rajaraman, S., Safronov, O., Safdari, P., et al. (2017). Genome sequencing and population genomic analyses provide insights into the adaptive landscape of silver birch. Nat. Gen. 49, 904–912. doi: 10.1038/ng.3862
Sellin, A., Alber, M., Keinänen, M., Kupper, P., Lihavainen, J., Lõhmus, K., et al. (2016). Growth of northern deciduous trees under increasing atmospheric humidity: possible mechanisms behind the growth retardation. Reg. Env. Change 17, 2135–2148. doi: 10.1007/s10113-016-1042-z
Silfver, T., Heiskanen, L., Aurela, M., Myller, K., Karhu, K., Meyer, N., et al. (2020). Insect herbivory control of Subarctic ecosystem CO2 exchange in present and future climates. Nature Commun. 11:2529. doi: 10.1038/s41467-020-16404-4
Tenkanen, A., Keski-Saari, S., Salojärvi, J., Oksanen, E., Keinänen, M., and Kontunen-Soppela, S. (2020). Differences in growth and gas exchange between southern and northern provenances of silver birch (Betula pendula) in northern Europe. Tree Phys. 40, 198–214. doi: 10.1093/treephys/tpz124
Tenkanen, A., Suprun, S., Oksanen, E., Keinänen, M., Keski-Saari, S., and Kontunen-Soppela, S. (2021). Strategy by latitude? Higher photosynthetic capacity and root mass fraction in northern than southern silver birch (Betula pendula Roth) in uniform growing conditions. Tree Phys.tpaa148. doi: 10.1093/treephys/tpaa148
Vanhellemont, M., Van Acker, J., and Verheyen, K. (2016). Exploring life growth patterns in birch (Betula pendula). Scand. J. For. Res. 31:7. doi: 10.1080/02827581.2016.1141978
Verkasalo, E. (2002). “Properties of domestic birch, aspen and alder and their utilisation in mechanical wood processing,” in Proceedings of the Finnish Forest Cluster Research Programme WOOD WISDOM (1998-2001). Final report, Helsinki, 215–229.
Viherä-Aarnio, A., Kostiainen, K., Piispanen, R., Saranpää, P., and Vapaavuori, E. (2013). Effects of seed transfers on yield and stem defects of silver birch (Betula pendula Roth). For. Ecol. Manag. 289, 133–142. doi: 10.1016/j.foreco.2012.10.030
Keywords: birch, warming, air humidity, water stress, CO2, ozone
Citation: Oksanen E (2021) Birch as a Model Species for the Acclimation and Adaptation of Northern Forest Ecosystem to Changing Environment. Front. For. Glob. Change 4:682512. doi: 10.3389/ffgc.2021.682512
Received: 18 March 2021; Accepted: 16 April 2021;
Published: 10 May 2021.
Edited by:
Andrea Ghirardo, Helmholtz Zentrum München, Helmholtz-Gemeinschaft Deutscher Forschungszentren (HZ), GermanyReviewed by:
Hilke Schroeder, Thünen Institute of Forest Genetics, GermanyMichael Eisenring, Swiss Federal Institute for Forest, Snow and Landscape Research (WSL), Switzerland
Copyright © 2021 Oksanen. This is an open-access article distributed under the terms of the Creative Commons Attribution License (CC BY). The use, distribution or reproduction in other forums is permitted, provided the original author(s) and the copyright owner(s) are credited and that the original publication in this journal is cited, in accordance with accepted academic practice. No use, distribution or reproduction is permitted which does not comply with these terms.
*Correspondence: Elina Oksanen, ZWxpbmEub2tzYW5lbkB1ZWYuZmk=