- 1Laboratory of Ecosystem Management, Graduate School of Agriculture, Hokkaido University, Sapporo, Japan
- 2Alaska Department of Natural Resources, Division of Forestry, Fairbanks, AK, United States
Questions have been raised about the application of conventional post-windthrow forest practices such as salvage logging, site preparation, and afforestation in response to the increase in wind disturbance caused by climate change. In particular, it is necessary to identify effective forest management practices that consider the pressure from deer browsing in forests in cold, snowy regions because the population of ungulates is expected to increase. The impacts of legacy destruction, i.e., the destruction of advance regeneration, microsites, and soil structure, caused by conventional post-windthrow practices have rarely been assessed separately from the impacts of subsequent deer browsing on forest regeneration or evaluated based on sufficiently long monitoring periods to assess vegetation succession. This lack of studies is one reason that alternative forest management practices to salvaging and planting have not been proposed. We conducted a field experiment at a large-scale windthrow site with a deer population to (1) assess the impact of legacy destruction and deer browsing on vegetation biomass and species composition after 15 years and (2) identify the effects of legacy retention. The study design allowed us to distinguish between and measure the impact of legacy destruction and that of subsequent deer browsing during a 15-year period. The results revealed the following: (1) Salvage logging and site preparation suppressed the development of biomass of shrub and tree layers in forested areas where harvest residues were piled up and shifted the plant communities in these areas to herbaceous plant communities. (2) Subsequent deer browsing suppressed the development of the biomass of shrub and tree layers throughout the forested site and shifted herbaceous communities to ruderal communities dominated by alien species; and 3. Compared with salvaging and planting, legacy retention enabled the windthrow sites to more quickly develop into a stand with characteristics similar to that of a mature, natural forest. Forest management practices that consider the presence of deer are necessary. We propose a policy shift from planting trees after salvaging to leaving downed trees to regenerate natural forests, unless there is concern about insect damage to the remaining forestry land in the vicinity.
Introduction
The frequency of intense tropical cyclones at the end of the twenty-first century (2075–99) was projected globally in the Intergovernmental Panel on Climate Change (IPCC) A1B scenario (Murakami et al., 2012). This scenario projects increasing amounts of severe damage to forests due to typhoons in East Asian countries with monsoon climates. It will be difficult to continue to implement conventional practices applied after windthrow, such as salvage logging, site preparation, and reforestation, in every stand because of limitations on available resources for forest management if the area of such disturbances increases (Thorn et al., 2018).
Recent research indicates that conventional post-windthrow practices may impede forest recovery (Thorn et al., 2018; Taeroe et al., 2019). First, salvage logging and site preparation for planting destroy windthrow legacies, including advance regeneration, various types of microsite, and soil structure (Tan et al., 2005; Peterson and Leach, 2008; Morimoto et al., 2011). Moreover, removing fallen logs facilitates deer foraging on planted and naturally regenerating saplings (Jonášová et al., 2010; Moriya et al., 2012; Götmark and Kiffer, 2014) because fallen logs prevent deer from accessing disturbed areas (de Chantal and Granström, 2007; Smit et al., 2012). These two impacts define the initial vegetation recovery process, which determines the future forest structure (Fischer and Fischer, 2012; Morimoto et al., 2019; Hotta et al., 2020).
The population density of ungulates may increase in boreal forests in North America because of the decrease in snow accumulation projected under climate change (Fisher et al., 2020; Laurent et al., 2021). The population of sika deer (Cervus nippon) in Japan is negatively related to snow depth (Ueno et al., 2018), and the possibility of a rapid intensification of deer impact is projected (Ohashi et al., 2014). These facts imply that forest managers should be vigilant about the increase in wind disturbance and increase in deer browsing pressure. Post-windthrow forest management, especially in cold, snowy regions with deer populations, needs to be approached carefully.
However, the effects of salvage logging and deer browsing on forest structure have never been assessed separately, nor have they been evaluated on the basis of monitoring over a sufficiently long period to assess subsequent forest succession processes (refer to Royo et al. (2010) for an assessment of those effects separately after fire disturbance). Therefore, it is not possible to determine whether a certain forest structure has been determined by legacy disturbance or by subsequent deer browsing. This situation makes it difficult to propose alternatives to salvaging and planting.
A field experiment was conducted in a cold, snowy region with a deer population to determine appropriate practices for achieving natural forest regeneration after catastrophic wind disturbance. The objectives of the study were (1) to assess the impact of legacy destruction and deer browsing on vegetation biomass and species composition after 15 years and (2) to identify the effects of legacy retention. We discuss the processes and consequences of post-windthrow forest practices on species composition and biomass 15 years after windthrow and the importance of legacy retention.
Materials and Methods
Study Area
Typhoon Songda hit Hokkaido Island (ca. 78,000 km2) in northern Japan in September 2004 and destroyed 399.6 km2 of forest (Tsushima et al., 2005). The study area was established in the national forest in Chitose city (Figure 1, 42°45′ N 141°30′ E; elevation of 150 m above sea level). In the study area, plantation patches of Abies sachalinensis planted in 1957 were completely destroyed by the typhoon, but no damage occurred in the natural old-growth mixed forests. The collapsed forest was an artificial forest, but the restoration goal was regeneration of the local natural forest in the windthrow area because the planned forest service in this area is conservation of biodiversity. The average annual temperature in the study area is 6.9°C, and the average annual precipitation is 1,649 mm (Lake Shikotsu, Japan Meteorological Agency [JMA], 2016). We established the experimental site in a damaged A. sachalinensis plantation (east–west (EW) 70 m × north–south (NS) 300 m) and the reference site (RF) in an undamaged mature, natural forest (ca. 4.5 km from the experimental site). The forest stand at the RF is more than 200 years old and is dominated by Picea jezoensis, A. sachalinensis, and Quercus crispula, all of which are original species in this region.
In the northern part of the experimental site (EW 70 m × NS 50 m), no manipulations were conducted, and all woody debris was left as it lay. In the southern part of the experimental site (EW 50 m × NS 150 m), salvage logging was conducted in September 2007 by bulldozers and harvesters with grapples. Thereafter, site preparation, flattening and clearing of a 4-m-wide row for tree planting were performed by backhoes. Woody debris scattered on the forest floor was piled along the sides of each planting row; these debris piles are referred to as residual rows. Approximately 10 cm of topsoil was removed from the site. Volcanic scoria ejected from Mount Tarumae (42°41′ N 141°22′ E; 1,041 m) emerged during site preparation. Seedlings of Q. crispula (∼60-cm height) were planted after the site preparation. This species was selected for planting to restore the mixed forest of Q. crispula and A. sachalinensis, which originally grew before manual forestation by humans. Deer fences (2 m in height) that enclose a part of the experimental site were installed in 2009.
The western and eastern sides of the experimental site were undamaged artificial Picea glehnii stands planted in 1979 and 1978. The forest that bordered the north side of the experimental site was natural mixed forest with minor damage. The forest that bordered the south side of the experimental site was A. sachalinensis plantations that were blown down by the typhoon; the downed trees were subsequently salvaged, and the area was planted with Q. crispula.
A subspecies of Japanese sika deer (Cervus nippon yesoensis) inhabits Hokkaido Island. The size of the deer population was estimated to be approximately 500,000–600,000 in 2013–2016 (Agetsuma, 2018). Their habitat comprises landscape that consists of both forests and grassland. Their foods seasonally change: grass leaves, tree leaves and nuts in the non-snow season and Sasa leaves, twigs and bark of woody plants in the snow season (Yokoyama et al., 2000; Campos-Arceiz and Takatsuki, 2005).
Study Design
We prepared four treatments without deer fences in 2007 and three additional treatments with deer fences in 2009 (Figure 1).
The treatments included legacy retention (LR), in which the trees were not salvaged and woody debris remained after the blowdown; legacy retention with deer fencing (LR-fenced), which involved LR enclosed by a deer fence; residual rows (RR), in which woody debris was piled up in rows during salvage logging; residual rows with deer fence (RR-fenced), which involved RR enclosed by a deer fence; planting rows (PR), in which salvaging, site preparation, and planting with Q. crispula seedlings were carried out; PR with deer fence (PR-fenced), which involved PR enclosed by a deer fence; and a reference (RF), which consisted of mature, natural mixed forest.
The size and number of quadrats in each treatment are shown in Figure 1. The quadrat size was determined by the maximum height of emerging plants (Braun-Blanquet, 1964). For the survey of the RF environment and vegetation, five quadrats (20 m × 20 m; 400 m2) were established. We then established five microquadrats (2 m × 2 m; 4 m2) within each quadrat. The quadrats were placed at least 200 m apart. One of the five microquadrats was placed at the center of the quadrat, and the other four microquadrats were placed at the centers of four 10 m × 10 m divided squares in each quadrat. For the survey of the environment and vegetation at the experimental treatment sites, microquadrats were established at the intersection points of the 10 m × 10 m grid system. The microquadrats were 2 m × 2 m (4 m2) in size for the LR and LR-fenced sites and 1 m × 4 m (4 m2) in size for the RR, RR-fenced, PR, and PR-fenced sites.
According to Japanese governmental policy, salvage logging should be implemented within a few years of a windthrow event (Ministry of Agriculture Forestry and Fisheries [MAFF], 2019). Thus, we were able to leave only a small portion of the study area unsalvaged for this study. Additionally, we were able to establish only 16 microquadrats for the LR site and 5 microquadrats for the LR-fenced site to avoid edge effects from the surrounding forest roads and to avoid the occurrence of autocorrelation among the quadrats. The minimal distance between any quadrat and roads was 10 m.
Field Survey
Environmental Conditions
The following were measured once at four random points in the microquadrats in July–August of 2018: the surface relative photon flux density (PFD); soil water content; cover % of materials that covered the ground; and the height, cover% and decay level of coarse woody debris (CWD). CWD was observed only in the LR, LR-fenced, RR, and RR-fenced treatments. The decay level was classified according to the shape of the CWD and the depth of knife penetration (Heilmann-Clausen, 2001; Fukasawa, 2012) according to established criteria (Forestry and Forest Products Research Institute [FFPRI], 2016). A decay level of 3 or greater corresponds to the status of dead wood that can serve as a nursery bed for seedlings (Graham and Cromack, 1982). An average value of four points was used as the representative of each microquadrat for later analysis. We also calculated an indicator to represent the amount of CWD, which is the maximum CWD height (cm) multiplied by the cover ratio (%), for each microquadrat. The unit was cm3.
The PFD was simultaneously measured using two light sensors (LI-250A light sensor; LI-COR, Lincoln, NE, United States) at 10 cm above the ground at four random points in each microquadrat and at a position to detect light from the whole sky. The measurements were conducted on sunny days in mid-August 2018. The ratio of the PFD at 10 cm above the ground to that of the whole sky was calculated, and the average ratio for each microquadrat was used to determine the relative PFD value.
The soil water content was measured using time-domain reflectometry (TDR; HydroSense, Campbell Scientific, Inc., Logan, UT, United States) on selected sunny days that followed three continuous sunny days in mid-August 2018. The soil water content was measured at a depth of 5 cm at four random points in each microquadrat, and the average was used in the subsequent analysis.
The % cover values were determined for litter, soil, gravel, and moss and lichen in the microquadrats in August 2018. Litter included leaves and small branches. Soil and gravel were classified by grain-size diameter: the diameter of gravel was larger than 1 cm, and that of soil was less than 1 cm.
Vegetation
The vascular plant species in each vegetation layer were surveyed once from late July to August in 2008 and 2018 in the experimental sites (LR, LR-fenced, PR, PR-fenced, RR, RR-fenced) and once from August to September in 2019 in the reference site (RF). Tree-layer species (≥10 m in height) were recorded only at the reference site because the vegetation at the experimental site had not yet reached the tree layer. The species in the tree layer in the quadrats and the species in the shrub (1.5–10 m) and herbaceous (<1.5 m) layers in the microquadrats were recorded. The % cover and maximum height of plants above and below the deer line (1.5 m) were measured at the experimental site in August 2018 and at the reference site in August 2019.
Analyses
The environmental and vegetation data were tabulated by microquadrat for the experimental treatment sites (LR, LR-fenced, PR, PR-fenced, RR, and RR-fenced) and by quadrat for the reference site (RF). To determine the representative value of each quadrat, we used the average value of the five microquadrats in the quadrat.
The Kruskal-Wallis test followed by the permutation test (P < 0.05) was used to examine the differences in soil water content, relative PFD,% cover of the various materials, and amounts of total CWD and CWD with a ≥ 3 decay level among the treatments (R version 3.5.1, package: rcompanion). The amount of CWD was analyzed only among the LR, LR-fenced, RR, and RR-fenced treatments because CWD was not observed in the other treatments.
An indicator of the vegetation biomass was calculated: the% cover of plants was separately multiplied by the maximum height (m) of the plants in the tree and shrub layers in each quadrat. We applied this indicator to express the volume of vegetation in the tree and shrub layers. The unit of the indicator was m3. Kruskal-Wallis test followed by the permutation test (P < 0.05) was used to examine the differences in vegetation biomass among the treatments.
A detrended correspondence analysis (DCA; Hill and Gauch, 1980) was used to examine the similarity between the species compositions in the windthrow treatments and those at the reference site. The DCA was also used to monitor the vegetation transit from 2008 to 2018 or 2019. The presence–absence data of each species were used for the analysis (R Core Team, 2018; R version 3.5.1, package: vegan). Any species present in fewer than two quadrats was excluded from the analysis. In total, 144 species were analyzed, including 47 woody, 84 herbaceous, and 13 fern species. We included all kinds of treatments for the analysis to determine which treatments would make the windfall site most similar to the target natural mixed forest.
Results
Environmental Conditions
Significant differences between certain treatments were observed for all environmental variables (Table 1).
To assess the impact of legacy destruction, we first compared the treatments with deer fences (LR-fenced, PR-fenced, RR-fenced) to the RF site. Most environmental variables at the planted sites (PR-fenced and RR-fenced) were significantly different from those at the RF site. In contrast, most environmental variables at the LR-fenced site were equivalent to those at the RF site.
Next, to assess the impact of deer browsing, we compared the treatments with and without deer fences (LR vs. LR-fenced, PR vs. PR-fenced, RR vs. RR-fenced). The installation of deer fences around the legacy retention sites (LR vs. LR-fenced) did not result in any differences in environmental variables. However, deer fence installation around the planted sites (PR vs. PR-fenced and RR vs. RR-fenced) resulted in differences in litter and moss cover. When the vegetation did not experience deer browsing, the litter cover increased, and the moss cover decreased.
Vegetation Biomass
The vegetation biomass in the shrub and tree layers, i.e., the vegetation biomass above the deer line, was significantly different among the different treatments (Figure 2). In contrast, the vegetation biomass in the herbaceous layer, i.e., the vegetation biomass below the deer line, varied only slightly among the treatments (Figure 3).
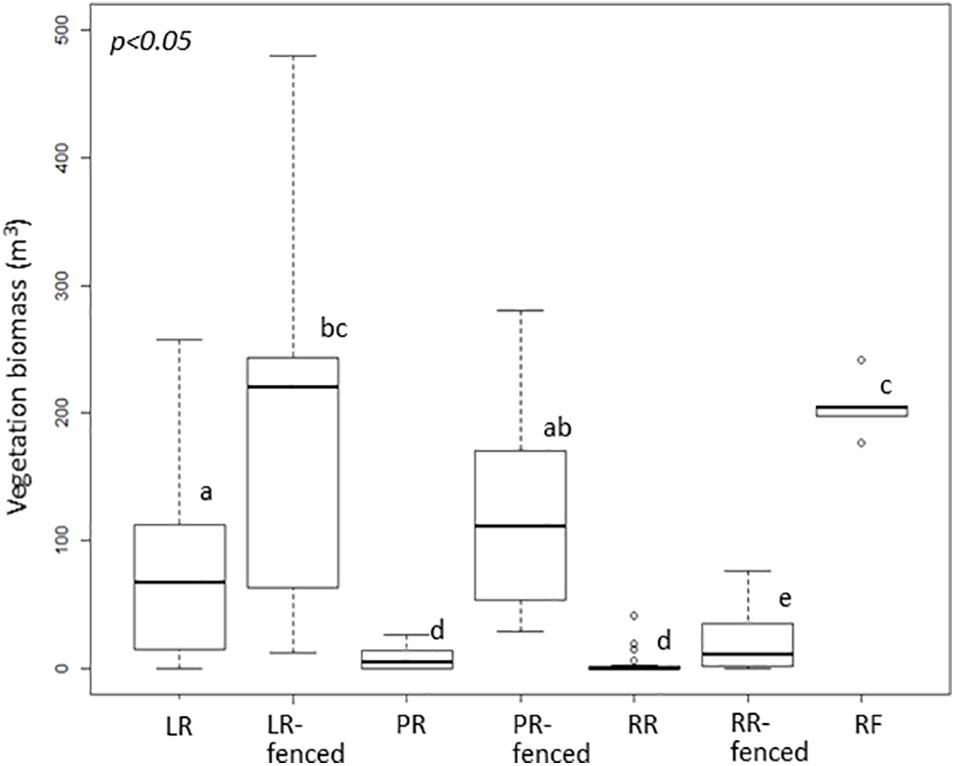
Figure 2. Boxplot of vegetation biomass above the deer line in each treatment after windthrow and in the mature, natural mixed forest. Significant differences according to a permutation test (p < 0.05) are indicated by different letters. LR, legacy retention; LR-fenced, LR enclosed by a deer fence; RR, residual rows; RR-fenced, RR enclosed by a deer fence; PR, planting rows; PR-fenced, PR enclosed by a deer fence; and RF, mature, natural mixed forest (reference site).
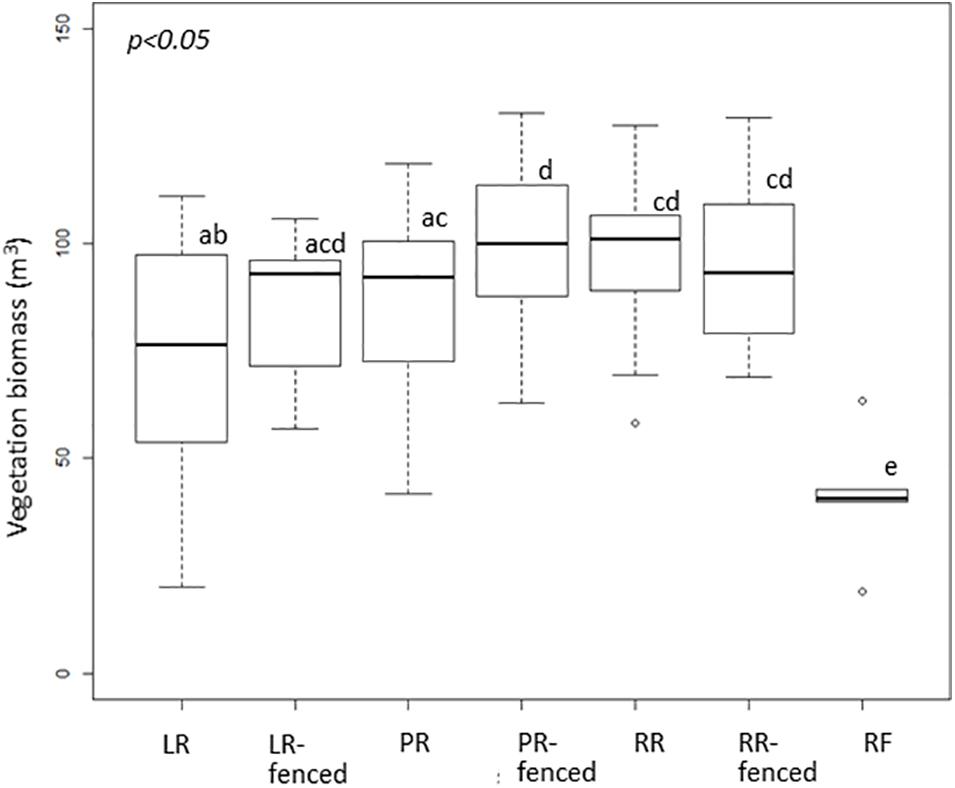
Figure 3. Boxplot of vegetation biomass below the deer line in each treatment after windthrow and in the mature, natural mixed forest. Significant differences according to a permutation test (p < 0.05) are indicated by different letters. LR, legacy retention; LR-fenced, LR enclosed by a deer fence; RR, residual rows; RR-fenced, RR enclosed by a deer fence; PR, planting rows; PR-fenced, PR enclosed by a deer fence; and RF, mature, natural mixed forest (reference site).
To assess the impact of legacy destruction, we first compared the treatments with deer fences (LR-fenced, PR-fenced, RR-fenced) to the RF site. Only at the LR-fenced site did the vegetation biomass in the shrub layer grow to a level comparable to that at the RF site. The vegetation biomass in the shrub layer was largest at the PR-fenced site, followed by that at the LR-fenced site, and that at the RR-fenced site was the lowest.
Next, to assess the impact of deer browsing, we compared the treatments with and without deer fences (LR vs. LR-fenced; PR vs. PR-fenced, RR vs. RR-fenced). In all comparisons, the vegetation biomass in the shrub and tree layers in the treatment that was free from deer browsing was significantly higher than that in the treatment exposed to deer browsing.
Species Richness and Composition
Immediately after the windthrow event in 2008, the planted sites (PR and RR) had higher species richness than the legacy retention site (LR) (Table 2). The planted sites (PR and RR) were dominated by shade-intolerant herbaceous and pioneer woody species, whereas shade-tolerant herbaceous and woody species dominated the legacy retention sites (LR) (Figure 4). The difference in species richness among the treatments at the windthrow experimental site disappeared by 2018 (Table 2). The species composition in LR and LR-fenced approached that in the mature natural forest (RF) over the studied decade. In contrast, the vegetation at the PR, PR-fenced, RR, and RR-fenced sites did not show any indications of a transition to RF vegetation.
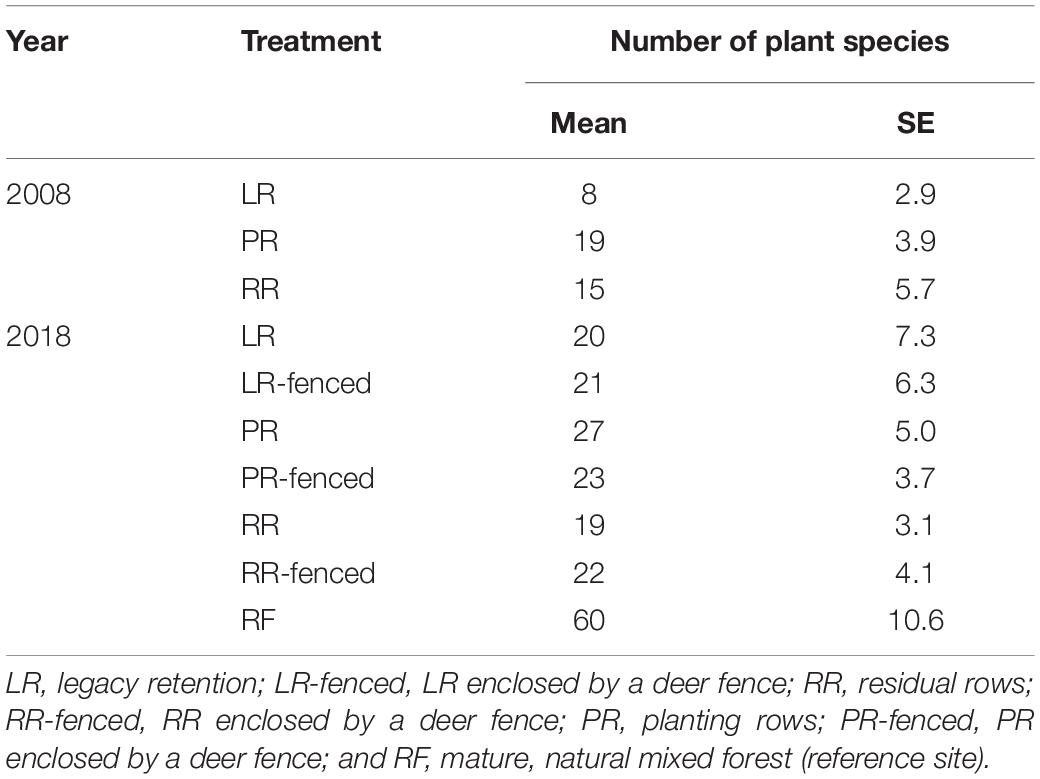
Table 2. Plant species richness in each treatment after windthrow and in the mature, natural mixed forest.
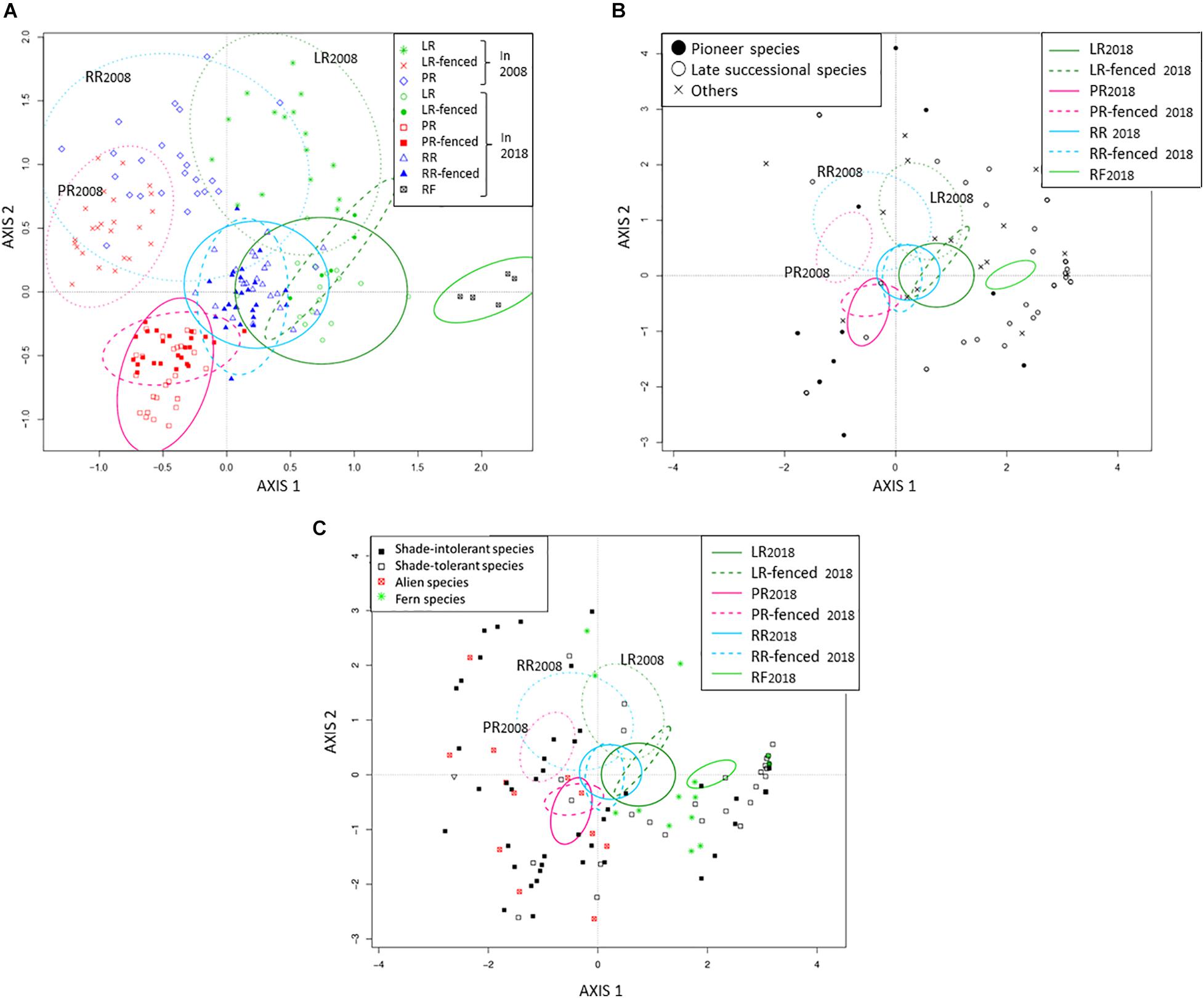
Figure 4. Biplot of species composition according to DCA. (A) Quadrat distribution, (B) Woody species with quadrat distribution, (C) Herbaceous species with quadrat distribution. LR, legacy retention; LR-fenced, LR enclosed by a deer fence; RR, residual rows; RR-fenced, RR enclosed by a deer fence; PR, planting rows; PR-fenced, PR enclosed by a deer fence; and RF, mature, natural mixed forest (reference site). The subscripts 2008 and 2018 indicate the year of the investigation. The 90% confidence interval of the quadrat distribution for each treatment is shown as an ellipse.
To assess the impact of legacy destruction, we compared the treatments with deer fences (LR-fenced, PR-fenced, RR-fenced) to the RF site. There was little difference in species richness among the treatments in the windthrow experimental site (LR-fenced, PR-fenced, RR-fenced), and all treatments had smaller species richness to the RF forest. The species composition in the LR-fenced forest was the closest to that in the RF forest and was characterized by shade-tolerant woody species and ferns. The species composition in the PR-fenced forest was the farthest from that in the RF forest and was characterized by shade-intolerant and alien herbaceous species like Solidago gigantea Aiton, Plantago asiatica L., and Chrysanthemum leucanthemum L (see Supplementary Table 1).
Next, we compared the treatments with and without deer fences (LR vs. LR-fenced; PR vs. PR-fenced, RR vs. RR-fenced) to assess the impact of deer browsing. The presence or absence of deer fences had no effect on the number of species (Table 2). We found slight differences in species composition between LR- and LR-fenced plants and between PR- and PR-fenced plants, but no difference was observed between RR- and RR-fenced plants.
Discussion
It is difficult to extrapolate a universal principle from an experiment conducted at a single windthrow site. However, having the same environmental conditions for the quadrats, including weather, topography, seed dispersal, and deer density, allowed us to make precise comparisons between treatments.
The Impact of Legacy Destruction and Deer Browsing on Vegetation Biomass
Legacy destruction suppressed the development of shrub- and tree-layer biomass only in the RR treatments. However, subsequent deer foraging suppressed the development of shrub- and tree-layer biomass in the unfenced RR and PR treatments. Shrub- and tree-layer biomass equivalent to that at the RF site developed only in the LR-fenced treatment.
Salvage logging and planting drastically changed the environmental conditions, including the soil water content, relative photon flux, cover of gravel, soil, and litter, immediately after the practice (Morimoto et al., 2011), and the effect remained even after 15 years. This result is compatible with a report (Lang et al., 2009) on the long-term (25 years) effect of salvage logging on soil in an old-growth hemlock northern hardwood forest. They also noted the importance of biological legacies that remained after salvage logging, such as root suckers and stump sprouts.
Because the residual row sites were densely piled with remaining woody debris and volcanic scoria after salvaging, many of the advanced seedlings were likely killed or damaged, and there was limited space available for the dispersal of new seeds. Therefore, the vegetation biomass in the RR-fenced treatment was much lower than that in the LR-fenced treatment, where developed seedlings and newly dispersed seeds regenerated, and in the PR-fenced treatment, where planted Q. crispula grew. The growth of advanced seedlings in the residual row was expected in the initial stages of regeneration (Morimoto et al., 2011), but they only slightly contributed to the vegetation biomass due to their low density.
The PR site had the lowest vegetation biomass probably due to the planting of Q. crispula, which is a species that deer preferentially consume. To develop stands of broadleaved tree species that are preferred by deer in areas with deer populations, creative planting methods are required, such as adjusting the size of the saplings and planting density and mixing broadleaved species with coniferous species (Borkowski et al., 2017).
One reason why the impact of deer browsing noticeably affected the vegetation biomass of the shrub and tree layers is that deer visit open stands more frequently than closed stands to forage for saplings (Kuijper et al., 2009). Deer frequented the sunny windthrow area and repeatedly foraged on Q. crispula saplings for 15 years, which probably suppressed tree growth and leaf development and thereby limited biomass development in the shrub and tree layers.
Although there are many reports speculating that deer browsing after salvaging suppresses the regeneration of broadleaved trees (e.g., in the early stage, Jonášová et al., 2010; 40 years after windthrow, Götmark and Kiffer, 2014), these studies were not designed to clearly distinguish the effects of deer browsing from the impacts of legacy destruction. Our study design allowed us to successfully quantify the effects of deer browsing and demonstrate that vegetation biomass of the shrub and tree layers was suppressed by deer browsing across the planted sites.
Impact of Legacy Destruction and Deer Browsing on Species Composition
Legacy destruction resulted in greater species richness, and the species composition was dominated by herbaceous, shade-intolerant species immediately after windthrow. Subsequent deer browsing did not alter the species richness but shifted the herbaceous community toward a community comprising more ruderal and alien species. The species richness in the LR treatment increased to the equivalent level of that in other treatments at the windthrow site, and the species composition approached the target species composition in the RF site during the 15 years, whereas the RR and PR sites maintained their herb-dominated species composition.
In the initial stages of regeneration after extreme forest management practices, such as scarification and site preparation with salvage logging, fern species decrease in abundance (Haeussler et al., 1999), herbaceous species dominate (Rumbaitis del Rio, 2006), and pioneer tree species establish among the herbaceous species (Morimoto et al., 2013). We found that these initial trends of regeneration were sustained even 15 years after the windthrow event. However, the effects of salvage logging on species richness were short lived, and the difference among treatments at the windthrow experimental site diminished during the 15 years. These findings correspond to those of a report in which the effects of wind disturbance and salvage logging on the diversity parameters were evaluated over 6 years (Oldfield and Peterson, 2019), stating that those disturbances altered the tree and sapling species compositions but not the alpha diversity, e.g., the species richness and Shannon diversity.
Conventional forestry practices shifted the plant community at the windthrow sites toward an herbaceous one, while legacy retention brought the community at the windthrow sites closer to the target mature natural forest community. The main reason for these opposite outcomes was the destruction of legacy forest remnants by heavy equipment rather than deer browsing. The impact of human disturbances on species composition may last even longer because they leave only a limited amount of decayed CWD that can serve as a substrate for seedling germination and growth in the forested area (Table 1). In particular, the regeneration of typical conifer tree species in boreal Picea forests is known to be dependent on the presence of well-decayed fallen logs 30–60 years after tree death (Zielonka, 2006). Therefore, Picea species are lost from the next generation of forests in forested areas. A statistical analysis showing that the impact of salvaging, site preparation, and planting on species diversity lasts for at least 50–80 years (Takafumi and Hiura, 2009) supports our inference.
During the 5 years between the windthrow event and the installation of the deer fence, the entire experimental site was in an open environment for deer to invade. In particular, deer were more likely to forage while walking at planting sites where windthrow trees had been removed. Therefore, the effect of deer foraging on the biomass and species composition in the PR-fenced and RR-fenced sites may be stronger than that if deer fences had been installed immediately after the windthrow event.
The Effect of Legacy Retention
The vegetation biomass and species composition of the LR treatment were similar to those of RF than those of the other treatments, and this trend was apparent during the first 15 years of succession. The LR treatment retained the advance regeneration of shade-tolerant species and fern species. The heterogeneous microsites retained at the LR sites also provided optimal conditions for the establishment of new seedlings. Moreover, the fallen logs at these sites acted as barriers to deer access and minimized browsing.
In the initial stages of recovery, the advance regeneration of shade-tolerant species and fern species that prefer various microsites characterizes legacy retention areas (Morimoto et al., 2011), in which the vegetation biomass and species composition become similar to those in mature natural forests (Morimoto et al., 2013). We found that these initial trends persisted even 15 years after the windthrow event.
Regeneration mortality and species composition are initially controlled by exogenous factors that are linked to storm severity and microsite heterogeneity and generate a degree of spatial partitioning within a microsite; gradually, species life-history traits and competition predominate, controlling forest dynamics (Vodde et al., 2015). Various types of structural legacies (e.g., downed trunks, branches, canopies, pits, and mounds) that create heterogeneous conditions on the ground (Rydgren et al., 1998; Ulanova, 2000) protect the propagule legacies of advance regeneration seedlings and saplings from deer browsing (Smit et al., 2012) and allow recruitment from newly dispersed seeds (Peterson and Pickett, 1995) as well as competition among them (Vodde et al., 2015). In the present study, these processes defined the species composition and biomass 15 years after the windthrow event.
Many studies have shown that fallen trees prevent deer from foraging on regenerating vegetation in the years immediately following a windthrow event. Our experimental design demonstrated that this is indeed the case. On the other hand, the vegetation biomass in the shrub and tree layers at the LR-fenced site was greater than that at the unfenced LR site, and the species composition at the LR-fenced site was slightly different from that at the unfenced LR site. These findings suggest that downed trees do not completely prevent deer from browsing. In fact, at our experimental site, the average height of CWD decreased significantly, from 0.98 m (0.00–2.15 m; Morimoto et al., 2011) in 2008 to 0.38 m (0.00–1.24 m) in 2018, because of the decay of downed logs. This suggests that the deer-excluding effect of fallen trees on legacy retention decreases over time. The consequences of these findings for future vegetation succession need to be considered.
We have shown the physical impact of fallen trees acting as barriers to deer access in this study; however, it is expected that deadwood also plays as a key role in the conservation of local biodiversity because deadwood provides an essential habitat for deadwood-dependent species such as saproxylic beetles, foraging woodpeckers, and fungi (Doerfler et al., 2020; Dufour-Pelletier et al., 2020).
Implications
The following suggestions are provided based on the analysis of the surveys conducted immediately after windfall and in the 15th year after windfall: after catastrophic wind disturbance in cold, snowy regions, leaving fallen trees is a more reasonable method to promote natural forest regeneration than conventional forest practices, which include salvaging, site preparation, and planting. However, in areas with high deer-browsing pressure, the stagnation of vegetative succession due to the decreasing deer-excluding effect of fallen trees over time must be taken into account.
In monsoon Asia, legacy retention has not been put into practical use due to the fear of bark beetles damaging the surrounding plantation trees, as these beetles inhabit the fallen trees that remain after windthrow. However, in the case of large-scale windfall disasters, it takes several years to completely remove fallen trees, and insect outbreaks are inevitable. Furthermore, vegetation recovery after salvage logging remains an issue (Morimoto et al., 2019). Hence, it is recommended to implement salvage logging only if there is a forestation area in the vicinity. The selection of species to plant is also an important issue since saplings planted to supplement destroyed advance regeneration may become food for deer in regions with a high deer density. Therefore, it is better to select tree species that are not preferred to be eaten by deer and plant those species after salvaging if it is not possible to protect them with deer fences.
Management practices must be considered on the basis of the presence of deer because the forest structure does not immediately recover from disturbance, even if the deer population is reduced and the browsing pressure decreases (Tanentzap et al., 2011). It is necessary to consider changing the reforestation policy of removing all downed trees to a policy of leaving downed trees to allow the regeneration of natural forests, unless there is a concern about insect damage to the remaining forestry land in the vicinity.
Data Availability Statement
The data analyzed in this study is subject to the following licenses/restrictions: The dataset belongs to the Laboratory of Forest Ecosystem Management, Graduate School of Agriculture, Hokkaido University. Requests to access these datasets should be directed to JM, jmo1219@for.agr.hokudai.ac.jp.
Author Contributions
JM: conceptualization, methodology, investigation, writing—original draft, and writing—review and editing. MS: methodology, investigation, data curation, visualization, and writing—review and editing. MM: methodology, investigation, and writing—review and editing. FN: writing—review and editing and supervision. All authors contributed to the article and approved the submitted version.
Funding
This study was supported by the Japan Society for the Promotion of Science KAKENHI Grant No. JP17H01516, the Ministry of the Environment Japan S-15 PANCES Grant No. JPMEERF16S11508, and the Ministry of Education, Culture, Sports, Science and Technology Japan TOUGOU Grant No. JPMXD0717935498.
Conflict of Interest
The authors declare that the research was conducted in the absence of any commercial or financial relationships that could be construed as a potential conflict of interest.
Acknowledgments
We would like to thank the Hokkaido Regional Forest Office of the Forestry Agency for supporting the long-term project.
Supplementary Material
The Supplementary Material for this article can be found online at: https://www.frontiersin.org/articles/10.3389/ffgc.2021.675475/full#supplementary-material
References
Agetsuma, N. (2018). A simple method for calculating minimum estimates of previous population sizes of wildlife from hunting records. PLoS One 13:e0198794. doi: 10.1371/journal.pone.0198794
Borkowski, J., Dobrowolska, D., Da̧browski, W., Banul, R., and Załuski, D. (2017). Young conifer stands form a deer browsing refuge for an oak admixture: silvicultural implications for forest regeneration under herbivore pressure. Eur. J. For. Res. 136, 787–800. doi: 10.1007/s10342-017-1070-3
Braun-Blanquet, J. (1964). Pflanzensoziologie, Grundzüge der Vegetationskunde. Berlin: Springer. doi: 10.1007/978-3-7091-8110-2
Campos-Arceiz, A., and Takatsuki, S. (2005). Food habits of sika deer in the Shiranuka Hills, eastern Hokkaido: a northern example from the north–south variations in food habits in sika deer. Ecol. Res. 20, 129–133. doi: 10.1007/s11284-004-0019-4
R Core Team (2018). R: A Language And Environment For Statistical Computing. Vienna, Austria: R Foundation for Statistical Computing.
de Chantal, M., and Granström, A. (2007). Aggregations of dead wood after wildfire act as browsing refugia for seedlings of Populus tremula and Salix caprea. For. Ecol. Manage. 250, 3–8. doi: 10.1016/j.foreco.2007.03.035
Doerfler, I., Cadotte, M. W., Weisser, W. W., Müller, J., Gossner, M. M., Heibl, C., et al. (2020). Restoration-oriented forest management affects community assembly patterns of deadwood-dependent organisms. J. Appl. Ecol. 57, 2429–2440. doi: 10.1111/1365-2664.13741
Dufour-Pelletier, S., Tremblay, J. A., Hébert, C., Lachat, T., and Ibarzabal, J. (2020). Testing the effect of snag and cavity supply on deadwood-associated species in a managed boreal forest. Forests 11:424. doi: 10.3390/f11040424
Fischer, A., and Fischer, H. S. (2012). Individual-based analysis of tree establishment and forest stand development within 25 years after wind throw. Eur. J. For. Res. 131, 493–501. doi: 10.1007/s10342-011-0524-2
Fisher, J. T., Burton, A. C., Nolan, L., and Roy, L. (2020). Influences of landscape change and winter severity on invasive ungulate persistence in the Nearctic boreal forest. Sci. Rep. 10:8742. doi: 10.1038/s41598-020-65385-3
Forestry and Forest Products Research Institute [FFPRI] (2016). The Methods of Forest Soil Inventory Ver. 3 (1) The Methods of Field Survey. Ibaraki: Forestry and Forest Products Research Institute.
Fukasawa, Y. (2012). Effects of wood decomposer fungi on tree seedling establishment on coarse woody debris. For. Ecol. Manage. 266, 232–238. doi: 10.1016/j.foreco.2011.11.027
Götmark, F., and Kiffer, C. (2014). Regeneration of oaks (Quercus robur/Q. petraea) and three other tree species during long-term succession after catastrophic disturbance (windthrow). Plant Ecol. 215, 1067–1080. doi: 10.1007/s11258-014-0365-4
Graham, R. L., and Cromack, K. (1982). Mass, nutrient content, and decay rate of dead boles in rain forests of Olympic National Park. Can. J. For. Res. 12, 511–521. doi: 10.1139/x82-080
Haeussler, S., Bedford, L., Boateng, J. O., and MacKinnon, A. (1999). Plant community responses to mechanical site preparation in northern interior British Columbia. Can. J. For. Res. 29, 1084–1100. doi: 10.1139/x99-057
Heilmann-Clausen, J. (2001). A gradient analysis of communities of macrofungi and slime moulds on decaying beech logs. Mycol. Res. 105, 575–596. doi: 10.1017/S0953756201003665
Hill, M. O., and Gauch, H. G. (1980). “Detrended correspondence analysis: an improved ordination technique,” in Classification and Ordination. Advances in Vegetation Science, ed. E. van der Maarel (Dordrecht: Springer), 47–58. doi: 10.1007/978-94-009-9197-2_7
Hotta, W., Morimoto, J., Inoue, T., Suzuki, S. N., Umebayashi, T., Owari, T., et al. (2020). Recovery and allocation of carbon stocks in boreal forests 64 years after catastrophic windthrow and salvage logging in northern Japan. For. Ecol. Manage. 468:118169. doi: 10.1016/j.foreco.2020.118169
Japan Meteorological Agency [JMA] (2016). Climate Statistics. Japan Meteorological Agency. Available Online at: http://www.data.jma.go.jp/obd/stats/etrn/index.php (accessed 8 February 2021)
Jonášová, M., Vávrová, E., and Cudlín, P. (2010). Western Carpathian mountain spruce forest after a windthrow: natural regeneration in cleared and uncleared areas. For. Ecol. Manage. 259, 1127–1134. doi: 10.1016/j.foreco.2009.12.027
Kuijper, D. P. J., Cromsigt, J. P. G. M., Churski, M., Adam, B., Jędrzejewska, B., and Jędrzejewski, W. (2009). Do ungulates preferentially feed in forest gaps in European temperate forest? For. Ecol. Manage. 258, 1528–1535. doi: 10.1016/j.foreco.2009.07.010
Lang, K. D., Schulte, L. A., and Guntenspergen, G. R. (2009). Windthrow and salvage logging in an old-growth hemlock-northern hardwoods forest. For. Ecol. Manage. 259, 56–64. doi: 10.1016/j.foreco.2009.09.042
Laurent, M., Dickie, M., Becker, M., Serrouya, R., and Boutin, S. (2021). Evaluating the mechanisms of landscape change on white-tailed deer populations. J. Wildl. Manage. 85, 340–353. doi: 10.1002/jwmg.21979
Ministry of Agriculture Forestry and Fisheries [MAFF] (2019). Ministry of Agriculture Forestry and Fisheries. Available Online at: https://www.rinya.maff.go.jp/j/saigai/saigai/attach/pdf/190326_5-1.pdf (accessed April 30, 2021).
Morimoto, J., Morimoto, M., and Nakamura, F. (2011). Initial vegetation recovery following a blowdown of a conifer plantation in monsoonal East Asia: impacts of legacy retention, salvaging, site preparation, and weeding. For. Ecol. Manage. 261, 1353–1361. doi: 10.1016/j.foreco.2011.01.015
Morimoto, J., Umebayashi, T., Suzuki, S. N., Owari, T., Nishimura, N., Ishibashi, S., et al. (2019). Long-term effects of salvage logging after a catastrophic wind disturbance on forest structure in northern Japan. Landsc. Ecol. Eng. 15, 133–141. doi: 10.1007/s11355-019-00375-w
Morimoto, M., Morimoto, J., Moriya, Y., and Nakamura, F. (2013). Forest restoration following a windthrow: how legacy retention versus plantation after salvaging alters the trajectory of initial recovery. Landsc. Ecol. Eng. 9, 259–270. doi: 10.1007/s11355-012-0206-3
Moriya, Y., Morimoto, M., Morimoto, J., and Nakamura, F. (2012). The effects of treatment following wind disturbance on deer browsing and its impact on vegetation. J. Jpn. For. Soc. 94, 10–16. doi: 10.4005/jjfs.94.10
Murakami, H., Wang, Y., Yoshimura, H., Mizuta, R., Sugi, M., Shindo, E., et al. (2012). Future changes in tropical cyclone activity projected by the new high-resolution MRI-AGCM. J. Clim. 25, 3237–3260. doi: 10.1175/JCLI-D-11-00415.1
Ohashi, K., Shibata, R., Murohara, T., and Ouchi, N. (2014). Role of anti-inflammatory adipokines in obesity-related diseases. Trends Endocrinol. Metab. 25, 348–355. doi: 10.1016/j.tem.2014.03.009
Oldfield, C. A., and Peterson, C. J. (2019). Woody species composition, diversity, and recovery six years after wind disturbance and salvage logging of a Southern Appalachian forest. Forests 10:129. doi: 10.3390/f10020129
Peterson, C. J., and Leach, A. D. (2008). Salvage logging after windthrow alters microsite diversity, abundance and environment, but not vegetation. Forestry 81, 361–376. doi: 10.1093/forestry/cpn007
Peterson, C. J., and Pickett, S. T. A. (1995). Forest reorganization: a case study in an old-growth forest catastrophic blowdown. Ecology 76, 763–774. doi: 10.2307/1939342
Royo, A. A., Collins, R., Adams, M. B., Kirschbaum, C., and Carson, W. P. (2010). Pervasive interactions between ungulate browsers and disturbance regimes promote temperate forest herbaceous diversity. Ecology 91, 93–105. doi: 10.1890/08-1680.1
Rumbaitis del Rio, C. M. (2006). Changes in understory composition following catastrophic windthrow and salvage logging in a subalpine forest ecosystem. Can. J. For. Res. 36, 2943–2954. doi: 10.1139/x06-169
Rydgren, K., Hestmark, G., and Økland, R. H. (1998). Revegetation following experimental disturbance in a boreal old-growth Picea abies forest. J. Veg. Sci. 9, 763–776. doi: 10.2307/3237042
Smit, C., Kuijper, D. P. J., Prentice, D., Wassen, M. J., and Cromsigt, J. P. G. M. (2012). Coarse woody debris facilitates oak recruitment in Białowieża Primeval Forest. Poland. For. Ecol. Manage. 284, 133–141. doi: 10.1016/j.foreco.2012.07.052
Taeroe, A., de Koning, J. H. C., Löf, M., Tolvanen, A., Heiðarsson, L., and Raulund-Rasmussen, K. (2019). Recovery of temperate and boreal forests after windthrow and the impacts of salvage logging. A quantitative review. For. Ecol. Manage. 446, 304–316. doi: 10.1016/j.foreco.2019.03.048
Takafumi, H., and Hiura, T. (2009). Effects of disturbance history and environmental factors on the diversity and productivity of understory vegetation in a cool-temperate forest in Japan. For. Ecol. Manage. 257, 843–857. doi: 10.1016/j.foreco.2008.10.020
Tan, X., Chang, S. X., and Kabzems, R. (2005). Effects of soil compaction and forest floor removal on soil microbial properties and N transformations in a boreal forest long-term soil productivity study. For. Ecol. Manage. 217, 158–170. doi: 10.1016/j.foreco.2005.05.061
Tanentzap, A. J., Bazely, D. R., Koh, S., Timciska, M., Haggith, E. G., Carleton, T. J., et al. (2011). Seeing the forest for the deer: do reductions in deer-disturbance lead to forest recovery? Biol. Conserv. 144, 376–382. doi: 10.1016/j.biocon.2010.09.015
Thorn, S., Bässler, C., Brandl, R., Burton, P. J., Cahall, R., Campbell, J. L., et al. (2018). Impacts of salvage logging on biodiversity: a meta-analysis. J. Appl. Ecol. 55, 279–289. doi: 10.1111/1365-2664.12945
Tsushima, T., Kanno, M., Terasawa, K., Kohata, Y., Abe, T., Sato, H., et al. (2005). Report of the Damage from Typhoon No. 18 in 2004. Koushunai-Kihou: Hokkaido Forestry Research Institute.
Ueno, M., Iijima, H., Takeshita, K., Takahashi, H., Yoshida, T., Uehara, H., et al. (2018). Robustness of adult female survival maintains a high-density sika deer (Cervus nippon) population following the initial irruption. Wildl. Res. 45, 143–154. doi: 10.1071/WR17103
Ulanova, N. G. (2000). The effects of windthrow on forests at different spatial scales: a review. For. Ecol. Manage. 135, 155–167. doi: 10.1016/S0378-1127(00)00307-8
Vodde, F., Jõgiste, K., Engelhart, J., Frelich, L. E., Moser, W. K., Sims, A., et al. (2015). Impact of wind-induced microsites and disturbance severity on tree regeneration patterns: results from the first post-storm decade. For. Ecol. Manage. 348, 174–185. doi: 10.1016/j.foreco.2015.03.052
Yokoyama, M., Kaji, K., and Suzuki, M. (2000). Food habits of sika deer and nutritional value of sika deer diets in eastern Hokkaido. Japan. Ecol. Res. 15, 345–355. doi: 10.1046/j.1440-1703.2000.00355.x
Keywords: windthrow, salvage logging, legacy retention, deer browsing, deer fence, vegetation biomass, species composition
Citation: Morimoto J, Sugiura M, Morimoto M and Nakamura F (2021) Restoration of Natural Forests After Severe Wind Disturbance in a Cold, Snowy Region With a Deer Population: Implications From 15 Years of Field Experiments. Front. For. Glob. Change 4:675475. doi: 10.3389/ffgc.2021.675475
Received: 03 March 2021; Accepted: 09 June 2021;
Published: 05 July 2021.
Edited by:
Barry Alan Gardiner, Institut Européen de la Forêt Cultivée (IEFC), FranceReviewed by:
Kaysandra Waldron, Canadian Forest Service, CanadaGolo Stadelmann, Swiss Federal Institute for Forest, Snow and Landscape Research (WSL), Switzerland
Copyright © 2021 Morimoto, Sugiura, Morimoto and Nakamura. This is an open-access article distributed under the terms of the Creative Commons Attribution License (CC BY). The use, distribution or reproduction in other forums is permitted, provided the original author(s) and the copyright owner(s) are credited and that the original publication in this journal is cited, in accordance with accepted academic practice. No use, distribution or reproduction is permitted which does not comply with these terms.
*Correspondence: Junko Morimoto, jmo1219@for.agr.hokudai.ac.jp